- 1Department of Human Ecology, Rutgers University, New Brunswick, NJ, USA
- 2Department of Community Sustainability, Michigan State University, East Lansing, MI, USA
- 3Department of Educational Psychology, Rutgers University, New Brunswick, NJ, USA
- 4Center for Mathematics, Science, and Computer Education, Rutgers University, New Brunswick, NJ, USA
- 5Department of Counseling and Educational Psychology, Indiana University, Bloomington, IN, USA
In response to recent educational imperatives in the United States, modeling and systems thinking have been identified as being critical for science learning. In this paper, we investigate models in the classroom from two important perspectives: (1) from the teacher perspective to understand how teachers perceive models and use models in the classroom and (2) from the students perspective to understand how student use model-based reasoning to represent their understanding in a classroom setting. Qualitative data collected from 19 teachers who attended a professional development workshop in the northeastern United States indicate that while teachers see the value in teaching to think with models (i.e., during inquiry practices), they tend to use models mostly as communication tools in the classroom. Quantitative data collected about the modeling practices of 42 middle school students who worked collaboratively in small groups (4–5 students) using a computer modeling program indicated that students tended to engage in more mechanistic and function-related thinking with time as they reasoned about a complex system. Furthermore, students had a typified trajectory of first adding and then next paring down ideas in their models. Implications for science education are discussed.
Introduction
Recent large-scale science education reform in the United States has been mainly guided by the Next Generation Science Standards (NGSS, 2013). In these standards, scientific practices such as modeling have been noted as being particularly important in the science classroom. Furthermore, the NGSS also highlights the value of not only integrating core concepts in science and engineering but also engaging students in learning about ideas that cross-cut disciplines, such as system-level and function-level understanding. Here, we will first discuss a study using qualitative data on teachers’ impressions of models and modeling and then we will share another study with empirical data to support the notion of pairing a conceptual representation with modeling to foster systems thinking skills in middle school students.
Many people inside and outside of the classroom do not have an appreciation for the purpose and the nature of models and modeling in science (e.g., Treagust et al., 2002; Crawford and Cullin, 2004). In the context of this manuscript, we define a model as a simplified abstraction that often represents a scientific phenomenon (Gilbert, 1993; Crawford and Jordan, 2013). Such representations enable the testing of ideas, for example, through simulation or as hypotheses related to how the world works. These representations can be physical, textual, verbal, or mathematical, but what is of interest to educators is the means by which students generate their models. Models and the practice of modeling provide students with an opportunity to think scientifically. They are central to science (Rosenblueth and Wiener, 1945) and, therefore, it has been suggested that they should be central to science teaching (Clement, 2000). Tools that enable sophisticated visualizations and simulations are now quite accessible, and student exposure to this technology has been found to be highly motivating (Shernoff, 2013). Model construction and revision in its simplicity is a process of posing and modifying explanations for particular phenomena. Models exist as hypotheses, conjectures, and often, sophisticated explanations.
Models also serve as communication tools. They provide a means for students to take non-linear (or non-narrative, non-story based) constructs and make them visible for themselves, their peers, and their instructors. Models lay out a means for looking at strong and weak links in conjectures, requiring evidence and reflecting on that evidence. Working with evidence encourages explicit thinking about epistemic aims and ideals, as well as encourage students to look for generic mechanisms and context specific elements. It is that generic-specific link that can possibly foster transfer of learning. Models provide pathways for formative and non-verbal assessment and can allow instructors to determine what is necessary to encourage more meaningful learning experiences.
Although models are an essential part of scientific inquiry, teachers often employ models as a way to directly communicate existing knowledge (Van Driel and Verloop, 1999; Treagust et al., 2002) rather than guiding inquiry to develop deep understanding of scientific phenomena. We argue that this predominant conception of a scientific model as a tool for communication can inhibit inference across large spatial and temporal scales and reasoning about dynamic phenomena leading to the under use of models in the scientific classroom. In addition, when models are used simply as static representations, students are rarely given the opportunity to use these representations for analysis, prediction or to understand reasons that underlie dynamic processes (Carey and Smith, 1993; Van Driel and Verloop, 1999), which represent more sophisticated model use that are common to science and scientific investigation. Such limitations will likely hinder a student’s attempt to integrate scientific concepts, and may promote an idea of a model as another manner in which to communicate, rather than a tool for exploration and reasoning. Below, we delved into this latter idea with our qualitative study of teachers’ ideas about models in a professional development context.
Part 1—Qualitative Study
The teachers voluntarily participated in this study as a part of a broader 3-day Woodrow Wilson Foundation supported National Teachers as Scholars (TAS) program. This program does not provide any pedagogically oriented professional development and rests entirely on the premise that teachers will gain from engaging in scholarly activity typified by university research. We report data from an east coast university’s TAS program, which focused on the role of models in the scientific enterprise and model use in the classroom. University scientists led the workshops. Grades 6–12 science teachers from urban/suburban schools were invited through letters sent to school principles. Teachers were not paid for their participation, and the school districts covered substitute teacher costs. While demographic information was not collected, the group was mostly female and their experience level teaching ranged from relatively new to the profession to 20+ years of experience.
During this workshop, we asked the teachers a series of questions regarding model use: (1) What do teachers report are beneficial about model use with regard to their students and in the scientific enterprise? (2) How do teachers develop models? and (3) How do teachers report their use of models in the classroom? To do so, open-ended questionnaires were distributed to 24 in-service teachers over three TAS sessions; however, we only report on the number of teachers that took part in all three sessions (N = 19). Teachers were also asked to provide a rationale for their choices. Responses were examined for emerging themes and categories; responses were then quantified based on their frequency.
Three TAS workshops were held, and teachers’ received professional development credits for each. Each workshop lasted 6 h and was led similarly: scholarly seminar led by a scientist about the possible definitions of models and how models are used in their scientific enterprise. After a short break, teachers discussed the earlier session and conversations ensued about how they implement, or might implement models further into their classroom instruction and activities. During the first session, teachers were asked to create their own models, both individually and in groups, and asked to have their students develop models (germane to their normal classroom practice) and take notes on the progress to be discussed during the next TAS workshop. During the second session, teachers were again given an activity to model phenomena, report on the use of models from the previous time. During the third session, they discussed how they used models in their classrooms.
Here, we report findings from the analysis of pencil-and-paper questionnaires delivered; one administered at the onset of the first session to gather initial perception of scientific models and the other at the end of the second session to evaluate their conception of models, and results from an activity where teacher worked in groups to develop a model. It should be made clear that the purpose of collecting these data was not to see if teacher perception of models changed over the intervention of the TAS workshop, but rather to document current understanding and use of models in their science classroom. While 25 individuals participated in at least 1 session, 19 teachers participated in all 3. In total, the questionnaires were 4 pages long, contained 8–10 open-ended questions, including a portion where teachers were asked to create their own model of some phenomena they cover in their course, and 8 Likert-type questions. Common to each questionnaire were standard questions to evaluate the program. These questions included probing participants about the extent to which the material was intellectually stimulating with requests to qualify their answer (i.e., why or why not).
Teachers were asked to describe their use of models in the classroom, their definition of models, how scientists use models, and then they were asked to develop a model themselves. Teachers were also asked to comment on the benefits and limitations of students using models in the classroom and the difficulties and ease at which they can evaluate student models and what they might look for. In addition to the open-ended questions, teachers also responded to Likert-type questions (disagree, somewhat disagree, agree, strongly agree), which included mixed questions that address the purpose of models in science and include; “Not all science fields use models,” “Models are too abstract for students to develop themselves,” “Models are mainly used for communicating ideas to other audiences outside of science,” and “it is relatively easy to explain to students how to develop models.”
Results
Generally, all teachers self-reported they found the material to be intellectually stimulating on all 3 days. Furthermore, teachers indicated that these workshops increased their enthusiasm for teaching. When asked why, responses differed but the commonality in all answers indicated that meeting other teachers, learning about the bigger picture of science, and gathering ideas for what to teach their students were particularly appealing. Repeated responses for limitations on their enthusiasm for teaching were time constraints, resources, and parent, student, and administration attitude.
What Teachers Say about Models
Teachers report that models are helpful in the classroom and, when asked, responded that model use was beneficial in several aspects of normal classroom activities. In total, the majority of teachers (80%) initially defined models as ways to show, illustrate, explain, or represent a scientific concept, while the rest (20%) discussed the dynamic properties of models that help with science inquiry and the development of ideas and hypotheses. The majority of teachers went on to give examples of models as physical representations of processes or unseen (i.e., too small, too big) structures, while one respondent said that models can be representations of hypotheses or ideas.
When asked about the role of models by scientists, respondents were split (50:45%) reporting that models are used to experiment, test, and revise ideas and as a tool for communicating concepts. Contrastingly, the Likert scale responses showed considerable disconnect between the limited answers given on the short answer portion of the survey (Table 1). For example, while the open-ended responses indicated that teachers believe that models are primarily communication and illustrative tools, Likert responses indicated the teachers strongly agreed that models are used for generating research questions and refining complicated ideas through experimentation. Results indicate that teachers define, for themselves, models as representational and communication tools, however, define models in scientific contexts as dynamic tools used to further scientific research (Table 1).
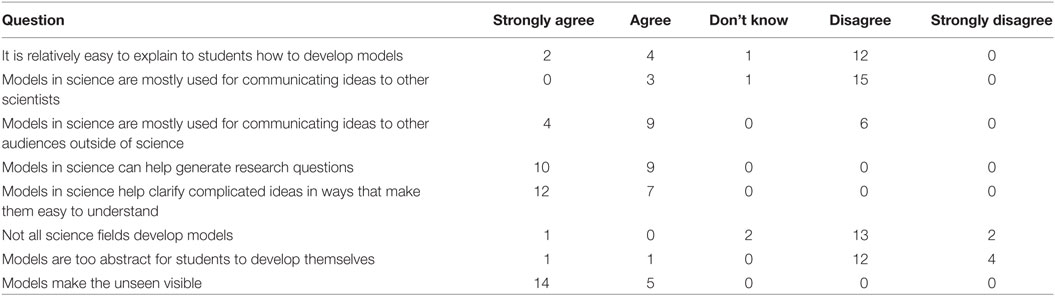
Table 1. Teacher response to Likert questions administered before the start of Teachers as Scholars sessions (N = 19).
How Teachers Develop Their Models
The science teachers were asked to create models. One task required them to individually draw a model of an aquarium (to have respondents all develop a model of the same phenomena), and the other activity involved them working in groups of three to four to model a sensory neuron cell given some background information.
In the first session, 20 teachers were asked to draw a model of an aquarium and explain their drawing. Seventeen of the 20 teachers’ drawings (85%) included structures of an aquarium where components (such as fish, filters, gravel, water, and plants) were labeled, while 1 included an explanation (with interrelated arrows) of an active process (CO2 and O2 exchange). In their written explanation, most of the teachers (70%) explained the processes that were illustrated in the model that were unseen (photosynthesis, respiration, filtration, etc.) or mentioned that the structures labeled in the drawings interact in some capacity.
Teachers were also asked to work in small groups (of three and four individuals). We present a synopsis of how each group interpreted the task of creating a model of a sensory neuron cell given some background information. The directions to the assignment asked the teachers to develop a model of the sensory neuron cell and provided some initial background information. The model building task entailed testing of potential hypotheses to elucidate how the cells function. All of the groups produced materials that were very similar to lab reports and largely mimicked the scientific method. Upon completion of the task, the groups presented their work models to the other groups. Most of the groups produced research questions, and data tables and all but one group chose to illustrate their experiment using pictures. None of the groups included a model of the actual cell, but rather, the process of how to design an experiment that might lead to the production of developing a cell model.
What Teachers Report They Do in the Classroom
The majority of teachers surveyed (85%) report using models in the classroom, and 80% report using pre-packaged science “kits” or “materials.” When asked about difficulties of using models in the classrooms, 60% mentioned lack of time and materials and 25% mentioned lack of student knowledge and deficiencies in student concentration. One teacher responded that their students have no difficulties. The majority of teachers (75%) reported that students developing their own models in the classroom helped the teachers because students tend to share knowledge with other students while working on “hands-on” model activities.
Likert responses showed that teachers disagreed with the statement “it is relatively easy to explain to students how to develop models” and indicated that they disagreed that “models are too abstract for students to develop themselves.” This suggests that a model being inherently abstract is not a factor of difficulty when teaching about models. Teachers agreed that models “made students more excited about subject material,” “helped students foster a deeper understanding,” and “helped students generate their own research questions.” Likert responses also indicated that teachers agreed that evaluating student models was easy and that drawings are often “easier to evaluate than written responses.” Open-ended question showed that teachers evaluate student models by looking for reproduction of the model used (52%) and reflecting some understanding of the concept covered (48%).
To gain further insight into how teachers’ evaluate models, 17 teachers were asked to think about a concept or process that they were currently covering in their class and asked to create a model that they would like to see from their students—and explain what aspects they saw as important when evaluating student work (Figures 1A,B). While 76% of the respondents drew a labeled process that was primarily descriptive in nature with the appropriate parts labeled (i.e., the water cycle, the nitrogen cycle, CO2–O2 exchange), two of the respondents included drawings that asked the student to represent a process and then present some argument as to why a model was developed—and provide justification for the way in which a phenomena was represented (i.e., justification for setting up a terrarium and the process of diffusion in an iodine experiment).
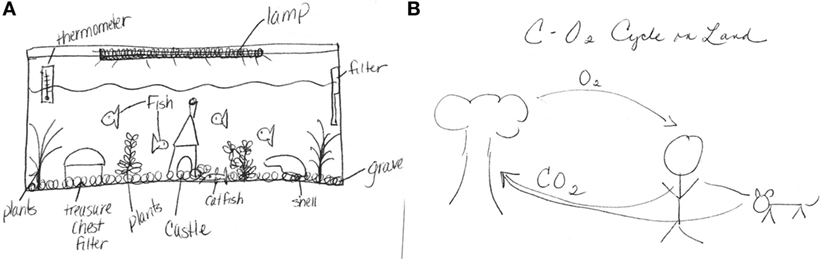
Figure 1. (A) Teacher drawing of a model of an aquarium. (B) Teacher drawing of a concept or process that they teach in their class. Drawing is an example of what they would consider a good student model.
Conclusion
The results of questionnaires indicate that teachers are torn about the utility of their model use in their classroom and in science. The majority of teachers indicated that models are useful for explaining and illustrating concepts that would otherwise be difficult to convey to their students because they are unseen (too large or too small). By contrast, the teachers seem to agree that models are useful to promoting inquiry and furthering scientific discovery when used by scientists (Table 2). These results suggest that teachers view classroom and scientific contexts has having different uses for models.
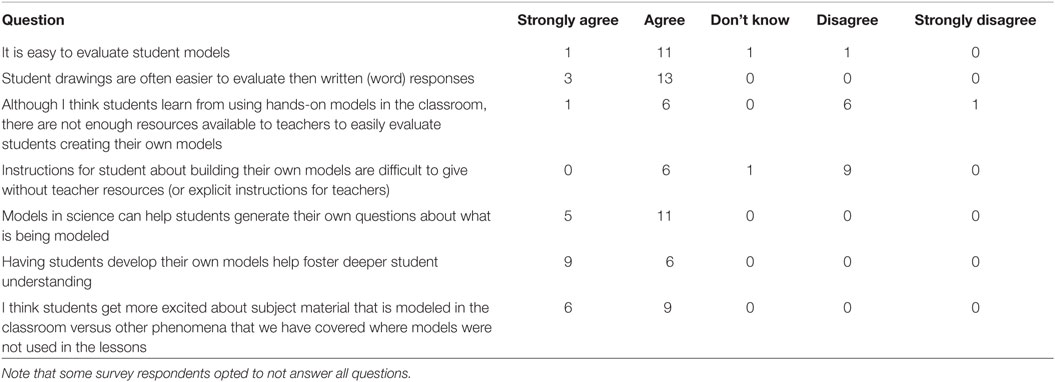
Table 2. Teacher response to Likert questions administered after second Teachers as Scholars session (N = 18).
Despite the fact that teachers are aware of the potential use of models in the classroom as thinking tools, they currently are not using them as such. While time and resource constraints are noted, it appears that the lack of models as inquiry tools is related to ease of implementation and possibly clarity on how teachers might actually teach with models. Given that teachers find model-based assessment amenable, perhaps providing model-based thinking and reasoning tools along with modeling software and curricula may increase model-based teaching among the teachers in our sample? Given that the latter are somewhat ubiquitous, we sought to address the former by creating tools that embed a conceptual representation (i.e., a cognitive framework that can help students organize complex information). Below we discuss our quantitative study, where we implemented a suite of technology-based modeling tools with an embedded complex system conceptual representation.
Part 2—Quantitative Study
Computer simulations provide an excellent context in which students can engage in dynamic modeling (Clement, 2000). These opportunities are especially important when teaching about complex systems because they provide a way in which students can represent multiple levels of abstraction and shape ideas that represent different temporal and spatial scales simultaneously (Hmelo et al., 2001; Jordan et al., 2013a,b). Model-based learning and its associated instruction is not solely teaching about models but with models. Learners should be steeped in the practice of making ideas visible through conceptual modeling, and in the practice of scientific modeling where they develop, test, and refine ideas.
For this section, we present findings from computer-generated models coded at the beginning, middle, and end of a curricular unit to determine the extent to which students represented dynamic ideas. The models analyzed were generated using a computer modeling program called Systems and Cycles which embeds the Structure, Behavior, and Function conceptual representation along with computer simulations and hypermedia to scaffold student understanding of complex systems.
The Structure–Behavior–Function (SBF) conceptual representation encourages individuals to explicitly represent a system by its structures (i.e., what are the parts?), and its behaviors (i.e., how do the parts do what they do?) its functions (i.e., what do these parts do?). Evidence suggests that this framework enables richer student explanations about complex systems (Goel et al., 1996; Hmelo-Silver et al., 2007). Furthermore, the use of computer simulations paired with direct SBF instruction has resulted in significant learning gains in the classroom in which this study takes place (Jordan et al., 2013a,b). These authors contend that it is the conceptual representation that provides for learners a framework that helps attach system function and processes to system structures. The latter, we argue, is critical to encouraging learners to move beyond the model as a representation or replication of static ideas.
Our Approach
In this study, SBF was scaffolded through the use of the Ecology Modeling Toolkit (EMT), which is part of the Systems and Cycles curricular unit. EMT combines an electronic journal, a modeling interface in which students are able to represent biotic and abiotic structures and the functional and behavioral relations between these structures, and tables for data collection and analysis. In addition, as part of the Systems and Cycles program, EMT links to RepTools. RepTools was designed to accompany a physical aquarium installed in the classroom and serve as a basis for understanding aquatic ecosystems. The kit provides digital tools which feature a function-centered hypermedia from which students can read about the structures, behaviors, and functions occurring within aquatic systems and includes a micro-level and macro-level NetLogo-based simulation. The macro-level simulation enables students to test ideas about fish spawning and water quality, and the micro-level simulation enables testing of ideas about the nitrification process that occurs within an aquarium as part of its biological filtration. In combination, these digital tools allow students to not only test ideas about a model complex system (e.g., aquatic ecosystems) but also to explain processes and outcomes that occur at multiple levels in aquatic systems.
Classroom Context
The Systems and Cycles intervention was used in one seventh-grade (middle school) science classroom in the northeastern United States as part of the routine classroom instruction for a period of 6 weeks. Forty-two students participated in this study, about 21 in each of 2 class periods. This intervention was integrated as a part of normal curricular instruction, aligned with curriculum goals for that unit period. No identifying information about student participants was collected, and the normal classroom teacher led the entire intervention, ensuring anonymity of students to the researchers. Students worked with the computer tools in small groups of four to five students for the duration of the unit. The teacher began the unit by introducing the concepts of modeling and complex systems before moving into detailed discussions of ecological content. The curricular unit covered aquatic ecosystems with a focus on aquariums and ponds.
To teach about aquatic ecology and to motivate students to reason about the relationship between structure, behaviors, and functions of pond and aquarium systems, the unit began with a problem-based scenario which students were expected to investigate and explain. Through dummy news reports based on real events, students were expected to gather evidence and model phenomena that explained a sudden fish kill in a local lake brought about because of intense eutrophication and a resulting hypoxic event. Students were asked to create an initial model that explained the phenomena and over the course of the unit revise this model as they were provided with content about important structural and functional aspects of healthy aquatic ecosystems. The unit was designed to motivate students to reason about the interactions between biotic and abiotic components of these systems at multiple scales using a facsimile of a real-world event.
Data Analysis
Student models were collected and analyzed at the beginning of the curricular unit, during the middle of the unit, and after unit completion yielding a total of 27 models for analysis (3 for each of the 9 groups). This allowed us to analyze student’s initial understanding the system, how students revised their models given new information, and how students ultimately decided to represent their understanding over a period of learning.
We applied the SBF coding schemes to all of the student models [see Hmelo-Silver et al. (2007) for review of coding scheme]. First, to understand the types and chronological order of the ideas students brought to the modeling interface, we applied a Structure, Behavior, and Function scheme which allowed us to uncover what types—and in what frequency and order—students tended to explain phenomena in the pond. For this analysis, student models were coded by the type and number of structures, type and number of behaviors, and type and number of functions included in each of the three models over time (see Figure 2 for an example). Second, to understand how organization of student ideas change over time and how students incorporated new information into their models, we looked how many ideas included in the models changed from the initial model to the final model. Two coders were trained to code based on these schemes and reached reliability rate of 0.92 for all 27 models.
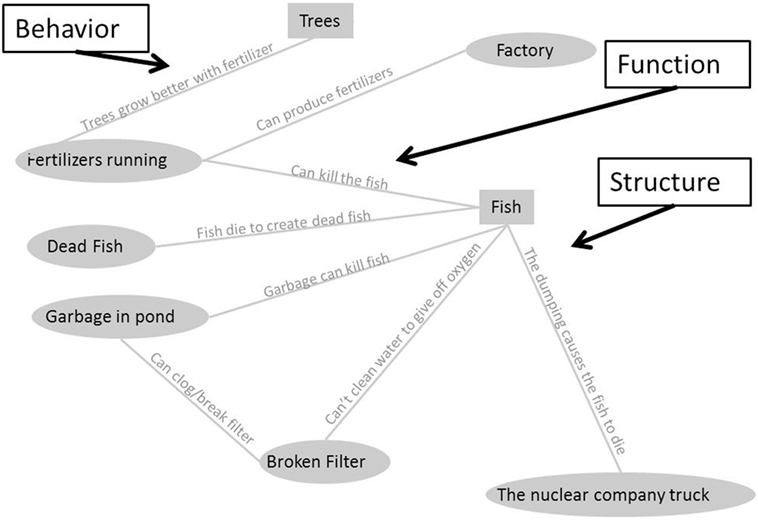
Figure 2. Examples of structures, behaviors, and functions included in a group’s Ecology Modeling Toolkit model.
Our Findings
Our first coding analyses indicated that the students’ initial models represented a structure-centric view of the system (Table 3). Students tended to focus on structural components of the system and included, on average, eight structures in their initial models, one function in their initial model, and most did not represent any behaviors. For their revised model, which was measured about half way through the curricular unit, students continued to represent the system structurally by increasing the structures represented on average to almost nine structures, two functions, and one behavior. In student’s final models, the structures included in student models decreased to around 6 on average, while functions increased slightly to 2 and behaviors increased to almost 3.
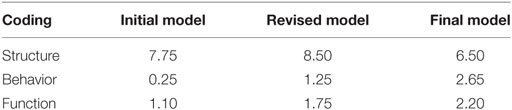
Table 3. Mean number of structures, behaviors, and function included in student’s initial revised and final models.
For our second coding analysis, by the simple measure of frequency of ideas represented across the three models, students began the modeling process by listing the structures potentially involved with the system operation based on the information presented and their prior knowledge to develop a hypothesis about what might have caused the fish kill. When measured in the revised models, students then added to their existing list of structures, new structures and components encountered in the curriculum. Finally, in the final models, students reduced the amount of structures while adding to their representations additional behaviors and functions. The increase in number of structures in their revised model before decreasing the structures in their final models may be an indication that students may add new information they encounter to their initial explanation before ultimately eliminating unnecessary structural components.
Conclusion
Through our model analyses, we conclude that as students move toward a more complex understanding of complex aquatic systems, they first establish structural components which comprise the system, then move to functional relationships which define the purpose of the system, and end by filling in the behavioral relationships which represent the mechanisms which allow these systems to function. Additionally, our data indicate that students begin the modeling task by representing their current ideas or hypotheses about the system, and then add to this understanding additional information they encounter about the system before ultimately subtracting non-relevant information and settling in on a final representation. The latter has been seen with SBF type models in the undergraduate classroom as well (Dauer et al., 2013).
Discussion
In the first study, we found that teachers appear to view model use in the classroom as distinct from scientific contexts. In the classroom, models are used as communication tools and not to support thinking, despite the fact that teachers recognize the value of thinking with models. In our second study, teachers’ view of the value of models was supported in that students were able to think more deeply about system-level mechanisms and functions in complex ecosystems. Therefore, it appears that ease of use and support in doing so are necessary to encourage models as tools for thinking in the classroom. We provided an example of a classroom implementation of a model-based curriculum supported by teacher professional development.
An Internet search reveals a large number of modeling tools for teachers, yet only a few are associated with professional development. Perhaps, given the suite of goals for science classroom teachers, professional development opportunities are not judged as being critically important? This may change as US states accept the NGSS.
A critical feature of the NGSS is the connection between classroom practice and the development of epistemic practices that are consistent with science. It has been shown that student beliefs that are more closely aligned with the epistemology of science are correlated with academic performance (e.g., Schommer, 1990; Linn and Hsi, 2000). Model-based instruction has been shown to support the epistemic links between data and evidence (Chinn and Malhotra, 2002).
It is clear that students need a sufficient understanding of concepts and claims in science but to truly engage in scientific thinking students also need also to understand the process by which scientists and they themselves can reliably know what they know. Therefore, the means to engage students in authentic scientific reasoning has gained considerable scrutiny in both the fields of science education and educational psychology. Indeed, some argue that scientists essentially use epistemic criteria to evaluate the validity and accuracy of scientific models and arguments (Kuhn and Weinstock, 1997; Chinn and Malhotra, 2002). The authors of the latter argue that at that time of publication, typical textbooks and even many researcher designed classroom tools did not support cognitive processes that are often used by scientists. Furthermore, they contend that the epistemology (i.e., the nature of the knowledge) that underlies many classroom tasks is very different from that which underlies authentic science.
A major area of distinction between the processes and epistemology between scientific reasoning and classroom reasoning tasks is the coordination between data and theory. Without a sense of how data are used as evidence to construct theory, students can be easily misled by issues of data variability, uncertainty, and conflict. Students’ epistemic beliefs are particularly important when confronting new ideas and evidence in science, which tend to be causal, mechanistic, and function driven. Chan et al. (1997) describe how students process scientific information that is inconsistent with their current ideas. Either students directly assimilate ideas by fitting them in their minds with what is already known or they engage in knowledge building. The latter refers to seeing inconsistent information as a problem that needs a solution. In searching for a solution, students will question their ideas and the new information about which they are learning. This active processing of information can help to regulate conceptual change and will likely elucidate science as a system versus a static enterprise. We argue that models provide an ideal platform for students to actively process new information about complex ideas about mechanisms and functions. Doing so can prove quite challenging, and therefore, the use of scaffolds such as the SBF representation in the study provided above can result in deeper multilevel integration of complex system ideas.
Our findings show that while modeling with a conceptual representation can be fruitful, teachers may not share our view of modeling as a workable classroom inquiry task. Most of the teachers recognized the value of models in scientific inquiry but models are used more for communication, not guiding inquiry, in the classroom. Certainly resource barriers are an issue, but it also appears that teachers may not know how to engage learners in modeling to learn tasks. Fortunately, over the past several years, educational researchers have been developing ways to make modeling a central feature in the scientific classroom. We suggest that the use of a conceptual representation as defined above may be another valuable tool in the resources now being made available for teachers. Additionally, while the idea of modeling can be conceptualized broadly by different users and audiences, we emphasize that modeling in the classroom should move beyond a graphical representation of a system as emphasized in this manuscript. However, these tools will not be used by teachers without proper professional development support. Given the value of modeling as an authentic practice in the understanding of complex system dynamics and other cross-cutting concepts featured in the NGSS, we recommend that teacher professional development on model use in the classroom be more ubiquitous.
Ethics Statement
All work was conducted in concordance with Rutgers University’s Institutional Review Board policy.
Author Contributions
All the authors contributed equally to the progress and submission of this manuscript.
Conflict of Interest Statement
The authors declare that the research was conducted in the absence of any commercial or financial relationships that could be construed as a potential conflict of interest.
Acknowledgments
The authors thank the numerous students and teachers involved with this project. In addition, they acknowledge their funding sources: Woodrow Wilson Foundation and the National Science Foundation (DRL: 0959756). All research was conducted with Rutgers University Institutional Review Board approval. The authors would also like to thank the reviewers of this manuscript for their thoughtful comments.
Funding
This research was supported by the National Science Foundation Grant # DRL 0632546.
References
Carey, S., and Smith, C. (1993). On understanding the nature of scientific knowledge. Educ. Psychol. 28, 235–251. doi: 10.1207/s15326985ep2803_4
Chan, C. K. K., Burtis, J., and Bereiter, C. (1997). Knowledge building as a mediator of conflict in conceptual change. Cognition Instruct. 15, 1–40. doi:10.1207/s1532690xci1501_1
Chinn, C. A., and Malhotra, B. A. (2002). Epistemologically authentic inquiry in schools: a theoretical framework for evaluating inquiry tasks. Sci. Educ. 86, 175–218. doi:10.1002/sce.10001
Clement, J. (2000). Model based learning as a key research area for science education. Int. J. Sci. Educ. 9, 1041–1053. doi:10.1080/095006900416901
Crawford, B., and Jordan, R. (2013). “Inquiry, models, and complex reasoning to transform learning in environmental education,” in Trading Zones in Environmental Education, eds M. Krasny and J. Dillon (New York, NY: Peter Lang), 105–127.
Crawford, B. A., and Cullin, M. J. (2004). Supporting prospective teachers’ conceptions of modeling in science. Int. J. Sci. Educ. 26, 1379–1140. doi:10.1080/09500690410001673775
Dauer, J. T., Momsen, J. L., Bray-Speth, E., Makohon-Moore, S., and Long, T. M. (2013). Analysis of student-constructed models of complex biological systems. J. Res. Sci. Teach. 50, 639–659. doi:10.1002/tea.21094
Gilbert, J. K. (1993). Models and Modeling in Science Education. UK: Association for Science Education.
Goel, A. K., Gomez, A., Grue, N., Murdock, W., Recker, M., and Govindaraj, T. (1996). “Towards design learning environments: explaining how devices work,” in Intelligent Tutoring Systems, eds C. Frasson, G. Gauthier, and A. Lesgold (Berlin: Springer), 493–501.
Hmelo, C. E., Ramakrishnan, S., Day, R. S., Shirey, W., Brufsky, A., Johnson, C., et al. (2001). The oncology thinking cap: scaffolded use of a simulation to learn about designing clinical trials. Teach. Learn. Med. 13, 183–191. doi:10.1207/S15328015TLM1303_8
Hmelo-Silver, C. E., Marathe, S., and Liu, L. (2007). Fish swim, rocks sit, and lungs breathe: expert-novice understanding of complex systems. J. Learn. Sci. 16, 307–331. doi:10.1080/10508400701413401
Jordan, R. C., Hmelo-Silver, C., Lei, L., and Gray, S. A. (2013a). Fostering reasoning about complex systems: using the aquarium to teach systems thinking. Appl. Environ. Educ. Commun. 12, 55–64. doi:10.1080/1533015X.2013.797860
Jordan, R. C., Gray, S. A., Brooks, W. R., Honwad, S., and Hmelo-Silver, C. E. (2013b). Process-based thinking in ecosystem education. Nat. Sci. Educ. 42, 68–74. doi:10.4195/nse.2012.0012
Kuhn, D., and Weinstock, M. (1997). “What is epistemological thinking and why does it matter?” in Personal Epistemology: The Psychology of Beliefs about Knowledge and Knowing, eds B. K. Hofer and P. R. Pintrich (NJ: Erlbaum), 121–144.
NGSS. (2013). Next Generation Science Standards: For States, By States. Washington, DC: The National Academies Press.
Rosenblueth, A., and Wiener, N. (1945). The role of models in science. Philos. Sci. 12, 316–321. doi:10.1086/286874
Schommer, M. (1990). Effects of beliefs about the nature of knowledge on comprehension. J. Educ. Psychol. 82, 498–504. doi:10.1037/0022-0663.82.3.498
Shernoff, D. (2013). Optimal Learning Environments to Promote Student Engagement. New York, NY: Springer.
Treagust, D. F., Chittleborough, G., and Mamiala, T. L. (2002). Students’ understanding of the role of scientific models in learning science. Int. J. Sci. Educ. 24, 357–368. doi:10.1080/09500690110066485
Keywords: modeling, conceptual representation, complex systems, education, technology
Citation: Jordan RC, Gray S, Sorensen AE, Pasewark S, Sinha S and Hmelo-Silver CE (2017) Modeling with a Conceptual Representation: Is It Necessary? Does It Work? Front. ICT 4:7. doi: 10.3389/fict.2017.00007
Received: 10 February 2017; Accepted: 31 March 2017;
Published: 20 April 2017
Edited by:
Fabrizio Consorti, Sapienza University of Rome, ItalyReviewed by:
Gabriella Agrusti, Libera Università Maria SS. Assunta, ItalyAdamantios Koumpis, University of Passau, Germany
Copyright: © 2017 Jordan, Gray, Sorensen, Pasewark, Sinha and Hmelo-Silver. This is an open-access article distributed under the terms of the Creative Commons Attribution License (CC BY). The use, distribution or reproduction in other forums is permitted, provided the original author(s) or licensor are credited and that the original publication in this journal is cited, in accordance with accepted academic practice. No use, distribution or reproduction is permitted which does not comply with these terms.
*Correspondence: Rebecca C. Jordan, rebecca.jordan@rutgers.edu