- 1Department of Immunology, University of Texas MD Anderson Cancer Center, Houston, TX, United States
- 2School of Life Science and Technology, University of Electronic Science and Technology of China, Chengdu, China
- 3Department of Microbiology and Molecular Cell Biology, Eastern Virginia Medical School, Norfolk, VA, United States
T follicular helper (Tfh) cells play key role in providing help to B cells during germinal center (GC) reactions. Generation of protective antibodies against various infections is an important aspect of Tfh-mediated immune responses and the dysregulation of Tfh cell responses has been implicated in various autoimmune disorders, inflammation, and malignancy. Thus, their differentiation and maintenance must be closely regulated to ensure appropriate help to B cells. The generation and function of Tfh cells is regulated by multiple checkpoints including their early priming stage in T zones and throughout the effector stage of differentiation in GCs. Signaling pathways activated downstream of cytokine and costimulatory receptors as well as consequent activation of subset-specific transcriptional factors are essential steps for Tfh cell generation. Thus, understanding the mechanisms underlying Tfh cell-mediated immunity and pathology will bring into spotlight potential targets for novel therapies. In this review, we discuss the recent findings related to the molecular mechanisms of Tfh cell differentiation and their role in normal immune responses and antibody-mediated diseases.
Introduction
Germinal centers (GCs) are secondary lymphoid structures within B cell follicles where B cells go through affinity maturation and class-switch recombination to generate high-affinity antibodies (1, 2). GC reactions play a critical role in the invasion of pathogens, while abnormal GC reactions are implicated in systemic autoimmune diseases, chronic inflammation, allergic responses, and B cell malignancies. The GC reaction is initiated and amplified by GC B cell and CD4+ T cell interactions followed by T follicular helper (Tfh) cell help to B cells which leads to the generation of long-lived serological memory (2, 3). Exaggerated expansion of Tfh cells results in excessive GC reactions, self-reactive B cell proliferation, and increased long-lived plasma cell differentiation, as well as an overproduction of high-affinity pathogenic autoantibodies (4). Understanding the development and function of Tfh cells is important for generating new vaccine strategies against pathogens as well as targeted approaches to abrogate the inappropriate activity of these cells in patients with various autoimmune diseases.
T follicular helper cell development occurs in a stepwise process (1, 2). Contact of naïve CD4+ T cells with antigen-presenting dendritic cells (DCs) within T cell follicles is the first step of commitment toward Tfh cell differentiation (Figure 1). This step of Th cell development, named as pre-Tfh is reflected by upregulation of CXC chemokine receptor 5 (CXCR5) expression as well as key genes in the Tfh pathway such as B-cell lymphoma 6 protein (Bcl6), Achaete-scute homolog 2 (Ascl2), ICOS, programmed cell death-1 (PD-1), and Batf, and the downregulation of CC chemokine receptor 7 (CCR7) expression. These changes guide the pre-Tfh cells to the T/B cell border where proper signals received from B cells trigger a further increase in the Tfh-associated gene expression pattern (Bcl6, PD-1, ICOS, and CXCR5), commitment to the functional GC Tfh cell program and subsequent GC formation. While in mice, IL-6, IL-21, and Bcl6 are essential for Tfh formation, in humans, Tfh generation relies on TGF-β, IL-12, IL-23, and Activin A signaling (5–9). In addition, Tfh cell differentiation is negatively controlled by cytokines (IL-2 and IL-7) costimulatory molecules [cytotoxic T lymphocyte antigen 4 (CTLA4) and PD-1], and transcriptional factors [signal transducers and activators of transcription (STAT)5, Blimp-1, FOXO1, Foxp1, and Krüppel-like factor 2 (Klf2)] (10–16).
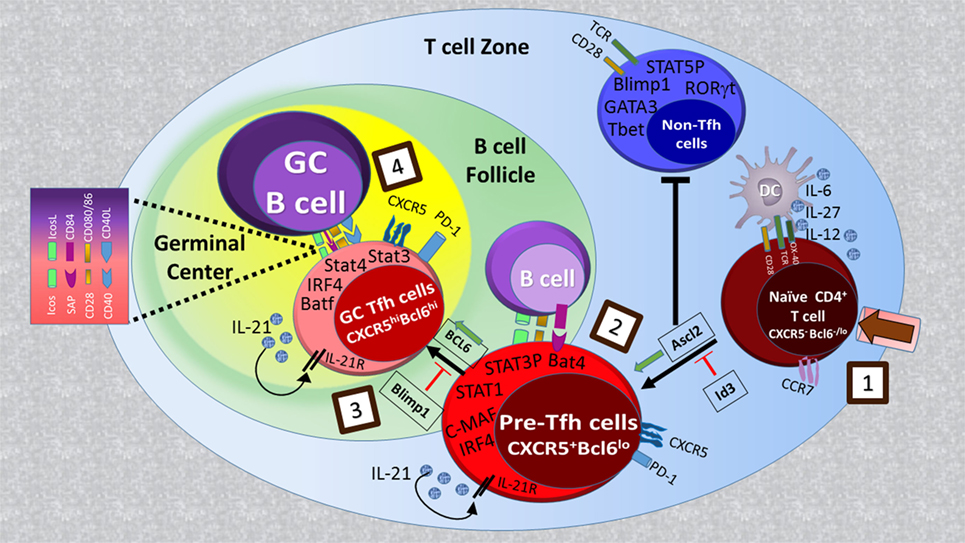
Figure 1. Regulatory signaling for T follicular helper (Tfh) cell development: naïve CD4+ T cell priming by MHC/antigen interaction on DCs (step 1) leads to the generation of CXCR5+Bcl6lo pre-Tfh cells with increased activity of transcriptional factors such as Achaete-scute homolog 2 (Ascl2), signal transducers and activators of transcription (STAT)1, STAT3, IFN-regulatory factor 4 (IRF4), and Batf (step 2). Ascl2 expression in activated T cells orchestrates them to migrate toward B cell follicles by upregulation of CXC chemokine receptor 5 (CXCR5) expression and repressing IL-2R-Blimp1 pathway as well as Th1, Th2, and Th17 cell differentiation. Upon interaction with cognate B cells at T-B border (step 3), CXCR5+ T cells begin to upregulate B-cell lymphoma 6 protein (Bcl6) expression, which in cooperation with other transcription factors determines the final differentiation state of Tfh cells within germinal centers (step 4).
In this review, we have discussed positive and negative regulation mechanisms of mouse and human Tfh differentiation including costimulation and cytokine driven signaling pathways and subsequent activation of downstream transcriptional factors. We also elaborate on the cellular requirement of other follicular T cells within GCs for Tfh cell development and GC formation. Moreover, we will review the current advances in Tfh cell biology in various disease settings.
Tfh Cells in Mice and Humans
Several seminal discoveries made in humans and mice in the early 2000s led to identification of B follicular helper T cells that are indispensable for GC formation and B cell function (17, 18). These cells express high levels of CXCR5 and low CCR7 in both humans and mice (17–20). CXCR5 expression is indispensable for T cell migration from T zones toward CXCL13-rich B-cell follicles, where they interact with B cells for further maturation and then provide help for the generation of high-affinity antibodies and long-lived plasma cells (21–24). Thus, based on their localization and function, CXCR5+ CD4+ T cells were designated as Tfh cells.
Profiling of cytokine and gene expression patterns provided the evidence that mouse and human Tfh cells are distinct from Th1 and Th2 subsets and help B cells by delivering activating signals with CD40L and the cytokine IL-21 (3, 6, 18, 25). Moreover, these mouse and human gene-profiling experiments helped to identify key molecules including Bcl6, Ascl2, IL-21, PD-1, and ICOS which play a substantial role in Tfh cell development, migration, homeostasis, and function in both species. In 2009, three independent groups identified the essential function of the transcription factor (TF) Bcl6 for Tfh cell development and function (7, 26, 27). Since then Tfh cells have been recognized as a distinct lineage of T helper cells.
Studies in mice and humans show that Tfh cells are localized in lymphoid organs and are composed of subsets that differ in their localization, phenotype, and function (28). In mice, after priming with DCs in the T zones of secondary lymphoid organs, a fraction of naive CD4+ T cells acquire CXCR5, PD-1, and Bcl6 expression, downregulate CCR7, and migrate toward B cell follicles (Figure 1) (21, 29–32). These CXCR5+Bcl6+CD4+ T cells, called Tfh precursors (pre-Tfh), interact with antigen-presenting B cells and further differentiate to fully programmed GC Tfh cells, which provide help to B cells within GCs. GC Tfh cells can be distinguished from Tfh precursors by high expression levels of CXCR5 and PD-1 (28). Sustained expression of Bcl-6 in GC Tfh cells is essential for GC formation (7, 26, 27). The origin of Tfh cells is not restricted to naive cells, and there is some evidence suggesting that other Th subsets including Th1, Th2, Th17, and regulatory T cells (Tregs) may become Tfh cells in GCs (3). This is consistent with the heterogeneity in cytokine expression patterns among GC Tfh cells developed under different immunization protocols and by different types of infectious agents (33). In human tonsils, CXCR5loICOSlo pre-Tfh cells (or extrafollicular helper T cells) express multiple Tfh molecules including CD40L, IL-21, and CXCL13 but not Bcl6 and are localized outside of GCs where they help naïve B cells to become immunoglobulin-producing cells (34). By contrast, CXCR5hiICOShiPD-1hi GC Tfh cells provide help to GC B cells and promote their survival, proliferation, and differentiation into immunoglobulin-producing cells (28). In human tonsils, GC Tfh cells contain subsets coexpressing Bcl-6 and RORγt, and Bcl-6 and T-bet (8). This suggests that other Th subsets may be able to differentiate into Tfh cells or that Tfh cells share the same developmental path with Th1 and Th17 cells as observed in mice (35, 36).
A CXCR5+ subset of CD4+ T cells can also be identified in the peripheral blood of mice and humans, subsequently referred to as circulating Tfh (cTfh) cells. Although these CCR7loPD-1+ cells express lower levels of ICOS and PD-1 and seldom express Bcl6 they are closely related to Tfh cells (37). Their differentiation depends on ICOS and Bcl6 but not SLAM-associated protein (SAP), suggesting that cTfh cells are primary Tfh precursors or early memory Tfh cells. The cTfh cell subset undergoes active Tfh differentiation into mature Tfh cells in secondary lymphoid organs upon antigen reencounter and their presence correlates with autoimmune diseases such as lupus and rheumatoid arthritis (RA) (37). In addition, based on the expression of CXCR3 and CCR6, human cTfh cells can also be subdivided into three main subsets, namely, CXCR3+CCR6− (Tfh1), CXCR3−CCR6− (Tfh2), and CXCR3−CCR6+ (Tfh17) cells (38, 39). Tfh2 and Tfh17 cells, but not Tfh1 cells, represent efficient B cell helper cells to regulate immunoglobulin isotype switching (38). A recent data showed that in addition to cTfh memory, Tfh cells could be local in the draining lymphoid organs and sustain B cell responses after reactivation (40). In contrast to cTfh cells, local memory Tfh cells promote plasma cell differentiation and could be released to the circulating memory compartment over time.
Tfh Cell Differentiation
T follicular helper cell differentiation is a complex process, which is tightly regulated (41) (Figure 1). It begins during naïve CD4+ T cell priming by DCs in the T cell zone of secondary lymphoid tissues and continues through the first cognate T cell:B cell interaction at the T–B junction until Tfh cells differentiate into mature GC Tfh cells when they enter follicles (2, 3, 42, 43). At each of these stages, Tfh cell development is influenced by signaling pathways downstream of cell surface molecules including the T cell receptor (TCR), costimulatory molecules, and cytokine receptors leading to the activation of specific transcriptional machinery (2, 3, 42, 43).
Costimulatory Molecules
T follicular helper cell differentiation from naive CD4+ T cell precursors is a multistep process which requires costimulatory signals. Positive [CD28, ICOS, SAP, glucocorticoid-induced tumor necrosis factor receptor-related protein (GITR), etc.] and negative [CTLA4, PD1, and B and T lymphocyte attenuator (BTLA)] costimulation works together with TCR signaling during Tfh cell development to guide activation, proliferation, differentiation, migration, survival, and effector functions (1–3, 33, 44). Imbalance between positive and negative costimulation signals leads to increased Tfh cell number and consequently to Tfh-driven autoimmunity (45, 46).
Positive Costimulation
CD28 and ICOS
CD28 and ICOS are two structurally and functionally related costimulatory molecules that are critical for Tfh cell differentiation (2, 47, 48). CD28, which is constitutively expressed on both naïve and resting T cells, specifically binds to its ligands CD80 or CD86 and regulates T-dependent B cell responses (49, 50). While previous studies suggest the overlapping function of CD80 and CD86 in antibody responses, during virus infection CD86 expression on B cells but not CD80 is critical for Tfh cell generation and function (51). CD28 signaling regulates (i) early key events of Tfh differentiation, especially the expression of PD-1, ICOS, OX-40, Bcl6, and CXCR5 (47); (ii) the late stage of Tfh differentiation as B7 ligand blockade during ongoing infection impairs Tfh cell response (52, 53); and (iii) Tfh cell survival (53).
ICOS, another CD28 family member, is highly expressed on Tfh cells and is critical for Tfh cell generation and GC formation (2, 48). Unlike CD28, ICOS does not regulate any of the early differentiation steps of naïve T cells into Tfh cells, particularly Bcl6 expression (47); however, ICOS is important to maintain the phenotype of already differentiated Tfh cells (47). Mice in which the ICOS–ICOSL interaction is disrupted as well as ICOS-deficient patients have fewer Tfh cells and smaller GCs (6, 47). ICOS exerts its costimulatory function through phosphoinositide 3-kinase (PI3K) signaling which results in the activation of Akt (47). Akt phosphorylates the transcriptional factor FOXO1 which thereby stays in the cytoplasm and becomes functionally inactive (47). This is critical because FOXO1 suppresses Tfh cell differentiation through the negative regulation of Bcl6 and positive regulation of Klf2 expression (15). Klf2 plays a negative role in Tfh differentiation by binding to promoter regions of CXCR5, CCR7, CD62L, PSGL-1, and S1pr1, which leads to the suppression of CXCR5, relocation of Tfh cells from B cell follicles back to T cell zone and their phenotype reversion to non-Tfh cells (44). Besides the ICOS–Klf2 axis, ICOS promotes interaction of the p85a regulatory subunit of PI3K with osteoponin (OPN-i), followed by translocation of OPN-I into the nucleus, its interaction with Bcl6 and protection of Bcl6 from proteasome degradation; thus, sustain responses by Tfh cells (54).
SLAM-Associated Protein
SLAM-associated protein is an intracellular adaptor protein essential for the function of SLAM family receptors (SFRs) to regulate immune responses (55, 56). SFRs consist of nine members, four of which are highly expressed on Tfh and B cells: CD150/SLAM, CD229/Ly9/SLAMF3, CD84/SLAMF5, and NTB-A/Ly108/SLAMF6 (57–59). High intrinsic SAP expression in both human and mouse GC Tfh cells, but not in B cells, is important for the development of long-term humoral immunity: particularly formation of GCs, long-lived plasma cells, and memory B cells (57, 60, 61). Bcl6 is required for SAP expression in GC Tfh cells (62). SAP through SFRs regulates T:B cell adhesion, cytokine production, and TCR signaling strength (63). Patients with X-linked lymphoproliferative disease (XLP) caused by a SAP gene mutation as well as mice lacking SAP expression, display defects in GC Tfh cell generation and GC reactions (3, 55). While SAP-deficient CD4+ T cells express normal levels of CXCR5, ICOS, and Bcl6, they have an impaired ability to stably interact with cognate B cells and sustain GC reactions (58, 61, 64, 65). Thus, SAP is not required for early Tfh cell differentiation (Bcl6+CXCR5+), but is indispensable for sustained T:B cell interactions and the full polarization to GC Tfh cells (Bcl6hiPD1hi) mainly by the following mechanisms: (1) SAP binds phosphotyrosines of the SLAM immunotyrosine switch motifs (ITSM) and mediates positive signaling by recruiting Src family kinase Fyn and PKCθ (66); (2) SAP competes with the tyrosine phosphatase SHP-1 for Ly108 ITSM binding, signaling through which contributes to reductions of T:B contacts and to an impairment in GC Tfh cell generation and GC responses (58). In addition to regulating T:B adhesion, positive signaling through SAP and PKCθ regulates cytokine secretion by GC Tfh cells, particularly IL-4 (60, 67). Moreover, Sap/Ly108 engagement sustains the TCR signaling that is required for establishing the Tfh cell:B cell synapse and thus allowing Tfh cells to provide help to B cells (68).
Glucocorticoid-Induced Tumor Necrosis Factor Receptor-Related Protein
Glucocorticoid-induced tumor necrosis factor receptor-related protein (TNFRSF18, and CD357), a member of the tumor necrosis factor receptor superfamily, is expressed at high levels in Tregs and activated T cells (69). Activation of GITR by its natural ligand GITRL enhances proliferation and effector T cell responses and inhibits Treg mediated suppression (70, 71). GITR is highly expressed on Tfh cells compared with non-Tfh cells in the spleens of collagen-induced arthritis (CIA) mice (72). GITR signaling promotes Tfh cell expansion and survival. Administration of GITR-Fc protein greatly reduces CIA severity by suppressing Tfh development and thereby humoral immune responses. In addition, in the chronic lymphocytic choriomeningitis virus (LCMV) infection model, a Tfh cell intrinsic role for GITR in sustaining Tfh cell responses and LCMV-specific antibody production has been identified (73). Thus, GITR signaling is considered as a positive regulator of Tfh generation (44).
Negative Costimulation
Programmed Cell Death-1
Programmed cell death-1 (PDCD1 and CD279) is a member of the CD28 superfamily which is transiently expressed by activated conventional T cells and delivers an inhibitory signal by engaging its ligands PD-L1/CD274/B7-H1 and PD-L2/CD237/B7-DC that are expressed on activated B cells, DCs, and macrophages (74). PD1 shows high and sustained expression on exhausted T cells, Tregs, Tfh, and T follicular regulatory (Tfr) cells (75–78). In addition to conventional T cells, PD-1 is expressed by B cells, natural killer cells, and myeloid cells as well (79, 80). Early studies utilizing complete knockouts of PD1, PD-L1, or PD-L2, or their respective blocking antibodies tried to address the role of the PD-1 pathway in controlling humoral immunity. Interestingly, some studies have shown attenuated humoral immune responses upon PD-1 signaling blockade, whereas others have found enhanced responses (74, 81–85). Later work using cell-type specific deletion of PD1 and its ligand helped to delineate the function of PD1 signaling in GC responses and gain insight into the individual role of PD1 in Tfr and Tfh cells and PD1 ligands in DCs and B cells in the regulation of humoral immunity (77). The PD-1–PD-L1 pathway plays an immunoregulatory role in limiting the differentiation and suppressive function of Tfr cells (77). PD-1 expression can also modulate Tfh differentiation and function. Tfh cells from aged mice express higher levels of PD-1 compared with Tfh cells in young mice; PD-1 blockade in aged Tfh cells restores Tfh cell function, suggesting a cell-intrinsic role of PD1 in Tfh cells (12, 86). Interestingly, PD-L1 expression on DCs, but not B cells, inhibits Tfh and Tfr cell differentiation (87).
Cytotoxic T Lymphocyte Antigen 4
Cytotoxic T lymphocyte antigen 4 is a key checkpoint in immune tolerance (88–92). While CTLA4 and CD28 share the same ligands CD80 and CD86, CTLA4 interacts with them with higher affinity and avidity compared with CD28 (48). The central tenet of CTLA4 function is to regulate CD28 signaling, since fatal multiorgan inflammation as well as increased antibody levels in CTLA4 KO mice are prevented by the blockade of CD80 and CD86 (93–95). CTLA4 is constitutively expressed in Tregs and upregulated after activation in conventional T cells and plays a key role in mediating Treg function and in controlling conventional T cells (48). In fact, a Treg-specific deletion of CTLA4 recapitulates the phenotype of germline CTLA4 KO mice including increase in antibody production, indicating the role of CTLA4 in Tregs to control B cell responses (96). Recently, multiple groups assessed the role of CTLA4 in B cell responses and identified the function of CTLA4 on multiple T cell subsets including Tfh, Tfr, and Tregs in regulating humoral immune responses (13, 97). While it was suggested that CTLA4-dependent suppression is the primary mechanism used by Treg and Tfr cells to control Tfh cell development and humoral immunity via CTLA4-dependent downregulation of CD80 and CD86 on B cells, Foxp1-dependent CTLA4 expression on non-Treg CD4+ cells has cell-intrinsic and negative regulatory functions in Tfh cell differentiation, maintenance, and function (13). CTLA4 controls Tfh cell differentiation by regulating the degree of CD28 engagement (52).
B and T Lymphocyte Attenuator
B and T lymphocyte attenuator (CD272) is an inhibitory receptor expressed on T and B cells that binds TNFR family member herpesvirus entry mediator and attenuates T and B cell activation and effector functions (98–100). Mice lacking BTLA exhibit increased antigen-specific IgG responses and with age gradually develop autoimmune hepatitis-like disease and autoantibody production to nuclear antigens (101), suggesting that BTLA negatively regulates humoral immune responses. BTLA is highly expressed in CXCR5+ Tfh cells compared with conventional CXCR5− CD4+ T cells. While Tfh cell development is not affected in BTLA-deficient mice, BTLA expression in Tfh cells but not in B cells is critical to control GC B cell development and antigen-specific IgG2a and IgG2b production (102). Moreover, BTLA controls Tfh-mediated B cell responses by suppressing IL-21 production (102).
Cytokines
Along with antigen and costimulation signaling, specific cytokine-dependent cues play a central role in governing naive CD4+ T cell differentiation into specific effector T helper cell subsets. For example, IL-12 and IFNγ promote Th1 differentiation, whereas IL-4 drives Th2 differentiation (42). In addition, IL6 and IL-21 in combination with TGFβ induce Th17 differentiation (42). There are multiple cytokines that exercise either positive or negative roles at different stages of Tfh development (1, 2). However, cytokine-dependent Tfh cell formation varies between mice and humans (1, 42). Particularly, while TGFβ signaling opposes Tfh development in mice, it is required for human Tfh cell development (42).
Cytokines That Support Tfh Cell Formation in Mice and Humans
IL-6, IL-21, and IL-27
IL-6, IL-21, and IL-27 have all been implicated in Tfh cell development, although with differing roles (1, 2, 6, 7, 103, 104). IL-6 is mainly derived from activated B cells, DCs, and follicular DCs and is required in the initial stage of Tfh cell formation by inducing Bcl6 and IL-21 expression (5, 103, 105, 106). Mice deficient in IL-6 or IL-6R show reduced or delayed Tfh cell formation due to impaired signaling through STAT3 and STAT1 (5, 107). In addition, at the late stage of chronic viral infection, IL-6 derived from activated follicular DCs is crucial for maintenance of Tfh cell by upregulation of Bcl6 and viral control (3). Similar to mice, in humans, IL-6 derived from circulating plasmablasts is also a potent inducer of Tfh differentiation (108). IL-21 is primarily produced by select CD4+ T cells including Tfh, Th17 cells, and natural killer T (NKT) cells and plays a more prominent role in sustaining Tfh cell identity and function (6, 7, 18, 36, 109). IL-21- and IL-21R-deficient mice display reduced numbers of Tfh cells after antigen immunization suggesting an autocrine role for IL-21 in the maintenance and augmentation of Tfh cell programming (6, 110). However, in mice deficient either in IL-6 or IL-21 signaling, Tfh cell development is only partially compromised, indicating that these cytokines may play redundant roles in Tfh cell development (5, 103). In fact, loss of both cytokines significantly diminished Tfh cell numbers compared with an IL-6 or IL-21 deficiency alone (5, 103). However, an IL-6/IL-21 deficiency does not cause the complete absence of Tfh cells, suggesting an existence of IL-6 and IL-21-independent mechanisms for Tfh cell generation. In fact, it has been reported that the cytokine IL-27 contributes to Tfh cell maintenance by promoting IL-21 expression (104). Mice deficient in IL-27 signaling show reduced IL-21 expression, Tfh cell number, and GC activity (104). Similar to mice, DC-derived IL-27 is critical for the induction of Tfh cell polarization, IL-21 secretion by Tfh cells, and Tfh-dependent production of IgG by B cells (111). In addition to IL-21 induction, it has been suggested that IL-27 may play an important role in Tfh cell development by antagonizing IL-2 signaling, which negatively regulates Tfh cell development (10, 112).
TGF-β, IL-12, IL-23, and Activin A
Recent data suggest that different groups of cytokines support Tfh cell formation in humans, with prominent roles for TGF-β, IL-12, and IL-23. While IL-12 and IL-23 are capable of inducing IL-21 expression in naïve CD4+ T cells from human tonsils and peripheral blood, only IL-12 could augment expression of CXR5, ICOS, CD40L, and Bcl6, thus IL-12 is likely to act at an early stage of human Tfh cell development. B cells co-cultured with IL-12-primed CD4+ T cells produce antibodies which in part is dependent on the expression of CD40L (113). In support of the importance of IL-12 and IL-23 for Tfh development, individuals with mutations disrupting the function of IL-12Rβ1 (receptor for IL-12 and IL-23) have fewer circulating and GC Tfh cells and memory B cells. In mice, acting through STAT4, IL-12 induced a transitional stage in Tfh–Th1 cells, which express IL-21 and Bcl6. However, the IL-12–STAT4 pathway also promotes Tbet expression, which ultimately represses Bcl6 expression and Tfh cell programming. Since IL-12 is also linked to the generation of human Th1 cells, additional factors may also contribute to human Tfh cell generation. Surprisingly, TGF-β acts as an important cofactor for the early differentiation of human Tfh cells, but not in mice (7, 8, 114). TGF-β synergizes with IL-12 and IL-23, activating STAT4 and STAT3, and promoting Tfh cell development through induction of Tfh key molecules including CXCR5, ICOS, IL-21, Bcl6, Batf, c-Maf, and the downregulation of Blimp-1 expression (8). Recently, Activin A was identified as a novel inducer of Tfh cell programming in human and non-human primate cells, but not in mice by upregulating CXCR5, CXCL13, and PD-1 expression and repressing CCR7 and Blimp-1 expression (9). Activin A, in combination with IL-12, promotes the generation of Tfh like cells that have high expression levels of CXCR5, Bcl6, PD-1, LAMF1, CXCL13, IL-21, LIF, LTA, and TNF (9). Activin-A-induced Tfh programming is dependent on signaling via SMAD2 and SMAD3 (9).
Cytokines Which Inhibit Tfh Cell Formation
IL-2, IL-10, and IL-7
The IL-2/STAT5 axis is shown to be inhibitory for Tfh cell formation. In an in vivo mouse model of influenza infection, it was shown that exogenous administration of IL-2 suppresses Tfh cell differentiation, GC formation, and neutralizes antibody production (10). T cell-intrinsic expansion of Tfh cells is mediated by loss of IL-2Rα (115, 116). Mechanistically, activation of STAT5 by IL-2 enhances Blimp-1 expression and prevents binding of STAT3 to the Bcl6 locus (10). In addition, IL-2 inhibits the expression of IL-6 receptor α-chain (IL-6rα) and to a lesser extent, gp130 and thus negatively regulates Tfh differentiation (117). Moreover, a recent study depicts that IL-2 drives cells toward Th1 rather than Tfh differentiation through the activation of Akt and mTORC1 kinase (118). IL-7 negatively regulates Tfh differentiation by activating STAT5 and thereby repressing Bcl6 and CXCR5 (11). An additional study showed that IL-10, which has traditionally been viewed as a costimulator of antibody production, could also inhibit antibody responses indirectly by suppressing a subset of Tfh cells that produce IL-17 and IL-21 (119).
Transcriptional Regulation of Tfh Cells
The differentiation of naïve CD4+ T cells into Tfh cells is regulated by coordinated interplay between cell-extrinsic factors and cell-intrinsic transcriptional networks. Since the discovery of Bcl6 as a key factor for Tfh differentiation, several other transcriptional factors that either support [IFN-regulatory factor 4 (IRF4), c-Maf, Batf, STATs, Ascl2, TCF1, LEF1, etc.] or oppose [FOXO1, FOXP1, BLIMP-1, STAT5, KLF2, and peroxisome proliferator-activated receptor gamma (PPARγ)] Tfh cell development, migration, and function have been defined (1–3). A dynamic balance between these multiple transcriptional factors is the main determinant of normal Tfh development and immunity, since dysfunction of negative transcriptional machinery triggers autoimmunity (120, 121).
TFs Positively Influencing Tfh Cell Differentiation
B-Cell Lymphoma 6 Protein
The Bcl6 has been recognized as a key transcriptional factor for Tfh cell development and for efficient GC responses (7, 26, 27). Expression of Bcl6 is driven by CD28 and IL-6/IL-21–STAT1/STAT3 signaling (47). Bcl6 overexpression leads to the upregulation of PD-1, CXCR5, CXCR4, and SAP which are essential for Tfh cell function in T and B cell interactions (106, 115). As a transcriptional repressor, Bcl6 functions to suppress genes that controls Tfh cell development by the following mechanisms: (i) Blimp-1 expression; (ii) genes encoding proteins that controls migration including EBI2, CCR7, CCR6, S1PR1, and PSGL1; (iii) Klf2 expression; (iv) genes that support Th1 (IFNGR1, T-bet, and STAT4), Th2 (Gata3), and Th17 (IL-17 and RORγt) cell development (7, 26, 27, 122–126).
c-Maf
c-Maf is a bZIP transcriptional factor that plays an important role in the regulation of cytokine production and Th2, Th17, and Tfh cell differentiation (87). Both Th17 and Tfh cells have higher expression of c-Maf, and loss of c-Maf in T cells results in a defect in IL-21 production and fewer Th17 and Tfh cells (36). Recent data further indicate an important and non-redundant role for c-Maf in the initiation of Tfh cell development and T-cell-mediated humoral responses. Loss of c-Maf expression in T cells leads to the decreased expression of key Tfh molecules, such as BCL6, CXCR5, and PD1 (127).
Batf
Batf is basic leucine zipper protein in the AP-1 family which was originally implicated in Th17 differentiation through direct regulation of transcription of RORγ, IL-21, and IL-22 (128). While Batf expression is moderately increased in Tfh cells compared with other T helper subsets, Batf is an essential component for Tfh development through combined regulation of Bcl6 and c-Maf (129, 130). Batf-deficient mice fail to generate Tfh cells, and Bcl6 and c-Maf overexpression in Batf-deficient T cells improves Tfh cell development but not to the level of Batf reconstitution, suggesting that additional targets are required for complete Tfh cell induction (130). In addition, the Batf/IRF4 complex in cooperation with STAT3 and STAT6 is required for IL-4 expression in Tfh cells (120).
IFN-Regulatory Factor 4
Together with the well-known function of IRF4 in Th2, Th17, Th9, and Treg differentiation as well in plasma cell maturation (131–139), IRF4 also plays a critical role in Tfh cell development and GC formation (140). Mechanistically, IRF4 may cooperate with other transcriptional factors that determine T cell fate decision: (i) IRF4 interacts with Jun and Batf to form an IRF4–Jun–Batf complex that binds to AP-1–IRF4 composite elements (141); (ii) binds with STATs (142), and Bcl6 (143). Recently, it has been acknowledged that TCR signaling strength controls IRF-4 concentration and consequently cell fate choice between Bcl6-expressing Tfh and Blimp-1 expressing Teff cells (144). Increased TCR signaling leads to elevated IRF4 levels that function to coordinate Teff cell fate choice at the expense of Tfh cell fate (144).
Signal Transducers and Activators of Transcriptions
There are several members of the STAT family including STAT1, STAT3, and STAT4 that contribute to Tfh cell development (1, 6, 14, 106). It has been reported that STAT1 is required for early Tfh differentiation (107). Besides, IL-6 signaling during Tfh differentiation is mediated by both STAT1 and STAT3 TFs (107). It has also been noted that STAT1 directly regulates expression of key Tfh genes including Bcl6, CXCR5, and PD-1 by binding to their promoter loci (145). IL-6–STAT3 and IL-21–STAT3 signaling can promote Tfh cell differentiation by inducing Bcl6 expression (6, 7, 146, 147). STAT3 cooperates with the Ikaros zinc finger TFs, Aiolos, and Ikaros, to regulate Bcl6 expression (148). IL-6-mediated STAT3 activation restricts IL-2Rα expression to limit Th1 cell differentiation (107). In humans, functional STAT3 deficiency compromises Tfh cell generation (149). STAT4 can promote the expression of Bcl6 and the classical Tfh cell cytokine IL-21 in both mouse and human Tfh cells in vitro (35, 150). However, continued IL-12-driven Stat4 signaling can decrease the expression of Bcl6 and IL-21 and strengthen T-bet-derived Th1 differentiation at the expense of Tfh cells (35). Interestingly after acute viral infection, T-bet is co-expressed with Bcl6 in Tfh cells and is required alongside STAT4 to coordinate IL-21 and IFN-γ production in Tfh cells and for promotion of the GC response (151).
Notch1 and Notch2
Notch proteins belong to the family of evolutionary conserved transmembrane-bound receptors and play an important role in CD4+ T helper cell differentiation and/or function including Tfh cells (152). Mice with T-cell-specific deletions of Notch1 and Notch2 display impaired Tfh cell differentiation, IL-4 secretion by Tfh cells and GC reactions after immunization with T-dependent antigens or infection with parasites (153). Notch1- and Notch2-deficient Tfh cells express reduced levels of Tfh-associated molecules (CXCR5, PD-1, BTLA, and Bcl6), but normal levels of ICOS and increased Blimp-1 expression (153). Notch receptors 1 and 2 are required for Tfh cell generation and IL-4 expression by Tfh cells, but are dispensable for Th2 cell differentiation in response to parasitic helminth infection. Thus, Notch signaling is an important checkpoint in the bifurcation between Tfh and Th2 cell-driven hallmarks of type-2 immunity (154).
NFAT
Nuclear factor of activated T cells 2 (NFAT2) is highly expressed in Tfh cells, NFAT2 deficiency in T cells leads to enhanced GC reactions due to the impairment of Tfr cells to upregulate CXCR5 but not Tfh cells (155). A loss of both NFAT1 and NFAT2 in CD4+ T cells leads to impaired GC reactions due to reduced Tfh cell differentiation and decreased expression of proteins such as ICOS, PD-1, and SFRs which are important players in T/B interactions and B cell help (156).
Achaete-Scute Homolog 2
Achaete-scute homolog 2, a bHLH-domain-containing TF, is selectively upregulated in Tfh cells and initiates Tfh cell development (157). Overexpression of Ascl2 can lead to a substantial induction of CXCR5 expression, but not Bcl6 and downregulation CCR7 expression in vitro, as well as accelerated T cell migration to the follicles and Tfh cell development in vivo in mice (157). Ascl2 inhibits expression of Th1 and Th17 signature genes (157). Ascl2 deletion as well as inhibition of its function with E-protein inhibitor Id3 leads to a total impairment of Tfh cell development and GC response (157).
T Cell Factor 1 (TCF-1) and LEF-1
T cell factor 1 is highly expressed in Tfh cells (158–160). TCF-1 plays an important role in the initiation of Tfh cell differentiation and the effector function of differentiated Tfh cells, as TCF-1 deficiency results in reduced generation of Tfh cells and impairs their function to provide B cell help (159, 160). Similar to TCF-1, LEF-1 is also known for its essential role in early Tfh cell development (161, 162). LEF-1 and TCF-1 are upstream of Bcl6 induction and directly target Tfh signaling molecules (Bcl6, IL-6R, gp130, and ICOS) to promote Tfh cell differentiation (158). TCF-1 also suppresses Blimp-1 and IL-2Ra expression (159).
Early Growth Response Gene 2 (EGR2) and EGR3
Early growth response gene 2 and EGR3 can directly regulate the expression of Bcl6 and differentiation of Tfh cells (163).
Bob1
It has been reported that B-cell-specific octamer-binding protein 1, Bob1 in cooperation with TFs Oct1/Oct2 can directly bind to Bcl6 and BTLA promoters and promote their expression and Tfh cell development (164). However, at the same time, other groups have reported that the function of Bob1 is to mainly restrict the cellular frequency of Tfh cells (165, 166).
NF-kB1
It has been reported that NF-kB1 promotes Tfh cell responses by facilitating CXCR5 expression but no other Tfh-related molecules (Bcl6, IL-21, and PD-1), and the NF-kB1-deficient T cells partially lose their ability to provide help to B cells in vivo (167). In addition, the non-canonical NF-kB pathway may also play an essential role in Tfh development through regulation of ICOSL expression in B cells (168).
TFs Negatively Influencing Tfh Cell Differentiation
FOXO1 and FOXP1
Early studies suggest that a decreased expression of FOXO1 either because of increased expression of ICOS (15) or because of ITCH-mediated degradation (169) may increase Tfh cell differentiation. Analysis of mice with a specific deletion of Foxo1 in T cells revealed the requirement for Foxo1 in the suppression of Bcl6 expression and Tfh cell differentiation (15). In addition, enforced nuclear localization of Foxo1 prevents Tfh cell differentiation (15). However, Foxo1 is required during final differentiation to GC Tfh cells as Foxo1 deficient GC Tfh cells are substantially reduced (15). Foxp1 is an additional negative regulator of Tfh cell development. It mainly plays a role in dampening ICOS and IL-21 expression by regulating CCR7 and CTLA4 expression (170).
Krüppel-Like Factor 2
The TF, Klf2, regulates naïve T cell trafficking to secondary lymphoid tissues by promoting the expression of CD62L and S1PR1 (16). KLF2 expression impairs Tfh cell differentiation, whereas ablation of KLF2 expression enhances Tfh cell differentiation (171). This effect is related to the capacity of KLF2 to promote the expression of genes that oppose Tfh cell differentiation (Blimp-1, Gata3, and T-bet) and to repress the transcription of CXCR5 (171).
Blimp1 and STAT5
Blimp1, which is encoded by Prdm1, is a transcriptional repressor that has the ability to inhibit Bcl6 expression in B and T cells (122). Blimp-1, induced by IL-2/IL-7 and STAT5 signaling, suppresses expression of Bcl6 and other Tfh-associated genes including CXCR5, c-Maf, Bcl6, Batf, and IL-21, thus preventing Tfh cell differentiation (14, 172).
Peroxisome Proliferator-Activated Receptor Gamma
Peroxisome proliferator-activated receptor gamma is a TF that regulates lipid and glucose metabolism (173). One-year-old T-cell-specific PPARγ-deficient mice exhibited a moderate autoimmune phenotype with increased Tfh cells, GC B cells, glomerular inflammation, and enhanced autoantibody production. Mechanistically, PPARγ by stabilizing the activity of Ikbα, Foxo1, and Sirt1 negatively regulates Bcl6 and IL-21 to inhibit Tfh cell differentiation and GC formation (173).
Other Follicular T Cells
Several types of follicular T cells that have been found in GCs and characterized such as Tfr cells, follicular regulatory CD8+ T cells (CD8 Tfr), natural killer T follicular helper (NKTfh) cells, and follicular CD8+ T cells (fCD8).
Tfr Cells
T follicular regulatory cells, a newly identified subset of Tregs, have been found in GCs where they control GC responses (75–77, 86, 174–176). Tfr cells originate from thymic-derived Foxp3+ T cells as well as from Foxp3− precursors rather than from Tfh cells (78, 177). They are different from Tfh cells and Tregs: on the one hand, Tfr cells express large amounts of Tfh-related factors including CXCR5, PD-1, Bcl6, CXCL13, and ICOS; on the other hand, they share numerous molecules that are expressed by Tregs, such as GITR, CTLA4, IL10, CD25, and Foxp3 (75, 76, 178).
T follicular regulatory cells similarly possess a multistage and multifactorial differentiation process (176) that requires CD28 and ICOS signaling (76, 77), Sap-dependent interaction with B cells (76), and expression of Bcl6 (178). TF NFAT2 contributes to the initial upregulation of CXCR5 in Tfr cells (155). In addition, recently it has been noted that the mTORC1–STAT3–TCF-1–Bcl6 axis and TRAF3 are essential for Tfr differentiation (179, 180). However, there are also some signals which inhibit Tfr-cell differentiation and function: PD-1, CTLA4, Blimp1, and helix-loop-helix proteins ID2 and ID3 (77, 78). In addition, the cytokine IL-21 can suppress Tfr cells through the upregulation of Bcl6 expression and downregulation of CD25 (103, 181, 182).
T follicular regulatory cells act to limit excessive GC responses by acting on both Tfh and GC B cells (13, 77, 86, 183). Moreover, Treg suppression is not limited to GC B cells and occurs at various steps during B cell differentiation, from B cell activation to class-switched B cells and plasma cells (78). The effect of Tfr cells to modulate GC reaction could be through several potential mechanisms. Tfr cells could suppress Tfh cells through CTLA-4, by dampening expression of CD28 ligands on GC B cells (13). Two immunosuppressive cytokines, IL-10 and TGF-β, may mediate the immune suppressive functions of Tfr within GCs (114, 119, 176, 184); however, a recent research showed that IL-10 is important for B cell survival and proliferation; thus, a detailed mechanism remains to be investigated (185). Interestingly, Tfr cells potentially have effects on antibody affinity, and their suppressive function could result in the selection of the highest affinity antigen-specific antibody and in the selection of higher affinity memory B cells (78).
Follicular Regulatory CD8 T Cells (CD8 Tfr)
In contrast to Foxp3+CD4+ Tregs, mouse CD8 Tregs do not constitutively express Foxp3 in the thymus and periphery (186), and the CD8+Foxp3+ T cells do not comprise CD8 Treg population due to lack of suppressive activity (187). Similarly, most of the human CD8 Tregs also lack Foxp3 (188). In mice, a specific subset of Qa-1-restricted CD8 Tregs with high expression levels of CXCR5 (named as CD8 Tfr) were found to possess the ability to limit GC size and prevent autoimmune disease in mice (189). Tfh cells are one of main targets of CD8 Tfr cells (189). In autoimmune-prone mice, CD8 Tfr cells can suppress the expansion of Tfh cells as well as autoantibody production (190). Recent data indicate on the importance of the TF STAT4 for CD8+ Tfr development, maintenance, and function toward Tfh and plasma B cells (191). Moreover, CD8 Tfr cells expressing IL-2Rβ are also shown to inhibit CD8 T cell function in an IL-10-dependent manner (192). Recently, cells with a CD8 Tfr phenotype (CD3+CD8+CXCR5hiCD44hi) have been identified in humans (193). In chronic CIV infection, CD8 Tfr cells localized in the follicles exhibit enhanced Tim-3 and IL-10 expression, but express less perforin compared with CD8 T cells. CD8 Tfr cells modestly limit HIV replication in Tfh cells by impairing IL-21 production via Tim-3 and inhibit B cell function (194). In addition, it has been reported that the KIR+CD8+ cells (KIR, killer cell immunoglobulin-like receptor, functional homolog of murine Ly49) exert inhibitory activity on CD4+CXCR5+ Tfh target cells in humans (193).
NKTfh Cells
Recently, a subset of invariant NKT cells, recognized as follicular helper NKT cells (NKTfh cells), was discovered (195). NKTfh cells express CXCR5, PD-1, and Bcl-6 and support B cell responses (196). Similar to the development of conventional Tfh cells, the formation of NKTfh cells is dependent on CD28-mediated cognate interactions with B cells and Bcl6 expression (196). Studies utilizing CD4+ T cell-specific loss of Bcl6 determined that both Tfh and iNKTfh cells contribute to B cell help (197). However, unlike Tfh-derived B cell responses, those driven by NKTfh cells have no potential to generate long-lived plasma cells and memory B cells (196). NKTfh cells also possess the ability to boost memory B cell responses to T-dependent antigens but not T-independent lipid antigens (198). In addition, the NKTfh cells can induce limited GC B cell responses in the absence of CD4+ cell help (199). Further studies are still needed for a deeper understanding of the mechanisms of NKTfh cells in immunity and their roles in disease.
Follicular CD8+ T Cells (fCD8)
In contrast to the previous opinion that CD8+ T cells are restricted to extrafollicular areas (200), recent studies have showed that the CD8+ T cells from human and non-human primates possess the ability to migrate to the lymphoid follicles and GCs to support B cells (201). CD8+ T cells localize in human tonsil follicles and possess follicular helper-like characteristics including high expression of Bcl6, CXCR5, ICOS, PD-1, CCR5, CD27, CD28, CD69, and CD95, but do not express CCR7, Blimp-1, Tim-3, and CD244 and are named as follicular CD8+ T cells (fCD8) (201–206). The current evidence suggests a crucial role of fCD8 in controlling intracellular pathogens and malignancies through the production of various cytokines (IFN-γ, TNF-α, and MIP1β) in LCMV-infected mice, HIV-infected individuals, and cancer patients (203–205, 207–211). These cytokines activate APCs, promote polarization of naïve CD4+ T cells to Th1 cells, sustain activation of CD8+ T cells; thus, contributing in the control of viral infection and tumor growth. In addition, similar to Tfh cells, IL-21-producing fCD8 cells promote Ag-specific antibody responses by stimulating B cells, as well as generating and maintaining follicles and GCs (201).
The Role of Tfh Cells in Diseases
The main function of Tfh cells is to control clonal selection of GC B cells and support B cell immunoglobulin synthesis, isotype switching, and somatic hypermutations. Pathological B cell activation and the production of autoantibodies is a hallmark of the defective immune response that accompanies autoimmunity. In the sections below, we discuss the role of Tfh cells in various disease settings (Figure 2).
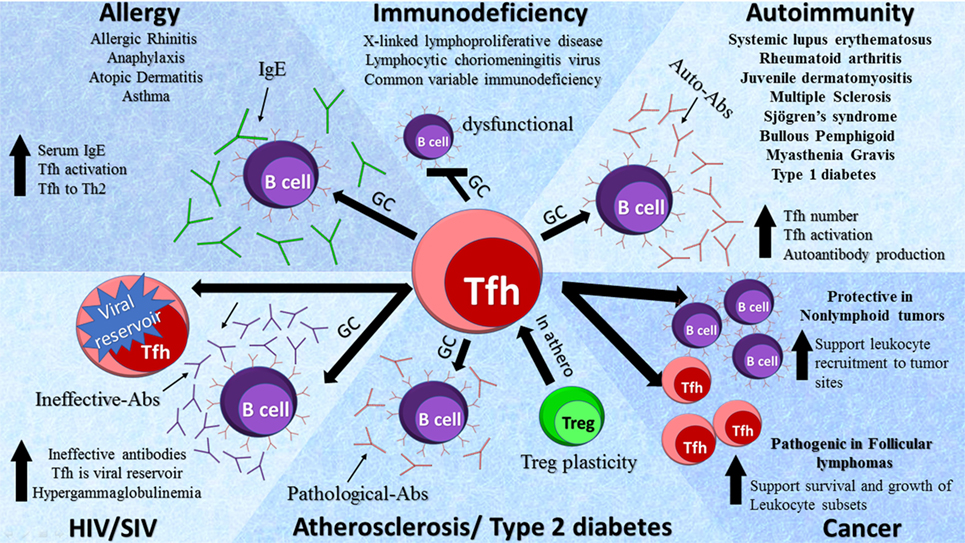
Figure 2. Role of T follicular helper (Tfh) cells in diseases. Tfh cells are involved in a variety of pathologies by diverse mechanisms as depicted in the schematic diagram. Elevated Tfh activity leads to an increase in germinal center (GC) formation, activity, and subsequent production of auto-Abs in autoimmune diseases. In primary immunodeficiencies, a decrease in Tfh cells has been detected with dysfunctional GC formation, whereas in acquired immunodeficiencies such as HIV and SIV an increase in Tfh cells serves as viral reservoirs. In atherosclerosis regulatory T cells (Tregs) transition to Tfh cells via GCs leading to the production of pathological Abs. In allergy, there is an increase in Tfh cell activation and GC formation causing the production of IgE. The role of Tfh cells in cancers is complex and dependent on the types of tumors.
Systemic Lupus Erythematosus (SLE)
Observations in animal models and in humans provide strong evidence that Tfh cells are important players in SLE pathology. Patients with SLE have an increased number of cTfh cells, which positively correlates with autoantibody titers (212). Furthermore, the proportion of peripheral blood T cells expressing ICOS is higher in patients with active SLE disease than in patients with inactive disease or in controls which probably leads to enhanced autoantibody production upon activation via ICOS–ICOSL interactions in these patients (45, 213). Production of high levels of IL-21 is a hallmark of Tfh cells and multiple studies have shown an increased frequency of CD4+IL-21+ T cells in SLE patients that was associated with disease severity (214). An increase in Tfh cells is also associated with a shift in the Th17/Treg populations with increases in Th17 cells and decreases in Tregs as well as an elevation of IgG+ class-switched memory B cells leading to a more inflammatory environment as observed in SLE (214–219). It is worthy to note that Tfh cells not only affect priming in secondary lymphoid tissues but can also impact local B cell activation and expansion. For example, within the tubulointerstitium of patients with lupus nephritis, Tfh-like cells are organized with B cells in structures resembling GCs (220, 221) suggesting that Tfh cells may also play a role in various complications that are associated with autoimmune pathologies.
Mice that have a single-amino-acid mutation in Roquin1 (a negative regulator of ICOS mRNA stability) demonstrate a spontaneous lupus-like phenotype that is accompanied by elevated numbers of Tfh cells expressing higher level of ICOS, IFN-γ OX40, and IL-21 and activated phenotypes of GCs (28, 222). Importantly, manipulation of Bcl6 expression and thus Tfh cell generation or adoptive transfer of lupus-associated Tfh cells from Roquinsan/san mice into healthy recipients induces the formation of GCs, highlighting a contributing role of Tfh cells in SLE (223). Another interesting model to study lupus-related pathophysiology is the BXSB mouse model, which displays lymphoid hyperplasia, monocytosis, immune complex-mediated glomerulonephritis, and an aberrant IL-21-dependent Tfh response (224). The MRL/lpr mouse model displays defective Fas signaling (225), which is characterized by high levels of autoantibodies and extrafollicular Tfh like cells that are dependent on ICOS, Bcl-6, and IL-21 signaling. The pathological functions of these T cells support extrafollicular B cell differentiation and plasmablast maturation (226). While increasing evidence suggests the importance of an extrafollicular Tfh-dependent response in the murine models of SLE (227–230), the importance of Tfh cells and extrafollicular sites in SLE patients is not well defined due to limitations in obtaining non-circulated follicular-resident Tfh cells.
There have been several relatively successful attempts to reduce the severity of SLE in humans via blockade of Tfh-cell differentiation and activity. Early results from SLE therapies targeting T cells showed that patients had reduced serum anti-dsDNA titers, but presented minimal reduction of protective antibodies, increased complement markers, and reduced nephritis scores (231). Studies using monoclonal antibodies against ICOS-L inhibited the development of Tfh and GC B cells resulting in decreases in anti-dsDNA Igs and improved kidney function. For years, the main therapy for SLE has been broad spectrum immunosuppressant’s; however, a growing body of work shows that Tfh cells may be a more precise and attractive target for treating SLE.
Sjögren’s Syndrome
Increased numbers of human peripheral blood CXCR5+ICOS+, CXCR5+PD-1+ Tfh cells and enhanced GC formation positively correlate with autoantibodies titers and severity of the primary Sjögren’s syndrome (pSS) (227–230). IL-6 levels are increased in the serum, tears, and salivary gland epithelial cells of patients with pSS. Interestingly, co-cultures of salivary gland epithelial cells with T cells induce Tfh cell differentiation (3, 232) suggesting a critical role for epithelial cell-derived IL-6 in Tfh cell differentiation in pSS. There are several treatment options for patients with pSS that target Tfh cell functions and therefore the humoral response. Abatacept is a fusion molecule combining CTLA-4 with IgG Fc that binds to CD80/86 and consequently impairs CD28-mediated T cell costimulation (233). It has been shown that abatacept inhibits T cell-dependent B cell activation either via DC-defective Th activation or via blockade of T/B cell interactions (234). Abatacept treatment in pSS patients reduces circulating numbers of ICOS+ cTfh cells resulting in an attenuated Tfh cell-dependent B cell hyperactivity (235) providing a promising therapy for pSS and some other autoimmune pathologies.
Juvenile Dermatomyositis (JDM) and Autoimmune Myasthenia Gravis (MG)
Serum autoantibodies can be found in up to 70% of patients with JDM. Furthermore, these patients display changes in cTfh cells subsets with a decrease in cTfh1 cells, and an increase in the cTfh2 and activated memory cTfh17 cell subpopulations; resulting in an overall increase in cTfh subsets with efficient helper functions. Significantly, these changes in the composition of cTfh cell subsets positively correlate with disease activity and the frequency of circulating plasmablasts (38).
Elevated levels of circulating CXCR5+CD57+, CXCR5+ICOShi, and CXCR5+PD1hi CD4+ T cells have also been reported in patients with MG (236). Functional studies demonstrate that cTfh cells from MG patients support autoantibody production, and thus, contribute to the development of disease. Interestingly, Tfh1 and Tfh17, but not Tfh2 cells, were found to be the major secretors of IL-21 (237). Thus, alterations in the composition of peripheral blood memory Tfh1, Tfh17, and Tfh2 subsets seems to be one of the distinct features of several autoimmune diseases including SLE (238), Sjogren’s syndrome, multiple sclerosis (239), and MG (237). While an increasing body of evidence reports a shift in the Tfh1/Tfh2/Tfh17 balance in the peripheral blood of patients with autoantibody-mediated pathologies, it is not clear whether the same shift in the Tfh subsets occurs within the inflamed tissues and GCs in the secondary lymphoid tissues. It would be important to identify molecular mechanisms and microenvironmental stimuli that direct a preferential repopulation of Tfh17 and Tfh2 cells in autoimmune settings.
Rheumatoid Arthritis
One of the other examples for the implication of Tfh cells in autoimmune disease setting is RA, which is characterized by high levels of autoantibodies and abnormal GC B cell responses, which contribute to inflammation in the joints. Tfh cells have been detected in the synovial tissue of patients with RA, and along with IL-21 are found in higher frequencies in the periphery (240–242). The increase in Tfh cells in RA patients is positively correlated with elevated serum level of anti-CCP antibodies and consequently with disease severity (240). In animal models, a T cell-specific CXCR5 deficiency results in a significant reduction in GC formation, decreased levels of collagen-specific IgG1 antibodies and a resistance to CIA induction (243). As Tfh cells play a significant role in the progression of RA, therapeutic targeting of Tfh cells could be a valuable option for treating patients with RA.
Type 1 Diabetes (T1D)
Type 1 diabetes is caused by the autoimmune destruction of insulin-producing β-cells in the pancreas. T1D patients have higher frequencies of circulating CD4+CXCR5+ICOS+Tfh cells and higher levels of IL-21 which positively correlates with an increase in plasmablasts, serum autoantibodies, and C-peptide levels (244–246). It is possible that Tfh cells may play a critical role in the initial stages of T1D with an increase of activated CXCR5+PD-1+ICOS+ cTfh cells being found in both children with newly diagnosed T1D and in children at late stages of preclinical T1D, characterized by impaired glucose tolerance (247, 248). Data from animal models also show that transfer of Tfh cells from a diabetic to a control animal induces elevated blood glucose levels and increased T cell infiltration into islets (244). Furthermore, Roquinsan/san mice that have overreactive Tfh cells display dramatically accelerated T1D induction. Collectively, there is direct evidence for a pathogenic role for Tfh cells in progression to T1D. Importantly, the increase in activated cTfh cells is strongly associated with positivity for multiple autoantibodies and could be used as a biomarker for the identification of a subgroup of patients with an active setting of T1D.
Type 2 Diabetes (T2D)
Evidence indicates a key contribution of the immune response in the manifestation of chronic low grade inflammation under the conditions of adipose tissue inflammation, islet β-cell dysfunction, and T2D. While the role of macrophages, CD4+, CD8+ cells, and follicular B cells is firmly established, there is limited knowledge about the Tfh cell role in obesity and T2D. Recently, Zhou and colleagues found that non-obese T2D patients vs BMI-matched healthy subjects have higher IgG levels and Tfh cells that are highly enriched in IFN-γ, but not IL-4 and IL-17 (249). Interestingly, overweight T2D patients (BMI ≥ 24.0) had higher levels of cTfh and the balance of cTfh cell subsets was shifter toward the Th17 subtype (52). Importantly, patients with abdominal obesity had additional increases in cTfh compared with patients without abdominal obesity (52), suggesting that cTfh may play a critical role in the modulation of adipose tissue inflammation in obesity-induced T2D.
Atherosclerosis
Recent reports revealed a critical role for Tfh cells and consequently GC B cells in the development and progression of human and mouse atherosclerosis. Tfh cells support atherogenesis via the production of pathological Abs and the generation of highly active GCs. The loss of Tfh cells via a conditional knockout of Bcl6 leads to a reduced atherosclerotic burden in atherosclerosis-prone mice (250, 251). Interestingly, a percentage of Tregs can switch the phenotype into pro-atherogenic Tfh cells but ApoAI can prevent Treg to Tfh cell conversion throughout atherosclerosis (250). It remains to be determined how atherosclerosis-prone conditions alter the memory pool of Tfh cells and how Tfh cells impact the processes of selection of high-affinity B cells and B cell memory development in atherosclerosis.
Allergy
Patients with allergic rhinitis (AR) and asthma preferentially have increased levels of cTfh cells that exhibit a Tfh2 cell phenotype (252). In patients with seasonal AR to ragweed pollen, activation of Tfh cells increases significantly during peak-season (253). It has been noted that the acute anaphylaxis response to peanut allergen is driven by IL-4+IL21+ Tfh cells and to a lesser extent by Th2 cells (254). Presence of Tfh cells but not Th2 cells is required for IgE production and for the development of an allergic response.
The increased levels of serum IgE is also a hallmark of atopic dermatitis (AD) (255–258). Peripheral Tfh cells in children with AD have significantly increased levels of ICOS, PD-1, and IL-21 suggesting a highly activated phenotype. Furthermore, a strong positive correlation has been detected between the numbers of IL-21+ Tfh-like cells, activated memory B cell pool, and disease severity (259, 260). Interestingly, patients with asthma also display elevated levels of PD-1+ICOS+ Tfh2 cells and the ratio of Tfh2:Tfh1 cells positively correlates with the total IgE levels in the blood (46). In correlation with the data on human samples, mRNA and protein expression levels of CXCR5, ICOS, ICOSL, and IL-21 were also elevated in mouse models of asthma. Our recent findings also identify an important role of the TF Batf toward the generation of IL-4-expressing Tfh cells rather that Th2 and to their pro-allergic function (120). We further demonstrated that the IL-4–STAT6 signaling contributes to the Batf induction in Tfh cells and the Batf/IRF4 complex along with Stat3 and Stat6 aids IL-4 production in Tfh cells. Recent studies have also shown that Tfh cells can sense specific microenvironmental conditions and differentiate into Th2 cells after repeat exposure with house dust mite (HDM) (261). At the initial stage of disease, Tfh cells are preferentially differentiated, but a second exposure leads to the Tfh switch into Th2 cells which migrate to the lung and produce an inflammatory response. These results suggest that targeting Tfh cells may be a good therapeutic strategy to prevent Th2-cell-mediated immunity to HDM (261).
Primary Immunodeficiency
There are several immunodeficiencies that directly affect the development and functions of Tfh cells and as a result alter B cell-dependent responses. XLP is a primary immunodeficiency caused by mutations in SH2D1A (encoding for SAP, signaling lymphocytic activation molecule-associated protein). A SAP deficiency does not impact CD4+ T cell development, but compromises Tfh cell differentiation (262). In line with this observation, XLP patients exhibited significant defects in GC formation, reductions in memory B cell responses, hypogammaglobulinemia, and impaired antigen-specific antibody responses (263). Mutations in CD40L, ICOS, and STAT3 also cause reduced number of CD4+CXCR5+ cells, defective GC formation, and impaired humoral immune responses. A recent study also highlighted the importance of the IL-12/IL-12R axis as patients with mutations in IL-12Rβ1 demonstrate fewer circulating memory Tfh, memory B cells, and defective GCs compared with control subject (264).
HIV and SIV Pathologies
Proper activation of Tfh cells and their interactions with GC B cells are essential for an effective humoral immune response and the extermination of pathogenic human and simian immunodeficiency viruses (HIV and SIV, respectively). Paradoxically, HIV-infected individuals (265) and monkeys infected with SIV (266) display a significantly higher frequency of ICOS+ Tfh, PD-1+ Tfh, and ICOS+PD-1+ Tfh cells among total CD4+ T cells compared with non-infected controls. Furthermore, Tfh cell frequency is significantly higher in non-treated HIV+ patients compared with HIV+ patients treated with combination anti-retroviral therapy, suggesting that an HIV viral persistence contributes to Tfh cell expansion (265–267). Interestingly, Tfh cells in humans and macaques show preferential infection with HIV and SIV, respectively (200, 267, 268), likely via the chemokine receptor CCR5 as CCR5 is expressed on a precursor subset of Tfh cells and may potentially serve as a co-receptor for HIV (269). GC Tfh cells have been implicated in HIV persistence by supporting viral replication during treated infection and serve as an important cellular reservoir of HIV-1 DNA (270, 271). One explanation is that cytotoxic CD8+ T cells are CXCR5 negative and thus unable to migrate to follicles and target HIV-infected GC Tfh cells (200, 272, 273). In line with an increased number of Tfh cells, hypergammaglobulinemia is detected in HIV+ patients (274), but these antibodies are ineffective (265, 273, 275–278). Studies have proposed that PD-1 triggering by PD-L1 on GC B cells is a mechanism for the abnormal Tfh functions and defective B cell help (277). In addition, due to the increased number of Tfh cells, the exacerbated interactions of Tfh and GC B cells may lower the threshold for B cell selection resulting in the selection of B cells with low affinities (223). Thus, the relationship between viral entrance into Tfh cells, number and functions of Tfh cells, and B cell activation/maturation is complex and requires further investigations.
Chronic Infections
Lymphocytic choriomeningitis virus persistence results in extended TCR engagement and an IL-6-driven shift from a Th1-induced response toward a Tfh response (279, 280). In addition to studies that identified a key role of Tfh cells in HIV and SIV infections, studies with the LCMV disease model further highlight the importance of timely expansion and sustained functions of Tfh cells in the orchestration of a proper humoral response for control of persistent viral infection (279, 280). Moreover, annual influenza virus studies determined an increase in the number and activation levels of cTfh cells. These studies not only show that an increased cTfh cell frequency contributes to circulating plasmablast responses and infection clearance but can also be used as a marker to monitor the efficacy of influenza vaccination (281, 282). In other infections such as chronic hepatitis B virus patients have an increase in circulating regulatory Tfh cells (283). Interestingly, long-term disruptions of proper T cell-dependent Ab production have been detected in the case of parasitic infections such as Leishmania (284), Litomosoides sigmodontis (285), and Plasmodium infection (286) suggesting the negative regulation of the number and functions of Tfh cells under these conditions. To date, the precise mechanisms that regulate differentiations and functions of Tfh and Tfr cells in different infection settings remain to be determined. Particularly, further studies are required to assess the mechanisms governing Tfh cell development, persistence, and function at the different stages of various infection diseases.
Cancer
Accumulating evidence suggests that Tfh cells are involved in peripheral T cell and B cell-associated tumors due to their high impact on growth and survival of different leukocyte subsets. Angioimmunoblastic T cell lymphoma (AITL) is an aggressive tumor and isolated neoplastic T cells express CXCL13, ICOS, CD154, CD40L, and NFATC1 (287, 288), making these T cells similar to Tfh cells (289). The expression of mutated RhoA G17V induces Tfh cell differentiation/activation; increased proliferation associated with ICOS upregulation, elevated PI3K, and mitogen-activated protein signaling (290). Loss-of-function mutations in epigenetic regulators such as TET2 and DNMT3A are frequent events in the pathogenesis of AITL. Interestingly, RhoA G17V expression accompanied with TET2 loss results in AITL development in mice. It is worth to note that altered RhoA GTPase activity has been linked with autoimmunity and studies in AITL further highlight a role of RhoA in shaping Tfh cell phenotype and response.
Like AITL, in follicular T cell lymphomas, infiltrating T cells resemble a phenotype of Tfh-like cells and express IL-4, TNF-α, IFN-γ, LT-α, CCL17, and CCL22 chemokines that play a role in the regulation of Treg and Th2 cell migration and modulate the activity of GC B cells within follicles as well. Not only Tfh-like cells but also Foxp3+ Tfr cells are found within neoplastic follicles and the number of Tfr cells is elevated during progression lymphomagenesis. Thus, there is a complex T cell response that is regulated by a delicate balance of CD4+, Tregs, and Tfh subsets. Probably one of the strongest predictors of survival would be the location pattern for T cell subsets, as accumulation within the follicles is linked with poor survival compared with a distribution pattern outside the follicle (291). To date, there is limited understanding in the functions of Tfh and Tfr subsets in lymphomagenesis and more detailed research in animal models and human samples will help to dissect the complex role of Tfh cells in cancer.
Unexpectedly, Tfh cells have protective roles in nonlymphoid tumors. Higher levels of Tfh cell infiltrates and their ability to organize tertiary lymphoid structures within tumors has been associated with increased survival and reduced immunosuppression which strongly correlate with an increased survival in breast cancer (292). Evidence suggest that IL-21 and CXCL13 may play a key role in the protective functions of Tfh cells via the modulation of local leukocyte recruitment. Infiltrating Tfh cells have also been reported in chronic lymphocytic leukemia, non-small cell lung cancer, osteosarcoma, and colorectal cancer (214, 293–297), where they positively correlated with patient survival (293). So far, very little is known about how Tfh cells impact the immune response involved in the suppression of tumor initiation and progression and further studies will be important for a better understanding of Tfh-related pathologies.
Conclusion
Since the identification of Tfh cells and the discovery of Bcl6 as a critical factor in their generation, there has been substantial progress made in understanding the molecular and cellular requirement for the development and function of mouse and human Tfh cells. To date, multiple cell-extrinsic and -intrinsic factors (costimulatory molecules, cytokines signaling, and transcriptional factors) have been determined to positively or negatively contribute to Tfh cell development. However, still many questions remain to be answered: (i) What is the exact composition and hierarchy of these factors and what are their stage-specific requirements? (ii) Is Bcl6 required complete Tfh cell commitment? (iii) How Tfh-specific transcriptional factors impact epigenetic mechanisms governing Tfh cell generation? (iv) Which factors contribute to Tfh cell maintenance and memory formation? (v) What are the appropriate Tfh-specific target(s) for therapy in Tfh mediated autoimmune disorders and cancer?
T follicular helper cells mainly localize in secondary lymphoid organs and circulate in the blood and are beginning to emerge as crucial players in maintaining a healthy balance between protective and pathogenic immunity (Figure 2). However, due to the localization of Tfh cells in secondary lymphoid tissues, the study of human Tfh cell heterogeneity and function in normal and disease settings has been difficult. Further analysis and comparison of human circulating counterparts to tissue-resident Tfh cells in various disease settings is critical, since it will help to reveal the level of Tfh cell heterogeneity at various stages of diseases as well as will determine whether the disease-associated alterations in Tfh cells are the main cause or result of disorders. Thus, in view of an emerging role of Tfh cells in various disease settings, we believe that current progress and further understanding of the heterogeneity and regulation of tissue-specific and cTfh cells in health and disease will lead to improved vaccine designs, better management of major autoimmune, inflammatory disorders, and cancer, and can be utilized as a novel prognostic biomarker for the identification of lymphoid and solid tumors.
Author Contributions
All authors listed contributed significantly toward the preparation of the manuscript. LQ, TW, SB, EG, and RN wrote the manuscript. AS helped in writing, extensive editing, and revising the manuscript. HZ provided critical comments on the manuscript.
Conflict of Interest Statement
The authors declare that the research was conducted in the absence of any commercial or financial relationships that could be construed as a potential conflict of interest.
Acknowledgments
This work is supported by NIH research grants (RN) (R03CA219760, R21AI120012, and R56AI125269), Institutional Research Grant (RN), and R01 HL139000 (EG and RN). RN and LQ are recipients of China Scholarship Council Fellowship (201706070066).
References
1. Vinuesa CG, Linterman MA, Yu D, MacLennan IC. Follicular helper T cells. Annu Rev Immunol (2016) 34:335–68. doi:10.1146/annurev-immunol-041015-055605
2. Wali S, Sahoo A, Puri S, Alekseev A, Nurieva R. Insights into the development and regulation of T follicular helper cells. Cytokine (2016) 87:9–19. doi:10.1016/j.cyto.2016.06.010
3. Crotty S. Follicular helper CD4 T cells (TFH). Annu Rev Immunol (2011) 29:621–63. doi:10.1146/annurev-immunol-031210-101400
4. Mesquita D Jr, Cruvinel WM, Resende LS, Mesquita FV, Silva NP, Camara NO, et al. Follicular helper T cell in immunity and autoimmunity. Braz J Med Biol Res (2016) 49:e5209. doi:10.1590/1414-431X20165209
5. Karnowski A, Chevrier S, Belz GT, Mount A, Emslie D, D’Costa K, et al. B and T cells collaborate in antiviral responses via IL-6, IL-21, and transcriptional activator and coactivator, Oct2 and OBF-1. J Exp Med (2012) 209:2049–64. doi:10.1084/jem.20111504
6. Nurieva RI, Chung Y, Hwang D, Yang XO, Kang HS, Ma L, et al. Generation of T follicular helper cells is mediated by interleukin-21 but independent of T helper 1, 2, or 17 cell lineages. Immunity (2008) 29:138–49. doi:10.1016/j.immuni.2008.05.009
7. Nurieva RI, Chung Y, Martinez GJ, Yang XO, Tanaka S, Matskevitch TD, et al. Bcl6 mediates the development of T follicular helper cells. Science (2009) 325:1001–5. doi:10.1126/science.1176676
8. Schmitt N, Liu Y, Bentebibel SE, Munagala I, Bourdery L, Venuprasad K, et al. The cytokine TGF-beta co-opts signaling via STAT3-STAT4 to promote the differentiation of human TFH cells. Nat Immunol (2014) 15:856–65. doi:10.1038/ni.2947
9. Locci M, Wu JE, Arumemi F, Mikulski Z, Dahlberg C, Miller AT, et al. Activin A programs the differentiation of human TFH cells. Nat Immunol (2016) 17:976–84. doi:10.1038/ni.3494
10. Ballesteros-Tato A, Leon B, Graf BA, Moquin A, Adams PS, Lund FE, et al. Interleukin-2 inhibits germinal center formation by limiting T follicular helper cell differentiation. Immunity (2012) 36:847–56. doi:10.1016/j.immuni.2012.02.012
11. McDonald PW, Read KA, Baker CE, Anderson AE, Powell MD, Ballesteros-Tato A, et al. IL-7 signalling represses Bcl-6 and the TFH gene program. Nat Commun (2016) 7:10285. doi:10.1038/ncomms10285
12. Lages CS, Lewkowich I, Sproles A, Wills-Karp M, Chougnet C. Partial restoration of T-cell function in aged mice by in vitro blockade of the PD-1/PD-L1 pathway. Aging Cell (2010) 9:785–98. doi:10.1111/j.1474-9726.2010.00611.x
13. Sage PT, Paterson AM, Lovitch SB, Sharpe AH. The coinhibitory receptor CTLA-4 controls B cell responses by modulating T follicular helper, T follicular regulatory, and T regulatory cells. Immunity (2014) 41:1026–39. doi:10.1016/j.immuni.2014.12.005
14. Nurieva RI, Podd A, Chen Y, Alekseev AM, Yu M, Qi X, et al. STAT5 protein negatively regulates T follicular helper (Tfh) cell generation and function. J Biol Chem (2012) 287:11234–9. doi:10.1074/jbc.M111.324046
15. Stone EL, Pepper M, Katayama CD, Kerdiles YM, Lai CY, Emslie E, et al. ICOS coreceptor signaling inactivates the transcription factor FOXO1 to promote Tfh cell differentiation. Immunity (2015) 42:239–51. doi:10.1016/j.immuni.2015.01.017
16. Weinreich MA, Takada K, Skon C, Reiner SL, Jameson SC, Hogquist KA. KLF2 transcription-factor deficiency in T cells results in unrestrained cytokine production and upregulation of bystander chemokine receptors. Immunity (2009) 31:122–30. doi:10.1016/j.immuni.2009.05.011
17. Kim CH, Rott LS, Clark-Lewis I, Campbell DJ, Wu L, Butcher EC. Subspecialization of CXCR5+ T cells: B helper activity is focused in a germinal center-localized subset of CXCR5+ T cells. J Exp Med (2001) 193:1373–81. doi:10.1084/jem.193.12.1373
18. Chtanova T, Tangye SG, Newton R, Frank N, Hodge MR, Rolph MS, et al. T follicular helper cells express a distinctive transcriptional profile, reflecting their role as non-Th1/Th2 effector cells that provide help for B cells. J Immunol (2004) 173:68–78. doi:10.4049/jimmunol.173.1.68
19. Breitfeld D, Ohl L, Kremmer E, Ellwart J, Sallusto F, Lipp M, et al. Follicular B helper T cells express CXC chemokine receptor 5, localize to B cell follicles, and support immunoglobulin production. J Exp Med (2000) 192:1545–52. doi:10.1084/jem.192.11.1545
20. Schaerli P, Willimann K, Lang AB, Lipp M, Loetscher P, Moser B. CXC chemokine receptor 5 expression defines follicular homing T cells with B cell helper function. J Exp Med (2000) 192:1553–62. doi:10.1084/jem.192.11.1553
21. Ansel KM, McHeyzer-Williams LJ, Ngo VN, McHeyzer-Williams MG, Cyster JG. In vivo-activated CD4 T cells upregulate CXC chemokine receptor 5 and reprogram their response to lymphoid chemokines. J Exp Med (1999) 190:1123–34. doi:10.1084/jem.190.8.1123
22. Forster R, Mattis AE, Kremmer E, Wolf E, Brem G, Lipp M. A putative chemokine receptor, BLR1, directs B cell migration to defined lymphoid organs and specific anatomic compartments of the spleen. Cell (1996) 87:1037–47. doi:10.1016/S0092-8674(00)81798-5
23. Ma CS, Deenick EK, Batten M, Tangye SG. The origins, function, and regulation of T follicular helper cells. J Exp Med (2012) 209:1241–53. doi:10.1084/jem.20120994
24. Gunn MD, Ngo VN, Ansel KM, Ekland EH, Cyster JG, Williams LT. A B-cell-homing chemokine made in lymphoid follicles activates Burkitt’s lymphoma receptor-1. Nature (1998) 391:799–803. doi:10.1038/35876
25. Kim CH, Lim HW, Kim JR, Rott L, Hillsamer P, Butcher EC. Unique gene expression program of human germinal center T helper cells. Blood (2004) 104:1952–60. doi:10.1182/blood-2004-03-1206
26. Johnston RJ, Poholek AC, DiToro D, Yusuf I, Eto D, Barnett B, et al. Bcl6 and Blimp-1 are reciprocal and antagonistic regulators of T follicular helper cell differentiation. Science (2009) 325:1006–10. doi:10.1126/science.1175870
27. Yu D, Rao S, Tsai LM, Lee SK, He Y, Sutcliffe EL, et al. The transcriptional repressor Bcl-6 directs T follicular helper cell lineage commitment. Immunity (2009) 31:457–68. doi:10.1016/j.immuni.2009.07.002
28. Ueno H, Banchereau J, Vinuesa CG. Pathophysiology of T follicular helper cells in humans and mice. Nat Immunol (2015) 16:142–52. doi:10.1038/ni.3054
29. Flynn S, Toellner KM, Raykundalia C, Goodall M, Lane P. CD4 T cell cytokine differentiation: the B cell activation molecule, OX40 ligand, instructs CD4 T cells to express interleukin 4 and upregulates expression of the chemokine receptor, Blr-1. J Exp Med (1998) 188:297–304. doi:10.1084/jem.188.2.297
30. Kerfoot SM, Yaari G, Patel JR, Johnson KL, Gonzalez DG, Kleinstein SH, et al. Germinal center B cell and T follicular helper cell development initiates in the interfollicular zone. Immunity (2011) 34:947–60. doi:10.1016/j.immuni.2011.03.024
31. Kitano M, Moriyama S, Ando Y, Hikida M, Mori Y, Kurosaki T, et al. Bcl6 protein expression shapes pre-germinal center B cell dynamics and follicular helper T cell heterogeneity. Immunity (2011) 34:961–72. doi:10.1016/j.immuni.2011.03.025
32. Haynes NM, Allen CD, Lesley R, Ansel KM, Killeen N, Cyster JG. Role of CXCR5 and CCR7 in follicular Th cell positioning and appearance of a programmed cell death gene-1high germinal center-associated subpopulation. J Immunol (2007) 179:5099–108. doi:10.4049/jimmunol.179.8.5099
33. Ramiscal RR, Vinuesa CG. T-cell subsets in the germinal center. Immunol Rev (2013) 252:146–55. doi:10.1111/imr.12031
34. Bentebibel SE, Schmitt N, Banchereau J, Ueno H. Human tonsil B-cell lymphoma 6 (BCL6)-expressing CD4+ T-cell subset specialized for B-cell help outside germinal centers. Proc Natl Acad Sci U S A (2011) 108:E488–97. doi:10.1073/pnas.1100898108
35. Nakayamada S, Kanno Y, Takahashi H, Jankovic D, Lu KT, Johnson TA, et al. Early Th1 cell differentiation is marked by a Tfh cell-like transition. Immunity (2011) 35:919–31. doi:10.1016/j.immuni.2011.11.012
36. Bauquet AT, Jin H, Paterson AM, Mitsdoerffer M, Ho IC, Sharpe AH, et al. The costimulatory molecule ICOS regulates the expression of c-Maf and IL-21 in the development of follicular T helper cells and TH-17 cells. Nat Immunol (2009) 10:167–75. doi:10.1038/ni.1690
37. He J, Tsai LM, Leong YA, Hu X, Ma CS, Chevalier N, et al. Circulating precursor CCR7(lo)PD-1(hi) CXCR5(+) CD4(+) T cells indicate Tfh cell activity and promote antibody responses upon antigen reexposure. Immunity (2013) 39:770–81. doi:10.1016/j.immuni.2013.09.007
38. Morita R, Schmitt N, Bentebibel SE, Ranganathan R, Bourdery L, Zurawski G, et al. Human blood CXCR5(+)CD4(+) T cells are counterparts of T follicular cells and contain specific subsets that differentially support antibody secretion. Immunity (2011) 34:108–21. doi:10.1016/j.immuni.2010.12.012
39. Schmitt N, Bentebibel SE, Ueno H. Phenotype and functions of memory Tfh cells in human blood. Trends Immunol (2014) 35:436–42. doi:10.1016/j.it.2014.06.002
40. Asrir A, Aloulou M, Gador M, Perals C, Fazilleau N. Interconnected subsets of memory follicular helper T cells have different effector functions. Nat Commun (2017) 8:847. doi:10.1038/s41467-017-00843-7
41. Scherm MG, Ott VB, Daniel C. Follicular helper T cells in autoimmunity. Curr Diab Rep (2016) 16:75. doi:10.1007/s11892-016-0770-2
42. Schmitt N, Ueno H. Regulation of human helper T cell subset differentiation by cytokines. Curr Opin Immunol (2015) 34:130–6. doi:10.1016/j.coi.2015.03.007
43. Weinstein JS, Hernandez SG, Craft J. T cells that promote B-cell maturation in systemic autoimmunity. Immunol Rev (2012) 247:160–71. doi:10.1111/j.1600-065X.2012.01122.x
44. Jogdand GM, Mohanty S, Devadas S. Regulators of Tfh cell differentiation. Front Immunol (2016) 7:520. doi:10.3389/fimmu.2016.00520
45. Yang JH, Zhang J, Cai Q, Zhao DB, Wang J, Guo PE, et al. Expression and function of inducible costimulator on peripheral blood T cells in patients with systemic lupus erythematosus. Rheumatology (Oxford) (2005) 44:1245–54. doi:10.1093/rheumatology/keh724
46. Gong F, Qian C, Zhu H, Zhu J, Pan Y, Dong Q, et al. Circulating follicular T-helper cell subset distribution in patients with asthma. Allergy Asthma Proc (2016) 37:154–61. doi:10.2500/aap.2016.37.3982
47. Weber JP, Fuhrmann F, Feist RK, Lahmann A, Al Baz MS, Gentz LJ, et al. ICOS maintains the T follicular helper cell phenotype by down-regulating Kruppel-like factor 2. J Exp Med (2015) 212:217–33. doi:10.1084/jem.20141432
48. Alegre ML, Frauwirth KA, Thompson CB. T-cell regulation by CD28 and CTLA-4. Nat Rev Immunol (2001) 1:220–8. doi:10.1038/35105024
49. Peach RJ, Bajorath J, Brady W, Leytze G, Greene J, Naemura J, et al. Complementarity determining region 1 (CDR1)- and CDR3-analogous regions in CTLA-4 and CD28 determine the binding to B7-1. J Exp Med (1994) 180(6):2049–58. doi:10.1084/jem.180.6.2049
50. Bluestone J. New perspectives of CD28-B7-mediated T cell costimulation. Immunity (1995) 2(6):555–9. doi:10.1016/1074-7613(95)90000-4
51. Salek-Ardakani S, Choi YS, Rafii-El-Idrissi Benhnia M, Flynn R, Arens R, Shoenberger S, et al. B cell-specific expression of B7-2 is required for follicular Th cell function in response to vaccinia virus. J Immunol (2011) 186:5294–303. doi:10.4049/jimmunol.1100406
52. Wang CJ, Heuts F, Ovcinnikovs V, Wardzinski L, Bowers C, Schmidt EM, et al. CTLA-4 controls follicular helper T-cell differentiation by regulating the strength of CD28 engagement. Proc Natl Acad Sci U S A (2015) 112:524–9. doi:10.1073/pnas.1414576112
53. Linterman MA, Denton AE, Divekar DP, Zvetkova I, Kane L, Ferreira C, et al. CD28 expression is required after T cell priming for helper T cell responses and protective immunity to infection. Elife (2014) 3:e03180. doi:10.7554/eLife.03180
54. Leavenworth JW, Verbinnen B, Yin J, Huang H, Cantor H. A p85alpha-osteopontin axis couples the receptor ICOS to sustained Bcl-6 expression by follicular helper and regulatory T cells. Nat Immunol (2015) 16:96–106. doi:10.1038/ni.3050
55. Chen S, Cai C, Li Z, Liu G, Wang Y, Blonska M, et al. Dissection of SAP-dependent and SAP-independent SLAM family signaling in NKT cell development and humoral immunity. J Exp Med (2017) 214:475–89. doi:10.1084/jem.20161312
56. Ma CS, Deenick EK. The role of SAP and SLAM family molecules in the humoral immune response. Ann N Y Acad Sci (2011) 1217:32–44. doi:10.1111/j.1749-6632.2010.05824.x
57. Cannons JL, Tangye SG, Schwartzberg PL. SLAM family receptors and SAP adaptors in immunity. Annu Rev Immunol (2011) 29:665–705. doi:10.1146/annurev-immunol-030409-101302
58. Cannons JL, Qi H, Lu KT, Dutta M, Gomez-Rodriguez J, Cheng J, et al. Optimal germinal center responses require a multistage T cell:B cell adhesion process involving integrins, SLAM-associated protein, and CD84. Immunity (2010) 32:253–65. doi:10.1016/j.immuni.2010.01.010
59. Kageyama R, Cannons JL, Zhao F, Yusuf I, Lao C, Locci M, et al. The receptor Ly108 functions as a SAP adaptor-dependent on-off switch for T cell help to B cells and NKT cell development. Immunity (2012) 36:986–1002. doi:10.1016/j.immuni.2012.05.016
60. Yusuf I, Kageyama R, Monticelli L, Johnston RJ, Ditoro D, Hansen K, et al. Germinal center T follicular helper cell IL-4 production is dependent on signaling lymphocytic activation molecule receptor (CD150). J Immunol (2010) 185:190–202. doi:10.4049/jimmunol.0903505
61. Crotty S, Kersh EN, Cannons J, Schwartzberg PL, Ahmed R. SAP is required for generating long-term humoral immunity. Nature (2003) 421:282–7. doi:10.1038/nature01318
62. Kroenke MA, Eto D, Locci M, Cho M, Davidson T, Haddad EK, et al. Bcl6 and Maf cooperate to instruct human follicular helper CD4 T cell differentiation. J Immunol (2012) 188:3734–44. doi:10.4049/jimmunol.1103246
63. Hu J, Havenar-Daughton C, Crotty S. Modulation of SAP dependent T:B cell interactions as a strategy to improve vaccination. Curr Opin Virol (2013) 3:363–70. doi:10.1016/j.coviro.2013.05.015
64. Cannons JL, Yu LJ, Jankovic D, Crotty S, Horai R, Kirby M, et al. SAP regulates T cell-mediated help for humoral immunity by a mechanism distinct from cytokine regulation. J Exp Med (2006) 203:1551–65. doi:10.1084/jem.20052097
65. Qi H, Cannons JL, Klauschen F, Schwartzberg PL, Germain RN. SAP-controlled T-B cell interactions underlie germinal centre formation. Nature (2008) 455:764–9. doi:10.1038/nature07345
66. Latour S, Roncagalli R, Chen R, Bakinowski M, Shi X, Schwartzberg PL, et al. Binding of SAP SH2 domain to FynT SH3 domain reveals a novel mechanism of receptor signalling in immune regulation. Nat Cell Biol (2003) 5:149–54. doi:10.1038/ncb919
67. Cannons JL, Wu JZ, Gomez-Rodriguez J, Zhang J, Dong B, Liu Y, et al. Biochemical and genetic evidence for a SAP-PKC-theta interaction contributing to IL-4 regulation. J Immunol (2010) 185:2819–27. doi:10.4049/jimmunol.0902182
68. Zhao F, Cannons JL, Dutta M, Griffiths GM, Schwartzberg PL. Positive and negative signaling through SLAM receptors regulate synapse organization and thresholds of cytolysis. Immunity (2012) 36:1003–16. doi:10.1016/j.immuni.2012.05.017
69. Nocentini G, Giunchi L, Ronchetti S, Krausz LT, Bartoli A, Moraca R, et al. A new member of the tumor necrosis factor/nerve growth factor receptor family inhibits T cell receptor-induced apoptosis. Proc Nat Acad Sci U S A (1997) 94:6216–21. doi:10.1073/pnas.94.12.6216
70. Knee DA, Hewes B, Brogdon JL. Rationale for anti-GITR cancer immunotherapy. Eur J Cancer (2016) 67:1–10. doi:10.1016/j.ejca.2016.06.028
71. Stephens GL, McHugh RS, Whitters MJ, Young DA, Luxenberg D, Carreno BM, et al. Engagement of glucocorticoid-induced TNFR family-related receptor on effector T cells by its ligand mediates resistance to suppression by CD4+CD25+ T cells. J Immunol (2004) 173:5008–20. doi:10.4049/jimmunol.173.8.5008
72. Butcher MJ, Filipowicz AR, Waseem TC, McGary CM, Crow KJ, Magilnick N, et al. Atherosclerosis-driven Treg plasticity results in formation of a dysfunctional subset of plastic IFNgamma+ Th1/Tregs. Circ Res (2016) 119:1190–203. doi:10.1161/CIRCRESAHA.116.309764
73. Clouthier DL, Zhou AC, Wortzman ME, Luft O, Levy GA, Watts TH. GITR intrinsically sustains early type 1 and late follicular helper CD4 T cell accumulation to control a chronic viral infection. PLoS Pathog (2015) 11:e1004517. doi:10.1371/journal.ppat.1004517
74. Good-Jacobson KL, Szumilas CG, Chen L, Sharpe AH, Tomayko MM, Shlomchik MJ. PD-1 regulates germinal center B cell survival and the formation and affinity of long-lived plasma cells. Nat Immunol (2010) 11:535–42. doi:10.1038/ni.1877
75. Chung Y, Tanaka S, Chu F, Nurieva RI, Martinez GJ, Rawal S, et al. Follicular regulatory T cells expressing Foxp3 and Bcl-6 suppress germinal center reactions. Nat Med (2011) 17:983–8. doi:10.1038/nm.2426
76. Linterman MA, Pierson W, Lee SK, Kallies A, Kawamoto S, Rayner TF, et al. Foxp3+ follicular regulatory T cells control the germinal center response. Nat Med (2011) 17:975–82. doi:10.1038/nm.2425
77. Sage PT, Francisco LM, Carman CV, Sharpe AH. The receptor PD-1 controls follicular regulatory T cells in the lymph nodes and blood. Nat Immunol (2013) 14:152–61. doi:10.1038/ni.2496
78. Sage PT, Sharpe AH. T follicular regulatory cells. Immunol Rev (2016) 271:246–59. doi:10.1111/imr.12411
79. Francisco LM, Sage PT, Sharpe AH. The PD-1 pathway in tolerance and autoimmunity. Immunol Rev (2010) 236:219–42. doi:10.1111/j.1600-065X.2010.00923.x
80. Keir ME, Butte MJ, Freeman GJ, Sharpe AH. PD-1 and its ligands in tolerance and immunity. Annu Rev Immunol (2008) 26:677–704. doi:10.1146/annurev.immunol.26.021607.090331
81. Hamel KM, Cao Y, Wang Y, Rodeghero R, Kobezda T, Chen L, et al. B7-H1 expression on non-B and non-T cells promotes distinct effects on T- and B-cell responses in autoimmune arthritis. Eur J Immunol (2010) 40:3117–27. doi:10.1002/eji.201040690
82. Kawamoto S, Tran TH, Maruya M, Suzuki K, Doi Y, Tsutsui Y, et al. The inhibitory receptor PD-1 regulates IgA selection and bacterial composition in the gut. Science (2012) 336:485–9. doi:10.1126/science.1217718
83. Hams E, McCarron MJ, Amu S, Yagita H, Azuma M, Chen L, et al. Blockade of B7-H1 (programmed death ligand 1) enhances humoral immunity by positively regulating the generation of T follicular helper cells. J Immunol (2011) 186:5648–55. doi:10.4049/jimmunol.1003161
84. Velu V, Titanji K, Zhu B, Husain S, Pladevega A, Lai L, et al. Enhancing SIV-specific immunity in vivo by PD-1 blockade. Nature (2009) 458:206–10. doi:10.1038/nature07662
85. Butler NS, Moebius J, Pewe LL, Traore B, Doumbo OK, Tygrett LT, et al. Therapeutic blockade of PD-L1 and LAG-3 rapidly clears established blood-stage Plasmodium infection. Nat Immunol (2011) 13:188–95. doi:10.1038/ni.2180
86. Sage PT, Tan CL, Freeman GJ, Haigis M, Sharpe AH. Defective TFH cell function and increased TFR cells contribute to defective antibody production in aging. Cell Rep (2015) 12:163–71. doi:10.1016/j.celrep.2015.06.015
87. Badell IR, La Muraglia GM II, Liu D, Wagener ME, Ding G, Ford ML. Selective CD28 blockade results in superior inhibition of donor-specific T follicular helper cell and antibody responses relative to CTLA4-Ig. Am J Transplant (2018) 18:89–101. doi:10.1111/ajt.14400
88. Walunas TL, Lenschow DJ, Bakker CY, Linsley PS, Freeman GJ, Green JM, et al. CTLA-4 can function as a negative regulator of T cell activation. Immunity (1994) 1:405–13. doi:10.1016/1074-7613(94)90071-X
89. Krummel MF. CD28 and CTLA-4 have opposing effects on the response of T cells to stimulation. J Exp Med (1995) 182:459–65. doi:10.1084/jem.182.2.459
90. Tivol EA, Borriello F, Schweitzer AN, Lynch WP, Bluestone JA, Sharpe AH. Loss of CTLA-4 leads to massive lymphoproliferation and fatal multiorgan tissue destruction, revealing a critical negative regulatory role of CTLA-4. Immunity (1995) 3:541–7. doi:10.1016/1074-7613(95)90125-6
91. Perez VL, Van Parijs L, Biuckians A, Zheng XX, Strom TB, Abbas AK. Induction of peripheral T cell tolerance in vivo requires CTLA-4 engagement. Immunity (1997) 6:411–7. doi:10.1016/S1074-7613(00)80284-8
92. Leach DR, Krummel MF, Allison JP. Enhancement of antitumor immunity by CTLA-4 blockade. Science (1996) 271:1734–6. doi:10.1126/science.271.5256.1734
93. Walker LS. Treg and CTLA-4: two intertwining pathways to immune tolerance. J Autoimmun (2013) 45:49–57. doi:10.1016/j.jaut.2013.06.006
94. Tivol EA, Boyd SD, McKeon S, Borriello F, Nickerson P, Strom TB, et al. CTLA4Ig prevents lymphoproliferation and fatal multiorgan tissue destruction in CTLA-4-deficient mice. J Immunol (1997) 158:5091–4.
95. Tang Q, Boden EK, Henriksen KJ, Bour-Jordan H, Bi M, Bluestone JA. Distinct roles of CTLA-4 and TGF-beta in CD4+CD25+ regulatory T cell function. Eur J Immunol (2004) 34:2996–3005. doi:10.1002/eji.200425143
96. Wing K, Onishi Y, Prieto-Martin P, Yamaguchi T, Miyara M, Fehervari Z, et al. CTLA-4 control over Foxp3+ regulatory T cell function. Science (2008) 322:271–5. doi:10.1126/science.1160062
97. Wing JB, Ise W, Kurosaki T, Sakaguchi S. Regulatory T cells control antigen-specific expansion of Tfh cell number and humoral immune responses via the coreceptor CTLA-4. Immunity (2014) 41:1013–25. doi:10.1016/j.immuni.2014.12.006
98. Cheung TC, Humphreys IR, Potter KG, Norris PS, Shumway HM, Tran BR, et al. Evolutionarily divergent herpesviruses modulate T cell activation by targeting the herpesvirus entry mediator cosignaling pathway. Proc Natl Acad Sci U S A (2005) 102:13218–23. doi:10.1073/pnas.0506172102
99. del Rio ML, Jones ND, Buhler L, Norris P, Shintani Y, Ware CF, et al. Selective blockade of herpesvirus entry mediator-B and T lymphocyte attenuator pathway ameliorates acute graft-versus-host reaction. J Immunol (2012) 188:4885–96. doi:10.4049/jimmunol.1103698
100. del Rio ML, Kaye J, Rodriguez-Barbosa JI. Detection of protein on BTLAlow cells and in vivo antibody-mediated down-modulation of BTLA on lymphoid and myeloid cells of C57BL/6 and BALB/c BTLA allelic variants. Immunobiology (2010) 215:570–8. doi:10.1016/j.imbio.2009.09.008
101. Oya Y, Watanabe N, Owada T, Oki M, Hirose K, Suto A, et al. Development of autoimmune hepatitis-like disease and production of autoantibodies to nuclear antigens in mice lacking B and T lymphocyte attenuator. Arthritis Rheum (2008) 58:2498–510. doi:10.1002/art.23674
102. Kashiwakuma D, Suto A, Hiramatsu Y, Ikeda K, Takatori H, Suzuki K, et al. B and T lymphocyte attenuator suppresses IL-21 production from follicular Th cells and subsequent humoral immune responses. J Immunol (2010) 185:2730–6. doi:10.4049/jimmunol.0903839
103. Eto D, Lao C, DiToro D, Barnett B, Escobar TC, Kageyama R, et al. IL-21 and IL-6 are critical for different aspects of B cell immunity and redundantly induce optimal follicular helper CD4 T cell (Tfh) differentiation. PLoS One (2011) 6:e17739. doi:10.1371/journal.pone.0017739
104. Batten M, Ramamoorthi N, Kljavin NM, Ma CS, Cox JH, Dengler HS, et al. IL-27 supports germinal center function by enhancing IL-21 production and the function of T follicular helper cells. J Exp Med (2010) 207:2895–906. doi:10.1084/jem.20100064
105. Nurieva RI, Chung Y. Understanding the development and function of T follicular helper cells. Cell Mol Immunol (2010) 7:190–7. doi:10.1038/cmi.2010.24
106. Liu X, Nurieva RI, Dong C. Transcriptional regulation of follicular T-helper (Tfh) cells. Immunol Rev (2013) 252:139–45. doi:10.1111/imr.12040
107. Choi YS, Eto D, Yang JA, Lao C, Crotty S. Cutting edge: STAT1 is required for IL-6-mediated Bcl6 induction for early follicular helper cell differentiation. J Immunol (2013) 190:3049–53. doi:10.4049/jimmunol.1203032
108. Chavele KM, Merry E, Ehrenstein MR. Cutting edge: circulating plasmablasts induce the differentiation of human T follicular helper cells via IL-6 production. J Immunol (2015) 194:2482–5. doi:10.4049/jimmunol.1401190
109. Nurieva R, Yang XO, Martinez G, Zhang Y, Panopoulos AD, Ma L, et al. Essential autocrine regulation by IL-21 in the generation of inflammatory T cells. Nature (2007) 448:480–3. doi:10.1038/nature05969
110. Vogelzang A, McGuire HM, Yu D, Sprent J, Mackay CR, King C. A fundamental role for interleukin-21 in the generation of T follicular helper cells. Immunity (2008) 29:127–37. doi:10.1016/j.immuni.2008.06.001
111. Gringhuis SI, Kaptein TM, Wevers BA, van der Vlist M, Klaver EJ, van Die I, et al. Fucose-based PAMPs prime dendritic cells for follicular T helper cell polarization via DC-SIGN-dependent IL-27 production. Nat Commun (2014) 5:5074. doi:10.1038/ncomms6074
112. Owaki T, Asakawa M, Kamiya S, Takeda K, Fukai F, Mizuguchi J, et al. IL-27 suppresses CD28-mediated [correction of medicated] IL-2 production through suppressor of cytokine signaling 3. J Immunol (2006) 176:2773–80. doi:10.4049/jimmunol.176.5.2773
113. Peng X, Remacle JE, Kasran A, Huylebroeck D, Ceuppens JL. IL-12 up-regulates CD40 ligand (CD154) expression on human T cells. J Immunol (1998) 160:1166–72.
114. McCarron MJ, Marie JC. TGF-beta prevents T follicular helper cell accumulation and B cell autoreactivity. J Clin Invest (2014) 124:4375–86. doi:10.1172/JCI76179
115. Choi YS, Kageyama R, Eto D, Escobar TC, Johnston RJ, Monticelli L, et al. ICOS receptor instructs T follicular helper cell versus effector cell differentiation via induction of the transcriptional repressor Bcl6. Immunity (2011) 34:932–46. doi:10.1016/j.immuni.2011.03.023
116. Pepper M, Pagan AJ, Igyarto BZ, Taylor JJ, Jenkins MK. Opposing signals from the Bcl6 transcription factor and the interleukin-2 receptor generate T helper 1 central and effector memory cells. Immunity (2011) 35:583–95. doi:10.1016/j.immuni.2011.09.009
117. Liao W, Lin JX, Wang L, Li P, Leonard WJ. Modulation of cytokine receptors by IL-2 broadly regulates differentiation into helper T cell lineages. Nat Immunol (2011) 12:551–9. doi:10.1038/ni.2030
118. Ray JP, Staron MM, Shyer JA, Ho PC, Marshall HD, Gray SM, et al. The interleukin-2-mTORc1 kinase axis defines the signaling, differentiation, and metabolism of T helper 1 and follicular B Helper T cells. Immunity (2015) 43:690–702. doi:10.1016/j.immuni.2015.08.017
119. Cai G, Nie X, Zhang W, Wu B, Lin J, Wang H, et al. A regulatory role for IL-10 receptor signaling in development and B cell help of T follicular helper cells in mice. J Immunol (2012) 189:1294–302. doi:10.4049/jimmunol.1102948
120. Sahoo A, Alekseev A, Tanaka K, Obertas L, Lerman B, Haymaker C, et al. Batf is important for IL-4 expression in T follicular helper cells. Nat Commun (2015) 6:7997. doi:10.1038/ncomms8997
121. Gong F, Su Q, Jiang D, Chen J, Pan Y, Huang X. High frequency of circulating follicular helper T cells in patients with bronchial asthma. Clin Lab (2014) 60:963–8. doi:10.7754/Clin.Lab.2013.130427
122. Crotty S, Johnston RJ, Schoenberger SP. Effectors and memories: Bcl-6 and Blimp-1 in T and B lymphocyte differentiation. Nat Immunol (2010) 11:114–20. doi:10.1038/ni.1837
123. Martins G, Calame K. Regulation and functions of Blimp-1 in T and B lymphocytes. Annu Rev Immunol (2008) 26:133–69. doi:10.1146/annurev.immunol.26.021607.090241
124. Hatzi K, Nance JP, Kroenke MA, Bothwell M, Haddad EK, Melnick A, et al. BCL6 orchestrates Tfh cell differentiation via multiple distinct mechanisms. J Exp Med (2015) 212:539–53. doi:10.1084/jem.20141380
125. Kusam S, Toney LM, Sato H, Dent AL. Inhibition of Th2 differentiation and GATA-3 expression by BCL-6. J Immunol (2003) 170:2435–41. doi:10.4049/jimmunol.170.5.2435
126. Liu X, Lu H, Chen T, Nallaparaju KC, Yan X, Tanaka S, et al. Genome-wide analysis identifies Bcl6-controlled regulatory networks during T follicular helper cell differentiation. Cell Rep (2016) 14:1735–47. doi:10.1016/j.celrep.2016.01.038
127. Andris F, Denanglaire S, Anciaux M, Hercor M, Hussein H, Leo O. The transcription factor c-Maf promotes the differentiation of follicular helper T cells. Front Immunol (2017) 8:480. doi:10.3389/fimmu.2017.00480
128. Schraml BU, Hildner K, Ise W, Lee WL, Smith WA, Solomon B, et al. The AP-1 transcription factor Batf controls T(H)17 differentiation. Nature (2009) 460:405–9. doi:10.1038/nature08114
129. Betz BC, Jordan-Williams KL, Wang C, Kang SG, Liao J, Logan MR, et al. Batf coordinates multiple aspects of B and T cell function required for normal antibody responses. J Exp Med (2010) 207:933–42. doi:10.1084/jem.20091548
130. Ise W, Kohyama M, Schraml BU, Zhang T, Schwer B, Basu U, et al. The transcription factor BATF controls the global regulators of class-switch recombination in both B cells and T cells. Nat Immunol (2011) 12:536–43. doi:10.1038/ni.2037
131. Brustle A, Heink S, Huber M, Rosenplanter C, Stadelmann C, Yu P, et al. The development of inflammatory T(H)-17 cells requires interferon-regulatory factor 4. Nat Immunol (2007) 8:958–66. doi:10.1038/ni1500
132. Huber M, Brustle A, Reinhard K, Guralnik A, Walter G, Mahiny A, et al. IRF4 is essential for IL-21-mediated induction, amplification, and stabilization of the Th17 phenotype. Proc Natl Acad Sci U S A (2008) 105:20846–51. doi:10.1073/pnas.0809077106
133. Lohoff M, Mittrucker HW, Prechtl S, Bischof S, Sommer F, Kock S, et al. Dysregulated T helper cell differentiation in the absence of interferon regulatory factor 4. Proc Natl Acad Sci U S A (2002) 99:11808–12. doi:10.1073/pnas.182425099
134. Rengarajan J, Mowen KA, McBride KD, Smith ED, Singh H, Glimcher LH. Interferon regulatory factor 4 (IRF4) interacts with NFATc2 to modulate interleukin 4 gene expression. J Exp Med (2002) 195:1003–12. doi:10.1084/jem.20011128
135. Zheng Y, Chaudhry A, Kas A, deRoos P, Kim JM, Chu TT, et al. Regulatory T-cell suppressor program co-opts transcription factor IRF4 to control T(H)2 responses. Nature (2009) 458:351–6. doi:10.1038/nature07674
136. Chen Q, Yang W, Gupta S, Biswas P, Smith P, Bhagat G, et al. IRF-4-binding protein inhibits interleukin-17 and interleukin-21 production by controlling the activity of IRF-4 transcription factor. Immunity (2008) 29:899–911. doi:10.1016/j.immuni.2008.10.011
137. Staudt V, Bothur E, Klein M, Lingnau K, Reuter S, Grebe N, et al. Interferon-regulatory factor 4 is essential for the developmental program of T helper 9 cells. Immunity (2010) 33:192–202. doi:10.1016/j.immuni.2010.07.014
138. Klein U, Casola S, Cattoretti G, Shen Q, Lia M, Mo T, et al. Transcription factor IRF4 controls plasma cell differentiation and class-switch recombination. Nat Immunol (2006) 7:773–82. doi:10.1038/ni1357
139. Sciammas R, Shaffer AL, Schatz JH, Zhao H, Staudt LM, Singh H. Graded expression of interferon regulatory factor-4 coordinates isotype switching with plasma cell differentiation. Immunity (2006) 25:225–36. doi:10.1016/j.immuni.2006.07.009
140. Bollig N, Brustle A, Kellner K, Ackermann W, Abass E, Raifer H, et al. Transcription factor IRF4 determines germinal center formation through follicular T-helper cell differentiation. Proc Natl Acad Sci U S A (2012) 109:8664–9. doi:10.1073/pnas.1205834109
141. Li P, Spolski R, Liao W, Wang L, Murphy TL, Murphy KM, et al. BATF-JUN is critical for IRF4-mediated transcription in T cells. Nature (2012) 490:543–6. doi:10.1038/nature11530
142. Kwon H, Thierry-Mieg D, Thierry-Mieg J, Kim HP, Oh J, Tunyaplin C, et al. Analysis of interleukin-21-induced Prdm1 gene regulation reveals functional cooperation of STAT3 and IRF4 transcription factors. Immunity (2009) 31:941–52. doi:10.1016/j.immuni.2009.10.008
143. Gupta S, Jiang M, Anthony A, Pernis AB. Lineage-specific modulation of interleukin 4 signaling by interferon regulatory factor 4. J Exp Med (1999) 190:1837–48. doi:10.1084/jem.190.12.1837
144. Krishnamoorthy V, Kannanganat S, Maienschein-Cline M, Cook SL, Chen J, Bahroos N, et al. The IRF4 gene regulatory module functions as a read-write integrator to dynamically coordinate T helper cell fate. Immunity (2017) 47:481–97.e7. doi:10.1016/j.immuni.2017.09.001
145. Nakayamada S, Poholek AC, Lu KT, Takahashi H, Kato M, Iwata S, et al. Type I IFN induces binding of STAT1 to Bcl6: divergent roles of STAT family transcription factors in the T follicular helper cell genetic program. J Immunol (2014) 192:2156–66. doi:10.4049/jimmunol.1300675
146. Zhong Z, Wen Z, Darnell JE Jr. Stat3: a STAT family member activated by tyrosine phosphorylation in response to epidermal growth factor and interleukin-6. Science (1994) 264:95–8. doi:10.1126/science.8140422
147. Asao H, Okuyama C, Kumaki S, Ishii N, Tsuchiya S, Foster D, et al. Cutting edge: the common gamma-chain is an indispensable subunit of the IL-21 receptor complex. J Immunol (2001) 167:1–5. doi:10.4049/jimmunol.167.1.1
148. Read KA, Powell MD, Baker CE, Sreekumar BK, Ringel-Scaia VM, Bachus H, et al. Integrated STAT3 and Ikaros zinc finger transcription factor activities regulate Bcl-6 expression in CD4(+) Th cells. J Immunol (2017) 199:2377–87. doi:10.4049/jimmunol.1700106
149. Ma CS, Avery DT, Chan A, Batten M, Bustamante J, Boisson-Dupuis S, et al. Functional STAT3 deficiency compromises the generation of human T follicular helper cells. Blood (2012) 119:3997–4008. doi:10.1182/blood-2011-11-392985
150. Schmitt N, Morita R, Bourdery L, Bentebibel SE, Zurawski SM, Banchereau J, et al. Human dendritic cells induce the differentiation of interleukin-21-producing T follicular helper-like cells through interleukin-12. Immunity (2009) 31:158–69. doi:10.1016/j.immuni.2009.04.016
151. Weinstein JS, Laidlaw BJ, Lu Y, Wang JK, Schulz VP, Li N, et al. STAT4 and T-bet control follicular helper T cell development in viral infections. J Exp Med (2018) 215:337–55. doi:10.1084/jem.2017045702062018c
152. Auderset F, Coutaz M, Tacchini-Cottier F. The role of Notch in the differentiation of CD4(+) T helper cells. Curr Top Microbiol Immunol (2012) 360:115–34. doi:10.1007/82_2012_227
153. Auderset F, Schuster S, Fasnacht N, Coutaz M, Charmoy M, Koch U, et al. Notch signaling regulates follicular helper T cell differentiation. J Immunol (2013) 191:2344–50. doi:10.4049/jimmunol.1300643
154. Dell’Aringa M, Reinhardt RL. Notch signaling represents an important checkpoint between follicular T-helper and canonical T-helper 2 cell fate. Mucosal Immunol (2018) 11:1079–91. doi:10.1038/s41385-018-0012-9
155. Vaeth M, Muller G, Stauss D, Dietz L, Klein-Hessling S, Serfling E, et al. Follicular regulatory T cells control humoral autoimmunity via NFAT2-regulated CXCR5 expression. J Exp Med (2014) 211:545–61. doi:10.1084/jem.20130604
156. Martinez GJ, Hu JK, Pereira RM, Crampton JS, Togher S, Bild N, et al. Cutting edge: NFAT transcription factors promote the generation of follicular helper T cells in response to acute viral infection. J Immunol (2016) 196:2015–9. doi:10.4049/jimmunol.1501841
157. Liu X, Chen X, Zhong B, Wang A, Wang X, Chu F, et al. Transcription factor achaete-scute homologue 2 initiates follicular T-helper-cell development. Nature (2014) 507:513–8. doi:10.1038/nature12910
158. Choi YS, Gullicksrud JA, Xing S, Zeng Z, Shan Q, Li F, et al. LEF-1 and TCF-1 orchestrate T(FH) differentiation by regulating differentiation circuits upstream of the transcriptional repressor Bcl6. Nat Immunol (2015) 16:980–90. doi:10.1038/ni.3226
159. Wu T, Shin HM, Moseman EA, Ji Y, Huang B, Harly C, et al. TCF1 is required for the T follicular helper cell response to viral infection. Cell Rep (2015) 12:2099–110. doi:10.1016/j.celrep.2015.08.049
160. Xu L, Cao Y, Xie Z, Huang Q, Bai Q, Yang X, et al. The transcription factor TCF-1 initiates the differentiation of T(FH) cells during acute viral infection. Nat Immunol (2015) 16:991–9. doi:10.1038/ni.3229
161. Weber BN, Chi AW, Chavez A, Yashiro-Ohtani Y, Yang Q, Shestova O, et al. A critical role for TCF-1 in T-lineage specification and differentiation. Nature (2011) 476:63–8. doi:10.1038/nature10279
162. Yu S, Zhou X, Steinke FC, Liu C, Chen SC, Zagorodna O, et al. The TCF-1 and LEF-1 transcription factors have cooperative and opposing roles in T cell development and malignancy. Immunity (2012) 37:813–26. doi:10.1016/j.immuni.2012.08.009
163. Ogbe A, Miao T, Symonds AL, Omodho B, Singh R, Bhullar P, et al. Early growth response genes 2 and 3 regulate the expression of Bcl6 and differentiation of T follicular helper cells. J Biol Chem (2015) 290:20455–65. doi:10.1074/jbc.M114.634816
164. Stauss D, Brunner C, Berberich-Siebelt F, Hopken UE, Lipp M, Muller G. The transcriptional coactivator Bob1 promotes the development of follicular T helper cells via Bcl6. EMBO J (2016) 35:881–98. doi:10.15252/embj.201591459
165. Barnett LG, Simkins HM, Barnett BE, Korn LL, Johnson AL, Wherry EJ, et al. B cell antigen presentation in the initiation of follicular helper T cell and germinal center differentiation. J Immunol (2014) 192:3607–17. doi:10.4049/jimmunol.1301284
166. Schwickert TA, Victora GD, Fooksman DR, Kamphorst AO, Mugnier MR, Gitlin AD, et al. T cell-limited checkpoint regulates affinity-dependent B cell entry into the germinal center. J Exp Med (2011) 208:1243–52. doi:10.1084/jem.20102477
167. Serre K, Mohr E, Benezech C, Bird R, Khan M, Caamano JH, et al. Selective effects of NF-kappaB1 deficiency in CD4(+) T cells on Th2 and TFh induction by alum-precipitated protein vaccines. Eur J Immunol (2011) 41:1573–82. doi:10.1002/eji.201041126
168. Hu H, Wu X, Jin W, Chang M, Cheng X, Sun SC. Noncanonical NF-kappaB regulates inducible costimulator (ICOS) ligand expression and T follicular helper cell development. Proc Natl Acad Sci U S A (2011) 108:12827–32. doi:10.1073/pnas.1105774108
169. Xiao N, Eto D, Elly C, Peng G, Crotty S, Liu YC. The E3 ubiquitin ligase Itch is required for the differentiation of follicular helper T cells. Nat Immunol (2014) 15:657–66. doi:10.1038/ni.2912
170. Wang H, Geng J, Wen X, Bi E, Kossenkov AV, Wolf AI, et al. The transcription factor Foxp1 is a critical negative regulator of the differentiation of follicular helper T cells. Nat Immunol (2014) 15:667–75. doi:10.1038/ni.2890
171. Lee JY, Skon CN, Lee YJ, Oh S, Taylor JJ, Malhotra D, et al. The transcription factor KLF2 restrains CD4(+) T follicular helper cell differentiation. Immunity (2015) 42:252–64. doi:10.1016/j.immuni.2015.01.013
172. Johnston RJ, Choi YS, Diamond JA, Yang JA, Crotty S. STAT5 is a potent negative regulator of TFH cell differentiation. J Exp Med (2012) 209:243–50. doi:10.1084/jem.20111174
173. Park HJ, Kim DH, Choi JY, Kim WJ, Kim JY, Senejani AG, et al. PPARgamma negatively regulates T cell activation to prevent follicular helper T cells and germinal center formation. PLoS One (2014) 9:e99127. doi:10.1371/journal.pone.0099127
174. Vanderleyden I, Linterman MA, Smith KG. Regulatory T cells and control of the germinal centre response. Arthritis Res Ther (2014) 16:471. doi:10.1186/s13075-014-0471-7
175. Wollenberg I, Agua-Doce A, Hernandez A, Almeida C, Oliveira VG, Faro J, et al. Regulation of the germinal center reaction by Foxp3+ follicular regulatory T cells. J Immunol (2011) 187:4553–60. doi:10.4049/jimmunol.1101328
176. Zhu Y, Zou L, Liu YC. T follicular helper cells, T follicular regulatory cells and autoimmunity. Int Immunol (2016) 28:173–9. doi:10.1093/intimm/dxv079
177. Dhaeze T, Stinissen P, Liston A, Hellings N. Humoral autoimmunity: a failure of regulatory T cells? Autoimmun Rev (2015) 14:735–41. doi:10.1016/j.autrev.2015.04.006
178. Sage PT, Sharpe AH. T follicular regulatory cells in the regulation of B cell responses. Trends Immunol (2015) 36:410–8. doi:10.1016/j.it.2015.05.005
179. Xu L, Huang Q, Wang H, Hao Y, Bai Q, Hu J, et al. The kinase mTORC1 promotes the generation and suppressive function of follicular regulatory T cells. Immunity (2017) 47:538–551.e5. doi:10.1016/j.immuni.2017.08.011
180. Chang JH, Hu H, Jin J, Puebla-Osorio N, Xiao Y, Gilbert BE, et al. TRAF3 regulates the effector function of regulatory T cells and humoral immune responses. J Exp Med (2014) 211:137–51. doi:10.1084/jem.20131019
181. Jandl C, Liu SM, Canete PF, Warren J, Hughes WE, Vogelzang A, et al. IL-21 restricts T follicular regulatory T cell proliferation through Bcl-6 mediated inhibition of responsiveness to IL-2. Nat Commun (2017) 8:14647. doi:10.1038/ncomms14647
182. Maceiras AR, Fonseca VR, Agua-Doce A, Graca L. T follicular regulatory cells in mice and men. Immunology (2017) 152:25–35. doi:10.1111/imm.12774
183. Sage PT, Alvarez D, Godec J, von Andrian UH, Sharpe AH. Circulating T follicular regulatory and helper cells have memory-like properties. J Clin Invest (2014) 124:5191–204. doi:10.1172/JCI76861
184. Vignali DA, Collison LW, Workman CJ. How regulatory T cells work. Nat Rev Immunol (2008) 8:523–32. doi:10.1038/nri2343
185. Laidlaw BJ, Lu Y, Amezquita RA, Weinstein JS, Vander Heiden JA, Gupta NT, et al. Interleukin-10 from CD4(+) follicular regulatory T cells promotes the germinal center response. Sci Immunol (2017) 2:eaan4767. doi:10.1126/sciimmunol.aan4767
186. Hori S, Nomura T, Sakaguchi S. Control of regulatory T cell development by the transcription factor Foxp3. Science (2003) 299:1057–61. doi:10.1126/science.1079490
187. Mayer CT, Floess S, Baru AM, Lahl K, Huehn J, Sparwasser T. CD8+ Foxp3+ T cells share developmental and phenotypic features with classical CD4+ Foxp3+ regulatory T cells but lack potent suppressive activity. Eur J Immunol (2011) 41:716–25. doi:10.1002/eji.201040913
188. Joosten SA, van Meijgaarden KE, Savage ND, de Boer T, Triebel F, van der Wal A, et al. Identification of a human CD8+ regulatory T cell subset that mediates suppression through the chemokine CC chemokine ligand 4. Proc Natl Acad Sci U S A (2007) 104:8029–34. doi:10.1073/pnas.0702257104
189. Kim HJ, Verbinnen B, Tang X, Lu L, Cantor H. Inhibition of follicular T-helper cells by CD8(+) regulatory T cells is essential for self tolerance. Nature (2010) 467:328–32. doi:10.1038/nature09370
190. Kim HJ, Wang X, Radfar S, Sproule TJ, Roopenian DC, Cantor H. CD8+ T regulatory cells express the Ly49 class I MHC receptor and are defective in autoimmune prone B6-Yaa mice. Proc Natl Acad Sci U S A (2011) 108:2010–5. doi:10.1073/pnas.1018974108
191. Taghavie-Moghadam PL, Waseem TC, Hattler J, Glenn LM, Dobrian AD, Kaplan MH, et al. STAT4 regulates the CD8(+) regulatory T cell/T follicular helper cell axis and promotes atherogenesis in insulin-resistant Ldlr(-/-) mice. J Immunol (2017) 199:3453–65. doi:10.4049/jimmunol.1601429
192. Endharti AT, Rifa IM, Shi Z, Fukuoka Y, Nakahara Y, Kawamoto Y, et al. Cutting edge: CD8+CD122+ regulatory T cells produce IL-10 to suppress IFN-gamma production and proliferation of CD8+ T cells. J Immunol (2005) 175:7093–7. doi:10.4049/jimmunol.175.11.7093
193. Holderried TA, Kim H-J, Lang PA, Cantor H. CD8+ Treg – from mouse to man. Blood (2013) 122:3474.
194. Miles B, Miller SM, Folkvord JM, Levy DN, Rakasz EG, Skinner PJ, et al. Follicular regulatory CD8 T cells impair the germinal center response in SIV and ex vivo HIV infection. PLoS Pathog (2016) 12:e1005924. doi:10.1371/journal.ppat.1005924
195. Rampuria P, Lang ML. CD1d-dependent expansion of NKT follicular helper cells in vivo and in vitro is a product of cellular proliferation and differentiation. Int Immunol (2015) 27:253–63. doi:10.1093/intimm/dxv007
196. Chang PP, Barral P, Fitch J, Pratama A, Ma CS, Kallies A, et al. Identification of Bcl-6-dependent follicular helper NKT cells that provide cognate help for B cell responses. Nat Immunol (2011) 13:35–43. doi:10.1038/ni.2166
197. Rampuria P, Lang GA, Devera TS, Gilmore C, Ballard JD, Lang ML. Coordination between T helper cells, iNKT cells, and their follicular helper subsets in the humoral immune response against Clostridium difficile toxin B. J Leukoc Biol (2017) 101:567–76. doi:10.1189/jlb.4A0616-271R
198. Lang ML. The influence of invariant natural killer T cells on humoral immunity to T-dependent and -independent antigens. Front Immunol (2018) 9:305. doi:10.3389/fimmu.2018.00305
199. Tonti E, Fedeli M, Napolitano A, Iannacone M, von Andrian UH, Guidotti LG, et al. Follicular helper NKT cells induce limited B cell responses and germinal center formation in the absence of CD4(+) T cell help. J Immunol (2012) 188:3217–22. doi:10.4049/jimmunol.1103501
200. Fukazawa Y, Lum R, Okoye AA, Park H, Matsuda K, Bae JY, et al. B cell follicle sanctuary permits persistent productive simian immunodeficiency virus infection in elite controllers. Nat Med (2015) 21:132–9. doi:10.1038/nm.3781
201. Perdomo-Celis F, Taborda NA, Rugeles MT. Follicular CD8(+) T cells: origin, function and importance during HIV infection. Front Immunol (2017) 8:1241. doi:10.3389/fimmu.2017.01241
202. Quigley MF, Gonzalez VD, Granath A, Andersson J, Sandberg JK. CXCR5+ CCR7- CD8 T cells are early effector memory cells that infiltrate tonsil B cell follicles. Eur J Immunol (2007) 37:3352–62. doi:10.1002/eji.200636746
203. He R, Hou S, Liu C, Zhang A, Bai Q, Han M, et al. Follicular CXCR5- expressing CD8(+) T cells curtail chronic viral infection. Nature (2016) 537:412–28. doi:10.1038/nature19317
204. Im SJ, Hashimoto M, Gerner MY, Lee J, Kissick HT, Burger MC, et al. Defining CD8+ T cells that provide the proliferative burst after PD-1 therapy. Nature (2016) 537:417–21. doi:10.1038/nature19330
205. Leong YA, Chen Y, Ong HS, Wu D, Man K, Deleage C, et al. CXCR5(+) follicular cytotoxic T cells control viral infection in B cell follicles. Nat Immunol (2016) 17:1187–96. doi:10.1038/ni.3543
206. Li S, Folkvord JM, Rakasz EG, Abdelaal HM, Wagstaff RK, Kovacs KJ, et al. Simian immunodeficiency virus-producing cells in follicles are partially suppressed by CD8+ cells in vivo. J Virol (2016) 90:11168–80. doi:10.1128/JVI.01332-16
207. Petrovas C, Ferrando-Martinez S, Gerner MY, Casazza JP, Pegu A, Deleage C, et al. Follicular CD8 T cells accumulate in HIV infection and can kill infected cells in vitro via bispecific antibodies. Sci Transl Med (2017) 9:eaag2285. doi:10.1126/scitranslmed.aag2285
208. Ferrando-Martinez S, Moysi E, Pegu A, Andrews S, Nganou Makamdop K, Ambrozak D, et al. Accumulation of follicular CD8+ T cells in pathogenic SIV infection. J Clin Invest (2018) 128:2089–103. doi:10.1172/JCI96207
209. Rahman MA, McKinnon KM, Karpova TS, Ball DA, Venzon DJ, Fan W, et al. Associations of simian immunodeficiency virus (SIV)-specific follicular CD8+ T cells with other follicular T cells suggest complex contributions to SIV viremia control. J Immunol (2018) 200:2714. doi:10.4049/jimmunol.1701403
210. Curran MA, Montalvo W, Yagita H, Allison JP. PD-1 and CTLA-4 combination blockade expands infiltrating T cells and reduces regulatory T and myeloid cells within B16 melanoma tumors. Proc Natl Acad Sci U S A (2010) 107:4275–80. doi:10.1073/pnas.0915174107
211. Jin Y, Lang C, Tang J, Geng J, Song HK, Sun Z, et al. CXCR5(+)CD8(+) T cells could induce the death of tumor cells in HBV-related hepatocellular carcinoma. Int Immunopharmacol (2017) 53:42–8. doi:10.1016/j.intimp.2017.10.009
212. Xu H, Liu J, Cui X, Zuo Y, Zhang Z, Li Y, et al. Increased frequency of circulating follicular helper T cells in lupus patients is associated with autoantibody production in a CD40L-dependent manner. Cell Immunol (2015) 295:46–51. doi:10.1016/j.cellimm.2015.01.014
213. Kawamoto M, Harigai M, Hara M, Kawaguchi Y, Tezuka K, Tanaka M, et al. Expression and function of inducible co-stimulator in patients with systemic lupus erythematosus: possible involvement in excessive interferon-gamma and anti-double-stranded DNA antibody production. Arthritis Res Ther (2006) 8:R62. doi:10.1186/ar1928
214. Wang L, Zhao P, Ma L, Shan Y, Jiang Z, Wang J, et al. Increased interleukin 21 and follicular helper T-like cells and reduced interleukin 10+ B cells in patients with new-onset systemic lupus erythematosus. J Rheumatol (2014) 41:1781–92. doi:10.3899/jrheum.131025
215. Terrier B, Costedoat-Chalumeau N, Garrido M, Geri G, Rosenzwajg M, Musset L, et al. Interleukin 21 correlates with T cell and B cell subset alterations in systemic lupus erythematosus. J Rheumatol (2012) 39:1819–28. doi:10.3899/jrheum.120468
216. Lan Y, Luo B, Wang JL, Jiang YW, Wei YS. The association of interleukin-21 polymorphisms with interleukin-21 serum levels and risk of systemic lupus erythematosus. Gene (2014) 538:94–8. doi:10.1016/j.gene.2014.01.012
217. Wong CK, Wong PT, Tam LS, Li EK, Chen DP, Lam CW. Elevated production of B cell chemokine CXCL13 is correlated with systemic lupus erythematosus disease activity. J Clin Immunol (2010) 30:45–52. doi:10.1007/s10875-009-9325-5
218. Lee J, Shin EK, Lee SY, Her YM, Park MK, Kwok SK, et al. Oestrogen up-regulates interleukin-21 production by CD4(+) T lymphocytes in patients with systemic lupus erythematosus. Immunology (2014) 142:573–80. doi:10.1111/imm.12263
219. Nakou M, Papadimitraki ED, Fanouriakis A, Bertsias GK, Choulaki C, Goulidaki N, et al. Interleukin-21 is increased in active systemic lupus erythematosus patients and contributes to the generation of plasma B cells. Clin Exp Rheumatol (2013) 31:172–9.
220. Liarski VM, Kaverina N, Chang A, Brandt D, Yanez D, Talasnik L, et al. Cell distance mapping identifies functional T follicular helper cells in inflamed human renal tissue. Sci Transl Med (2014) 6:230ra46. doi:10.1126/scitranslmed.3008146
221. Chang A, Henderson SG, Brandt D, Liu N, Guttikonda R, Hsieh C, et al. In situ B cell-mediated immune responses and tubulointerstitial inflammation in human lupus nephritis. J Immunol (2011) 186:1849–60. doi:10.4049/jimmunol.1001983
222. Vinuesa CG, Cook MC, Angelucci C, Athanasopoulos V, Rui L, Hill KM, et al. A RING-type ubiquitin ligase family member required to repress follicular helper T cells and autoimmunity. Nature (2005) 435:452–8. doi:10.1038/nature03555
223. Linterman MA, Rigby RJ, Wong RK, Yu D, Brink R, Cannons JL, et al. Follicular helper T cells are required for systemic autoimmunity. J Exp Med (2009) 206:561–76. doi:10.1084/jem.20081886
224. Bubier JA, Sproule TJ, Foreman O, Spolski R, Shaffer DJ, Morse HC III, et al. A critical role for IL-21 receptor signaling in the pathogenesis of systemic lupus erythematosus in BXSB-Yaa mice. Proc Natl Acad Sci U S A (2009) 106:1518–23. doi:10.1073/pnas.0807309106
225. Mandik-Nayak L, Seo SJ, Sokol C, Potts KM, Bui A, Erikson J. MRL-lpr/lpr mice exhibit a defect in maintaining developmental arrest and follicular exclusion of anti-double-stranded DNA B cells. J Exp Med (1999) 189:1799–814. doi:10.1084/jem.189.11.1799
226. Odegard JM, Marks BR, DiPlacido LD, Poholek AC, Kono DH, Dong C, et al. ICOS-dependent extrafollicular helper T cells elicit IgG production via IL-21 in systemic autoimmunity. J Exp Med (2008) 205:2873–86. doi:10.1084/jem.20080840
227. Simpson N, Gatenby PA, Wilson A, Malik S, Fulcher DA, Tangye SG, et al. Expansion of circulating T cells resembling follicular helper T cells is a fixed phenotype that identifies a subset of severe systemic lupus erythematosus. Arthritis Rheum (2010) 62:234–44. doi:10.1002/art.25032
228. Bohnhorst JO, Bjorgan MB, Thoen JE, Jonsson R, Natvig JB, Thompson KM. Abnormal B cell differentiation in primary Sjogren’s syndrome results in a depressed percentage of circulating memory B cells and elevated levels of soluble CD27 that correlate with serum IgG concentration. Clin Immunol (2002) 103:79–88. doi:10.1006/clim.2002.5199
229. Szabo K, Papp G, Barath S, Gyimesi E, Szanto A, Zeher M. Follicular helper T cells may play an important role in the severity of primary Sjögren’s syndrome. Clin Immunol (2013) 147:95–104. doi:10.1016/j.clim.2013.02.024
230. Bombardieri M, Barone F, Humby F, Kelly S, McGurk M, Morgan P, et al. Activation-induced cytidine deaminase expression in follicular dendritic cell networks and interfollicular large B cells supports functionality of ectopic lymphoid neogenesis in autoimmune sialoadenitis and MALT lymphoma in Sjogren’s syndrome. J Immunol (2007) 179:4929–38. doi:10.4049/jimmunol.179.7.4929
231. Sidiropoulos PI, Boumpas DT. Lessons learned from anti-CD40L treatment in systemic lupus erythematosus patients. Lupus (2004) 13:391–7. doi:10.1191/0961203304lu1032oa
232. Pertovaara M, Silvennoinen O, Isomaki P. Cytokine-induced STAT1 activation is increased in patients with primary Sjogren’s syndrome. Clin Immunol (2016) 165:60–7. doi:10.1016/j.clim.2016.03.010
233. Linsley PS, Nadler SG. The clinical utility of inhibiting CD28-mediated costimulation. Immunol Rev (2009) 229:307–21. doi:10.1111/j.1600-065X.2009.00780.x
234. Patakas A, Ji RR, Weir W, Connolly SE, Benson RA, Nadler SG, et al. Abatacept inhibition of T cell priming in mice by induction of a unique transcriptional profile that reduces their ability to activate antigen-presenting cells. Arthritis Rheumatol (2016) 68:627–38. doi:10.1002/art.39470
235. Verstappen GM, Meiners PM, Corneth OBJ, Visser A, Arends S, Abdulahad WH, et al. Attenuation of follicular helper T cell-dependent B cell hyperactivity by abatacept treatment in primary Sjogren’s syndrome. Arthritis Rheumatol (2017) 69:1850–61. doi:10.1002/art.40165
236. Wang L, Zhang Y, He M. Clinical predictors for the prognosis of myasthenia gravis. BMC Neurol (2017) 17:77. doi:10.1186/s12883-017-0857-7
237. Luo C, Li Y, Liu W, Feng H, Wang H, Huang X, et al. Expansion of circulating counterparts of follicular helper T cells in patients with myasthenia gravis. J Neuroimmunol (2013) 256:55–61. doi:10.1016/j.jneuroim.2012.12.001
238. Le Coz C, Joublin A, Pasquali JL, Korganow AS, Dumortier H, Monneaux F. Circulating TFH subset distribution is strongly affected in lupus patients with an active disease. PLoS One (2013) 8:e75319. doi:10.1371/journal.pone.0075319
239. Romme Christensen J, Bornsen L, Ratzer R, Piehl F, Khademi M, Olsson T, et al. Systemic inflammation in progressive multiple sclerosis involves follicular T-helper, Th17- and activated B-cells and correlates with progression. PLoS One (2013) 8:e57820. doi:10.1371/journal.pone.0057820
240. Liu R, Wu Q, Su D, Che N, Chen H, Geng L, et al. A regulatory effect of IL-21 on T follicular helper-like cell and B cell in rheumatoid arthritis. Arthritis Res Ther (2012) 14:R255. doi:10.1186/ar4100
241. An LM, Li J, Ji LL, Li GT, Zhang ZL. [Detection of peripheral follicular helper T cells in rheumatoid arthritis]. Beijing Da Xue Xue Bao Yi Xue Ban (2016) 48:951–7. doi:10.3969/j.issn.1671-167X.2016.06.006
242. Rao DA, Gurish MF, Marshall JL, Slowikowski K, Fonseka CY, Liu Y, et al. Pathologically expanded peripheral T helper cell subset drives B cells in rheumatoid arthritis. Nature (2017) 542:110–4. doi:10.1038/nature20810
243. Moschovakis GL, Bubke A, Friedrichsen M, Falk CS, Feederle R, Förster R. T cell specific Cxcr5 deficiency prevents rheumatoid arthritis. Sci Rep (2017) 7:8933. doi:10.1038/s41598-017-08935-6
244. Kenefeck R, Wang CJ, Kapadi T, Wardzinski L, Attridge K, Clough LE, et al. Follicular helper T cell signature in type 1 diabetes. J Clin Invest (2015) 125:292–303. doi:10.1172/JCI76238
245. Xu X, Shi Y, Cai Y, Zhang Q, Yang F, Chen H, et al. Inhibition of increased circulating Tfh cell by anti-CD20 monoclonal antibody in patients with type 1 diabetes. PLoS One (2013) 8:e79858. doi:10.1371/journal.pone.0079858
246. Ferreira RC, Simons HZ, Thompson WS, Cutler AJ, Dopico XC, Smyth DJ, et al. IL-21 production by CD4(+) effector T cells and frequency of circulating follicular helper T cells are increased in type 1 diabetes patients. Diabetologia (2015) 58:781–90. doi:10.1007/s00125-015-3509-8
247. Viisanen T, Ihantola E-L, Näntö-Salonen K, Hyöty H, Nurminen N, Selvenius J, et al. Circulating CXCR5+PD-1+ICOS+ follicular T helper cells are increased close to the diagnosis of type 1 diabetes in children with multiple autoantibodies. Diabetes (2017) 66:437–47. doi:10.2337/db16-0714
248. Arif S, Leete P, Nguyen V, Marks K, Nor NM, Estorninho M, et al. Blood and islet phenotypes indicate immunological heterogeneity in type 1 diabetes. Diabetes (2014) 63:3835–45. doi:10.2337/db14-0365
249. Zhou J, Wang Y, He Y, Gao Y, Wan R, Cai M, et al. Non-obese type 2 diabetes patients present intestinal B cell dysregulations associated with hyperactive intestinal Tfh cells. Mol Immunol (2018) 97:27–32. doi:10.1016/j.molimm.2018.03.008
250. Gaddis DE, Padgett LE, Wu R, McSkimming C, Romines V, Taylor AM, et al. Apolipoprotein AI prevents regulatory to follicular helper T cell switching during atherosclerosis. Nat Commun (2018) 9:1095. doi:10.1038/s41467-018-03493-5
251. Clement M, Guedj K, Andreata F, Morvan M, Bey L, Khallou-Laschet J, et al. Control of the T follicular helper-germinal center B-cell axis by CD8(+) regulatory T cells limits atherosclerosis and tertiary lymphoid organ development. Circulation (2015) 131:560–70. doi:10.1161/CIRCULATIONAHA.114.010988
252. Kamekura R, Shigehara K, Miyajima S, Jitsukawa S, Kawata K, Yamashita K, et al. Alteration of circulating type 2 follicular helper T cells and regulatory B cells underlies the comorbid association of allergic rhinitis with bronchial asthma. Clin Immunol (2015) 158:204–11. doi:10.1016/j.clim.2015.02.016
253. Jin J, Kobayashi T, Bachman KA, Kita H. T follicular helper (Tfh) cells are activated by natural exposure to pollens during the Ragweed Hay fever season. J Allergy Clin Immunol (2018) 141:AB176. doi:10.1016/j.jaci.2017.12.561
254. Dolence JJ, Kobayashi T, Iijima K, Krempski J, Drake LY, Dent AL, et al. Airway exposure initiates peanut allergy by involving the IL-1 pathway and T follicular helper cells in mice. J Allergy Clin Immunol (2017). doi:10.1016/j.jaci.2017.11.020
255. Akdis CA, Akdis M, Bieber T, Bindslev-Jensen C, Boguniewicz M, Eigenmann P, et al. Diagnosis and treatment of atopic dermatitis in children and adults: European Academy of Allergology and Clinical Immunology/American Academy of Allergy, Asthma and Immunology/PRACTALL Consensus Report. J Allergy Clin Immunol (2006) 118:152–69. doi:10.1016/j.jaci.2006.03.045
256. Eyerich K, Novak N. Immunology of atopic eczema: overcoming the Th1/Th2 paradigm. Allergy (2013) 68:974–82. doi:10.1111/all.12184
257. Werfel T, Allam JP, Biedermann T, Eyerich K, Gilles S, Guttman-Yassky E, et al. Cellular and molecular immunologic mechanisms in patients with atopic dermatitis. J Allergy Clin Immunol (2016) 138:336–49. doi:10.1016/j.jaci.2016.06.010
258. Eyerich K, Huss-Marp J, Darsow U, Wollenberg A, Foerster S, Ring J, et al. Pollen grains induce a rapid and biphasic eczematous immune response in atopic eczema patients. Int Arch Allergy Immunol (2008) 145:213–23. doi:10.1159/000109290
259. Szabó K, Gáspár K, Dajnoki Z, Papp G, Fábos B, Szegedi A, et al. Expansion of circulating follicular T helper cells associates with disease severity in childhood atopic dermatitis. Immunol Lett (2017) 189:101–8. doi:10.1016/j.imlet.2017.04.010
260. Czarnowicki T, Esaki H, Gonzalez J, Renert-Yuval Y, Brunner P, Oliva M, et al. Alterations in B-cell subsets in pediatric patients with early atopic dermatitis. J Allergy Clin Immunol (2017) 140:134–44.e9. doi:10.1016/j.jaci.2016.09.060
261. Ballesteros-Tato A, Randall TD, Lund FE, Spolski R, Leonard WJ, León B. T follicular helper cell plasticity shapes pathogenic T helper 2 cell-mediated immunity to inhaled house dust mite. Immunity (2016) 44:259–73. doi:10.1016/j.immuni.2015.11.017
262. Ma CS, Nichols KE, Tangye SG. Regulation of cellular and humoral immune responses by the SLAM and SAP families of molecules. Annu Rev Immunol (2007) 25:337–79. doi:10.1146/annurev.immunol.25.022106.141651
263. Ma CS, Hare NJ, Nichols KE, Dupré L, Andolfi G, Roncarolo M-G, et al. Tangye, Impaired humoral immunity in X-linked lymphoproliferative disease is associated with defective IL-10 production by CD4(+) T cells. J Clin Invest (2005) 115:1049–59. doi:10.1172/JCI200523139
264. Schmitt N, Bustamante J, Bourdery L, Bentebibel SE, Boisson-Dupuis S, Hamlin F, et al. IL-12 receptor beta1 deficiency alters in vivo T follicular helper cell response in humans. Blood (2013) 121:3375–85. doi:10.1182/blood-2012-08-448902
265. Lindqvist M, van Lunzen J, Soghoian DZ, Kuhl BD, Ranasinghe S, Kranias G, et al. Expansion of HIV-specific T follicular helper cells in chronic HIV infection. J Clin Invest (2012) 122:3271–80. doi:10.1172/JCI64314
266. Petrovas C, Yamamoto T, Gerner MY, Boswell KL, Wloka K, Smith EC, et al. CD4 T follicular helper cell dynamics during SIV infection. J Clin Invest (2012) 122:3281–94. doi:10.1172/JCI63039
267. Perreau M, Savoye AL, De Crignis E, Corpataux JM, Cubas R, Haddad EK, et al. Follicular helper T cells serve as the major CD4 T cell compartment for HIV-1 infection, replication, and production. J Exp Med (2013) 210:143–56. doi:10.1084/jem.20121932
268. Xu Y, Weatherall C, Bailey M, Alcantara S, De Rose R, Estaquier J, et al. Simian immunodeficiency virus infects follicular helper CD4 T cells in lymphoid tissues during pathogenic infection of pigtail macaques. J Virol (2013) 87:3760–73. doi:10.1128/JVI.02497-12
269. Xu Y, Phetsouphanh C, Suzuki K, Aggrawal A, Graff-Dubois S, Roche M, et al. HIV-1 and SIV predominantly use CCR5 expressed on a precursor population to establish infection in T follicular helper cells. Front Immunol (2017) 8:376. doi:10.3389/fimmu.2017.00376
270. Hufert FT, van Lunzen J, Janossy G, Bertram S, Schmitz J, Haller O, et al. Germinal centre CD4+ T cells are an important site of HIV replication in vivo. AIDS (1997) 11:849–57. doi:10.1097/00002030-199707000-00003
271. Kohler SL, Pham MN, Folkvord JM, Arends T, Miller SM, Miles B, et al. Germinal center T follicular helper cells are highly permissive to HIV-1 and alter their phenotype during virus replication. J Immunol (2016) 196:2711–22. doi:10.4049/jimmunol.1502174
272. Connick E, Mattila T, Folkvord JM, Schlichtemeier R, Meditz AL, Ray MG, et al. CTL fail to accumulate at sites of HIV-1 replication in lymphoid tissue. J Immunol (2007) 178:6975–83. doi:10.4049/jimmunol.178.11.6975
273. Hong JJ, Amancha PK, Rogers K, Ansari AA, Villinger F. Spatial alterations between CD4(+) T follicular helper, B, and CD8(+) T cells during simian immunodeficiency virus infection: T/B cell homeostasis, activation, and potential mechanism for viral escape. J Immunol (2012) 188:3247–56. doi:10.4049/jimmunol.1103138
274. Lu J, Lv Y, Lv Z, Xu Y, Huang Y, Cui M, et al. Expansion of circulating T follicular helper cells is associated with disease progression in HIV-infected individuals. J Infect Public Health (2018). doi:10.1016/j.jiph.2018.01.005
275. De Milito A, Nilsson A, Titanji K, Thorstensson R, Reizenstein E, Narita M, et al. Mechanisms of hypergammaglobulinemia and impaired antigen-specific humoral immunity in HIV-1 infection. Blood (2004) 103:2180–6. doi:10.1182/blood-2003-07-2375
276. Hart M, Steel A, Clark SA, Moyle G, Nelson M, Henderson DC, et al. Loss of discrete memory B cell subsets is associated with impaired immunization responses in HIV-1 infection and may be a risk factor for invasive pneumococcal disease. J Immunol (2007) 178:8212–20. doi:10.4049/jimmunol.178.12.8212
277. Cubas RA, Mudd JC, Savoye A-L, Perreau M, van Grevenynghe J, Metcalf T, et al. Inadequate T follicular cell help impairs B cell immunity during HIV infection. Nat Med (2013) 19:494–9. doi:10.1038/nm.3109
278. Moir S, Fauci AS. B cells in HIV infection and disease. Nat Rev Immunol (2009) 9:235–45. doi:10.1038/nri2524
279. Fahey LM, Wilson EB, Elsaesser H, Fistonich CD, McGavern DB, Brooks DG. Viral persistence redirects CD4 T cell differentiation toward T follicular helper cells. J Exp Med (2011) 208:987–99. doi:10.1084/jem.20101773
280. Harker JA, Lewis GM, Mack L, Zuniga EI. Late interleukin-6 escalates T follicular helper cell responses and controls a chronic viral infection. Science (2011) 334:825–9. doi:10.1126/science.1208421
281. Herati RS, Muselman A, Vella L, Bengsch B, Parkhouse K, Del Alcazar D, et al. Successive annual influenza vaccination induces a recurrent oligoclonotypic memory response in circulating T follicular helper cells. Sci Immunol (2017) 2:eaag2152. doi:10.1126/sciimmunol.aag2152
282. Koutsakos M, Wheatley AK, Loh L, Clemens EB, Sant S, Nussing S, et al. Circulating TFH cells, serological memory, and tissue compartmentalization shape human influenza-specific B cell immunity. Sci Transl Med (2018) 10: eaan8405. doi:10.1126/scitranslmed.aan8405
283. Wang R, Xie R, Song Z. Circulating regulatory Tfh cells are enriched in patients with chronic hepatitis B infection and induce the differentiation of regulatory B cells. Exp Cell Res (2018) 365:171–6. doi:10.1016/j.yexcr.2018.02.031
284. Rodrigues V, Laforge M, Campillo-Gimenez L, Soundaramourty C, Correia-de-Oliveira A, Dinis-Oliveira RJ, et al. Abortive T follicular helper development is associated with a defective humoral response in Leishmania infantum-infected macaques. PLoS Pathog (2014) 10:e1004096. doi:10.1371/journal.ppat.1004096
285. Haben I, Hartmann W, Breloer M. Nematode-induced interference with vaccination efficacy targets follicular T helper cell induction and is preserved after termination of infection. PLoS Negl Trop Dis (2014) 8:e3170. doi:10.1371/journal.pntd.0003170
286. Perez-Mazliah D, Nguyen MP, Hosking C, McLaughlin S, Lewis MD, Tumwine I, et al. Follicular helper T cells are essential for the elimination of Plasmodium infection. EBioMedicine (2017) 24:216–30. doi:10.1016/j.ebiom.2017.08.030
287. de Leval L, Rickman DS, Thielen C, Reynies A, Huang YL, Delsol G, et al. The gene expression profile of nodal peripheral T-cell lymphoma demonstrates a molecular link between angioimmunoblastic T-cell lymphoma (AITL) and follicular helper T (TFH) cells. Blood (2007) 109:4952–63. doi:10.1182/blood-2006-10-055145
288. Grogg KL, Attygalle AD, Macon WR, Remstein ED, Kurtin PJ, Dogan A. Expression of CXCL13, a chemokine highly upregulated in germinal center T-helper cells, distinguishes angioimmunoblastic T-cell lymphoma from peripheral T-cell lymphoma, unspecified. Mod Pathol (2006) 19:1101–7. doi:10.1038/modpathol.3800625
289. Vinuesa CG, Tangye SG, Moser B, Mackay CR. Follicular B helper T cells in antibody responses and autoimmunity. Nat Rev Immunol (2005) 5:853–65. doi:10.1038/nri1714
290. Cortes JR, Ambesi-Impiombato A, Couronné L, Quinn SA, Kim CS, da Silva Almeida AC, et al. RHOA G17V induces T follicular helper cell specification and promotes lymphomagenesis. Cancer Cell (2018) 33:259–73.e7. doi:10.1016/j.ccell.2018.01.001
291. Ochando J, Braza MS. T follicular helper cells: a potential therapeutic target in follicular lymphoma. Oncotarget (2017) 8:112116–31. doi:10.18632/oncotarget.22788
292. Gu-Trantien C, Loi S, Garaud S, Equeter C, Libin M, de Wind A, et al. CD4+ follicular helper T cell infiltration predicts breast cancer survival. J Clin Invest (2013) 123:2873–92. doi:10.1172/JCI67428
293. Bindea G, Mlecnik B, Tosolini M, Kirilovsky A, Waldner M, Obenauf AC, et al. Spatiotemporal dynamics of intratumoral immune cells reveal the immune landscape in human cancer. Immunity (2013) 39:782–95. doi:10.1016/j.immuni.2013.10.003
294. Wang Z, Wang Z, Diao Y, Qian X, Zhu N, Dong W. Circulating follicular helper T cells in Crohn’s disease (CD) and CD-associated colorectal cancer. Tumour Biol (2014) 35:9355–9. doi:10.1007/s13277-014-2208-2
295. Cha Z, Zang Y, Guo H, Rechlic JR, Olasnova LM, Gu H, et al. Association of peripheral CD4+ CXCR5+ T cells with chronic lymphocytic leukemia. Tumour Biol (2013) 34:3579–85. doi:10.1007/s13277-013-0937-2
296. Shi W, Li X, Cha Z, Sun S, Wang L, Jiao S, et al. Dysregulation of circulating follicular helper T cells in nonsmall cell lung cancer. DNA Cell Biol (2014) 33:355–60. doi:10.1089/dna.2013.2332
Keywords: T follicular helper cells, germinal center, transcription, autoimmunity, cancer
Citation: Qin L, Waseem TC, Sahoo A, Bieerkehazhi S, Zhou H, Galkina EV and Nurieva R (2018) Insights Into the Molecular Mechanisms of T Follicular Helper-Mediated Immunity and Pathology. Front. Immunol. 9:1884. doi: 10.3389/fimmu.2018.01884
Received: 15 June 2018; Accepted: 31 July 2018;
Published: 15 August 2018
Edited by:
Maria Pia Cicalese, San Raffaele Scientific Institute (IRCCS), ItalyReviewed by:
Jinfang Zhu, National Institute of Allergy and Infectious Diseases (NIAID), United StatesJohn R. Lukens, University of Virginia, United States
Copyright: © 2018 Qin, Waseem, Sahoo, Bieerkehazhi, Zhou, Galkina and Nurieva. This is an open-access article distributed under the terms of the Creative Commons Attribution License (CC BY). The use, distribution or reproduction in other forums is permitted, provided the original author(s) and the copyright owner(s) are credited and that the original publication in this journal is cited, in accordance with accepted academic practice. No use, distribution or reproduction is permitted which does not comply with these terms.
*Correspondence: Roza Nurieva, rnurieva@mdanderson.org
†These authors have contributed equally to this work.