- 1International Genomics Research Center (IGRC), Institute of Immunology, Jiangsu University, Zhenjiang, China
- 2Department of Microbiology and Immunology, Faculty of Medicine, Al-Azhar University, Assiut, Egypt
- 3Department of Neuroimmunology, Brigham and Women’s Hospital, Harvard Medical School, Boston, MA, United States
- 4Department of Clinical Laboratory Diagnostics, School of Medicine, Jiangsu University, Zhenjiang, China
- 5Division of Pharmaceutical Microbiology, Department of Pharmaceutics and Industrial Pharmacy, College of Pharmacy, Taif University, Taif, Saudi Arabia
Bronchial asthma is one of the most chronic pulmonary diseases and major public health problems. In general, asthma prevails in developed countries than developing countries, and its prevalence is increasing in the latter. For instance, the hygiene hypothesis demonstrated that this phenomenon resulted from higher household hygienic standards that decreased the chances of infections, which would subsequently increase the occurrence of allergy. In this review, we attempted to integrate our knowledge with the hygiene hypothesis into beneficial preventive approaches for allergic asthma. Therefore, we highlighted the studies that investigated the correlation between allergic asthma and the two different types of infections that induce the two major antagonizing arms of T cells. This elucidation reflects the association between various types of natural infections and the immune system, which is predicted to support the main objective of the current research on investigating of the benefits of natural infections, regardless their immune pathways for the prevention of allergic asthma. We demonstrated that natural infection with Mycobacterium tuberculosis (Mtb) prevents the development of allergic asthma, thus Bacille Calmette-Guérin (BCG) vaccine is suggested at early age to mediate the same prevention particularly with increasing its efficiency through genetic engineering-based modifications. Likewise, natural helminth infections might inhabit the allergic asthma development. Therefore, helminth-derived proteins at early age are good candidates for designing vaccines for allergic asthma and it requires further investigation. Finally, we recommend imitation of natural infections as a general strategy for preventing allergic asthma that increased dramatically over the past decades.
Background
Bronchial asthma is regarded as the most chronic pulmonary disease and a major public health problem, affecting more than 350 million people worldwide with a high mortality rate in severe cases. Also, it is likely to afflict an additional 100 million by 2025. Among the various forms of asthma (minor forms due to air pollution, exercise, aspirin and cold), allergic or atopic asthma is the most prevalent (1, 2). T helper 2 (Th2) cells are the major effector cells in the pathogenesis of allergic asthma. Upon allergic exposure and via their signature cytokines, they stimulate Eosinophils and IgE-producing B cells with subsequent mast cells degranulation, resulting in the characteristic airway narrowing and airway hyperreactivity (AHR) (2). Generally, asthma is more prevalent in developed countries (range between 8.5% to 32%) than in developing countries (range between 4.1% to 4.2%) and its prevalence is increasing in the developing countries as they become more westernized (1, 3). The first study investigated this phenomenon was in 1976, when the authors found that allergic diseases increase more in urban areas than in rural areas, which resulted from less exposure to viruses, bacteria and helminths in urban areas (4). In 1989, a study reported similar findings, and concluded that higher household hygienic standards lead to decreased chances of infections, which may subsequently increase the occurrence of allergy, and it has been referred as the hygiene hypothesis (5). This hypothesis suggest that the removal of the regulatory effects of infectious microorganisms from populations tended to lead to an imbalance in the immune system (6), which acts through two patterns of acquired immune response: Th1 and Th2 immune responses (7). In addition, over the past 20–30 years, it has become increasingly clear that, in Western countries, a strong correlation exists between improved sanitation and hygiene and a dramatic increase in atopic disorders (8). Moreover, this hypothesis was supported by the global rise in allergy associated with a decreased infection burden (1, 6, 9–11). This hypothesis has been extended to include different autoimmune diseases, such as type I diabetes mellitus, inflammatory bowel disease, and multiple sclerosis (8, 11, 12). Moreover, there is another hypothesis derived from the hygiene hypothesis and called old friends that implies the relationship between various types of infections and chronic inflammatory diseases (13). Furthermore, the biodiversity hypothesis, another hypothesis derived from the hygiene hypothesis, demonstrates that reduced natural environmental biodiversity adversely affects human commensal microbiota, which is consequently associated with higher prevalence of atopy (14). In general, these illustrations indicate the significance of the hygiene hypothesis to gain further knowledge concerning allergic asthma.
The main attempt of the current study was to integrate existing knowledge with the hygiene hypothesis to attain beneficial preventive approaches for allergic asthma. Therefore, we highlighted the studies that illustrated the correlation between allergic asthma and two different types of infections (mycobacteria and helminths) that induce the two major antagonizing arms of T cells (Th1 and Th2). This elucidation reflects the relationship between various types of natural infections and the immune system, which consequently supports our main attempt to investigate the potential advantages of various natural infections and the practical applications of these benefits for developing efficient approaches to control allergic asthma.
Mycobacterium tuberculosis and Allergic Asthma
Tuberculosis (TB) is a highly infectious granulomatous lung disease induced by Mtb, affects 10 million new cases annually with 1.5 million associated deaths predominantly in developing countries, representing the most lethal pathogenic organism worldwide (15). Mtb is a potent Th1 response inducer. After Mtb reaches the respiratory tract, it is deposited within the alveoli, to which the immune system responds by releasing pro-inflammatory cytokines that recruit monocytes and macrophages. Mtb begins to multiply within alveolar macrophages secreting interleukin (IL)-12, the latter activates IFN-γ-secreted Th1, and thus activates macrophages and enhances its intracellular killing of phagocytosed Mtb. This immune response can control the infection in 90% of cases. However, Mtb are not completely eradicated and their intracellular persistence inside macrophages induces Th1 hypersensitivity, that stimulate the formation of chronic granuloma, which is a structure consisting of a central zone of multinucleated giant cells containing the organisms, in addition to the peripheral zone of fibroblasts, lymphocytes and monocytes to limit bacterial spread (15). Th1-directed response is mainly induced by certain antigenic structures of cell wall of Mtb, such as the mycolyl arabinogalactan peptidoglycan complex and their associated lipoarabinomannan. These structures give Mtb their acidic stability and prolong their survival inside macrophages (16). Notably, T-regulatory cells (Tregs) are involved in the immune response to Mtb via potentially suppressing the pathogenic hyperactivation of Th1 cells (17, 18).
In an asthmatic mice model, Mtb infection alleviates allergic inflammation and reduces Th2 cytokines. These effects were suggested to be mediated through the conversion of the allergen-specific Th2 into Th1 cells, which was supported by increased IFN-γ secretion of allergen-specific T cells, and not by Mtb-stimulated expanded T cells. In addition, this switch was induced by IL-12, which is the classical cytokine associated with Mtb infection (15, 19). These findings indicate an inverse correlation between murine experimental allergic asthma and Mtb infection. Concurrently, for human allergic asthma, an international ecological study was conducted by the International Study of Asthma and Allergies in Childhood (ISAAC), using data from 23 countries in Europe, USA, Canada, Australia and New Zealand, and found that childhood Mtb infection may protect against the subsequent development of allergic asthma (20). Another ecological study included world health organization and ISAAC program data for standardized asthma symptoms and TB prevalence collected from Asian, central and south American, and African countries. This study concluded a preventive effect of Mtb infection against the development of asthma through the induction of strong Th1 immune response (21). Moreover, the protective role of Mtb was concluded by other studies (22–24). Interestingly, as reported in mice, this effect might be induced by the switching of allergen specific Th2 into Th1 cells in human (23). Furthermore, there was an inverse association between tuberculin test positivity and the incidence of allergic asthma (25, 26). In general, early childhood Mtb infection can prevent the subsequent development of allergic asthma.
BCG and Allergic Asthma
The protective role of Mtb infection against allergic asthma indicates the elucidation of the potential role of the TB vaccine in preventing asthma. The only licensed vaccine for TB is the BCG that is named after Albert Calmette and Camille Guérin who in 1908 attenuated the living virulent mycobacterium bovis (M. bovis) through continuous passages (231 passages) on culture media that lost the virulence and maintained the antigenicity of the organism. This vaccine was made throughout 13 years at the Institute Pasteur in Lille, France, and was used for the first time in humans in 1921 for TB prevention, with more than 100 million children vaccinated annually worldwide (27, 28). However, the protective efficacy of BCG is variable, ranging from 0% to 80% in different countries. Nonetheless, the exact reason for this variation remains unclear (27, 29, 30).
Interestingly, BCG is not a single strain, as the original strain in Pasteur institute generated several offspring strains via continuous passages in the countries that received the vaccine that reached 60 countries by 1927. For example, the Danish strain originated in 1931 in the name of 423rd transfer, whereas the Glaxo strain was derived from the 1077th transfer of the Danish strain. Many other strains were used, for instance; Pasteur, Tokyo, Prague, Russian, Moreau, …etc. (30, 31). The mechanism of the attenuation process of M. bovis resulting from the serial passages is not well interpreted. Nevertheless, it may involve deletion of a chromosomal region, called region of difference 1 (RD1), which is found only in virulent mycobacteria and absent in BCG, containing the encoding genes for ESAT-6 and CFP-10 and their secretion apparatus which are two fundamental virulence factors for M. bovis. Furthermore, other RD regions such as RD2, RD3 and RD14 to RD16 may be omitted. Moreover, single nucleotide polymorphisms are also involved in chromosomal changes (27, 28). Therefore, the genetic variability between the various BCG vaccine strains may explain the variable protection. Also, they induced different degrees of immune cell responses in vitro. However, there is no clear evidence that one strain produces more protection against pulmonary TB than the remaining strains (30). Another variable for the efficacy of BCG is the prior exposure to environmental mycobacteria, which are distributed differently among countries. These mycobacteria affect the efficacy of BCG through its pre-existing immune response that may block BCG replication, hence called the blocking hypothesis. In addition, it may induce a certain level of protection against TB, which masks any protective effect of subsequent BCG, thus called the masking hypothesis (28, 32).
BCG is considered very safe and the following reactions following intradermal (i.d.) administration are mild in the form of erythema and papule or ulceration that develops into scar, and generally do not require any treatment (33). BCG stimulates the immune response via binding its antigenic structures, such as lipoarabinomannan, phosphatidylinositol mannoside and trehalose 6,60-dimycolate, to toll-like receptor (TLR)2 and TLR4 on innate immune cells as macrophages that secrete IL-12 to induce Th1 cells polarization. The latter cells secrete IFN-γ that activates macrophages in a positive feedback loop (34, 35). Consequently, BCG is a potent inducer of Th1 cells which is, also, converted into memory cells to maintain life-long protection against Mtb infection. However, BCG, also, stimulates polarization of Tregs, as the response to Mtb infection, to prevent an exaggerated Th1 response (34). Due to the immunostimulatory effects of BCG, it is used as an immunotherapy for bladder cancer, multiple sclerosis and type 1 diabetes mellitus, and it also used as an adjuvant (35).
In experimental murine allergic asthma, we divided the potential effects of BCG into three categories (Table 1): 1) preventive, 2) preventive and/or therapeutic, and 3) therapeutic, according to three time sets of vaccine administration: 1) before allergen sensitization (36–43), 2) with allergen sensitization (44–46), and 3) with/after aerosol allergen challenge (47, 48), respectively. In several studies, administration of BCG after birth or at an early age prevents the subsequent development of murine allergic asthma regardless of strain, route and number of doses (36–46). In addition, the BCG protective response is supported by the ability of BCG adoptive transferred stimulated dendritic cells (DCs) with different subsets to suppress the established allergic inflammation in murine asthma (37, 49–51). Moreover, the BCG administration after the establishment of allergic asthma still has the potential to suppress allergic inflammation and AHR (47, 48), indicating that BCG is not only a preventive agent, but also a therapeutic candidate for allergic asthma. Correspondingly, for human allergic asthma (Table 2), several epidemiological studies with various study designs concluded that early BCG vaccination significantly decreases the subsequent development of asthma in different countries, such as England, France, Turkey, Germany and Spain in Europe, and Japan, Thailand and India in Asia (25, 52–57). Moreover, this conclusion was supported by the inverse correlation between BCG scar dimeter and atopic asthma in Brazil and Korea (65, 66). Interestingly, BCG scar diameter is a significant reflection of the immune response to BCG as a Th1 enhanced response with the subsequent increase of IFN-γ (66). Furthermore, BCG administration to asthmatic patients demonstrated therapeutic efficiency in the form of improved pulmonary functions and reduced medications. This occurs via the attenuation of Th2 response (67, 68), which proved the inhibitory effects of BCG even after developing atopic asthma.
The protective effect of BCG against allergic asthma can be mediated through two potential pathways (Figure 1A). First, promoted Th1 cells polarization with the subsequent increase in Th1/Th2 homeostasis along with their signature cytokines as IFN-γ/IL-5 ratio. This promotion could be induced by either polarization of naïve CD4+ to Th1 cells or switching of allergen specific Th2 into Th1 cells (38, 41–43). Another pathway is the upregulation of Tregs differentiation with the subsequent increase of IL-10, which is induced by interaction with stimulated TLR2, TLR4 and PD-1 that was expressed in DCs (37, 39, 40, 46). Interestingly, BCG can stimulate differentiation of naïve DCs into different subsets as CD8α+ and CD8α- DCs that can induce both Tregs and Th1 cells through secretion of IL-10 and IL-12, respectively (37, 49–51). These two pathways provide protection in the form of suppression of Th2 cytokines secretion, eosinophilia, allergic inflammation, allergen specific-IgE and AHR (36–48). Moreover, BCG protected against asthma through reduced IL-17 production, the signature cytokine of Th17, that played a critical role in inducing neutrophilia and airway inflammation and correlated with AHR and disease severity (45, 69, 70). However, the complex action of Th17 in eosinophilic airway inflammation requires further elucidations.
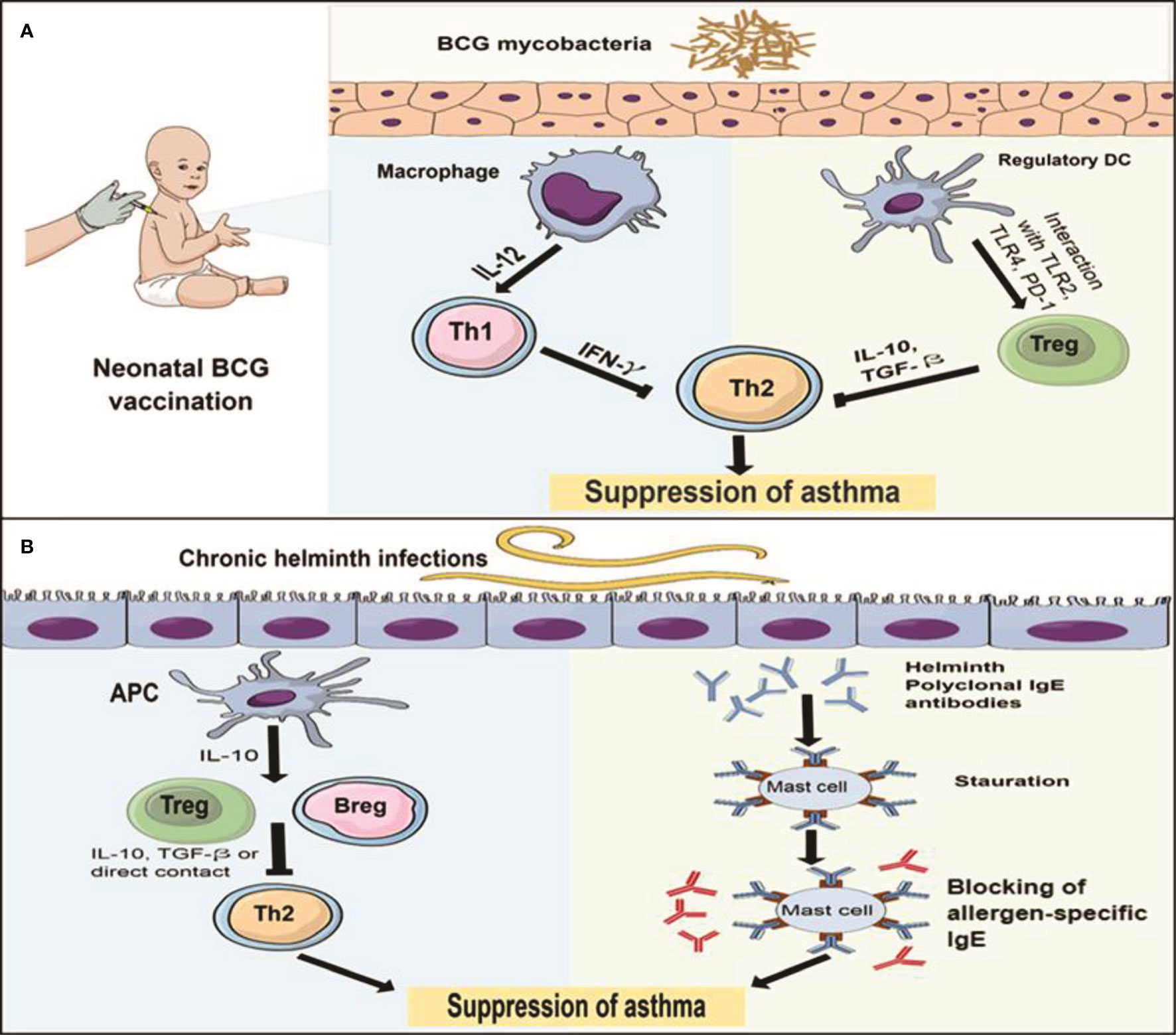
Figure 1 The immune mechanisms of BCG and helminths protect against allergic asthma (A) The protective effect of neonatal BCG is mediated through two pathways: the first pathway (left) is induction of Th1 cells polarization by IL-12 secreting macrophages, the resultant IFN-γ inhibits Th2 response with subsequent suppression of asthma. Another pathway (right) is the upregulation of Tregs polarization through interaction with TLR2, TLR4 and PD-1 expressed on DCs, the resultant IL-10 and TGF-β reduce TH2 response and suppress asthma. (B) The protective effect of chronic helminth infection is mediated through 2 pathways: the first pathway (left) is the induction of T and Bregs differentiation by IL-10 secreting antigen presenting cells (APCs). Then, Th2 response is inhabited by IL-10 and TGF-β or the direct contact between Tregs and Th2 cells, which leads to suppressing asthma. The second pathway (right) is the saturation hypothesis in which helminth infections induce polyclonal IgE (blue) that binds to and saturate high affinity FcϵR on mast cells, preventing binding of allergen-specific IgE (red) and subsequent blocking of mast cell degranulation.
On the contrary, several epidemiological studies found that early BCG vaccination did not decrease the risk of subsequent development of atopic asthma, these studies were performed in European countries such as Germany, Sweden, Netherland and a North American country; Canada (Table 2) (58–64). However, there weren’t adequate animal research that supported this claim unlike the BCG protective effects that were reinforced by several animal studies as mentioned previously. The discrepancy between the preventive role of BCG vaccination in the development of human asthma reported in different countries is attributed to some factors. First, BCG strains contrasted with different immunogenicity (28); however, as was shown in Table 2, the lack of information about the specific strains used in six of the 14 studies that supported (53–55) or did not support (59, 61, 62) the negative association between asthma and BCG, resulted in a severe difficulty to interpret the role of strains.
Nonetheless, four of seven studies (Table 2) supported the protective role of BCG against asthma and reported strains, such as Pasteur (52), Tokyo 172 (25) and Copenhagen 1331 strains (56, 57), while the latter induced poor protective effects. Interestingly, for the seven studies that did not support the protective role of BCG (Table 2), Copenhagen strain 1331 was utilized in three studies in Germany (60), the Netherlands (63) and Sweden (58). However, the other three studies, which did not mention the strain were also performed in the same three countries (59, 61, 62), with some potential to use the Copenhagen strain. The effect of this particular strain has not been investigated through animal research regarding its effect on murine allergic asthma unlike other strains shown in Table 1. Thus, among the different BCG strains, Copenhagen strain may not induce protection against allergic asthma, and this requires further investigations. In addition, the seventh non-supportive study used the Pasteur strain and was conducted in Canada (64). However, the study did not exclude the subjects who received the vaccine after the neonatal period. Consequently, this leads to the second point of the controversy, which is that late administration of BCG during the neonatal period may increase the chances of prior exposure to environmental mycobacteria, that might decrease the efficacy of BCG as previously mentioned. In same context, another study was implemented in Sweden that did not exclude receivers (59).
Atopic hereditary can potentially interfere with the effects of BCG, particularly in the seven studies that did not support the protective function. Four of these seven studies did not detect a significant difference between vaccinated and non-vaccinated or asthmatic and non-asthmatic subjects regarding family history of atopy (58, 60, 63, 64). Three other studies did not apply this comparison, which weakens the potential interference of the genetic background with the BCG effects in those studies. In contrast, studies demonstrating the protective effects of BCG on allergic asthma in developed countries such as England and France (53, 54), which consequently contradicts the assumption that the preventive effects of BCG against asthma are mediated by the natural infection with Mtb in developing countries, where the incidence of tuberculosis is high, and not by the BCG itself (58). However, the preventive role of Mtb infection against the development of asthma, which was previously mentioned, is not controversial as BCG. Interestingly, Mtb infection generates four times more IFN-γ than BCG (45). Despite the widespread use of BCG as a TB vaccine, TB remains the leading cause of death from an infectious agent worldwide (71). Therefore, imitating natural Mtb infection through enhancing the efficacy of BCG and its administration at the neonatal period may induce protection against asthma and end this controversy.
In general, the conventional method to increase the efficacy of vaccines is the booster dosing (72), since repeated exposure to both antigen and polarizing cytokine is required for an effective immune response (73). In this regard, the BCG efficacy ranges between 0-80% and wanes over time (29), and it is classified as moderately effective vaccine (74). In addition, repeated intradermal injection of live attenuated vaccine is not acceptable due to the associated adverse effects. Thus, intranasal administration of recombinant immunodominant proteins is a better option (75). Antigen 85A (Ag85A) is a major immunodominant secretory protein in both Mtb and BCG (76), and can be incorporated with Mtb32 protein (an immunodominant mycobacterial protein) into an adenoviral vector, as its intranasal administration to asthmatic mice significantly suppresses allergic airway inflammation compared to BCG effect. This suppression occurs via increased IFN-γ and IL-10 and decreased IL-4, IL-5, IL-13 and IL-33 (77). In addition, antigen 85B (Ag85B), which is another major secretory mycobacterial protein, was recombined with pMG plasmid and administrated nasally to asthmatic mice inducing a protective effect by increasing IFN-γ/IL-4 ratio (75). Moreover, general Mtb secretory proteins, particularly Ag85A, have showed promising results for TB prevention in animals and humans and may replace the primary vaccine in the near future (76, 78, 79). It is expected that developed countries will replace the primary BCG vaccine with the recombinant protein vaccine because they cannot see any necessity for injecting live attenuated BCG concurrent with their low TB prevalence (60). However, there is a growing need for further investigation of intranasal use of Ag85A and Ag85B in humans. Additionally, intranasal administration of recombinant BCG producing genetically detoxified S1 subunit of pertussis toxin can prevent murine allergic asthma by increasing IL-12 and IFN-γ (80). Another method to increase BCG efficacy is the combination of BCG with CpG oligodeoxynucleotide, which is a synthetic TLR9 agonist that stimulates both macrophages (classical type) and DCs with subsequent induction of Th1 response. This combination inhibits allergic inflammation in murine asthma compared to BCG alone and may be a protective candidate effective for allergic asthma (34). In addition, it induces more protection against murine TB compared with BCG alone (81). Likewise, recombinant BCG strains producing IL-12 or IL-18 can further mediate protection against murine allergic asthma by switching from a Th2 response to a Th1 response (82, 83). All these genetic engineering-based modifications of BCG can improve its efficacy and provide enhanced protection against murine allergic asthma when administrated at early age. Thus, their use as boosters or as primary candidate vaccines for the prevention of human atopic asthma is promising for preventing one of the most prevalent chronic disease in the world and must be considered in future research.
Helminths and Allergic Asthma
Helminths have infected human for thousands of years (84). At present, it is estimated that approximately 30% of the world is infected with at least one species of helminths particularly in poor and less developed communities. There are several types of helminths; the most common worldwide are intestinal nematodes including Ascaris lumbricoides (A. lumbricoides), Trichuris trichiura (T. trichiura) and hookworm and schistosomes (9). Basically, helminthic infection activates Th2 cells that secrete IL-4, IL-5 and IL-13, then IL-4 stimulates B cells to produce helminth-specific Ig-E, which opsonize the helminths and promote binding to IL-5 activated eosinophils via FcϵR. Eosinophils release their granular contents including the main primary and cationic proteins which destroy the helminths, besides binding to FcϵR on mast cells inducing inflammatory response through the release of vasoactive amines, the production of inflammatory cytokines e.g. tumor necrosis factor (TNF) and lipid mediators that contribute to anti-helminths responses. In addition, IL-4 and IL-13 induce mucus secretion and peristalsis that promotes the expulsion of helminths from the mucosal organs (barrier immunity) (10, 85). On the contrary, helminth infection, also, induces T and B regulatory cells that suppress the immune response through the secretion of IL-10, and transforming growth factor (TGF)-β. This process results in a state of hypo-responsiveness which enables organisms to overcome host resistance, and allows chronic infections (10, 86). However, these regulatory mechanisms may protect the host from an excessive immune response against helminths, repair damage that occurred during migration and feeding of these helminths and enhance resistance to further colonization (9, 87).
In experimental murine allergic asthma (Table 3), early and chronic infection of different species of helminths e.g., Schistosoma mansoni (S. mansoni) (88–91), Schistosoma japonicum (S. japonicum) (92), Trichinella spiralis (T. spiralis) (93, 94), Heligosomoides polygyrus (H. polygyrus) (95, 96), Litomosoides sigmodontis (97), Nippostrongylus brasiliensis (N. brasiliensis) (99) and Strongyloides stercoralis (S. stercoralis) (98), can prevent disease progression. Also, administration of helminth eggs, particularly S. mansoni (90, 100), mediates the same effects. In addition, adoptive transfer of immune cell from infected animals such as S. mansoni-induced B regulatory cells (101, 102), S. japonicum-induced DCs (103) and H. polygyus-induced B cells (104), can protect against murine asthma. With regard to humans, in general, variable helminthic infections among different countries can reduce the risk of developing atopic diseases (105–112). Furthermore, the increased allergic reactivity following long term anti-helminths treatment, supports the hypothesis of the protective role conferred by helminths in atopic diseases (113, 114). Concurrently, there is an inverse correlation between human allergic asthma and helminth infections (Table 4) of various species such as S. mansoni (115–117), A. lumbricoides (24, 117–119), Necator americanus (N. americanus) (hookworm) (117–120), and Entrobius vermicularis (E. vermicularis) (pinworm) (121). This reverse association can be attributed to the protective effect against the development of allergic asthma.
Although the Th2 immune responses to helminths and allergic asthma seem to be identical, the helminths-induced responses have different characteristics (9, 10), which may constitute the protective mechanisms against atopic asthma. There are two hypotheses for these mechanisms in mice and human (Figure 1B). First, the regulatory network hypothesis (the most widely accepted), in which chronic helminth infections induce regulatory immune response in the form of T and B regulatory cells that suppress Th2 cell activation and subsequent allergic inflammation via the production of anti-inflammatory IL-10 and TGF-β (10, 86, 102, 132–134). The helminths induction of the adaptive regulatory (T and B) cells may be mediated by the initial interaction of the helminths with innate cells as DCs (103) and macrophages (132) to induce regulatory DCs and M2 macrophages, respectively. Then, the latter cells secrete IL-10 which induces T and B regulatory cells. In addition, these regulatory cells, by secreting IL-10 can inhibit DCs, resulting in an alleviation of allergen presentation by DCs and further inhibition of Th2 cells polarization (132, 134). Moreover, via IL-10 and TFG-β, they induce more M2 macrophages (2), which reflects regulatory feedback mechanisms between adaptive and innate cells. Since suppressive activities in some studies are continued with regulatory cells after IL-10 depletion, the regulatory function of T and B cells does not always depend on their signature cytokines. Nonetheless, direct interaction with other immune cells such as Th2 or innate cells may play a significant role in the suppressive functions (90, 104). Furthermore, helminth-induced inhibition can occur even with depletion of adaptive regulatory cells, through direct inhibition of DCs (100), and thus the helminth-induced regulation will find its way through either adaptive or innate systems. Interestingly, in allergic asthma, Tregs are dysfunctional with limited inhibitory activities and may exaggerate the pathogenesis of the disease (10, 135). Consequently, helminths-induced regulatory network to mediate the protective effects against atopic asthma is proposed. The second hypothesis is termed the saturation hypothesis and it has been proposed earlier than the first hypothesis, in which helminth infections induce polyclonal IgE that bind to and saturate high affinity FcϵR on mast cells and basophils, preventing binding of aeroallergen-specific IgE and block mast cell and basophils degranulation (Figure 1B). This is based on the findings that helminth-induced polyclonal IgE is significantly greater than asthma-induced IgE (11, 98, 132, 133, 136). In addition, helminths may induce specific IgG4 that can interact and compete with aeroallergen-specific IgE, which provides another illustration of the saturation hypothesis (136–138). In general, these two hypotheses provide an explanation of the protective mechanisms of helminth-induced immune response against atopic asthma, which also represent the fundamental differences between Th2 immune response for helminths and allergic asthma. Therefore, the helminth response is called the modified immune response type 2 (10, 137, 138). In addition, this protection is associated with chronic helminth infections, since acute infection may exacerbate the atopy (100, 132). Interestingly, the immune response to helminth infections has been hypothesized to be the primary objective of the evolution of type 2 immune response arm, not the allergy (139). Therefore, the immune response to helminths represents the normal side of type 2 arm (sensitivity), while the response to allergens, represents the pathogenic side (hypersensitivity). One simple explanation for helminth-induced suppression of allergy is that the type 2 immune response has a limited capacity to respond, that could be depleted by helminth immunity or allergy. In addition, through competition or splitting efforts, the anti-helminthic response will reduce the allergic reactions (137).
In contrast, based on human studies in different countries (Table 4), helminth infections, such as A. lumbricoides, Hookworm, T. trichiura, E. vermicularis, Toxocara canis (T. canis) and Ancylostoma duodenale, do not induce any protective effects against allergic asthma (122–131). Furthermore, A. lumbricoides (122, 126) and T. canis (129, 130) may increase the risk of developing asthma. Moreover, in asthmatics, experimental hookworm infection neither improve bronchial responsiveness nor other asthma measures (140). Conflicting results concerning the protective effect of helminth infections against human atopic asthma can be attributed to several factors. First, the variation of helminth species among studies (133). However, in the previously mentioned human studies, there was a controversy about A. lumbricoides (that showed the most conflicting results), hookworm, T. trichiura and E. vermicularis, whereas there was no conflict regarding the negative association between S. mansoni infection and atopic asthma in human. This may be related to helminth chronicle (the second factor of conflict) (133), and the associated granulomatous inflammation and fibrosis (141). In addition, S. mansoni is the most studied helminths in mice demonstrating a protective effect against asthma (88–91, 100, 102). Therefore, acute or light helminth infections do not protect against asthma and may exacerbate the allergic inflammation, while chronic infections are more often associated with protection (100) as previously mentioned in the regulatory network hypothesis. Thus, the undetermined course of helminth infections, whether acute or chronic, in the previously mentioned human studies is one of the reasons for the controversy concerning the protective effect against atopic asthma. Third, the undetermined host age when acquiring infection in most human epidemiological studies, is another cause of conflict (133), because the infections should occur earlier than the onset of the allergic sensitization, in order to induce preventive effects. Interestingly, all the previously mentioned animal studies supported the protective effects of helminth infections (88–91, 93, 94, 99, 102, 104) (Table 3). The courses of infection and the host age upon acquisition of infection, were clearly determined. This indicates that the early and chronic helminth infections is necessary to protect against the development of experimental allergic asthma. Fourth, most human studies did not compare allergic hereditary risk between the infected and non-infected or asthmatic and non-asthmatic subjects, since the significant difference in atopic family history between these groups may interfere with the proposed effects of helminths in human asthma (130). Only two studies (of non-supportive studies) performed this comparison and found a significant association of atopic family history with the asthmatic group (126, 127). Ultimately, these adverse outcomes may be because some helminths possess allergy-inducing and/or anti-allergic molecules, and thus the effect of helminth in asthma will depend on the predominant secretory molecule. For example, Ascaris suum (A. suum) contains both the allergenic protein of A. suum-3 (APAS-3) that induces/exacerbates allergic reactions and the suppressive protein of A. suum-1 (PAS-1) that suppresses asthma (142). This highlights the significance of identifying the helminth effector proteins against asthma, which is the next subject of discussion.
Helminth-Derived Proteins and Allergic Asthma
It is unlikely that infection with live worms or their eggs could be delivered into children as a vaccine to prevent asthma (132). In order to solve this prospective issue, identification of helminth proteins that mediate anti-allergic properties, followed by the production of similar recombinant proteins that mediate the same immune responses observed in live worm infection would be a better option for developing a future vaccine for allergic asthma (143). Many commercial companies and private entities have produced and marketed helminth-derived molecules for the treatment of inflammatory diseases (144). Therefore, several studies demonstrated the role of different helminthic proteins in the prevention and therapy of murine allergic asthma (Table 5). One of these effectual proteins is S. mansoni schistosomula (Smteg), extracted from the outer layer of the parasite. Smteg administration reduces eosinophilia, Th2 cytokines and allergen specific-IgE, and these effects can be mediated by a high level of IL-10 secreted by alveolar M2 macrophages and DCs. This indicates a potential preventive and/or therapeutic effects of Smteg protein in asthmatic mice (146). In addition, Sm22·6, which is a soluble protein associated with the tegument of S. mansoni, induces the same suppressive effects as Smteg through the induction of IL-10 secreted by Tregs. PIII, also derived from S. mansoni, mediates the same effects, but without elevation of IL-10. In addition, S. mansoni-derived Sm29 induces suppressive effects; however, lesser than the other two molecules (145). Another schistosome is S. japonicum containing the SJMHE1 protein, which is an HSP60‐derived peptide. SJMHE1 administration suppresses the development of murine asthma through inhibiting eosinophilia and Th2 cells and inducing Th1 and Treg cells (149). Likewise, soluble S. japonicum egg antigen mediates the same effects without inducing Th1 response (147). While P6, P25, and P30 peptides in SjP40 protein, which is the dominant protein of S. japonicum eggs, could prevent murine asthma by activating Th1 and alleviating Th2 cells (148). For A. lumbricoides, total protein extracts reduce IL-5 and eosinophilia and induce IL-10 to protect mice against experimental asthma (150). Another type of ascaris is A. suum that contains three different components. Each component can prevent murine asthma, namely; total protein extracts (151), pseudocoelomic fluid (152) and PAS-1 (142). They reduce eosinophilic inflammation and Th2 activities through the induction of Tregs and Th1 (142) or inhibition of DCs (152). Interestingly, as mentioned previously, A. suum has allergenic protein that can induce and exacerbate allergic reaction (142), indicating that identifying effective anti-allergic components in the worm extracts would be a crucial approach for developing novel preventive strategies. Moreover, soluble extracts of adult worms of T. spiralis have more potent suppressive impact on allergic asthma than those of soluble extracts of the larvae muscle of the same helminth (11). Another important consideration is the timing of protein injection, as the effective suppressive action of some helminth derived protein depends on early administration prior to developing asthma, which also indicates the importance of elucidating the preventive and therapeutic potentials of each candidate protein. The excretory/secretory products of Fasciola hepatica are a clear example of this indication, the administration of which during experimental murine asthma development, induces significant suppressive effects, while their administration after the establishment of the disease has no apparent effect (165). Another significant protein derived from helminth is AIP-2 secreted by Ancylostoma caninum (hookworm). AIP-2 has both preventive and therapeutic potentials on murine asthma through inhibition of DCs and induction of Tregs (164). ES-62, another promising molecule secreted by the filarial nematode Acanthocheilonema viteae (A. viteae). ES-62 has preventive and/or therapeutic potentials through the direct inhibition by mast cells FcϵRI-induced release of allergy mediators through blocking of key signal transduction molecules (153, 155). Moreover, several proteins derived from different species of helminths demonstrate preventive or therapeutic abilities against murine allergic asthma, such as Trichuris suis (162), H. polygyrus (156, 157), N. brasiliensis (158), Caenorhabditis elegans (161), Clonorchis sinensis (160), Angiostrongylus cantonensis (163) and Toxascaris leonine (159) (Table 5). Thus, worm-derived proteins might be exploited to prevent allergic asthma, since they suppress asthma through the same mechanisms that are induced with living worm infections without entailing their undesired side effects.
There are many studies investigating the protective effects of helminth-derived proteins in murine allergic asthma. Nevertheless, there are only few studies that detected these protein actions in human allergic asthma. S. mansoni antigens, Sm29 and Sm29TSP-2 reduce Th2 cells while inducing Tregs with subsequent high IL-10 production in the cell cultures from asthmatic patients (166). In same vein, other S. mansoni recombinant antigens as Sm22.6, Sm14, P24, and PIII antigen increase IL-10 secretion in cell cultures from subjects with asthma (167). Additionally, AIP-2, from the hookworm, suppresses human DCs activation and proliferation from asthmatic subjects in-vitro (164). In addition, ES-62 from A. viteae when co-cultured with sensitized human mast cells from healthy subjects, mediates the same effects previously mentioned with ES-62 with murine mast cells (153). However, these studies only demonstrate in-vitro therapeutic potentials for human asthma. Therefore, further research is needed to investigate both in-vitro and in-vivo preventive potentials of helminth-derived proteins against the development of human allergic asthma, to design novel strategies for potential allergic asthma vaccines.
Summary and Conclusions
In this study, we discussed the immunomodulatory effects of different natural infections on the development of asthma, and the benefits of imitating these phenomena via the use of effective proteins-based vaccines for future disease control. Natural infection with Mtb prevents the development of allergic asthma. Therefore, BCG vaccine is suggested at an early age to mediate the same prevention particularly with increasing its efficiency through genetic engineering-based modifications, which are beneficial for tuberculosis prevention as well. Similarly, natural helminthic infections may prevent allergic asthma development. Therefore, helminth-derived protein at early age is an effective candidate for designing allergic asthma vaccines and requires further investigation. We revealed the beneficial features of the hygiene hypothesis for preventing allergic asthma via simulating the natural infections that either induce Th1 or Th2 cells primarily as nature will find its way regardless of the immune pathway, and this can also be applied to other allergic diseases. In addition, since the hygiene hypothesis also includes autoimmune diseases as mentioned earlier, imitation of the nature could be the missing key for such diseases. We, therefore, recommend mimicking nature to be a general strategy for preventing allergic asthma and other diseases that increased dramatically over the past decades.
Author Contributions
MA conceptualized the idea. MA, XJ, JW and FA performed the literature study and wrote the original draft. MA, SA and HX discussed, reviewed and edited the manuscript. All authors contributed to the article and approved the submitted version.
Funding
This work was supported by National Natural Science Foundation of China (Grant No. 81771756) and the Doctorial Innovation Projects of Jiangsu Province (Grant no. KYCX17_1816).
Conflict of Interest
The authors declare that the research was conducted in the absence of any commercial or financial relationships that could be construed as a potential conflict of interest.
Publisher’s Note
All claims expressed in this article are solely those of the authors and do not necessarily represent those of their affiliated organizations, or those of the publisher, the editors and the reviewers. Any product that may be evaluated in this article, or claim that may be made by its manufacturer, is not guaranteed or endorsed by the publisher.
Acknowledgments
We thank Professor Ahmed Sadek at Assiut University for his advice and discussion. Also, we thank Dr. Yu Tian at Jiangsu University for his valuable comments.
Abbreviations
A. lumbricoides, Ascaris lumbricoides; A. suum, Ascaris suum; A. viteae, Acanthocheilonema viteae; Ag85A, Antigen 85A; Ag85B, Antigen 85B; AHR, Airway hyperreactivity; AIP-2, anti-inflammatory protein-2; APC, Antigen presenting cell; BCG, Bacille Calmette-Guérin; DCs, Dendritic cells; E. vermicularis, Entrobius vermicularis; ES, Excretory/secretory protein; H. polygyrus, Heligosomoides polygyrus; i.d., Intradermal; i.n., Intranasal; IL, Interleukin; ISAAC, International Study of Asthma and Allergies in Childhood; M. bovis, Mycobacterium bovis; Mtb, Mycobacterium tuberculosis bacteria; N. americanus, Necator americanus; N. brasiliensis, Nippostrongylus brasiliensis; OVA, Ovalbumin; RD, Region of difference; S. japonicum, Schistosoma japonicum; S. mansoni, Schistosoma mansoni; S. stercoralis, Strongyloides stercoralis; Smteg, schistosomula tegument; T. canis, Toxocara canis; T. spiralis, Trichinella spiralis; T. trichiura, Trichuris trichiura; TB, Tuberculosis; TGF, Transforming growth factor; Th, T helper; TLR, Toll-like receptor; TNF, Tumor necrosis factor; TP, Total protein; Tregs, T regulatory cells.
References
1. Dharmage SC, Perret JL, Custovic A. Epidemiology of Asthma in Children and Adults. Front Pediatr (2019) 7:246. doi: 10.3389/fped.2019.00246
2. Abdelaziz MH, Abdelwahab SF, Wan J, Cai W, Huixuan W, Jianjun C, et al. Alternatively Activated Macrophages; A Double-Edged Sword in Allergic Asthma. J Transl Med (2020) 18(1):58. doi: 10.1186/s12967-020-02251-w
3. Beasley R, Crane J, Lai CK, Pearce N. Prevalence and Etiology of Asthma. J Allergy Clin Immunol (2000) 105(2 Pt 2):S466–72. doi: 10.1016/s0091-6749(00)90044-7
4. Gerrard JW, Geddes CA, Reggin PL, Gerrard CD, Horne S. Serum IgE Levels in White and Metis Communities in Saskatchewan. Ann Allergy (1976) 37(2):91–100.
5. Strachan DP. Hay Fever, Hygiene, and Household Size. BMJ (1989) 299(6710):1259–60. doi: 10.1136/bmj.299.6710.1259
6. Wammes LJ, Mpairwe H, Elliott AM, Yazdanbakhsh M. Helminth Therapy or Elimination: Epidemiological, Immunological, and Clinical Considerations. Lancet Infect Dis (2014) 14(11):1150–62. doi: 10.1016/S1473-3099(14)70771-6
7. Ponte EV, Rizzo JA, Cruz AA. Interrelationship Among Asthma, Atopy, and Helminth Infections. J Bras Pneumol (2007) 33(3):335–42. doi: 10.1590/s1806-37132007000300016
8. Liu Z, Liu Q, Bleich D, Salgame P, Gause WC. Regulation of Type 1 Diabetes, Tuberculosis, and Asthma by Parasites. J Mol Med (Berl) (2010) 88(1):27–38. doi: 10.1007/s00109-009-0546-0
9. Fernandes JS, Cardoso LS, Pitrez PM, Cruz AA. Helminths and Asthma: Risk and Protection. Immunol Allergy Clin North Am (2019) 39(3):417–27. doi: 10.1016/j.iac.2019.03.009
10. Logan J, Navarro S, Loukas A, Giacomin P. Helminth-Induced Regulatory T Cells and Suppression of Allergic Responses. Curr Opin Immunol (2018) 54:1–6. doi: 10.1016/j.coi.2018.05.007
11. Sun S, Li H, Yuan Y, Wang L, He W, Xie H, et al. Preventive and Therapeutic Effects of Trichinella Spiralis Adult Extracts on Allergic Inflammation in an Experimental Asthma Mouse Model. Parasit Vectors (2019) 12(1):326. doi: 10.1186/s13071-019-3561-1
12. Helmby H. Human Helminth Therapy to Treat Inflammatory Disorders - Where Do We Stand? BMC Immunol (2015) 16:12. doi: 10.1186/s12865-015-0074-3
13. Rook GA. 99th Dahlem Conference on Infection, Inflammation and Chronic Inflammatory Disorders: Darwinian Medicine and the ‘Hygiene’ or ‘Old Friends’ Hypothesis. Clin Exp Immunol (2010) 160(1):70–9. doi: 10.1111/j.1365-2249.2010.04133.x
14. Hanski I, von Hertzen L, Fyhrquist N, Koskinen K, Torppa K, Laatikainen T, et al. Environmental Biodiversity, Human Microbiota, and Allergy Are Interrelated. Proc Natl Acad Sci U S A (2012) 109(21):8334–9. doi: 10.1073/pnas.1205624109
15. Tarancon R, Uranga S, Martin C, Aguilo N. Mycobacterium Tuberculosis Infection Prevents Asthma and Abrogates Eosinophilopoiesis in an Experimental Model. Allergy (2019) 74(12):2512–14. doi: 10.1111/all.13923
16. Scanga CB, Le Gros G. Development of an Asthma Vaccine: Research Into BCG. Drugs (2000) 59(6):1217–21. doi: 10.2165/00003495-200059060-00002
17. Guyot-Revol V, Innes JA, Hackforth S, Hinks T, Lalvani A. Regulatory T Cells are Expanded in Blood and Disease Sites in Patients With Tuberculosis. Am J Respir Crit Care Med (2006) 173(7):803–10. doi: 10.1164/rccm.200508-1294OC
18. Wergeland I, Assmus J, Dyrhol-Riise AM. T Regulatory Cells and Immune Activation in Mycobacterium Tuberculosis Infection and the Effect of Preventive Therapy. Scand J Immunol (2011) 73(3):234–42. doi: 10.1111/j.1365-3083.2010.02496.x
19. Sano K, Haneda K, Tamura G, Shirato K. Ovalbumin (OVA) and Mycobacterium Tuberculosis Bacilli Cooperatively Polarize Anti-OVA T-Helper (Th) Cells Toward a Th1-Dominant Phenotype and Ameliorate Murine Tracheal Eosinophilia. Am J Respir Cell Mol Biol (1999) 20(6):1260–7. doi: 10.1165/ajrcmb.20.6.3546
20. von Mutius E, Pearce N, Beasley R, Cheng S, von Ehrenstein O, Bjorksten B, et al. International Patterns of Tuberculosis and the Prevalence of Symptoms of Asthma, Rhinitis, and Eczema. Thorax (2000) 55(6):449–53. doi: 10.1136/thorax.55.6.449
21. Shirtcliffe P, Weatherall M, Beasley R. An Inverse Correlation Between Estimated Tuberculosis Notification Rates and Asthma Symptoms. Respirology (2002) 7(2):153–5. doi: 10.1046/j.1440-1843.2002.00372.x
22. von Hertzen L, Klaukka T, Mattila H, Haahtela T. Mycobacterium Tuberculosis Infection and the Subsequent Development of Asthma and Allergic Conditions. J Allergy Clin Immunol (1999) 104(6):1211–4. doi: 10.1016/s0091-6749(99)70015-1
23. Jones PD, Gibson PG, Henry RL. The Prevalence of Asthma Appears to be Inversely Related to the Incidence of Typhoid and Tuberculosis: Hypothesis to Explain the Variation in Asthma Prevalence Around the World. Med Hypotheses (2000) 55(1):40–2. doi: 10.1054/mehy.1999.0997
24. Vargas MH, Becerril-Angeles M, Medina-Reyes IS, Rascon-Pacheco RA. Altitude Above 1500m is a Major Determinant of Asthma Incidence. An Ecological Study. Respir Med (2018) 135:1–7. doi: 10.1016/j.rmed.2017.12.010
25. Shirakawa T, Enomoto T, Shimazu S, Hopkin JM. The Inverse Association Between Tuberculin Responses and Atopic Disorder. Science (1997) 275(5296):77–9. doi: 10.1126/science.275.5296.77
26. Miyake Y, Arakawa M, Tanaka K, Sasaki S, Ohya Y. Tuberculin Reactivity and Allergic Disorders in Schoolchildren, Okinawa, Japan. Clin Exp Allergy (2008) 38(3):486–92. doi: 10.1111/j.1365-2222.2007.02869.x
27. Joung SM, Ryoo S. BCG Vaccine in Korea. Clin Exp Vaccine Res (2013) 2(2):83–91. doi: 10.7774/cevr.2013.2.2.83
28. Kowalewicz-Kulbat M, Locht C. BCG and Protection Against Inflammatory and Auto-Immune Diseases. Expert Rev Vaccines (2017) 16(7):1–10. doi: 10.1080/14760584.2017.1333906
29. Abebe F. Is Interferon-Gamma the Right Marker for Bacille Calmette-Guerin-Induced Immune Protection? The Missing Link in Our Understanding of Tuberculosis Immunology. Clin Exp Immunol (2012) 169(3):213–9. doi: 10.1111/j.1365-2249.2012.04614.x
30. Dockrell HM, Smith SG. What Have We Learnt About BCG Vaccination in the Last 20 Years? Front Immunol (2017) 8:1134. doi: 10.3389/fimmu.2017.01134
31. Oettinger T, Jorgensen M, Ladefoged A, Haslov K, Andersen P. Development of the Mycobacterium Bovis BCG Vaccine: Review of the Historical and Biochemical Evidence for a Genealogical Tree. Tuber Lung Dis (1999) 79(4):243–50. doi: 10.1054/tuld.1999.0206
32. Andersen P, Doherty TM. The Success and Failure of BCG - Implications for a Novel Tuberculosis Vaccine. Nat Rev Microbiol (2005) 3(8):656–62. doi: 10.1038/nrmicro1211
33. El-Zein M, Parent ME, Benedetti A, Rousseau MC. Does BCG Vaccination Protect Against the Development of Childhood Asthma? A Systematic Review and Meta-Analysis of Epidemiological Studies. Int J Epidemiol (2010) 39(2):469–86. doi: 10.1093/ije/dyp307
34. Mohammadi-Shahrokhi V, Rezaei A, Andalib A, Rahnama A, Jafarzadeh A, Eskandari N. Immunomodulatory Effects of Adjuvants CPG, MPLA, and BCG on the Derp2-Induced Acute Asthma at Early Life in an Animal Model of BALB/c Mice. Inflammation (2017) 40(1):259–74. doi: 10.1007/s10753-016-0476-2
35. Counoupas C, Pinto R, Nagalingam G, Britton WJ, Triccas JA. Protective Efficacy of Recombinant BCG Over-Expressing Protective, Stage-Specific Antigens of Mycobacterium Tuberculosis. Vaccine (2018) 36(19):2619–29. doi: 10.1016/j.vaccine.2018.03.066
36. Nahori MA, Lagranderie M, Lefort J, Thouron F, Joseph D, Winter N, et al. Effects of Mycobacterium Bovis BCG on the Development of Allergic Inflammation and Bronchial Hyperresponsiveness in Hyper-IgE BP2 Mice Vaccinated as Newborns. Vaccine (2001) 19(11-12):1484–95. doi: 10.1016/s0264-410x(00)00345-5
37. Kim YJ, Kim HJ, Kang MJ, Yu HS, Seo JH, Kim HY, et al. Bacillus Calmette-Guerin Suppresses Asthmatic Responses via CD4(+)CD25(+) Regulatory T Cells and Dendritic Cells. Allergy Asthma Immunol Res (2014) 6(3):201–7. doi: 10.4168/aair.2014.6.3.201
38. Choi IS, Lin XH, Koh YA, Koh YI, Lee HC. Strain-Dependent Suppressive Effects of BCG Vaccination on Asthmatic Reactions in BALB/c Mice. Ann Allergy Asthma Immunol (2005) 95(6):571–8. doi: 10.1016/S1081-1206(10)61021-6
39. Samary Cdos S, Antunes MA, Silva JD, Silva AL, Araujo CC, Bakker-Abreu I, et al. Impact of Bacillus Calmette-Guerin Moreau Vaccine on Lung Remodeling in Experimental Asthma. Respir Physiol Neurobiol (2013) 189(3):614–23. doi: 10.1016/j.resp.2013.07.025
40. Gouveia ACC, Braga FG, Mota M, Silva FMC, Brugiolo ASS, Oliveira EE, et al. Enhanced Expression of PD-L1 and IFN-Gamma on Dendritic Cells is Associated With BCG-Induced Th2 Inhibition. Cytokine (2017) 99:163–72. doi: 10.1016/j.cyto.2017.09.005
41. Herz U, Gerhold K, Gruber C, Braun A, Wahn U, Renz H, et al. BCG Infection Suppresses Allergic Sensitization and Development of Increased Airway Reactivity in an Animal Model. J Allergy Clin Immunol (1998) 102(5):867–74. doi: 10.1016/s0091-6749(98)70030-2
42. Shen H, Huang H, Wang J, Ye S, Li W, Wang K, et al. Neonatal Vaccination With Bacillus Calmette-Guerin Elicits Long-Term Protection in Mouse-Allergic Responses. Allergy (2008) 63(5):555–63. doi: 10.1111/j.1398-9995.2008.01637.x
43. Zhang G, Wang P, Qiu Z, Qin X, Lin X, Li N, et al. Distant Lymph Nodes Serve as Pools of Th1 Cells Induced by Neonatal BCG Vaccination for the Prevention of Asthma in Mice. Allergy (2013) 68(3):330–8. doi: 10.1111/all.12099
44. Choi IS, Lin XH, Koh YA, Cui Y. Inoculation Route-Dependent and Allergen-Specific Suppressive Effects of Bacille Calmette-Guerin Vaccination on Asthmatic Reactions in BALB/c Mice. Lung (2007) 185(3):179–86. doi: 10.1007/s00408-007-9003-4
45. Han ER, Choi IS, Eom SH, Kim HJ. Preventive Effects of Mycobacteria and Their Culture Supernatants Against Asthma Development in BALB/c Mice. Allergy Asthma Immunol Res (2010) 2(1):34–40. doi: 10.4168/aair.2010.2.1.34
46. Gong P, Li Y, Tan YP, Li H. Pretreatment With Inactivated Bacillus Calmette-Guerin Increases CD4+CD25+ Regulatory T Cell Function and Decreases Functional and Structural Effects of Asthma Induction in a Rat Asthma Model. Artif Organs (2016) 40(4):360–7. doi: 10.1111/aor.12566
47. Hopfenspirger MT, Agrawal DK. Airway Hyperresponsiveness, Late Allergic Response, and Eosinophilia Are Reversed With Mycobacterial Antigens in Ovalbumin-Presensitized Mice. J Immunol (2002) 168(5):2516–22. doi: 10.4049/jimmunol.168.5.2516
48. Gouveia AC, Brugiolo AS, Alves CC, Silva FM, Mesquita FP, Gameiro J, et al. Th2 Responses in OVA-Sensitized BALB/c Mice Are Down-Modulated by Mycobacterium Bovis BCG Treatment. J Clin Immunol (2013) 33(1):235–45. doi: 10.1007/s10875-012-9746-4
49. Ahrens B, Gruber C, Rha RD, Freund T, Quarcoo D, Awagyan A, et al. BCG Priming of Dendritic Cells Enhances T Regulatory and Th1 Function and Suppresses Allergen-Induced Th2 Function In Vitro and In Vivo. Int Arch Allergy Immunol (2009) 150(3):210–20. doi: 10.1159/000222673
50. Bilenki L, Gao X, Wang S, Yang J, Fan Y, Han X, et al. Dendritic Cells From Mycobacteria-Infected Mice Inhibits Established Allergic Airway Inflammatory Responses to Ragweed via IL-10- and IL-12-Secreting Mechanisms. J Immunol (2010) 184(12):7288–96. doi: 10.4049/jimmunol.0902829
51. Gao X, Bai H, Cheng J, Fan Y, Wang S, Jiao L, et al. CD8alpha+ and CD8alpha- DC Subsets From BCG-Infected Mice Inhibit Allergic Th2-Cell Responses by Enhancing Th1-Cell and Treg-Cell Activity Respectively. Eur J Immunol (2012) 42(1):165–75. doi: 10.1002/eji.201141833
52. Townley RG, Barlan IB, Patino C, Vichyanond P, Minervini MC, Simasathien T, et al. The Effect of BCG Vaccine at Birth on the Development of Atopy or Allergic Disease in Young Children. Ann Allergy Asthma Immunol (2004) 92(3):350–5. doi: 10.1016/S1081-1206(10)61574-8
53. Martignon G, Oryszczyn MP, Annesi-Maesano I. Does Childhood Immunization Against Infectious Diseases Protect From the Development of Atopic Disease? Pediatr Allergy Immunol (2005) 16(3):193–200. doi: 10.1111/j.1399-3038.2005.00254.x
54. Linehan MF, Frank TL, Hazell ML, Francis HC, Morris JA, Baxter DN, et al. Is the Prevalence of Wheeze in Children Altered by Neonatal BCG Vaccination? J Allergy Clin Immunol (2007) 119(5):1079–85. doi: 10.1016/j.jaci.2006.12.672
55. Singh M, Das RR, Kumar L, Kumar R. Bacille Calmette-Guerin Vaccination is Associated With Lower Prevalence of Allergic Diseases in Indian Children. Am J Rhinol Allergy (2013) 27(4):e107–12. doi: 10.2500/ajra.2013.27.3940
56. Gruber C, Meinlschmidt G, Bergmann R, Wahn U, Stark K. Is Early BCG Vaccination Associated With Less Atopic Disease? An Epidemiological Study in German Preschool Children With Different Ethnic Backgrounds. Pediatr Allergy Immunol (2002) 13(3):177–81. doi: 10.1034/j.1399-3038.2002.01006.x
57. Garcia-Marcos L, Suarez-Varela MM, Canflanca IM, Garrido JB, Quiros AB, Lopez-Silvarrey Varela A, et al. BCG Immunization at Birth and Atopic Diseases in a Homogeneous Population of Spanish Schoolchildren. Int Arch Allergy Immunol (2005) 137(4):303–9. doi: 10.1159/000086461
58. Alm JS, Lilja G, Pershagen G, Scheynius A. Early BCG Vaccination and Development of Atopy. Lancet (1997) 350(9075):400–3. doi: 10.1016/S0140-6736(97)02207-1
59. Strannegard IL, Larsson LO, Wennergren G, Strannegard O. Prevalence of Allergy in Children in Relation to Prior BCG Vaccination and Infection With Atypical Mycobacteria. Allergy (1998) 53(3):249–54. doi: 10.1111/j.1398-9995.1998.tb03884.x
60. Gruber C, Kulig M, Bergmann R, Guggenmoos-Holzmann I, Wahn U, Group MASS. Delayed Hypersensitivity to Tuberculin, Total Immunoglobulin E, Specific Sensitization, and Atopic Manifestation in Longitudinally Followed Early Bacille Calmette-Guerin-Vaccinated and Nonvaccinated Children. Pediatrics (2001) 107(3):E36. doi: 10.1542/peds.107.3.e36
61. Mommers M, Weishoff-Houben M, Swaen GM, Creemers H, Freund H, Dott W, et al. Infant Immunization and the Occurrence of Atopic Disease in Dutch and German Children: A Nested Case-Control Study. Pediatr Pulmonol (2004) 38(4):329–34. doi: 10.1002/ppul.20089
62. Mohrenschlager M, Haberl VM, Kramer U, Behrendt H, Ring J. Early BCG and Pertussis Vaccination and Atopic Diseases in 5- to 7-Year-Old Preschool Children From Augsburg, Germany: Results From the MIRIAM Study. Pediatr Allergy Immunol (2007) 18(1):5–9. doi: 10.1111/j.1399-3038.2006.00485.x
63. Steenhuis TJ, van Aalderen WM, Bloksma N, Nijkamp FP, van der Laag J, van Loveren H, et al. Bacille-Calmette-Guerin Vaccination and the Development of Allergic Disease in Children: A Randomized, Prospective, Single-Blind Study. Clin Exp Allergy (2008) 38(1):79–85. doi: 10.1111/j.1365-2222.2007.02859.x
64. El-Zein M, Conus F, Benedetti A, Menzies D, Parent ME, Rousseau MC. Association Between Bacillus Calmette-Guerin Vaccination and Childhood Asthma in the Quebec Birth Cohort on Immunity and Health. Am J Epidemiol (2017) 186(3):344–55. doi: 10.1093/aje/kwx088
65. Sarinho E, Schor D, Veloso M, Lima M. BCG Scar Diameter and Asthma: A Case-Control Study. J Allergy Clin Immunol (2000) 106(6):1199–200. doi: 10.1067/mai.2000.111241
66. Park SS, Heo EY, Kim DK, Chung HS, Lee CH. The Association of BCG Vaccination With Atopy and Asthma in Adults. Int J Med Sci (2015) 12(8):668–73. doi: 10.7150/ijms.12233
67. Choi IS, Koh YI. Therapeutic Effects of BCG Vaccination in Adult Asthmatic Patients: A Randomized, Controlled Trial. Ann Allergy Asthma Immunol (2002) 88(6):584–91. doi: 10.1016/S1081-1206(10)61890-X
68. Datau EA, Mewengkang H, Matheos JC, Purnawan I, Wibisono M, Wongdjaja K, et al. Clinical Efficacy and Laboratory Improvement of Bacillus Calmette-Guerin Vaccination on Adult Atopic Asthma: A Cohort Study. World Allergy Organ J (2008) 1(4):63–9. doi: 10.1097/WOX.0b013e31816c8b85
69. Deng Y, Chen W, Zang N, Li S, Luo Y, Ni K, et al. The Antiasthma Effect of Neonatal BCG Vaccination Does Not Depend on the Th17/Th1 But IL-17/IFN-Gamma Balance in a BALB/c Mouse Asthma Model. J Clin Immunol (2011) 31(3):419–29. doi: 10.1007/s10875-010-9503-5
70. Deng Y, Li W, Luo Y, Wang LJ, Xie XH, Luo J, et al. Inhibition of IFN-Gamma Promotes Anti-Asthma Effect of Mycobacterium Bovis Bacillus Calmette-Guerin Neonatal Vaccination: A Murine Asthma Model. Vaccine (2014) 32(18):2070–8. doi: 10.1016/j.vaccine.2014.02.007
71. Ogongo P, Porterfield JZ, Leslie A. Lung Tissue Resident Memory T-Cells in the Immune Response to Mycobacterium Tuberculosis. Front Immunol (2019) 10:992. doi: 10.3389/fimmu.2019.00992
72. Gabutti G, Trucchi C, Conversano M, Zivelonghi G, Zoppi G. Booster Vaccination: The Role of Reduced Antigen Content Vaccines as a Preschool Booster. BioMed Res Int (2014) 2014:541319. doi: 10.1155/2014/541319
73. Bajenoff M, Wurtz O, Guerder S. Repeated Antigen Exposure is Necessary for the Differentiation, But Not the Initial Proliferation, of Naive CD4(+) T Cells. J Immunol (2002) 168(4):1723–9. doi: 10.4049/jimmunol.168.4.1723
74. Steigler P, Verrall AJ, Kirman JR. Beyond Memory T Cells: Mechanisms of Protective Immunity to Tuberculosis Infection. Immunol Cell Biol (2019) 97(7):647–55. doi: 10.1111/imcb.12278
75. Wu J, Xu J, Cai C, Gao X, Li L, Zhong N. Ag85B DNA Vaccine Suppresses Airway Inflammation in a Murine Model of Asthma. Respir Res (2009) 10(1):51. doi: 10.1186/1465-9921-10-51
76. Zhang Y, Feng L, Li L, Wang D, Li C, Sun C, et al. Effects of the Fusion Design and Immunization Route on the Immunogenicity of Ag85A-Mtb32 in Adenoviral Vectored Tuberculosis Vaccine. Hum Vaccin Immunother (2015) 11(7):1803–13. doi: 10.1080/21645515.2015.1042193
77. Zhang Y, Feng Y, Li L, Ye X, Wang J, Wang Q, et al. Immunization With an Adenovirus-Vectored TB Vaccine Containing Ag85A-Mtb32 Effectively Alleviates Allergic Asthma. J Mol Med (Berl) (2018) 96(3-4):249–63. doi: 10.1007/s00109-017-1614-5
78. Horwitz MA, Lee BW, Dillon BJ, Harth G. Protective Immunity Against Tuberculosis Induced by Vaccination With Major Extracellular Proteins of Mycobacterium Tuberculosis. Proc Natl Acad Sci USA (1995) 92(5):1530–4. doi: 10.1073/pnas.92.5.1530
79. Smaill F, Jeyanathan M, Smieja M, Medina MF, Thanthrige-Don N, Zganiacz A, et al. A Human Type 5 Adenovirus-Based Tuberculosis Vaccine Induces Robust T Cell Responses in Humans Despite Preexisting Anti-Adenovirus Immunity. Sci Transl Med (2013) 5(205):205ra134. doi: 10.1126/scitranslmed.3006843
80. Christ AP, Rodriguez D, Bortolatto J, Borducchi E, Keller A, Mucida D, et al. Enhancement of Th1 Lung Immunity Induced by Recombinant Mycobacterium Bovis Bacillus Calmette-Guerin Attenuates Airway Allergic Disease. Am J Respir Cell Mol Biol (2010) 43(2):243–52. doi: 10.1165/rcmb.2009-0040OC
81. Freidag BL, Melton GB, Collins F, Klinman DM, Cheever A, Stobie L, et al. CpG Oligodeoxynucleotides and Interleukin-12 Improve the Efficacy of Mycobacterium Bovis BCG Vaccination in Mice Challenged With M. Tuberculosis. Infect Immun (2000) 68(5):2948–53. doi: 10.1128/iai.68.5.2948-2953.2000
82. Biet F, Duez C, Kremer L, Marquillies P, Amniai L, Tonnel AB, et al. Recombinant Mycobacterium Bovis BCG Producing IL-18 Reduces IL-5 Production and Bronchoalveolar Eosinophilia Induced by an Allergic Reaction. Allergy (2005) 60(8):1065–72. doi: 10.1111/j.1398-9995.2005.00826.x
83. Ke X, Huang J, Chen Q, Hong S, Zhu D. Protective Effects of Combined Mycobacterium Bovis BCG and Interleukin-12 Vaccination on Airway Inflammation in a Murine Model of Allergic Asthma. Clin Invest Med (2010) 33(3):E196–202. doi: 10.25011/cim.v33i3.13726
84. Cooper AJR, Dholakia S, Holland CV, Friend PJ. Helminths in Organ Transplantation. Lancet Infect Dis (2017) 17(6):e166–e76. doi: 10.1016/S1473-3099(16)30533-3
85. Anthony RM, Rutitzky LI, Urban JF Jr, Stadecker MJ, Gause WC. Protective Immune Mechanisms in Helminth Infection. Nat Rev Immunol (2007) 7(12):975–87. doi: 10.1038/nri2199
86. Evans H, Mitre E. Worms as Therapeutic Agents for Allergy and Asthma: Understanding Why Benefits in Animal Studies Have Not Translated Into Clinical Success. J Allergy Clin Immunol (2015) 135(2):343–53. doi: 10.1016/j.jaci.2014.07.007
87. Yap GS, Gause WC. Helminth Infections Induce Tissue Tolerance Mitigating Immunopathology But Enhancing Microbial Pathogen Susceptibility. Front Immunol (2018) 9:2135. doi: 10.3389/fimmu.2018.02135
88. Mangan NE, van Rooijen N, McKenzie AN, Fallon PG. Helminth-Modified Pulmonary Immune Response Protects Mice From Allergen-Induced Airway Hyperresponsiveness. J Immunol (2006) 176(1):138–47. doi: 10.4049/jimmunol.176.1.138
89. Smits HH, Hammad H, van Nimwegen M, Soullie T, Willart MA, Lievers E, et al. Protective Effect of Schistosoma Mansoni Infection on Allergic Airway Inflammation Depends on the Intensity and Chronicity of Infection. J Allergy Clin Immunol (2007) 120(4):932–40. doi: 10.1016/j.jaci.2007.06.009
90. Pacifico LG, Marinho FA, Fonseca CT, Barsante MM, Pinho V, Sales-Junior PA, et al. Schistosoma Mansoni Antigens Modulate Experimental Allergic Asthma in a Murine Model: A Major Role for CD4+ CD25+ Foxp3+ T Cells Independent of Interleukin-10. Infect Immun (2009) 77(1):98–107. doi: 10.1128/IAI.00783-07
91. Layland LE, Straubinger K, Ritter M, Loffredo-Verde E, Garn H, Sparwasser T, et al. Schistosoma Mansoni-Mediated Suppression of Allergic Airway Inflammation Requires Patency and Foxp3+ Treg Cells. PLoS Negl Trop Dis (2013) 7(8):e2379. doi: 10.1371/journal.pntd.0002379
92. Mo HM, Lei JH, Jiang ZW, Wang CZ, Cheng YL, Li YL, et al. Schistosoma Japonicum Infection Modulates the Development of Allergen-Induced Airway Inflammation in Mice. Parasitol Res (2008) 103(5):1183–9. doi: 10.1007/s00436-008-1114-1
93. Park HK, Cho MK, Choi SH, Kim YS, Yu HS. Trichinella Spiralis: Infection Reduces Airway Allergic Inflammation in Mice. Exp Parasitol (2011) 127(2):539–44. doi: 10.1016/j.exppara.2010.10.004
94. Aranzamendi C, de Bruin A, Kuiper R, Boog CJ, van Eden W, Rutten V, et al. Protection Against Allergic Airway Inflammation During the Chronic and Acute Phases of Trichinella Spiralis Infection. Clin Exp Allergy (2013) 43(1):103–15. doi: 10.1111/cea.12042
95. Kitagaki K, Businga TR, Racila D, Elliott DE, Weinstock JV, Kline JN. Intestinal Helminths Protect in a Murine Model of Asthma. J Immunol (2006) 177(3):1628–35. doi: 10.4049/jimmunol.177.3.1628
96. Hartmann S, Schnoeller C, Dahten A, Avagyan A, Rausch S, Lendner M, et al. Gastrointestinal Nematode Infection Interferes With Experimental Allergic Airway Inflammation But Not Atopic Dermatitis. Clin Exp Allergy (2009) 39(10):1585–96. doi: 10.1111/j.1365-2222.2009.03290.x
97. Dittrich AM, Erbacher A, Specht S, Diesner F, Krokowski M, Avagyan A, et al. Helminth Infection With Litomosoides Sigmodontis Induces Regulatory T Cells and Inhibits Allergic Sensitization, Airway Inflammation, and Hyperreactivity in a Murine Asthma Model. J Immunol (2008) 180(3):1792–9. doi: 10.4049/jimmunol.180.3.1792
98. Wang CC, Nolan TJ, Schad GA, Abraham D. Infection of Mice With the Helminth Strongyloides Stercoralis Suppresses Pulmonary Allergic Responses to Ovalbumin. Clin Exp Allergy (2001) 31(3):495–503. doi: 10.1046/j.1365-2222.2001.01044.x
99. Wohlleben G, Trujillo C, Muller J, Ritze Y, Grunewald S, Tatsch U, et al. Helminth Infection Modulates the Development of Allergen-Induced Airway Inflammation. Int Immunol (2004) 16(4):585–96. doi: 10.1093/intimm/dxh062
100. Obieglo K, Schuijs MJ, Ozir-Fazalalikhan A, Otto F, van Wijck Y, Boon L, et al. Isolated Schistosoma Mansoni Eggs Prevent Allergic Airway Inflammation. Parasite Immunol (2018) 40(10):e12579. doi: 10.1111/pim.12579
101. Amu S, Saunders SP, Kronenberg M, Mangan NE, Atzberger A, Fallon PG. Regulatory B Cells Prevent and Reverse Allergic Airway Inflammation via FoxP3-Positive T Regulatory Cells in a Murine Model. J Allergy Clin Immunol (2010) 125(5):1114–24 e8. doi: 10.1016/j.jaci.2010.01.018
102. van der Vlugt LE, Labuda LA, Ozir-Fazalalikhan A, Lievers E, Gloudemans AK, Liu KY, et al. Schistosomes Induce Regulatory Features in Human and Mouse CD1d(hi) B Cells: Inhibition of Allergic Inflammation by IL-10 and Regulatory T Cells. PLoS One (2012) 7(2):e30883. doi: 10.1371/journal.pone.0030883
103. Liu JY, Li LY, Yang XZ, Li J, Zhong G, Wang J, et al. Adoptive Transfer of Dendritic Cells Isolated From Helminth-Infected Mice Enhanced T Regulatory Cell Responses in Airway Allergic Inflammation. Parasite Immunol (2011) 33(10):525–34. doi: 10.1111/j.1365-3024.2011.01308.x
104. Wilson MS, Taylor MD, O’Gorman MT, Balic A, Barr TA, Filbey K, et al. Helminth-Induced CD19+CD23hi B Cells Modulate Experimental Allergic and Autoimmune Inflammation. Eur J Immunol (2010) 40(6):1682–96. doi: 10.1002/eji.200939721
105. Hagel I, Lynch NR, DiPrisco MC, Lopez RI, Garcia NM. Allergic Reactivity of Children of Different Socioeconomic Levels in Tropical Populations. Int Arch Allergy Immunol (1993) 101(2):209–14. doi: 10.1159/000236521
106. Araujo MI, Lopes AA, Medeiros M, Cruz AA, Sousa-Atta L, Sole D, et al. Inverse Association Between Skin Response to Aeroallergens and Schistosoma Mansoni Infection. Int Arch Allergy Immunol (2000) 123(2):145–8. doi: 10.1159/000024433
107. van den Biggelaar AH, van Ree R, Rodrigues LC, Lell B, Deelder AM, Kremsner PG, et al. Decreased Atopy in Children Infected With Schistosoma Haematobium: A Role for Parasite-Induced Interleukin-10. Lancet (2000) 356(9243):1723–7. doi: 10.1016/S0140-6736(00)03206-2
108. van den Biggelaar AH, Lopuhaa C, van Ree R, van der Zee JS, Jans J, Hoek A, et al. The Prevalence of Parasite Infestation and House Dust Mite Sensitization in Gabonese Schoolchildren. Int Arch Allergy Immunol (2001) 126(3):231–8. doi: 10.1159/000049519
109. Cooper PJ, Chico ME, Rodrigues LC, Ordonez M, Strachan D, Griffin GE, et al. Reduced Risk of Atopy Among School-Age Children Infected With Geohelminth Parasites in a Rural Area of the Tropics. J Allergy Clin Immunol (2003) 111(5):995–1000. doi: 10.1067/mai.2003.1348
110. Schafer T, Meyer T, Ring J, Wichmann HE, Heinrich J. Worm Infestation and the Negative Association With Eczema (Atopic/Nonatopic) and Allergic Sensitization. Allergy (2005) 60(8):1014–20. doi: 10.1111/j.1398-9995.2005.00801.x
111. Flohr C, Tuyen LN, Lewis S, Quinnell R, Minh TT, Liem HT, et al. Poor Sanitation and Helminth Infection Protect Against Skin Sensitization in Vietnamese Children: A Cross-Sectional Study. J Allergy Clin Immunol (2006) 118(6):1305–11. doi: 10.1016/j.jaci.2006.08.035
112. Rodrigues LC, Newcombe PJ, Cunha SS, Alcantara-Neves NM, Genser B, Cruz AA, et al. Early Infection With Trichuris Trichiura and Allergen Skin Test Reactivity in Later Childhood. Clin Exp Allergy (2008) 38(11):1769–77. doi: 10.1111/j.1365-2222.2008.03027.x
113. Lynch NR, Hagel I, Perez M, Di Prisco MC, Lopez R, Alvarez N. Effect of Anthelmintic Treatment on the Allergic Reactivity of Children in a Tropical Slum. J Allergy Clin Immunol (1993) 92(3):404–11. doi: 10.1016/0091-6749(93)90119-z
114. van den Biggelaar AH, Rodrigues LC, van Ree R, van der Zee JS, Hoeksma-Kruize YC, Souverijn JH, et al. Long-Term Treatment of Intestinal Helminths Increases Mite Skin-Test Reactivity in Gabonese Schoolchildren. J Infect Dis (2004) 189(5):892–900. doi: 10.1086/381767
115. Medeiros M Jr., Figueiredo JP, Almeida MC, Matos MA, Araujo MI, Cruz AA, et al. Schistosoma Mansoni Infection Is Associated With a Reduced Course of Asthma. J Allergy Clin Immunol (2003) 111(5):947–51. doi: 10.1067/mai.2003.1381
116. Araujo MI, Hoppe B, Medeiros M Jr., Alcantara L, Almeida MC, Schriefer A, et al. Impaired T Helper 2 Response to Aeroallergen in Helminth-Infected Patients With Asthma. J Infect Dis (2004) 190(10):1797–803. doi: 10.1086/425017
117. Ponte EV, Rasella D, Souza-Machado C, Stelmach R, Barreto ML, Cruz AA. Reduced Asthma Morbidity in Endemic Areas for Helminth Infections: A Longitudinal Ecological Study in Brazil. J Asthma (2014) 51(10):1022–7. doi: 10.3109/02770903.2014.936454
118. Selassie FG, Stevens RH, Cullinan P, Pritchard D, Jones M, Harris J, et al. Total and Specific IgE (House Dust Mite and Intestinal Helminths) in Asthmatics and Controls From Gondar, Ethiopia. Clin Exp Allergy (2000) 30(3):356–8. doi: 10.1046/j.1365-2222.2000.00706.x
119. Dagoye D, Bekele Z, Woldemichael K, Nida H, Yimam M, Hall A, et al. Wheezing, Allergy, and Parasite Infection in Children in Urban and Rural Ethiopia. Am J Respir Crit Care Med (2003) 167(10):1369–73. doi: 10.1164/rccm.200210-1204OC
120. Scrivener S, Yemaneberhan H, Zebenigus M, Tilahun D, Girma S, Ali S, et al. Independent Effects of Intestinal Parasite Infection and Domestic Allergen Exposure on Risk of Wheeze in Ethiopia: A Nested Case-Control Study. Lancet (2001) 358(9292):1493–9. doi: 10.1016/S0140-6736(01)06579-5
121. Huang SL, Tsai PF, Yeh YF. Negative Association of Enterobius Infestation With Asthma and Rhinitis in Primary School Children in Taipei. Clin Exp Allergy (2002) 32(7):1029–32. doi: 10.1046/j.1365-2745.2002.01424.x
122. Palmer LJ, Celedon JC, Weiss ST, Wang B, Fang Z, Xu X. Ascaris Lumbricoides Infection is Associated With Increased Risk of Childhood Asthma and Atopy in Rural China. Am J Respir Crit Care Med (2002) 165(11):1489–93. doi: 10.1164/rccm.2107020
123. Cooper PJ, Chico ME, Bland M, Griffin GE, Nutman TB. Allergic Symptoms, Atopy, and Geohelminth Infections in a Rural Area of Ecuador. Am J Respir Crit Care Med (2003) 168(3):313–7. doi: 10.1164/rccm.200211-1320OC
124. Davey G, Venn A, Belete H, Berhane Y, Britton J. Wheeze, Allergic Sensitization and Geohelminth Infection in Butajira, Ethiopia. Clin Exp Allergy (2005) 35(3):301–7. doi: 10.1111/j.1365-2222.2005.02181.x
125. Ponte EV, Lima F, Araujo MI, Oliveira RR, Cardoso LS, Cruz AA. Skin Test Reactivity and Der P-Induced Interleukin 10 Production in Patients With Asthma or Rhinitis Infected With Ascaris. Ann Allergy Asthma Immunol (2006) 96(5):713–8. doi: 10.1016/S1081-1206(10)61070-8
126. Takeuchi H, Zaman K, Takahashi J, Yunus M, Chowdhury HR, Arifeen SE, et al. High Titre of Anti-Ascaris Immunoglobulin E Associated With Bronchial Asthma Symptoms in 5-Year-Old Rural Bangladeshi Children. Clin Exp Allergy (2008) 38(2):276–82. doi: 10.1111/j.1365-2222.2007.02890.x
127. Wordemann M, Diaz RJ, Heredia LM, Collado Madurga AM, Ruiz Espinosa A, Prado RC, et al. Association of Atopy, Asthma, Allergic Rhinoconjunctivitis, Atopic Dermatitis and Intestinal Helminth Infections in Cuban Children. Trop Med Int Health (2008) 13(2):180–6. doi: 10.1111/j.1365-3156.2007.01988.x
128. Alcantara-Neves NM, Veiga RV, Dattoli VC, Fiaccone RL, Esquivel R, Cruz AA, et al. The Effect of Single and Multiple Infections on Atopy and Wheezing in Children. J Allergy Clin Immunol (2012) 129(2):359–6767 e1–3. doi: 10.1016/j.jaci.2011.09.015
129. Jogi NO, Svanes C, Siiak SP, Logan E, Holloway JW, Igland J, et al. Zoonotic Helminth Exposure and Risk of Allergic Diseases: A Study of Two Generations in Norway. Clin Exp Allergy (2018) 48(1):66–77. doi: 10.1111/cea.13055
130. Ferreira MU, Rubinsky-Elefant G, de Castro TG, Hoffmann EH, da Silva-Nunes M, Cardoso MA, et al. Bottle Feeding and Exposure to Toxocara as Risk Factors for Wheezing Illness Among Under-Five Amazonian Children: A Population-Based Cross-Sectional Study. J Trop Pediatr (2007) 53(2):119–24. doi: 10.1093/tropej/fml083
131. Bager P, Vinkel Hansen A, Wohlfahrt J, Melbye M. Helminth Infection Does Not Reduce Risk for Chronic Inflammatory Disease in a Population-Based Cohort Study. Gastroenterology (2012) 142(1):55–62. doi: 10.1053/j.gastro.2011.09.046
132. Erb KJ. Can Helminths or Helminth-Derived Products be Used in Humans to Prevent or Treat Allergic Diseases? Trends Immunol (2009) 30(2):75–82. doi: 10.1016/j.it.2008.11.005
133. Flohr C, Quinnell RJ, Britton J. Do Helminth Parasites Protect Against Atopy and Allergic Disease? Clin Exp Allergy (2009) 39(1):20–32. doi: 10.1111/j.1365-2222.2008.03134.x
134. Zakeri A, Borji H, Haghparast A. Interaction Between Helminths and Toll-Like Receptors: Possibilities and Potentials for Asthma Therapy. Int Rev Immunol (2016) 35(3):219–48. doi: 10.3109/08830185.2015.1096936
135. Singh R, Alape D, de Lima A, Ascanio J, Majid A, Gangadharan SP. Regulatory T Cells in Respiratory Health and Diseases. Pulm Med (2019) 2019:1907807. doi: 10.1155/2019/1907807
136. Carvalho EM, Bastos LS, Araujo MI. Worms and Allergy. Parasite Immunol (2006) 28(10):525–34. doi: 10.1111/j.1365-3024.2006.00894.x
137. Maizels RM. Infections and Allergy - Helminths, Hygiene and Host Immune Regulation. Curr Opin Immunol (2005) 17(6):656–61. doi: 10.1016/j.coi.2005.09.001
138. Schwartz C, Hams E, Fallon PG. Helminth Modulation of Lung Inflammation. Trends Parasitol (2018) 34(5):388–403. doi: 10.1016/j.pt.2017.12.007
139. Allen JE, Wynn TA. Evolution of Th2 Immunity: A Rapid Repair Response to Tissue Destructive Pathogens. PLoS Pathog (2011) 7(5):e1002003. doi: 10.1371/journal.ppat.1002003
140. Feary JR, Venn AJ, Mortimer K, Brown AP, Hooi D, Falcone FH, et al. Experimental Hookworm Infection: A Randomized Placebo-Controlled Trial in Asthma. Clin Exp Allergy (2010) 40(2):299–306. doi: 10.1111/j.1365-2222.2009.03433.x
141. Wu GY, Halim MH. Schistosomiasis: Progress and Problems. World J Gastroenterol (2000) 6(1):12–9. doi: 10.3748/wjg.v6.i1.12
142. Itami DM, Oshiro TM, Araujo CA, Perini A, Martins MA, Macedo MS, et al. Modulation of Murine Experimental Asthma by Ascaris Suum Components. Clin Exp Allergy (2005) 35(7):873–9. doi: 10.1111/j.1365-2222.2005.02268.x
143. Cruz AA, Cooper PJ, Figueiredo CA, Alcantara-Neves NM, Rodrigues LC, Barreto ML. Global Issues in Allergy and Immunology: Parasitic Infections and Allergy. J Allergy Clin Immunol (2017) 140(5):1217–28. doi: 10.1016/j.jaci.2017.09.005
144. Smallwood TB, Giacomin PR, Loukas A, Mulvenna JP, Clark RJ, Miles JJ. Helminth Immunomodulation in Autoimmune Disease. Front Immunol (2017) 8:453. doi: 10.3389/fimmu.2017.00453
145. Cardoso LS, Oliveira SC, Goes AM, Oliveira RR, Pacifico LG, Marinho FV, et al. Schistosoma Mansoni Antigens Modulate the Allergic Response in a Murine Model of Ovalbumin-Induced Airway Inflammation. Clin Exp Immunol (2010) 160(2):266–74. doi: 10.1111/j.1365-2249.2009.04084.x
146. Marinho FV, Alves CC, de Souza SC, da Silva CM, Cassali GD, Oliveira SC, et al. Schistosoma Mansoni Tegument (Smteg) Induces IL-10 and Modulates Experimental Airway Inflammation. PLoS One (2016) 11(7):e0160118. doi: 10.1371/journal.pone.0160118
147. Yang J, Zhao J, Yang Y, Zhang L, Yang X, Zhu X, et al. Schistosoma Japonicum Egg Antigens Stimulate CD4 CD25 T Cells and Modulate Airway Inflammation in a Murine Model of Asthma. Immunology (2007) 120(1):8–18. doi: 10.1111/j.1365-2567.2006.02472.x
148. Ren J, Hu L, Yang J, Yang L, Gao F, Lu P, et al. Novel T-Cell Epitopes on Schistosoma Japonicum SjP40 Protein and Their Preventive Effect on Allergic Asthma in Mice. Eur J Immunol (2016) 46(5):1203–13. doi: 10.1002/eji.201545775
149. Zhang W, Li L, Zheng Y, Xue F, Yu M, Ma Y, et al. Schistosoma Japonicum Peptide SJMHE1 Suppresses Airway Inflammation of Allergic Asthma in Mice. J Cell Mol Med (2019) 23(11):7819–29. doi: 10.1111/jcmm.14661
150. Pitrez PM, Gualdi LP, Barbosa GL, Sudbrack S, Ponzi D, Cao RG, et al. Effect of Different Helminth Extracts on the Development of Asthma in Mice: The Influence of Early-Life Exposure and the Role of IL-10 Response. Exp Parasitol (2015) 156:95–103. doi: 10.1016/j.exppara.2015.06.004
151. Lima C, Perini A, Garcia ML, Martins MA, Teixeira MM, Macedo MS. Eosinophilic Inflammation and Airway Hyper-Responsiveness are Profoundly Inhibited by a Helminth (Ascaris Suum) Extract in a Murine Model of Asthma. Clin Exp Allergy (2002) 32(11):1659–66. doi: 10.1046/j.1365-2222.2002.01506.x
152. McConchie BW, Norris HH, Bundoc VG, Trivedi S, Boesen A, Urban JF Jr., et al. Ascaris Suum-Derived Products Suppress Mucosal Allergic Inflammation in an Interleukin-10-Independent Manner via Interference With Dendritic Cell Function. Infect Immun (2006) 74(12):6632–41. doi: 10.1128/IAI.00720-06
153. Melendez AJ, Harnett MM, Pushparaj PN, Wong WS, Tay HK, McSharry CP, et al. Inhibition of Fc Epsilon RI-Mediated Mast Cell Responses by ES-62, a Product of Parasitic Filarial Nematodes. Nat Med (2007) 13(11):1375–81. doi: 10.1038/nm1654
154. Schnoeller C, Rausch S, Pillai S, Avagyan A, Wittig BM, Loddenkemper C, et al. A Helminth Immunomodulator Reduces Allergic and Inflammatory Responses by Induction of IL-10-Producing Macrophages. J Immunol (2008) 180(6):4265–72. doi: 10.4049/jimmunol.180.6.4265
155. Rzepecka J, Coates ML, Saggar M, Al-Riyami L, Coltherd J, Tay HK, et al. Small Molecule Analogues of the Immunomodulatory Parasitic Helminth Product ES-62 Have Anti-Allergy Properties. Int J Parasitol (2014) 44(9):669–74. doi: 10.1016/j.ijpara.2014.05.001
156. McSorley HJ, O’Gorman MT, Blair N, Sutherland TE, Filbey KJ, Maizels RM. Suppression of Type 2 Immunity and Allergic Airway Inflammation by Secreted Products of the Helminth Heligmosomoides Polygyrus. Eur J Immunol (2012) 42(10):2667–82. doi: 10.1002/eji.201142161
157. McSorley HJ, Blair NF, Smith KA, McKenzie AN, Maizels RM. Blockade of IL-33 Release and Suppression of Type 2 Innate Lymphoid Cell Responses by Helminth Secreted Products in Airway Allergy. Mucosal Immunol (2014) 7(5):1068–78. doi: 10.1038/mi.2013.123
158. Trujillo-Vargas CM, Werner-Klein M, Wohlleben G, Polte T, Hansen G, Ehlers S, et al. Helminth-Derived Products Inhibit the Development of Allergic Responses in Mice. Am J Respir Crit Care Med (2007) 175(4):336–44. doi: 10.1164/rccm.200601-054OC
159. Lee KH, Park HK, Jeong HJ, Park SK, Lee SJ, Choi SH, et al. Immunization of Proteins From Toxascaris Leonina Adult Worm Inhibits Allergic Specific Th2 Response. Vet Parasitol (2008) 156(3-4):216–25. doi: 10.1016/j.vetpar.2008.06.016
160. Jeong YI, Kim SH, Ju JW, Cho SH, Lee WJ, Park JW, et al. Clonorchis Sinensis-Derived Total Protein Attenuates Airway Inflammation in Murine Asthma Model by Inducing Regulatory T Cells and Modulating Dendritic Cell Functions. Biochem Biophys Res Commun (2011) 407(4):793–800. doi: 10.1016/j.bbrc.2011.03.102
161. Kim SE, Kim JH, Min BH, Bae YM, Hong ST, Choi MH. Crude Extracts of Caenorhabditis Elegans Suppress Airway Inflammation in a Murine Model of Allergic Asthma. PLoS One (2012) 7(4):e35447. doi: 10.1371/journal.pone.0035447
162. Ebner F, Hepworth MR, Rausch S, Janek K, Niewienda A, Kuhl A, et al. Therapeutic Potential of Larval Excretory/Secretory Proteins of the Pig Whipworm Trichuris Suis in Allergic Disease. Allergy (2014) 69(11):1489–97. doi: 10.1111/all.12496
163. Ji P, Hu H, Yang X, Wei X, Zhu C, Liu J, et al. AcCystatin, an Immunoregulatory Molecule From Angiostrongylus Cantonensis, Ameliorates the Asthmatic Response in an Aluminium Hydroxide/Ovalbumin-Induced Rat Model of Asthma. Parasitol Res (2015) 114(2):613–24. doi: 10.1007/s00436-014-4223-z
164. Navarro S, Pickering DA, Ferreira IB, Jones L, Ryan S, Troy S, et al. Hookworm Recombinant Protein Promotes Regulatory T Cell Responses That Suppress Experimental Asthma. Sci Transl Med (2016) 8(362):362ra143. doi: 10.1126/scitranslmed.aaf8807
165. Finlay CM, Stefanska AM, Coleman MM, Jahns H, Cassidy JP, McLoughlin RM, et al. Secreted Products of Fasciola Hepatica Inhibit the Induction of T Cell Responses That Mediate Allergy. Parasite Immunol (2017) 39(10). doi: 10.1111/pim.12460
166. de Almeida T, Fernandes JS, Lopes DM, Andrade LS, Oliveira SC, Carvalho EM, et al. Schistosoma Mansoni Antigens Alter Activation Markers and Cytokine Profile in Lymphocytes of Patients With Asthma. Acta Trop (2017) 166:268–79. doi: 10.1016/j.actatropica.2016.12.002
Keywords: allergy, asthma, BCG, helminths, immunotherapy, mycobacteria, Th1/Th2, vaccines
Citation: Abdelaziz MH, Ji X, Wan J, Abouelnazar FA, Abdelwahab SF and Xu H (2021) Mycobacterium-Induced Th1, Helminths-Induced Th2 Cells and the Potential Vaccine Candidates for Allergic Asthma: Imitation of Natural Infection. Front. Immunol. 12:696734. doi: 10.3389/fimmu.2021.696734
Received: 17 April 2021; Accepted: 19 July 2021;
Published: 03 August 2021.
Edited by:
Pere-Joan Cardona, Hospital Universitari Germans Trias i Pujol, SpainReviewed by:
Mohamed Ahmed El-Mokhtar, Assiut University, EgyptRicardo Wesley Alberca, University of São Paulo, Brazil
Copyright © 2021 Abdelaziz, Ji, Wan, Abouelnazar, Abdelwahab and Xu. This is an open-access article distributed under the terms of the Creative Commons Attribution License (CC BY). The use, distribution or reproduction in other forums is permitted, provided the original author(s) and the copyright owner(s) are credited and that the original publication in this journal is cited, in accordance with accepted academic practice. No use, distribution or reproduction is permitted which does not comply with these terms.
*Correspondence: Huaxi Xu, xuhx@ujs.edu.cn; orcid.org/0000-0002-2568-7393; Sayed F. Abdelwahab, icpminia@yahoo.com; sayed.awahab@mu.edu.eg; orcid.org/0000-0002-9636-7485