- 1Translational Cell Therapy Research Group, Department of Clinical Sciences, Intervention and Technology (CLNTEC), Division of Pediatrics, Karolinska Institutet, Stockholm, Sweden
- 2Berlin Institute of Health (BIH) Center for Regenerative Therapies (BCRT) and Berlin-Brandenburg School for Regenerative Therapies (BSRT), Berlin, Germany
- 3Department of Nephrology and Internal Intensive Care Medicine, All Charité Universitätsmedizin Berlin, Corporate Member of Freie Universität Berlin and Humboldt-Universität zu Berlin, Berlin, Germany
- 4Department of Women’s and Children’s Health, Karolinska Institutet, Stockholm, Sweden
Mesenchymal stromal cells (MSCs) possess profound immunomodulatory and regenerative properties that are of clinical use in numerous clinical indications with unmet medical need. Common sources of MSCs include among others, bone marrow (BM), fat, umbilical cord, and placenta-derived decidua stromal cells (DSCs). We here summarize our more than 20-years of scientific experience in the clinical use of MSCs and DSCs in different clinical settings. BM-MSCs were first explored to enhance the engraftment of autografts in hematopoietic cell transplantation (HCT) and osteogenesis imperfecta around 30 years ago. In 2004, our group reported the first anti-inflammatory use of BM-MSCs in a child with grade IV acute graft-versus-host disease (GvHD). Subsequent studies have shown that MSCs appear to be more effective in acute than chronic GvHD. Today BM-MSC-therapy is registered for acute GvHD in Japan and for GvHD in children in Canada and New Zeeland. MSCs first home to the lung following intravenous injection and exert strong local and systemic immunomodulatory effects on the host immune system. Thus, they were studied for ameliorating the cytokine storm in acute respiratory distress syndrome (ARDS). Both, MSCs and DSCs were used to treat SARS-CoV-2 coronavirus-induced disease 2019 (COVID-19)-induced ARDS. In addition, they were also used for other novel indications, such as pneumomediastinum, colon perforation, and radiculomyelopathy. MSC and DSCs trigger coagulation and were thus explored to stop hemorrhages. DSCs appear to be more effective for acute GvHD, ARDS, and hemorrhages, but randomized studies are needed to prove superiority. Stromal cell infusion is safe, well tolerated, and only gives rise to a slight fever in a limited number of patients, but no major side effects have been reported in multiple safety studies and metaanalysis. In this review we summarize current evidence from in vitro studies, animal models, and importantly our clinical experience, to support stromal cell therapy in multiple clinical indications. This encloses MSC’s effects on the immune system, coagulation, and their safety and efficacy, which are discussed in relation to prominent clinical trials within the field.
Introduction
Mesenchymal stromal cells (MSCs) were first described by Friedenstein and co-workers (1). MSCs are rare precursor cells that can be found in the bone marrow (BM) and all vascularized tissues in the body, such as adipose tissue (AT) and perinatal tissues (PT) (2, 3). They cannot regenerate and maintain a whole tissue compartment and are therefore not considered true stem cells (4). Many early studies used MSCs in regenerative medicine because of their capacity to differentiate into several mesenchymal tissue lineages, such as cartilage, bone, tendon, muscle and fat (5, 6).
However, it has recently become clear that MSCs exert their beneficial effects mainly through paracrine mechanisms (7). This entails both, cell contact-dependent and independent mechanisms, e.g. the secretion of various immunomodulatory and regenerative mediators that polarize the host immune system and promote tissue repair (8). MSCs suppress lymphocyte proliferation in mixed lymphocyte cultures (MLCs) in vitro and prolong skin allograft survival in vivo (9). Koc et al. demonstrated that autologous MSCs are safe to infuse in doses of 1-5x106 cells/kg in patients who have undergone autologous hematopoietic cell transplantation (HCT) for breast cancer (10).
These investigations inspired us to apply MSCs in a 9-year-old boy with acute lymphoblastic leukemia in third remission, who had received HCT from an HLA-matched unrelated female donor. The boy had developed severe grade IV acute graft-versus-host disease (GvHD) that did not respond to cyclosporine, prednisolone, methylprednisolone, extracorporeal psoralen with ultraviolet light, Infliximab/Daclizumab. He was deteriorating, presented with bloody diarrhea 20 times/day, and had a bilirubin of 250 mmol/L. After 4 weeks we had expanded enough BM-MSCs from his mother to employ a dose of 2x106 cells/kg. After MSCs infusion the patient had a dramatic response with normal stools and normal bilirubin within a week (11). This was followed by a BM-MSC pilot study in 8 patients with severe acute GvHD at our center (12) and a European multicenter study (13).
MSCs have been investigated in a large number of trials for acute GvHD since then (14). For severe acute GvHD, MSCs were more encouraging in children than adults (13, 15). BM-MSCs are now registered as a therapy for acute GvHD in Japan and for children in Canada and New Zeeland (16). Despite preliminary encouraging findings, controlled trials did not show significant efficacy of using BM-MSCs for the treatment of steroid-refractory acute GvHD (17). In this review article, we summarize our scientific and clinical experience on the use of BM-MSCs and placenta-derived DSCs in different clinical indications in relation to prominent clinical trials (Figure 1), entailing studies in HCT, GvHD, hemorrhagic cystitis, acute respiratory distress syndrome (ARDS) and coronavirus-induced disease 2019 (COVID-19).
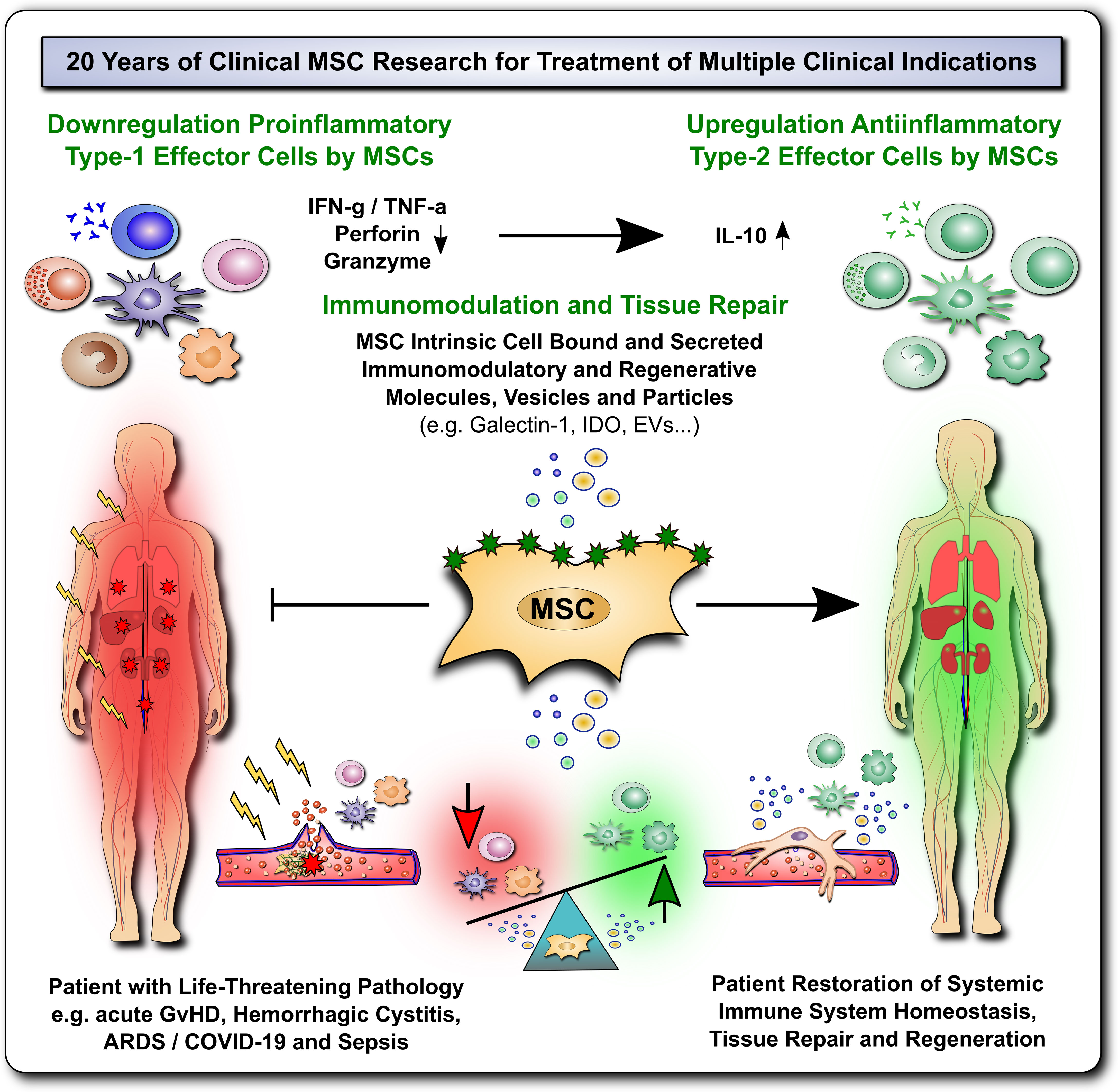
Figure 1 20 Years of Clinical MSC Research for Treatment for Multiple Life-Threatening Clinical Indications. The past 20 years of clinical mesenchymal stromal cell (MSC) research have shown that these cells can elicit profound immunomodulation and tissue repair upon therapeutic transfer into severely ill patients with multiple life-threatening clinical indications, such as acute Graft-versus-Host Disease (GvHD), Hemorrhagic Cystitis, Acute Respiratory Distress Syndrome (ARDS), Coronavirus 2019 Induced Disease (COVID-19) and Sepsis. This presumably occurs due to down-regulation of proinflammatory type-1 effector cells and upregulation of anti-inflammatory type-2 effector cells by MSCs through multiple MSC intrinsic cell bound and secreted immunomodulatory and regenerative mediators that restore and promote the patients' immune system homeostasis, tissue repair and regeneration.
MSC Immunomodulation and Immunological Properties
The immunomodulatory and immunological properties of MSCs are diverse and affect almost every major arm of the human immune system (Figures 1–3). They have been reviewed earlier (8). We here only briefly summarize this important aspect of MSC biology.
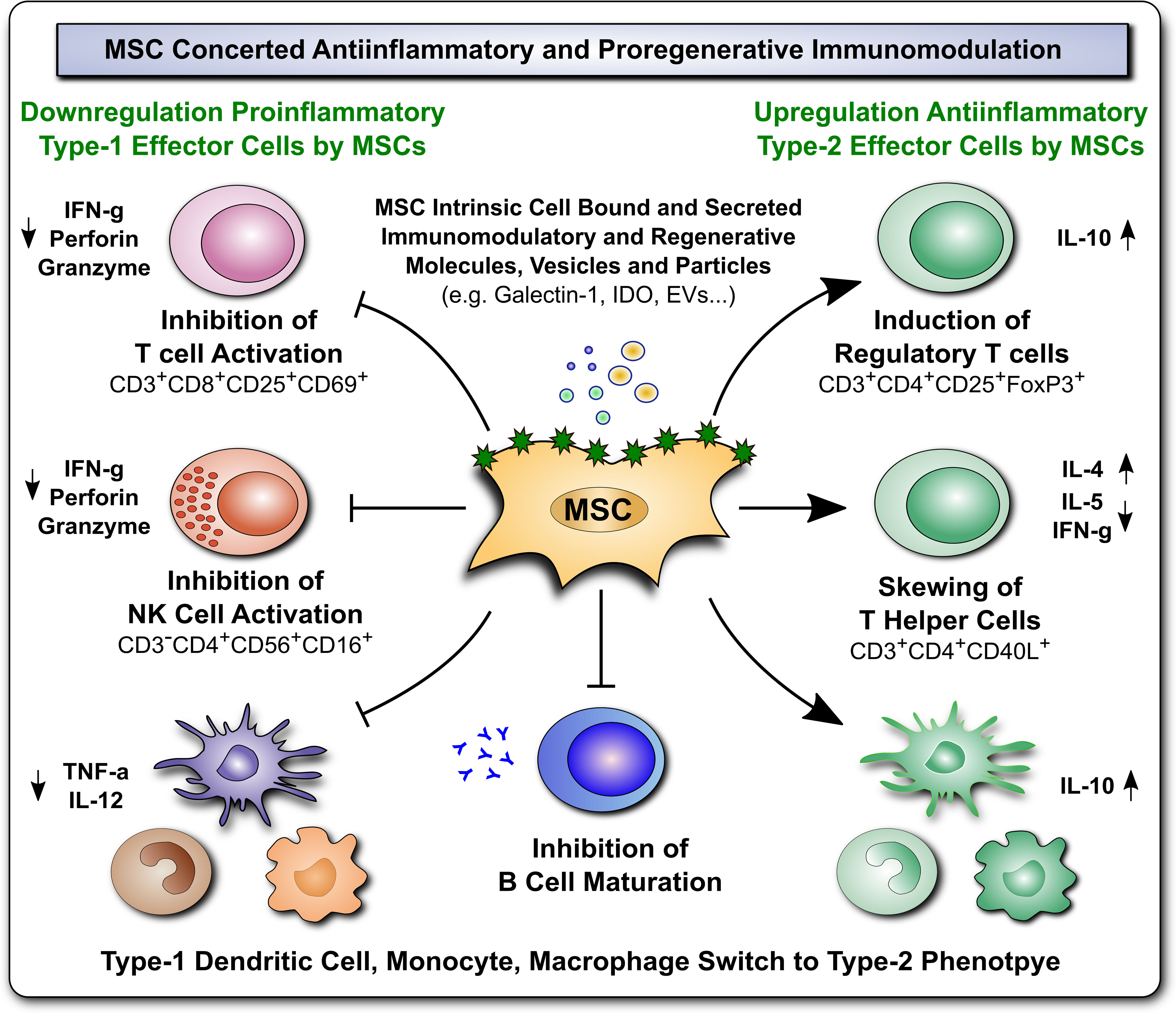
Figure 2 Immunomodulatory and Regenerative Properties of MSCs. Mesenchymal stromal cells (MSCs) employ a broad array of antiinflammatory and proregenerative immunomodulatory mechanisms, which are exerted either by MSCs directly, through intrinsic cell associated/bound, or secreted immunomodulatory and regenerative molecules, vesicles/particles (e.g. Galectin-1, indoleamine-2,3-dioxygenase (IDO), and extracellular vesicles, (EVs), respectively), or alternatively indirectly through MSCmediated favorable polarization of innate and adaptive immune responses (e.g. downregulation of proinflammatory type-1 effector cells, such as inhibition of T and NK cell activation, and upregulation of antiinflammatory type 2 effector cells, such as induction of regulatory T cells, skewing of T helper cells, and switch in type 1 dendritic cell, monocyte, and macrophage phages to type 2 phenotype, and inhibition of B cell maturation), that both lead to favorable changes in the predominant cytokine milieu (e.g. downregulation of proinflammatory mediators TNF-a, IFN-g, perforin and granzyme, but induction of antiinflammatory IL-10, in conjunction with secretion of multiple trophic and regenerative factors, not shown here) that altogether mediate beneficial tissue repair and proregenerative responses.
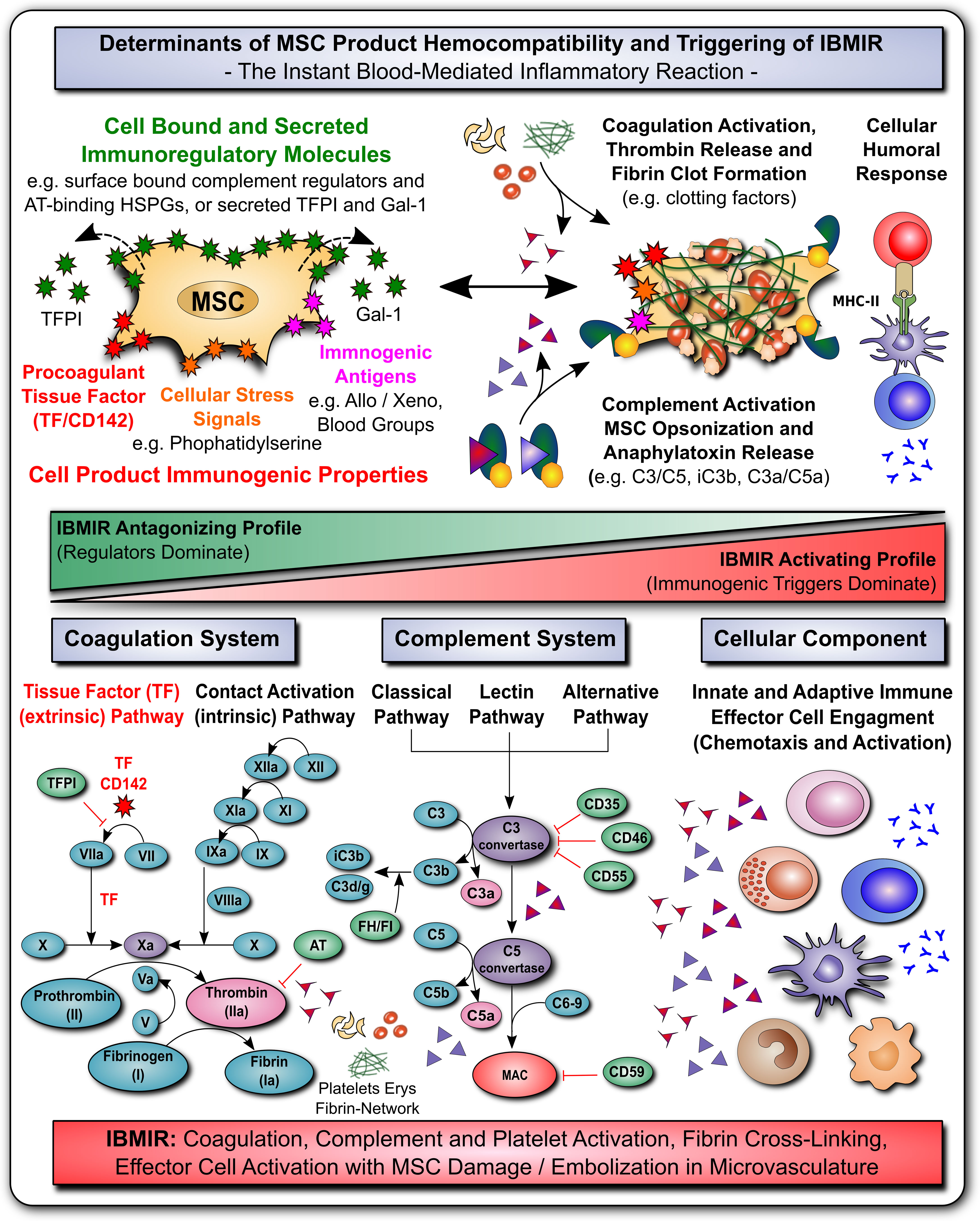
Figure 3 MSC Determinants of Immunogenicity, Hemocompatibility and Interaction with the Innate Immune Cascade Systems. (A) The safety and efficacy of infused mesenchymal stromal cell (MSC) products depends on their hemocompatibility profile and concomitant triggering of the instant blood-mediated inflammatory reaction (IBMIR). In analogy to their immunomodulatory features, the cells display a broad array of either regulatory elements or immunogenic triggering factors (e.g. cell bound or secreted regulators of complement and coagulation cascade and cellular immunity). A tight balance between triggering and regulatory elements is decisive for the triggering of IBMIR (incompatibility with blood) or prevention thereof (hemocompatibility). While multiple blood regulatory elements employed by MSCs prevent blood activation akin to mechanism employed by hemocompatible endothelial cells, therapeutic MSC products can also display varying levels of immunogenic triggers, such as highly procoagulant tissue factor (TF/CD142), cellular stress signals (e.g. cell surface exposure of complement and coagulation activating phosphatidylserine resulting from membrane asymmetry upon freeze-thawing), and immunogenic antigens (e.g. allo-, xeno-, and blood group antigens). If these triggers prevail over the regulatory elements, blood-incompatible MSC products that are introduced into the blood stream can trigger the IBMIR, entailing the activation of complement, coagulation, and cellular immune responses, which may compromise MSC product safety and functionality. (B) Considering the coagulation cascade, regulatory elements entail amongst others the secreted tissue factor pathway inhibitor (TFPI) and surface localized heparan-sulfate proteoglycans (HSPGs) that bind antithrombin (AT), which are both strong negative-regulators of the highly procoagulant thrombin (activator of fibrin and platelets). Thrombin is formed upon triggering of the clotting cascade by activation of the extrinsic tissue factor pathway of coagulation (initiated by conversion of factor FVII to FVIIa), or the intrinsic contact activation pathway of coagulation (initiated through conversion of FXII to FXIIa, e.g. upon blood exposure of highly negatively charged basement membrane contained collagen residues). Both arms of the coagulation cascade converge where FX is turned into FXa, that promotes the conversion of prothrombin to thrombin, which in turn elicits conversion of fibrinogen to fibrin, that will form the fibrin clot through cross-linking fibrin fibers, and incorporating activated platelets, erythrocytes, and nucleated white blood cells. (C) Considering the complement cascade, regulatory elements entail the cell surface bound complement regulators CD35, CD46, CD55, CD59 and the secreted complement regulators factor H and I (FH/FI), that can regulate the cascade at different steps, as indicated in the figure, and may thus prevent formation of the final membrane attack complex (MAC) albeit initiation of the earlier steps of complement cascade activation. Activation of the complement cascade can occur via the classical, lectin, and alternative pathways of coagulation, triggered among others via recognition of aberrant cell surface features (e.g. phosphatidylserine exposure upon freeze-thawing) or bound immunoglobulins via C1q (classical pathway), which is then transmitted through activation of the central complement components 3 and 5 (C3/C5), with concomitant formation of cell surface bound opsonins C3b/iC3b/C3dg and soluble chemotactic anaphylatoxins C3a and C5a, that can attract and activate various types of nucleated effector cells, such as T, NK, B cells and various phagocytes (e.g. PMNs, monocytes, macrophages and dendritic cells). (D) Considering the cellular component, as shown in Figures 1 and 2, MSC possess very potent immunoregulatory features to modulate adaptive and humoral branches of cellular immunity, that may however be compromised or skewed in an unfavorable direction if IBMIR-mediated killing of infused cells occurs to rapidly for the cells to exert their beneficial effects (e.g. induction of cellular humoral response and alloimunization in response to third-party cells). Overall, the triggering of IBMIR by infused therapeutic cell products may lead to coagulation, complement, and platelet activation, fibrin-cross-linking, and clot formation, with concomitant effector cell activation, and consecutive MSC damage and embolization in the microvasculature.
First of all, MSCs exhibit profound immunomodulation of both the adaptive and innate immune system, which is an integral part of their regenerative and therapeutic properties (8). Initial in vitro studies documented that MSCs’ inhibitory effect in alloantigen and mitogen-activated mixed lymphocyte culture (MLCs) did not depend on human leukocyte antigen (HLA)-compatibility between responder cells and MSCs (18). Interestingly, the cells also inhibit MLCs after differentiation to bone or cartilage (19). Indeed, it is a common property of different types of stromal cells from various tissue sources, including placenta-derived decidua stromal cells (DSCs) and fibroblasts, that these cells can inhibit MLCs (20–22). However, differences in intensity/quality of suppression may be apparent.
On a cellular level, CD4+ CD25+ regulatory T cells (Tregs) and IL-10 production are increased by both MSCs and DSCs (23–27). Type I dendritic cells decrease their TNF-α and IL-12 production when co-cultured with MSCs, and MSCs promote IL-10 secretion by LPS-stimulated type 2 dendritic cells. Both, MSCs and DSCs produce numerous immunomodulatory and immunosuppressive factors (8, 27), including HLA-G5 (28), prostaglandin E2 (23), several Galectin’s (e.g. Galectin-1) (29), and indoleamine-2,3-dioxygenase (IDO) (30). IDO inhibits T cells by conversion of tryptophan to kynurenine (31). MSCs can inhibit MLCs by soluble factors and also by direct contact with lymphocytes (27).
DSCs mainly suppress lymphocyte proliferation through both paracrine mechanisms and cell-to-cell contact (27, 32). Importantly, DSCs from the placental fetal membrane induce substantially stronger inhibition of MLCs compared to BM-MSCs and other sources of stromal cells from the placenta (33), which may also relate to their strong potency to modulate immune responses in vivo (7, 34). Apart from the secretion of soluble mediators, their direct contact-dependent effects include activation of the PD-1 pathway (35) and the activation of VCAM-1 and ICAM-1 (36). MSCs upregulate CD39 and adenosine production to suppress activated T cells (37) and they also induce Fas-mediated T cell apoptosis (38).
Sophisticated mechanistic studies by Galleu et al. have shown that allogeneic MSCs undergo perforin-dependent apoptosis by recipient cytotoxic T-cells after infusion, to reverse GvHD in a mouse model and this may also be part of their MoA in respective patients (39). Recipient phagocytes engulf apoptotic MSCs and produce IDO, necessary for suppression and to prevent GvHD (40). BM-MSCs produce exosomes with immunosuppressive and homing abilities (41). Exosomes from MSCs were also shown to be able to suppress and reverse acute GvHD (42). As opposed to BM-MSC, DSCs do not need IFN-γ licensing to be activated and show its immunomodulatory effect (43).
From an immunophenotypic point, MSCs are negative for common hematopoietic, myeloid, and endothelial markers (e.g. CD34, CD45, CD14, and CD31) and they are positive for a panel of commonly expressed markers (e.g. CD29, CD73, CD90, CD105, and CD166) (44, 45). Indeed, there is no specific commonly accepted surface marker for MSCs thoroughly established yet. DSCs have a similar immunophenotype as BM-MSCs (e.g. positive for CD29, CD73, CD105, and absence of CD34, etc) with only minor differences in surface antigen expression. These entail higher expression of PDL-1, PDL-2, and complement regulatory molecule CD55 (DAF, decay-accelerating factor) and procoagulant tissue factor (TF/CD142) (44). The latter two have an impact on hemocompatibility and triggering of the instant blood mediated inflammatory reaction (IBMIR; see below) (44, 46–49).
Initial studies suggested that MSCs are immune-privileged and do not induce strong immune stimulation (9, 19, 20). A recent review suggested that MSCs are rather immune evasive than truly immune privileged (50). MSCs express regular levels of HLA class I molecules and only low to absent levels of HLA class II. Both can be upregulated by inflammatory mediators, such as IFN-γ. Fas-ligand and co-stimulatory molecules, B-71, B-72, CD40, and CD40L are not expressed (20). DSCs only express HLA class I, and exposure to IFN-γ does not induce HLA class II expression (33).
Nonetheless, xenogeneic, and allogeneic MSCs/DSCs are rejected (51–53). After systemic infusion, neither MSCs nor DSCs are long-lived and are found in the circulation only shortly after infusion, partly due to a lung-entrapment effect (10, 53, 54). Only low levels of gene-marked MSCs were found in the BM of children with osteogenesis imperfecta (OI) (55). In a baboon model alloantibodies were induced by allogeneic MSCs (56). However, we did not detect any anti-HLA antibodies in 12 GvHD patients treated with BM-MSCs (57). Similarly, DSCs did not induce alloantibodies in patients with GvHD (58).
MSC Hemocompatibility and Innate Immune Cascade Systems
The majority of clinical applications involving both MSCs and DSCs employ systemic infusion as a mode of clinical delivery (46, 59, 60). Thus, it is of crucial importance to study hemocompatibility aspects of the infused cell products (46–48, 61–66) and to understand their interaction with the innate immune defense cascade system, which are crucial for the processes of hemostasis and thrombosis. Therapeutic stromal cells can impact on existing pathology, such as hemorrhages, by modulating these cascade systems in vivo (60, 67–69). Thus, hemocompatibility aspects impact both safety and efficacy (Figure 3) (46–48, 61, 70).
The triggering of instant immune responses to stromal and other cell products infused into the bloodstream has been summarized under the term “IBMIR” – The instant blood-mediated inflammatory reaction (49). The intensity of IBMIR-triggering differs between stromal cell products from different sources and is influenced by several manufacturing parameters, such as their degree of in vitro expansion and cryopreservation. There are high variations between tissue sources and minimal expanded MSCs trigger less IBMIR than highly expanded cell products. Freeze-thawed cells trigger more IBMIR than fresh, culture-derived cells, which may correlate to some degree with their safety and efficacy profile (7, 45–47, 49, 62, 71–73).
The molecular mechanisms underlying the regulation of the innate immune cascade systems and their crosstalk with infused cells is highly complex and interlinked, involving a multitude of factors and crosstalk/feedback mechanisms that are hard to predict (70). Thus, hemocompatibility assessment of MSC products requires whole blood exposure in vitro and in vivo testing to understand the ultimate outcome (46–48). The complement, coagulation, and fibrinolytic cascades involve >30 soluble and cell bound molecules (Figure 3). This includes the primary cascade factors and a multitude of regulatory elements at different steps of the cascade, from the initial triggering or starting point to the final effector pathways, with the highly complex interaction between the three primary systems (70).
The coagulation system is primarily initiated/triggered via two effector pathways: 1) The Tissue Factor (TF/CD142) Pathway and 2) The Contact Activation Pathway, involving the recognition of blood exposed TF/CD142 and highly negatively charged collagen residues by coagulation factors VII and XII, respectively. We have studied in detail the pro- and anti-coagulant mechanisms/features employed by MSCs from different tissue sources (46). This includes their differential expression of highly procoagulant TF/CD142 (e.g. lowest to highest expression for BM-MSCs, PT-MSCs, and AT-MSCs, respectively) (46, 48) and collagens as typical pro-coagulant effectors, their expression of regulatory elements, such as tissue factor pathway inhibitor (TFPI) and prostacyclin (PGI2; a potent inhibitor of platelet activation), and surface expression of antithrombin-binding heparan sulfate proteoglycans (44, 49, 62, 74). Although principle generalizations can be made, each cell product may differ due to different underlying manufacturing processes, and may thus have to be assessed independently to study its distinct hemocompatibility profile (48). This is further impacted by the treatment indication and underlying coagulopathies/anticoagulation regimens (47, 62, 75–77).
Another key cascade system is the complement system, which is primarily triggered through three pathways: 1) The Classical Pathway (e.g. triggered by C1q), 2) The Lectin Pathway (e.g. triggered by MBL), and 3) The Alternative Pathway (“Tickover”). The complement system can recognize aberrant cell surface features of infused MSCs that are unfamiliar to the whole blood environment (45, 71, 72, 74, 78–81) when their complement regulatory features are insufficient to counterbalance activation (78, 80, 81). Thawed cells directly derived from cryostorage before clinical use can exhibit cell membrane asymmetry and phosphatidylserine exposure, which can be recognized by C1q/MBL (45, 71, 74). Cell surface binding of immunoglobulins of various specificities (e.g. towards immunogenic blood type ABO antigens, foreign donor alloantigen, or xenogeneic supplements/fetal calf serum) can promote complement activation (71, 74, 79). In addition, MSCs exhibit potent fibrinolytic properties through their expression of a diverse array of matrix metalloproteinases (46).
Safety and Studies on Potential Side Effects of Stromal Cells
Stromal cells are used clinically in doses ranging from 1 to 10 x 106 cells/kg. Three large meta-analyses including thousands of BM-MSC-treated patients found only a slight increase in body temperature upon MSCs infusion in a few patients (82–84). In several well-controlled clinical studies, there was no other major infusion-related toxicity. While administration of third-party MSCs can occasionally induce minimal allo-immunization in an immunocompetent host, no major severe adverse events and no major infusion-related toxicity have been reported with BM-MSCs (82–84).
Among patients receiving 88 infusions of placenta-derived DSCs, there were three transient, spontaneously reversible events (fever, dyspnea, and vertigo) (60). However, we did not find any thromboembolic events in patients treated with DSCs (60). In addition, in a comprehensive prospective safety and toxicity study in rats and mice using human DSCs, no thrombosis, organ damage, or dysfunction and toxicity on vital organs (e.g. liver, kidney, and lung) was observed with doses as high as 40x106 cells/kg (53).
This may be due to highly optimized DSC application procedures, involving several important safety measures, such as removal of larger cell aggregates by filtration through a synthetic micromesh (70μm) before cell injection, heparinized syringes, prophylactic anticoagulation in patients and animals, and substitution of immunogenic AB-plasma with nonimmunogenic human serum albumin (7, 34, 46, 48, 60, 69, 71, 79).
Nonetheless, cases of thromboembolic events (e.g. compromised hemocompatibility, micro-and macro-thrombosis, and thromboembolism) in response to highly procoagulant TF/CD142 expressing MSC products in conjunction with suboptimal application routines have been reported in the past and call for increased caution when employing AT- and UC-derived MSCs products with high TF/CD142 expression (48, 85–89).
Furthermore, three fairly recent meta-analyses found no association with MSCs therapy and organ system complications, infections, deaths, or malignancies (82–84). Autopsy of 18 patients treated with BM-MSCs following HCT showed no ectopic tissue formation (45, 90) when treated with fresh-from-culture-derived or cryopreserved freeze-thawed MSCs (45, 71).
Various sources of MSCs and DSCs have been used for medical treatment and they have some differences in features, e.g. DSCs are only half the size compared to BM-MSCs and do not differentiate well to the bone, cartilage, and fat as do BM-MSCs (44, 54). This may reduce microvascular entrapment and the risk for ectopic tissue formation. Pilot studies and meta-analyses support the safety of both MSCs and DSCs. In contrast to pharmacological immunosuppressive drugs, stromal cells have very little if any side effects or toxicity.
Co-infusion of MSCs in HCT
In 46 HLA-identical sibling transplants, MSCs were infused at the time of transplant (91). Graft failure was not seen in any of the patients and grades III-IV acute GvHD was noted in 15% of patients. Ball et al. co-infused MSCs in children given HLA-haploidentical grafts (92) and none of the patients experienced graft failure compared to 10% among retrospective controls. We infused MSCs to enhance the engraftment of HCT in seven patients (93). Three of them were re-grafted for previous graft failure. Neutrophil counts >0.5x109/L and platelet counts >30x109/L were both achieved at a medium of 12 days. All patients achieved 100% donor chimerism.
Co-transplantation of MSCs was compared to placebo in a prospective randomized study in HCT patients (94). Engraftment of neutrophils and platelets was similar in the two groups. There was a decreased risk of acute GvHD and an increased probability of leukemic relapse in the group receiving MSCs. Two children were given haploidentical MSCs and HLA-identical sibling HCT (95). The two patients with previous graft failure had prompt engraftment, no severe GvHD, and were in good condition two years after HCT.
Baron et al. suggested that transplantation of MSCs derived from umbilical cord blood (UCB) may prevent lethal GvHD without increasing the risk of leukemic relapse following HCT (96). In a pediatric study, parental haploidentical MSCs were used to promote engraftment of UCB grafts with a survival advantage relative to controls (97). In 13 children receiving UCB transplants, co-infusion of third-party MSCs was performed (98). Compared to historical controls there were no differences in hematological recovery or rejection rate. However, the rate of grade III-IV acute GvHD was significantly decreased in the MSC group compared to the controls (p=0.05).
Six children with aplastic anemia were given MSCs together with HLA-haploidentical or unrelated HLA-matched grafts (99). Acute GvHD grade II developed only in one case, but no chronic GvHD was documented. All were alive at a median follow-up of 15 months. In a pediatric study of UCB transplants, third-party UCB-MSCs were co-transplanted (100). Neutrophil engraftment was achieved with a median of 19 days in MSC-treated patients, as compared to 24 days in the historical controls (p=0.03). Acute and chronic GvHD were comparable between the two groups. Forty-four children with severe aplastic anemia were treated with haploidentical HCT and co-transplantation of MSCs (101). The incidence was 29% for grade II-IV acute GvHD and 15% for chronic GvHD and overall survival was 77%.
In a multicenter study, 35 children with aplastic anemia underwent HLA-haploidentical HCT with co-transplantation of donor-derived BM-MSCs (102). All had 100% donor engraftment and incidence of grade II-IV acute GvHD and chronic GvHD was 26% and 23%, respectively. Overall survival at 22 months was 86%. A combination of haplo-HCT and allogeneic MSCs was used for the treatment of severe aplastic anemia in 103 pediatric patients (103). Cumulative incidence of grade II-IV acute GvHD was 26% and grade III-IV acute GvHD was 7%. The cumulative incidence of chronic GvHD was 26% and overall survival was 87% at a median follow-up of 40 months.
A recent meta-analysis, including children as well as adults, showed improved survival in patients treated with MSCs as prophylaxis in HCT patients (104). A low MSC content of the HCT graft, as measured as MSCs expansion at the second passage, was correlated to transplant-related mortality and acute GvHD III-IV (105). Overall, these data indicate that MSC prophylaxis in HCT is associated with decreased graft failure, development of less acute but not chronic GvHD, and maybe an overall survival benefit.
Treatment of Acute GVHD
Early studies paved the way for BM-MSCs as a treatment for severe acute GvHD (11–13). In a multicenter study, patients with steroid-refractory acute GvHD who had a complete response had a two-year survival of 53% in contrast to 16% among partial- and non-responders (13). There was a trend for a better complete response of 64% in pediatric patients in contrast to 47% in adults in this and other studies, and we thus put a focus on children in the following sections. Several studies confirmed the benefit using MSCs for children with severe acute GvHD (106–108). In a study by Prasad et al. commercially available MSCs (Prochymal™) were given to 12 pediatric patients with steroid-refractory severe acute GvHD, with a complete response in 7 children (58%). Survival at 100 days was also 58%. Two children had a partial response, and no response was seen in 3 children.
In a study by Ball et al. using BM-MSCs in 37 pediatric patients treated for steroid-refractory acute GvHD, the response was 65% and 3-year survival was 57% (109). In a mixed study of children and adults in several Brazilian centers response rate was 50% and survival was 20% in severe acute GvHD (110). Platelet-lysate-expanded MSCs were used for the treatment of steroid-refractory acute GvHD in 8 pediatric patients and 22 adults (111). Complete and partial response was seen in 88% of children compared to 50% in adults (p=0.099). Survival was 88% and 29% in the two groups, respectively (p=0.003).
Bonig et al. used BM-MSCs generated from pooled mononuclear cells from multiple donors and the overall response was 82% and 180-day survival was 64%. In children, the response was 77% and two-year survival was 77% (112). A Turkish study including 33 children found that MSCs induced a complete response in 18 patients (54%), a partial response in 7 (21%), and no response in 8 children (24%) (113). Two-year survival was 63% in patients with complete or partial response (75%).
In a multicenter study of 260 children and adults, who were randomized in a double-blind controlled study using Prochymal™ for the treatment of severe acute GvHD (114). The primary end-point, complete response at day 28, was the same in the two study groups. In a later analysis among patients with acute GvHD III-IV, MSC-treated patients had an overall response rate of 65% compared to 23% in the placebo-treated patients (p=0.05) (115). Children had a better overall response compared to adults.
Kurtzberg et al. used Remestemcel-L (Commercial BM-MSC) in 241 pediatric patients with steroid-refractory acute GvHD (116). The children were given 2x106 MSCs/kg of Remestemcel-L twice a week for 4 weeks. Overall response at day +28 was 65% and 100-day survival was 82% among responders and 39% among non-responders (p<0.001). Meta-Analysis in children and adults showed that overall survival was positively correlated to MSC-dose (p=0.02) (104). Children treated with Remestemcel-L were compared to matched patients from the Mouth Sinai Acute GvHD International Consortium (MAGIC) (117). Clinical response after 28 days was 18/25 (72%) in the Remestemcel-L group compared to 13/27 (48%) among the retrospective controls (p=0.08). When children with high levels of biomarkers Reg3a and ST2 for severe acute GvHD were compared, the MSC group had better survival at 180 days, 64% as opposed to 10% in children treated with best available therapy (P=0.01), which included extracorporeal photopheresis, etanercept, infliximab, ruxolitinib, anti-thymocyte globulin, MMF, alemtuzumab, basiliximab, and tocilizumab.
DSCs were used to treat six pediatric patients with severe acute GvHD, grade III-IV and all six responded (118). Unfortunately, one of the patients, a teenage boy with Sickle Cell Disease (SS) died of severe brain hemorrhages a few months post HCT, but at time of HCT, he had severe sequelae due to untreated SS. Another child died of leukemia relapse. Four children are still alive and well with a Lansky score of 100% and five-year survival was 67%.
Overall, these data indicate partial effectiveness of MSCs and DSCs particularly in the treatment of severe acute GvHD in children with room for improvement. Our experience regarding DSCs in the pediatric population is limited, and further studies are needed (118). The outcome with DSCs in adults with acute GvHD is most promising (34, 46, 119). In mainly adult patients, we reported that with an improved handling of DSCs (7, 46), cell viability was median 95% and all 21 patients treated for severe acute GvHD responded with a one-year survival of 76% (34). Long-term survival among those patients at four years was 54%, which was not statistically inferior to the survival among 453 patients without severe acute GvHD undergoing HCT during the same time-period (119).
Treatment of Chronic GVHD
The use of MSCs is much more limited in chronic GvHD than in acute GvHD and most pilot studies using MSCs for chronic GvHD were performed in adult patients (12, 120, 121). Transient effects on chronic GvHD were reported in four children using platelet-lysate-expanded MSCs (107). Only one child had a complete response (the others flared). A Meta-Analysis on the use of MSCs in 76 pediatric and adult patients reported an overall response of 66% with complete responses in 23% of the patients and overall survival of 64% (104).
We used DSCs to treat 3 pediatric patients with severe grade 3 chronic GvHD according to National Institute of Health criteria (54). The three children presented with GvHD in the skin, mouth, eyes, gastrointestinal tract, liver, lungs, joints, and fascia. Two of the children had a response in the liver with normalization of elevated liver enzymes. In one child esophageal varices disappeared. However, there was no overall improvement and grading after therapy sustained very severe grade 3 (54).
A polish study reported treatment of 9 pediatric patients with steroid-refractory acute or chronic GvHD (122). The children were given BM-, AT- or UC-MSC from 1 up to 6 infusions with an overall partial or complete response rate of 56%. Three patients with overlap syndrome showed amelioration, but the condition was not permanent.
A Phase II multicenter randomized, double blind controlled study was performed using UC-derived MSCs as prophylaxis against chronic GvHD (123). The two-year cumulative incidence of chronic GvHD in the MSC group was 27.4% as compared with 49% in the non-MSC-treated controls (p=0.02). After MSCs infusions, increasing memory B-lymphocytes and regulatory T cells were observed.
A meta-analysis including children and adults with GvHD treated with MSCs suggested improved survival using MSCs (104). In conclusion, the data on MSC and DSC treatment for chronic GvHD are much more scarce than acute GvHD, but preliminary studies indicate partial effectiveness.
Treatment of Hemorrhages
Hemorrhagic cystitis occurs in up to 70% of recipients of HCT (124) and is classified as early-onset (within 72 hours after transplantation) or late-onset. Early-onset hemorrhagic cystitis is mainly caused by direct toxic effects of irradiation, cyclophosphamide, busulfan, and other cytotoxic drugs used for conditioning. Late-onset hemorrhagic cystitis may be due to viral infections, e.g. polyomavirus (BK and JC virus) and adenovirus. Transfusions are given to maintain platelet and hemoglobin levels. Hyperhydration and diuretics are given in stages beyond mild disease. For advanced hemorrhagic cystitis, there is no established therapy, but several interventions have been tried, including urethral catheterization, nephrostomy, dialysis, ligation of hypogastric arteries, cystectomy, and hyperbaric oxygen.
Stromal cells may be an attractive alternative and we used BM-MSCs to treat children and adults with hemorrhagic cystitis following HCT (67, 125). Among 10 patients with moderate-to-severe pathology 8 had a complete response after infusion of MSCs (68). Two patients with life-threatening hemorrhagic cystitis and several medical problems died of multi-organ failure (67). Tong et al. used UCB- MSCs in 13 pediatric patients with severe BK-virus-induced hemorrhagic cystitis (126). The children were given a median of 2 (1-3) MSC infusions with 8 cured cases. The copy number of BK virus DNA was 4.43x108 ml before MSCs treatment and 2.67x108 ml after infusion (ns).
Placenta-derived DSCs have a stronger effect on coagulation and hemostasis than BM-MSCs due to higher expression of procoagulant TF/CD142 and thus stronger reduction of the clotting time (44, 46). We treated 11 patients (median age 33 years, range 8-50 years; 4 on pain-requiring opiates) with grades III-IV hemorrhagic cystitis with DSCs (median 1 dose, range 1-4 doses) (69). In 5 patients hematuria disappeared within 5 days after DSC infusion and patients who received DSCs within three days after the start of hematuria had a shorter duration of hematuria and pain than those who were given DSCs later. One-year survival among the 11 patients with severe hemorrhagic cystitis following HCT given DSCs was 82%. These data are promising and presently we plan a prospective placebo-controlled study.
Treatment of ARDS
Acute respiratory distress syndrome (ARDS) is a life-threatening lung condition with high mortality (127–129). The syndrome consists of acute hypoxic respiratory failure with bilateral pulmonary infiltrates associated with sepsis, pneumonia, shock, or aspiration.
Acute lung injury (ALI) and ARDS are characterized by rapid alveolar injury, inflammation, cytokine storm, and neutrophil accumulation. This causes pulmonary edema, leading to severe hypoxemia and impaired carbon dioxide excretion. ALI/ARDS may occur during the pancytopenia phase after HCT. The rationale to use stromal cells for ALI/ARDS is that these cells have a profound anti-inflammatory effect and first home to the lung after intravenous infusion (46, 54, 130). The hope is that MSCs ameliorate the ARDS-induced cytokine storm in the lungs.
We treated an adolescent boy with BM-MSCs in 2004 due to ARDS (131). The pulmonary infiltrates cleared, but the patient had multiple severe medical problems and died of multi-organ failure. We treated a 13-year-old boy with granulocyte transfusions, due to neutropenia after HCT. Subsequently, he developed ARDS and was treated with 1x106 BM-MSCs/kg. He required ventilator assistance and died of massive aspergillosis pneumonia as revealed by autopsy.
AT-derived MSCs were used in a prospective randomized placebo-controlled study in 12 patients with ARDS (132). There was no difference in outcome between the two groups. In a phase I study, 3 patients each received 1x106, 5x106, or 10x106 cryopreserved BM-MSCs/kg as a single infusion for ARDS (133). The dose of 10x106 MSCs/kg was selected for a randomized phase II safety study (134). Survival was the same in the MSC and placebo groups. MSC viability was low post-cryo-recovery (7, 47, 71, 135).
We treated a young adult, with DSCs, who developed α–streptococci septicemia during pancytopenia after HCT (136). With 15 L/min oxygen using a face mask and an oxygen saturation of 92%, he was in imminent need of ventilator assistance. He received 1x106 DSCs/kg. After DSCs infusion oxygen saturation instantly increased to 98%. Requirements for oxygen decreased and the oxygen supply was discontinued 5 days later. Chest radiography normalized. The patient was discharged a few days later, is alive and shows 100% Karnofysky`s score.
The COVID-19 pandemic has led to the death of 5 million people worldwide with more than 245 million infections (University JH. Corona-Virus Resource Center 2021, available from https://coronavirus.jhu.edu/). Death by COVID-19 is mainly due to ARDS (137) and the pronounced coagulopathy leading to cardiovascular demise with lung- and multi-organ failure (47). Thus, particular attention should be paid to thromboprophylaxis, MSC product quality and mode of delivery, when employing experimental MSCs therapies for treatment of COVID-19 ARDS (47). ARDS is caused by a cytokine storm in the lungs, inflammation, neutrophil accumulation and alveolar damage (128). The main rational to use MSCs for treating ARDS is their strong anti-inflammatory effect and that MSCs home to the lung after intravenous cell infusion, which is one of the organs most strongly compromised by this pathology (9, 47, 54, 130). It may be reasoned, that the stronger antiinflammatory and regenerative properties/effects of specific MSC products, the better their therapeutic effect may be on ARDS (comparative studies lacking so far). Thus, it could be reasoned that DSCs may be favored over BM-MSCs, due to their stronger immunosuppressive and regenerative properties in treatment of acute immune-related pathology (7, 34, 46). Indeed, several MSC studies are registered to treat COVID-19-induced ARDS (47, 138–140). UC-derived MSCs were used in a double-blind pilot study including 24 patients with mild COVID-19-ARDS. Survival was 91% in the MSCs group compared to 42% among the controls (p=0.05).
DSCs were given to 10 patients with ARDS induced by COVID-19 (77). Inflammatory cytokines (IL-6 and CRP) significantly decreased after DSCs infusion. The median oxygen saturation level increased from 80% to 95% (P=0.012). Pulmonary infiltrates disappeared in all patients and 7 patients survived and were discharged. No toxicity or infusion-related adverse effects were seen. In a study from Toronto (Mattsson, J., personal communication), DSCs were used to treat 10 patients with ARDS induced by Covid-19. All 10 survived and were discharged from the hospital.
Exosomes derived from BM-MSC were used to treat COVID-19 induced ARDS in 24 patients. The exosomes were safe, and recovery was seen in 17 (71%) of the patients (141). A case study of 3 severe Covid-19 patients treated with concentrated secretomes of hypoxia-conditioned MSCs reported safety and beneficial effects (142).
Discussion
Although MSCs were first explored to enhance hematopoietic engraftment, they are not established for this indication yet (10). However, MSCs are now registered for the treatment of acute GvHD in Japan and in pediatric patients in Canada and New Zeeland (16), but failed to get FDA approval in the US. It is now almost two decades since we treated the 9-year-old boy with life-threatening acute GvHD with BM-MSCs (11). We were lucky to choose a child as the first patient since an adult with such advanced GvHD would not have survived the four weeks it took to isolate and expand enough MSCs for therapy.
MSCs are not established as a treatment for acute GvHD in adults, because a prospective randomized study by Osiris did not meet the primary endpoint, response at 28 days after HCT (115). In this study, children had a better outcome than adults. Also, in the first multi-center study using MSCs for acute GvHD, children tended to have a better outcome than adults (13).
An early multi-center study also demonstrated a favorable outcome in children as opposed to adults (15). This was not found in a more recent meta-analysis, where children and adults had the same response rate to MSCs (14). There is conflicting data and another meta-analysis suggested that children had a better outcome by MSCs-therapy for acute GvHD than adults (143). Regardless of age, it seems that BM-MSC is not the optimal source of stromal cells for acute GvHD. DSCs have a stronger immunomodulatory effect compared to BM-MSC in vitro in MLC and in vivo in patients treated for acute GVHD (33, 34).
MSCs were more successful in acute than chronic GvHD (107, 120, 121). Indeed, MSCs have a better effect on acute inflammatory disorders. In contrast, in chronic GvHD, there is a strong fibrotic component in addition to the underlying inflammatory pathology, that seems to be rather unaffected by MSC treatment. However, we identified a good response to DSCs in patients with chronic GvHD of the liver (54). This may be due to that stromal cell home to the lung after intravenous infusion and are then distributed to the liver and spleen, where they may exert their beneficial effects more efficiently than in peripheral fibrotic tissues (54).
Recently, Ruxolitinib was approved for steroid refractory acute GvHD in adults and pediatric patients above 12 years of age (144). Overall response at day 28 was 62% in the Ruxolitinib group, which was better than 39% in the control group (p<0.001). Ruxolitinib was associated with side effects such as neutropenia, thrombocytopenia, anemia, and infections. The study only included 5 children treated with Ruxolitinib. Ruxolitinib was not so encouraging in children with acute GvHD (145). This may be due to that children have different pharmacokinetics than adults and probably require higher dosages/kg.
Most studies employing MSCs reported that the treatment is safe (12, 115, 116, 146). Similarly, DSCs also seem safe (60). The safety of MSCs therapy is supported by three recent meta-analyses (82–84). A slight increase in temperature was noted following MSCs infusion, but no serious adverse events. This distinguishes stromal cells from many other immunosuppressive drugs, which typically report several adverse events (144). When MSCs are infused systemically, attention should be paid to differing hemostatic/procoagulant properties from different tissue sources, resulting from their differential expression of highly procoagulant TF/C142. A few cases of thromboembolism were reported (7, 46–48, 85–88). The risks for thrombosis can be minimized using well-characterized, high-quality, GMP-compliant MSC products (46, 48), with proper hemocompatibility screening prior to clinical use (7, 46–48). Considering the choice of MSC products for specific indications and mode of delivery, potential benefits need to be weighed against potential side effects (7, 46, 62).
MSCs’ procoagulant properties may be beneficial in the treatment of hemorrhages. MSCs and DSCs exhibit strong modulatory properties on coagulation and hemostasis and these effects are more profound for DSCs than BM-MSCs (44). Both cell types were used successfully to treat hemorrhages following HCT (67–69, 125, 126). BM-MSCs stopped gastrointestinal hemorrhages in a patient refractory to platelet transfusions. Despite encouraging studies, MSCs have only been used to treat hemorrhages in a few reports to date.
For hemorrhagic cystitis, both BM-MSCs as well as DSCs seem to be a superior option, compared to conventional treatments, as these traditional interventions are associated with high morbidity and mortality (147). As shown in our pilot studies, treatment with BM-MSCs and DSCs is an attractive alternative (67–69, 125). DSCs exhibit a stronger effect on coagulation and hemostasis (44, 46) and it seems to be an attractive option to use them in future studies, especially in the pediatric population when a child suffers from severe hemorrhagic cystitis stage III-IV. MSCs also have the potential to be used to stop fatal gastro-intestinal hemorrhages in very old patients with severe co-morbidities, where surgical intervention cannot be undertaken (125).
A concern with BM-MSCs has been possible ectopic tissue formation, because these cells differentiate well into cartilage, fat, and bone, among other mesenchymal tissues (6, 90, 115). Analysis of human autopsy material found no ectopic tissue following MSC infusion (90). DSCs may be even safer because they are half the size with a lower tendency for microvascular entrapment, and they do not differentiate well into cartilage and bone (44, 54).
Cell dosing and cryopreservation may be decisive factors in the safe and efficient clinical use of stromal cells (7, 45, 46, 71, 79). A wide range of MSCs cell doses were used safely, from 0.5 to 10 x 106 cells/kg (46, 148, 149). Wilson and co-workers tested three different doses, 1x106, 6x106 and 10x106 MSCs/kg and selected 10x106 cells/kg in a prospective study for ARDS (133, 134). In the latter study, cell viability varied between 36% and 85% post thawing for clinical use, and low viability may have compromised clinical efficacy (135).
However, with optimal handling both fresh and freeze-thawed cells may have similar viability and immunomodulatory effects (Sadeghi B. et al. to be published) (71, 73). Indeed, the full impact of cryopreservation and freeze-thawing on MSCs’ therapeutic efficacy is controversially discussed in the moment, since several studies also reported that both fresh and freeze-thawed MSCs have similar immunomodulatory properties in preclinical models (150–154). Some recent studies even argued that frozen cells may be more advantageous since recognition of apoptotic cells by the host immune system may be part of their MoA (39).
With therapeutic DSCs, the median cell viability was more than 95% and cell batches with high viability had a better clinical response in treatment of severe acute GvHD (7, 34). Fresh BM-MSCs performed better than freeze-thawed cells (45). Thus, the highest possible cell viability should be aimed for, but above 80% may be sufficient. A cell dose of 1x106 cell/kg seems to be effective in many clinical trials.
Many different sources of stromal cells were explored during the past decades (7, 46, 48). Most experience stems from BM-MSCs, but this requires rather invasive/painful harvesting by aspirating BM from patients or voluntary donors with limited material for cell expansion. In contrast, AT-derived MSCs are popular because they can be easily sourced from leftover material obtained during plastic surgery. In addition, perinatal and endometrial tissue sources (155), such as placenta, umbilical cord matrix, umbilical cord, or menstrual blood are non-invasive sources, with excellent expansion potential and high potency, including DSCs (33).
Our pilot studies indicate that DSCs are superior for inhibition of alloreactivity and have stronger paracrine activity compared to other sources of MSCs, which makes them valuable for immunosuppression. In contrast, a major advantage of BM-MSCs is that they differentiate very well into fat, cartilage, and bone (156), and therefore may be more valuable for tissue engineering and in regenerative medicine.
Due to the ongoing SARS-CoV2 coronavirus pandemic, where ARDS has been a frequent cause of death, many studies were registered for this indication using various sources of MSCs (47, 138). Two randomized placebo-controlled studies using AT- and BM-MSCs failed to prove efficacy for ARDS (132, 134). So far, PT-derived MSCs and DSCs seem to be promising for ARDS induced by COVID-19 (77, 140, 157, 158). Regarding DSCs a prospective randomized placebo- controlled trial is needed. Overall, more data and efficacy studies are needed, before ARDS can be established as an indication using MSCs (47).
MSCs are mainly used to treat acute GvHD, but not as extensively in chronic GvHD. Recent studies show that it may be possible to reverse severe acute GvHD by using exosomes from MSCs (42). Other indications where MSCs may be useful, such as pneumomediastinum, colon perforation, and radiculomyelopathy have been explored in adult HCT patients so far (67, 159). In children with osteogenesis imperfecta, HCT combined with MSCs treatment showed donor-osteoblast engraftment new dense bone, increased bone mineral content, and improved growth velocity (55, 160–162). In addition, patients who have undergone HCT for Hurler’s disease and metachromatic leukodystrophy were treated with MSCs to enhance enzyme production (163). Among children with metachromatic leukodystrophy, 4 of 5 had improvement in nerve conduction velocity.
Conclusion
In this paper, we have discussed the use of MSCs to enhance hematopoietic engraftment, and as treatment for chronic and acute GvHD, hemorrhagic cystitis and ARDS. The later three pathologies are characterized by high levels of proinflammatory mediators. MSCs may be of particular use here, since they have potent immunomodulatory and regenerative effects, with a favorable safety profile compared to other conventional immunosuppressive drugs. The application of BM-MSCs and DSCs appears to be safe, although their procoagulant properties need to be anticipated for clinical use. Further improvements in clinical efficacy by stromal cells therapy may be achieved by optimizing the cell production process and by using stromal cell derived exosomes and extracellular vesicles instead of whole cell therapy. Product improvements may be achieved by optimizing cell viability and cryopreservation, the mode of cell delivery, innovative cell engineering concepts, and more potent products, such as DSCs. In the future, many different types of MSCs products tailored to specific clinical indications, but also exosomes derived thereof, may be used for several inflammatory disorders. Primary indications for MSCs and DSCs include among others acute GvHD and hemorrhages (e.g. hemorrhagic cystitis) and ARDS, but many other indications, e.g. osteogenesis imperfecta, rheumatic inflammatory disorders, cardiovascular diseases, sepsis and septic shock, inflammatory bowel disease, multiple sclerosis, neuroinflammation and other acute inflammatory disorders may join the line of established therapies (164, 165).
Data Availability Statement
The original contributions presented in the study are included in the article/supplementary material. Further inquiries can be directed to the corresponding authors.
Author Contributions
OR wrote the first draft of the article, complemented and revised by GM, BG, and BS. All authors contributed to the article and approved the submitted version.
Funding
The study was supported with grants from the Swedish Cancer Foundation (CAN 2018, 419) and the Cancer Society in Stockholm (111293). OR was the recipient of a Distinguished Professor Award from the Karolinska Institutet. GM’s contributions were made possible by funding from the German Federal Ministry for Education and Research (BMBF) and German Research Foundation (DFG) through the Berlin Institute of Healthy (BIH)-Center for Regenerative Therapies (BCRT) and the Berlin-Brandenburg School for Regenerative Therapies (BSRT, GSC203), respectively, and in part by the European Union’s Horizon 2020 Research and Innovation Program under grant agreements No 733006 (PACE) and 779293 (HIPGEN). We acknowledge support from Frontiers Immunology and the DFG and the Open Access Publication Fund of Charité – Universitätsmedizin Berlin.
Conflict of Interest
The authors declare that the research was conducted in the absence of any commercial or financial relationships that could be construed as a potential conflict of interest.
Publisher’s Note
All claims expressed in this article are solely those of the authors and do not necessarily represent those of their affiliated organizations, or those of the publisher, the editors and the reviewers. Any product that may be evaluated in this article, or claim that may be made by its manufacturer, is not guaranteed or endorsed by the publisher.
Acknowledgments
We want to thank Gunilla Tillinger for the excellent typing of this manuscript.
References
1. Friedenstein AJ, Petrakova KV, Kurolesova AI, Frolova GP. Heterotopic of Bone Marrow. Analysis of Precursor Cells for Osteogenic and Hematopoietic Tissues. Transplantation (1968) 6(2):230–47.
2. Pittenger MF, Mackay AM, Beck SC, Jaiswal RK, Douglas R, Mosca JD, et al. Multilineage Potential of Adult Human Mesenchymal Stem Cells. Science (1999) 284(5411):143–7. doi: 10.1126/science.284.5411.143
3. Pittenger MF, Discher DE, Péault BM, Phinney DG, Hare JM, Caplan AI. Mesenchymal Stem Cell Perspective: Cell Biology to Clinical Progress. NPJ Regenerative Med (2019) 4(1):22. doi: 10.1038/s41536-019-0083-6
4. Bianco P, Cao X, Frenette PS, Mao JJ, Robey PG, Simmons PJ, et al. The Meaning, the Sense and the Significance: Translating the Science of Mesenchymal Stem Cells Into Medicine. Nat Med (2013) 19(1):35–42. doi: 10.1038/nm.3028
6. Andrzejewska A, Catar R, Schoon J, Qazi TH, Sass FA, Jacobi D, et al. Multi-Parameter Analysis of Biobanked Human Bone Marrow Stromal Cells Shows Little Influence for Donor Age and Mild Comorbidities on Phenotypic and Functional Properties. Front Immunol (2019) 10:2474. doi: 10.3389/fimmu.2019.02474
7. Moll G, Hoogduijn MJ, Ankrum JA. Editorial: Safety, Efficacy and Mechanisms of Action of Mesenchymal Stem Cell Therapies. Front Immunol (2020) 11:243. doi: 10.3389/fimmu.2020.00243
8. Doorn J, Moll G, Le Blanc K, van Blitterswijk C, de Boer J. Therapeutic Applications of Mesenchymal Stromal Cells: Paracrine Effects and Potential Improvements. Tissue Eng Part B Rev (2012) 18(2):101–15. doi: 10.1089/ten.teb.2011.0488
9. Bartholomew A, Sturgeon C, Siatskas M, Ferrer K, McIntosh K, Patil S, et al. Mesenchymal Stem Cells Suppress Lymphocyte Proliferation In Vitro and Prolong Skin Graft Survival In Vivo. Exp Hematol (2002) 30(1):42–8. doi: 10.1016/S0301-472X(01)00769-X
10. Koç ON, Gerson SL, Cooper BW, Dyhouse SM, Haynesworth SE, Caplan AI, et al. Rapid Hematopoietic Recovery After Coinfusion of Autologous-Blood Stem Cells and Culture-Expanded Marrow Mesenchymal Stem Cells in Advanced Breast Cancer Patients Receiving High-Dose Chemotherapy. J Clin Oncol Off J Am Soc Clin Oncol (2000) 18(2):307–16. doi: 10.1200/JCO.2000.18.2.307
11. Le Blanc K, Rasmusson I, Sundberg B, Gotherstrom C, Hassan M, Uzunel M, et al. Treatment of Severe Acute Graft-Versus-Host Disease With Third Party Haploidentical Mesenchymal Stem Cells. Lancet (2004) 363(9419):1439–41. doi: 10.1016/S0140-6736(04)16104-7
12. Ringden O, Uzunel M, Rasmusson I, Remberger M, Sundberg B, Lonnies H, et al. Mesenchymal Stem Cells for Treatment of Therapy-Resistant Graft-Versus-Host Disease. Transplantation (2006) 81(10):1390–7. doi: 10.1097/01.tp.0000214462.63943.14
13. Le Blanc K, Frassoni F, Ball L, Locatelli F, Roelofs H, Lewis I, et al. Mesenchymal Stem Cells for Treatment of Steroid-Resistant, Severe, Acute Graft-Versus-Host Disease: A Phase II Study. Lancet (2008) 371(9624):1579–86. doi: 10.1016/S0140-6736(08)60690-X
14. Hashmi S, Ahmed M, Murad MH, Litzow MR, Adams RH, Ball LM, et al. Survival After Mesenchymal Stromal Cell Therapy in Steroid-Refractory Acute Graft-Versus-Host Disease: Systematic Review and Meta-Analysis. Lancet Haematol (2016) 3(1):e45–52. doi: 10.1016/S2352-3026(15)00224-0
15. Wernicke CM, Grunewald TG, Hendrik J, Kuci S, Kuci Z, Koehl U, et al. Mesenchymal Stromal Cells for Treatment of Steroid-Refractory GvHD: A Review of the Literature and Two Pediatric Cases. Int Arch Med (2011) 4(1):27. doi: 10.1186/1755-7682-4-27
16. Murata M, Terakura S, Wake A, Miyao K, Ikegame K, Uchida N, et al. Off-The-Shelf Bone Marrow-Derived Mesenchymal Stem Cell Treatment for Acute Graft-Versus-Host Disease: Real-World Evidence. Bone Marrow Transplant (2021) 56(10):2355–66. doi: 10.1038/s41409-021-01304-y
17. Fisher SA, Cutler A, Doree C, Brunskill SJ, Stanworth SJ, Navarrete C, et al. Mesenchymal Stromal Cells as Treatment or Prophylaxis for Acute or Chronic Graft-Versus-Host Disease in Haematopoietic Stem Cell Transplant (HSCT) Recipients With a Haematological Condition. Cochrane Database System Rev (2019) 1:CD009768. doi: 10.1002/14651858.CD009768.pub2
18. Le Blanc K, Tammik L, Sundberg B, Haynesworth SE, Ringdén O. Mesenchymal Stem Cells Inhibit and Stimulate Mixed Lymphocyte Cultures and Mitogenic Responses Independently of the Major Histocompatibility Complex. Scand J Immunol (2003) 57(1):11–20. doi: 10.1046/j.1365-3083.2003.01176.x
19. Le Blanc K, Tammik C, Rosendahl K, Zetterberg E, Ringden O. HLA Expression and Immunologic Properties of Differentiated and Undifferentiated Mesenchymal Stem Cells. Exp Hematol (2003) 31(10):890–6. doi: 10.1016/S0301-472X(03)00110-3
20. Tse WT, Pendleton JD, Beyer WM, Egalka MC, Guinan EC. Suppression of Allogeneic T-Cell Proliferation by Human Marrow Stromal Cells: Implications in Transplantation. Transplantation (2003) 75(3):389–97. doi: 10.1097/01.TP.0000045055.63901.A9
21. Jones S, Horwood N, Cope A, Dazzi F. The Antiproliferative Effect of Mesenchymal Stem Cells is a Fundamental Property Shared by All Stromal Cells. J Immunol (2007) 179(5):2824–31. doi: 10.4049/jimmunol.179.5.2824
22. Gregoire C, Ritacco C, Hannon M, Seidel L, Delens L, Belle L, et al. Comparison of Mesenchymal Stromal Cells From Different Origins for the Treatment of Graft-Vs.-Host-Disease in a Humanized Mouse Model. Front Immunol (2019) 10:619. doi: 10.3389/fimmu.2019.00619
23. Aggarwal S, Pittenger MF. Human Mesenchymal Stem Cells Modulate Allogeneic Immune Cell Responses. Blood (2005) 105(4):1815–22. doi: 10.1182/blood-2004-04-1559
24. Maccario R, Podestà M, Moretta A, Cometa A, Comoli P, Montagna D, et al. Interaction of Human Mesenchymal Stem Cells With Cells Involved in Alloantigen-Specific Immune Response Favors the Differentiation of CD4+ T-Cell Subsets Expressing a Regulatory/Suppressive Phenotype. Haematologica (2005) 90(4):516–25.
25. Rasmusson I, Ringdén O, Sundberg B, Le Blanc K. Mesenchymal Stem Cells Inhibit Lymphocyte Proliferation by Mitogens and Alloantigens by Different Mechanisms. Exp Cell Res (2005) 305(1):33–41. doi: 10.1016/j.yexcr.2004.12.013
26. Nemeth K, Leelahavanichkul A, Yuen PST, Mayer B, Parmelee A, Doi K, et al. Bone Marrow Stromal Cells Attenuate Sepsis via Prostaglandin E-2-Dependent Reprogramming of Host Macrophages to Increase Their Interleukin-10 Production (Vol 15, Pg 42, 2009). Nat Med (2009) 15(4):462–2. doi: 10.1038/nm0409-462b
27. Erkers T, Nava S, Yosef J, Ringden O, Kaipe H. Decidual Stromal Cells Promote Regulatory T Cells and Suppress Alloreactivity in a Cell Contact-Dependent Manner. Stem Cells Dev (2013) 22(19):2596–605. doi: 10.1089/scd.2013.0079
28. Selmani Z, Naji A, Gaiffe E, Obert L, Tiberghien P, Rouas-Freiss N, et al. HLA-G is a Crucial Immunosuppressive Molecule Secreted by Adult Human Mesenchymal Stem Cells. Transplantation (2009) 87(9 Suppl):S62–66. doi: 10.1097/TP.0b013e3181a2a4b3
29. Gieseke F, Böhringer J, Bussolari R, Dominici M, Handgretinger R, Müller I. Human Multipotent Mesenchymal Stromal Cells Use Galectin-1 to Inhibit Immune Effector Cells. Blood (2010) 116(19):3770–9. doi: 10.1182/blood-2010-02-270777
30. Meisel R, Zibert A, Laryea M, Göbel U, Däubener W, Dilloo D. Human Bone Marrow Stromal Cells Inhibit Allogeneic T-Cell Responses by Indoleamine 2,3-Dioxygenase-Mediated Tryptophan Degradation. Blood (2004) 103(12):4619–21. doi: 10.1182/blood-2003-11-3909
31. Munn DH, Zhou M, Attwood JT, Bondarev I, Conway SJ, Marshall B, et al. Prevention of Allogeneic Fetal Rejection by Tryptophan Catabolism. Science (1998) 281(5380):1191–3. doi: 10.1126/science.281.5380.1191
32. Sadeghi B, Heshmati Y, Khoein B, Kaipe H, Uzunel M, Walfridsson J, et al. Xeno-Immunosuppressive Properties of Human Decidual Stromal Cells in Mouse Models of Alloreactivity In Vitro and In Vivo. Cytotherapy (2015) 17(12):1732–45. doi: 10.1016/j.jcyt.2015.09.001
33. Karlsson H, Erkers T, Nava S, Ruhm S, Westgren M, Ringden O. Stromal Cells From Term Fetal Membrane are Highly Suppressive in Allogeneic Settings In Vitro. Clin Exp Immunol (2012) 167(3):543–55. doi: 10.1111/j.1365-2249.2011.04540.x
34. Ringden O, Baygan A, Remberger M, Gustafsson B, Winiarski J, Khoein B, et al. Placenta-Derived Decidua Stromal Cells for Treatment of Severe Acute Graft-Versus-Host Disease. Stem Cells Transl Med (2018) 7(4):325–31. doi: 10.1002/sctm.17-0167
35. Augello A, Tasso R, Negrini SM, Amateis A, Indiveri F, Cancedda R, et al. Bone Marrow Mesenchymal Progenitor Cells Inhibit Lymphocyte Proliferation by Activation of the Programmed Death 1 Pathway. Eur J Immunol (2005) 35(5):1482–90. doi: 10.1002/eji.200425405
36. Ren G, Zhao X, Zhang L, Zhang J, L'Huillier A, Ling W, et al. Inflammatory Cytokine-Induced Intercellular Adhesion Molecule-1 and Vascular Cell Adhesion Molecule-1 in Mesenchymal Stem Cells are Critical for Immunosuppression. J Immunol (2010) 184(5):2321–8. doi: 10.4049/jimmunol.0902023
37. Saldanha-Araujo F, Ferreira FI, Palma PV, Araujo AG, Queiroz RH, Covas DT, et al. Mesenchymal Stromal Cells Up-Regulate CD39 and Increase Adenosine Production to Suppress Activated T-Lymphocytes. Stem Cell Res (2011) 7(1):66–74. doi: 10.1016/j.scr.2011.04.001
38. Akiyama K, Chen C, Wang D, Xu X, Qu C, Yamaza T, et al. Mesenchymal-Stem-Cell-Induced Immunoregulation Involves FAS-Ligand-/FAS-Mediated T Cell Apoptosis. Cell Stem Cell (2012) 10(5):544–55. doi: 10.1016/j.stem.2012.03.007
39. Galleu A, Riffo-Vasquez Y, Trento C, Lomas C, Dolcetti L, Cheung TS, et al. Apoptosis in Mesenchymal Stromal Cells Induces In Vivo Recipient-Mediated Immunomodulation. Sci Trans Med (2017) 9(416):eaam7828. doi: 10.1126/scitranslmed.aam7828
40. Galipeau J. Macrophages at the Nexus of Mesenchymal Stromal Cell Potency: The Emerging Role of Chemokine Cooperativity. Stem Cells (2021) 39(9):1145–54. doi: 10.1002/stem.3380
41. Théry C, Ostrowski M, Segura E. Membrane Vesicles as Conveyors of Immune Responses. Nat Rev Immunol (2009) 9(8):581–93. doi: 10.1038/nri2567
42. Kordelas L, Rebmann V, Ludwig AK, Radtke S, Ruesing J, Doeppner TR, et al. MSC-Derived Exosomes: A Novel Tool to Treat Therapy-Refractory Graft-Versus-Host Disease. Leukemia (2014) 28(4):970–3. doi: 10.1038/leu.2014.41
43. Kaipe H, Erkers T, Sadeghi B, Ringdén O. Stromal Cells-are They Really Useful for GVHD? Bone Marrow Transplant (2014) 49(6):737–43. doi: 10.1038/bmt.2013.237
44. Moll G, Ignatowicz L, Catar R, Luecht C, Sadeghi B, Hamad O, et al. Different Procoagulant Activity of Therapeutic Mesenchymal Stromal Cells Derived From Bone Marrow and Placental Decidua. Stem Cells Dev (2015) 24(19):2269–79. doi: 10.1089/scd.2015.0120
45. Moll G, Alm JJ, Davies LC, von Bahr L, Heldring N, Stenbeck-Funke L, et al. Do Cryopreserved Mesenchymal Stromal Cells Display Impaired Immunomodulatory and Therapeutic Properties? Stem Cells (2014) 32(9):2430–42. doi: 10.1002/stem.1729
46. Moll G, Ankrum JA, Kamhieh-Milz J, Bieback K, Ringden O, Volk HD, et al. Intravascular Mesenchymal Stromal/Stem Cell Therapy Product Diversification: Time for New Clinical Guidelines. Trends Mol Med (2019) 25(2):149–63. doi: 10.1016/j.molmed.2018.12.006
47. Moll G, Drzeniek N, Kamhieh-Milz J, Geissler S, Volk H-D, Reinke P. MSC Therapies for COVID-19: Importance of Patient Coagulopathy, Thromboprophylaxis, Cell Product Quality and Mode of Delivery for Treatment Safety and Efficacy. Front Immunol (2020) 11(1091). doi: 10.3389/fimmu.2020.01091
48. Moll G, Ankrum JA, Olson SD, Nolta JA. Improved MSC Minimal Criteria to Maximize Patient Safety: A Call to Embrace Tissue Factor and Hemocompatibility Assessment of MSC Products. Stem Cells Trans Med (2022) szab005. doi: 10.1093/stcltm/szab005
49. Moll G, Rasmusson-Duprez I, von Bahr L, Connolly-Andersen AM, Elgue G, Funke L, et al. Are Therapeutic Human Mesenchymal Stromal Cells Compatible With Human Blood? Stem Cells (2012) 30(7):1565–74. doi: 10.1002/stem.1111
50. Ankrum JA, Ong JF, Karp JM. Mesenchymal Stem Cells: Immune Evasive, Not Immune Privileged. Nat Biotechnol (2014) 32(3):252–60. doi: 10.1038/nbt.2816
51. Grinnemo KH, Mansson A, Dellgren G, Klingberg D, Wardell E, Drvota V, et al. Xenoreactivity and Engraftment of Human Mesenchymal Stem Cells Transplanted Into Infarcted Rat Myocardium. J Thorac Cardiovasc Surg (2004) 127(5):1293–300. doi: 10.1016/j.jtcvs.2003.07.037
52. Eliopoulos N, Stagg J, Lejeune L, Pommey S, Galipeau J. Allogeneic Marrow Stromal Cells are Immune Rejected by MHC Class I- and Class II-Mismatched Recipient Mice. Blood (2005) 106(13):4057–65. doi: 10.1182/blood-2005-03-1004
53. Sadeghi B, Moretti G, Arnberg F, Samen E, Kohein B, Catar R, et al. Preclinical Toxicity Evaluation of Clinical Grade Placenta-Derived Decidua Stromal Cells. Front Immunol (2019) 10:2685. doi: 10.3389/fimmu.2019.02685
54. Erkers T, Kaipe H, Nava S, Molldén P, Gustafsson B, Axelsson R, et al. Treatment of Severe Chronic Graft-Versus-Host Disease With Decidual Stromal Cells and Tracing With (111)Indium Radiolabeling. Stem Cells Dev (2015) 24(2):253–63. doi: 10.1089/scd.2014.0265
55. Horwitz EM, Gordon PL, Koo WK, Marx JC, Neel MD, McNall RY, et al. Isolated Allogeneic Bone Marrow-Derived Mesenchymal Cells Engraft and Stimulate Growth in Children With Osteogenesis Imperfecta: Implications for Cell Therapy of Bone. Proc Natl Acad Sci USA (2002) 99(13):8932–7. doi: 10.1073/pnas.132252399
56. Beggs KJ, Lyubimov A, Borneman JN, Bartholomew A, Moseley A, Dodds R, et al. Immunologic Consequences of Multiple, High-Dose Administration of Allogeneic Mesenchymal Stem Cells to Baboons. Cell Transplant (2006) 15(8-9):711–21. doi: 10.3727/000000006783981503
57. Sundin M, Ringden O, Sundberg B, Nava S, Gotherstrom C, Le Blanc K. No Alloantibodies Against Mesenchymal Stromal Cells, But Presence of Anti-Fetal Calf Serum Antibodies, After Transplantation in Allogeneic Hematopoietic Stem Cell Recipients. Haematologica (2007) 92(9):1208–15. doi: 10.3324/haematol.11446
58. Kaipe H, Carlson LM, Erkers T, Nava S, Mollden P, Gustafsson B, et al. Immunogenicity of Decidual Stromal Cells in an Epidermolysis Bullosa Patient and in Allogeneic Hematopoietic Stem Cell Transplantation Patients. Stem Cells Dev (2015) 24(12):1471–82. doi: 10.1089/scd.2014.0568
59. Kabat M, Bobkov I, Kumar S, Grumet M. Trends in Mesenchymal Stem Cell Clinical Trials 2004-2018: Is Efficacy Optimal in a Narrow Dose Range? Stem Cells Trans Med (2020) 9(1):17–27. doi: 10.1002/sctm.19-0202
60. Baygan A, Aronsson-Kurttila W, Moretti G, Tibert B, Dahllof G, Klingspor L, et al. Safety and Side Effects of Using Placenta-Derived Decidual Stromal Cells for Graft-Versus-Host Disease and Hemorrhagic Cystitis. Front Immunol (2017) 8:795. doi: 10.3389/fimmu.2017.00795
61. Nilsson B, Korsgren O, Lambris JD, Ekdahl KN. Can Cells and Biomaterials in Therapeutic Medicine be Shielded From Innate Immune Recognition? Trends Immunol (2010) 31(1):32–8. doi: 10.1016/j.it.2009.09.005
62. Caplan H, Olson SD, Kumar A, George M, Prabhakara KS, Wenzel P, et al. Mesenchymal Stromal Cell Therapeutic Delivery: Translational Challenges to Clinical Application. Front Immunol (2019) 10:1645. doi: 10.3389/fimmu.2019.01645
63. Coppin L, Sokal E, Stéphenne X. Thrombogenic Risk Induced by Intravascular Mesenchymal Stem Cell Therapy: Current Status and Future Perspectives. Cells (2019) 8(10):1160. doi: 10.3390/cells8101160
64. Levy O, Kuai R, Siren EMJ, Bhere D, Milton Y, Nissar N, et al. Shattering Barriers Toward Clinically Meaningful MSC Therapies. Sci Adv (2020) 6(30):eaba6884. doi: 10.1126/sciadv.aba6884
65. Moll G, Drzeniek N, Kamhieh-Milz J, Geissler S, Reinke P. Editorial Comment: Variables Affecting the Presence of Mesenchymal Stromal Cells in the Peripheral Blood and Their Relationship With Apheresis Product. Br J Haematol (2020) 189(4):593–6. doi: 10.1111/bjh.16389
66. Giri J, Moll G. MSCs in Space: Mesenchymal Stromal Cell Therapeutics as Enabling Technology for Long-Distance Manned Space Travel. Curr Stem Cell Rep (2022) 8:1–13. doi: 10.1007/s40778-022-00207-y
67. Ringden O, Uzunel M, Sundberg B, Lonnies L, Nava S, Gustafsson J, et al. Tissue Repair Using Allogeneic Mesenchymal Stem Cells for Hemorrhagic Cystitis, Pneumomediastinum and Perforated Colon. Leukemia (2007) 21(11):2271–6. doi: 10.1038/sj.leu.2404833
68. Ringden O, Le Blanc K. Mesenchymal Stem Cells for Treatment of Acute and Chronic Graft-Versus-Host Disease, Tissue Toxicity and Hemorrhages. Best Pract Res Clin Haematol (2011) 24(1):65–72. doi: 10.1016/j.beha.2011.01.003
69. Aronsson-Kurttila W, Baygan A, Moretti G, Remberger M, Khoein B, Moll G, et al. Placenta-Derived Decidua Stromal Cells for Hemorrhagic Cystitis After Stem Cell Transplantation. Acta Haematol (2018) 139(2):106–14. doi: 10.1159/000485735
70. Markiewski MM, Nilsson B, Ekdahl KN, Mollnes TE, Lambris JD. Complement and Coagulation: Strangers or Partners in Crime? Trends Immunol (2007) 28(4):184–92. doi: 10.1016/j.it.2007.02.006
71. Moll G, Geissler S, Catar R, Ignatowicz L, Hoogduijn MJ, Strunk D, et al. Cryopreserved or Fresh Mesenchymal Stromal Cells: Only a Matter of Taste or Key to Unleash the Full Clinical Potential of MSC Therapy? Adv Exp Med Biol (2016) 951:77–98. doi: 10.1007/978-3-319-45457-3_7
72. Hoogduijn MJ, de Witte SF, Luk F, van den Hout-van Vroonhoven MC, Ignatowicz L, Catar R, et al. Effects of Freeze-Thawing and Intravenous Infusion on Mesenchymal Stromal Cell Gene Expression. Stem Cells Dev (2016) 25(8):586–97. doi: 10.1089/scd.2015.0329
73. Cottle C, Porter AP, Lipat A, Moll G, Chinnadurai R. Impact of Cryopreservation and Freeze-Thawing on Therapeutic Properties of Mesenchymal Stromal Cells and Other Common Cellular Therapeutics. Curr Stem Cell Rep (2022).
74. Moll G, Le Blanc K. Engineering More Efficient Multipotent Mesenchymal Stromal (Stem) Cells for Systemic Delivery as Cellular Therapy. ISBT Sci Ser (2015) 10(S1):357–65. doi: 10.1111/voxs.12133
75. George MJ, Prabhakara K, Toledano-Furman NE, Wang YW, Gill BS, Wade CE, et al. Clinical Cellular Therapeutics Accelerate Clot Formation. Stem Cells Trans Med (2018) 7(10):731–9. doi: 10.1002/sctm.18-0015
76. George MJ, Prabhakara K, Toledano-Furman NE, Gill BS, Wade CE, Cotton BA, et al. Procoagulant In Vitro Effects of Clinical Cellular Therapeutics in a Severely Injured Trauma Population. Stem Cells Trans Med (2020) 9(4):491–8. doi: 10.1002/sctm.19-0206
77. Sadeghi B, Roshandel E, Pirsalehi A, Kazemi S, Sankanian G, Majidi M, et al. Conquering the Cytokine Storm in COVID-19-Induced ARDS Using Placenta-Derived Decidua Stromal Cells. J Cell Mol Med (2021) 25(22):10554–64. doi: 10.1111/jcmm.16986
78. Moll G, Jitschin R, von Bahr L, Rasmusson-Duprez I, Sundberg B, Lonnies L, et al. Mesenchymal Stromal Cells Engage Complement and Complement Receptor Bearing Innate Effector Cells to Modulate Immune Responses. PloS One (2011) 6(7):e21703. doi: 10.1371/journal.pone.0021703
79. Moll G, Hult A, von Bahr L, Alm JJ, Heldring N, Hamad OA, et al. Do ABO Blood Group Antigens Hamper the Therapeutic Efficacy of Mesenchymal Stromal Cells? PloS One (2014) 9(1):e85040. doi: 10.1371/journal.pone.0085040
80. Li Y, Lin F. Mesenchymal Stem Cells are Injured by Complement After Their Contact With Serum. Blood (2012) 120(17):3436–43. doi: 10.1182/blood-2012-03-420612
81. Li Y, Qiu W, Zhang L, Fung J, Lin F. Painting Factor H Onto Mesenchymal Stem Cells Protects the Cells From Complement- and Neutrophil-Mediated Damage. Biomaterials (2016) 102:209–19. doi: 10.1016/j.biomaterials.2016.05.055
82. Lalu MM, McIntyre L, Pugliese C, Fergusson D, Winston BW, Marshall JC, et al. Safety of Cell Therapy With Mesenchymal Stromal Cells (SafeCell): A Systematic Review and Meta-Analysis of Clinical Trials. PloS One (2012) 7(10):e47559. doi: 10.1371/journal.pone.0047559
83. Lalu MM, Sullivan KJ, Mei SH, Moher D, Straus A, Fergusson DA, et al. Evaluating Mesenchymal Stem Cell Therapy for Sepsis With Preclinical Meta-Analyses Prior to Initiating a First-in-Human Trial. Elife (2016) 5:e17850. doi: 10.7554/eLife.17850
84. Thompson M, Mei SHJ, Wolfe D, Champagne J, Fergusson D, Stewart DJ, et al. Cell Therapy With Intravascular Administration of Mesenchymal Stromal Cells Continues to Appear Safe: An Updated Systematic Review and Meta-Analysis. EClinicalMedicine (2020) 19:100249. doi: 10.1016/j.eclinm.2019.100249
86. Jung JW, Kwon M, Choi JC, Shin JW, Park IW, Choi BW, et al. Familial Occurrence of Pulmonary Embolism After Intravenous, Adipose Tissue-Derived Stem Cell Therapy. Yonsei Med J (2013) 54(5):1293–6. doi: 10.3349/ymj.2013.54.5.1293
87. Acosta L, Hmadcha A, Escacena N, Perez-Camacho I, de la Cuesta A, Ruiz-Salmeron R, et al. Adipose Mesenchymal Stromal Cells Isolated From Type 2 Diabetic Patients Display Reduced Fibrinolytic Activity. Diabetes (2013) 62(12):4266–9. doi: 10.2337/db13-0896
88. Wu Z, Zhang S, Zhou L, Cai J, Tan J, Gao X, et al. Thromboembolism Induced by Umbilical Cord Mesenchymal Stem Cell Infusion: A Report of Two Cases and Literature Review. Transplant Proc (2017) 49(7):1656–8. doi: 10.1016/j.transproceed.2017.03.078
89. Goldsobel G, von Herrath C, Schlickeiser S, Brindle N, Stähler F, Reinke P, et al. RESTORE Survey on the Public Perception of Advanced Therapies and ATMPs in Europe—Why the European Union Should Invest More! Front Med (2021) 8(1900). doi: 10.3389/fmed.2021.739987
90. von Bahr L, Batsis I, Moll G, Hagg M, Szakos A, Sundberg B, et al. Analysis of Tissues Following Mesenchymal Stromal Cell Therapy in Humans Indicates Limited Long-Term Engraftment and No Ectopic Tissue Formation. Stem Cells (2012) 30(7):1575–8. doi: 10.1002/stem.1118
91. Lazarus HM, Koc ON, Devine SM, Curtin P, Maziarz RT, Holland HK, et al. Cotransplantation of HLA-Identical Sibling Culture-Expanded Mesenchymal Stem Cells and Hematopoietic Stem Cells in Hematologic Malignancy Patients. Biol Blood Marrow Transplant (2005) 11(5):389–98. doi: 10.1016/j.bbmt.2005.02.001
92. Ball LM, Bernardo ME, Roelofs H, Lankester A, Cometa A, Egeler RM, et al. Cotransplantation of Ex Vivo Expanded Mesenchymal Stem Cells Accelerates Lymphocyte Recovery and may Reduce the Risk of Graft Failure in Haploidentical Hematopoietic Stem-Cell Transplantation. Blood (2007) 110(7):2764–7. doi: 10.1182/blood-2007-04-087056
93. Le Blanc K, Samuelsson H, Gustafsson B, Remberger M, Sundberg B, Arvidson J, et al. Transplantation of Mesenchymal Stem Cells to Enhance Engraftment of Hematopoietic Stem Cells. Leukemia (2007) 21(8):1733–8. doi: 10.1038/sj.leu.2404777
94. Ning H, Yang F, Jiang M, Hu L, Feng K, Zhang J, et al. The Correlation Between Cotransplantation of Mesenchymal Stem Cells and Higher Recurrence Rate in Hematologic Malignancy Patients: Outcome of a Pilot Clinical Study. Leukemia (2008) 22(3):593–9. doi: 10.1038/sj.leu.2405090
95. Fang B, Li N, Song Y, Li J, Zhao RC, Ma Y. Cotransplantation of Haploidentical Mesenchymal Stem Cells to Enhance Engraftment of Hematopoietic Stem Cells and to Reduce the Risk of Graft Failure in Two Children With Severe Aplastic Anemia. Pediatr Transplant (2009) 13(4):499–502. doi: 10.1111/j.1399-3046.2008.01002.x
96. Baron F, Lechanteur C, Willems E, Bruck F, Baudoux E, Seidel L, et al. Cotransplantation of Mesenchymal Stem Cells Might Prevent Death From Graft-Versus-Host Disease (GVHD) Without Abrogating Graft-Versus-Tumor Effects After HLA-Mismatched Allogeneic Transplantation Following Nonmyeloablative Conditioning. Biol Blood Marrow Transplant J Am Soc Blood Marrow Transplant (2010) 16(6):838–47. doi: 10.1016/j.bbmt.2010.01.011
97. Macmillan ML, Blazar BR, DeFor TE, Wagner JE. Transplantation of Ex-Vivo Culture-Expanded Parental Haploidentical Mesenchymal Stem Cells to Promote Engraftment in Pediatric Recipients of Unrelated Donor Umbilical Cord Blood: Results of a Phase I-II Clinical Trial. Bone Marrow Transplant (2009) 43(6):447–54. doi: 10.1038/bmt.2008.348
98. Bernardo ME, Ball LM, Cometa AM, Roelofs H, Zecca M, Avanzini MA, et al. Co-Infusion of Ex Vivo-Expanded, Parental MSCs Prevents Life-Threatening Acute GVHD, But Does Not Reduce the Risk of Graft Failure in Pediatric Patients Undergoing Allogeneic Umbilical Cord Blood Transplantation. Bone Marrow Transplant (2011) 46(2):200–7. doi: 10.1038/bmt.2010.87
99. Wang H, Yan H, Wang Z, Zhu L, Liu J, Guo Z. Cotransplantation of Allogeneic Mesenchymal and Hematopoietic Stem Cells in Children With Aplastic Anemia. Pediatrics (2012) 129(6):e1612–5. doi: 10.1542/peds.2011-2091
100. Lee SH, Lee MW, Yoo KH, Kim DS, Son MH, Sung KW, et al. Co-Transplantation of Third-Party Umbilical Cord Blood-Derived MSCs Promotes Engraftment in Children Undergoing Unrelated Umbilical Cord Blood Transplantation. Bone Marrow Transplant (2013) 48(8):1040–5. doi: 10.1038/bmt.2013.7
101. Liu Z, Zhang Y, Xiao H, Yao Z, Zhang H, Liu Q, et al. Cotransplantation of Bone Marrow-Derived Mesenchymal Stem Cells in Haploidentical Hematopoietic Stem Cell Transplantation in Patients With Severe Aplastic Anemia: An Interim Summary for a Multicenter Phase II Trial Results. Bone Marrow Transplant (2017) 52(5):704–10. doi: 10.1038/bmt.2016.347
102. Wang Z, Yu H, Cao F, Liu Z, Liu Z, Feng W, et al. Donor-Derived Marrow Mesenchymal Stromal Cell Co-Transplantation Following a Haploidentical Hematopoietic Stem Cell Transplantation Trail to Treat Severe Aplastic Anemia in Children. Ann Hematol (2019) 98(2):473–9. doi: 10.1007/s00277-018-3523-2
103. Ding L, Han DM, Zheng XL, Yan HM, Xue M, Liu J, et al. A Study of Human Leukocyte Antigen-Haploidentical Hematopoietic Stem Cells Transplantation Combined With Allogenic Mesenchymal Stem Cell Infusion for Treatment of Severe Aplastic Anemia in Pediatric and Adolescent Patients. Stem Cells Trans Med (2021) 10(2):291–302. doi: 10.1002/sctm.20-0345
104. Morata-Tarifa C, Macías-Sánchez MDM, Gutiérrez-Pizarraya A, Sanchez-Pernaute R. Mesenchymal Stromal Cells for the Prophylaxis and Treatment of Graft-Versus-Host Disease-a Meta-Analysis. Stem Cell Res Ther (2020) 11(1):64. doi: 10.1186/s13287-020-01592-z
105. Berger M, Mareschi K, Castiglia S, Rustichelli D, Mandese A, Migliore E, et al. In Vitro Mesenchymal Progenitor Cell Expansion is a Predictor of Transplant-Related Mortality and Acute GvHD III-IV After Bone Marrow Transplantation in Univariate Analysis: A Large Single-Center Experience. J Pediatr Hematol/Oncol (2019) 41(1):42–6. doi: 10.1097/MPH.0000000000001281
106. Fang B, Song Y, Lin Q, Zhang Y, Cao Y, Zhao RC, et al. Human Adipose Tissue-Derived Mesenchymal Stromal Cells as Salvage Therapy for Treatment of Severe Refractory Acute Graft-vs.-Host Disease in Two Children. Pediatr Transplant (2007) 11(7):814–7. doi: 10.1111/j.1399-3046.2007.00780.x
107. Lucchini G, Introna M, Dander E, Rovelli A, Balduzzi A, Bonanomi S, et al. Platelet-Lysate-Expanded Mesenchymal Stromal Cells as a Salvage Therapy for Severe Resistant Graft-Versus-Host Disease in a Pediatric Population. Biol Blood Marrow Transplant J Am Soc Blood Marrow Transplant (2010) 16(9):1293–301. doi: 10.1016/j.bbmt.2010.03.017
108. Prasad VK, Lucas KG, Kleiner GI, Talano JA, Jacobsohn D, Broadwater G, et al. Efficacy and Safety of Ex Vivo Cultured Adult Human Mesenchymal Stem Cells (Prochymal™) in Pediatric Patients With Severe Refractory Acute Graft-Versus-Host Disease in a Compassionate Use Study. Biol Blood Marrow Transplant J Am Soc Blood Marrow Transplant (2011) 17(4):534–41. doi: 10.1016/j.bbmt.2010.04.014
109. Ball LM, Bernardo ME, Roelofs H, van Tol MJD, Contoli B, Zwaginga JJ, et al. Multiple Infusions of Mesenchymal Stromal Cells Induce Sustained Remission in Children With Steroid-Refractory, Grade III-IV Acute Graft-Versus-Host Disease. Br J Haematol (2013) 163(4):501–9. doi: 10.1111/bjh.12545
110. Dotoli GM, De Santis GC, Orellana MD, de Lima Prata K, Caruso SR, Fernandes TR, et al. Mesenchymal Stromal Cell Infusion to Treat Steroid-Refractory Acute GvHD III/IV After Hematopoietic Stem Cell Transplantation. Bone Marrow Transplant (2017) 52(6):859–62. doi: 10.1038/bmt.2017.35
111. Salmenniemi U, Itälä-Remes M, Nystedt J, Putkonen M, Niittyvuopio R, Vettenranta K, et al. Good Responses But High TRM in Adult Patients After MSC Therapy for GvHD. Bone Marrow Transplant (2017) 52(4):606–8. doi: 10.1038/bmt.2016.317
112. Bonig H, Kuçi Z, Kuçi S, Bakhtiar S, Basu O, Bug G, et al. Children and Adults With Refractory Acute Graft-Versus-Host Disease Respond to Treatment With the Mesenchymal Stromal Cell Preparation "MSC-FFM"-Outcome Report of 92 Patients. Cells (2019) 8(12). doi: 10.3390/cells8121577
113. Erbey F, Atay D, Akcay A, Ovali E, Ozturk G. Mesenchymal Stem Cell Treatment for Steroid Refractory Graft-Versus-Host Disease in Children: A Pilot and First Study From Turkey. Stem Cells Int (2016) 2016:1641402. doi: 10.1155/2016/1641402
114. Martin PJ, Uberti JP, Soiffer RJ, Klingemann H, Waller EK, Daly AS, et al. Prochymal Improves Response Rates In Patients With Steroid-Refractory Acute Graft Versus Host Disease (SR-GVHD) Involving The Liver And Gut: Results Of A Randomized, Placebo-Controlled, Multicenter Phase III Trial In GVHD. Biol Blood Marrow Transplant 16(2):S169–70. doi: 10.1016/j.bbmt.2009.12.057
115. Kebriaei P, Hayes J, Daly A, Uberti J, Marks DI, Soiffer R, et al. A Phase 3 Randomized Study of Remestemcel-L Versus Placebo Added to Second-Line Therapy in Patients With Steroid-Refractory Acute Graft-Versus-Host Disease. Biol Blood Marrow Transplant J Am Soc Blood Marrow Transplant (2020) 26(5):835–44. doi: 10.1016/j.bbmt.2019.08.029
116. Kurtzberg J, Prockop S, Chaudhury S, Horn B, Nemecek E, Prasad V, et al. Study 275: Updated Expanded Access Program for Remestemcel-L in Steroid-Refractory Acute Graft-Versus-Host Disease in Children. Biol Blood Marrow Transplant J Am Soc Blood Marrow Transplant (2020) 26(5):855–64. doi: 10.1016/j.bbmt.2020.01.026
117. Kasikis S, Baez J, Gandhi I, Grupp S, Kitko CL, Kowalyk S, et al. Mesenchymal Stromal Cell Therapy Induces High Responses and Survival in Children With Steroid Refractory GVHD and Poor Risk Biomarkers. Bone Marrow Transplant (2021) 56(11):2869–70. doi: 10.1038/s41409-021-01442-3
118. Ringdén O, Gustafsson B, Sadeghi B. Mesenchymal Stromal Cells in Pediatric Hematopoietic Cell Transplantation a Review and a Pilot Study in Children Treated With Decidua Stromal Cells for Acute Graft-Versus-Host Disease. Front Immunol (2020) 11:567210. doi: 10.3389/fimmu.2020.567210
119. Sadeghi B, Remberger M, Gustafsson B, Winiarski J, Moretti G, Khoein B, et al. Long-Term Follow-Up of a Pilot Study Using Placenta-Derived Decidua Stromal Cells for Severe Acute Graft-Versus-Host Disease. Biol Blood Marrow Transplant J Am Soc Blood Marrow Transplant (2019) 25(10):1965–9. doi: 10.1016/j.bbmt.2019.05.034
120. Weng JY, Du X, Geng SX, Peng YW, Wang Z, Lu ZS, et al. Mesenchymal Stem Cell as Salvage Treatment for Refractory Chronic GVHD. Bone Marrow Transplant (2010) 45(12):1732–40. doi: 10.1038/bmt.2010.195
121. Pérez-Simon JA, López-Villar O, Andreu EJ, Rifón J, Muntion S, Diez Campelo M, et al. Mesenchymal Stem Cells Expanded In Vitro With Human Serum for the Treatment of Acute and Chronic Graft-Versus-Host Disease: Results of a Phase I/II Clinical Trial. Haematologica (2011) 96(7):1072–6. doi: 10.3324/haematol.2010.038356
122. Krasowska-Kwiecien A, Gozdzik J, Jarocha D, Wozniak M, Czogala W, Wiecha O, et al. Mesenchymal Stem Cells as a Salvage Treatment for Severe Refractory Graft-Vs-Host Disease in Children After Bone Marrow Transplantation. Transplant Proc (2019) 51(3):880–9. doi: 10.1016/j.transproceed.2019.01.023
123. Gao L, Zhang Y, Hu B, Liu J, Kong P, Lou S, et al. Phase II Multicenter, Randomized, Double-Blind Controlled Study of Efficacy and Safety of Umbilical Cord-Derived Mesenchymal Stromal Cells in the Prophylaxis of Chronic Graft-Versus-Host Disease After HLA-Haploidentical Stem-Cell Transplantation. J Clin Oncol (2016) 34(24):2843–50. doi: 10.1200/JCO.2015.65.3642
124. Hassan Z, Remberger M, Svenberg P, Elbander M, Omazic B, Mattsson J, et al. Hemorrhagic Cystitis: A Retrospective Single-Center Survey. Clin Transplant (2007) 21(5):659–67. doi: 10.1111/j.1399-0012.2007.00705.x
125. Ringdén O, Leblanc K. Pooled MSCs for Treatment of Severe Hemorrhage. Bone Marrow Transplant (2011) 46(8):1158–60. doi: 10.1038/bmt.2010.262
126. Tong J, Liu H, Zheng C, Zhu X, Tang B, Wan X, et al. Effects and Long-Term Follow-Up of Using Umbilical Cord Blood-Derived Mesenchymal Stromal Cells in Pediatric Patients With Severe BK Virus-Associated Late-Onset Hemorrhagic Cystitis After Unrelated Cord Blood Transplantation. Pediatr Transplant (2020) 24(2):e13618. doi: 10.1111/petr.13618
127. Rubenfeld GD, Caldwell E, Peabody E, Weaver J, Martin DP, Neff M, et al. Incidence and Outcomes of Acute Lung Injury. New Engl J Med (2005) 353(16):1685–93. doi: 10.1056/NEJMoa050333
128. Matthay MA, Zemans RL. The Acute Respiratory Distress Syndrome: Pathogenesis and Treatment. Annu Rev Pathol (2011) 6:147–63. doi: 10.1146/annurev-pathol-011110-130158
129. Meyer NJ, Gattinoni L, Calfee CS. Acute Respiratory Distress Syndrome. Lancet (2021) 398(10300):622–37. doi: 10.1016/S0140-6736(21)00439-6
130. Gao J, Dennis JE, Muzic RF, Lundberg M, Caplan AI. The Dynamic In Vivo Distribution of Bone Marrow-Derived Mesenchymal Stem Cells After Infusion. Cells Tissues Organs (2001) 169(1):12–20. doi: 10.1159/000047856
131. Ringden O. Mesenchymal Stem (Stromal) Cells for Treatment of Acute Respiratory Distress Syndrome. Lancet Respir Med (2015) 3(4):e12. doi: 10.1016/S2213-2600(15)00047-8
132. Zheng G, Huang L, Tong H, Shu Q, Hu Y, Ge M, et al. Treatment of Acute Respiratory Distress Syndrome With Allogeneic Adipose-Derived Mesenchymal Stem Cells: A Randomized, Placebo-Controlled Pilot Study. Respir Res (2014) 15(1):39. doi: 10.1186/1465-9921-15-39
133. Wilson JG, Liu KD, Zhuo H, Caballero L, McMillan M, Fang X, et al. Mesenchymal Stem (Stromal) Cells for Treatment of ARDS: A Phase 1 Clinical Trial. Lancet Respir Med (2015) 3(1):24–32. doi: 10.1016/S2213-2600(14)70291-7
134. Matthay MA, Calfee CS, Zhuo H, Thompson BT, Wilson JG, Levitt JE, et al. Treatment With Allogeneic Mesenchymal Stromal Cells for Moderate to Severe Acute Respiratory Distress Syndrome (START Study): A Randomised Phase 2a Safety Trial. Lancet Respir Med (2019) 7(2):154–62. doi: 10.1016/S2213-2600(18)30418-1
135. Cruz FF, Rocco PRM. Cell Therapy for Acute Respiratory Distress Syndrome Patients: The START Study. J Thorac Dis (2019) 11(Suppl 9):S1329–32. doi: 10.21037/jtd.2019.04.22
136. Ringdén O, Solders M, Erkers T, Nava S, Mollden P, Hultkrantz M, et al. Successful Reversal of Acute Lung Injury Using Placenta-Derived Decidual Stromal Cells. J Stem Cell Res Ther (2014) 4:244. doi: 10.4172/2157-7633.1000244
137. Mehta P, McAuley DF, Brown M, Sanchez E, Tattersall RS, Manson JJ. COVID-19: Consider Cytokine Storm Syndromes and Immunosuppression. Lancet (2020) 395(10229):1033–4. doi: 10.1016/S0140-6736(20)30628-0
138. Hamdan H, Hashmi SK, Lazarus H, Gale RP, Qu W, El Fakih R. Promising Role for Mesenchymal Stromal Cells in Coronavirus Infectious Disease-19 (COVID-19)-Related Severe Acute Respiratory Syndrome? Blood Rev (2021) 46:100742. doi: 10.1016/j.blre.2020.100742
139. Magunia H, Guerrero AM, Keller M, Jacoby J, Schlensak C, Haeberle H, et al. Extubation and Noninvasive Ventilation of Patients Supported by Extracorporeal Life Support for Cardiogenic Shock: A Single-Center Retrospective Observational Cohort Study. J Intensive Care Med (2021) 36(7):783–92. doi: 10.1177/0885066620918171
140. Hashemian SR, Aliannejad R, Zarrabi M, Soleimani M, Vosough M, Hosseini SE, et al. Mesenchymal Stem Cells Derived From Perinatal Tissues for Treatment of Critically Ill COVID-19-Induced ARDS Patients: A Case Series. Stem Cell Res Ther (2021) 12(1):91. doi: 10.1186/s13287-021-02165-4
141. Sengupta V, Sengupta S, Lazo A, Woods P, Nolan A, Bremer N. Exosomes Derived From Bone Marrow Mesenchymal Stem Cells as Treatment for Severe COVID-19. Stem Cells Dev (2020) 29(12):747–54. doi: 10.1089/scd.2020.0080
142. Putra A, Widyatmoko A, Ibrahim S, Amansyah F, Amansyah F, Berlian M, et al. Case Series of the First Three Severe COVID-19 Patients Treated With the Secretome of Hypoxia-Mesenchymal Stem Cells in Indonesia [Version 1; Peer Review: 1 Approved, 1 Approved With Reservations]. F1000Research (2021) 10(228). doi: 10.12688/f1000research.51191.3
143. Chen X, Wang C, Yin J, Xu J, Wei J, Zhang Y. Efficacy of Mesenchymal Stem Cell Therapy for Steroid-Refractory Acute Graft-Versus-Host Disease Following Allogeneic Hematopoietic Stem Cell Transplantation: A Systematic Review and Meta-Analysis. PloS One (2015) 10(8):e0136991. doi: 10.1371/journal.pone.0136991
144. Zeiser R, von Bubnoff N, Butler J, Mohty M, Niederwieser D, Or R, et al. Ruxolitinib for Glucocorticoid-Refractory Acute Graft-Versus-Host Disease. N Engl J Med (2020) 382(19):1800–10. doi: 10.1056/NEJMoa1917635
145. Khandelwal P, Teusink-Cross A, Davies SM, Nelson AS, Dandoy CE, El-Bietar J, et al. Ruxolitinib as Salvage Therapy in Steroid-Refractory Acute Graft-Versus-Host Disease in Pediatric Hematopoietic Stem Cell Transplant Patients. Biol Blood Marrow Transplant J Am Soc Blood Marrow Transplant (2017) 23(7):1122–7. doi: 10.1016/j.bbmt.2017.03.029
146. Kuci Z, Bonig H, Kreyenberg H, Bunos M, Jauch A, Janssen JW, et al. Mesenchymal Stromal Cells Generated From Pooled Mononuclear Cells of Multiple Bone Marrow Donors as a Rescue Therapy for Children With Severe Steroid-Refractory Graft Versus Host Disease: A Multicenter Survey. Haematologica (2016). doi: 10.3324/haematol.2015.140368
147. Gander R, Asensio M, Guillén G, Royo GF, Bolaños A, Pérez M, et al. Hemorrhagic Cystitis After Hematopoietic Stem Cell Transplantation: A Challenge for the Pediatric Urologist. J Pediatr Urol (2018) 14(5):366–73. doi: 10.1016/j.jpurol.2018.03.018
148. Le Blanc K, Ringdén O. Immunomodulation by Mesenchymal Stem Cells and Clinical Experience. J Internal Med (2007) 262(5):509–25. doi: 10.1111/j.1365-2796.2007.01844.x
149. Matthay MA, Zhuo H, Gotts JE, Liu KD, Calfee CS. Precision Medicine for Cell Therapy in Acute Respiratory Distress Syndrome - Authors' Reply. Lancet Respir Med (2019) 7(4):e14. doi: 10.1016/S2213-2600(19)30083-9
150. Luetzkendorf J, Nerger K, Hering J, Moegel A, Hoffmann K, Hoefers C, et al. Cryopreservation Does Not Alter Main Characteristics of Good Manufacturing Process-Grade Human Multipotent Mesenchymal Stromal Cells Including Immunomodulating Potential and Lack of Malignant Transformation. Cytotherapy (2015) 17(2):186–98. doi: 10.1016/j.jcyt.2014.10.018
151. Gramlich OW, Burand AJ, Brown AJ, Deutsch RJ, Kuehn MH, Ankrum JA. Cryopreserved Mesenchymal Stromal Cells Maintain Potency in a Retinal Ischemia/Reperfusion Injury Model: Toward an Off-the-Shelf Therapy. Sci Rep (2016) 6:26463. doi: 10.1038/srep26463
152. Burand AJ, Gramlich OW, Brown AJ, Ankrum JA. Function of Cryopreserved Mesenchymal Stromal Cells With and Without Interferon-Gamma Prelicensing is Context Dependent. Stem Cells (2017) 35(5):1437–9. doi: 10.1002/stem.2528
153. Tan Y, Salkhordeh M, Wang JP, McRae A, Souza-Moreira L, McIntyre L, et al. Thawed Mesenchymal Stem Cell Product Shows Comparable Immunomodulatory Potency to Cultured Cells In Vitro and in Polymicrobial Septic Animals. Sci Rep (2019) 9(1):18078. doi: 10.1038/s41598-019-54462-x
154. Oja S, Kaartinen T, Ahti M, Korhonen M, Laitinen A, Nystedt J. The Utilization of Freezing Steps in Mesenchymal Stromal Cell (MSC) Manufacturing: Potential Impact on Quality and Cell Functionality Attributes. Front Immunol (2019) 10:1627. doi: 10.3389/fimmu.2019.01627
155. Parolini O, Alviano F, Bagnara GP, Bilic G, Buhring HJ, Evangelista M, et al. Concise Review: Isolation and Characterization of Cells From Human Term Placenta: Outcome of the First International Workshop on Placenta Derived Stem Cells. Stem Cells (2008) 26(2):300–11. doi: 10.1634/stemcells.2007-0594
156. Prockop DJ. Marrow Stromal Cells as Stem Cells for Nonhematopoietic Tissues. Science (1997) 276(5309):71–4. doi: 10.1126/science.276.5309.71
157. Shi L, Huang H, Lu X, Yan X, Jiang X, Xu R, et al. Effect of Human Umbilical Cord-Derived Mesenchymal Stem Cells on Lung Damage in Severe COVID-19 Patients: A Randomized, Double-Blind, Placebo-Controlled Phase 2 Trial. Signal Transduct Target Ther (2021) 6(1):58. doi: 10.1038/s41392-021-00488-5
158. Xu X, Jiang W, Chen L, Xu Z, Zhang Q, Zhu M, et al. Et Al: Evaluation of the Safety and Efficacy of Using Human Menstrual Blood-Derived Mesenchymal Stromal Cells in Treating Severe and Critically Ill COVID-19 Patients: An Exploratory Clinical Trial. Clin Trans Med (2021) 11(2):e297.
159. Sadeghi B, Ersmark B, Moretti G, Mattsson J, Ringdén O. Treatment of Radiculomyelopathy in Two Patients With Placenta-Derived Decidua Stromal Cells. Int J Hematol (2020) 111(4):591–4. doi: 10.1007/s12185-019-02804-w
160. Horwitz EM, Prockop DJ, Gordon PL, Koo WW, Fitzpatrick LA, Neel MD, et al. Clinical Responses to Bone Marrow Transplantation in Children With Severe Osteogenesis Imperfecta. Blood (2001) 97(5):1227–31. doi: 10.1182/blood.V97.5.1227
161. Horwitz EM, Prockop DJ, Fitzpatrick LA, Koo WW, Gordon PL, Neel M, et al. Transplantability and Therapeutic Effects of Bone Marrow-Derived Mesenchymal Cells in Children With Osteogenesis Imperfecta. Nat Med (1999) 5(3):309–13. doi: 10.1038/6529
162. Otsuru S, Gordon PL, Shimono K, Jethva R, Marino R, Phillips CL, et al. Transplanted Bone Marrow Mononuclear Cells and MSCs Impart Clinical Benefit to Children With Osteogenesis Imperfecta Through Different Mechanisms. Blood (2012) 120(9):1933–41. doi: 10.1182/blood-2011-12-400085
163. Koç ON, Day J, Nieder M, Gerson SL, Lazarus HM, Krivit W. Allogeneic Mesenchymal Stem Cell Infusion for Treatment of Metachromatic Leukodystrophy (MLD) and Hurler Syndrome (MPS-Ih). Bone Marrow Transplant (2002) 30(4):215–22.
164. Valade G, Libert N, Martinaud C, Vicaut E, Banzet S, Peltzer J. Therapeutic Potential of Mesenchymal Stromal Cell-Derived Extracellular Vesicles in the Prevention of Organ Injuries Induced by Traumatic Hemorrhagic Shock. Front Immunol (2021) 12:749659. doi: 10.3389/fimmu.2021.749659
Keywords: cellular therapy, mesenchymal stromal cells (MSCs), immunomodulation, regeneration, hematopoietic engraftment, graft-versus host disease (GvHD), acute respiratory distress syndrome (ARDS), coronavirus-induced disease 2019 (COVID-19)
Citation: Ringdén O, Moll G, Gustafsson B and Sadeghi B (2022) Mesenchymal Stromal Cells for Enhancing Hematopoietic Engraftment and Treatment of Graft-Versus-Host Disease, Hemorrhages and Acute Respiratory Distress Syndrome. Front. Immunol. 13:839844. doi: 10.3389/fimmu.2022.839844
Received: 20 December 2021; Accepted: 17 February 2022;
Published: 18 March 2022.
Edited by:
Selim Kuci, University Hospital Frankfurt, GermanyReviewed by:
Philippe Lewalle, Jules Bordet Institute, BelgiumMehdi Najar, Université libre de Bruxelles, Belgium
Copyright © 2022 Ringdén, Moll, Gustafsson and Sadeghi. This is an open-access article distributed under the terms of the Creative Commons Attribution License (CC BY). The use, distribution or reproduction in other forums is permitted, provided the original author(s) and the copyright owner(s) are credited and that the original publication in this journal is cited, in accordance with accepted academic practice. No use, distribution or reproduction is permitted which does not comply with these terms.
*Correspondence: Olle Ringdén, olle.ringden@ki.se; Guido Moll, guido.moll@charite.de; Britt Gustafsson, britt.gustafsson@ki.se; Behnam Sadeghi, behnam.sadeghi@ki.se
†ORCID: Olle Ringdén, orcid.org/0000-0002-6092-1536
Guido Moll, orcid.org/0000-0001-6173-5957
Britt Gustafsson, orcid.org/0000-0002-7080-5053
Behnam Sadeghi, orcid.org/0000-0002-8721-0400