- 1Department of Microbiology and Immunology, Graduate Institute of Biomedical Sciences, Chang Gung University, Taoyuan, Taiwan
- 2Department of Pediatrics, Molecular Infectious Disease Research Center, Chang Gung Memorial Hospital at Linkou, Taoyuan, Taiwan
- 3Department of Microbiology, School of Medicine, China Medical University, Taichung, Taiwan
- 4Department of Nursing, Asia University, Taichung, Taiwan
- 5Department of Medical Biotechnology and Laboratory Science, College of Medicine, Chang Gung University, Taoyuan, Taiwan
- 6Microbiota Research Center and Emerging Viral Infections Research Center, Chang Gung University, Taoyuan, Taiwan
- 7Molecular Medicine Research Center, Chang Gung University, Taoyuan, Taiwan
- 8Department of Laboratory Medicine, Chang Gung Memorial Hospital at Linkou, Taoyuan, Taiwan
- 9Department of Otolaryngology-Head and Neck Surgery, Chang Gung Memorial Hospital, Taoyuan, Taiwan
- 10Medical Research Center, Xiamen Chang Gung hospital, Xiamen, China
- 11Research Center for Chinese Herbal Medicine, College of Human Ecology, Chang Gung University of Science and Technology, Taoyuan, Taiwan
Helicobacter pylori infection is closely associated with various gastrointestinal diseases and poses a serious threat to human health owing to its increasing antimicrobial resistance. H. pylori possesses two major virulence factors, vacuolating cytotoxin A (VacA) and cytotoxin-associated gene A (CagA), which are involved in its pathogenesis. Probiotics have recently been used to eradicate H. pylori infection and reduce the adverse effects of antibiotic-based therapies. Parabacteroides goldsteinii MTS01 is a novel next-generation probiotic (NGP) with activities that can alleviate specific diseases by altering the gut microbiota. However, the mechanism by which P. goldsteinii MTS01 exerts its probiotic effects against H. pylori infection remains unclear. Our results showed that administration of P. goldsteinii MTS01 to H. pylori-infected model mice altered the composition of the gut microbiota and significantly reduced serum cholesterol levels, which mitigated H. pylori-induced gastric inflammation. In addition, the pathogenic effects of H. pylori VacA and CagA on gastric epithelial cells were markedly abrogated by treatment with P. goldsteinii MTS01. These results indicate that P. goldsteinii MTS01 can modulate gut microbiota composition and has anti-virulence factor functions, and thus could be developed as a novel functional probiotic for reducing H. pylori-induced pathogenesis.
Introduction
Persistent Helicobacter pylori infection in the human stomach is closely associated with the development of various gastrointestinal diseases, including chronic gastritis, peptic ulcers, and gastric cancer (1). Combination therapies using several antibiotics and either a proton pump inhibitor (PPI) or bismuth are widely employed to eradicate H. pylori and relieve related gastrointestinal illnesses (2). However, a consequent increase in antibiotic resistance and changes in the gut microbiota often occur (3, 4).
The two main virulence factors of H. pylori, vacuolating cytotoxin A (VacA) and cytotoxin-associated gene A (CagA), are involved in its pathogenesis (5). VacA is a pore-forming toxin that promotes acidic vacuole formation in host cells and inhibits mitochondrial function, resulting in apoptosis (6). CagA, which is encoded on the cag-pathogenicity island (cag-PAI), is delivered to host cells through the type IV secretion system (TFSS) and activates nuclear factor-kappa B (NF-κB) and IL-8 secretion, leading to inflammation and, in some cases, oncogenesis (7). Notably, these two virulence factors exploit cholesterol-rich microdomains in membranes, which are referred to as lipid rafts, for cell binding, intracellular delivery, and cell intoxication (8, 9). Therefore, depleting cholesterol to disrupt lipid rafts is considered an ideal strategy for alleviating H. pylori-related diseases (10).
Probiotics and their metabolites play crucial roles in promoting host health, including maintaining physiological homeostasis, improving intestinal integrity, and producing antimicrobial peptides (11). Recently, several traditional probiotics were used to eradicate H. pylori infection and reduce the adverse effects of antibiotic-based therapies (2). However, the effectiveness of probiotics for eradicating H. pylori is controversial (12, 13). The conflicts regarding the clinical effectiveness of traditional probiotics could be resolved by next-generation probiotics (NGP), which have fully defined genetic backgrounds and physiological characteristics that target specific diseases (14).
Parabacteroides goldsteinii MTS01, a newly discovered NGP, exerts multiple disease-alleviating effects, including obesity-reversing, insulin resistance-controlling, and COPD pathogenesis-ameliorating effects, by altering the gut microbiota (15–17). A recent study reported that a loss of P. goldsteinii may exacerbate colitis in model mice (18). However, whether P. goldsteinii MTS01 can alter this microbial ecosystem and mitigate H. pylori-induced pathogenesis in the stomach remains to be explored. In this study, we comprehensively investigated the mechanisms by which P. goldsteinii MTS01 inhibits H. pylori infection and ameliorates inflammation in the stomach using a mouse model. Our results showed that administration of P. goldsteinii MTS01 to the model mice altered the composition of the gut microbiota, reduced serum cholesterol, and effectively attenuated the actions of H. pylori VacA and CagA. These findings suggest that P. goldsteinii MTS01 could be developed as a functional probiotic against H. pylori-associated pathogenesis.
Materials and Methods
Bacterial Culture
H. pylori strain 26695 (ATCC 700392) was cultured on the blood agar plates (Brucella agar with 10% defibrinated sheep blood) and incubated at 37°C in microaerophilic environment (5% O2, 10% CO2, and 85% N2). P. goldsteinii MTS01 was isolated from the feces of a healthy adult who has taken P. goldsteinii MTS01 previously isolated from a healthy mouse (15) for half a year. It was cultured in thioglycollate medium (BD Biosciences) at 37°C in an anaerobic chamber as described previously (15). Culture supernatant from P. goldsteinii MTS01 was filtrated using a 0.22 μm filter. Heat-inactivated P. goldsteinii MTS01 was prepared by boiling 1×108 CFU/ml of bacterial suspension in PBS for 30 min. Live, heat-inactivated, and culture broth of P. goldsteinii MTS01 were collected for following analyses.
Animal Experiments
Six-week-old male BALB/c mice were purchased from the National Laboratory Animal Center (Taipei, Taiwan). The mouse experiments were performed in accordance with the Animal Care and Use Guidelines for Chang Gung University under a protocol approved by the Institutional Animal Care Use Committee (IACUC Approval No.: CGU107-141). The body weight and rectal temperature were recorded every morning during the study period (Supplementary Figure 1). Mice were randomly divided into 4 groups for the treatments with vehicle control (PBS) (n = 9), P. goldsteinii MTS01 (n = 10), H. pylori (n = 8), and P. goldsteinii MTS01 + H. pylori (n = 10), respectively (Figure 1). Mice were fed with P. goldsteinii MTS01 (2×108 CFU/100 μl) by intragastric gavage once daily for a total of 9 weeks. Mice were then inoculated with H. pylori (1×109 CFU/100 μl) once every two days and continuously fed with P. goldsteinii MTS01 (2×108 CFU/100 μl) once daily for additional two weeks. After completing the treatment course, mice were euthanized and the blood samples, gastric tissues, and intestinal stool were prepared for further analysis, as described previously (19).
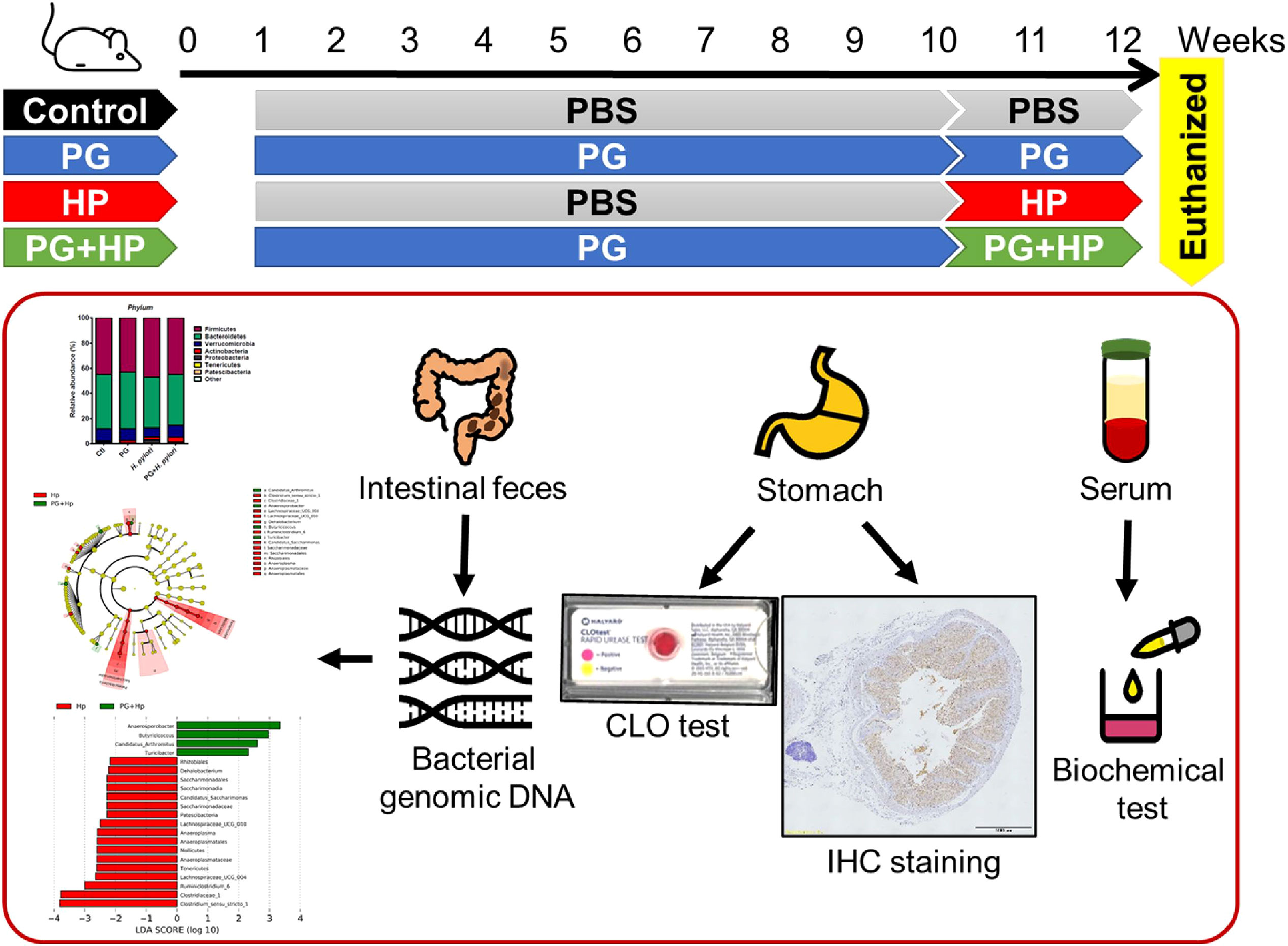
Figure 1 Murine model for experimental studies. Mice were divided into four groups for the treatments with vehicle-control (PBS, n = 9), P. goldsteinii MTS01 (PG, n = 10), H. pylori (HP, n = 8), and P. goldsteinii MTS01 + H. pylori (n = 10). Mice were intragastrically administered P. goldsteinii MTS01 (2×108 CFU/100 μl) once daily for a total of nine weeks and continuously inoculated with P. goldsteinii MTS01 and H. pylori (1×109 CFU/100 μl) once daily for additional two weeks. Mice were euthanized and the samples of blood, stomach, and stool were prepared for further analysis.
Serum Cholesterol/Triglyceride Analysis
Mouse blood was collected by cardiac puncture and centrifuged immediately (7000 rpm for 10 min) to prepare the serum. Serum triglyceride was analyzed using a Triglyceride Assay Kit (ab65336, Abcam). The levels of total cholesterol, high-density lipoprotein (HDL), low-density lipoprotein (LDL), and very-low-density lipoproteins (VLDL) in serum were assayed by a Cholesterol Assay ELISA Kit (ab65390, Abcam).
Rapid Urease Test
CLO test (Kimberly Clark), a rapid urease test used to determine H. pylori infection. A gastric biopsy from mice was added into the CLO test microtube and incubated at room temperature for 3 h. The change in color of the gastric biopsies from yellow to red, which indicated the positive colonization of H. pylori (20).
Histopathological Analysis
Murine gastric tissues were prepared for immunohistochemistry (IHC) staining as described previously (16). The tissue sections were stained with monoclonal antibodies (Abcam) against COX-2 (1:1000), IL-1β (1:100), and TNF-α (1:100) at 4°C for 18 h. After washing, the tissue sections were incubated with a horseradish peroxidase (HRP)-conjugated goat anti-rabbit secondary antibody (1:000, GBI Labs) and developed with an ABC kit (Vector Laboratories). The stained tissues were analyzed by a histopathologist using a light microscope (Carl Zeiss).
Gut Microbiota Analysis
Gut or fecal microbiota containing both intestinal and gastric microbiota may affect the pathology of H. pylori infection (21, 22), and we analyzed their composition. Microbiota DNA in mouse stool was extracted using the QIAamp PowerFecal Pro DNA Kit (Qiagen) and applied to the amplification of 16S rRNA V3–V4 region using PCR, as described previously (23, 24). In brief, 16S amplicon libraries were constructed by two-step PCR reactions and the concentrations of each library was assessed using an HT DNA High Sensitivity LabChip kit (Caliper). The multiplexed amplified libraries were sequenced using the Illumina MiSeq system. The amplicon sequences were analyzed by following the MiSeq SOP (25). As a result, a total of 986 amplicon sequence variants (ASVs) was obtained, then ASVs were annotated into taxonomic orders using the get_taxa_unique function in phyloseq (26). The species richness among the gut microbiota was determined using the alpha diversity measures: observed species richness, Shannon, and Fisher’s diversity index by phyloseq. Two-dimensional PCoA plots were used to analyze the bacterial community populations between two groups using phyloseq. Beta diversity was conducted by using weighted Unifrac phylogenetic distance matrices (27). A PERMANOVA (α = 0.05) with 999 random permutations was run to determine differences between groups with the Vegan package. The cladogram functionality of the linear discriminant analysis (LDA) effect size (LEfSe) method was used to illustrate differences in gut microbiota composition between un-treatment and treatment with P. goldsteinii MTS01. In the cladogram, differences between groups were illustrated at phyla, class, order, family, and genus levels. PICRUSt was used to predict the microbial functionality profiles by generating the Kyoto Encyclopedia of Genes and Genomes (KEGG) pathway according to 16S rRNA gene sequencing (28). The predicted genes were aligned to KEGG database and the different pathways among groups were compared using STAMP (version 2.1.3) with two-side Welch’s t-test.
Cell Culture
AGS cells (ATCC CRL-1739, human gastric epithelial cell line) were cultured in F12 medium (Hyclone) containing 10% fetal bovine serum (Hyclone) and incubated at 37°C in an incubator with 5% CO2.
Determination of Cell Elongated Phenotype
AGS cells were pretreated with P. goldsteinii MTS01 (MOI = 200) followed by infection with H. pylori (MOI = 100) for 6 h. H. pylori VacA-induced cell vacuolation and CagA-induced elongated cells (hummingbird phenotype) were observed. The percentage of elongated cells was determined as reported previously (29).
CagA Phosphorylation Assay
AGS cells were pretreated with P. goldsteinii MTS01 (MOI 200) for 30 min followed by infection with H. pylori (MOI = 100) for additional 6 h. The cell extracts were prepared and subjected to 6% SDS-PAGE then transferred onto polyvinylidene difluoride membranes (1:1000, Millipore). The membranes were incubated with anti-phosphotyrosine (4G10) antibody (1:2000, Millipore) overnight at 4°C, and then probed with a horseradish peroxidase-conjugated secondary antibody (Millipore). The expression level of phospho-CagA was analyzed using ECL western blotting detection reagents (GE Healthcare) and recorded by AzureSpot Analysis Software with Azure 400 (Azure Biosystems).
NF-κB Luciferase Activity Assay
AGS cells were transfected with NF-κB-luciferase reporter followed by treatment with P. goldsteinii MTS01 (MOI = 200) and H. pylori (MOI = 100) for 6 h. The cell lysates were prepared and subjected to luciferase assays using Dual-Luciferase Reporter Assay System (Promega). Luciferase activity was normalized by co-transfection of β-galactosidase expression vector (Promega), as described previously (29).
Analysis of IL-8 Production
Culture supernatant from AGS cells was collected. The IL-8 level was analyzed using enzyme-linked immunosorbent (ELISA) assay according to the manufacturer’s instructions (Invitrogen) (30).
Statistical Analysis
Differential abundance was analyzed using the Kruskal-Wallis to detect main effect differences and then applied to Wilcoxon rank-sum test for pairwise comparisons (24). Mann–Whitney U test was used to evaluate differences in microbiota abundance (31). In the biochemistry and cell-based studies, statistical significance was determined by Student’s t-test between two groups. One-way ANOVA test with Tukey post-hoc test was used to assess the statistical significance of the experimental results for more than two studied groups. The statistical analysis was conducted using SPSS program (version 18.0 for Windows, SPSS Inc.) and the figures were performed by Prism program (version 9.0.0 for Windows, GraphPad Software). A P value less than 0.05 was considered statistically significant.
Results
P. goldsteinii MTS01 Influences the Gut Microbiota Diversity
To assess the effects of P. goldsteinii MTS01 on H. pylori-induced gastric inflammation in vivo, mice were inoculated with live P. goldsteinii MTS01 via intragastric gavage before H. pylori infection. Mice were divided into the following four groups: control (PBS), P. goldsteinii MTS01 (PG), H. pylori (HP), and PG+HP (Figure 1). After treatment, the mice were euthanized, and intestinal feces were collected to characterize the gut microbiota using 16S rRNA V3–V4 sequencing. The average number of raw reads per sample was 198,893 for the 37 specimens tested (Supplementary Table 1). A total of 4,767,390 high-quality filtered reads (mean, 128,848.4 ± 23,599.6 reads per sample) were obtained. The reads were constructed into 986 ASVs, and these sequence variants were used for taxonomic classification. The ASVs were then categorized into 8 phyla, 13 classes, 19 orders, 29 families, and 75 genera. The taxonomic and phylogenetic data for ASVs are shown in Supplementary Table 2.
We then compared the composition and diversity of the microbiota in mice subjected to the different treatments. Compared to the control group, the gut microbiota communities of H. pylori-infected mice had significantly higher richness indices, including the abundance-based coverage estimator (ACE)-diversity index and Fisher’s index (Figures 2A, B). In contrast, the microbiota communities in P. goldsteinii MTS01-treated and H. pylori-infected (PG+HP) mice were less diverse than those in H. pylori-infected (HP) mice, as shown by the four alpha diversity indices (Figures 2A–D). Principal coordinate analysis (PCoA) was used to examine the beta diversity of the four treatment groups. Our results showed that the spatial separation of the gut microbiota composition in the PCoA plot varied among the different treatments (Adonis test, P=0.001 and R2 = 0.37; Figure 2E). These results indicated that the gut microbiota profiles differed among the four treatment groups.
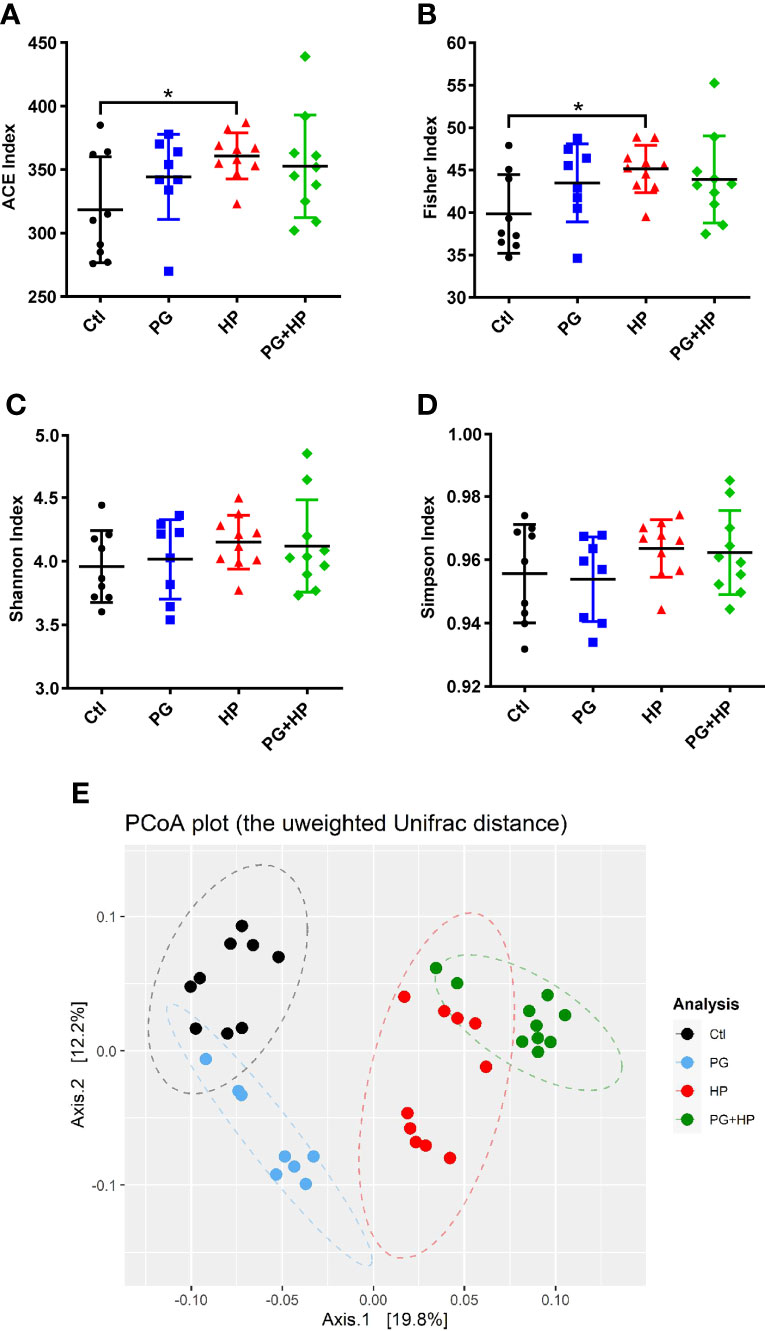
Figure 2 P. goldsteinii MTS01 and H pylori alter the gut microbiota communities. Diversity index of (A) ACE, (B) Fisher, (C) Shannon, and (D) Simpson were performed to analyze microbiota diversity in murine intestinal stool. *P < 0.05 was assessed using Student’s t-test. (E) Analysis of the gut microbiota diversity. PCoA was conducted to analyze the gut microbiota composition in mice administered with vehicle-control (black), P. goldsteinii MTS01 (blue), H. pylori (red), and P. goldsteinii MTS01 + H. pylori (green).
P. goldsteinii MTS01 Alters Relative Abundance of Gut Microbiota
Next, we investigated the profiles of the major microbiota at phylum and genus levels in the four treatment groups. In the control group, the top seven most abundant phyla were Firmicutes (44.8 ± 8.1%), Bacteroidetes (43.2 ± 4.6%), Verrucomicrobia (9.7 ± 6.9%), Actinobacteria (0.98 ± 0.4%), Proteobacteria (1.1 ± 1.7%), Tenericutes (0.1 ± 0.1%), and Patescibacteria (0.02 ± 0.01%; Figure 3). There were no significant differences in the levels of Firmicutes, Bacteroidetes, Verrucomicrobia, and Proteobacteria among the four treatment groups (Figures 3B−D, F). However, in the H. pylori-infected group, the abundance of Firmicutes (47.1%, P=0.45) increased, while the abundance of Bacteroidetes (40.2%, P=0.18) decreased compared to their abundances in control mice, but these differences were not significant (Figure 3B, C). Notably, the Firmicutes/Bacteroidetes ratio was lower in P. goldsteinii MTS01-fed H. pylori-infected mice than that in H. pylori-infected mice. Moreover, Tenericutes (0.02%, P=0.028) and Patescibacteria (0.02%, P=0.029) were significantly decreased in P. goldsteinii MTS01-fed H. pylori-infected mice compared to their levels in H. pylori-infected mice (Figure 3G, H).
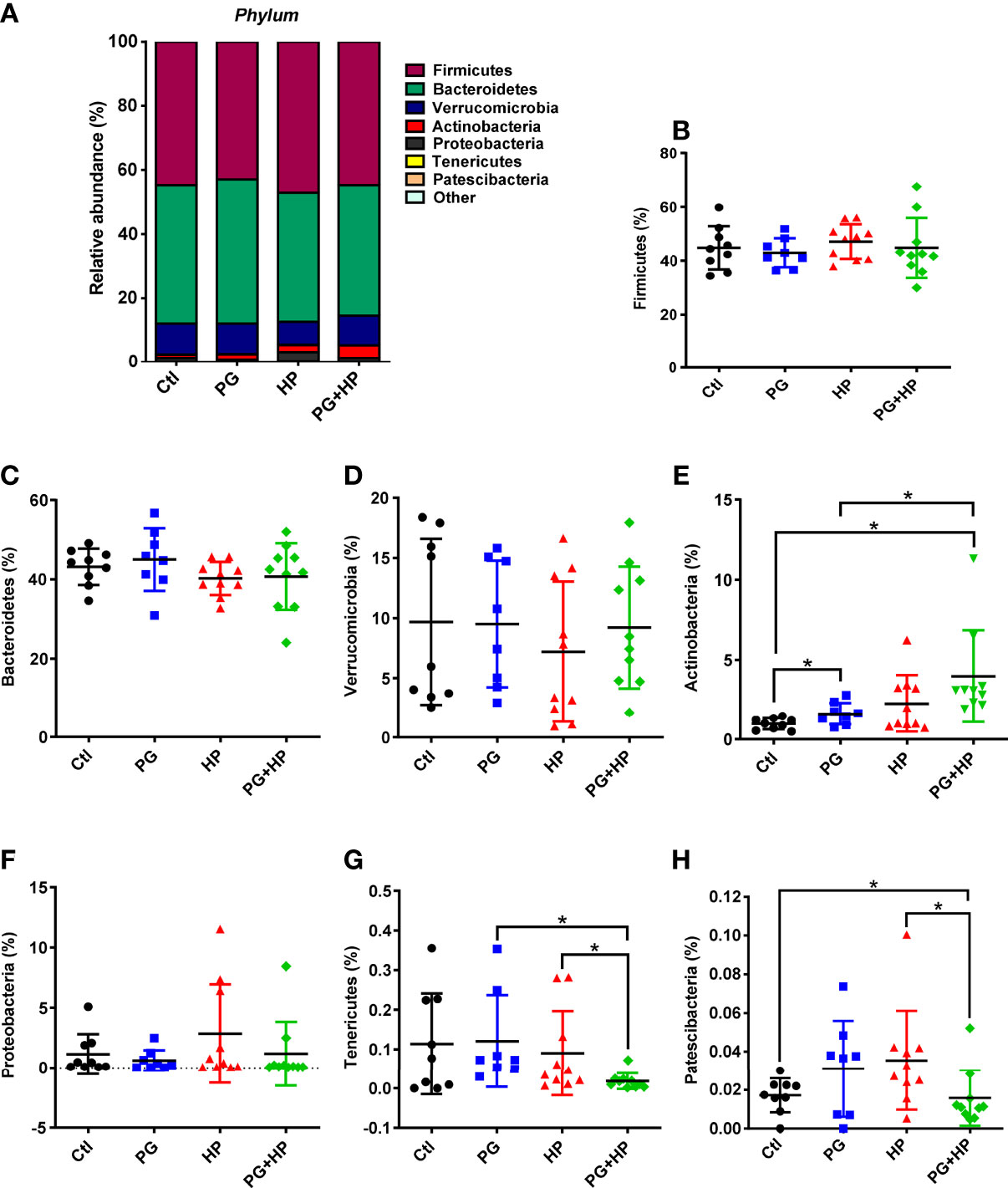
Figure 3 Relative abundance of the gut microbiota in phyla. The bacteria taxonomic profiles at phylum in the gut microbiota in P. goldsteinii MTS01-treated and H. pylori-infected mice. (A) Top seven most abundant phyla in the four murine groups were analyzed. Relative abundance of (B) Firmicutes, (C) Bacteroidetes, (D) Verrucomicrobia, (E) Actinobacteria, (F) Proteobacteria, (G) Tenericutes, and (H) Patescibacteria in the gut microbiota community were assessed. *P < 0.05.
The most abundant bacteria were then analyzed at the genus level, and the top 20 genera were identified (frequency > 0.01%; Figure 4 and Supplementary Figure 2). Compared to the control group, seven genera were significantly changed in the H. pylori infection group; six genera were increased (Bifidobacterium, Candidatus_Saccharimonas, Clostridium_sensu_stricto_1, Lachnospiraceae_UCG-010, Dehalobacterium, and Ruminiclostridium), and one genus (Butyricicoccus) was decreased (P < 0.05). Comparison of PG+HP and HP mice showed that eleven genera were different; five genera were increased (Bifidobacterium, Butyricicoccus, Anaerosporobacter, Candidatus_Arthromitus, and Turicibacter), and six genera were decreased (Lachnospiraceae_UCG-004, Candidatus_Saccharimonas, Clostridium_sensu_stricto_1, Lachnospiraceae_UCG-010, Dehalobacterium, and Anaeroplasma). These results indicate that the gut bacterial community was modulated in mice exposed to either P. goldsteinii MTS01 and/or H. pylori.
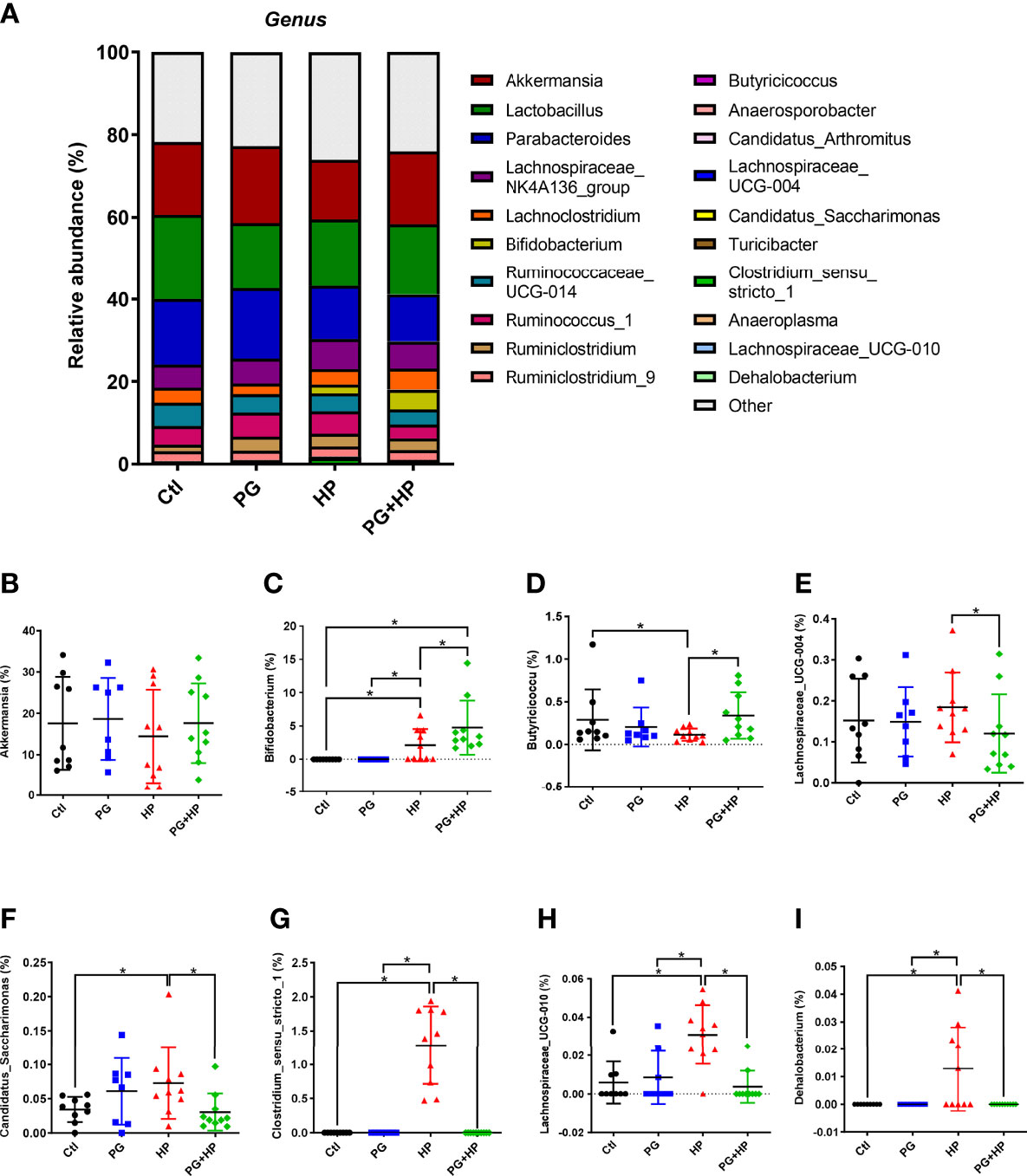
Figure 4 Relative abundance of the gut microbiota in genera. The bacteria taxonomic profiles at genus in the gut microbiota in mice administrated with P. goldsteinii MTS01 and H. pylori. (A) Top twenty most abundant genera among four treated groups were shown. Relative abundance of (B) Akkermansia, (C) Bifidobacterium, (D) Butyricicoccus, (E) Lachnospiraceae_UCG-004, (F) Candidatus_Saccharimonas, (G) Clostridium_sensu_stricto_1, (H) Lachnospiraceae_UCG-010, (I) Dehalobacterium in the gut microbiota were analyzed. *P < 0.05.
P. goldsteinii MTS01 Changes the Genera of Gut Microbiota
We next analyzed the relative abundance of bacteria at the genus level among the experimental groups using LEfSe analysis (Figure 5 and Suplementary Figure 3). Our results showed that 17 genera were altered between the control and HP groups and 10 genera were altered between the HP and PG+HP groups. The abundances of the top six enriched bacterial genera, Actinobacteria, Bifidobacterium, Ruminiclostridium, Oscillibacter, Proteus, and Intestinimonas, were significantly more abundant in H. pylori-infected mice than in control mice (Figure 5A, Supplementary Table 3). The relative abundances of Anaerosporobacter, Butyricicoccus, Candidatus_Arthromitus, and Turicibacter were significantly higher in the PG+HP group than in the HP group (Figure 5B). We generated a cladogram of the circular taxonomic and phylogenetic relationships based on the relative abundance data, which identified the bacterial genera that were differentially present in the HP and PG+HP groups (Figure 6 and Supplementary Figure 4). These results indicate that the gut microbiota of H. pylori-infected mice was influenced by treatment with P. goldsteinii MTS01.
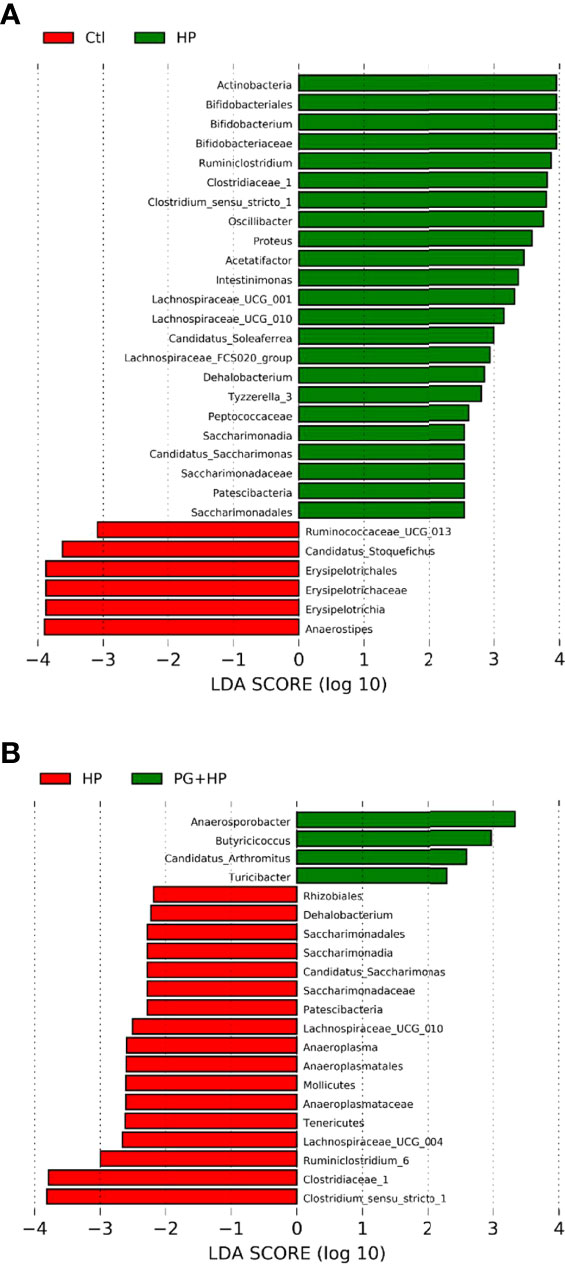
Figure 5 Differences in the gut microbiota composition in P. goldsteinii MTS01-treated and H pylori-infected mice. LEfSe analysis exhibited the abundance bacterial species compared with (A) control and H. pylori; (B) H. pylori and P. goldsteinii MTS01+H. pylori.
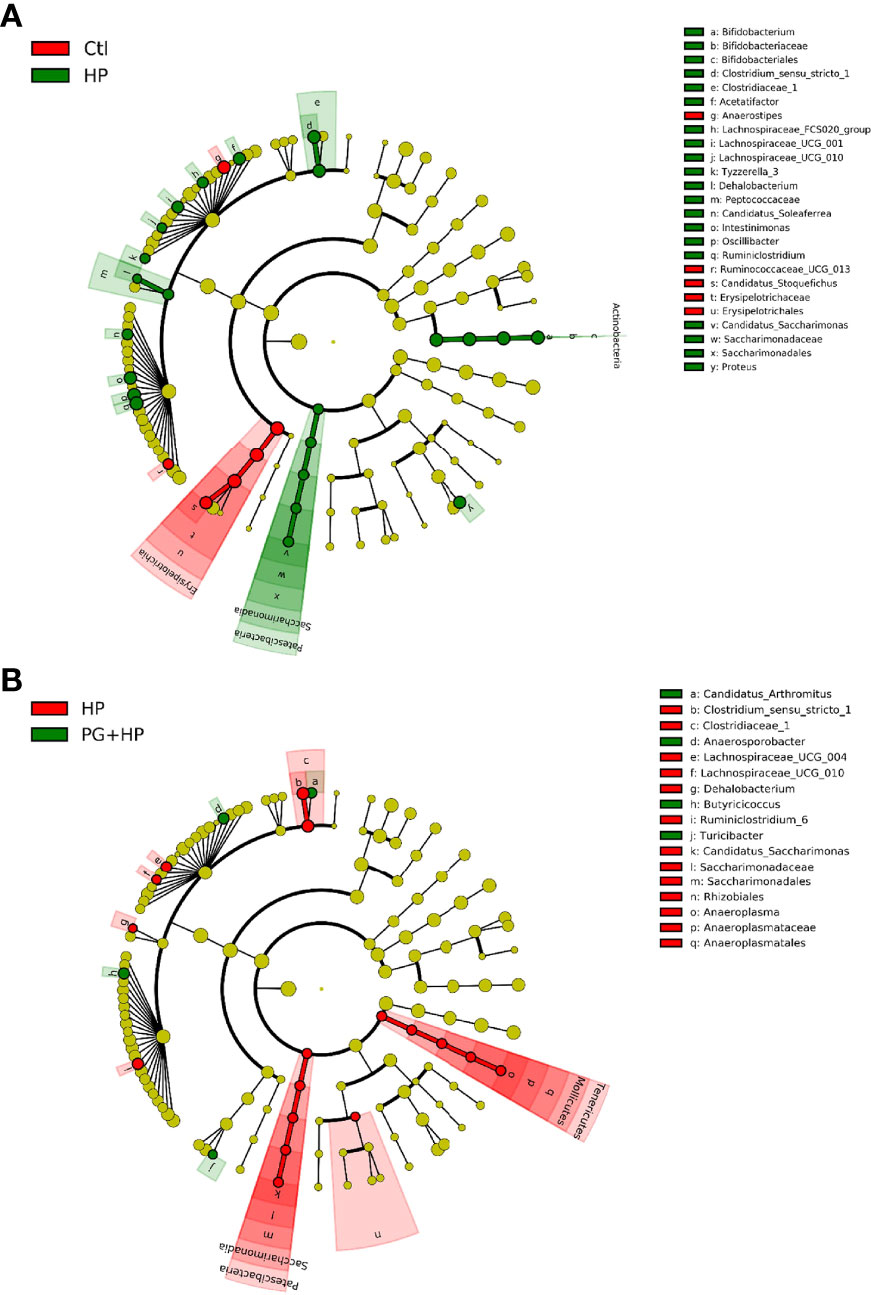
Figure 6 Circular taxonomic and phylogenetic tree of the gut microbiota diversity. Compared the effect of P. goldsteinii MTS01 and H. pylori altered microbiota composition in each group. Cladogram showed enriched taxa of gut microbiome in mice treated with (A) control and H. pylori; (B) H. pylori and P. goldsteinii MTS01+H. pylori.
Functional Analysis of the Bacterial Communities
We used PICRUSt2 to analyze the differences in microbiome function resulting from P. goldsteinii MTS01 treatment in H. pylori-infected mice (Figure 7 and Supplementary Figure 5). The major pathways correlated with H. pylori infection were thiamine metabolism, two-component system, and N-glycan biosynthesis (Figure 7). The predominant pathways that were altered following P. goldsteinii MTS01 treatment in H. pylori-infected mice were lipoic acid metabolism, lysine biosynthesis, NOD-like receptor signaling pathway, and inositol phosphate metabolism. These findings indicate that the differentially abundant bacteria in mice administered P. goldsteinii MTS01 were associated with lipid metabolism and the host immune response, which may contribute to the amelioration of H. pylori-induced pathogenesis.
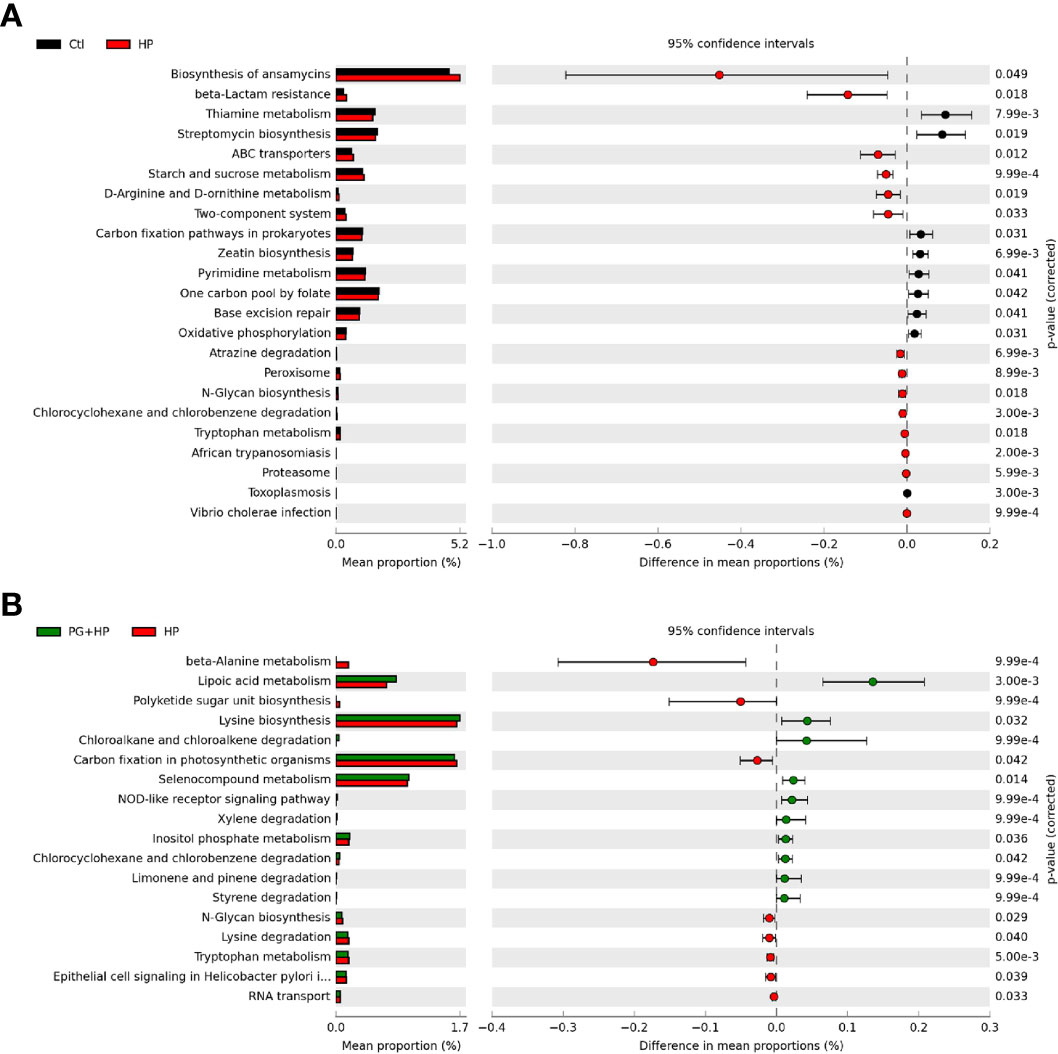
Figure 7 Putative functions of microbiota community. The microbial functionality profiles altered by P. goldsteinii MTS01 and H. pylori were analyzed using PICRUSt2 to generate KEGG pathway. Functional features showed the comparison between (A) control and H. pylori, and (B) H. pylori and P. goldsteinii MTS01+H. pylori.
P. goldsteinii MTS01 Reduces Serum Cholesterol Level and Mitigates Gastric Inflammation in H. pylori-Infected Mice
To further investigate whether P. goldsteinii MTS01 alleviates H. pylori-induced pathogenesis mediated by reducing cholesterol levels, we analyzed mouse serum triglyceride/cholesterol levels and prepared gastric tissues for histopathological analysis. Our results showed that serum levels of triglycerides and total cholesterol were significantly increased in mice infected with H. pylori compared to those in the control mice (Figures 8A, B). P. goldsteinii MTS01 treatment effectively inhibited these increases in serum triglyceride and cholesterol levels in H. pylori-infected mice, but did not affect LDL/VLDL and HDL levels when compared to those in H. pylori-infected mice. Mouse gastric tissues were subjected to histopathological analysis. IHC analysis showed faint expression of pro-inflammatory cytokines (COX-2, IL-1β, and TNF-α) in the gastric tissues of control mice, indicating an absence of inflammation (Figure 9). In contrast, the expression of these proinflammatory cytokines was much higher in H. pylori-infected tissues but was remarkably reduced in H. pylori-infected mice fed P. goldsteinii MTS01. However, the CLO test indicated that H. pylori infection in the stomach was unaffected by P. goldsteinii MTS01 treatment (data not shown). Together, these results demonstrate that P. goldsteinii MTS01 treatment decreased serum cholesterol, which is associated with the amelioration of stomach inflammation caused by H. pylori.
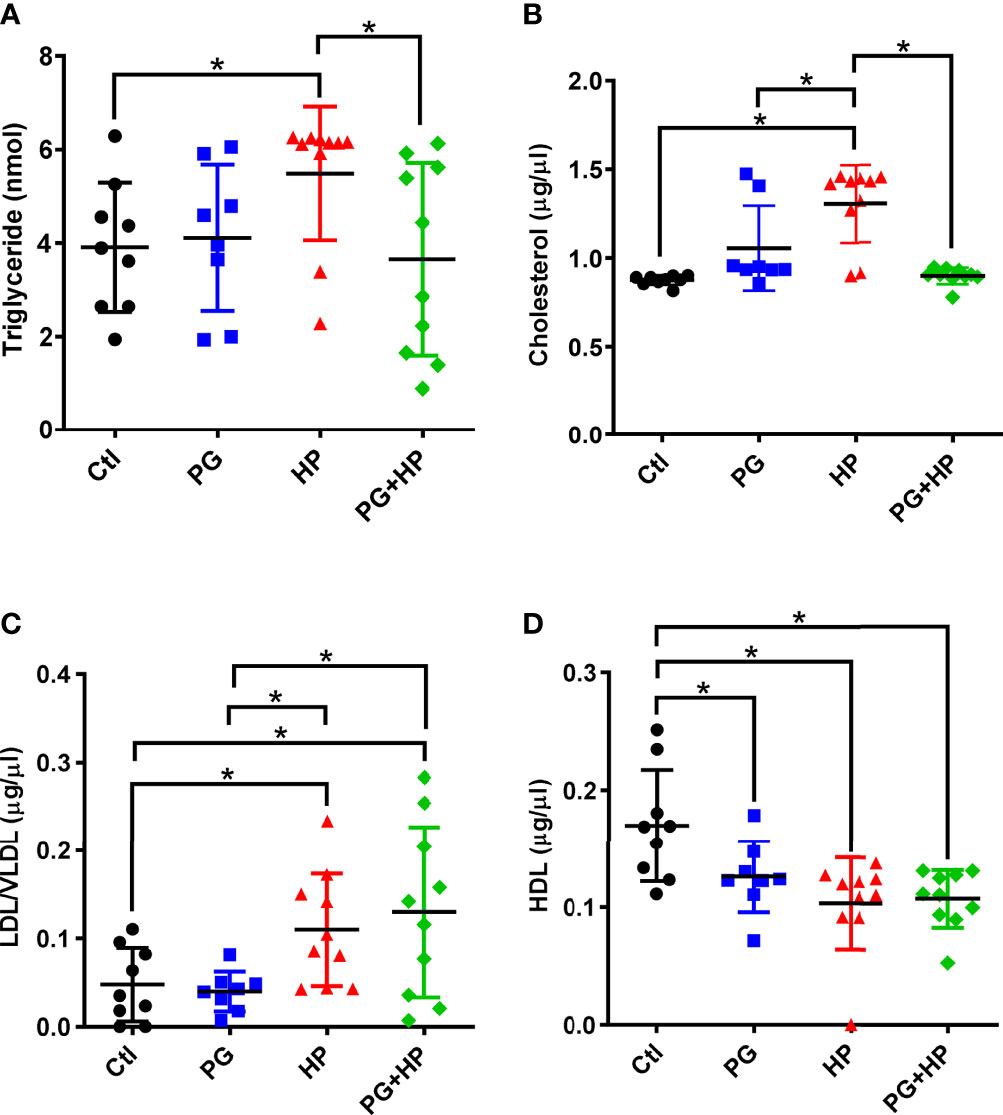
Figure 8 P. goldsteinii MTS01 lowers serum triglyceride/cholesterol. Sera from mice were collected and the levels of (A) triglyceride, (B) total cholesterol, (C) LDL/VLDL, and (D) HDL were analyzed. *P < 0.05.
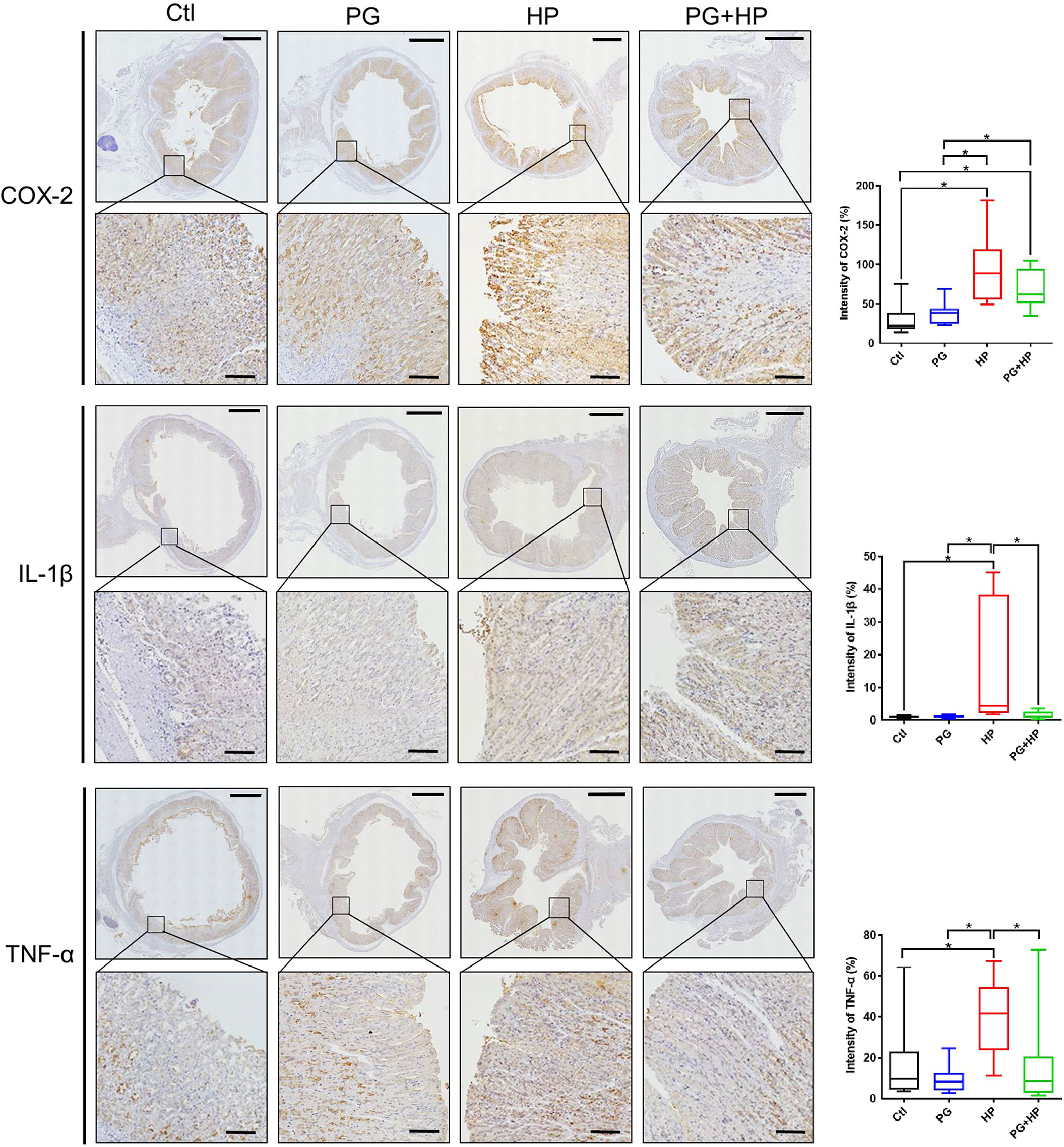
Figure 9 P. goldsteinii MTS01 mitigates H. pylori-induced gastric inflammation. Mouse gastric tissues were subjected to IHC staining with specific antibodies against COX-2, IL-1β, and TNF-α (original magnification: 200×). The magnified images are displayed below each cropped area. Scale in each panel, 1000 μm, and in each magnified image, 100 μm. The intensity of COX-2, IL-1β, and TNF-α expression for IHC staining in gastric tissues are shown in the right panels. "*" indicated P < 0.05.
P. goldsteinii MTS01 Alleviates H. pylori-Induced Pathogenesis
We investigated whether P. goldsteinii MTS01 lowers cholesterol levels and consequently attenuates VacA and CagA activity in cells. AGS cells were pretreated with P. goldsteinii MTS01 for 30 min and infected with H. pylori for 6 h. As shown in Figure 10A, H. pylori VacA-induced vacuole formation was effectively diminished in cells pretreated with P. goldsteinii MTS01. Similarly, the H. pylori CagA-induced scattering (hummingbird) phenotype was significantly decreased in P. goldsteinii MTS01-pretreated cells (Figure 10B). We then investigated whether P. goldsteinii MTS01 inhibited H. pylori CagA phosphorylation in cells. Our results showed that phosphorylation of CagA was increased in H. pylori-infected cells, but was significantly decreased in cells pretreated with either live or heat-inactivated P. goldsteinii MTS01 (Figure 10C). This trend was also observed for H. pylori-induced NF-κB activation (Figure 10D). Collectively, our results demonstrate that P. goldsteinii MTS01 inhibits the pathogenic effects of H. pylori VacA and CagA in gastric epithelial cells, which may be attributed to its microbiota-altering and cholesterol-lowering effects.
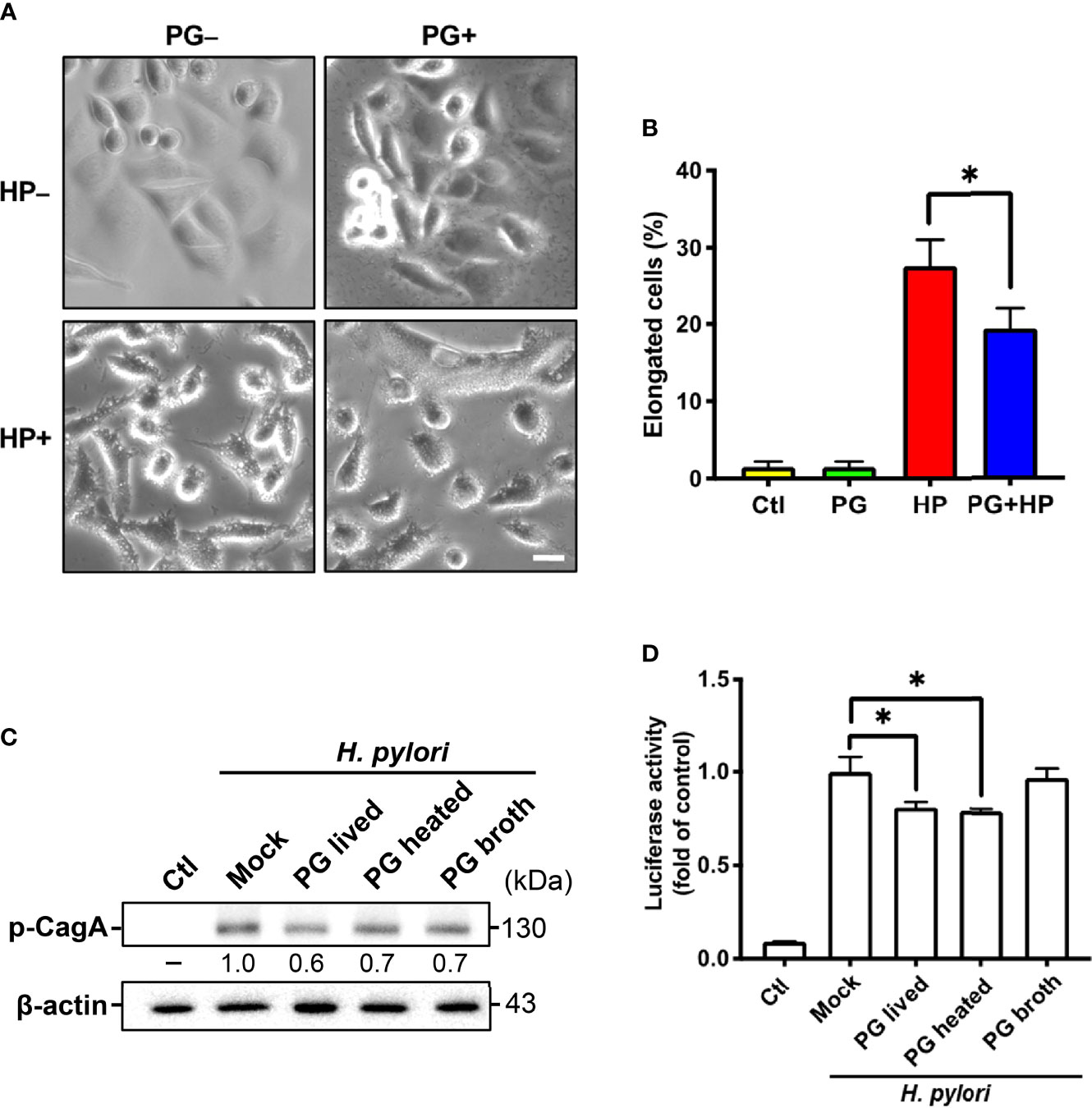
Figure 10 P. goldsteinii MTS01 ameliorates H. pylori-induced pathogenesis. (A) AGS cells were pretreated with P. goldsteinii MTS01 for 30 min and then infected with H. pylori at an MOI of 100 for 6 h. Cell vacuolation and elongation were observed by using a phase-contrast microscope. (B) The proportion of elongated cells were counted. (C) CagA phosphorylation were analyzed by western blot assay. Relative protein expression levels were normalized to β-actin and indicated under each lane. (D) The level of NF-κB activation was assessed using luciferase assay. *P < 0.05.
Discussion
Several bacteria use virulence factors that hijack cellular cholesterol to gain access to host cells (32, 33). Two virulence factors of H. pylori, VacA and CagA, exploit cholesterol for cellular internalization and consequent disease development (8, 9). Lowering cellular cholesterol has been shown to effectively abrogate the progression of diseases induced by pathogenic bacteria (34). For instance, cholesterol-lowering agents, such as statins, have been used to inhibit bacterial infectivity (35–37). In our recent studies, we found that statin therapy reduced serum cholesterol levels and decreased the incidence of H. pylori-related diseases, indicating that cholesterol plays a pivotal role in the function of H. pylori virulence factors (38–40). In the present study, we showed that P. goldsteinii MTS01 has potent serum triglyceride/cholesterol-lowering activity, which is associated with reduced H. pylori-induced gastric inflammation in mouse models. In addition, treatment of cells with P. goldsteinii MTS01 remarkably inhibited the effects of VacA and CagA, which in turn decreased NF-κB activation and proinflammatory cytokine production. In our previous study, we showed that administration of live P. goldsteinii MTS01 reversed obesity in HFD-fed mice, mitigated endotoxemia, and relieved inflammation (15). Given these beneficial effects, P. goldsteinii MTS01 could be developed into a beneficial probiotic to combat H. pylori virulence factors and alleviate related inflammation and disease.
Eradication therapy using a combination of antibiotics and PPI is the gold standard for the treatment of H. pylori infections (2). However, with the widespread use of antibiotics, antimicrobial resistance of H. pylori has increased significantly, increasing the incidence of treatment failure (41). In addition, antibiotics can alter the gut microbiota and cause dysbiosis, which adversely affects physiological functions and may exacerbate disease progression (42). However, co-treatment of patients with both antibiotics and probiotics has been shown to prevent the adverse effects associated with antibiotic use and increase the rates of H. pylori eradication (43–45). This effect can be explained by the fact that probiotic supplements improve the dysbiosis caused by antibiotic eradication therapy (46). Consistent with previous reports, the results of the current study indicate that P. goldsteinii MTS01 has the potential to be developed as a functional probiotic for ameliorating H. pylori-induced pathogenesis.
An increased ratio of Firmicutes/Bacteroidetes was associated with H. pylori-positive gastritis (47) and obesity (48), and a reversal of the increased Firmicutes/Bacteroidetes ratio has been shown to be associated with reductions in total cholesterol, obesity, and related inflammation (17, 49, 50). Our results showed an increase in Bifidobacterium and a decrease in Clostridium in H. pylori-infected mice administered P. goldsteinii MTS01. In addition, P. goldsteinii MTS01 treatment decreased the Firmicutes/Bacteroidetes ratio, demonstrating that P. goldsteinii MTS01 could help maintain gut normobiosis and restore the disruption in the intestinal microbiota caused by H. pylori infection.
H. pylori CagA co-localized with the tight junction scaffolding protein ZO-1 at sites of bacterial attachment, resulting in the disruption of epithelial barrier function (51). Destruction of tight junctions by CagA resulted in a wider cell gap and altered cell-cell contact, which in turn enhanced cell migration (52). Consequently, sequelae may occur, particularly peptic ulcer disease and gastric cancer. Disruption of gastrointestinal barrier integrity caused leakage of intestinal bacterial LPS and proinflammatory cytokines into the circulation, leading to exacerbation of endotoxemia and inflammation (53). The present results show that P. goldsteinii MTS01 has inhibitory effects against H. pylori-CagA functions, including CagA phosphorylation and cell morphology scattering. We recently reported that P. goldsteinii MTS01 treatment effectively alleviated LPS-induced monolayer disruption and restored ZO-1 expression, which are crucial for maintaining gut integrity and homeostasis (15). Collectively, these results indicate that P. goldsteinii MTS01 possesses both antibacterial virulence factor effects and gut barrier-maintaining functions.
LPS, which is produced by gram-negative bacteria, is recognized by TLR4 and activates MD-2 to trigger signaling in response to inflammation (54). LPS derived from H. pylori is involved in the activation of the TLR4/MD-2 signalling pathway, which induces bacterial-elicited inflammation in gastric epithelial cells (55, 56). Notably, we recently reported that P. goldsteinii MTS01-LPS antagonizes E. coli LPS-induced activation, and the effect was attributed to competition for TLR4 (16). This is similar to the findings with LPS isolated from Bacteroides and Rhodobacter spp., which possess potent antagonism against pathogenic LPS-induced inflammation (57–59). Since the present study demonstrated the inhibitory effects of P. goldsteinii MTS01 against H. pylori-associated pathogenesis, whether the mechanism underlying the alleviation of H. pylori-induced inflammation is mediated by P. goldsteinii MTS01-LPS deserves further investigation.
In conclusion, we employed murine models to investigate the potential inhibitory effects of P. goldsteinii MTS01 on H. pylori infection and its physiological effects in vivo and to elucidate the detailed mechanism. Our results showed that P. goldsteinii MTS01 lowered cholesterol levels, significantly decreased the effects of H. pylori virulence factors on cells, and diminished bacteria-induced inflammation. P. goldsteinii MTS01 also altered the microbiota composition; increasing the number of beneficial bacteria and maintaining gut microbiota normobiosis. Our results demonstrate that P. goldsteinii MTS01 could be developed as a novel NGP to target H. pylori infection and alleviate its pathogenesis.
Data Availability Statement
The data presented in the study are deposited in the NCBI repository, accession number PRJNA833917.
Ethics Statement
The animal study was reviewed and approved by the Institutional Animal Care Use Committee of Chang Gung University (IACUC Approval No.: CGU107-141).
Author Contributions
C-HL, C-HC, and H-CL designed this work. T-LL, M-ZH, H-YW, and Y-FC performed the experimental study. T-LL, M-ZH, H-YW, and Y-FC, S-WL, and C-YY performed data analysis and interpretation. C-HL, T-LL, C-YY, C-HC, and H-CL. wrote the manuscript. All authors reviewed and approved the manuscript.
Conflict of Interest
The authors declare that the research was conducted in the absence of any commercial or financial relationships that could be construed as a potential conflict of interest.
Publisher’s Note
All claims expressed in this article are solely those of the authors and do not necessarily represent those of their affiliated organizations, or those of the publisher, the editors and the reviewers. Any product that may be evaluated in this article, or claim that may be made by its manufacturer, is not guaranteed or endorsed by the publisher.
Acknowledgments
The authors would like to thank Dr. Chia-Chen Lu for her editorial assistance and valuable comments. The authors sincerely appreciate the assistance in analyzing 16S rRNA gene sequences and bioinformatic analysis from Next Generating Sequencing Core, Bioinformatics Core, and Molecular Medicine Research Center, Chang Gung University, Taoyuan, Taiwan.
Supplementary Material
The Supplementary Material for this article can be found online at: https://www.frontiersin.org/articles/10.3389/fimmu.2022.916848/full#supplementary-material
Supplementary Figure 1 | Body weight and temperature of mice during experimental studies.Mice were divided into four groups for the treatments with vehicle-control (PBS, n = 9), P. goldsteinii MTS01 (PG, n = 10), H. pylori (HP, n = 8), and P. goldsteinii MTS01 + H. pylori (n = 10). The body weight and temperature of the mice were recorded every week for a total of twelve weeks.
Supplementary Figure 2 | Relative abundance of the gut microbiota in genera. The bacteria taxonomic profiles at genus level in the gut microbiota of the experimental mice. Relative abundance of (A) Lactobacillus, (B) Parabacteroides, (C) Lachnospiraceae_NK4A136_group, (D) Lachnoclostridium, (E) Ruminococcus_UCG014, (F) Ruminococcus_1, (G) Ruminiclostridium, (H) Ruminiclostridium_9, (I) Anaerosporobacter, (J) Candidatus_Arthromitus, (K) Turicibacter, (L) Anaeroplasma in the gut microbiota were analyzed. *P < 0.05.
Supplementary Figure 3 | Differences in gut microbiota composition in mice administrated with P. goldsteinii MTS01 and H. pylori. LEfSe analysis exhibited the abundance bacterial species compared with (A) control and P. goldsteinii MTS01+H. pylori; (B) H. pylori and P. goldsteinii MTS01.
Supplementary Figure 4 | Circular taxonomic and phylogenetic tree of gut microbiota diversity. Compared the effect of P. goldsteinii MTS01 and H. pylori altered microbiota composition in each group. Cladogram showed enriched taxa of gut microbiome in mice treated with (A) control and P. goldsteinii MTS01+H. pylori; (B) H. pylori and P. goldsteinii MTS01.
Supplementary Figure 5 | Putative functions of microbiota community. The microbial functionality profiles altered by P. goldsteinii MTS01 and H. pylori were analyzed using PICRUSt2 to generate KEGG pathway. Functional features showed the comparison between (A) control and P. goldsteinii MTS01+H. pylori; (B) H. pylori and P. goldsteinii MTS01.
References
1. Crowe SE. Helicobacter Pylori Infection. N Engl J Med (2019) 380(12):1158–65. doi: 10.1056/NEJMcp1710945
2. Malfertheiner P, Megraud F, O'Morain CA, Gisbert JP, Kuipers EJ, Axon AT, et al. Management of Helicobacter Pylori Infection-The Maastricht V/Florence Consensus Report. Gut (2017) 66(1):6–30. doi: 10.1136/gutjnl-2016-312288
3. Megraud F, Bruyndonckx R, Coenen S, Wittkop L, Huang TD, Hoebeke M, et al. Helicobacter Pylori Resistance to Antibiotics in Europe in 2018 and Its Relationship to Antibiotic Consumption in the Community. Gut (2021) 70(10):1815–22. doi: 10.1136/gutjnl-2021-324032
4. Fallone CA, Moss SF, Malfertheiner P. Reconciliation of Recent Helicobacter Pylori Treatment Guidelines in a Time of Increasing Resistance to Antibiotics. Gastroenterology (2019) 157(1):44–53. doi: 10.1053/j.gastro.2019.04.011
5. Backert S, Neddermann M, Maubach G, Naumann M. Pathogenesis of Helicobacter Pylori Infection. Helicobacter (2016) 21(Suppl 1):19–25. doi: 10.1111/hel.12335
6. Foegeding NJ, Caston RR, McClain MS, Ohi MD, Cover TL. An Overview of Helicobacter Pylori Vaca Toxin Biology. Toxins (2016) 8(6). doi: 10.3390/toxins8060173
7. Tegtmeyer N, Neddermann M, Asche CI, Backert S. Subversion of Host Kinases: A Key Network in Cellular Signaling Hijacked by Helicobacter Pylori Caga. Mol Microbiol (2017) 105(3):358–72. doi: 10.1111/mmi.13707
8. Schraw W, Li Y, McClain MS, van der Goot FG, Cover TL. Association of Helicobacter Pylori Vacuolating Toxin (Vaca) With Lipid Rafts. J Biol Chem (2002) 277(37):34642–50. doi: 10.1074/jbc.M203466200
9. Lai CH, Chang YC, Du SY, Wang HJ, Kuo CH, Fang SH, et al. Cholesterol Depletion Reduces Helicobacter Pylori Caga Translocation and Caga-Induced Responses in Ags Cells. Infect Immun (2008) 76(7):3293–303. doi: 10.1128/IAI.00365-08
10. Sit WY, Chen YA, Chen YL, Lai CH, Wang WC. Cellular Evasion Strategies of Helicobacter Pylori in Regulating Its Intracellular Fate. Semin Cell Dev Biol (2020) 101:59–67. doi: 10.1016/j.semcdb.2020.01.007
11. Belkaid Y, Hand TW. Role of the Microbiota in Immunity and Inflammation. Cell (2014) 157(1):121–41. doi: 10.1016/j.cell.2014.03.011
12. Navarro-Rodriguez T, Silva FM, Barbuti RC, Mattar R, Moraes-Filho JP, de Oliveira MN, et al. Association of a Probiotic to a Helicobacter Pylori Eradication Regimen Does Not Increase Efficacy or Decreases the Adverse Effects of the Treatment: A Prospective, Randomized, Double-Blind, Placebo-Controlled Study. BMC Gastroenterol (2013) 13:56. doi: 10.1186/1471-230X-13-56
13. Lu C, Sang J, He H, Wan X, Lin Y, Li L, et al. Probiotic Supplementation Does Not Improve Eradication Rate of Helicobacter Pylori Infection Compared to Placebo Based on Standard Therapy: A Meta-Analysis. Sci Rep (2016) 6:23522. doi: 10.1038/srep23522
14. Chang CJ, Lin TL, Tsai YL, Wu TR, Lai WF, Lu CC, et al. Next Generation Probiotics in Disease Amelioration. J Food Drug Anal (2019) 27(3):615–22. doi: 10.1016/j.jfda.2018.12.011
15. Wu TR, Lin CS, Chang CJ, Lin TL, Martel J, Ko YF, et al. Gut Commensal Parabacteroides Goldsteinii Plays a Predominant Role in the Anti-Obesity Effects of Polysaccharides Isolated From Hirsutella Sinensis. Gut (2019) 68(2):248–62. doi: 10.1136/gutjnl-2017-315458
16. Lai HC, Lin TL, Chen TW, Kuo YL, Chang CJ, Wu TR, et al. Gut Microbiota Modulates Copd Pathogenesis: Role of Anti-Inflammatory Parabacteroides Goldsteinii Lipopolysaccharide. Gut (2021) 71(2):309–21. doi: 10.1136/gutjnl-2020-322599
17. Chang CJ, Lin CS, Lu CC, Martel J, Ko YF, Ojcius DM, et al. Ganoderma Lucidum Reduces Obesity in Mice by Modulating the Composition of the Gut Microbiota. Nat Commun (2015) 6:7489. doi: 10.1038/ncomms8489
18. Tsou AM, Goettel JA, Bao B, Biswas A, Kang YH, Redhu NS, et al. Utilizing a Reductionist Model to Study Host-Microbe Interactions in Intestinal Inflammation. Microbiome (2021) 9(1):215. doi: 10.1186/s40168-021-01161-3
19. Chen YH, Tsai WH, Wu HY, Chen CY, Yeh WL, Chen YH, et al. Probiotic Lactobacillus Spp. Act Against Helicobacter Pylori-Induced Inflammation. J Clin Med (2019) 8(1). doi: 10.3390/jcm8010090
20. Marshall BJ, Warren JR, Francis GJ, Langton SR, Goodwin CS, Blincow ED. Rapid Urease Test in the Management of Campylobacter Pyloridis-Associated Gastritis. Am J Gastroenterol (1987) 82(3):200–10.
21. Martin-Nunez GM, Cornejo-Pareja I, Clemente-Postigo M, Tinahones FJ. Gut Microbiota: The Missing Link Between Helicobacter Pylori Infection and Metabolic Disorders? Front Endocrinol (Lausanne) (2021) 12:639856. doi: 10.3389/fendo.2021.639856
22. Ozbey G, Sproston E, Hanafiah A. Helicobacter Pylori Infection and Gastric Microbiota. Euroasian J Hepatogastroenterol (2020) 10(1):36–41. doi: 10.5005/jp-journals-10018-1310
23. Yang CY, Yeh YM, Yu HY, Chin CY, Hsu CW, Liu H, et al. Oral Microbiota Community Dynamics Associated With Oral Squamous Cell Carcinoma Staging. Front Microbiol (2018) 9:862. doi: 10.3389/fmicb.2018.00862
24. Chen YW, Li SW, Lin CD, Huang MZ, Lin HJ, Chin CY, et al. Fine Particulate Matter Exposure Alters Pulmonary Microbiota Composition and Aggravates Pneumococcus-Induced Lung Pathogenesis. Front Cell Dev Biol (2020) 8:570484. doi: 10.3389/fcell.2020.570484
25. Kozich JJ, Westcott SL, Baxter NT, Highlander SK, Schloss PD. Development of a Dual-Index Sequencing Strategy and Curation Pipeline for Analyzing Amplicon Sequence Data on the Miseq Illumina Sequencing Platform. Appl Environ Microbiol (2013) 79(17):5112–20. doi: 10.1128/AEM.01043-13
26. McMurdie PJ, Holmes S. Phyloseq: An R Package for Reproducible Interactive Analysis and Graphics of Microbiome Census Data. PLoS One (2013) 8(4):e61217. doi: 10.1371/journal.pone.0061217
27. Lozupone C, Knight R. Unifrac: A New Phylogenetic Method for Comparing Microbial Communities. Appl Environ Microbiol (2005) 71(12):8228–35. doi: 10.1128/AEM.71.12.8228-8235.2005
28. Langille MG, Zaneveld J, Caporaso JG, McDonald D, Knights D, Reyes JA, et al. Predictive Functional Profiling of Microbial Communities Using 16s Rrna Marker Gene Sequences. Nat Biotechnol (2013) 31(9):814–21. doi: 10.1038/nbt.2676
29. Yeh JY, Lin HJ, Kuo CJ, Feng CL, Chou CH, Lin CD, et al. Campylobacter Jejuni Cytolethal Distending Toxin C Exploits Lipid Rafts to Mitigate Helicobacter Pylori-Induced Pathogenesis. Front Cell Dev Biol (2021) 8:617419. doi: 10.3389/fcell.2020.617419
30. Lin HJ, Jiang ZP, Lo HR, Feng CL, Chen CJ, Yang CY, et al. Coalescence of Rage in Lipid Rafts in Response to Cytolethal Distending Toxin-Induced Inflammation. Front Immunol (2019) 10:109. doi: 10.3389/fimmu.2019.00109
31. Segata N, Izard J, Waldron L, Gevers D, Miropolsky L, Garrett WS, et al. Metagenomic Biomarker Discovery and Explanation. Genome Biol (2011) 12(6):R60. doi: 10.1186/gb-2011-12-6-r60
32. Frisan T. Bacterial Genotoxins: The Long Journey to the Nucleus of Mammalian Cells. Biochim Biophys Acta (2016) 1858(3):567–75. doi: 10.1016/j.bbamem.2015.08.016
33. Chen Y, Huang K, Chen LK, Wu HY, Hsu CY, Tsai YS, et al. Membrane Cholesterol Is Crucial for Clostridium Difficile Surface Layer Protein Binding and Triggering Inflammasome Activation. Front Immunol (2020) 11:1675. doi: 10.3389/fimmu.2020.01675
34. Gagliardi MC, Iwabuchi K, Lai CH. Editorial: Role of Lipid Rafts in Anti-Microbial Immune Response. Front Immunol (2021) 12:654776. doi: 10.3389/fimmu.2021.654776
35. Motzkus-Feagans CA, Pakyz A, Polk R, Gambassi G, Lapane KL. Statin Use and the Risk of Clostridium Difficile in Academic Medical Centres. Gut (2012) 61(11):1538–42. doi: 10.1136/gutjnl-2011-301378
36. Skerry C, Pinn ML, Bruiners N, Pine R, Gennaro ML, Karakousis PC. Simvastatin Increases the in Vivo Activity of the First-Line Tuberculosis Regimen. J Antimicrob Chemother (2014) 69(9):2453–7. doi: 10.1093/jac/dku166
37. Chow OA, von Kockritz-Blickwede M, Bright AT, Hensler ME, Zinkernagel AS, Cogen AL, et al. Statins Enhance Formation of Phagocyte Extracellular Traps. Cell Host Microbe (2010) 8(5):445–54. doi: 10.1016/j.chom.2010.10.005
38. Lin CJ, Liao WC, Lin HJ, Hsu YM, Lin CL, Chen YA, et al. Statins Attenuate Helicobacter Pylori Caga Translocation and Reduce Incidence of Gastric Cancer: In Vitro and Population-Based Case-Control Studies. PLoS One (2016) 11(1):e0146432. doi: 10.1371/journal.pone.0146432
39. Lin CJ, Liao WC, Chen YA, Lin HJ, Feng CL, Lin CL, et al. Statin Therapy Is Associated With Reduced Risk of Peptic Ulcer Disease in the Taiwanese Population. Front Pharmacol (2017) 8:210. doi: 10.3389/fphar.2017.00210
40. Hsu CY, Yeh JY, Chen CY, Wu HY, Chiang MH, Wu CL, et al. Helicobacter Pylori Cholesterol-Alpha-Glucosyltransferase Manipulates Cholesterol for Bacterial Adherence to Gastric Epithelial Cells. Virulence (2021) 12(1):2341–51. doi: 10.1080/21505594.2021.1969171
41. Alba C, Blanco A, Alarcon T. Antibiotic Resistance in Helicobacter Pylori. Curr Opin Infect Dis (2017) 30(5):489–97. doi: 10.1097/QCO.0000000000000396
42. Vangay P, Ward T, Gerber JS, Knights D. Antibiotics, Pediatric Dysbiosis, and Disease. Cell Host Microbe (2015) 17(5):553–64. doi: 10.1016/j.chom.2015.04.006
43. Francavilla R, Polimeno L, Demichina A, Maurogiovanni G, Principi B, Scaccianoce G, et al. Lactobacillus Reuteri Strain Combination in Helicobacter Pylori Infection: A Randomized, Double-Blind, Placebo-Controlled Study. J Clin Gastroenterol (2014) 48(5):407–13. doi: 10.1097/MCG.0000000000000007
44. Yang YJ, Sheu BS. Probiotics-Containing Yogurts Suppress Helicobacter Pylori Load and Modify Immune Response and Intestinal Microbiota in the Helicobacter Pylori-Infected Children. Helicobacter (2012) 17(4):297–304. doi: 10.1111/j.1523-5378.2012.00941.x
45. Sheu BS, Cheng HC, Kao AW, Wang ST, Yang YJ, Yang HB, et al. Pretreatment With Lactobacillus- and Bifidobacterium-Containing Yogurt Can Improve the Efficacy of Quadruple Therapy in Eradicating Residual Helicobacter Pylori Infection After Failed Triple Therapy. Am J Clin Nutr (2006) 83(4):864–9. doi: 10.1093/ajcn/83.4.864
46. Yuan Z, Xiao S, Li S, Suo B, Wang Y, Meng L, et al. The Impact of Helicobacter Pylori Infection, Eradication Therapy, and Probiotics Intervention on Gastric Microbiota in Young Adults. Helicobacter (2021) 26(6)e12848. doi: 10.1111/hel.12848
47. Yang L, Zhang J, Xu J, Wei X, Yang J, Liu Y, et al. Helicobacter Pylori Infection Aggravates Dysbiosis of Gut Microbiome in Children With Gastritis. Front Cell Infect Microbiol (2019) 9:375. doi: 10.3389/fcimb.2019.00375
48. Turnbaugh PJ, Ley RE, Mahowald MA, Magrini V, Mardis ER, Gordon JI. An Obesity-Associated Gut Microbiome With Increased Capacity for Energy Harvest. Nature (2006) 444(7122):1027–31. doi: 10.1038/nature05414
49. Turnbaugh PJ, Hamady M, Yatsunenko T, Cantarel BL, Duncan A, Ley RE, et al. A Core Gut Microbiome in Obese and Lean Twins. Nature (2009) 457(7228):480–4. doi: 10.1038/nature07540
50. Lee NY, Shin MJ, Youn GS, Yoon SJ, Choi YR, Kim HS, et al. Lactobacillus Attenuates Progression of Nonalcoholic Fatty Liver Disease by Lowering Cholesterol and Steatosis. Clin Mol Hepatol (2021) 27(1):110–24. doi: 10.3350/cmh.2020.0125
51. Amieva MR, Vogelmann R, Covacci A, Tompkins LS, Nelson WJ, Falkow S. Disruption of the Epithelial Apical-Junctional Complex by Helicobacter Pylori Caga. Science (2003) 300(5624):1430–4. doi: 10.1126/science.1081919
52. Song X, Chen HX, Wang XY, Deng XY, Xi YX, He Q, et al. H. Pylori-Encoded Caga Disrupts Tight Junctions and Induces Invasiveness of Ags Gastric Carcinoma Cells Via Cdx2-Dependent Targeting of Claudin-2. Cell Immunol (2013) 286(1-2):22–30. doi: 10.1016/j.cellimm.2013.10.008
53. Cani PD, Possemiers S, Van de Wiele T, Guiot Y, Everard A, Rottier O, et al. Changes in Gut Microbiota Control Inflammation in Obese Mice Through a Mechanism Involving Glp-2-Driven Improvement of Gut Permeability. Gut (2009) 58(8):1091–103. doi: 10.1136/gut.2008.165886
54. Shimazu R, Akashi S, Ogata H, Nagai Y, Fukudome K, Miyake K, et al. Md-2, a Molecule That Confers Lipopolysaccharide Responsiveness on Toll-Like Receptor 4. J Exp Med (1999) 189(11):1777–82. doi: 10.1084/jem.189.11.1777
55. Yokota S, Okabayashi T, Rehli M, Fujii N, Amano K. Helicobacter Pylori Lipopolysaccharides Upregulate Toll-Like Receptor 4 Expression and Proliferation of Gastric Epithelial Cells Via the Mek1/2-Erk1/2 Mitogen-Activated Protein Kinase Pathway. Infect Immun (2010) 78(1):468–76. doi: 10.1128/IAI.00903-09
56. Lu DY, Chen HC, Yang MS, Hsu YM, Lin HJ, Tang CH, et al. Ceramide and Toll-Like Receptor 4 Are Mobilized Into Membrane Rafts in Response to Helicobacter Pylori Infection in Gastric Epithelial Cells. Infect Immun (2012) 80(5):1823–33. doi: 10.1128/IAI.05856-11
57. Lin TL, Shu CC, Chen YM, Lu JJ, Wu TS, Lai WF, et al. Like Cures Like: Pharmacological Activity of Anti-Inflammatory Lipopolysaccharides From Gut Microbiome. Front Pharmacol (2020) 11:554. doi: 10.3389/fphar.2020.00554
58. Tan H, Zhao J, Zhang H, Zhai Q, Chen W. Novel Strains of Bacteroides Fragilis and Bacteroides Ovatus Alleviate the Lps-Induced Inflammation in Mice. Appl Microbiol Biotechnol (2019) 103(5):2353–65. doi: 10.1007/s00253-019-09617-1
Keywords: Parabacteroides goldsteinii, gut microbiota, Helicobacter pylori, cholesterol, pathogenesis
Citation: Lai C-H, Lin T-L, Huang M-Z, Li S-W, Wu H-Y, Chiu Y-F, Yang C-Y, Chiu C-H and Lai H-C (2022) Gut Commensal Parabacteroides goldsteinii MTS01 Alters Gut Microbiota Composition and Reduces Cholesterol to Mitigate Helicobacter pylori-Induced Pathogenesis. Front. Immunol. 13:916848. doi: 10.3389/fimmu.2022.916848
Received: 10 April 2022; Accepted: 01 June 2022;
Published: 30 June 2022.
Edited by:
Juarez Antonio Simões Quaresma, Universidade do Estado do Pará, BrazilReviewed by:
Feng Zhang, Affiliated Hospital of Jiangnan University, ChinaJinfeng Miao, Nanjing Agricultural University, China
Copyright © 2022 Lai, Lin, Huang, Li, Wu, Chiu, Yang, Chiu and Lai. This is an open-access article distributed under the terms of the Creative Commons Attribution License (CC BY). The use, distribution or reproduction in other forums is permitted, provided the original author(s) and the copyright owner(s) are credited and that the original publication in this journal is cited, in accordance with accepted academic practice. No use, distribution or reproduction is permitted which does not comply with these terms.
*Correspondence: Chih-Ho Lai, chlai@mail.cgu.edu.tw; Hsin-Chih Lai, hclai@mail.cgu.edu.tw