- 1Department of Immunology, Moffitt Cancer Center, Tampa, FL, United States
- 2Department of Integrated Mathematical Oncology, Moffitt Cancer Center, Tampa, FL, United States
- 3Comparative Medicine, University of South Florida, Tampa, FL, United States
- 4Cell Therapy Facility, Moffitt Cancer Center, Tampa, FL, United States
- 5Department of Genitourinary Oncology, Moffitt Cancer Center, Tampa, FL, United States
Background: New therapeutics in development for bladder cancer need to address the recalcitrant nature of the disease. Intravesical adoptive cell therapy (ACT) with tumor infiltrating lymphocytes (TIL) can potentially induce durable responses in bladder cancer while maximizing T cells at the tumor site. T cells infused into the bladder directly encounter immunosuppressive populations, such as myeloid derived suppressor cells (MDSCs), that can attenuate T cell responses. Intravesical instillation of gemcitabine can be used as a lymphodepleting agent to precondition the bladder microenvironment for infused T cell products.
Methods: Urine samples from bladder cancer patients and healthy donors were analyzed by flow cytometry and cytometric bead array for immune profiling and cytokine quantification. MDSCs were isolated from the urine and cocultured with stimulated T cells to assess effects on proliferation. An orthotopic murine model of bladder cancer was established using the MB49-OVA cell line and immune profiling was performed. MDSCs from tumor-bearing mice were cocultured with OT-I splenocytes to assess T cell proliferation. Mice received intravesical instillation of gemcitabine and depletion of immune cells was measured via flow cytometry. Bladder tumor growth of mice treated with intravesical gemcitabine, OT-I transgenic T cells, or combination was monitored via ultrasound measurement.
Results: In comparison to healthy donors, urine specimen from bladder cancer patients show high levels of MDSCs and cytokines associated with myeloid chemotaxis, T cell chemotaxis, and inflammation. T cells isolated from healthy donors were less proliferative when cocultured with MDSCs from the urine. Orthotopic murine bladder tumors also presented with high levels of MDSCs along with enrichment of cytokines found in the patient urine samples. MDSCs isolated from spleens of tumor-bearing mice exerted suppressive effects on the proliferation of OT-I T cells. Intravesical instillation of gemcitabine reduced overall immune cells, MDSCs, and T cells in orthotopic bladder tumors. Combination treatment with gemcitabine and OT-I T cells resulted in sustained anti-tumor responses in comparison to monotherapy treatments.
Conclusion: MDSCs are enriched within the microenvironment of bladder tumors and are suppressive to T cells. Gemcitabine can be used to lymphodeplete bladder tumors and precondition the microenvironment for intravesical ACT.
Introduction
Bladder cancer is the sixth most common cancer in the United States (1–3). Patients diagnosed with non-muscle invasive bladder cancer (NMIBC) are treated with a combination of transurethral resection of bladder tumor (TURBT) and intravesical instillation of chemotherapy and/or Bacillus Calmette–Guérin (BCG), depending on risk stratification (4, 5). On average, 50% of NMIBC patients will experience a recurrence and approximately 15-20% of patients will progress to muscle invasive bladder cancer (MIBC), where the prognosis is significantly worse (6–8). The high recurrence rates in bladder cancer highlights the clinical gaps in bladder cancer management and calls for new therapeutics that can induce durable responses. One therapy that can potentially produce such responses is adoptive cell therapy (ACT) with tumor infiltrating lymphocytes (TIL).
ACT with TIL is a cancer immunotherapy that involves the expansion and infusion of a patient’s endogenous, tumor-specific T cells to treat cancer (9, 10). ACT with TIL has shown to produce durable anti-tumor responses in patients with metastatic melanoma. Due to the high mutational burden of both malignancies, ACT with TIL may induce similar responses in patients with bladder cancer (11, 12). Feasibility studies of growing TIL from bladder tumors support that TIL can be expanded from bladder cancer patient samples (13, 14). Additionally, previous work in murine models show that intravesical ACT with TIL is an effective method of treating smaller bladder tumors (15). However, preconditioning the tumor microenvironment prior to ACT has yet to be investigated.
The microenvironment of tumors is highly suppressive to T cells. The accumulation of immune populations, such as myeloid derived suppressor cells (MDSCs), regulatory T cells (T regs), and tumor associated macrophages (TAMs) can induce direct and indirect means of T cell suppression and is correlated with more aggressive disease (16–19). These immunosuppressive populations are more likely to attenuate anti-tumor responses of T cells transferred locally compared to T cells transferred systemically. We predict that the depletion of these accumulated populations in bladder tumors is likely to augment anti-tumor responses of TIL. One potential method to deplete these cells is using gemcitabine. Gemcitabine is an antimetabolite chemotherapy that is used routinely to treat bladder cancer and can have lymphodepleting properties at low doses (20, 21). In this work, we show that gemcitabine can be used as a preconditioning agent to deplete these immunosuppressive populations. When gemcitabine pretreatment is used in mice with larger tumors that are resistant to ACT with OT-I transgenic T cells, the anti-tumor responses of T cells instilled into the bladder are enhanced.
Materials and methods
Clinical specimens
All samples were collected under an IRB-approved study (MCC18142 and MCC20106). Patient demographics of samples were blinded until after analysis was complete.
Urine collection and analysis
Urine samples were centrifuged at 500 x g for 5 minutes and supernatants were frozen for < 2 years at -80C before cytokine analysis. Cell pellets were treated with Red Blood Cell (RBC) lysis buffer (Biolegend, 420301) for 5 minutes and neutralized with PBS. Urine samples were centrifuged again at 500 x g for 5 minutes and passed through a 100 µm filter to remove cell clumps. An additional 70 µm filter was used if cell clumping was still present. Cells were resuspended in PBS and prepared for flow staining.
Human tumor processing
Tumors were processed in a petri dish with human tumor digest buffer (human enzyme media), described previously (14). Tumors were mechanically digested using scalpels and the GentleMACS dissociator (Miltenyi). The digest was incubated with human enzyme media for one hour at 37° C. Cells were strained using a mesh cup and a 100 µm filter and subsequently treated with RBC lysis buffer.
PBMC processing
Blood from healthy donors and bladder cancer patients were prepped by pooling sample tubes and diluting 2X with PBS. SepMate™ Tubes (STEMCELL Technologies, 85460) were used to isolate PBMCs by pipetting Ficoll into the bottom of the tube and layering with blood. The tubes were centrifuged at 290 x g for 10 minutes. The PBMC layer was isolated and centrifuged at 500 x g for 5 minutes. Cells were treated with RBC lysis buffer, as described above.
Animals
Female C57BL/6 mice from Charles River were used for all in vivo experiments. Female OT-I transgenic mice (C57BL/6-Tg (TcraTcrb) 1100Mjb/J) from Jackson Laboratory were used for all experiments in which OT-I transgenic T cells or splenocytes were needed. All animals were housed at the Comparative Medicine Facility at the University of South Florida and humanely euthanized with CO2 inhalation followed by cervical dislocation when orthotopic tumors reached 250-350 mm3, subcutaneous tumors reached 1 cm in diameter, or when animals were in poor health.
Cell lines and cell culture
We received MB49 bladder tumor cell line as a kind gift from Dr. Jeffery Schlom (National Cancer Institute, Bethesda, MA) (22). An ovalbumin (OVA) expressing fluorescent MB49 cell line (MB49OVA) was generated as described (15). Cells were cultured in complete media (CM), as previously described (15). Cells were passaged at 80% confluency, tested routinely for mycoplasma, and passaged no more than 10 times for experiments.
Murine tumor models
For subcutaneous models, mice received subcutaneous injections of 5×105 MB49-OVA cells. For orthotopic models, mice were anesthetized with isoflurane and kept warm using a heating pad. Gentle pressure was placed on bladders to evacuate any residual urine. Mice were then catheterized with 24G x 0.75 in catheters (Terumo) and pre-treated orthotopically with poly-L-lysine (Sigma) in PBS (50 µL at 1 µg/mL) for 10 minutes. Gentle pressure was used to empty bladders. Bladders were washed with 50 µL PBS and mice received intravesical instillation of 1×105 MB49-OVA murine bladder cancer cells in PBS for 30 minutes. After the incubation period, catheters were removed, and mice were placed in recovery cages and monitored.
Ultrasound
Six to ten days after tumor cell instillation, bladder tumors were monitored via the Vevo 2100 ultrasound system (FUJIFILM VisualSonics Inc.). Tumor measurements were recorded 1-2 times per week and tumor volumes were analyzed and calculated via the Vevo LAB ultrasound software (FUJIFILM VisualSonics Inc.).
Murine tissue processing
After harvest, tumors were processed as previously described (15). Spleens were mechanically digested through a 100 µm filter using a syringe plunger and treated with RBC lysis buffer, described previously. Tumors used for initial immune profiling experiments were harvested at murine endpoint.
Flow cytometry staining
Human urine cells, tumor digest, and PBMCs were stained with Zombie NIR (Biolegend, 423106) (1:1000 dilution) for live/dead staining. Cells were incubated in the dark at room temperature for 30 minutes and later washed with flow buffer (1.0 L DPBS/Modified without Calcium & Magnesium (HyClone, Cat No. SH30028.03), 5% Fetal Bovine Serum (FBS),1mM EDTA, 0.1% Sodium Azide). Fc block (Miltenyi, 130-059-901) was administered to each sample (1:100) and incubated in the dark at 4 C for 10 minutes. Samples were stained with the following antibodies for 20 minutes at 4 C: HLA-DR (BD, 555811), CD3 (Invitrogen, 17-0036-42), CD19 (Biolegend, 302212), CD56 (BD, 555518), CD14 (BD, 550787), CD11b, CD33 (Biolegend, 366618), LOX1 (Biolegend, 358604), and CD15 (Biolegend, 323040). Cells were washed with flow buffer and fixed with 2% paraformaldehyde (PFA) for 15 minutes at 4° C. Murine tumor digest was stained similarly to the above using murine Fc block (Tonbo, 70-0161-M001) and murine antibodies. Samples were stained with two panels: the first panel staining for CD4 (Biolegend, 116012), CD8 (BD, 553035), Nk1.1 (BioLegend, 108714), OVA-specific T cell receptors (MBL international, TB-5001-1), and CD45 (BioLegend, 103140) and the second panel staining for Ly6C (BioLegend, 128014), MHCII (BioLegend, 107636), CD45 (BioLegend, 103140), F4/80 (BioLegend, 123141), CD11b (BioLegend, 101228), CD19 (BD, 557399), CD103 (BioLegend, 121426), Ly6G (Tonbo, 20-1276-U100), and CD11c (Biolegend, 117339). For comparison of immune populations across murine treatment groups antibodies against CD45, CD4, CD8, Gr-1, and CD11b were used. All samples were run on FACSCelesta (BD) flow cytometer and analyzed using FlowJo V10 software.
Cytokine analysis
All samples used for cytokine analysis were stored at -80C and analyzed < 2 years from sample collection. Human urine supernatants from bladder cancer patients and healthy donors were analyzed via the LEGENDplex HU Essential Immune Response Panel (Biolegend, 740929). The 13-plex was used to identify sample concentrations of IL-4, IL-2, CXCL10, IL-1b, TNF-a, CCL2, IL-17a, IL-6, IL-10, IFN-g, IL-12p70, and TGF-b. Murine urine and bladder fragment supernatants were analyzed via the LEGENDplex MU Cytokine Release Syndrome Panel (Biolegend, 741023). The 13-plex was used to identify sample concentrations of IFN-g, IL-10, CCL4, IFN-a, CXCL9, CXCL10, TNF-a, IL-6, VEGF, IL-4, CCL3, CCL2, and GM-CSF. All assays were performed according to manufacturer’s protocol and data analysis was performed using The LEGENDplex Data Analysis Software Suite (Biolegend).
MDSC suppressor assay
Human MDSCs were isolated using CD15 MicroBeads (Miltenyi, 130-046-601). T cells were isolated from healthy donor PBMCs using an EasySep T cell isolation kit (STEMCELL, 17951) according to the manufacturer’s protocol. T cells were labeled with 5 µM CellTrace Violet (CTV) (Invitrogen, C34557) and incubated for 20 min, at 37° C, in the dark. T cells were stimulated with OKT-3 (10 µg/ml) and plated at decreasing ratios of MDSC: T cells. Cells were collected 72 hours later and analyzed by flow cytometry for CTV labeled T cells. Murine MDSCs were obtained from spleens of mice bearing subcutaneous MB49-OVA tumors. MDSCs were isolated from splenocytes using the MDSC Isolation Kit (Miltenyi, 130-094-538) according to manufacturer’s protocol. Splenocytes were obtained from OT-I TCR transgenic mice and labeled with CTV, as described above. T cells were stimulated with OVA-SIINFEKL peptide (1 µg/mL) and cocultured with MDSCs in a decreasing ratio of MDSCs to splenocytes. Cocultures were incubated for 66-72 hours and analyzed via flow cytometry for CTV labeled cells.
Thymidine incorporation assay
Human T cells and MDSCs isolated previously were cocultured in decreasing ratios of MDSCs to T cells. Approximately 48 hours after plating, 1µCi of 3H thymidine in TIL CM was spiked into each well and left to incubate overnight. Cells were washed and harvested onto a filter mat and left to dry in an oven at 60° C. The dried filter mat was placed into a plastic sleeve, saturated with betaplate scint (PerkinElmer) and read using the MicroBeta Microplate Counter (PerkinElmer).
IHC map of MDSCs
A portion of the resected tumors were cut into 4μm sections and stained for H&E, Ly6G, and CD31. These serial slide sections were scanned with a Leica Aperio AT2 digital Pathology Slide Scanner (Leica Biosystems, Vista, CA) with a 20x/0.7NA objective lens. The Tissuealign tool from Visiopharm version 2022.02 (Visiopharm A/S, Denmark) was used to co-register the whole slide SVS image files for the 3 IHC biomarkers with the H&E image. The H&E image was used to segment tumor and non-tumor regions under the guidance of the study pathologist. Next, a cell detection algorithm was used for each biomarker based on the pattern of DAB and Hematoxylin stains to label each cell as biomarker positive or negative with the thresholds set to be consistent for all samples. Finally, the intensity and spatial coordinates for each cell and vessel were reported. These were used for graphical reconstruction of the IHC maps for MDSCs, using the MATLAB software.
Profiling immune populations and cytokines post gemcitabine treatment
To evaluate the effect of gemcitabine on immune cells in bladder tumors, mice with orthotopic MB49-OVA tumors (>50 mm3) were randomized and treated with intravesical instillation of gemcitabine (500 µg). Four days after instillation of therapy, bladders were harvested and processed as described above. Bladder digest was stained for overall immune cells, lymphocytes, and MDSCs. Samples were analyzed by flow cytometry and results were normalized to cell count and bladder mass. Using the same experimental design above, murine tumor-bearing bladders were cut into equal size fragments and plated in 1 mL of CM overnight. The supernatants were collected and analyzed for cytokines using the LEGENDplex kit previously described. In the untreated group, urine was also obtained by catheterizing mice and pulling out the liquid with a syringe. Urine samples were analyzed using the same LEGENDplex kit as the tumor supernatants.
Treatment models
Mice received intravesical instillation of 1×105 MB49-OVA cells for 30 minutes to establish orthotopic bladder tumors. Bladder tumor growth was measured via ultrasound and mice were separated into untreated, gemcitabine only (GEM), OT-I only (OT-I), and combination (GEM + OT-I) groups. Ten days after instillation of tumor cells, the GEM and GEM+OT-I groups received intravesical instillation of gemcitabine (500 µg in 50 µL PBS) for 30 minutes. Fourteen days after instillation of tumor cells, the OT-I and GEM+OT-I groups received intravesical instillation of 5×106 OT-I transgenic T cells for a 3-hour incubation period. OT-I T cells were obtained by running splenocytes through T cell isolation columns (R&D Systems). Tumor volumes were recorded two times per week until endpoint of study.
Statistical analysis
For comparisons between human specimens and in vitro experimental groups, a two-tailed Student’s t test was used. For in vivo experimental groups, a one-tailed Student’s t test was used. For experimental tumor growth curves, a Compare Groups of Growth Curves (CGGC) test was used. Outliers were identified using the ROUT method with a Q value of 0.1%. All statistical analyses were performed using GraphPad Prism and all P values ≤ 0.05 were determined to be statistically significant.
Results
MDSCs are enriched within the urine of bladder cancer patients and are suppressive to T cells
Previous reports have highlighted the importance of MDSCs in the pathogenesis and progression of bladder cancer (18, 23, 24). Therefore, we decided to focus on the impact of this specific population on T cells. We first wanted to assess whether there is an accumulation of MDSCs within the bladder microenvironment of cancer patients. Urine samples from healthy donors and bladder cancer patients were analyzed via flow cytometry. Demographics data regarding patient-derived samples are listed in Table 1.
Polymorphonuclear MDSCs (PMN-MDSCs) were identified as CD3-CD19-CD56-CD14-LOX1+CD15+ populations and monocytic MDSCs (M-MDSCs) were identified as CD3-CD19-CD56-CD14+CD33+HLA-DR- populations (25) (Figure 1A). The sum of these populations equals the total amount of MDSCs in the sample. In comparison to the healthy cohort, the urine of bladder cancer patients was enriched for MDSCs (Figure 1B), with PMN-MDSCs making up most of these cells (Figure 1C). Interestingly, the levels of MDSCs within the bladder tumors were not as high as in the urine, potentially indicating that MDSCs may be shed from the tumor and released into the urine (Figure 1D). To determine whether this enrichment was specific to the bladder microenvironment or systemic, we obtained blood samples from patients and isolated the peripheral blood mononuclear cells (PBMCs). In comparison to the urine, PBMCs from bladder cancer patients contained very few MDSCs (Figure 1E).
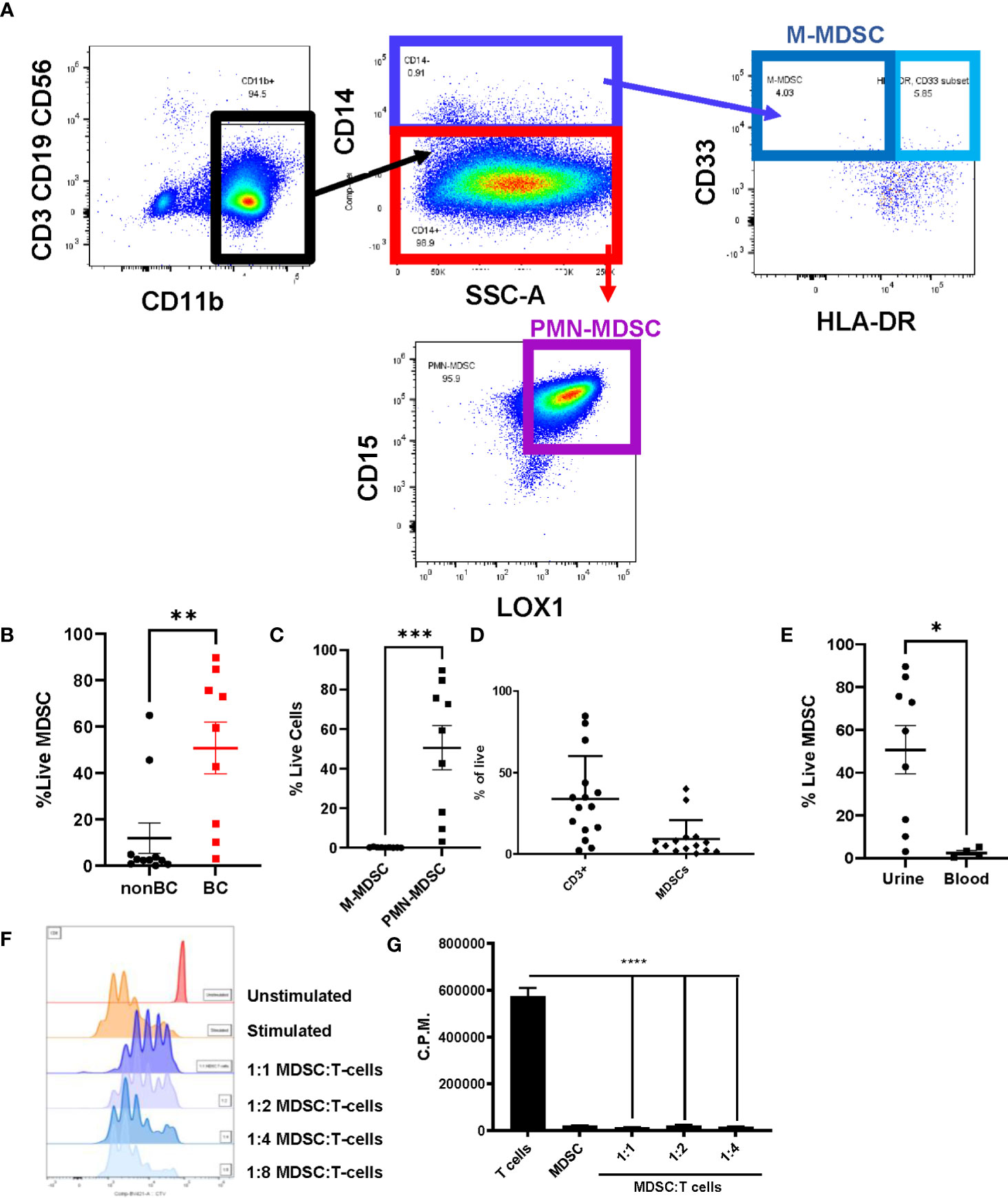
Figure 1 Urine of bladder cancer patients is enriched for MDSCs. (A) Gating strategy for MDSCs using urine cells from bladder cancer patient (B) Graph showing the percentage of live MDSCs in the urine of bladder cancer patients and healthy donors. n=9-11 per group. (C) Comparison of percentages of live PMN-MDSC and M-MDSC in the urine of bladder cancer patients, n=9. Composition of (D) immune cells in bladder tumors (n=15) and (E) MDSCs in urine and blood (n=4-9). (F) CTV flow cytometry analysis of 1:1, 1:2, 1:4, 1:8 coculture ratios of urinary MDSCs to T cells from healthy donor PBMCs. (G) Measurement of incorporation of tritiated thymidine in 1:1, 1:2, and 1:4 coculture ratios of MDSCs to T cells. All statistical analysis performed using two-tailed Student’s t tests. *, p ≤ 0.05; **, p ≤ 0.01; ***, p ≤ 0.001; ****, p ≤ 0.0001. With P values < 0.05.
To interrogate whether the accumulated MDSCs in bladder cancer patients are suppressive to T cells, coculture assays were performed using MDSCs isolated from the urine of patients and T cells isolated from healthy donor PBMCs. After stimulation of T cells with OKT-3, T cell proliferation was assessed through dilution of Cell Trace Violet (CTV). Coculture conditions with higher ratios of MDSCs resulted in less proliferation of T cells in comparison to cultures with fewer MDSCs (Figure 1F). Similar results were obtained by performing the same cocultures and measuring the incorporation of tritiated thymidine to assess T cell proliferation (Figure 1G). After determining that MDSCs accumulate in the bladder microenvironment and are suppressive to T cells, we next wanted to profile cytokine levels to better understand the microenvironment encountered by adoptively transferred T cells. Urinary cytokines have previously been used as a surrogate for immune activity in bladder cancer (26, 27). In comparison to healthy donors, CCL2, IL-6, CXCL10, IL-1b, IFN-g, TGF-b, and IL-8 were enriched within the urine of bladder cancer patients (Figure 2). CCL2 is associated with migration of monocytes and can serve as a chemoattractant for immunosuppressive cells (28, 29). IL-8 is associated with migration of neutrophils and has been associated with tumor progression in bladder cancer (30, 31). Enrichment of both cytokines may be supplementing further migration of MDSCs into the bladder microenvironment. IL-6 and IL-1b are proinflammatory cytokines that have been linked to bladder cancer progression (32, 33). CXCL10 promotes the migration of CXCR3 expressing cells, such as T cells, monocytes, NK cells, and tumor cells (34). The enrichment of CXCL10 is of particular interest due to its association with T cell infiltration into solid tumors (35–37). We have previously shown that CXCR3 signaling drives T cell infiltration into murine bladder tumors after intravesical ACT (15). We predict that these high CXCL10 levels are beneficial to intravesical ACT with TIL and warrants further study. Altogether, this data suggests that the urine of bladder cancer patients is enriched for MDSCs that are suppressive to T cells and that the microenvironment contains elevated levels of cytokines associated with inflammation, myeloid chemotaxis, and T cell chemotaxis.
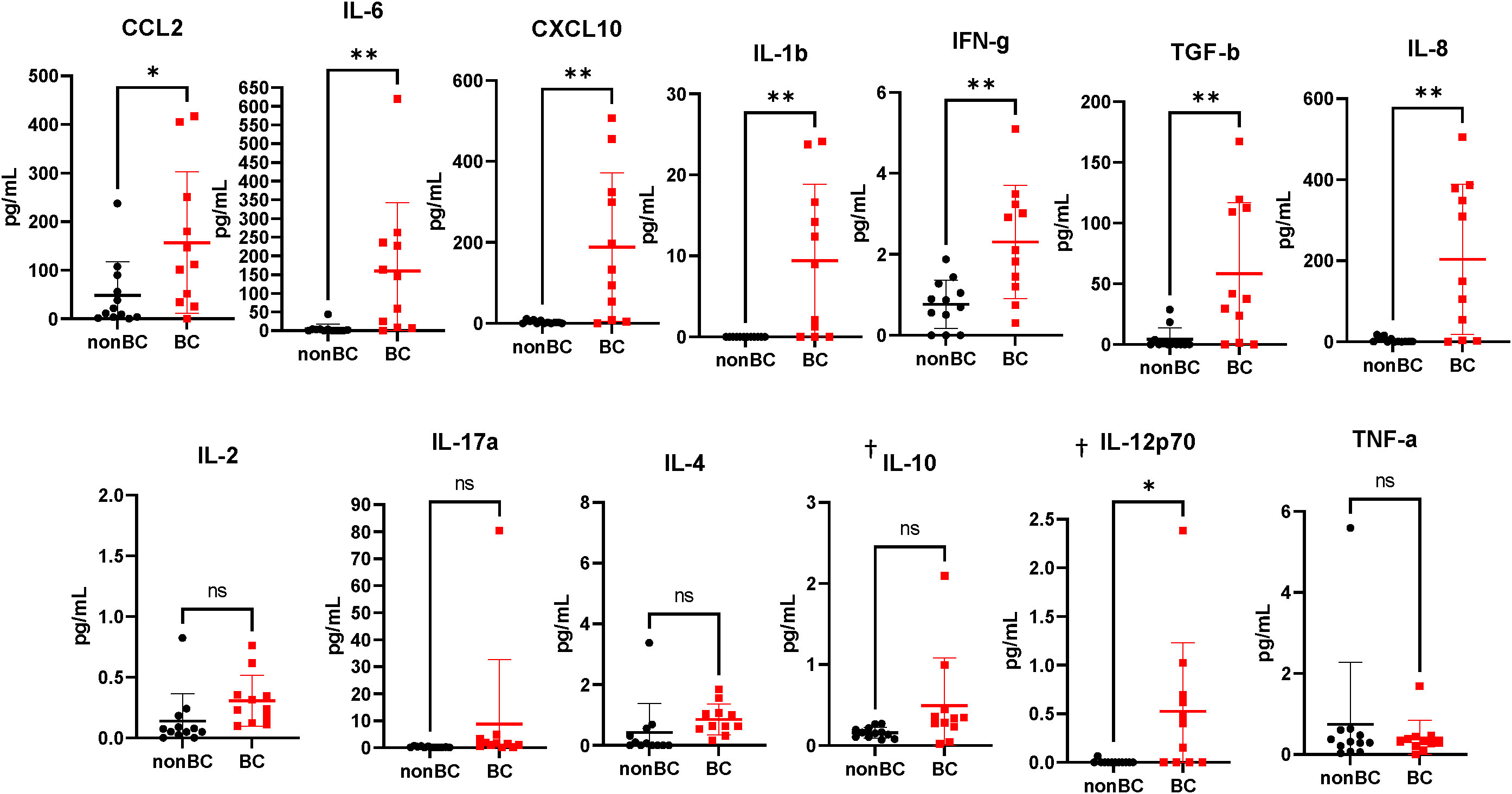
Figure 2 Urine supernatants from bladder cancer patients are highly enriched for cytokines. Cytometric bead array analysis of urine supernatants from bladder cancer patients and healthy donors. n=11-12 per group. Graphs marked with † are outside the limits of detection of the assay. All statistical analysis performed using two-tailed Student’s t tests. ns, non-significant; p > 0.05; *, p ≤ 0.05; **, p ≤ 0.01. With P values < 0.05.
MDSCs are enriched within MB49-OVA tumors and are suppressive to T cells
In our previous manuscript, we have shown that ACT with OT-I transgenic T cells is sufficient to control small MB49-OVA bladder tumors (15). In this work, we have adjusted our treatment schedules to use a tumor model that represents a larger tumor burden and fails to respond to ACT with OT-I T cells. We first wanted to validate that our murine bladder cancer model recapitulates the key characteristics of the human tumors highlighted in Figure 1. We profiled the immune microenvironment of orthotopic bladder tumors to assess relative MDSC enrichment. Briefly, orthotopic tumors were established through intravesical instillation of MB49-OVA murine bladder cancer cells. Bladder tumor growth was monitored via ultrasound measurement and bladders were harvested and processed and analyzed via flow cytometry to profile the immune infiltrate (Figure 3A). MDSCs were found to make up most of the immune cells in the tumor microenvironment and were about four times as prevalent as T cells. PMN-MDSCs made up the majority of the MDSCs; however, M-MDSCs seem to be more prevalent in the murine model in comparison to the human samples.
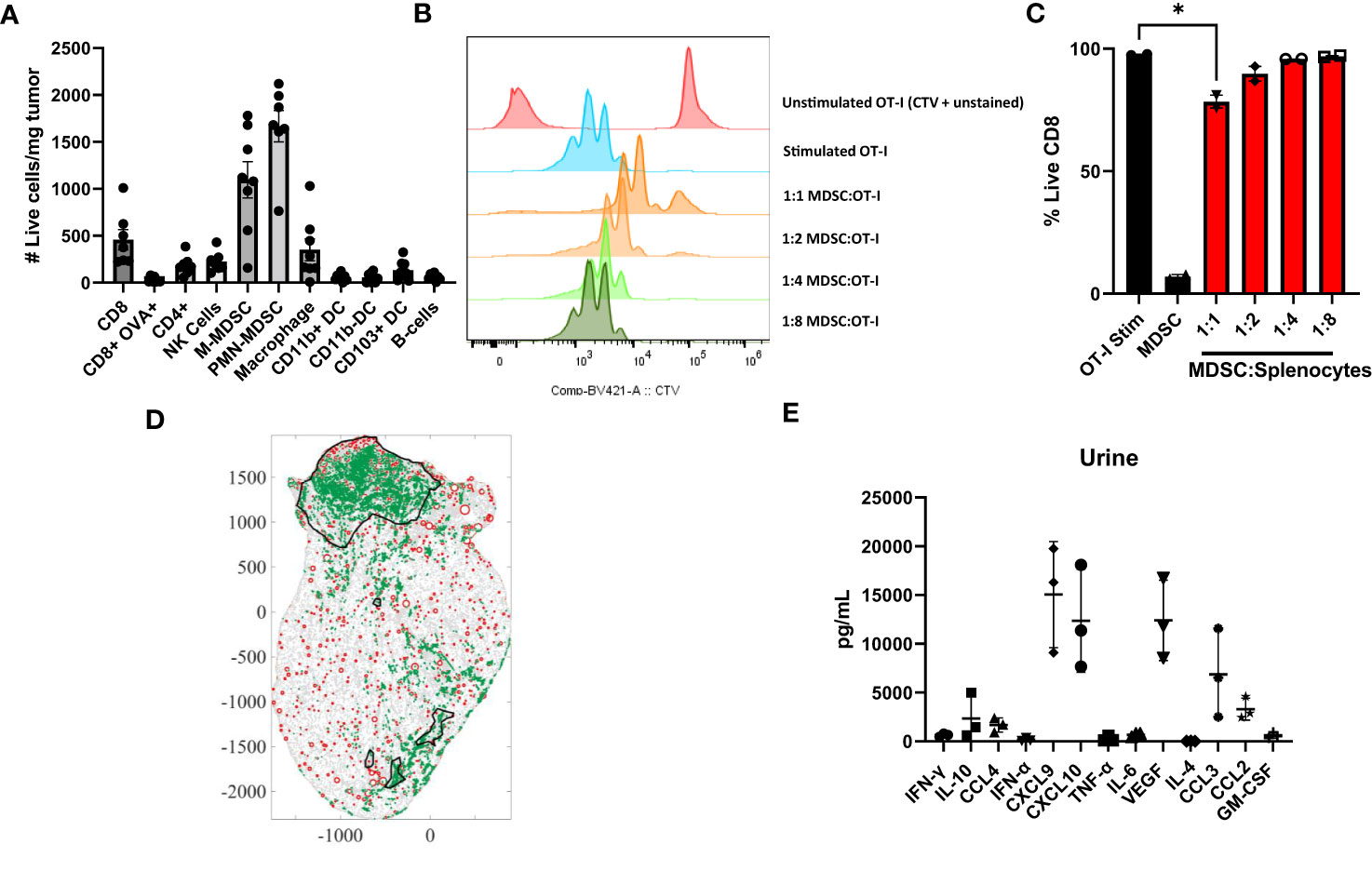
Figure 3 Murine bladder tumors are enriched for MDSCs that are suppressive to T cells. (A) Profile of immune populations in orthotopic MB49-OVA tumors normalized to number of live cells per milligram of tumor, n=7-8. (B) CTV flow cytometry analysis of 1:1, 1:2, 1:4, 1:8 coculture ratios of splenic MDSCs to OT-I T cells. (C) Graph showing expansion of CD8+ T cells in same coculture conditions. (D) Scanned tumor-bearing bladder tissue slides from mouse treated with intravesical instillation of OT-I T cells. Tumors are outlined in black, MDSCs are represented as green dots (identified via Ly6G staining), and blood vessels are represented as red circles (identified via CD31 staining). (E) Cytometric bead array analysis of urine supernatants from mice bearing orthotopic MB49-OVA tumors, n=3. All statistical analysis performed using two-tailed Student’s t tests. *, p ≤ 0.05. With P values < 0.05.
To determine whether murine MDSCs are suppressive to T cells, MDSCs isolated from spleens of mice bearing subcutaneous tumors were cocultured with OT-I splenocytes. T cells were stimulated with cognate ovalbumin antigen and proliferation was assessed through dilution of CTV. Matching the human data, murine T cells cocultured with higher ratios of MDSCs were less proliferative (Figure 3B). There was also an overall reduction in CD8+ T cells in coculture conditions where numbers of MDSCs matched the number of splenocytes (Figure 3C). Although we see a significant reduction in T cell proliferation at the 1:1 coculture condition. The most accurate representation within the bladder microenvrionment would be a 4:1 ratio of MDSCs to T cells. To show accumulation of MDSCs post-intravesical ACT, bladders of treated mice were fixed, stained, and digitized to visualize MDSC localization. A representative image is shown (Figure 3D). Mice that have received intravesical administration of OT-I T cells show a significant accumulation of MDSCs within their tumor (2986.2/mm2). The accumulation of these cells may contribute to immunosuppression within the tumor microenvironment, thus providing further rationale for incorporating a preconditioning regimen.
We next wanted to examine whether the cytokines in murine bladder tumors were similarly enriched to the cytokines in urine samples from the bladder cancer patients. Bladder fragments from mice bearing orthotopic tumors were plated and cultured overnight in media. Supernatants were collected the following day for analysis. Urine from bladder tumor bearing mice was also collected. Cytokine levels were assessed by comparing relative abundance across the same sample types. Within the urine samples, the relative abundance of CXCL9, CXCL10, VEGF, CCL3, and CCL2 was higher in comparison to the other cytokines tested (Figure 3E). Similarly, in the supernatant, the relative abundance of CXCL10, IL-6, and CCL2 was higher in comparison to the other cytokines tested (not shown). From the human data, the urine of bladder cancer patients had significantly upregulated levels of CCL2, IL-6, CXCL10, IL-1b, IFN-g, TGF-b, and IL-8 in comparison to the healthy donors. Therefore, the overexpression of CCL2, CXCL10, and IL-6 is shared in both murine and human samples. Mice do not have the IL-8 gene, and therefore we cannot make comparisons with the human data (38). Taken together, bladder tumors in our murine model have similar characteristics to the human samples through enrichment of MDSCs that are suppressive to T cells and an accumulation of cytokines that are associated with inflammation, myeloid chemotaxis, and T cell chemotaxis.
Gemcitabine depletes immune cells in orthotopic bladder tumors
Gemcitabine is an antimetabolite chemotherapy that is already used in the clinic for the treatment of bladder cancer (39, 40). In addition to being tumoricidal, gemcitabine has been shown to have lymphodepleting properties at low concentrations and can deplete MDSCs (41). To assess the effects of gemcitabine on the immune microenvironment of murine bladder tumors, mice bearing orthotopic MB49-OVA tumors were treated with intravesical instillation of gemcitabine (500 µg/mouse). Four days later, bladder tumors were harvested and stained for overall immune cells (CD45+) (Figure 4A), MDSCs (Figure 4B), CD8+ T cells (Figure 4C) and CD4+ T cells (Figure 4D). In general, mice treated with intravesical instillation of gemcitabine had a significant reduction in overall immune cells, MDSCs, and T cells. Although gemcitabine is used to ablate MDSCs, the overall result is lymphodepletion as cell populations cannot be selectively depleted by the chemotherapy. However, the depletion of these bystander populations to make room for the infusion product may be an added benefit for T cell anti-tumor responses.
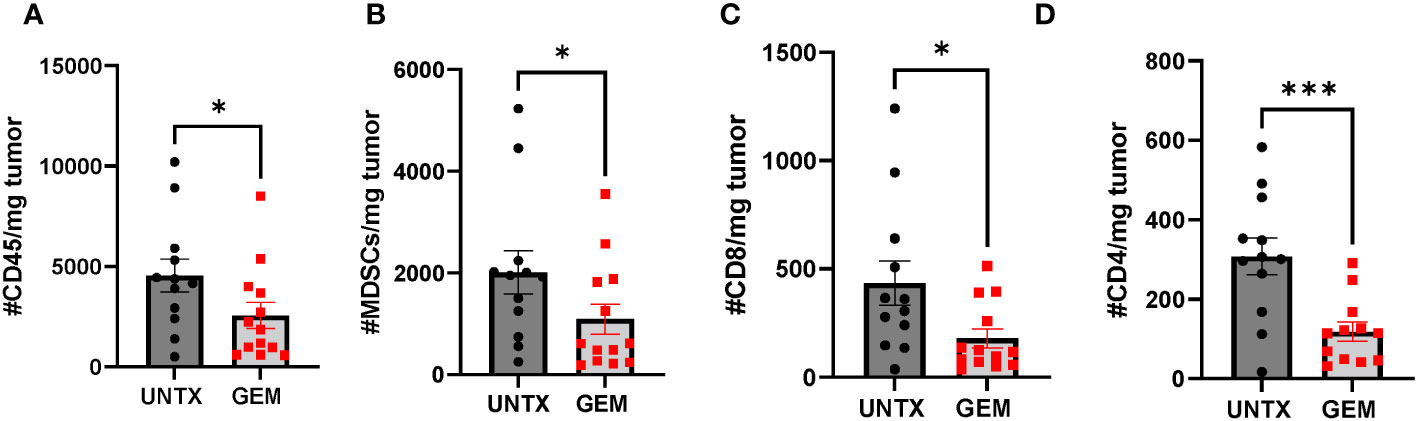
Figure 4 Intravesical instillation of gemcitabine reduces total number of immune cells. Graphs comparing total number of (A) CD45+ (B) MDSCs (C) CD8+ (D) CD4+ cells normalized to tumor mass in untreated mice and mice treated with intravesical instillation of 500 µg gemcitabine. n=12-13 per group. All statistical analysis performed using one-tailed Student’s t tests. *, p ≤ 0.05; ***, p ≤ 0.001. With P values < 0.05.
We observed that intravesical instillation of gemcitabine results in an overall lymphodepleting effect in the bladder microenvironment. We next wanted to determine whether this loss in immune cells results in an alteration in the cytokine levels in the bladder. Bladder tumor fragments of untreated mice and mice treated with intravesical gemcitabine were plated in media and the supernatants were collected 24 hours after plating for cytokine analysis. The only change in cytokine levels was a reduction in VEGF in the gemcitabine group (Figure 5). VEGF is important for tumor angiogenesis and promotes the expansion and migration of MDSCs (41, 42). There was a trend in an increase in GM-CSF in the gemcitabine group; however, this was not significant. While we see a reduction in immune cells in the bladder microenvironment, we do not see a reduction in the levels of cytokines important for T cell migration, namely CXCL9 and CXCL10. This may suggest that after treatment with gemcitabine, the availability of these cytokines do not change. Altogether, intravesical instillation of gemcitabine has a lymphodepleting effect on the bladder microenvironment with little impact to the availability of cytokines to T cells.
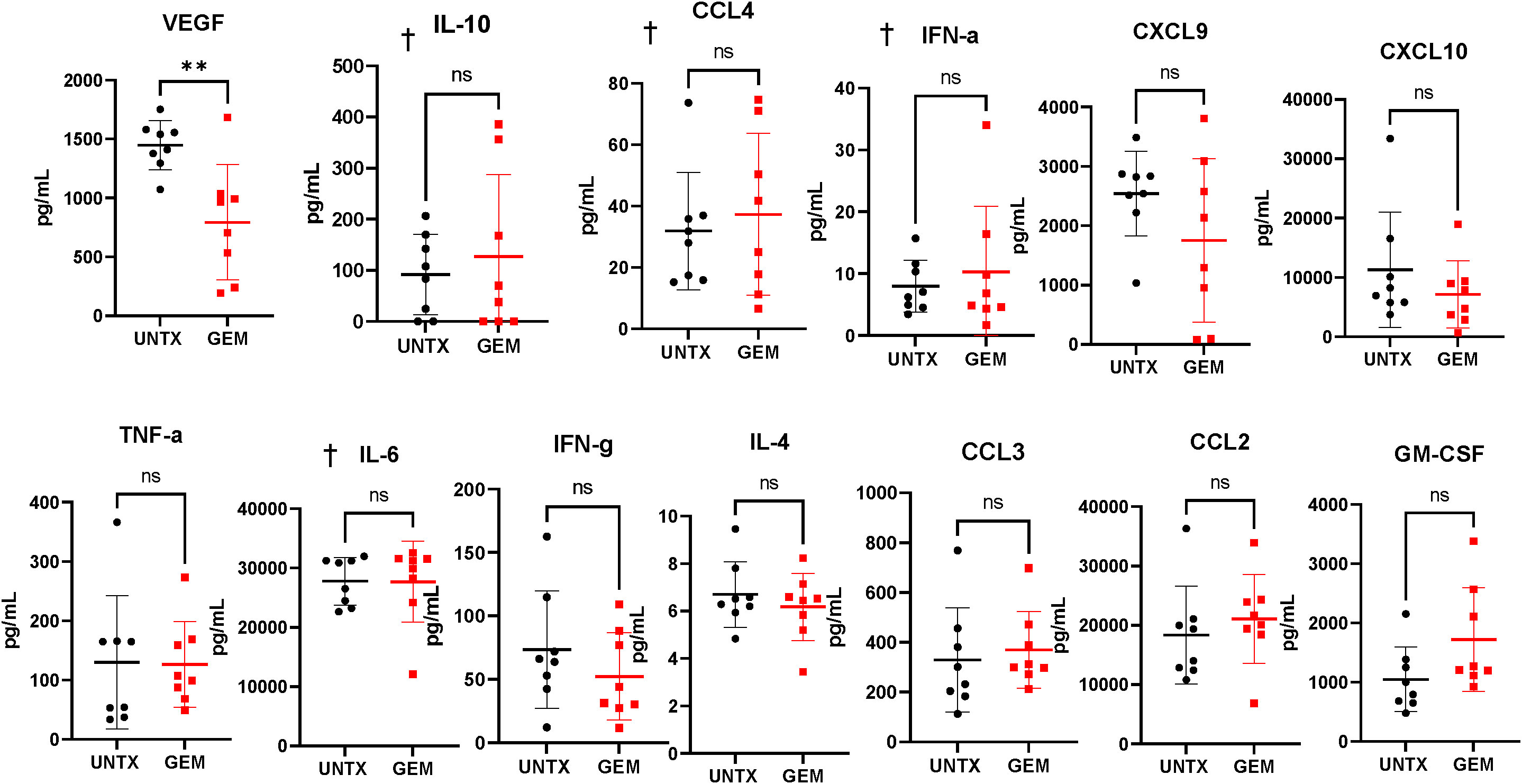
Figure 5 Gemcitabine treatment induces reduction of VEGF in tumor supernatants. Cytokine bead array analysis of supernatants collected from overnight cultures of tumor-bearing bladder fragments. n=8 per group. Graphs marked with † are outside the limits of detection of the assay. All statistical analysis performed using two-tailed Student’s t tests. ns, non-significant; p > 0.05; **, p ≤ 0.01. With P values < 0.05.
Pretreatment of bladder tumors with gemcitabine enhances anti-tumor effects of ACT with tumor-specific T cells
We have shown that intravesical instillation of gemcitabine has local lymphodepleting effects on the bladder tumor microenvironment. We predict that this pretreatment step will enhance the anti-tumor responses of adoptively transferred OT-I T cells into mice bearing MB49-OVA bladder tumors that are refractory to ACT. To test this, we established orthotopic MB49-OVA bladder tumors in mice and created four treatment groups: untreated (UNTX), gemcitabine treatment only (GEM), OT-I treatment only (OT-I), and combination gemcitabine and OT-I treatment (GEM+OT-I). Ten days after instillation of bladder tumor cells, mice in the GEM and GEM+OT-I groups received intravesical instillation of gemcitabine (500 µg). Four days after instillation of gemcitabine, mice in the OT-I and GEM+OT-I groups received intravesical instillation of OT-I T cells for a three-hour incubation period (5×106 T cells). This represents a timepoint where ACT with OT-I T cells provides minimal anti-tumor responses due to the large size of the tumors. All mice underwent ultrasound two times per week and tumor growth was monitored until 20 days post tumor cell instillation (Figure 6A). Overall, monotherapies with either gemcitabine or OT-I T cells resulted in a small reduction in bladder tumor growth in comparison to untreated mice (Figure 6B). However, the combination of GEM and OT-I resulted in a significant reduction in tumor growth. By day 20, the monotherapy groups and the untreated group had similar tumor volumes while the combination group had a reduction in tumor volume (Figures 6C, D). Taken together, pretreating larger bladder tumors with gemcitabine before intravesical instillation of OT-I T cells results in enhanced anti-tumor responses in comparison to monotherapies of gemcitabine or ACT.
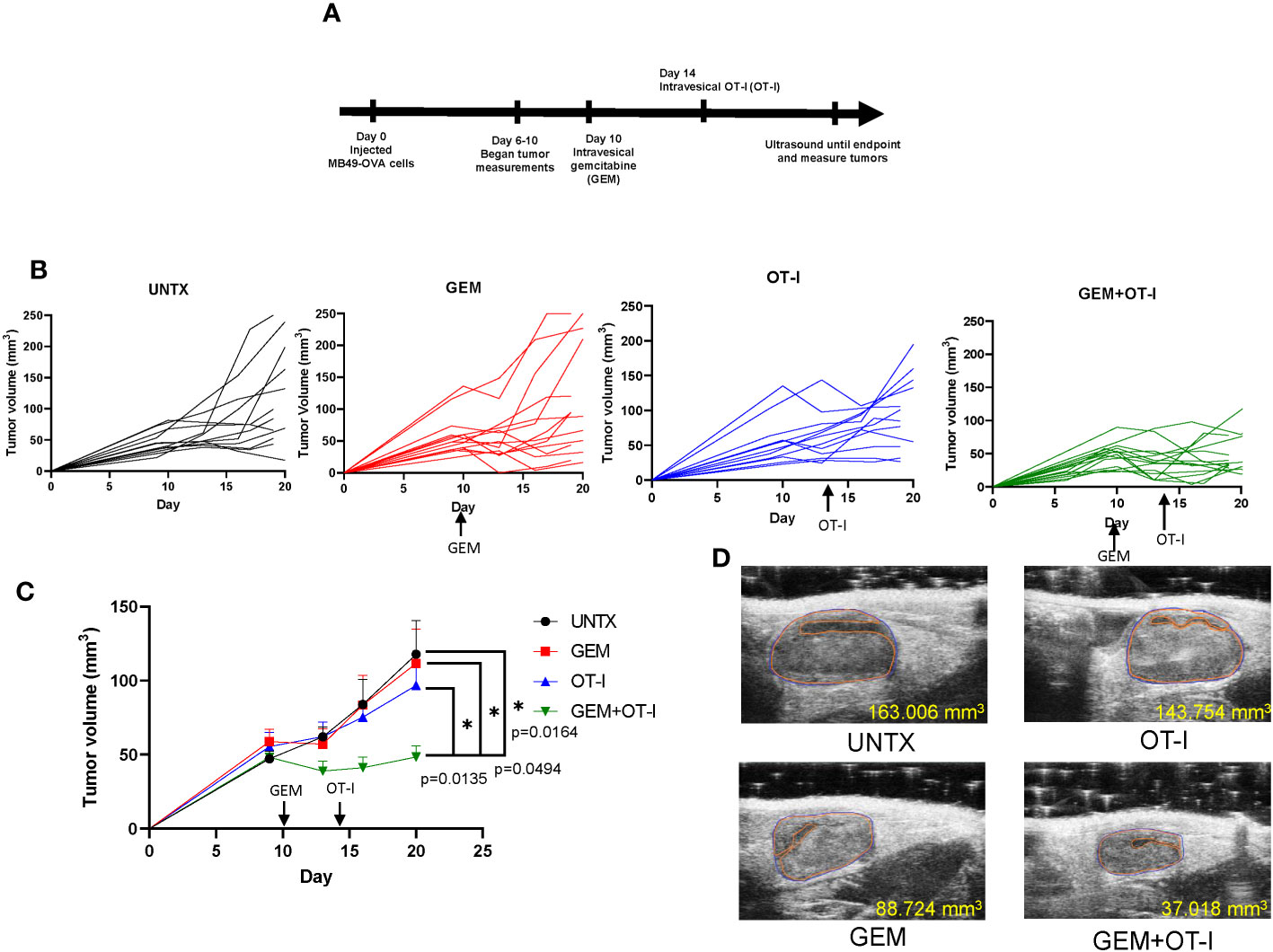
Figure 6 Pretreatment of gemcitabine combined with ACT improves anti-tumor responses. (A) Schematic showing timeline of treatments. (B) Individual growth curves of mice in the UNTX, GEM, OT-I, and GEM+OT-I groups. n=12-14 per group. (C) Average tumor growth curves for each treatment group. Statistical analysis performed via Compare Groups of Growth Curves (CGGC) test using 10,000 permutations. (D) Representative ultrasound images of each treatment group at day 20. *, p ≤ 0.05.
Discussion
The tumor microenvironment is made up of several immune populations, many of which are suppressive to T cells (16). Specifically, MDSCs in bladder cancer have been associated with poorer clinical responses to BCG and neoadjuvant chemotherapy. Additionally, higher levels of MDSCs have been associated with higher grade malignancies in bladder cancer (23, 43). In this work, we have shown that MDSCs are enriched within the microenvironment of both human and murine bladder tumors. These MDSCs suppress activated T cells and exist in a microenvironment that is enriched for inflammatory cytokines, myeloid chemokines, and T cell chemokines. We show that intravesical instillation of gemcitabine can be used to locally lymphodeplete the bladder microenvironment with little impact to cytokines important for T cell migration. We predict that the high levels of CXCL10 can enhance migration of T cells into the bladder through interactions with CXCR3 (15). However, we do see a reduction in VEGF after treatment with gemcitabine, which warrants further investigation as to how this may impact ACT. We did expect to see changes in other myeloid cytokines and chemokines; however, it is possible that the kinetics of the other cytokines tested may require more time to show differences in the microenvironment. In our previous manuscript, we show that intravesical instillation of OT-I T cells is sufficient to control growth of bladder tumors. In this work, by design our bladder tumor burden was larger and ACT with OT-I alone was not able to control tumor growth. This was done intentionally to determine additive value of altering the tumor microenvironment. When gemcitabine is used to precondition the bladder microenvironment, we see an added anti-tumor benefit in combination with intravesical instillation of OT-I transgenic T cells. Even though our murine model had a larger tumor burden than our previous studies and with a human corollary of mixed stage disease, we still found an anti-tumor effect with combination intravesical therapy. This provides baseline data for the use of combination therapy for therapeutic pathway for smaller lower stage disease. Larger sample sizes correlating MDSCs to grade of tumor for smaller tumors would be a next step.
Cancer patients receiving T cell therapies typically undergo a systemic, nonmyeloablative preconditioning step with cyclophosphamide and fludarabine (44). This allows for the depletion of cytokine sinks and immunosuppressive populations that attenuate the activity of adoptively transferred T cells (45, 46). While a necessary preconditioning step, this lymphodepletion regimen is a significant source of patient toxicity and can lead to an overall reduction in quality of life (47, 48). Patients with bladder cancer who receive intravesical ACT would not require systemic chemotherapy due to local administration of the infusion product. Nonetheless, T cells adoptively transferred into the bladder are more likely to be affected by the immunosuppressive populations present in the bladder microenvironment. Here, we use gemcitabine to precondition the bladder microenvironment before administration of ACT. This allows for the local depletion of immunosuppressive populations that would attenuate T cell responses in the bladder without the toxicities associated with systemic chemotherapy.
One potential limitation in using gemcitabine as a lymphodepleting agent is separating out the tumoricidal effects from the lymphodepleting effects. One study using gemcitabine as a lymphodepleting agent showed that the tumoricidal properties of gemcitabine dominate when the tumors are small and the lymphodepleting properties dominate when the tumors are larger due to the accumulation of MDSCs and other immune populations (49). In this work, we allow tumors to grow larger (50 mm3) before we perform intravesical instillation of gemcitabine to optimize for lymphodepletion. Additionally, this is the tumor size where monotherapy with OT-I T cells has limited antitumor responses. Despite these limitations, gemcitabine is already used as a treatment for bladder cancer and its implementation as a preconditioning agent likely requires minimal adjustments to clinical practice (50). Another potential limitation of this work is the use of the OVA model for ACT. The immunogenicity of the OVA257-264 antigen expressed by the tumor cells may not be representative of the typical immunogenicity of tumor antigens in bladder cancer. Ideally, TIL from murine bladder tumors would be expanded ex vivo and used for ACT to more closely model the therapy administered in the clinic. Here, we use the OVA antigen specificity model to control for antigen recognition and reactivity of the infused T cell product.
We show that gemcitabine can be used as a preconditioning agent and that one round of instillation is sufficient to induce added benefits to ACT. Given that gemcitabine is administered locally to alter the bladder microenvironment, we expect the MDSCs to reconstitute back into the bladder over time. More work will need to be done to map out the optimal number of preconditioning cycles to enhance efficacy of ACT. Additionally, multiple infusions of T cells can be performed, allowing for the development of a highly personalized treatment regimen for each patient. Overall, using gemcitabine as a lymphodepleting agent can augment the anti-tumor responses of ACT.
Data availability statement
The original contributions presented in the study are included in the article/supplementary material. Further inquiries can be directed to the corresponding author.
Ethics statement
The studies involving humans were approved by Moffitt Scientific Research Committee and Advarra IRB. The studies were conducted in accordance with the local legislation and institutional requirements. The human samples used in this study were acquired from a by- product of routine care or industry. Written informed consent was received from participants in accordance with national legislation and institutional requirements. The animal study was approved by Institutional Animal Care and Use Committee (IACUC). The study was conducted in accordance with the local legislation and institutional requirements.
Author contributions
SB: Conceptualization, Data curation, Formal Analysis, Methodology, Project administration, Supervision, Writing – original draft. BB: Conceptualization, Data curation, Formal Analysis, Methodology, Project administration, Supervision, Writing – review & editing. AO: Data curation, Formal Analysis, Supervision, Writing – original draft. JB: Methodology, Project administration, Writing – review & editing. AL: Data curation, Writing – review & editing. JA: Data curation, Writing – review & editing. DA: Project administration, Writing – review & editing. CC: Data curation, Project administration, Writing – review & editing. AH: Project administration, Writing – review & editing. MB: Conceptualization, Formal Analysis, Supervision, Writing – review & editing. MP: Formal Analysis, Supervision, Writing – review & editing. KR: Formal Analysis, Funding acquisition, Project administration, Supervision, Writing – review & editing. SP-T: Conceptualization, Formal Analysis, Funding acquisition, Methodology, Project administration, Supervision, Writing – review & editing.
Funding
The authors declare financial support was received for the research, authorship, and/or publication of this article. This work was supported by the National Institutes of Health, National Cancer Institute grants R01CA259387, and the Department of Defense grant W81XWH-22-1-0341. W81XWH-22-1-0339 and W81XWH-22-1-0340. There should be a total of three DOD grants ending in -0339, -0340, -0341 and one R01.
Acknowledgments
The authors would like to acknowledge the individuals Ahmet Murat Aydin and Sarah Asby for their assistance. This work was supported in part by the Shared Resources, including Flow Cytometry Core Facility, Small Animal Imaging Lab, Tissue Core Facility, Microscopy Core Facility, and Cell Therapy Facility, at the H. Lee Moffitt Cancer Center and Research Institute, an NCI designated Comprehensive Cancer Center through the National Institutes of Health Grant P30-CA076292.
Conflict of interest
BB is currently employed at Iovance Biotherapeutics. AH reports common stock holdings in AbbVie, Inc., Amgen, Inc., BioHaven Pharmaceuticals, and Bristol Myers Squibb. KR has received research support that is not related to this research from NIH-NCI R01CA272601 and R01CA230610. SP-T receives salary support on sponsored research agreements between Moffitt Cancer Center and Iovance Biotherapeutics, Turnstone Biologics, Intellia Therapeutics, and Dyve Biosciences. SP-T is a member of the SAB for KSQ Therapeutics, Inc. SP-T and MB are consultants for Morphogenesis, Inc. Moffitt Cancer Center and Research Institute has licensed intellectual property related to the proliferation and expansion of tumor infiltrating lymphocytes TIL to Iovance Biotherapeutics.
The remaining authors declare that the research was conducted in the absence of any commercial or financial relationships that could be construed as a potential conflict of interest.
The author(s) declared that they were an editorial board member of Frontiers, at the time of submission. This had no impact on the peer review process and the final decision.
Publisher’s note
All claims expressed in this article are solely those of the authors and do not necessarily represent those of their affiliated organizations, or those of the publisher, the editors and the reviewers. Any product that may be evaluated in this article, or claim that may be made by its manufacturer, is not guaranteed or endorsed by the publisher.
References
1. Sharma S, Ksheersagar P, Sharma P. Diagnosis and treatment of bladder cancer. Am Fam Physician (2009) 80(7):717–23.
2. Saginala K, Barsouk A, Aluru JS, Rawla P, Padala SA, Barsouk A. Epidemiology of bladder cancer. Med Sci (Basel) (2020) 8(1). doi: 10.3390/medsci8010015
3. Jordan B, Meeks JJ. T1 bladder cancer: current considerations for diagnosis and management. Nat Rev Urol (2019) 16(1):23–34. doi: 10.1038/s41585-018-0105-y
4. Shore ND, Palou Redorta J, Robert G, Hutson TE, Cesari R, Hariharan S, et al. Non-muscle-invasive bladder cancer: An overview of potential new treatment options. Urol Oncol (2021) 39(10):642–63. doi: 10.1016/j.urolonc.2021.05.015
5. Joice GA, Bivalacqua TJ, Kates M. Optimizing pharmacokinetics of intravesical chemotherapy for bladder cancer. Nat Rev Urol (2019) 16(10):599–612. doi: 10.1038/s41585-019-0220-4
6. Patel VG, Oh WK, Galsky MD. Treatment of muscle-invasive and advanced bladder cancer in 2020. CA Cancer J Clin (2020) 70(5):404–23. doi: 10.3322/caac.21631
7. Lu M, Chen S, Zhou Q, Wang L, Peng T, Wang G. Predicting recurrence of nonmuscle-invasive bladder cancer (Ta-T1): A study based on 477 patients. Med (Baltimore) (2019) 98(28):e16426. doi: 10.1097/MD.0000000000016426
8. Bree KK, Shan Y, Hensley PJ, Lobo N, Hu C, Tyler DS, et al. Management, surveillance patterns, and costs associated with low-grade papillary stage ta non-muscle-invasive bladder cancer among older adults, 2004-2013. JAMA Netw Open (2022) 5(3):e223050. doi: 10.1001/jamanetworkopen.2022.3050
9. Forget MA, Haymaker C, Hess KR, Meng YJ, Creasy C, Karpinets T, et al. Prospective analysis of adoptive TIL therapy in patients with metastatic melanoma: response, impact of anti-CTLA4, and biomarkers to predict clinical outcome. Clin Cancer Res (2018) 24(18):4416–28. doi: 10.1158/1078-0432.CCR-17-3649
10. Hall M, Liu H, Malafa M, Centeno B, Hodul PJ, Pimiento J, et al. Expansion of tumor-infiltrating lymphocytes (TIL) from human pancreatic tumors. J Immunother Cancer (2016) 4:61. doi: 10.1186/s40425-016-0164-7
11. Rosenberg SA, Dudley ME. Adoptive cell therapy for the treatment of patients with metastatic melanoma. Curr Opin Immunol (2009) 21(2):233–40. doi: 10.1016/j.coi.2009.03.002
12. Seitter SJ, Sherry RM, Yang JC, Robbins PF, Shindorf ML, Copeland AR, et al. Impact of prior treatment on the efficacy of adoptive transfer of tumor-infiltrating lymphocytes in patients with metastatic melanoma. Clin Cancer Res (2021) 27(19):5289–98. doi: 10.1158/1078-0432.CCR-21-1171
13. Aydin AM, Bunch BL, Beatty M, Hajiran A, Dhillon J, Sarnaik AA, et al. The factors affecting expansion of reactive tumor infiltrating lymphocytes (TIL) from bladder cancer and potential therapeutic applications. Front Immunol (2021) 12:628063. doi: 10.3389/fimmu.2021.628063
14. Poch M, Hall M, Joerger A, Kodumudi K, Beatty M, Innamarato PP, et al. Expansion of tumor infiltrating lymphocytes (TIL) from bladder cancer. Oncoimmunol (2018) 7(9):e1476816. doi: 10.1080/2162402X.2018.1476816
15. Bunch BL, Morse J, Asby S, Blauvelt J, Aydin AM, Innamarato P, et al. Systemic and intravesical adoptive cell therapy of tumor-reactive T cells can decrease bladder tumor growth in vivo. J Immunother Cancer (2020) 8(2). doi: 10.1136/jitc-2020-001673
16. Labani-Motlagh A, Ashja-Mahdavi M, Loskog A. The tumor microenvironment: A milieu hindering and obstructing antitumor immune responses. Front Immunol (2020) 11:940. doi: 10.3389/fimmu.2020.00940
17. Vasievich EA, Huang L. The suppressive tumor microenvironment: a challenge in cancer immunotherapy. Mol Pharm (2011) 8(3):635–41. doi: 10.1021/mp1004228
18. Crispen PL, Kusmartsev S. Mechanisms of immune evasion in bladder cancer. Cancer Immunol Immunother (2020) 69(1):3–14. doi: 10.1007/s00262-019-02443-4
19. Lechner MG, Liebertz DJ, Epstein AL. Characterization of cytokine-induced myeloid-derived suppressor cells from normal human peripheral blood mononuclear cells. J Immunol (2010) 185(4):2273–84. doi: 10.4049/jimmunol.1000901
20. Prasanna T, Craft P, Balasingam G, Haxhimolla H, Pranavan G. Intravesical gemcitabine versus intravesical bacillus calmette-guerin for the treatment of non-muscle invasive bladder cancer: an evaluation of efficacy and toxicity. Front Oncol (2017) 7:260. doi: 10.3389/fonc.2017.00260
21. Bracci L, Schiavoni G, Sistigu A, Belardelli F. Immune-based mechanisms of cytotoxic chemotherapy: implications for the design of novel and rationale-based combined treatments against cancer. Cell Death Differ (2014) 21(1):15–25. doi: 10.1038/cdd.2013.67
22. Zaharoff DA, Hance KW, Rogers CJ, Schlom J, Greiner JW. Intratumoral immunotherapy of established solid tumors with chitosan/IL-12. J Immunother (2010) 33(7):697–705. doi: 10.1097/CJI.0b013e3181eb826d
23. Yang G, Shen W, Zhang Y, Liu M, Zhang L, Liu Q, et al. Accumulation of myeloid-derived suppressor cells (MDSCs) induced by low levels of IL-6 correlates with poor prognosis in bladder cancer. Oncotarget (2017) 8(24):38378–88. doi: 10.18632/oncotarget.16386
24. Fallah J, Diaz-Montero CM, Rayman P, Wei W, Finke JH, Kim JS, et al. Myeloid-derived suppressor cells in nonmetastatic urothelial carcinoma of bladder is associated with pathologic complete response and overall survival. Clin Genitourin Cancer (2020) 18(6):500–8. doi: 10.1016/j.clgc.2020.03.004
25. Joseph M, Enting D. Immune responses in bladder cancer-role of immune cell populations, prognostic factors and therapeutic implications. Front Oncol (2019) 9:1270. doi: 10.3389/fonc.2019.01270
26. Kumari N, Agrawal U, Mishra AK, Kumar A, Vasudeva P, Mohanty NK, et al. Predictive role of serum and urinary cytokines in invasion and recurrence of bladder cancer. Tumour Biol (2017) 39(4):1010428317697552. doi: 10.1177/1010428317697552
27. Salmasi A, Elashoff DA, Guo R, Upfill-Brown A, Rosser CJ, Rose JM, et al. Urinary cytokine profile to predict response to intravesical BCG with or without HS-410 therapy in patients with non-muscle-invasive bladder cancer. Cancer Epidemiol Biomarkers Prev (2019) 28(6):1036–44. doi: 10.1158/1055-9965.EPI-18-0893
28. Eckstein M, Epple E, Jung R, Weigelt K, Lieb V, Sikic D, et al. CCL2 expression in tumor cells and tumor-infiltrating immune cells shows divergent prognostic potential for bladder cancer patients depending on lymph node stage. Cancers (Basel) (2020) 12(5). doi: 10.3390/cancers12051253
29. Chen C, He W, Huang J, Wang B, Li H, Cai Q, et al. LNMAT1 promotes lymphatic metastasis of bladder cancer via CCL2 dependent macrophage recruitment. Nat Commun (2018) 9(1):3826. doi: 10.1038/s41467-018-06152-x
30. Reis ST, Leite KR, Piovesan LF, Pontes-Junior J, Viana NI, Abe DK, et al. Increased expression of MMP-9 and IL-8 are correlated with poor prognosis of Bladder Cancer. BMC Urol (2012) 12:18. doi: 10.1186/1471-2490-12-18
31. Morizawa Y, Miyake M, Shimada K, Hori S, Tatsumi Y, Nakai Y, et al. Correlation of immune cells and cytokines in the tumor microenvironment with elevated neutrophil-to-lymphocyte ratio in blood: an analysis of muscle-invasive bladder cancer. Cancer Invest (2018) 36(7):395–405. doi: 10.1080/07357907.2018.1506800
32. Chen MF, Lin PY, Wu CF, Chen WC, Wu CT. IL-6 expression regulates tumorigenicity and correlates with prognosis in bladder cancer. PloS One (2013) 8(4):e61901. doi: 10.1371/journal.pone.0061901
33. Matsumoto R, Tsuda M, Yoshida K, Tanino M, Kimura T, Nishihara H, et al. Aldo-keto reductase 1C1 induced by interleukin-1beta mediates the invasive potential and drug resistance of metastatic bladder cancer cells. Sci Rep (2016) 6:34625. doi: 10.1038/srep34625
34. Tokunaga R, Zhang W, Naseem M, Puccini A, Berger MD, Soni S, et al. CXCL9, CXCL10, CXCL11/CXCR3 axis for immune activation - A target for novel cancer therapy. Cancer Treat Rev (2018) 63:40–7. doi: 10.1016/j.ctrv.2017.11.007
35. Muthuswamy R, Wang L, Pitteroff J, Gingrich JR, Kalinski P. Combination of IFNalpha and poly-I:C reprograms bladder cancer microenvironment for enhanced CTL attraction. J Immunother Cancer (2015) 3:6. doi: 10.1186/s40425-015-0050-8
36. Kohli K, Pillarisetty VG, Kim TS. Key chemokines direct migration of immune cells in solid tumors. Cancer Gene Ther (2022) 29(1):10–21. doi: 10.1038/s41417-021-00303-x
37. Reschke R, Yu J, Flood B, Higgs EF, Hatogai K, Gajewski TF. Immune cell and tumor cell-derived CXCL10 is indicative of immunotherapy response in metastatic melanoma. J Immunother Cancer (2021) 9(9). doi: 10.1136/jitc-2021-003521
38. Alfaro C, Teijeira A, Onate C, Perez G, Sanmamed MF, Andueza MP, et al. Tumor-produced interleukin-8 attracts human myeloid-derived suppressor cells and elicits extrusion of neutrophil extracellular traps (NETs). Clin Cancer Res (2016) 22(15):3924–36. doi: 10.1158/1078-0432.CCR-15-2463
39. Shelley MD, Jones G, Cleves A, Wilt TJ, Mason MD, Kynaston HG. Intravesical gemcitabine therapy for non-muscle invasive bladder cancer (NMIBC): a systematic review. BJU Int (2012) 109(4):496–505. doi: 10.1111/j.1464-410X.2011.10880.x
40. Velaer KN, Steinberg RL, Thomas LJ, O'Donnell MA, Nepple KG. Experience with sequential intravesical gemcitabine and docetaxel as salvage therapy for non-muscle invasive bladder cancer. Curr Urol Rep (2016) 17(5):38. doi: 10.1007/s11934-016-0594-2
41. Law AMK, Valdes-Mora F, Gallego-Ortega D. Myeloid-derived suppressor cells as a therapeutic target for cancer. Cells (2020) 9(3). doi: 10.3390/cells9030561
42. Gabrilovich DI, Nagaraj S. Myeloid-derived suppressor cells as regulators of the immune system. Nat Rev Immunol (2009) 9(3):162–74. doi: 10.1038/nri2506
43. Puttmann K. The role of myeloid derived suppressor cells in urothelial carcinoma immunotherapy. Bladder Cancer (2019) 5(2):103–14. doi: 10.3233/BLC-190219
44. Zhao L, Cao YJ. Engineered T cell therapy for cancer in the clinic. Front Immunol (2019) 10:2250. doi: 10.3389/fimmu.2019.02250
45. Nissani A, Lev-Ari S, Meirson T, Jacoby E, Asher N, Ben-Betzalel G, et al. Comparison of non-myeloablative lymphodepleting preconditioning regimens in patients undergoing adoptive T cell therapy. J Immunother Cancer (2021) 9(5). doi: 10.1136/jitc-2020-001743
46. Rosenberg SA, Restifo NP, Yang JC, Morgan RA, Dudley ME. Adoptive cell transfer: a clinical path to effective cancer immunotherapy. Nat Rev Cancer (2008) 8(4):299–308. doi: 10.1038/nrc2355
47. Dawicki W, Allen KJH, Garg R, Geoghegan EM, Berger MS, Ludwig DL, et al. Targeted lymphodepletion with a CD45-directed antibody radioconjugate as a novel conditioning regimen prior to adoptive cell therapy. Oncotarget (2020) 11(39):3571–81. doi: 10.18632/oncotarget.27731
48. Lowe KL, Mackall CL, Norry E, Amado R, Jakobsen BK, Binder G. Fludarabine and neurotoxicity in engineered T-cell therapy. Gene Ther (2018) 25(3):176–91. doi: 10.1038/s41434-018-0019-6
49. Le HK, Graham L, Cha E, Morales JK, Manjili MH, Bear HD. Gemcitabine directly inhibits myeloid derived suppressor cells in BALB/c mice bearing 4T1 mammary carcinoma and augments expansion of T cells from tumor-bearing mice. Int Immunopharmacol (2009) 9(7-8):900–9. doi: 10.1016/j.intimp.2009.03.015
Keywords: tumor infiltrating lymphocytes (TIL), adoptive cell therapy (ACT), non-muscle invasive bladder cancer (NMIBC), neoadjuvant, gemcitabine, lymphodepletion
Citation: Bazargan S, Bunch B, Ojwang‘ AME, Blauvelt J, Landin A, Ali J, Abrahams D, Cox C, Hall AM, Beatty MS, Poch M, Rejniak KA and Pilon-Thomas S (2023) Targeting myeloid-derived suppressor cells with gemcitabine to enhance efficacy of adoptive cell therapy in bladder cancer. Front. Immunol. 14:1275375. doi: 10.3389/fimmu.2023.1275375
Received: 09 August 2023; Accepted: 28 September 2023;
Published: 12 October 2023.
Edited by:
Dev Karan, Medical College of Wisconsin, United StatesReviewed by:
Thomas A. Mace, The Ohio State University, United StatesWilliam Tabayoyong, University of Rochester, United States
Gregory B. Lesinski, Emory University, United States
Copyright © 2023 Bazargan, Bunch, Ojwang‘, Blauvelt, Landin, Ali, Abrahams, Cox, Hall, Beatty, Poch, Rejniak and Pilon-Thomas. This is an open-access article distributed under the terms of the Creative Commons Attribution License (CC BY). The use, distribution or reproduction in other forums is permitted, provided the original author(s) and the copyright owner(s) are credited and that the original publication in this journal is cited, in accordance with accepted academic practice. No use, distribution or reproduction is permitted which does not comply with these terms.
*Correspondence: Shari Pilon-Thomas, Shari.Pilon-Thomas@moffitt.org