- 1Department of Respiratory Medicine, Children’s Hospital of Nanjing Medical University, Nanjing, China
- 2Pediatric Department, Northern Jiangsu People’s Hospital, Yangzhou, China
- 3Department of Pharmacy, The Affiliated Cancer Hospital of Nanjing Medical University and Jiangsu Cancer Hospital and Jiangsu Institute of Cancer Research, Nanjing, China
- 4Research Division of Clinical Pharmacology, The First Affiliated Hospital of Nanjing Medical University, Nanjing, China
- 5National Vaccine Innovation Platform, Nanjing Medical University, Nanjing, China
Background: The house dust mite (HDM) is widely recognized as the most prevalent allergen in allergic diseases. Allergen-specific immunotherapy (AIT) has been successfully implemented in clinical treatment for HDM. Hypoallergenic B-cell epitope-based vaccine designed by artificial intelligence (AI) represents a significant progression of recombinant hypoallergenic allergen derivatives.
Method: The three-dimensional protein structure of Der f 36 was constructed using Alphafold2. AI-based tools were employed to predict B-cell epitopes, which were subsequently verified through IgE-reaction testing. Hypoallergenic Der f 36 was then synthesized, expressed, and purified. The reduced allergenicity was assessed by enzyme-linked immunosorbent assay (ELISA), immunoblotting, and basophil activation test. T-cell response to hypoallergenic Der f 36 and Der f 36 was evaluated based on cytokine expression in the peripheral blood mononuclear cells (PBMCs) of patients. The immunogenicity was evaluated and compared through rabbit immunization with hypoallergenic Der f 36 and Der f 36, respectively. The inhibitory effect of the blocking IgG antibody on the specific IgE-binding activity and basophil activation of Der f 36 allergen was also examined.
Results: The final selected non-allergic B-cell epitopes were 25–48, 57–67, 107–112, 142–151, and 176–184. Hypoallergenic Der f 36 showed significant reduction in IgE-binding activity. The competitive inhibition of IgE-binding to Der f 36 was investigated using the hypoallergenic Der f 36, and only 20% inhibition could be achieved, which is greatly reduced when compared with inhibition by Der f 36 (98%). The hypoallergenic Der f 36 exhibited a low basophil-stimulating ratio similar to that of the negative control, and it could induce an increasing level of IFN‐γ but not Th2 cytokines IL-5 and IL-13 in PBMCs. The vaccine-specific rabbit blocking IgG antibodies could inhibit the patients’ IgE binding and basophil stimulation activity of Derf 36.
Conclusion: This study represents the first application of an AI strategy to facilitate the development of a B-cell epitope-based hypoallergenic Der f 36 vaccine, which may become a promising immunotherapy for HDM-allergic patients due to its reduced allergenicity and its high immunogenicity in inducing blocking of IgG.
1 Introduction
The house dust mite (HDM) has been widely recognized as a primary contributor to indoor aeroallergens, which is capable of eliciting IgE-mediated allergic diseases including allergic rhinitis (AR), asthma (AS), and atopic dermatitis (AD). Both AR and AS are the most common allergic diseases in children with an estimated prevalence range of 2%–25% and 3%–38%, respectively (1, 2). In China, HDM emerged as the leading allergen among children diagnosed with AR and/or asthma with approximately 60% of patients exhibiting sensitization to it (3). A previous study indicated that 74.5% of children diagnosed with AD were hypersensitive to HDM extracts as determined by skin prick test (4). The high prevalence of sensitization to HDM necessitates the development of effective therapeutics with substantial efficacy.
Allergen‐specific immunotherapy (AIT) is the only disease-modifying therapy for mite allergy (5). It was demonstrated to impede the progression of symptoms from mild to severe in children with mite allergy (6). The current vaccine used in AIT for mite allergy is still based on the natural HDM extracts. However, the quality of natural HDM extracts is limited (7–9). A previous study has reported significant variability among 10 commercially available HDM extracts, attributed to the absence of crucial allergens in the extract products and the varying IgE reactivity of different mite allergens (4), or which might contain several proteases, which can degrade proteins in HDM extracts (7).
The use of purified recombinant allergens for diagnosis enables the identification of specific allergens facilitating precision immunotherapy (10). However, native‐like recombinant allergens still retain IgE reactivity and T‐cell epitopes leading to immediate and late‐phase adverse reactions. Efficient approach might be achieved by recombinant hypoallergenic allergen derivatives. B-cell epitope-based hypoallergenic vaccines represent a further improvement for hypoallergenic derivatives of recombinant vaccine. These vaccines have the capability to induce a significant increase in allergen-specific IgG antibodies, which in turn can provide protection against allergic inflammation by inhibiting the reactivity of specific IgE and the activation of basophils in response to native-like allergens (11). The prediction of epitopes is a significant concern in the field of immunoinformatics and holds great practical implications for the development of epitope-based vaccines (12). Artificial intelligence (AI), defined as the utilization of computer algorithms and programs to mimic human intelligence (13), presents a promising avenue for enhancing the efficacy and success rate of traditional vaccine development. In the field of vaccine development, AI has been developed to forecast protein structure, predict B-cell epitopes (14, 15), determine class II major histocompatibility complex (MHC-II) epitopes, and facilitate the design of vaccines targeting the MHC-II immune peptidome (16, 17). Additionally, AI has been employed to identify potential biomarkers for seasonal flu vaccines (17), and analyze immune system response (18). Compared to the conventional ways, AI can be more accurate than animal models by taking human anatomy and physiology into consideration when simulating the effect of a new product (19). This would increase efficiency and reduce the cost for each drug.
Previous studies have highlighted the significance of groups 1, 2, and 23 in HDM as the most crucial allergens (20). Most hypoallergenic vaccine studies focused on the major allergens and individualized therapy for mite allergy (21). Der f 36 is a newly identified HDM allergen in recent years. It is a new allergen group without significant amino acid sequence similarity with known allergens. The IgE-binding frequency was determined as 42%–60% in France based on the recombinant Der f 36 and natural molecule (22), which made Der f 36 an important component for desensitization. However, there was no report focusing on the development of a hypoallergenic vaccine specifically targeting Der f 36.
In this research, we applied the AI strategy to facilitate the design of recombinant hypoallergenic B-cell epitope-based Der f 36 vaccine. The reduced allergenicity of the vaccine was characterized by enzyme-linked immunosorbent assay (ELISA), Western blot (WB), inhibition ELISA, basophil activation test, and peripheral blood mononuclear cell (PBMC) cytokine expression. The immunogenicity was evaluated through a rabbit immunization study. The IgG blocking of IgE-binding test and basophil activation test were also used to characterize the IgE inhibition ability of hypoallergic Der f 36 vaccine. We believe that the AI-driven designed hypoallergenic Der f 36 represents a promising strategy and vaccine candidate for immunotherapy of mite-allergic patients.
2 Method
2.1 Expression and purification of recombinant Der f 36
Der f 36 was already produced from the recombinant expression plasmid pET-28a (+) in Escherichia coli and stored at −80°C for the follow-up experiment. Briefly, the Der f 36 clone was inoculated in Luria–Bertani (LB) medium containing 50 μg/ml of kanamycin at 37°C and shaken at 200 rpm. When the optical density (OD) at 600 nm reached 0.6, isopropyl β-D-1-thiogalactopyranoside (IPTG) was added at a final concentration of 1 mM (shaking at 180 rpm at 37°C for another 4 h). The culture was harvested by centrifugation and disrupted by sonication for 20 min. The lysate was centrifuged at 12,000 × g for 10 min at 4°C, and the liquid supernatant was purified using HisTrap HP column (GE Healthcare, Uppsala, Sweden) and HiTrap Q HP column (GE Healthcare, Uppsala, Sweden) according to the manufacturer’s instructions. The concentration of endotoxin was determined by chromogenic method (details are presented in the Supplementary Material). Reverse-phase high-performance liquid chromatography (RP-HPLC) was used to determine the purity of Der f 36 (details are presented in the Supplementary Material).
2.2 Patients and samples
The Der f 36-positive serum was selected from 100 HDM positive serum in our preparatory work by ELISA (detailed procedures shown as below). HDM-positive sera were selected based on positive case history of HDM allergy and specific IgE to Dermatophagoides farinae > 0.35 kUA/L in serum, as determined by the ImmunoCAP System (Thermo Fisher Scientific/Phadia, Uppsala, Sweden). The non-allergic sera were used as the control group. HDM-positive sera with IgE antibodies specific to Der f 36 (n = 24) were selected for the assessment of hypoallergenic Der f 36. The study was approved by the ethics committee of the Children’s Hospital of Nanjing Medical University.
2.3 Construction of hypoallergenic B-cell epitope-based Der f 36 vaccine
2.3.1 The tertiary structure construction and quality assessment of Der f 36
To design the hypoallergenic vaccine, the information of amino acid sequence of Der f 36 was retrieved from WHO/IUIS (http://www.allergen.org) and GenBank (protein ID: ATI08931.1). The amino acid sequence was submitted to Alphafold2 to build a three-dimensional (3D) model (23). PROCHECK (24) and ERRAT (25) in SAVES v6.0 serve (https://saves.mbi.ucla.edu/) was used to evaluate the model’s quality. Structural visualization was performed in PyMOL software (https://pymol.org/2/).
2.3.2 Prediction of T-cell epitopes
T-cell epitopes were aimed to be avoided from the B-cell epitope-based hypoallergen to reduce the T-cell epitope-mediated late-phase side effects (26, 27). T-cell epitopes containing regions were predicted by TepiTool (http://tools.iedb.org/tepitool/) (28). The 26 most frequent human class II alleles from HLA-DR, HLA-DQ, and HLA-DP with a percentile rank ≤ 10 were set. The final T-cell epitope was selected from those containing two or more MHC-II alleles.
2.3.3 Prediction of B-cell epitopes and allergenicity of synthesized B-cell epitope
Three methods, including AI and traditional computational prediction, were selected for linear B-cell epitope prediction. The 3D structure of Der f 36 was submit to the ElliPro server (http://tools.iedb.org/ellipro/) (29). To identify regions containing B-cell epitopes, the score was set at >0.5. Bepipred 2.0 (http://tools.iedb.org/bcell/) (30) and Graphbepi (http://bio-web1.nscc-gz.cn/app/graphbepi) were AI methods that were also used to predict B-cell epitopes (31). In Bepipred 2.0, the threshold was set at 0.5. The final linear B-cell epitopes were selected from the shared sequence from two or more prediction methods.
Conformational epitopes relied on the 3D structure of the protein. Conformational epitopes make essential contributions to allergenicity in inhalant allergens (32). Two AI methods, Seppa 3.0 (http://www.badd-cao.net/seppa3/index.html) (33) and SEMA (http://sema.airi.net) (34), were employed to predict the key residues contributed for discontinuous B-cell epitopes. The threshold of Seppa 3.0 was set at 0.064, and the SEMA value should be more than 1.1. All the results were integrated.
The final selected B-cell epitope peptides were synthesized by GenScript (Nanjing, China). Two milligrams of these epitope peptides was dissolved in 50 µl of ultrapure water, and a 2-μl sample was spotted on a nitrocellulose membrane to determine each epitope’s IgE-binding activity. Membranes were blocked with 1% BSA and incubated with 10 Der f 36-positive serum and one nonallergic sera (1:10 dilution) overnight at 4°C. The membranes were incubated with HRP-conjugated anti-human IgE antibody (1:5,000 dilution; KPL, MD, USA) and visualized by adding Immobilon™ Western HRP Substrate Luminol Reagent (Merck Millipore, MA, USA).
Moreover, the same method was used to detect these epitopes’ binding activity to human IgG4 except the membranes were finally incubated with mouse anti-human IgG4 antibody (1:5,000 dilution; Thermo Fisher Scientific, Massachusetts, USA).
2.3.4 Design of hypoallergenic B-cell epitope-based Der f 36 vaccine
The PreS domain derived from hepatitis B virus was selected as a qualified carrier to integrate B-cell epitopes based on its immunogenicity and safety (35–37). The candidate fragments without IgE reactivity and without T-cell epitope regions were linked by KK linker at the N and C terminal of the PreS carrier (38, 39). The physicochemical properties were calculated using ProtParam (http://web.expasy.org/protparam/).
2.4 Expression and purification of constructed hypoallergenic Der f 36
The designed hypoallergenic B-cell vaccine gene combined with the C-terminal His-tag was synthesized by GENCEFE Biotech (Wuxi, China) and cloned into the pET-28a and transformed into E. coli strain BL21(DE3) (Tsingke, Beijing, China).
The expression of hypoallergenic Der f 36 was the same as Der f 36. The targeted protein was expressed as inclusion bodies and solubilized with 10 mM of Tris-HCL, pH 8.0, 100 mM of NaH2PO3 containing 8 M of urea, then purified by HisTrap™ HP affinity column. The protein was dialyzed into the refolding solution [0.5 M arginine, 1 mM of glutathione reduced (GSH), 0.1 mM of glutathione oxidized (GSSG), 50 mM of Tris, 0.5 M of NaCl, 5 mM of EDTA, 10% glycerol, pH 9.5] for 24 h at 4°C. The refolded protein was further purified by HiTrap™ SP HP cation exchange column (GE Healthcare, Uppsala, Sweden). The detailed elution condition is provided in the Supporting Material. The concentration of endotoxin was also determined. The purity of hypoallergenic Der f 36 was tested by RP-HPLC as described above. Anti-6×tis-tag antibody was used to verify the targeted protein by Western blot as described in the Supporting Material.
2.5 IgE reactivity of hypoallergenic Der f 36
2.5.1 IgE reactivity analyzed by ELISA
The 96-well plates (Corning Costar, Maine, USA) were separately coated with 1 µg of hypoallergenic Der f 36 or Der f 36 in phosphate-buffered saline (PBS) per well then incubated at 4°C overnight. The plate was blocked with 1% BSA at 37°C for 2 h. The 24 IgE-positive sera, 100 µL/well (diluted in PBST PBS containing 0.1% Tween-20 1:10), were added and incubated at 37°C for 1 h. HRP-conjugated goat anti-human IgE (KPL, MD, USA) was added for 1 h, and chromogenic reaction was developed using TMB substrate (Beyotime, Shanghai, China) for 10 min. Then the reaction was stopped by 1 M of H2SO4, and the absorbance was measured at 450 nm using Multiskan GO (Thermo Fisher Scientific, MA, USA). The cut-off value was presented as mean OD value +3 standard deviations of control serum from healthy donors.
2.5.2 IgE reactivity detected by Western blot
Both Der f 36 and hypoallergenic Der f 36 were electrophoresed on 12% SDS-PAGE and transferred onto 0.22-µm PVDF membranes (Merck Millipore, MA, USA). Membranes were blocked with 5% skimmed milk, then incubated with five representative sera from the set of Der f 36-reactive patients mentioned above and one negative control serum (1:10 dilution) at 4°C overnight. the membranes were detected with HRP-conjugated anti-human IgE. Visualization of the membranes was performed using the Tanon 5200 multi-imaging system (Tanon, Shanghai, China).
2.6 Inhibition of IgE binding to Der f 36
2.6.1 IgE competition ELISA test
IgE competition ELISA test was used to analyze the ability of hypoallergenic Der f 36 to inhibit the IgE binding to Der f 36, which indicated the decreasing degree of IgE-binding activity of the designed vaccine. The plate was coated by Der f 36 (10 µg/ml) and diluted in PBS overnight at 4°C. Four samples were selected from the 24 positive sera to conduct a mixed serum pool diluted in PBST + 1% BSA (1:10). The mixed serum was pre-incubated with gradient concentration of Der f 36 and hypoallergenic Der f 36 at 4°C overnight, respectively. Each well was blocked with 1% BSA at 37°C for 2 h, then incubated by 100 μl of pre-incubated sera at 37°C for 1 h. The chromogenic reaction was the same as above. The formula for calculating inhibition rate was (OD450 uninhibited − OD450 inhibited)/(OD450 uninhibited − OD450 control) × 100%.
2.6.2 IgG blocking of IgE-binding test
The immunogenicity of the hypoallergenic Der f 36 was evaluated, and its specific IgG was prepared by immunizing a rabbit using purified hypoallergenic Der f 36 to induce IgG (details are described in the Supporting Material). The specific IgG blocking of IgE binding to Der f 36 was also determined by ELISA. The ELISA plate wells were coated with Der f 36. After BSA incubation, 100 µl/well of 1:10 diluted rabbit serum was added and incubated for 1 h before reacting with the patients’ sera. The chromogenic reaction was the same as above.
2.7 Basophil activation test
Whole blood within donated by four volunteers who had Der f 36 specific-IgE was screened by dot blot and lysed with red blood cell lysis buffer (Fcmacs, Nanjing, China) and washed twice using PBS based on a previously published reported (40, 41). Basophils were stimulated with anti-human IgE antibody as a positive control, Der f 36, hypoallergenic Der f 36 (final concentration, 10 µg/ml), and PBS alone as a negative control at 37°C for 30 min. Then, pre-cooled PBS, including 2.5 mM EDTA, was used as ending solution, followed by centrifugation at 1,000 rpm for 5 min at 4°C, and the pellets were resuspended with 100 μl of the staining buffer containing 5 μl of FITC-conjugated anti-CD63 mAb (Biolegend, CA, USA) and 5 μl of PE-conjugated anti-CCR3 mAb (Biolegend, CA, USA) and followed by incubation on ice for 20 min. The expression of CD63 and CCR3 in basophil was evaluated by FACSCanto Plus and analyzed by FlowJo V10 software (Oregon, USA).
Inhibition of basophil activation by blocking IgG was further analyzed based on BAT. Briefly, the blocking IgG was purified from rabbit serum by Protein A affinity chromatography as described in the Supporting Material. The obtained blocking IgG (at 50 μg/ml) was mixed with Der f 36 for 30 min at 37°C, then the mixture or Der f 36 alone was added to the patients’ basophils for stimulating. Other steps were the same as described above.
2.8 PBMC cytokine expression
Fresh blood was donated from five Der f 36 allergic individuals. PBMCs were isolated by Ficoll density gradient centrifugation (Fcmacs Biotech China) and cultured with a cell count of 2 × 105 in triplicate in 96-well plates (LabServ, Thermo Fisher Scientific, MA, USA) in 200 μl of serum-free Ultra Culture Medium (Gibco, Thermo Fisher Scientific, MA, USA) and stimulated with Der f 36, hypoallergenic Der f 36 (final concentration of 10 μg/ml) for 6 days at 37°C in 5% CO2 with a humidified atmosphere. The recombinant Der f 2 or PreS carrier was used as control protein. The supernatants were collected for cytokine ELISA using an ELISA kit of IL-5, 10, 13, and IFN-γ (Thermo Fisher Scientific, MA, USA).
2.9 Statistical analysis
The continuous variable was analyzed by paired sample t-test. The Mann–Whitney test was used for unpaired non-normal data. The paired non-normal data were analyzed by Wilcoxon test. A value of p < 0.05 was considered as statistically significant. Charts were completed by GraphPad Prism version 8.0 software (GraphPad Software, Inc., San Diego, CA, USA).
3 Results
3.1 Expression and purification of Der f 36
The recombinant Der f 36 was expressed and purified by Ni-NTA resin combined with HiTrap Q HP column. The major eluted fraction at 80 –90 ml was collected (Supplementary Figure S1A). The purified Der f 36 was migrated as a single band in SDS-PAGE with a molecular weight slightly below 25 kDa (Supplementary Figure S1A). The concentration of endotoxin was 0.11 EU/ml in Der f 36. The purity of Der f 36 assessed by RP-HPLC was 91.2% (Supplementary Figure S1B).
3.2 Construction of B-cell epitope-based hypoallergenic Der f 36
3.2.1 3D modeling and evaluation of Der f 36 structure
The 3D model of hypoallergenic Der f 36 protein was built by Alphafold2 (Figure 1A). The overall quality factor evaluated by ERRAT program is 96.757 (Figure 1B). None of the amino acid residues of Der f 36 were in disallowed regions of Ramachandran plot of tertiary structure (Figure 1C).
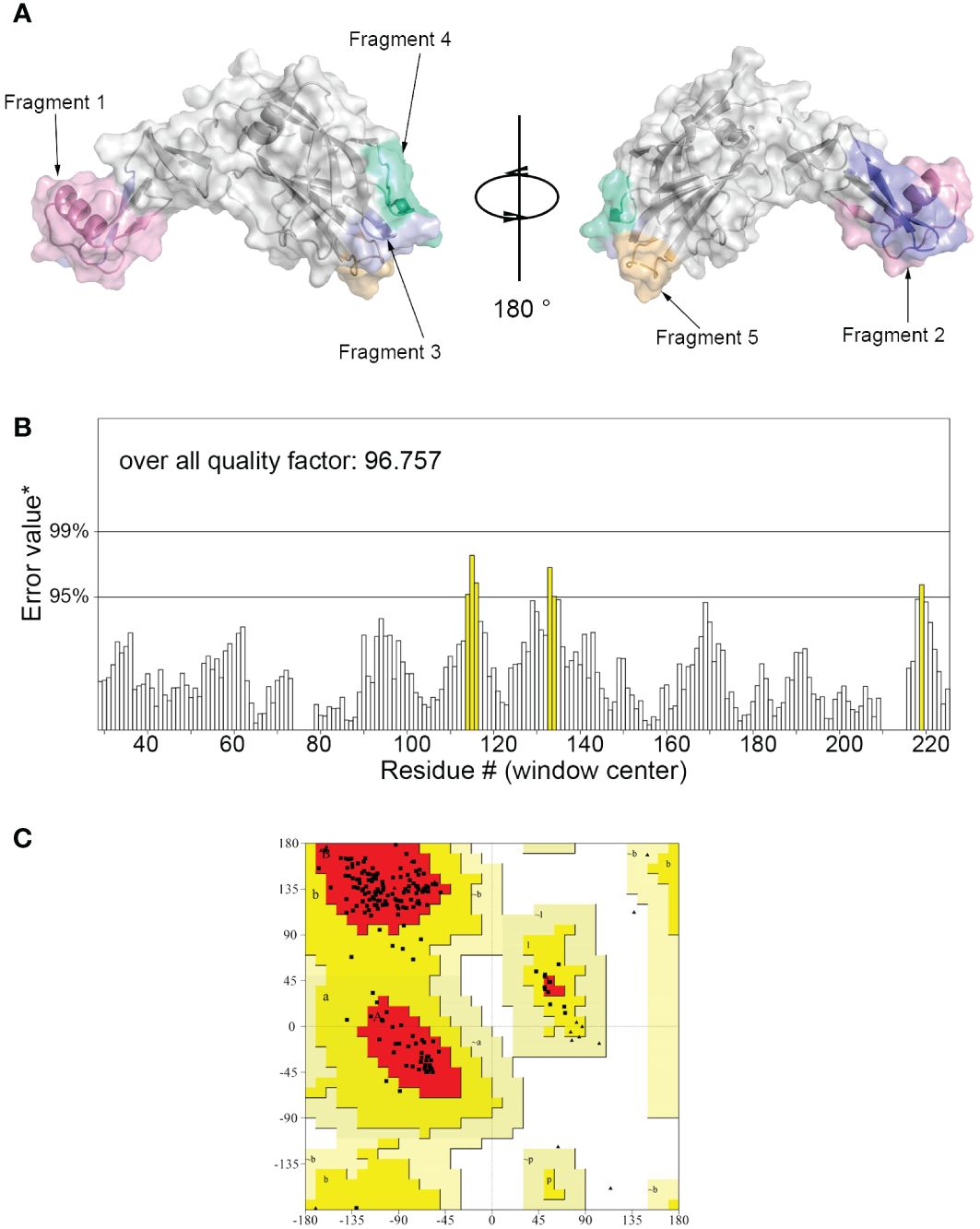
Figure 1 Three-dimensional (3D) modeling of Der f 36 and quality evaluation. (A) The 3D structure of Der f 36 allergen. The finally predicted linear B-cell epitopes are also displayed with different colors. (B) Validation of Der f 36 model by the ERRAT program (color figure online). (C) Validation of the Der f 36 model by Ramachandran plot. Residues in most favored regions (red); residues in allowed regions (yellow); residues in generally allowed regions (light yellow); residues in disallowed regions (white).
3.2.2 Computational prediction of T-cell epitopes
TepiTool was used to predict the T-cell epitope of Der f 36. The regions of final predicted T-cell epitopes were 16–30, 21–35, 27–41, 33–47, 39–53, 44–58, 50–64, 55–69, 60–74, 67–81, 72–86, 77–91, 84–98, 91–105, 100–114, 105–119, 111–125, 116–130, 121–135, 129–143, 134–148, 139–153, 144–158, 149–163, 154–168, 167–181, 172–186, 181–195, 186–200, 193–207, 200–214, 205–219, 210–224, and 215–229. A detailed information of each epitope and binding alleles are presented in Table 1.
3.2.3 Prediction of B-cell linear and conformational epitopes
We used three servers to predict the linear B-cell epitopes of Der f 36. According to the ElliPro server, seven linear epitopes were predicted as follows: residues 25–46, 92–97, 106–112, 141–164, 176–187, 198–207, and 212–218. Nine epitopes (residues 22–50, 64–70, 86–98, 107–110, 125–137, 142–151, 172–182, 196–204, and 213–221) were predicted by the Bepipred 2.0 server. Graphbepi showed eight epitopes (residues 25–48, 57–66, 68–72, 74–80, 122–135, 142–146, 177–184, and 209–216) (Table 2).
Forty-seven residues of conformational epitopes were predicted by Seppa 3.0 and SEMA. Most of them were included in the linear epitopes (Table 3). All of the linear epitopes and conformational epitopes were overlayed to get the final sequence. The final selected fragments containing B-cell epitopes without T-cell epitopes were colored in the 1D and 3D structure as follows: Fragment 1 (25–48), Fragment 2 (57–67), Fragment 3 (107–112), Fragment 4 (142–151), and Fragment 5 (176–184) (Figures 1A, 2A).
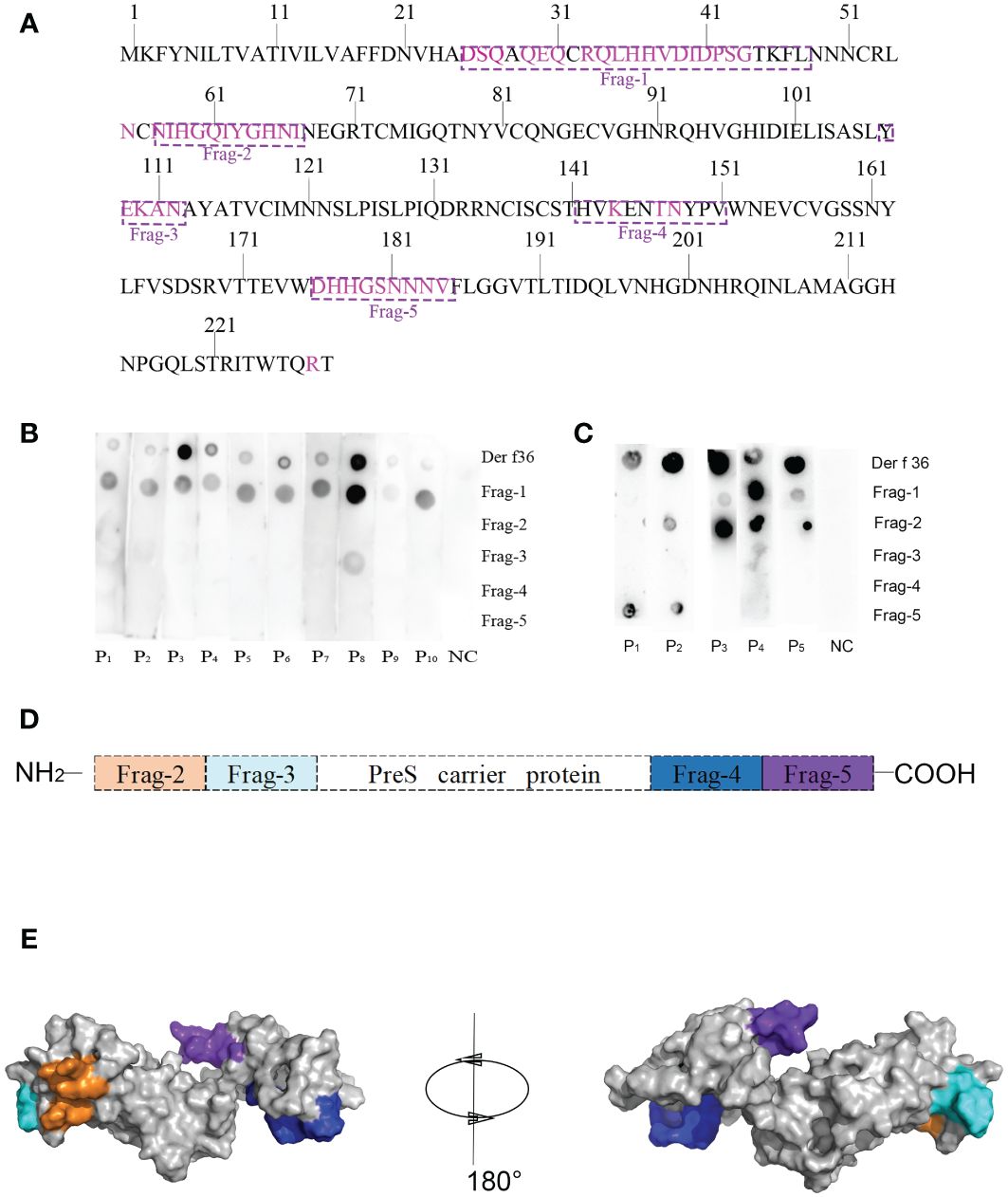
Figure 2 Design of B-cell epitope-based hypoallergenic Der f 36. (A) Distribution of B-cell epitopes in the amino acid sequence of Der f 36. The linear B-cell epitopes are boxed with dotted lines, and discontinuous B-cell epitopes are highlighted in purple. (B) Dot-blot assays were performed to detect each peptide’s IgE reactivity. (C) Dot-blot assays were performed to detect each peptide’s IgG4 reactivity. (D) Schematic representation of finally designed hypoallergenic Der f 36. Frag-2 to Frag-5 were used in hypoallergenic Der f 36 design. (E) The distribution of selected fragments on the 3D structure of the hypoallergenic Der f 36.
3.2.4 Specific IgE and IgG4 reaction of synthesized B-cell epitope
Dot-blot assays were performed to detect IgE reactivity of the synthesized B-cell epitope fragments using sera from 10 Der f 36 allergic patients and one nonallergic serum. Fragment 1 showed 10 positive IgE-binding spots, Fragment 3 only weakly reacted with serum from patient 8, while others did not exhibit IgE reactivity (Figure 2B).
The top five sera with high IgE reactivity to Der f 36 in dot blot were selected to detect IgG4 reaction, and Fragments 1, 2, and 5 showed positive binding results (Figure 2C).
3.3 Design of hypoallergenic B-cell epitope-based Der f 36
To construct a B-cell epitope-based hypoallergenic Der f 36 vaccine, Fragment 1 with strong positive IgE reaction was deleted, and the other fragments (Fragments 2–5) were linked to the PreS carrier by the KK linker (Figures 2D, E). The ProtParam results showed that the vaccine has 217 amino acid residues with a molecular weight of 23 kDa and theoretical pI of 9.94. The total number of negatively charged residues (Asp + Glu) and positively charged residues (Arg + Lys) were 11 and 20, respectively. The grand average of hydropathicity (GRAVY) was −0.777.
3.4 Expression and purification of B-cell epitope-based hypoallergenic Der f 36
The majority of the target proteins were located in inclusion bodies (Figures 3A, B, lane 4). The targeted protein was purified by nickel affinity chromatography under denaturing conditions containing 250 mM of imidazole (Figure 3D). The denaturant was gradually removed by dialysis, and the protein was refolded with redox refolding buffer. The folded protein was further purified by cation exchange chromatography and eluted as the main peak (Figure 3C). The molecular weight of the hypoallergenic Der f 36 was approximately 25 kDa by SDS-PAGE analysis. It was also verified using anti-6×His-tag antibodies by Western blot (Figures 3B, E). The concentration of endotoxin was 0.05 EU/mL in hypoallergenic Der f 36. The purity of hypoallergenic Der f 36 was 90.6% assessed using RP-HPLC (Supplementary Figure S2).
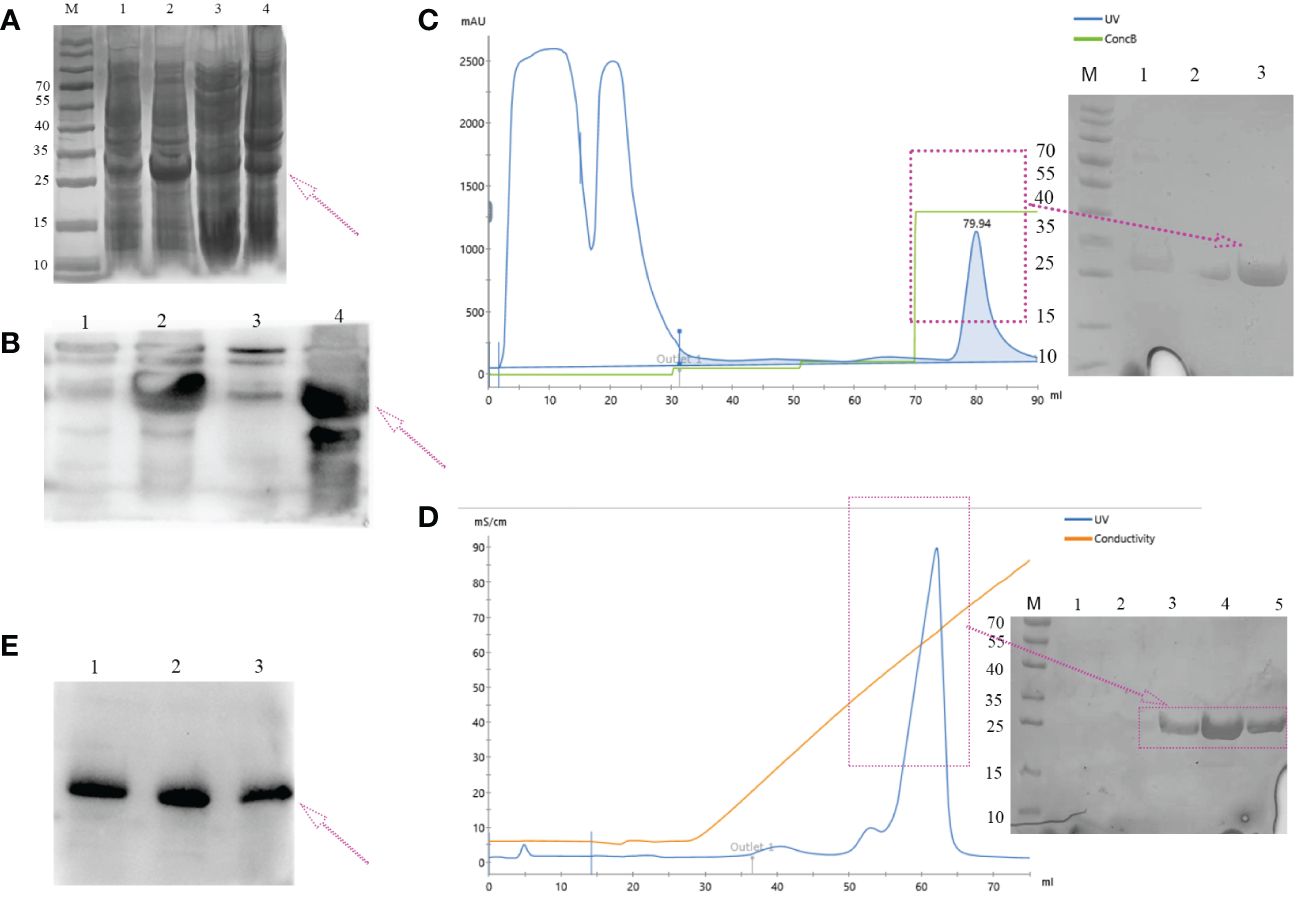
Figure 3 Expression and purification of hypoallergenic Der f 36 in (E) coli. (A) The recombinant protein resolved on 12% SDS-PAGE gel was visualized with Coomassie Blue G-250. Lane M, standard marker; lanes 1, 2 represent lysates of whole cells induced by 0 mM, 1 mM of IPTG, respectively; lane 3, the supernatant of cell lysate after ultrasonication; lane 4, the precipitants (inclusion bodies) of cell lysate after ultrasonication. (B) Non-induced (lane 1), induced (lane 2), supernatant (lane 3), and precipitated samples (lane 4) were verified by WB using anti-6*His antibodies. (C) Elution profile of hypoallergenic Der f 36 inclusion bodies on HisTrap™ HP affinity column. The fractions highlighted with an arrow were pooled for further purification. (D) The pooled fractions were further purified by loading onto a HiTrap SP HP column. The purified protein was highlighted with a purple box. (E) Final purified protein was verified by WB using anti-6*His antibodies.
3.5 IgE reactivity of B-cell epitope-based hypoallergenic Der f 36
ELISA and immunoblotting were performed to determine the allergenicity of the constructed vaccine. We compared the allergenicity of hypoallergenic Der f 36 with Der f 36 using 24 individual serum samples from Der f 36-allergic patients. All samples revealed a significant reduction in IgE-binding activity to hypoallergenic Der f 36 when compared to wild-type Der f 36 (4), (p < 0.001) (Figure 4A). The five representative allergic sera and one negative serum sample were analyzed using Western blot to confirm the result, in which only Der f 36 showed obvious IgE-binding bands, while no band was detected by hypoallergenic Der f 36 (Figure 4B).
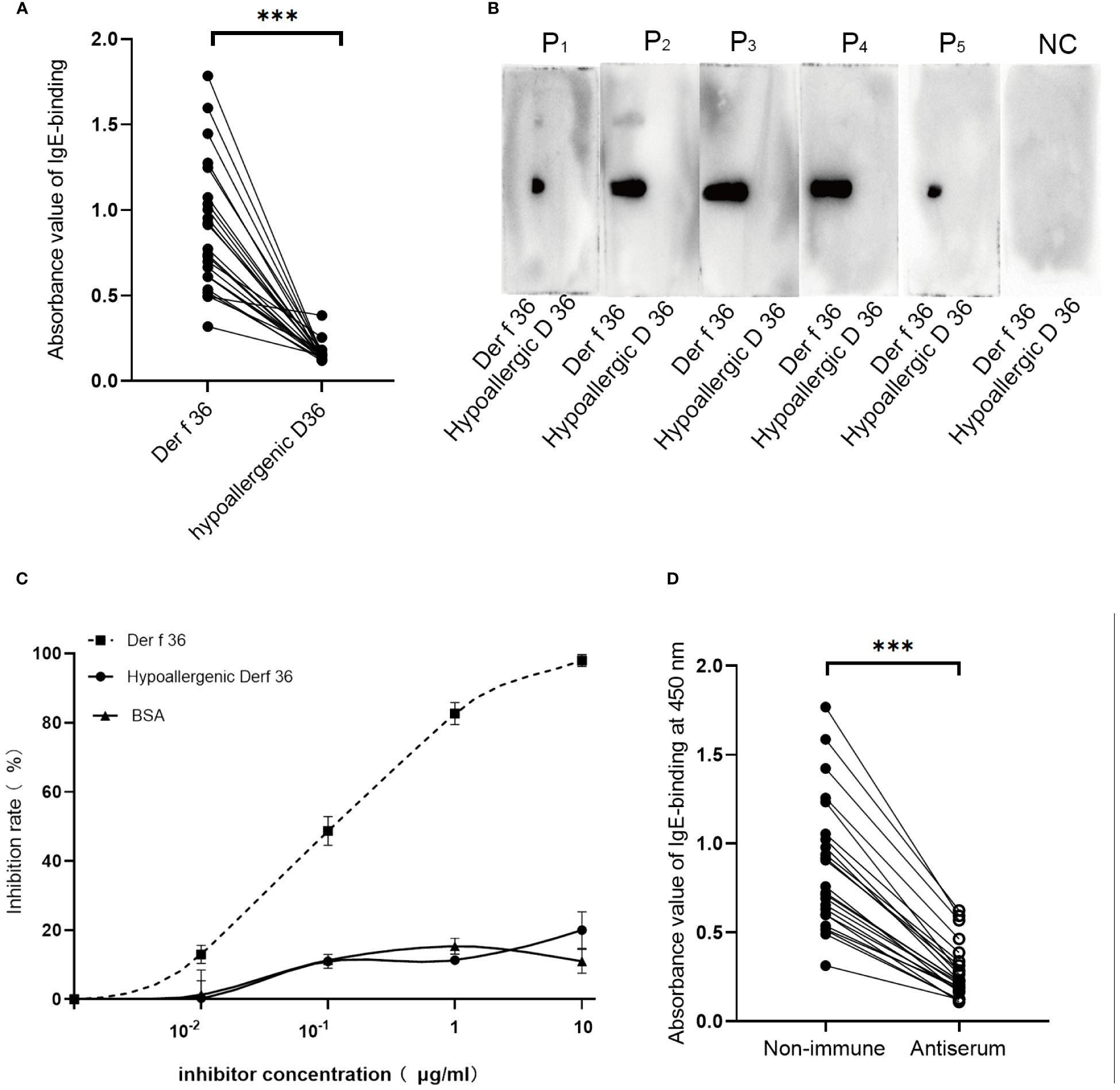
Figure 4 Sensitization and immunogenicity of hypoallergenic Der f 36. (A) Distinction of immunoreactivity to IgE between hypoallergenic Der f 36 and Der f 36 was assessed by ELISA. The result was analyzed using paired-sample t-test (***p < 0.001). (B) Confirmation of IgE reactivity by Western blot. The membranes were incubated with five positive serum samples and one negative control serum samples. (C) Competitive inhibition ELISA test with hypoallergenic Der f 36 and Der f 36. Pooled sera from four positive-reaction samples were used to conduct the inhibition ELISA with the increasing concentrations of the inhibitor. (D) Rabbit-specific IgG inhibits allergic patients’ IgE binding to Der f 36. The data were analyzed using paired-sample t-test (***p < 0.001).
3.6 IgE competition and inhibition test
A mixed serum pool of five high-positive-reaction sera was used to conduct a competition ELISA between the hypoallergenic Der f 36 and Der f 36. Only 20% of IgE binding to Der f 36 could be inhibited by hypoallergenic Der f 36 at 10 μg/ml, which is greatly reduced when compared with inhibition by Der f 36 (98%) (Figure 4C).
We then tested whether hypoallergenic Der f 36 IgG antibodies could inhibit the allergic patients’ IgE binding to Der f 36 in ELISA assays using 24 Der f 36-positive sera mentioned above. The blocking antibodies significantly inhibited the patients’ IgE binding to Der f 36 in each serum (p < 0.001) (Figure 4D). The inhibition rates of the blocking IgG were calculated between 57.0% and 81.7% (mean: 68.2%).
3.7 Basophil activation test
Basophil activation test was performed in 4 Der f 36-positive-reaction blood and a healthy donor. Proportions (%) of CD63-positive and CCR3-positive cells were detected to evaluate the basophil activation (Figure 5A). In comparison with Der f 36, the hypoallergenic Der f 36 causes less activation of basophils in the same cell sample, just as low as the negative control. The recombinant Der f 36 at 10 μg/ml induced an approximate 2.18- to 6.47-fold increase in the proportion (%) of CCR3 and CD63 double-positive cells compared with hypoallergenic Der f 36 (p < 0.05) (Figure 5B).
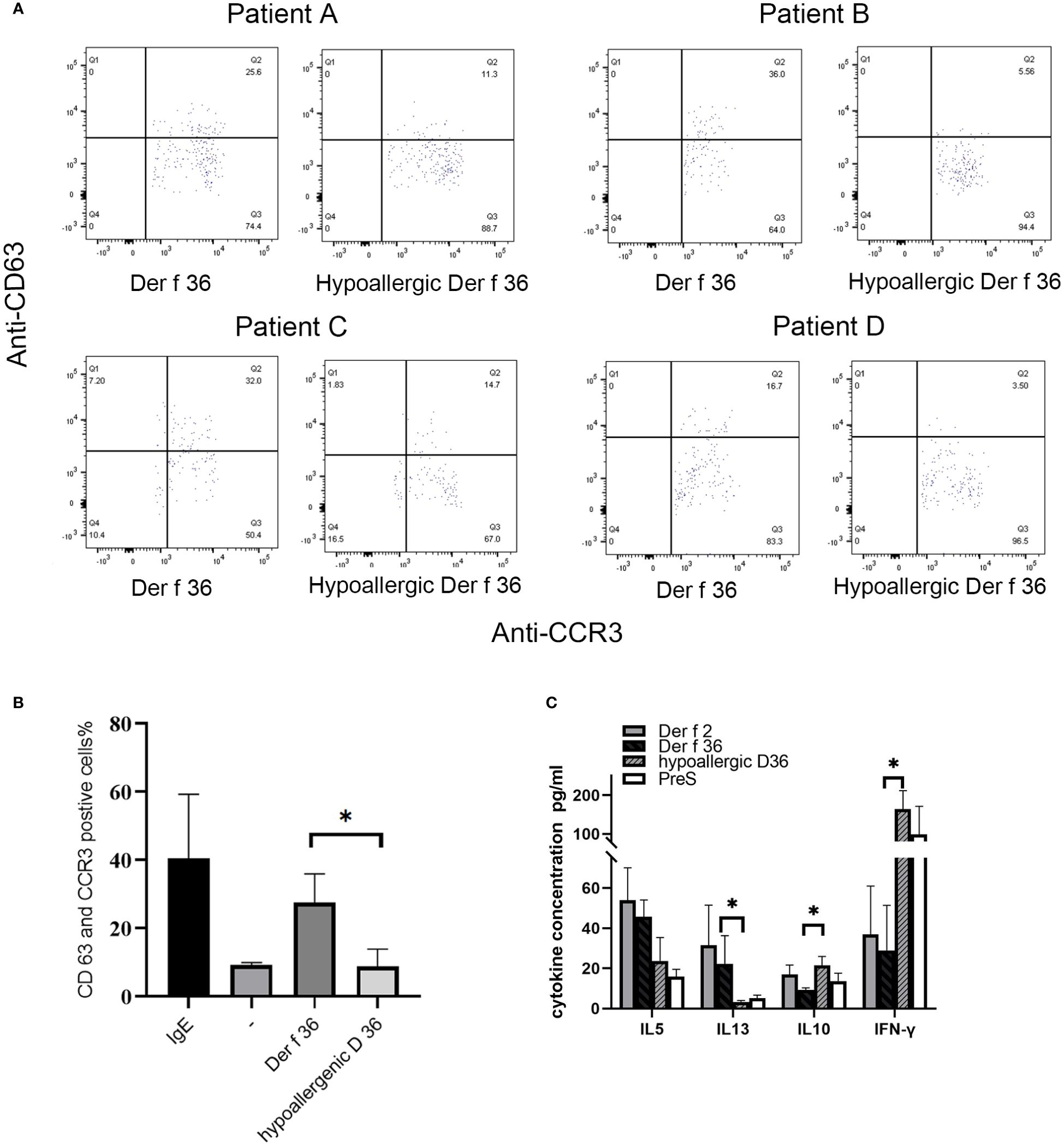
Figure 5 Induction of basophil activation by hypoallergenic Der f 36. (A) Basophil gating strategy and representative flow cytometry plots. (B) The basophils of Der f 36 allergic patients were stimulated with PBS, anti-IgE, hypoallergenic Der f 36, or Der f 36. The difference in stimulating rate between hypoallergenic Der f 36 and Der f 36 was analyzed using paired-sample t-test (*p < 0.05). (C) Comparison of PBMC cytokine expression between Der f 36 and hypo Der f 36. Stimulated with Der f 2, Der f 36, hypo Der f 36, and Pre S (final concentration of 10 μg/ml), supernatant fluids were collected for cytokine ELISA. Values were analyzed by the paired Wilcoxon test (*p < 0.05).
The ability of blocking IgG to inhibit basophil activation was analyzed to confirm the protective efficacy of hypoallergenic Der f 36. Der f 36 pre-incubated with hypoallergenic Der f 36 IgG antibodies could lead to less activated basophils (5.17%–6.25%) when compared with non-blocking Der f 36 group (14.3%–18.1%) (p < 0.01) (Figure 6).
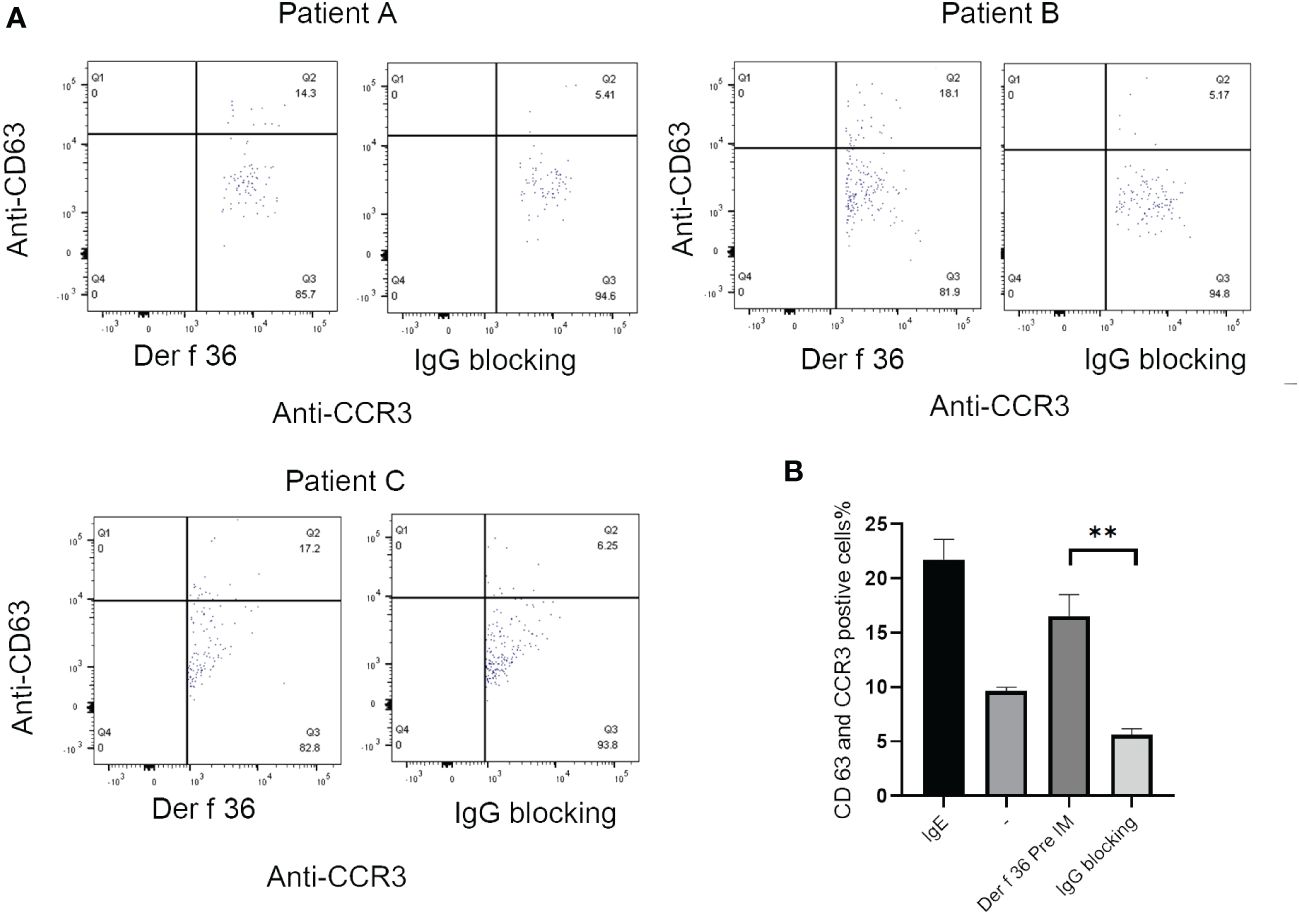
Figure 6 The inhibition of basophil activation of Der f 36 by specific IgG antibodies against hypoallergenic Der f 36. (A) The patients’ basophils pre-incubated with hypoallergenic Der f 36 IgG antibodies could lead to less activated basophils than non-blocking group. (B) The difference of stimulating rate between Der f 36 group and Der f 36 with IgG blocking group was analyzed by paired sample T-test (**P < 0.01).
3.8 Cytokine responses of PBMC
The wild-type Der f 36 induced a higher level of Th2 cytokines IL‐5 (45.65 ± 8.34 pg/ml) and IL‐13 (22.27 ± 14 pg/ml) than hypoallergenic Der f 36 (23.68 ± 11.65 pg/ml for IL-5, p = 0.08; 3.23 ± 0.75 pg/ml for IL-13, p < 0.05). The hypoallergenic Der f 36 could induce a higher level of Th1 cytokine IFN‐γ (164 ± 47.88 pg/ml) than wild-type Der f 36 (28.92 ± 22.51 pg/ml) (p < 0.05). An increasing level of regulatory cytokine IL-10 was observed with the hypoallergenic Der f 36 (21.42 ± 4.48 pg/ml) than with wild-type Der f 36 (9.31 ± 0.91 pg/ml) (p < 0.05) (Figure 5C). Concurrently, Der f 2 induced the highest level of IL-5 (53.93 ± 16.18 pg/ml) and IL-13 (31.48 ± 20.03 pg/ml), but exhibited a lower level of IFN‐γ (36.91 ± 24.09 pg/ml) and IL-10 (16.98 ± 4.65 pg/ml) when compared with the hypoallergenic Der f 36 (Figure 5C). A lower level of Th2 cytokines was induced by the PreS protein since it is a non-allergenic carrier but could provide additional T-cell help activity and thus induce a high level of IFN-γ (Figure 5C).
4 Discussion
HDM is the most common allergen for allergic diseases. AIT was an effective treatment for HDM allergy (5). However, current AIT used in clinics has disadvantages such as the quality of the allergen preparation is uncontrollable and the risk of inducing adverse reactions (7–9). Recombinant and synthetic allergen derivatives have been applied to AIT to make it more safe and convenient. Clinical immunotherapy studies showed that the molecules contained IgE-reactive epitope or T-cell epitope, which may cause IgE-mediated or T-cell epitope-mediated late-phase side effects (26, 27). To improve the vaccine’s safety, non-allergic B-cell epitope-based hypoallergenic vaccine was developed (42). This type of hypoallergen is characterized by the lack of IgE reactivity without inducing any immediate side effects but preserving the ability to produce blocking IgG antibodies upon immunization (36, 43); for example, BM32 is a grass pollen allergy B-cell epitope-carrier vaccine, which has been reported to be safe and induces beneficial clinical effects in several clinical trials (36).
AI tools have been widely used in vaccine research that can facilitate a rational design and increase efficiency (19). In this study, we first applied AI strategy to facilitate B-cell epitope screening of Der f 36. For inhaled allergens, conformational epitopes appear to be the primary targets of IgE responses (32), so we integrated the predicted linear and conformational B-cell epitopes and excluded T-cell epitopes. The allergen’s candidate B-cell epitopes were further verified by serum-specific IgE reactions, and Fragment 1 showed a strong IgE reaction, which suggested that Fragment 1 made a great contribution on the allergenicity of Der f 36. Although Fragment 1 also showed positive IgG4 response, we still exclude it in the later construction of hypoallergenic vaccine for decreasing IgE-binding activity. The final selected Fragments of Der f 36 with low or without IgE reactivity were then fused to the Pres-carrier protein with the goal to reduce allergenicity while preserving high immunogenicity.
The constructed hypoallergenic Der f 36 was expressed in E. coli and finally purified as a high-purity vaccine. The majority of the target proteins were located in inclusion bodies. Only a weak His-tag-positive band could be observed in the supernatant. We deduced that the band with the same molecular weight as the supernatant may mainly be the endogenous protein of E.coli. Inclusion bodies also have advantages, such as easier isolation, resistance to proteolytic degradation, and very high amount of overexpressed protein (44). Such properties may also be suitable for the large-scale production of vaccines. The protein was migrated at approximately 25 kDa on SDS-PAGE, which was slightly higher than the calculated result and may be due to the additional 6×His-tag at the C-terminal of the expressed protein. The 6×His-tag facilitated the subsequent purification based on its affinity to Ni2+. Moreover, the theoretical pI of the hypoallergenic Der f 36 was calculated as 9.94, which indicated that it is a basic protein and would be positively charged under neutral or acidic conditions. Such property provided the possibility for further purification of the vaccine. Moreover, amebocyte lysate analysis showed that both Der f 36 and hypoallergenic Der f 36 were almost endotoxin-free molecules according to a previous study (45), which is crucial for animal experiment and PBMC cytokine expression.
This hypoallergenic Der f 36 showed reduction in IgE reactivity compared with Der f 36, as demonstrated by ELISA and immunoblotting. Competitive-inhibition ELISA also confirmed that the hypoallergenic Der f 36 lost most of its IgE-binding ability, and only 20% inhibition could be achieved, which is greatly reduced when compared with inhibition by Der f 36 (98%). Basophil activation test showed that the stimulating ratio of basophils in hypoallergenic Der f 36 was reduced as low as that of the negative control. Allergic individuals whose sera contain specific IgE have a bias in generating T-helper 2 (Th2) cytokines (46–49). IL-5 (50, 51) and IL-13 (52) are recognized as Th2 cytokines. IL-10 (53) and IFN-γ (54) have been reported to have a “protective” role in asthma. Hypoallergenic Der f 36 induced a lower level of the Th2 cytokines IL‐5 and IL‐13 than Der f 36. This indicated that the T-cell-mediated side effects of the vaccine may be decreased even though the difference of IL-5 was not statistically different. This is also the advantages of B-cell epitope vaccines due to the elimination of T-cell epitopes (26). We found that the hypoallergenic Der f 36 could induce a higher level of Th1 cytokine IFN‐γ than the wild-type Der f 36. We think the effect was attributed by PreS carrier based on its beneficial modulation of immune responses toward a Th1 phenotype according to previous studies (27, 55). Moreover, we also observed an increasing level of regulatory cytokine IL-10 by the hypoallergenic Der f 36 than wild-type Der f 36, which may be helpful for T-cell tolerance based on the immune-suppressive functions of IL-10 (56, 57). Moreover, as the controls, Der f 2 induced the highest levels of IL-5 and IL-13. This is similar to a previous study on carrier-bound Der p 2 peptide vaccine (58). The IFN‐γ and IL-10 induced by Der f 2 in our study was lower than Der p 2 in that study, which may be due to the different extent of sensitizations to Der f 2 from the patients in different studies. The PreS carrier is a non-allergenic carrier and induced very low levels of Th2 cytokines, but could provide additional T-cell help activity that is helpful for the production of blocking antibodies against the non-allergenic peptide. These results all confirmed that our constructed vaccine has low allergenicity and would be safer during therapy. To certify whether hypoallergenic Der f 36 could induce blocking IgG to inhibit IgE-binding activity of Der f 36, we used this vaccine to immunize rabbits and induced a high titer of IgG antibodies. This hypoallergenic Der f 36-specific IgG antibodies not only inhibited allergic patients’ IgE binding to Der f 36 but also inhibited the activation of basophils stimulated by Der f 36. All the evidence indicated that hypoallergenic Der f 36 has the potential to show protective efficacy as a qualified desensitization vaccine.
Individualized therapy using recombinant allergens has become popular (59). For HDM immunotherapy, several hypoallergenic allergen derivatives have been developed with reduced IgE reactivity (27, 60, 61). The application of AI has accelerated the clinical trial process and reduced the cost and time of vaccine development (19). Der f 36 is a newly identified allergen with a unique structure type. Its IgE-positive rate cannot be ignored. Overall, the hypoallergenic Der f 36 constructed using AI strategy represents a promising molecular vaccine candidate for immunotherapy of mite-allergic patients as well as an efficient paradigm strategy for the development of allergen vaccines.
Data availability statement
The original contributions presented in the study are included in the article/Supplementary Material. Further inquiries can be directed to the corresponding authors.
Ethics statement
The studies involving humans were approved by Children’s Hospital of Nanjing Medical University. The studies were conducted in accordance with the local legislation and institutional requirements. Written informed consent for participation in this study was provided by the participants’ legal guardians/next of kin. Ethical approval was not required for the study involving animals in accordance with the local legislation and institutional requirements because it was commercially available.
Author contributions
Q-ZQ: Data curation, Formal analysis, Methodology, Project administration, Validation, Writing – original draft. JT: Data curation, Methodology, Software, Writing – original draft. C-YW: Data curation, Writing – original draft. Z-QX: Conceptualization, Investigation, Methodology, Writing – review & editing. MT: Writing – review & editing, Funding acquisition.
Funding
The author(s) declare financial support was received for the research, authorship, and/or publication of this article. This work was supported by the National Natural Science Foundation of China (82201967) and the Clinical research project of Children’s Hospital of Nanjing Medical University (LCYJZ202302).
Conflict of interest
The authors declare that the research was conducted in the absence of any commercial or financial relationships that could be construed as a potential conflict of interest.
Publisher’s note
All claims expressed in this article are solely those of the authors and do not necessarily represent those of their affiliated organizations, or those of the publisher, the editors and the reviewers. Any product that may be evaluated in this article, or claim that may be made by its manufacturer, is not guaranteed or endorsed by the publisher.
Supplementary material
The Supplementary Material for this article can be found online at: https://www.frontiersin.org/articles/10.3389/fimmu.2024.1325998/full#supplementary-material
References
1. Brożek JL, Bousquet J, Agache I, Agarwal A, Bachert C, Bosnic-Anticevich S, et al. Allergic Rhinitis and its Impact on Asthma (ARIA) guidelines-2016 revision. J Allergy Clin Immunol. (2017) 140:950–8. doi: 10.1016/j.jaci.2017.03.050
2. Aaron SD, Boulet LP, Reddel HK, Gershon AS. Underdiagnosis and overdiagnosis of asthma. Am J Respir Crit Care Med. (2018) 198:1012–20. doi: 10.1164/rccm.201804-0682CI
3. Dai L, Liu J, Zhao Q, Li M, Zhou Y, Chen Z, et al. Investigation of allergic sensitizations in children with allergic rhinitis and/or asthma. Front Pediatr. (2022) 10:842293. doi: 10.3389/fped.2022.842293
4. Yang L, Zhu R. Immunotherapy of house dust mite allergy. Hum Vaccin Immunother. (2017) 13:2390–6. doi: 10.1080/21645515.2017.1364823
5. Niederberger V, Valenta R. Molecular approaches for new vaccines against allergy. Expert Rev Vaccines. (2006) 5:103–10. doi: 10.1586/14760584.5.1.103
6. Des Roches A, Paradis L, Menardo JL, Bouges S, Daurés JP, Bousquet J. Immunotherapy with a standardized Dermatophagoides pteronyssinus extract. VI. Specific immunotherapy prevents the onset of new sensitizations in children. J Allergy Clin Immunol. (1997) 99:450–3. doi: 10.1016/s0091-6749(97)70069-1
7. Casset A, Mari A, Purohit A, Resch Y, Weghofer M, Ferrara R, et al. Varying allergen composition and content affects the in vivo allergenic activity of commercial Dermatophagoides pteronyssinus extracts. Int Arch Allergy Immunol. (2012) 159:253–62. doi: 10.1159/000337654
8. Twaroch TE, Curin M, Sterflinger K, Focke-Tejkl M, Swoboda I, Valenta R. Specific antibodies for the detection of alternaria allergens and the identification of cross-reactive antigens in other fungi. Int Arch Allergy Immunol. (2016) 170:269–78. doi: 10.1159/000449415
9. Durham SR, Shamji MH. Allergen immunotherapy: past, present and future. Nat Rev Immunol. (2023) 23:317–28. doi: 10.1038/s41577-022-00786-1
10. Valenta R, Duchene M, Vrtala S, Birkner T, Ebner C, Hirschwehr R, et al. Recombinant allergens for immunoblot diagnosis of tree-pollen allergy. J Allergy Clin Immunol. (1991) 88:889–94. doi: 10.1016/0091-6749(91)90245-J
11. Chen H, Li J, Cheng L, Gao Z, Lin X, Zhu R, et al. China consensus document on allergy diagnostics. Allergy Asthma Immunol Res. (2021) 13:177–205. doi: 10.4168/aair.2021.13.2.177
12. Sanchez-Trincado JL, Gomez-Perosanz M, Reche PA. Fundamentals and methods for T- and B-cell epitope prediction. J Immunol Res. (2017) 2017:2680160. doi: 10.1155/2017/2680160
13. Mondal B. Artificial Intelligence: State of the Art. In: Balas VE, Kumar R, Srivastava R, editors. Recent Trends and Advances in Artificial Intelligence and Internet of Things. Springer International Publishing, Cham (2020). doi: 10.1007/978-3-030-32644-9_32
14. Lundegaard C, Lund O, Nielsen M. Prediction of epitopes using neural network based methods. J Immunol Methods. (2011) 374:26–34. doi: 10.1016/j.jim.2010.10.011
15. Nanni L, Brahnam S, Lumini A. Artificial intelligence systems based on texture descriptors for vaccine development. Amino Acids. (2011) 40:443–51. doi: 10.1007/s00726-010-0654-8
16. Jabbari P, Rezaei N. Artificial intelligence and immunotherapy. Expert Rev Clin Immunol. (2019) 15:689–91. doi: 10.1080/1744666x.2019.1623670
17. Mak KK, Pichika MR. Artificial intelligence in drug development: present status and future prospects. Drug Discov Today. (2019) 24:773–80. doi: 10.1016/j.drudis.2018.11.014
18. Pappalardo F, Flower D, Russo G, Pennisi M, Motta S. Computational modelling approaches to vaccinology. Pharmacol Res. (2015) 92:40–5. doi: 10.1016/j.phrs.2014.08.006
19. Russo G, Reche P, Pennisi M, Pappalardo F. The combination of artificial intelligence and systems biology for intelligent vaccine design. Expert Opin Drug Discov. (2020) 15:1267–81. doi: 10.1080/17460441.2020.1791076
20. Thomas WR. Hierarchy and molecular properties of house dust mite allergens. Allergol Int. (2015) 64:304–11. doi: 10.1016/j.alit.2015.05.004
21. Yu P-Y, Zhu Y, Tan L-X, Xu Z-Q, Lu C, Guan X-W. Immunoinformatics construction of B cell epitope-based hypoallergenic der f 34 vaccine for immunotherapy of house dust mite allergy. Int J Pept Res Ther. (2021) 28:17. doi: 10.1007/s10989-021-10337-2
22. Bordas-Le Floch V, Le Mignon M, Bussières L, Jain K, Martelet A, Baron-Bodo V, et al. A combined transcriptome and proteome analysis extends the allergome of house dust mite Dermatophagoides species. PloS One. (2017) 12:e0185830. doi: 10.1371/journal.pone.0185830
23. Cramer P. AlphaFold2 and the future of structural biology. Nat Struct Mol Biol. (2021) 28:704–5. doi: 10.1038/s41594-021-00650-1
24. Laskowski RA, MacArthur MW, Moss DS, Thornton JM. PROCHECK: a program to check the stereochemical quality of protein structures. J Appl Crystallogr. (1993) 26(2):283–91. doi: 10.1107/S0021889892009944
25. Colovos C, Yeates TO. Verification of protein structures: patterns of nonbonded atomic interactions. Protein Sci. (1993) 2:1511–9. doi: 10.1002/pro.5560020916
26. Zieglmayer P, Focke-Tejkl M, Schmutz R, Lemell P, Zieglmayer R, Weber M, et al. Mechanisms, safety and efficacy of a B cell epitope-based vaccine for immunotherapy of grass pollen allergy. EBioMedicine. (2016) 11:43–57. doi: 10.1016/j.ebiom.2016.08.022
27. Banerjee S, Weber M, Blatt K, Swoboda I, Focke-Tejkl M, Valent P, et al. Conversion of Der p 23, a new major house dust mite allergen, into a hypoallergenic vaccine. J Immunol. (2014) 192:4867–75. doi: 10.4049/jimmunol.1400064
28. Paul S, Sidney J, Sette A, Peters B. TepiTool: A pipeline for computational prediction of T cell epitope candidates. Curr Protoc Immunol. (2016) 114:18.19.11–18.19.24. doi: 10.1002/cpim.12
29. Ponomarenko J, Bui HH, Li W, Fusseder N, Bourne PE, Sette A, et al. ElliPro: a new structure-based tool for the prediction of antibody epitopes. BMC Bioinf. (2008) 9:514. doi: 10.1186/1471-2105-9-514
30. Jespersen MC, Peters B, Nielsen M, Marcatili P. BepiPred-2.0: improving sequence-based B-cell epitope prediction using conformational epitopes. Nucleic Acids Res. (2017) 45:W24–w29. doi: 10.1093/nar/gkx346
31. Zeng Y, Wei Z, Yuan Q, Chen S, Yu W, Lu Y, et al. Identifying B-cell epitopes using AlphaFold2 predicted structures and pretrained language model. Bioinformatics. (2023) 39. doi: 10.1093/bioinformatics/btad187
32. Pomés A. Relevant B cell epitopes in allergic disease. Int Arch Allergy Immunol. (2010) 152:1–11. doi: 10.1159/000260078
33. Zhou C, Chen Z, Zhang L, Yan D, Mao T, Tang K, et al. SEPPA 3.0-enhanced spatial epitope prediction enabling glycoprotein antigens. Nucleic Acids Res. (2019) 47:W388–w394. doi: 10.1093/nar/gkz413
34. Shashkova TI, Umerenkov D, Salnikov M, Strashnov PV, Konstantinova AV, Lebed I, et al. SEMA: Antigen B-cell conformational epitope prediction using deep transfer learning. Front Immunol. (2022) 13:960985. doi: 10.3389/fimmu.2022.960985
35. Gattinger P, Kratzer B, Tulaeva I, Niespodziana K, Ohradanova-Repic A, Gebetsberger L, et al. Vaccine based on folded receptor binding domain-PreS fusion protein with potential to induce sterilizing immunity to SARS-CoV-2 variants. Allergy. (2022) 77:2431–45. doi: 10.1111/all.15305
36. Rauber MM, Möbs C, Campana R, Henning R, Schulze-Dasbeck M, Greene B, et al. Allergen immunotherapy with the hypoallergenic B-cell epitope-based vaccine BM32 modifies IL-10- and IL-5-secreting T cells. Allergy. (2020) 75:450–3. doi: 10.1111/all.13996
37. Bublin M, Kostadinova M, Radauer C, Varga EM, Hafner C, Schmidthaler K, et al. Engineering of structural variants of the major peanut allergens Ara h 2 and Ara h 6 for allergen-specific immunotherapy. J Allergy Clin Immunol. (2019) 143:1226–1229.e1210. doi: 10.1016/j.jaci.2018.10.039
38. Ayyagari VS, CV T, AP K, Srirama K. Design of a multi-epitope-based vaccine targeting M-protein of SARS-CoV2: an immunoinformatics approach. J Biomol Struct Dyn. (2022) 40:2963–77. doi: 10.1080/07391102.2020.1850357
39. Sarkar B, Ullah MA, Johora FT, Taniya MA, Araf Y. Immunoinformatics-guided designing of epitope-based subunit vaccines against the SARS Coronavirus-2 (SARS-CoV-2). Immunobiology. (2020) 225:151955. doi: 10.1016/j.imbio.2020.151955
40. Yang YS, Xu ZQ, Zhu W, Zhu DX, Jiao YX, Zhang LS, et al. Molecular and immunochemical characterization of profilin as major allergen from Platanus acerifolia pollen. Int Immunopharmacol. (2022) 106:108601. doi: 10.1016/j.intimp.2022.108601
41. Jiao YX, Song LB, Xu ZQ, Zhu DX, Yang YS, Tian M, et al. Purification and characterization of enolase as a novel allergen in Platanus acerifolia pollen. Int Immunopharmacol. (2022) 113:109313. doi: 10.1016/j.intimp.2022.109313
42. Tulaeva I, Kratzer B, Campana R, Curin M, van Hage M, Karsonova A, et al. Preventive allergen-specific vaccination against allergy: mission possible? Front Immunol. (2020) 11:1368. doi: 10.3389/fimmu.2020.01368
43. Weber M, Niespodziana K, Linhart B, Neubauer A, Huber H, Henning R, et al. Comparison of the immunogenicity of BM32, a recombinant hypoallergenic B cell epitope-based grass pollen allergy vaccine with allergen extract-based vaccines. J Allergy Clin Immunol. (2017) 140:1433–1436.e1436. doi: 10.1016/j.jaci.2017.03.048
44. Rinas U, Garcia-Fruitós E, Corchero JL, Vázquez E, Seras-Franzoso J, Villaverde A. Bacterial inclusion bodies: discovering their better half. Trends Biochem Sci. (2017) 42:726–37. doi: 10.1016/j.tibs.2017.01.005
45. Velickovic TC, Thunberg S, Polovic N, Neimert-Andersson T, Grönlund H, van Hage M, et al. Low levels of endotoxin enhance allergen-stimulated proliferation and reduce the threshold for activation in human peripheral blood cells. Int Arch Allergy Immunol. (2008) 146:1–10. doi: 10.1159/000112497
46. Kapsenberg ML, Wierenga EA, Bos JD, Jansen HM. Functional subsets of allergen-reactive human CD4+ T cells. Immunol Today. (1991) 12:392–5. doi: 10.1016/0167-5699(91)90137-I
47. Mosmann TR, Sad S. The expanding universe of T-cell subsets: Th1, Th2 and more. Immunol Today. (1996) 17:138–46. doi: 10.1016/0167-5699(96)80606-2
48. Wambre E, James EA, Kwok WW. Characterization of CD4+ T cell subsets in allergy. Curr Opin Immunol. (2012) 24:700–6. doi: 10.1016/j.coi.2012.07.009
49. Scanlon ST, McKenzie AN. Type 2 innate lymphoid cells: new players in asthma and allergy. Curr Opin Immunol. (2012) 24:707–12. doi: 10.1016/j.coi.2012.08.009
50. Till S, Dickason R, Huston D, Humbert M, Robinson D, Larché M, et al. IL-5 secretion by allergen-stimulated CD4+ T cells in primary culture: relationship to expression of allergic disease. J Allergy Clin Immunol. (1997) 99:563–9. doi: 10.1016/S0091-6749(97)70085-X
51. Hales BJ, Shen H, Thomas WR. Cytokine responses to Der p 1 and Der p 7: house dust mite allergens with different IgE-binding activities. Clin Exp Allergy. (2000) 30:934–43. doi: 10.1046/j.1365-2222.2000.00901.x
52. Woodfolk JA. T-cell responses to allergens. J Allergy Clin Immunol. (2007) 119:280–94. doi: 10.1016/j.jaci.2006.11.008
53. Kearley J, Barker JE, Robinson DS, Lloyd CM. Resolution of airway inflammation and hyperreactivity after in vivo transfer of CD4+CD25+ regulatory T cells is interleukin 10 dependent. J Exp Med. (2005) 202:1539–47. doi: 10.1084/jem.20051166
54. Iwamoto I, Nakajima H, Endo H, Yoshida S. Interferon gamma regulates antigen-induced eosinophil recruitment into the mouse airways by inhibiting the infiltration of CD4+ T cells. J Exp Med. (1993) 177:573–6. doi: 10.1084/jem.177.2.573
55. Marth K, Breyer I, Focke-Tejkl M, Blatt K, Shamji MH, Layhadi J, et al. A nonallergenic birch pollen allergy vaccine consisting of hepatitis PreS-fused Bet v 1 peptides focuses blocking IgG toward IgE epitopes and shifts immune responses to a tolerogenic and Th1 phenotype. J Immunol. (2013) 190:3068–78. doi: 10.4049/jimmunol.1202441
56. Akdis CA, Akdis M. Mechanisms of immune tolerance to allergens: role of IL-10 and Tregs. J Clin Invest. (2014) 124:4678–80. doi: 10.1172/jci78891
57. Coomes SM, Kannan Y, Pelly VS, Entwistle LJ, Guidi R, Perez-Lloret J, et al. CD4(+) Th2 cells are directly regulated by IL-10 during allergic airway inflammation. Mucosal Immunol. (2017) 10:150–61. doi: 10.1038/mi.2016.47
58. Chen KW, Focke-Tejkl M, Blatt K, Kneidinger M, Gieras A, Dall’Antonia F, et al. Carrier-bound nonallergenic Der p 2 peptides induce IgG antibodies blocking allergen-induced basophil activation in allergic patients. Allergy. (2012) 67:609–21. doi: 10.1111/j.1398-9995.2012.02794.x
59. Jutel M, Kosowska A, Smolinska S. Allergen immunotherapy: past, present, and future. Allergy Asthma Immunol Res. (2016) 8:191–7. doi: 10.4168/aair.2016.8.3.191
60. Boonpiyathad T, van de Veen W, Wirz O, Sokolowska M, Rückert B, Tan G, et al. Role of Der p 1-specific B cells in immune tolerance during 2 years of house dust mite-specific immunotherapy. J Allergy Clin Immunol. (2019) 143:1077–1086.e1010. doi: 10.1016/j.jaci.2018.10.061
Keywords: house dust mite, hypoallergenic vaccine, Der f 36, artificial intelligence, B cell epitopes
Citation: Qin Q-Z, Tang J, Wang C-Y, Xu Z-Q and Tian M (2024) Construction by artificial intelligence and immunovalidation of hypoallergenic mite allergen Der f 36 vaccine. Front. Immunol. 15:1325998. doi: 10.3389/fimmu.2024.1325998
Received: 22 October 2023; Accepted: 12 March 2024;
Published: 27 March 2024.
Edited by:
Alain Jacquet, Chulalongkorn University, ThailandReviewed by:
Alexis Labrada, National Center of Bioproducts (BIOCEN), CubaEduardo Santos Da Silva, Federal University of Bahia (UFBA), Brazil
Copyright © 2024 Qin, Tang, Wang, Xu and Tian. This is an open-access article distributed under the terms of the Creative Commons Attribution License (CC BY). The use, distribution or reproduction in other forums is permitted, provided the original author(s) and the copyright owner(s) are credited and that the original publication in this journal is cited, in accordance with accepted academic practice. No use, distribution or reproduction is permitted which does not comply with these terms.
*Correspondence: Man Tian, tmsweet@163.com; Zhi-Qiang Xu, xu.zhiqiang@outlook.com
†These authors have contributed equally to this work