- 1Department of Entomology, University of California, Riverside, CA, United States
- 2Department of Botany & Plant Sciences, University of California, Riverside, CA, United States
- 3Center for Invasive Species Research, University of California, Riverside, CA, United States
Lycorma delicatula (Hemiptera: Fulgoridae), the spotted lanternfly, native to China, invaded and established in the northeast U.S. in 2014. Since this time, populations have grown and spread rapidly, and invasion bridgeheads have been detected in mid-western states (i.e., Indiana in 2021). This invasive pest presents a significant threat to Californian agriculture. Therefore, a proactive classical biological control program using Anastatus orientalis (Hymenoptera: Eupelmidae), a L. delicatula egg parasitoid native to China, was initiated in anticipation of eventual establishment of L. delicatula in California. In support of this proactive approach, the potential host range of A. orientalis was investigated. Eggs of 34 insect species either native or non-native to the southwestern U.S. were assessed for suitability for parasitism and development of A. orientalis. Of the native species tested, 10, 13, and one were Hemiptera, Lepidoptera, and Mantodea, respectively. Of the non-native species, eight Hemiptera and two Lepidoptera were evaluated. Host range tests conducted in a quarantine facility, exposed individually mated A. orientalis females (Haplotype C) to non-target and target (i.e., L. delicatula) eggs in sequential no-choice and static choice experiments to determine suitability for parasitization and development. Additionally, the sex ratio, fertility, and size of offspring obtained from non-target and target eggs were evaluated. Results of host range testing indicated that A. orientalis is likely polyphagous and can successfully parasitize and develop in host species belonging to at least two different orders (i.e., Hemiptera, Lepidoptera) and seven families (Coreidae, Erebidae, Fulgoridae, Lasiocampidae, Pentatomidae, Saturniidae and Sphingidae). Prospects for use of A. orientalis as a classical biological control agent of L. delicatula in the southwestern U.S. are discussed.
1 Introduction
Classical or introduction biological control is the intentional importation, release, and establishment of natural enemies for suppressing damaging populations of invasive non-native organisms to densities that no longer cause economic or ecological harm. This approach aims to reduce pest population densities by re-associating safe (i.e., host-specific) and efficacious natural enemies with the target pest (1). Host range and host specificity testing are important primary steps in identifying natural enemy species that may have deleterious impacts on pest populations while presenting minimal risk to non-target species (1). Host use evaluation studies are mandatory in the United States of America (U.S.) and provide safety data for review by Federal agencies (i.e., United States Department of Agriculture, Animal and Plant Health Inspection Service [USDA APHIS]), that regulate the importation and release of natural enemies for use in classical biological control programs (2). Host range and host specificity testing evaluations are time consuming, often taking years to complete (3). During this time, newly established invasive pest populations tend to increase in density and spread as management plans are slowly developed and implemented. Proactive biological control attempts to reduce or eliminate this window of opportunity for an invasive pest by evaluating candidate natural enemies for potential use in a classical biological control program in advance of the anticipated incursion and establishment of the target pest in the area of concern (3).
Spotted lanternfly, Lycorma delicatula (White) (Hemiptera: Fulgoridae), native to China (4), was detected for the first time in the U.S. in Berks County Pennsylvania, in September 2014 (5). By November 2022, L. delicatula infestations were confirmed in an additional 13 states in eastern and mid-western areas of the U.S. (Connecticut, Delaware, Indiana, Maryland, Massachusetts, Michigan, New Jersey, New York, North Carolina, Ohio, Rhode Island, Virginia, and West Virginia) (6). Lycorma delicatula is a phloem-feeding fulgorid that has a broad host range (7). Direct feeding damage can cause mortality to highly preferred hosts like Ailanthus altissima (Miller) (Sapindales: Simaroubaceae) and grapevines (Vitis vinifera L. [Vitales: Vitaceae]). Indirect damage results from the excretion of high quantities of honeydew that promote sooty mold growth (7, 8). Lycorma delicatula has been recorded infesting forest and ornamental shade tree species in natural and urban areas, respectively (7, 9), and presents a significant risk to economically important perennial agricultural crops (e.g., grapes and nuts) (10, 11). Long-distance dispersal by L. delicatula is almost entirely human-assisted. This occurs primarily through the accidental translocation of egg masses that are often laid indiscriminately on inert substrates (e.g., wooden pallets and railcars) that undergo subsequent transportation into uninfested areas (12, 13). This type of inadvertent relocation in the U.S. likely resulted in the establishment of invasion bridgeheads in Indiana (2021) and Michigan and North Carolina (2022) (6). Ecological niche models indicate that L. delicatula has a potential distribution that includes large areas of the west coast of the U.S., and other parts of the world (e.g., Europe) (14). For California, a western U.S. state, with an agricultural economy worth ~$50 billion per year (15), L. delicatula is viewed as a significant invasion threat that could cause major problems for producers of specialty crops, like grapes and nuts, which are multi-billion-dollar industries (15).
The egg parasitoid, Anastatus orientalis Yang and Choi (Hymenoptera: Eupelmidae), was found parasitizing L. delicatula eggs in northern China in 2011during foreign exploration for natural enemies for use in South Korea, where L. delicatula is also invasive (16, 17). Following the invasion and spread of L. delicatula on the east coast of the U.S. there was renewed interest in the potential use of A. orientalis as a classical biological control agent (18, 19). Molecular analyses identified six different haplotypes of A. orientalis collected from the native range. Importations of A. orientalis into the U.S. were initially comprised of Haplotype C, which was first evaluated as a classical biological control agent against L. delicatula (20). The majority of Anastatus spp. Motschulsky are primary endoparasitoids attacking eggs of Diptera, Dictyoptera, Coleoptera, Hemiptera, Lepidoptera, Orthoptera, and Mantodea (21–27). Numerous Anastatus species have been considered or used as classical biological control agents against various pests around the world. For example, A. japonicus Ashmead was released in the eastern U.S. for control of Lymantria dispar L. (Lepidoptera: Erebidae) (27, 28) and against Tessaratoma papillosa Drury (Hemiptera: Pentatomidae) in China (29, 30). Anastatus sp. was released in Nepal to control Rhynchocoris humeralis (Thunberg) (Hemiptera: Pentatomidae) (31). Anastatus bifasciatus (Geoffroy) was evaluated to study levels of biotic resistance of central European natural enemies against invasive Halyomorpha halys Stål (Hemiptera: Pentatomidae) populations (32, 33). In the eastern U.S., A. reduvii, a native species, has been detected as one of the most common parasitoids emerging from eggs of invasive H. halys (34). Some Anastatus spp. are commercially-available and used for augmentative biological control of Amblypelta nitida Stål and A. lutescens lutescens Distant (Hemiptera: Coreidae) in Australia (35, 36).
Given the obvious threat posed to California agriculture by the westward migration of L. delicatula in the U.S., a proactive biological control program was initiated. Proactive research efforts focused on the suitability of A. orientalis (Haplotype C) as a potential classical biological control agent of L. delicatula in advance of its expected establishment in California (3). Consequently, the objective of this study was to investigate the physiological host range of A. orientalis on native and non-native non-target species from the southwestern U.S. (i.e., California and Arizona) to determine whether or not this natural enemy would be a suitable candidate to release for classical biological control of L. delicatula should it eventually establish in California. The results of these studies are presented here.
2 Materials and methods
2.1 Source and collection of test insects
Anastatus orientalis Haplotype C specimens were obtained from colonies established at USDA APHIS PPQ (Plant Protection and Quarantine) Forest Pest Methods Laboratory in Massachusetts, U.S. Initial A. orientalis populations were shipped to the University of California Riverside Insectary and Quarantine Facility (UCR-IQF) as parasitized L. delicatula egg masses under USDA-APHIS permit P526P-22-03022 and P526P-22-04208 and California Department of Food and Agriculture (CDFA) permit 3888. Colonies of A. orientalis were established in UCR-IQF in October 2019 and reared continuously on cold stored L. delicatula egg masses.
Lycorma delicatula egg masses were field collected in winter (December to March) of 2019 to 2022. Collections were made in Pennsylvania, U.S. (Berks, Dauphin, Huntingdon, Lancaster and Lebanon Counties) predominantly from A. altissima (>90%). Entire egg masses attached to underlying bark were removed using chisels and shipped or hand carried to the UCR-IQF under USDA-APHIS permit P526P-19-02058 and CDFA Permit 3458. In quarantine, all field collected egg masses were stored at 5°C and 60-75% R.H. Egg masses were randomly selected and used for experiments reported here.
Selection of non-target species for host range testing was made based on phylogenetic relationships amongst species within the family Fulgoridae and their representation in the southwestern U.S. Five native fulgorid genera in California and Arizona, Amcyle spp., Cyrpoptus spp., Poblicia spp., Scaralina spp. (described incorrectly as genus Alphina spp Stål, Yanega et al. unpublished), and Scolopsella spp (37). were targeted for field collections and use in host range tests. Previous host range studies on Anastatus suggested that species may potentially have broad host ranges and are capable of utilizing hosts from different orders (32). Consequently, to determine if A. orientalis potentially exhibits oligophagy or polyphagy, additional non-target species belonging to Hemiptera (Cicadellidae, Coreidae, Liviidae, Pentatomidae, Reduviidae, and Rhopalidae), Lepidoptera (Erebidae, Lasiocampidae, Saturniidae, and Sphingidae), and Mantodea (Mantidae) were included in host range testing for A. orientalis (Table 1). These families were also selected to compliment simultaneous testing conducted by collaborators at the USDA APHIS PPQ Forest Pest Methods Laboratory of potential native and non-native non-target species found in the eastern U.S. All non-target insect colonies used for host range testing, unless otherwise stated, were maintained on each test species preferred host plant species held in cages (BugDorm-2120 61×61×61 cm, MegaView Science Co. Ltd., Taiwan) at the UCR-IQF at 25°C, 65%RH, L:D 16:8. Colonies were checked daily for egg masses which were harvested and used immediately or held at 10°C until used for experiments.
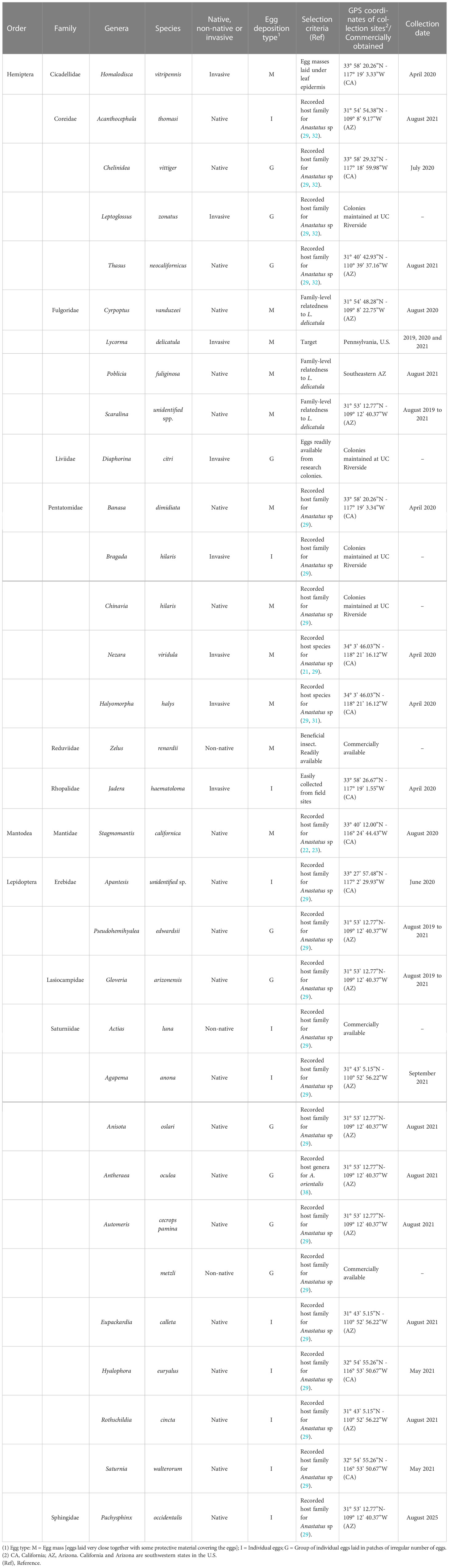
Table 1 Non-target species tested, selection criteria for use in evaluations, and collection information.
2.2.1 Hemiptera
2.2.1.1 Fulgoridae collections
Fulgorids collected in the Chiricahua mountains near Portal, Santa Cruz County, in southeastern Arizona included Scaralina spp. (comprised of three undescribed species and incorrectly placed as Alphina genera, Yanega et al. unpublished) and Cyrpoptus vanduzeei Ball (Table 1). Adult Scaralina spp. were hand collected as they were attracted to mercury vapor and UV lights. Immediately after capture, adult males and females were caged (i.e., sleeve cages made of mesh with fiber spacing of 160 µm (Figure 1) on trunks of oak trees, Quercus arizonica Sarg. (Fagales: Fagaceae), at the American Museum of Natural History’s Southwestern Research Station, Portal Arizona. Cages were inspected daily for oviposited egg masses which were collected and maintained at ~10°C until use in experiments with A. orientalis. Three species of Scaralina were collected and relatively low numbers of egg masses per species were obtained. Therefore, all egg masses (n = 9) used for experiments were pooled and referred to as “Scaralina spp.”. Poblicia fuliginosa (Olivier) and C. vanduzeei adults were collected during the day from Baccharis sarothroides Gray (Asterales: Asteraceae) from different locations in Arizona (Table 1). Adult P. fuliginosa and C. vanduzeei were maintained on potted B. sarothroides plants held in cages. Live insects used for experiments were moved to UCR-IQF under USDA-APHIS Permit number P526P-19-00766 and CDFA Permit number 3457.
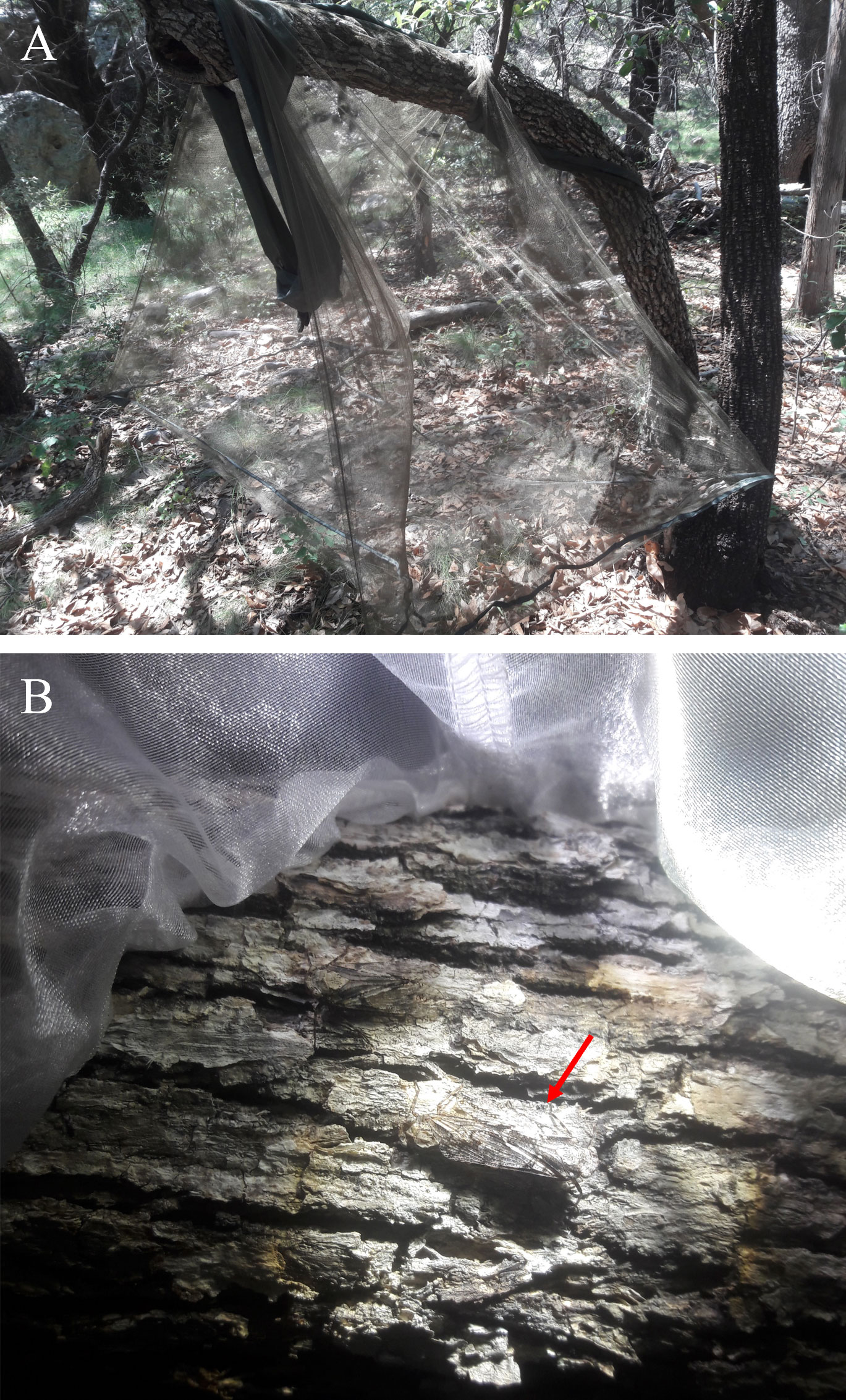
Figure 1 (A) Sleeve cages set up on branches of Quercus arizonica in Portal, AZ were used to confine adult native fulgorids on putative host plants for mating and oviposition. (B) A Scaralina sp. specimen captured at night by black lighting is seen resting on the bark of Q. arizonica branch enclosed by a sleeve cage (the red arrow indicates position of Scaralina sp.).
2.2.1.2 Cicadellidae collections
Homalodisca vitripennis Germar (Hemiptera: Cicadellidae), a pest of grapes, were collected with sweep nets in citrus orchards in Riverside, California and maintained on potted basil, Ocimum basilicum (Lamiales: Lamiaceae), a host plant that supports adult feeding and oviposition.
2.2.1.3 Coreidae collections
Acanthocephala thomasi Uhler, Chelinidea vittiger Uhler, Leptoglossus zonatus (Dallas) and Thasus neocalifornicus Brailovsky and Barrera were included in host range tests. Acanthocephala thomasi specimens were hand collected in Portal Arizona (Table 1) and maintained on potted B. sarothroides plants. Chelinidea vittiger specimens were collected in Riverside, California and maintained on Opuntia sp. (Caryophyllales: Cactaceae). Leptoglossus zonatus specimens were obtained from research colonies maintained in the Department of Entomology at UC Riverside. Thasus neocalifornicus were collected in Sonoita, Arizona and maintained on Prosopis velutina Wooton (Fabales: Fabaceae) trees (Table 1).
2.2.1.4 Liviidae collections
Diaphorina citri Kuwayama (Hemiptera: Liviidae) eggs were obtained from colonies maintained in the UCR-IQF building of the Department of Entomology at UCR. Diaphorina citri, collected in southern California in 2011 and certified free of the citrus killing bacterium Candidatus Liberibacter asiaticus (CLas), were reared on Murraya koenigii (Sapindales: Rutaceae), a non-propagative host for CLas.
2.2.1.5 Pentatomidae collections
Banasa dimidiata (Say) (native), Bragada hilaris (Burmeister) (invasive), Chinavia hilaris Say (native), Nezara viridula L. (invasive) and H. halys (invasive) were used in host range tests. Banasa dimidiata specimens were collected in Riverside, California and maintained on Hirschfeldia incana (Brassicales: Brassicaceae) (Table 1). Bragada hilaris and Chinavia hilaris were obtained from research colonies maintained in the Department of Entomology at UCR. Halyomorpha halys and N. viridula were established from adults collected from Hancock Park, in Los Angeles, California. All live insects were transported to UCR-IQF under USDA-APHIS Permit number P526P-22-03011 and CDFA Permit number 3887 (Table 1). Halyomorpha halys colonies were maintained on a mixed diet of avocados, carrots, apples, green beans, table grapes and A. altissima. Nezara viridula colonies were maintained on green bean plants and raw peanuts.
2.2.1.6 Reduviidae collections
Egg masses of Zelus renardii Kolenati were purchased from Arbico Organics (Oro Valley, Arizona). Purchased eggs were approximately 2 days of age upon receipt. Egg masses were exposed to A. orientalis immediately.
2.2.1.7 Rhopalidae collections
Jadera haematoloma Herrich-Schäffer adults were collected in the Botanic Gardens at the University of California Riverside campus, Riverside, California. Specimens were not feed, adults were kept on ventilated plastic containers, provided with a water-saturated cotton wick, and eggs were collected daily and exposed immediately to A. orientalis.
2.2.2 Lepidoptera
All Lepidoptera (Erebidae, Lasiocampidae, Saturniidae, and Sphingidae) species, used in host range testing (except for Automeris metzli Sallé which were purchased from an online vendor as pupae) were field collected as adults (Table 1). Automeris metzli pupae were held at 10°C for two months to simulate exposure to winter temperatures. After this chilling period, pupae were moved to a temperature cabinet set at 25 ± 2°C and 60% R.H. until adults emerged. Field captured adult moths were kept in bug-dorms (BugDorm-2120 61×61×61 cm, MegaView Science Co. Ltd., Taiwan) and maintained outdoors near collection sites or in a temperature chamber (25 ± 2°C; 60% of R.H.) when moved into UCR-IQF for mating and oviposition. Adults were not provided with food or water as test species do not feed in adult stage (except Apantesis sp. which was provided 50% honey water solution). Eggs oviposited onto walls of cages or on cardboard oviposition strips were collected daily and either used immediately or maintained at 10°C until used for experiments.
2.2.3 Mantodea
2.2.3.1 Mantidae collections
Adult female Stagmomantis californica Rehn & Hebard were collected in Riverside County, California (Table 1) and fed with H. halys nymphs and adults. Ootheca, ~48 h of age, were collected and presented to A. orientalis.
2.3 No-choice sequential host-testing
Five female A. orientalis, less than 24 hours of age, were placed in a test unit with one male and a thin smear of pure honey on the mesh of the unit’s lid as a carbohydrate source. Each experimental egg mass-parasitoid test arena was comprised of a clear plastic container 3 cm × 4 cm × 5 cm (180 mL clear RPTE hinged lid deli containers, AD16 GenPak, Charlotte, NC) with a modified lid that had a ventilated mesh window (1.5 cm x 2.5 cm) to facilitate air exchange. One L. delicatula egg mass was placed in the test unit and exposed to the five females and the male of A. orientalis for seven days. This seven day period is a pre-oviposition period during which host feeding occurs (pers. obs. F. Gomez Marco) at temperatures that simulate the fall (i.e., September when parasitoid oviposition in the field occurs) in Beijing (average daily high 25°C, average daily low 14°C, lights on 6:00 AM, lights off 6:30 PM [i.e., L:D 12.5:11.5], 75% R.H.; referred to as Beijing-fall regimen), the general area where A. orientalis was collected for colony establishment in the U.S (16–19). All experiments were conducted under the Beijing-fall regimen. After this one-week pre-oviposition period, females were moved individually and placed singly without males in new separate test units for a total of 274 A. orientalis females. One non-target host egg mass [number of eggs in the egg mass and the physical size of the egg mass varied on species being tested (Table 2)] was placed into each test unit containing a single mated female for seven days. After the seven-day exposure period, non-target eggs were removed, and replaced with L. delicatula egg masses, and females were left to host feed and oviposit for an additional seven days. Thus, the sequential non-choice tests were performed in this order; target host (pre-oviposition period, seven days) – non-target host (seven days) – target host (seven days). Parasitism of L. delicatula egg masses in the exposure trial following exposure to non-target eggs confirmed female competency if no parasitism was observed from non-target exposures. The total time taken to complete each no choice sequential host test cycle for each female was 21 days.
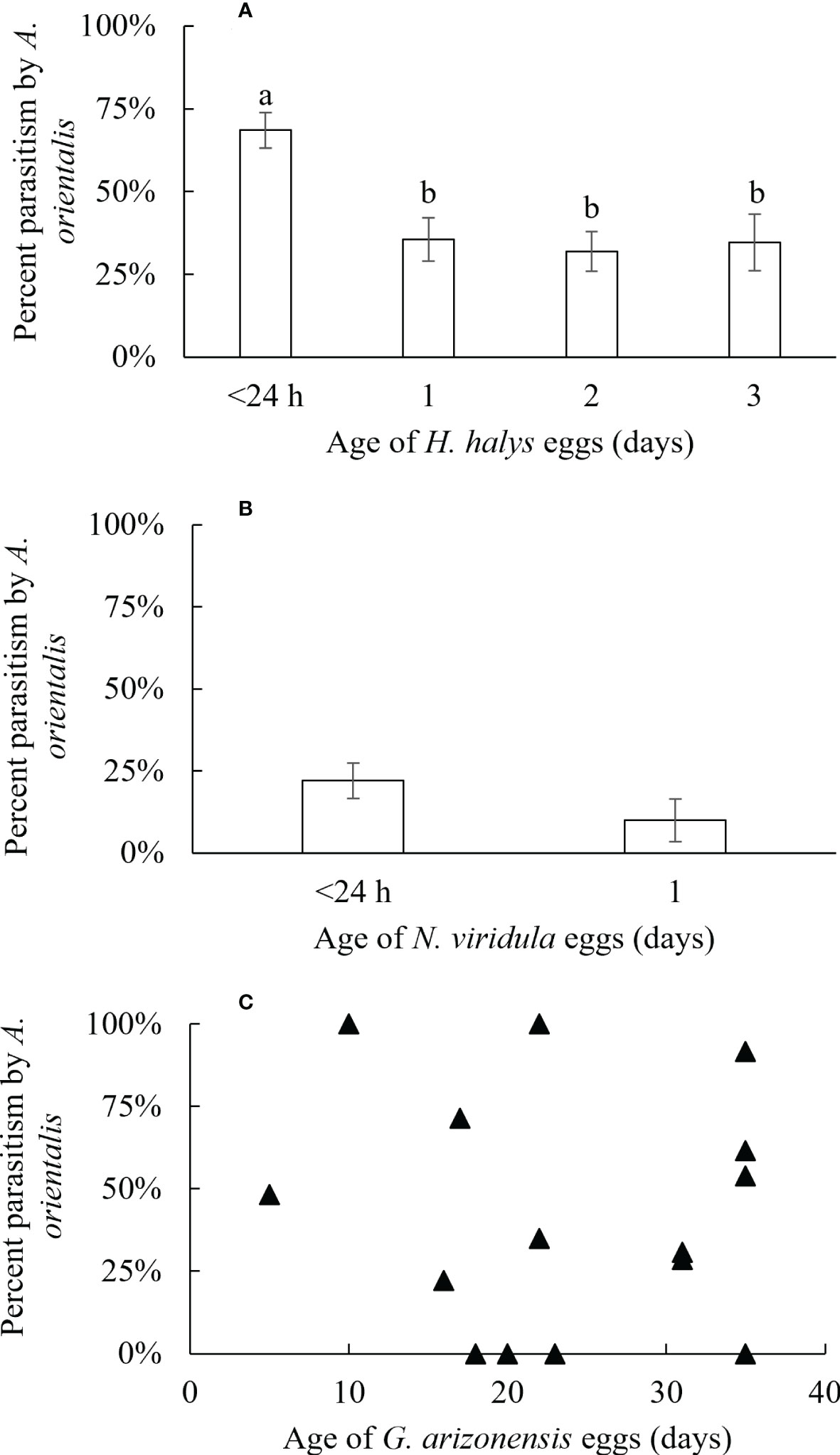
Figure 2 Percentage of parasitism (± SE) by A orientalis on non-target host eggs of (A) H. halys (<24 hours; n = 27, 1 day; n = 23; 2 days; n = 27, 3 days; n = 17), (B) N. viridula (<24 hours; n = 11, 1 day; n = 6) and (C) G. arizonensis. Different letters indicate significant differences for percent parasitism of eggs of different ages.
A variation of this experiment that reduced female exposure time to non-target egg masses from five species [C. hilaris, Gloveria arizonensis Packard, H. halys, N. viridula and Saturnia walterorum Hogue & Johnson (Table 1)] was conducted. In this set of experiments, five A. orientalis females had a seven day pre-oviposition period with a male and access to a L. delicatula egg mass. Following this seven-day exposure period, individual mated females without males were exposed to non-target eggs (Tables 1, 2) for 24 hours then moved to a target L. delicatula egg mass for 24 hours to confirm competency.
For both experimental designs, female A. orientalis, that did not produce offspring either on the non-target eggs or on the second exposure to the L. delicatula egg mass, were classified as “incompetent” and discarded from analyses. This rule was not followed for Anisota oslari Rothschild due to the high mortality (100%) of A. orientalis females following exposure to eggs of this species, and for C. vittiger and C. vanduzeei, due the low number of repetitions due to low egg availability (Table 2), which resulted from difficulty in acquiring sufficient test eggs of non-target species for experiments. Each L. delicatula egg mass (from pre-oviposition and post-non-target exposure) and non-target species eggs were held under the Beijing-fall regimen for one month, for development of parasitoid larvae. After this four-week period, eggs were transferred to 25°C, 16:8 L:D, and 75% R.H. for emergence of parasitoid offspring following previously published protocols (19). The host species from which A. orientalis emerged were recorded. Target eggs that did not produce parasitoids were dissected to detect failed parasitism (i.e., presence of dead parasitoid larvae or pupae were recorded). Percentage of parasitism was calculated as:
Mortality of non-target host species due to exposure to A. orientalis was calculated using the Henderson–Tilton formula (Equation 2) (38), which calculates percent mortality based on the initial and final insect counts in the control relative to treatments with parasitoid exposure. Rates of naturally occurring mortality for non-target species host eggs were calculated with control eggs that were held under similar ambient conditions to test eggs but were not exposed to A. orientalis. The percentage of mortality by parasitoids was calculated as:
Using percent mortality caused by parasitoids and percent parasitism, percent mortality of non-target hosts resulting from parasitoid activity, but that did not result in A. orientalis offspring (i.e., excess mortality [due to host feeding and/or oviposition attempts]) was calculated with the equation:
Percent excess mortality caused by parasitoids to non-target hosts was compared with percent mortality in controls not exposed to parasitoids when the number of successfully completed repetitions for each treatment exceeded a minimum of three.
2.4 Choice host-testing
To assess host preference on parasitization by A. orientalis when given a simultaneous choice between eggs of non-target species and L. delicatula, choice host-tests were performed with two non-target species, G. arizonensis and H. halys. Pairs of egg masses of target and non-target species were presented simultaneously to female parasitoids ~48 hours of age in exposure arenas which were constructed using two stacked transparent U-shaped acrylic risers 15cm×15cm×15cm (SW Plastics F2191, Riverside, CA), that formed a rectangular cage 15cm×15cm×30cm with two open sides. One open face was covered with white semi-opaque no-see-um netting (Skeeta, Bradenton, FL) and the other was fitted with a 30cm-long sleeve sewn from no-see-um netting). Choice tests were run either for 24 hours or seven days. Inside arenas, egg masses were separated by 26 cm and randomly placed on the floor of arenas for each repetition. After exposure time, each group of eggs (target, non-target, and control eggs not exposed to parasitoids) were isolated in ventilated clear plastic test arenas (3 cm × 4 cm × 5 cm, see section 2) and held under the Beijing-fall regimen for four weeks before being moved to 25°C until parasitoids or immature non-target species emerged from eggs, or eggs were classified as dead and dissected for evidence of parasitism when possible.
2.5 Anastatus orientalis offspring sex ratio, fertility and size when reared from target and non-target hosts
Anastatus orientalis offspring that emerged from non-target host species and target host (i.e., L. delicatula) eggs were evaluated for offspring sex ratio, fertility of males and females, and adult size. Parasitoid sex ratio was calculated as the number of female parasitoids divided by the total number of female and male parasitoids combined that emerged from each experimental egg mass. Three different offspring fertility evaluations were performed on five non-target host species: Actias luna L., Agapema anona Ottolengui, G. arizonensis, H. halys and P. fuliginosa. First, males and females emerging from the same non-target host species (< 48 hours of age) were set up in test arenas (see section 2 for details). Second, males that emerged from non-target host species were coupled with unmated A. orientalis females that emerged from L. delicatula eggs. Finally, females that emerged from non-target host species were coupled with A. orientalis males that emerged from L. delicatula egg masses. All mating couples were exposed to L. delicatula egg masses for seven days and a thin smear of pure honey on the mesh of the ventilated lid of the test arena as a carbohydrate source. After seven days, male-female pairs were removed, and each egg mass was held under the Beijing-fall regimen for four weeks then moved to 25°C for the emergence of parasitoids and non-target hosts.
To evaluate the effect of host species on the size of A. orientalis male and female parasitoids that successfully emerged from non-target and target host eggs, measurements of right hind tibia lengths were used as a proxy for parasitoid size and subsequent fitness (i.e., parasitoids with larger hind tibia are assumed to be bigger and more fit than parasitoids with smaller tibia) (39, 40). Excised right hind tibiae were placed onto glass slides and covered with a second glass slide. Hind tibia length was measured from its point of attachment on the femur to the attachment point with the tarsi using a Leica S8AP0 microscope. Slide mounted hind tibiae were photographed at a magnification of 25 × with an attached Leica DMC2900 camera and length was measured using the Leica Application Suite version 4.6.2. A total of ~10 randomly selected A. orientalis males and females from each non-target host were measured and compared to 10 randomly selected males and females reared from L. delicatula.
To evaluate the effects of non-target host egg age on parasitism rates/host acceptance of A. orientalis, the age of eggs from all non-target host species exposed to A. orientalis was recorded. Data from eggs exposed to A. orientalis for seven days in the non-choice experiment (see section 2) were used. Three species, G. arizonensis, N. viridula, and H. halys resulted in sufficient repetitions and/or age variability to be analyzed. Gloveria arizonensis Packard eggs age ranged from 5 to 35 days old. Nezara viridula egg age used in this study were ≤24 hours of age. Finally, H. halys egg age ranged from <24 hours to three days of age. Percent parasitism was compared between egg age for each of these three species.
2.6 Statistical analysis
All statistical analyses were conducted in R version 4.1.3 (41) using the development environment RStudio (42). Percent excess mortality that resulted from non-reproductive behavior of A. orientalis was compared with natural-occurring mortality rates in the paired controls using a generalized linear model (GLM) with a quasi-binomial distribution to account for high variance in data sets. The relation between the hind tibia size of the male parasitoids offspring and the sex ratio of parasitoid offspring emerging from the same host was analyzed using linear regression. Differences in mean hind tibiae lengths between male and female parasitoids emerging from different non-target species eggs and target eggs were analyzed using ANOVA followed by a Tukey posthoc test at the 0.05 level of significance using the package ‘multcomp’. All means are presented ± standard error (SE).
7 Results
7.1 No-choice sequential host-testing experiments
In addition to the target host L. delicatula, eggs from a total of 34 non-target host species, however, eggs of three Scaralina spp. were pooled as Scaralina sp. giving a functional total of 32 species that were exposed to A. orientalis females in no-choice sequential host testing experiments. From the total of 244 female A. orientalis that completed the entire sequential exposure series (non-target and target), 23 (9.4%) females failed to parasitize the non-target host and the target host. They were considered incompetent and were excluded from data analyses. There were three exceptions for non-target hosts; C. vittiger and C. vanduzeei, due to the low number of repetitions because of the low numbers of non-target eggs available for testing, and A. oslari due to the high mortality of parasitoids (n =16; 100% of females tested died) after exposure to non-target eggs (Table 2). Additionally, 41 (16.8%) parasitoids were able to parasitize non-target host eggs and then failed to parasitize L. delicatula eggs. The results from these trials were included in data analyses.
Anastatus orientalis parasitized five species in the order Hemiptera: A. thomasi (Coreidae), C. hilaris, H. halys and N. viridula (all Pentatomidae) and P. fuliginosa (Fulgoridae) (Table 2). Anastatus orientalis parasitized 10 species in the order Lepidoptera: A. luna, A. anona, Automeris cecrops pamina Neumoegen, Eupackardia calleta Westwood, Hyalophora euryalus Boisduval, Rothschildia cincta Tepper, S. walterorum (Saturniidae), G. arizonensis (Lasiocampidae), Pseudohemihyalea edwardsii Packard (Erebidae) and Pachysphinx occidentalis Edwards (Sphingidae) (Table 2). The maximum percent parasitism of eggs for non-target hosts in Hemiptera and Lepidoptera were obtained on the native pentatomid, C. hilaris, and the native saturniid, A. anona, at 68.79% ± 12.41 and 76.48% ± 14.10, respectively.
Percent non-target host mortality of eggs exposed to A. orientalis was corrected with their paired controls (Equation 2) and excess mortality for non-target hosts following exposure to parasitoids was calculated (Equation 3). Excess mortality was compared across nine non-target hosts species (four suitable for parasitism and five unsuitable for parasitism, Figure 3) with their paired controls. Percent excess mortality resulting from death other than parasitism that resulted in the emergence of an adult parasitoid (i.e., mortality from host feeding and/or failed parasitism) for non-target host species were not affected by exposure to A. orientalis and no significant differences were found for nine species (A. oslari; F1,18 = 0.184, P = 0.67, C. hilaris; F1,19 = 0.11, P = 0.75, D. citri; F1,4 = 0.82, P = 0.41, H. halys; F1,116 = 0.097, P = 0.75, J. haematoloma; F1,18 = 3.5, P = 0.13, L. zonatus; F1,13 = 0.69, P = 0.42, N. viridula; F1,30 = 1.98, P = 0.16, S. walterorum; F1,6 = 0.71, P = 0.43, T. neocalifornicus; F1,8 = 4.89, P = 0.058) (Figure 3).
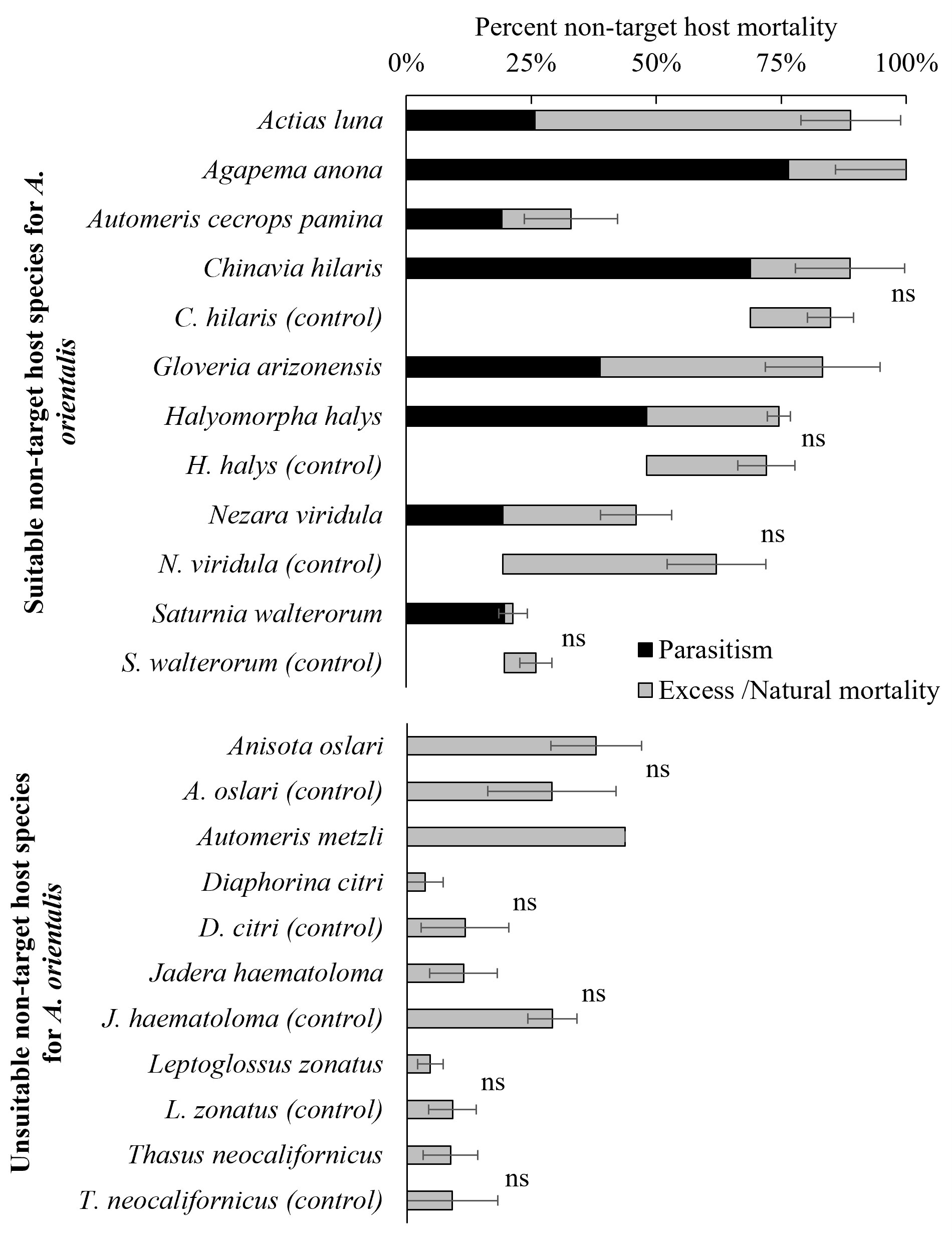
Figure 3 Percent non-target host mortality after exposure to A. orientalis from which parasitoids emerged (suitable hosts) and failed to emerge (unsuitable hosts). Species with no excess/natural mortality following parasitoid exposure were excluded from figure (Correction of mortality for exposed non-target host was null [Equation 2]). Total percent mortality of non-target hosts is the sum of the percentage of parasitism (in black) and the excess mortality (in grey) (± SE). (ns) Indicates no significant differences between the percent excess mortality after exposure to A. orientalis and the percentage of eggs alive without exposure to A. orientalis (control or naturally-occurring mortality).
Of the five non-target host species exposed to A. orientalis females for 24 hours, three pentatomids, C. hilaris, H. halys and N. viridula, and two Lepidoptera species, G. arizonensis (Lasiocampidae) and S. walterorum (Saturniidae), were all parasitized by A. orientalis. The maximum average percent parasitism was observed for S. walterorum (42.96 ± 25.7) and the minimum average percent parasitism was recorded for N. viridula (4.79 ± 1.91) (Table 2).
The effect of non-target host egg age on parasitism by A. orientalis was evaluated for three species, G. arizonensis, H. halys and N. viridula. Rates of parasitism decreased as non-target host egg age increased for H. halys (F1,92 = 1.54, P < 0.001), and no effect of egg age on parasitism was observed for N. viridula (F1,17 = 1.54, P = 0.23). Similarly, increasing age of G. arizonensis eggs did not affect parasitism rates (F1,13 = 0.07, P = 0.79) (Figure 2).
7.2 Choice host-testing experiments
The two non-target species used in choice experiments, G. arizonensis and H. halys, were parasitized in both exposure periods, 24 hours and 7 days, when exposed to A. orientalis in the presence of L. delicatula egg masses (Figure 4). For all the G. arizonensis vs L. delicatula choice trials (n = 12), four parasitoids failed to parasitize one of the two hosts species exposed; G. arizonensis and L. delicatula eggs were not parasitized three times and one time, respectively. For H. halys vs L. delicatula experiments (n = 19), three A. orientalis females did not parasitize either the target or the non-target host. Eight parasitoids failed to parasitize one of the two host species exposed; H. halys eggs were not parasitized in three trials and L. delicatula eggs were not parasitized in five trials.
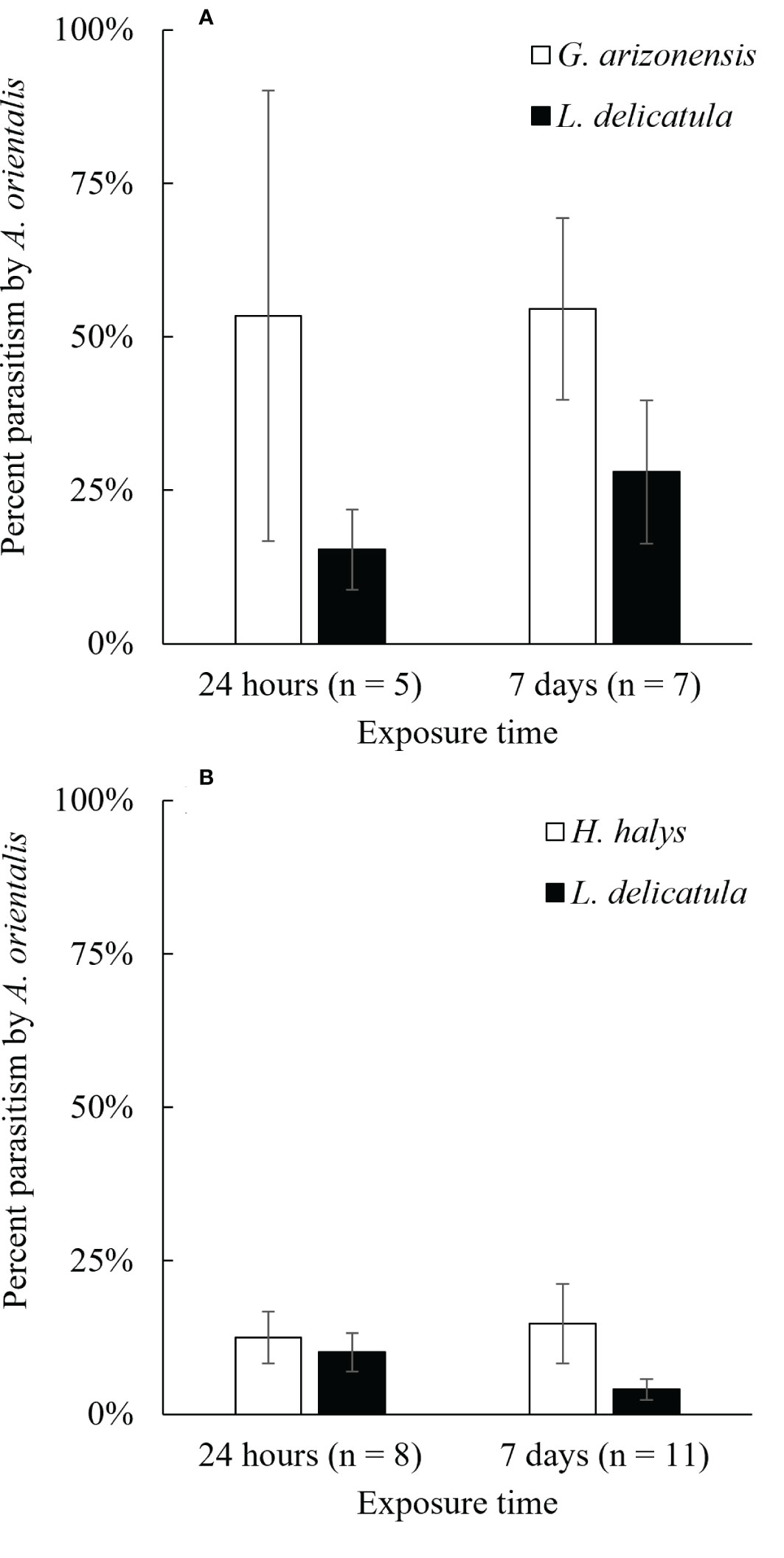
Figure 4 Average percent parasitism of non-target host eggs (A) G. arizonensis and (B) H. halys, when presented simultaneously with the target host, L. delicatula for two different exposure times, 24 hours and 7 days (n = number of repetitions).
7.3 Anastatus orientalis offspring from non-target hosts; sex ratio, male and female fertility, and size
7.3.1 Sex ratio
A total of 15 non-target host species were suitable hosts for A. orientalis and a total of 1,870 parasitoids, 1,780 males and 90 females, emerged from susceptible non-target species following seven-day exposure time. The highest female sex ratio was obtained from S. walterorum eggs (0.5 ± 0.32) (there was one exception, A. thomasi, which only one egg was exposed and parasitized and resulted in a female parasitoid). Seven non-target species did not produce females after being parasitized by A. orientalis (i.e., parasitism of A. anona, A. cecrops pamina, E. calleta, P. edwardsii, P. fuliginosa, P. occidentalis and R. cincta eggs produced only male offspring) (Table 2).
The five non-target species exposed to A. orientalis for 24 hours resulted in a total of 137 parasitoids, 110 males, 24 females and three larvae. The highest sex ratio between these five species was obtained from G. arizonensis eggs (0.72 ± 0.21). Only C. hilaris and H. halys did not produce females (i.e., male offspring only produced) after being parasitized by A. orientalis (Table 2).
7.3.2 Fertility
Male parasitoids were observed mating with females reared from the same non-target host species and female offspring were subsequently produced confirming mating was successful (A. orientalis is arrhenotokous and female offspring are produced from fertilized eggs). Consequently, male-female pairs of A. orientalis that emerged from the same non-target host species produced male and female offspring. (Table 3). Males that emerged from non-target host species successfully inseminated females emerging from L. delicatula egg masses as female offspring were produced from these male-female pairings (Table 3).
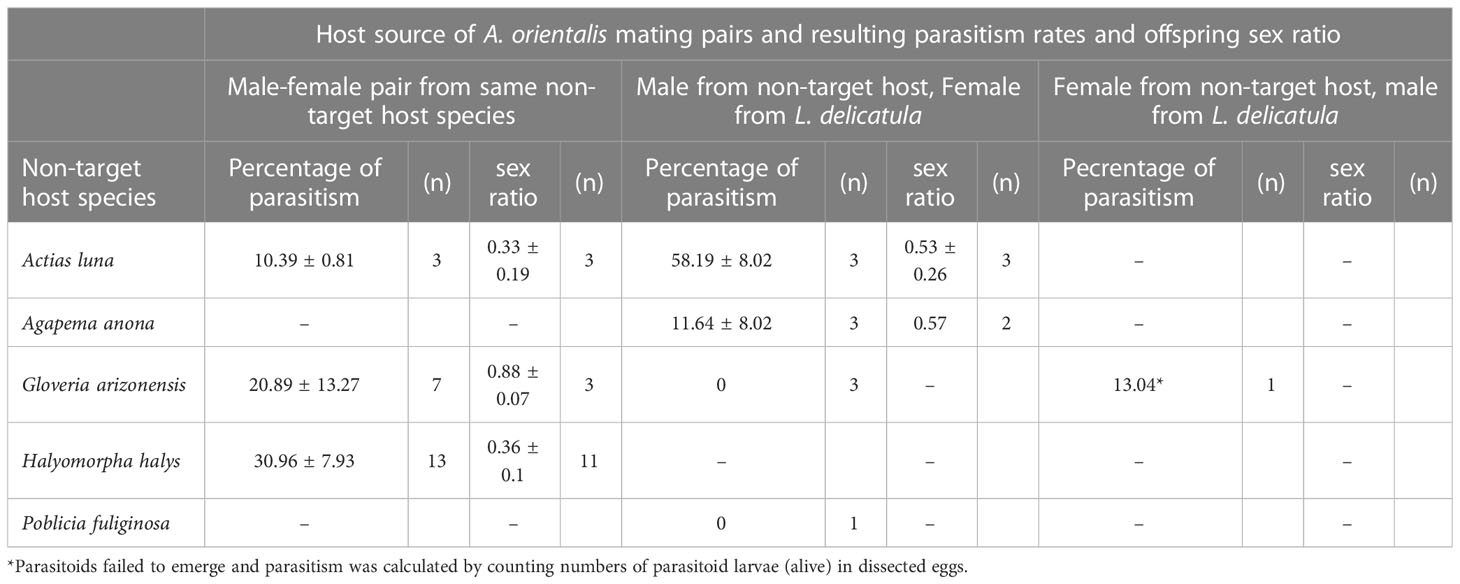
Table 3 Parasitism rates and offspring sex ratio (i.e., proportion of female offspring) produced by i) A. orientalis couples that emerged from five different non-target host species or ii) males that emerged from non-target host species mated with unmated females that emerged from L. delicatula eggs or iii) females that emerged from non-target host species mated with males that emerged from L. delicatula eggs (n = number of repetitions).
7.3.3 Size
A wide range of offspring sizes as well as a pronounced sexual dimorphism with larger females and smaller males was observed for A. orientalis when reared from non-target host species (Table 4). Average male hind tibia lengths ranged from 0.309 ± 0.005 mm (host: P. edwardsii) to 0.587 ± 0.022 mm (host: G. arizonensis). Average hind tibia lengths for females ranged from 0.613 ± 0.007 mm (host: C. hilaris) to 1.04 mm (host: A. thomasi) (Table 4). The largest A. orientalis males emerged from the target host, L. delicatula (F6,62 = 100.9, P < 0.001). The largest female emerged from the non-target host, A. thomasi. All other non-target hosts from which females emerged were smaller when compared with females that emerged from L. delicatula eggs (F3,26 = 285.4, P < 0.001) (Table 4). The average size of male offspring was significantly correlated with the sex ratio of the parasitoids emerging from the same host species (F1,6 = 7.668, P = 0.032; R2 = 0.561) (Figure 5). Non-target hosts that produced A. orientalis males were smaller and the sex ratio of emerged parasitoids was lower (i.e., male biased) and larger parasitoids typically emerged from host eggs that had female –biased sex ratios (Figure 5).
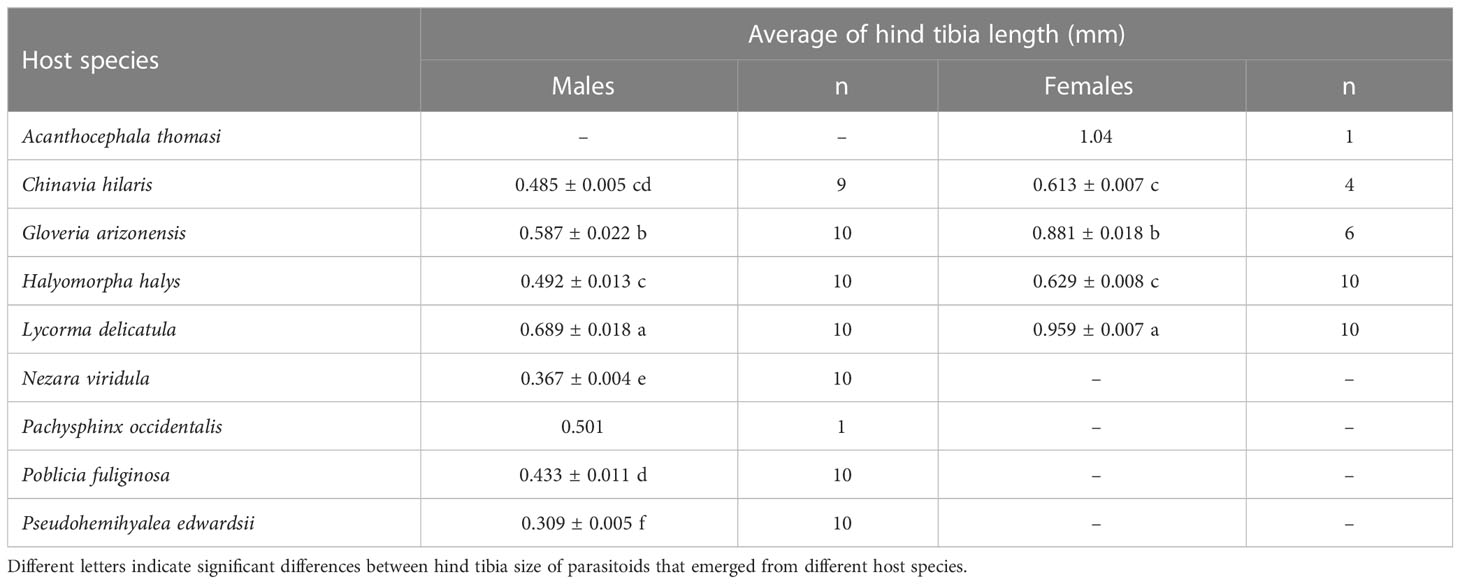
Table 4 Average hind tibia length (mm) of A. orientalis females and males that emerged from eggs of non-target species.
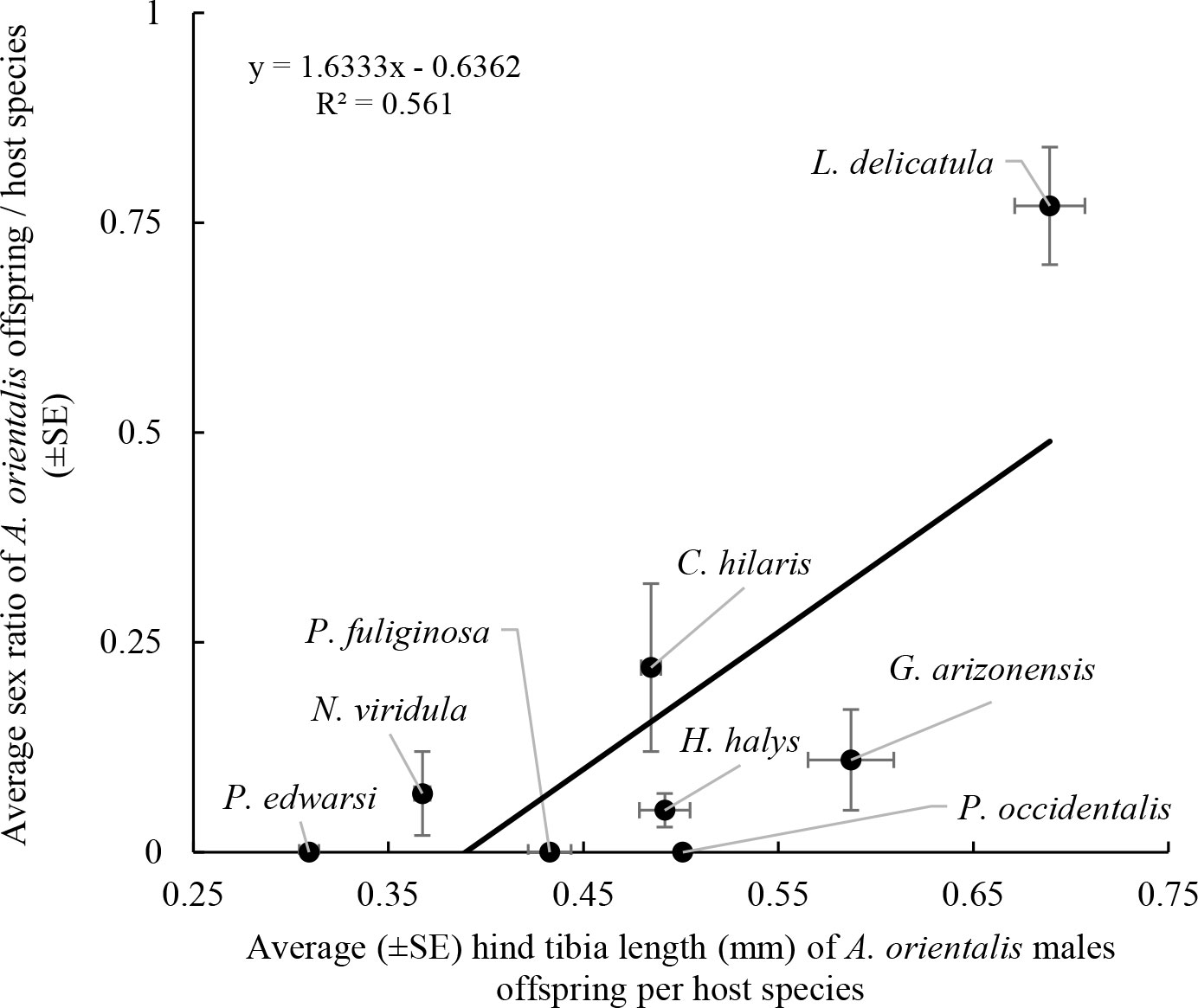
Figure 5 Relation between hind tibia length of A. orientalis offspring males emerging from each host (average values from Table 4) and sex ratio obtained from the same host (average values from Table 2).
8 Discussion
This is the first study to assess the physiological host range and specificity of A. orientalis (Haplotype C) with respect to potential non-target species that occur in the southwestern U.S. In the quarantine laboratory, no-choice tests assessing the developmental suitability of non-target host species for A. orientalis demonstrated that 15 out of 34 non-target host species were suitable hosts for A. orientalis. This work indicated H. halys eggs could successfully support development of A. orientalis (offspring sex ratio was strongly male biased), a finding contrary to previous reports (43). Antheraea sp. (Saturniidae) eggs (i.e., A. pernyi) have been reported to be suitable reproductive hosts for A. orientalis (43). However, parasitization of Antheraea oculea Neumoegen eggs by A. orientalis was not recorded in this study. Five new hemipteran hosts in three families (Coreidae [A. thomasi {native}], Fulgoridae [P. fuliginosa {native}], and Pentatomidae [C. hilaris {native}, H. halys {invasive} N. viridula {invasive}]) and ten lepidopteran hosts in four families (Erebidae [P. edwardsii {native}], Lasiocampidae [G. arizonensis {native}], Saturniidae [A. luna {non-native}, A. anona {native}, A. cecrops pamina {native}, E. calleta {native}, H. euryalus {native}, R. cincta {native}, S. walterorum {native}] and Sphingidae [P. occidentalis {native}]) were identified as suitable hosts and are added to an increasing list of identified species that A. orientalis can successfully use as reproductive hosts in the laboratory (Table 2).
In terms of percent parasitism, eggs of two non-target species, C. hilaris (Pentatomidae [native]) and A. anona (Saturniidae [native]), were similar to or better hosts, than the target, L. delicatula. In the first case, C. hilaris, percent parasitism (68.79% ± 12.41; sex ratio 0.22 ± 0.1) was similar to the target, L. delicatula (58.24 ± 10.09; sex ratio 0.77 ± 0.07). This result may need to be interpreted cautiously, because C. hilaris eggs masses had on average a smaller number of eggs (17.73 ± 2.51) when compared to the average number of eggs per egg mass (39.08 ± 3.84) from the target, L. delicatula. In the second case, A. anona, percent parasitism was higher (76.48 ± 14.10; sex ratio 0) than the target L. delicatula (58.24 ± 10.09), and both egg masses were approximately equal in size (i.e., 42.2 ± 7.43 eggs and 39.08 ± 3.84 eggs for A. anona and L. delicatula, respectively). Collectively, data reported here and findings from companion studies (i.e., Broadley et al. [USDA], Submitted) suggest that A. orientalis, is at a minimum oligophagous, but more likely to be a polyphagous species.
Similar results from host specificity tests from other Anastatus spp. have been reported further supporting findings that Anastatus spp. potentially have broad host ranges. Stahl et al. (32, 33) studied the physiological host range of A. bifasciatus (this species is native to Europe) for use as a biological control agent against H. halys, an invasive pest in Europe. In this study, A. bifasciatus successfully parasitized eggs of eight pentatomid species (including N. viridula [tested in this study]) and 14 lepidopteran species belonging to seven different families (Endromidae, Erebidae, Lasiocampidae, Notodontidae, Papilionidae, Saturniidae, and Sphingidae). Results reported here indicate that A. orientalis can also parasitize hosts eggs from Erebidae, Lasiocampidae, Saturniidae and Sphingidae. Host range tests that expose A. orientalis females to eggs of Notodontidae and Papilionidae, two lepidopteran families with species representation in the southwest U.S., may be warranted to further understanding of potential non-target host use (the family Endromidae is not present in North America). For Anastatus spp., results reported here and those of Stahl et al. (32, 33), indicate strongly that selecting non-target species which are restricted to close taxonomic relatedness to the target pest (e.g., families in the Fulgoroidea [Hemiptera: Auchenorrhyncha]) may be inadequate as Anastatus spp. may tend to be generalists capable of parasitizing species across different orders.
No additional significant levels of excess mortality (i.e., mortality from causes other than parasitism) to non-target species exposed to A. orientalis was observed (Figure 3). Failure to detect excess mortality of non-target eggs exposed to A. orientalis when compared to levels of naturally-occurring mortality in control eggs not exposed to A. orientalis may have at least two explanations: i) parasitized non-target eggs develop successfully when parasitoid larvae and/or envenomation failed to kill the host egg (host eggs are incapable of encapsulating parasitoid eggs and larvae), or ii) A. orientalis females only host fed on eggs that were parasitized and it is possible that A. orientalis is a concurrent parasitoid (i.e., host feeds on parasitized eggs). Thus, egg mortality from host feeding alone was not observed and egg mortality was attributed to solely to parasitism. Additional studies confirming the lack of excess mortality due to unsuccessful parasitization or host feeding following exposure of non-target host species to A. orientalis are needed. Excess mortality of non-target host eggs following exposure to A. orientalis should be considered an important deleterious non-target impact if it occurs (44).
For three non-target host species tested, A. oslari (Saturniidae [native]), C. vanduzeei (Fulgoridae [native]) and C. vittiger (Coreidae [native]), all parasitoid females exposed to egg masses of these species failed to parasitize L. delicatula egg masses in sequential exposure tests. For C. vanduzeei and C. vittiger, too few non-target eggs were available for experiments and replication was low for each test species and therefore were not excluded from the results. Interestingly, for female A. orientalis exposed to A. oslari eggs excess egg mortality increased slightly but not significantly, and all females (n=16) died following exposure to A. oslari eggs prior to sequential exposure to L. delicatula egg masses. These results, a slight but non-significant increase in excess egg mortality and premature mortality of females, suggest that A. orientalis females may have host fed on A. oslari eggs and egg contents were possibly toxic to parasitoids. This could be explained by chemical protection of eggs by secondary plant compounds, like tannins, which are used as chemical defenses by host plants (i.e., Quercus spp.) of A. oslari (45). Sequestration of protective compounds, like tannins, in A. oslari eggs could reduce survivorship rates of third trophic level organisms like A. orientalis (46).
To evaluate a more realistic exposure time of A. orientalis to non-target species, 24 hour exposure tests (as opposed to a 7 day exposure period) were performed with five non-target species (C. hilaris, G. arizonensis, H. halys, N. viridula and S. walterorum). Anastatus orientalis was able to parasitize the five non-target species in this shorter exposure period following the seven-day pre-oviposition exposure period on L. delicatula eggs. These findings suggest that prior host exposure, especially to the target, L. delicatula, does not deter use of subsequent non-target host eggs. In addition, when non-target and target host eggs were exposed to A. orientalis females at the same time (i.e., choice experiments), that non-target species were attacked under both exposure time scenarios (i.e., 24 hours and 7 days). These results further suggest that A. orientalis is probably a generalist parasitoid capable of using any suitable non-target host species upon encounter. In many instances, female parasitoids engaged in parasitism within minutes of introduction into test arenas and contact with non-target eggs (pers. obs. F. Gomez Marco).
The age of non-target host eggs can affect the acceptance behavior of A. orientalis females and rates of successful parasitism. For example, percent parasitism by A. orientalis was higher on young eggs (<24 hours of age) vs. older eggs (>24 hours to 3 days) of H. halys. However, for two other non-target species, G. arizonensis and N. viridula, tested in egg age acceptance studies, no significant effect of egg age on parasitism was found. Therefore, age of non-target eggs and the non-target species may be an important covariables to consider when host tests are being designed and executed. Additionally, defensive chemical compounds (see above) may also affect the acceptance behavior of parasitoids (and survivorship rates) and this may also warrant consideration in design, analysis, and interpretation of host range tests (46).
The sex ratio of A. orientalis offspring was strongly dependent on host egg size. All non-target host eggs which were parasitized by A. orientalis with an average egg size visibly (not measured in this study) smaller than L. delicatula produced a male biased sex ratio (< 0.5) and smaller adult males and females. However, the sex ratio from hosts which produced smaller A. orientalis males decreased, indicating that there was a strong correlation between offspring size and offspring sex ratio. Only one non-target host with eggs larger than L. delicatula eggs (pers. obs. F. Gomez Marco) was used in our tests (A. thomasi) and this resulted in the largest female parasitoid (hind tibia size of 1.04 mm) obtained from host-range studies. These findings tentatively support conditional sex allocation theory, where, with decreasing host quality (i.e., host egg size in this study), parasitoid offspring sex ratio becomes more male biased and males are generally smaller, both of which correlate with decreased fitness (47). In support of results presented here, two previous studies, Hou et al. (48) and Stahl et al. (32), reported more male-biased sex ratios when Anastatus spp. were reared on host eggs that were smaller than the target host. Interestingly, differences in A. orientalis offspring sex ratio from two non-target host species, C. hilaris and H. halys, existed when egg masses were exposed for 24 hours (C. hilaris and H. halys: 0, no females) or seven days (C. hilaris: 0.22 ± 0.1, H. halys: 0.05 ± 0.02). This finding might indicate a preference of A. orientalis females to first oviposit (within at least the first 24 hours) non-fertilized eggs (i.e., produce male offspring) and then oviposit fertilized eggs (i.e., produce female offspring) when exposure times are longer and there is more time to repeatedly assess host quality. Sex ratio of offspring has important demographic implications as it affects rates of population grown. Male-biased progeny production on non-target hosts of marginal quality may limit or negate adverse population-level impacts on non-target species (32).
Anastatus orientalis offspring reared from non-target host species successfully parasitized L. delicatula egg masses. Percent parasitism and sex ratios resulting from mated couples that emerged from the same non-target host species were similar to values recorded for A. orientalis reared continuously on L. delicatula. Interestingly, males that emerged from G. arizonensis and P. fuliginosa when paired with unmated A. orientalis females that emerged from L. delicatula failed to produce any offspring. Due to the low number of repetitions for these experiments this finding should be viewed with caution. However, no obvious biological explanation (i.e., size of the males or inability to copulate with females) was observed to explain these results as unmated A. orientalis females should be able to oviposit unfertilized eggs that produce male offspring without mating with males that emerged from G. arizonensis and P. fuliginosa.
In laboratory studies in a quarantine facility, sequential no-choice and choice exposure studies that exposed female A. orientalis to non-target eggs of 34 native and non-native species in three orders and 12 families and target eggs (L. delicatula) for either 24 hours or 7 days, indicate that this egg parasitoid potentially has a wide host range as it successfully parasitized 15 species in 6 families in two orders (Hemiptera and Lepidoptera). Results presented here are for A. orientalis (Haplotype C). Other haplotypes of A. orientalis have been identified and are being assessed to determine if differences (i.e., greater specificity) in host preferences exist (20). A well-founded criticism of host-range tests is that parasitoids are constrained in small ventilated containers spaces with easily accessible hosts for long periods of time (i.e., 24 hours to 7 days) and are unable to engage in behaviors (e.g., rapid abandonment of patches with sub-optimal hosts) that could reduce or eliminate non-target use (49–52). Consequently, in the absence of comprehensive field data on host use and non-target species - target species - parasitoid phenology in the native range (i.e., China) from where A. orientalis was sourced, it is difficult to determine if high levels of non-target host use observed in host range tests reported here occurs in the field. Until detailed field data from the native range are available and based on results of host range tests reported here that suggest A. orientalis [and possibly most species of Anastatus (23–27, 32, 33)] has a very broad host range, use of this natural enemy in classical biological control of L. delicatula in the western U.S. should be assessed critically and with extreme caution.
Data availability statement
The raw data supporting the conclusions of this article will be made available by the authors, without undue reservation.
Author contributions
FG-M conceived and designed the experiments, performed collections and experiments, analyzed the data, prepared figures and/or tables, authored or reviewed drafts of the paper, and approved the final draft. DY performed collections, reviewed drafts of the paper, and approved the final draft. MR performed collections, reviewed drafts of the paper, and approved the final draft. MH obtained the funding, conceived and designed the experiments, performed collections authored or reviewed drafts of the paper, and approved the final draft. All authors contributed to the article and approved the submitted version.
Funding
Funding for this work was provided by the California Department of Agriculture Office of Environmental Farming and Innovation’s Proactive Integrated Pest Management Solutions Grant Program, award number 18-0632-000-SG “Proactive Biological Control of Spotted Lantern Fly, Lycorma delicatula (Hemiptera: Fulgoridae)”.
Acknowledgments
We thank Dr. Juli Gould, Dr. Hannah Broadley (USDA APHIS PPQ, Forest Pest Methods Laboratory), and Dr. Kim Hoelmer (USDA ARS, Beneficial Insects Introduction Research Unit) for their assistance and contributions in starting and maintaining this project and for providing A. orientalis to start colonies at UC Riverside. We are indebted to USDA APHIS field crews for their enthusiastic assistance with identification of infested field sites and L. delicatula egg mass collections at field sites in Pennsylvania over winter. We acknowledge Prof. Jocelyn Millar, Prof. Kent Deena, Prof. Richard Stouthamer and Prof. Thomas Perring and their respective lab members for providing non-target eggs. Ruth Amrich and Ishika Sameth provided assistance with colony maintenance and experiments in the Insectary & Quarantine facility at UC Riverside. We thank Bryan David, Dr. Serguei Triapitsyn (Principal Museum Scientist at UC Riverside) Michael Wilson, and Dr. Steve McElfresh for their help to collect non-target native fauna. Dr. Jorge Torres, Departamento de Agronomia, Entomologia, Universidade Federal Rural de Pernambuco, Recife, Brazil provided extraordinary assistance with establishment and maintenance of pentatomid colonies during his 12-month sabbatical program with the Hoddle lab. We thank the American Museum of Natural History’s Southwestern Research Station (Portal, Arizona) team for generous assistance and access to field sites. Dr. A. James Downer, Barbara Roth, and Reed Peters (Cave Creek Ranch) for hosting us during our summer field trips to Portal, Arizona.
Conflict of interest
The authors declare that the research was conducted in the absence of any commercial or financial relationships that could be construed as a potential conflict of interest.
Publisher’s note
All claims expressed in this article are solely those of the authors and do not necessarily represent those of their affiliated organizations, or those of the publisher, the editors and the reviewers. Any product that may be evaluated in this article, or claim that may be made by its manufacturer, is not guaranteed or endorsed by the publisher.
References
1. Hoddle MS, Lake EC, Minteer CR, Daane KM. Importation biological control. In: Mason PG (ed). Biological control: Global impacts, challenges and future directions of pest management. Clayton South: CSIRO PUBLISHING. (2021). 67–89.
2. United States Department of Agriculture (USDA). Technical advisory group for biological control agents of weeds manual Washington DC. (2017) (Accessed October 15, 2022).
3. Hoddle MS, Mace K, Steggall J. Proactive biological control: a cost-effective management option for invasive pests. California Agric (2018) 72:148–50. doi: 10.3733/ca.2018a0027
4. Kim H, Kim M, Kwon DH, Park S, Lee Y, Huang J, et al. Molecular comparison of Lycorma delicatula (Hemiptera: Fulgoridae) isolates in Korea, China, and Japan. J Asia-Pac Entomol (2013) 16:503–6. doi: 10.1016/j.aspen.2013.07.003
5. Barringer LE, Donovall LR, Spichiger S-E, Lynch D, Henry D. The first new world record of Lycorma delicatula (Insecta: Hemiptera: Fulgoridae). Entomol News (2015) 125:20–4. doi: 10.3157/021.125.0105
7. Dara SK, Barringer L, Arthurs SP. Lycorma delicatula (Hemiptera: Fulgoridae): a new invasive pest in the united states. J Int Pest Manag (2015) 6:1–6. doi: 10.1093/jipm/pmv021
8. Urban JM, Calvin D, Hills-Stevenson J. Early response (2018–2020) to the threat of spotted lanternfly, Lycorma delicatula (Hemiptera: Fulgoridae) in Pennsylvania. Ann Entomol Soc Am (2021) 114:709–18. doi: 10.1093/aesa/saab030
9. Liu H, Hartlieb RJ. Spatial distribution of Lycorma delicatula (Hemiptera: Fulgoridae) egg masses on tree-of-heaven, black walnut, and Siberian elm in north America. J Econ Entomol (2020) 113:1028–32. doi: 10.1093/jee/toz350
10. Nixon LJ, Jones SK, Tang L, Urban J, Felton K, Leskey TC. Survivorship and development of the invasive Lycorma delicatula (Hemiptera: Fulgoridae) on wild and cultivated temperate host plants. Environ Entomol (2022) 51:222–8. doi: 10.1093/ee/nvab137
11. Lee D-H, Park Y-L, Leskey TC. A review of biology and management of Lycorma delicatula (Hemiptera: Fulgoridae), an emerging global invasive species. J Asia-Pac Entomol (2019) 22:589–96. doi: 10.1016/j.aspen.2019.03.004
12. Baker TC, Smyers EC, Urban JM, Meng Z, Pagadala Damadaram KJ, Myrick AJ, et al. Progression of seasonal activities of adults of the spotted lanternfly, lycorma delicatula, during the 2017 season of mass flight dispersal behavior in eastern Pennsylvania. J Asia-Pac Entomol (2019) 22:705–13. doi: 10.1016/j.aspen.2019.05.006
13. Urban JM, Leach H. Biology and management of the spotted lanternfly, Lycorma delicatula (Hemiptera: Fulgoridae), in the united states. Ann Rev Entomol (2023) 68:151–67. doi: 10.1146/annurev-ento-120220-111140
14. Wakie TT, Neven LG, Yee WL, Lu Z. The establishment risk of Lycorma delicatula (Hemiptera: Fulgoridae) in the united states and globally. J Econ Entomol (2019) 113:306–14. doi: 10.1093/jee/toz259
15. California Deparment of Food and Agriculture. California Agricultural production statistics (2019) (Accessed 2022 February).
16. Choi M-Y, Yang Z-Q, Wang X-Y, Tang Y-L, Hou Z-R, Kim JH, et al. Parasitism rate of egg parasitoid Anastatus orientalis (Hymenoptera: Eupelmidae) on Lycorma delicatula (Hemiptera: Fulgoridae) in China. K J App Entomol (2014) 53:135–9. doi: 10.5656/KSAE.2014.01.1.075
17. Yang Z-Q, Choi W-Y, Cao L-M, Wang X-Y, Hou Z-R. A new species of Anastatus (Hymenoptera: Eulpelmidae) from China, parasitizing eggs of Lycorma delicatula (Homoptera: Fulgoridae). Zool Syst (2015) 40:290–302. doi: 10.11865/zs.20150305
18. Xin B, Zhang Y-l, Wang X-y, Cao L-m, Hoelmer KA, Broadley HJ, et al. Exploratory survey of spotted lanternfly (Hemiptera: Fulgoridae) and its natural enemies in China. Environ Entomol (2020) 50:36–45. doi: 10.1093/ee/nvaa137
19. Broadley HJ, Gould JR, Sullivan LT, Wang X-y, Hoelmer KA, Hickin ML, et al. Life history and rearing of Anastatus orientalis (Hymenoptera: Eupelmidae), an egg parasitoid of the spotted lanternfly (Hemiptera: Fulgoridae). Environ Entomol (2021) 50:28–35. doi: 10.1093/ee/nvaa124
20. Wu Y, Broadley HJ, Vieira KA, McCormack JJ, Losch C, McGraw AR, et al. Cryptic genetic diversity and associated ecological differences of anastatus orientalis, an egg parasitoid from China of the spotted lanternfly. Front Insect Sci – Invasive Insect Species. (Submitted).
21. Askew A. A new european species of Anastatus motschulsky (Hym., eupelmidae). Entomol Monthly Mag (2005) 141:1697–9.
22. Jones WA. World review of the parasitoids of the southern green stink bug, Nezara viridula (L.) (Heteroptera: Pentatomidae). Ann Entomol Soc Am (1988) 81:262–73. doi: 10.1093/aesa/81.2.262
23. Burks BD. The north american species of Anastatus motschulsky (Hymenoptera, eupelmidae). Trans Am Entomological Soc (1967) . 93:423–32.
24. , Pieltain BC. Estudio monográfico de las especies españolas del género anastatus Motsch.(Hym. chalc.). Revista Española de Entmologia 10. (1935). 273–92.
25. Chen YM, Gibson GAP, Peng LF, Iqbal A, Zang LS. Anastatus motschulsky (Hymenoptera, eupelmidae): egg parasitoids of Caligula japonica Moore (Lepidoptera, saturniidae) in China. Zookeys (2019) 881:109–34. doi: 10.3897/zookeys.881.34646
26. Noyes JS. Universal chalcidoidea database. World Wide Web electronic publication (2019). (Accessed October 15, 2022).
27. Hayat M. Some indian species of Anastatus (Hiymenoptera: Chalcidoidea, eupelmidae). Oriental Insects (1975) 9:261–71. doi: 10.1080/00305316.1975.10434497
28. Crossman S. Two imported egg parasites of the gipsy moth, Anastatus bifasciatus fonsc and Schedius kuvanae Howard. J Agric Res (1925) 30:643–75.
29. Chen Y, Lin L, Chen J. Preliminary report of study on the release of Anastatus for the control of the stink bug (Tessaratoma papillosa). Fujian Agric Sci Technol (1990) 2:15–6.
30. Huang M, Mai S, Wu W, Poo C. The bionomics of Anastatus sp. and its utilization for the control of lichee stink bug, Tessaratoma papillosa Drury. Act Entomol Sin (1974) 17:362–75.
31. Shrestha R. Productivity improvement of citrus fruits through effective fruit drop management technique in the mid and far Western development region of Nepal. Tech Rep (2011).
32. Stahl JM, Babendreier D, Haye T. Using the egg parasitoid Anastatus bifasciatus against the invasive brown marmorated stink bug in Europe: Can non-target effects be ruled out? J Pest Sci (2018) 91:1005–17. doi: 10.1007/s10340-018-0969-x
33. Stahl JM, Babendreier D, Marazzi C, Caruso S, Costi E, Maistrello L, et al. Can Anastatus bifasciatus be used for augmentative biological control of the brown marmorated stink bug in fruit orchards? Insects (2019) 10(4):108. doi: 10.3390/insects10040108
34. Ogburn EC, Bessin R, Dieckhoff C, Dobson R, Grieshop M, Hoelmer KA, et al. Natural enemy impact on eggs of the invasive brown marmorated stink bug, Halyomorpha halys (Stål) (Hemiptera: Pentatomidae), in organic agroecosystems: a regional assessment. Biol Control (2016) 101:39–51. doi: 10.1016/j.biocontrol.2016.06.002
35. Govender AW. Australian Fruitspotting bugs, amblypelta nitida stål and a. lutescens lutescens distant (Hemiptera: Coreidae), and the potential for their biologically based management in macadamia orchards. Queensland, Australia: University of Queensland (2015).
36. Huwer R, Maddox C, Fay H, Llewellyn R, Danne A, Hickey M, et al. A multi target approach to fruitspotting bug management. In: Proceedings of the VII world avocado congress. Cairns, Australia (2011).
38. Henderson CF, Tilton EW. Tests with acaricides against the brown wheat mite. J Econom Entomol (1955) 48:157–61. doi: 10.1093/jee/48.2.157
39. Gebiola M, Gomez-Marco F, Simmons GS, Stouthamer R. Effect of host feeding on life history traits of Tamarixia radiata, parasitoid of the Asian citrus psyllid, diaphorina citri. BioControl (2018) 63:763–71. doi: 10.1007/s10526-018-9903-7
40. Ameri M, Rasekh A, Michaud JP, Allahyari H. Morphometric indicators for quality assessment in the aphid parasitoid, Lysiphlebus fabarum (Braconidae: Aphidiinae). Eur J Entomol (2013) 110:519–25. doi: 10.14411/eje.2013.069
41. R Core Team. R: A language and environment for statistical computing. Vienna, Austria: R Foundation for Statistical Computing (2022).
43. Seo M, Kim JH, Seo BY, Park C, Choi BR, Kim KH, et al. Mass-rearing techniques of Anastatus orientalis (Hymenoptera: Eupelmidae), as the egg-parasitoid of Lycorma delicatula (Hemiptera: Fulgoridae): an using method of Antheraea pernyi (Lepidoptera: Saturniidae) and l. delicatula eggs in laboratory. K J App Entomol (2018) 57:243–51. doi: 10.5656/KSAE.2018.08.0.035
44. Abram PK, Brodeur J, Urbaneja A, Tena A. Nonreproductive effects of insect parasitoids on their hosts. Annu Rev Entomol (2019) 64:259–76. doi: 10.1146/annurev-ento-011118-111753
45. Forkner RE, Marquis RJ, Lill JT. Feeny revisited: condensed tannins as anti-herbivore defences in leaf-chewing herbivore communities of quercus. Ecol Entomol (2004) 29:174–87. doi: 10.1111/j.1365-2311.2004.0590.x
46. Fatouros NE, Cusumano A, Bin F, Polaszek A, van Lenteren JC. How to escape from insect egg parasitoids: A review of potential factors explaining parasitoid absence across the insecta. Proc R Soc B: Biol Sci (2020) 287:20200344. doi: 10.1098/rspb.2020.0344
47. Charnov EL, Los-den Hartogh RL, Jones WT, van den Assem J. Sex ratio evolution in a variable environment. Nature (1981) 289:27–33. doi: 10.1038/289027a0
48. Hou Z, Liang H, Chen Q, Hu Y, Tian H. Application of Anastatus sp. against Halyomorpha halys. For Pest Dis (2009) 4:39.
49. Haye T, Goulet H, Mason PG, Kuhlmann U. Does fundamental host range match ecological host range? a retrospective case study of a lygus plant bug parasitoid. Biol Control (2005) 35:55–67. doi: 10.1016/j.biocontrol.2005.06.008
50. Haye T, Mason PG, Gillespie DR, Miall JH, Gibson GAP, Diaconu A, et al. Determining the host specificity of the biological control agent Trichomalus perfectus (Hymenoptera: Pteromalidae): The importance of ecological host range. Biocontrol Sci Techn (2015) 25:21–47. doi: 10.1080/09583157.2014.945900
51. van Lenteren JC, Loomans AJ. Environmental risk assessment: Methods for comprehensive evaluation and quick scan. In: Bigler F, Babendreier D, Kuhlmann U, editors. Environmental impact of invertebrates for biological control of arthropods: methods and risk assessment, vol. 10 . Wallingford: CABI Publishing (2006). p. 254–72.
52. Whiters T, Mansfield S. Choice or no-choice tests? effects of experimental design on the expression of host range. In: Hoddle M (ed), Second international symposium on biological control of arthropods. Davos, Switzerland: USDA Forest Service Bulletin, Morgantown, West Virginia, USA (2005). 620–32.
Keywords: Coreidae, Lasiocampidae, Spotted lanternfly, Saturniidae, parasitoid, polyphagy, southwestern U.S
Citation: Gómez Marco F, Yanega D, Ruiz M and Hoddle MS (2023) Proactive classical biological control of Lycorma delicatula (Hemiptera: Fulgoridae) in California (U.S.): Host range testing of Anastatus orientalis (Hymenoptera: Eupelmidae). Front. Insect Sci. 3:1134889. doi: 10.3389/finsc.2023.1134889
Received: 31 December 2022; Accepted: 08 February 2023;
Published: 20 February 2023.
Edited by:
Miriam Cooperband, USDA APHIS PPQ, United StatesReviewed by:
Jana Collatz, Agroscope (Switzerland), SwitzerlandTeodora Borislavova Toshova, Bulgarian Academy of Sciences, Bulgaria
Copyright © 2023 Gómez Marco, Yanega, Ruiz and Hoddle. This is an open-access article distributed under the terms of the Creative Commons Attribution License (CC BY). The use, distribution or reproduction in other forums is permitted, provided the original author(s) and the copyright owner(s) are credited and that the original publication in this journal is cited, in accordance with accepted academic practice. No use, distribution or reproduction is permitted which does not comply with these terms.
*Correspondence: Francesc Gómez Marco, ZnJhbmNlZ21AdWNyLmVkdQ==