- Department of Clinical Research, Indian Council of Medical Research (ICMR)-National Institute for Research in Tuberculosis, Chennai, India
Tuberculosis (TB) is a communicable disease that is a major source of illness, one of the ten causes of mortality worldwide, and the largest cause of death from a single infectious agent Mycobacterium tuberculosis. HIV infection and TB are a fatal combination, with each speeding up the progression of the other. Barriers to integrated treatment as well as safety concerns on the co-management of HIV- TB co-infection do exist. Many HIV TB co-infected people require concomitant anti-retroviral therapy (ART) and anti-TB medication, which increases survival but also introduces certain management issues, such as drug interactions, combined drug toxicities, and TB immune reconstitution inflammatory syndrome which has been reviewed here. In spite of considerable pharmacokinetic interactions between antiretrovirals and antitubercular drugs, when the pharmacological characteristics of drugs are known and appropriate combination regimens, dosing, and timing of initiation are used, adequate clinical response of both infections can be achieved with an acceptable safety profile. To avoid undesirable drug interactions and side effects in patients, anti TB treatment and ART must be closely monitored. To reduce TB-related mortality among HIV-TB co-infected patients, ART and ATT (Anti Tuberculosis Treatment) outcomes must improve. Clinical practise should prioritise strategies to promote adherence, such as reducing treatment duration, monitoring and treating adverse events, and improving treatment success rates, to reduce the mortality risk of HIV-TB co-infection.
Introduction
Tuberculosis (TB), a communicable disease, is one of the ten major causes of mortality worldwide, and the largest cause of death from a single infectious agent Mycobacterium tuberculosis. In 2019, an estimated 10.0 million people had active TB worldwide, with 1.2 million HIV-negative individuals and 208 000 HIV-positive people dying from the disease (1).
Drug-resistant (DR) TB occurs when bacteria become resistant to the drugs used to treat TB.
Rifampicin resistance includes any resistance to rifampicin detected using phenotypic or genotypic method whereas multidrug resistance is resistance to at least both isoniazid and rifampicin. Drug-resistant TB is a major health concern globally with about half a million persons diagnosed with rifampicin-resistant tuberculosis (RR-TB), with 78% having multidrug-resistant tuberculosis (MDR-TB). India (27 percent), China (14 percent), and the Russian Federation (14 percent) were the three countries with the highest share of the global burden. MDR/RR-TB was found among 3.3 percent of new TB patients and 17.7 percent of previously treated cases globally (1).
HIV and TB constitute a fatal combination, with each speeding up the progression of the other. HIV impairs the immune system, increasing the risk of TB in HIV-positive individuals. Even when HIV-infected individuals are on antiretroviral therapy (ART), the risk for developing active TB increases by 2-5 times during early HIV-1 infection and by a significant margin of more than 20 times in advanced HIV-1 disease. Even while on ART there is an approximately 4-fold increased risk of TB for HIV-infected patients (2). Also, one of the major risk factors for latent tuberculosis infection (LTBI) progression to active TB is HIV infection. LTBI in immunocompromised individuals may serve as a reservoir for future progression to TB disease (3).
Many HIV infected TB patienst have few symptoms or less specific symptoms of TB (productive cough, chest pain, shortness of breath, hemoptysis, fever, night sweats, and/or weight loss). They have atypical chest radiographic findings. As they are less likely to have cavitory lesions, smear negative TB is more common. Evaluate all HIV patients for TB and offer HIV testing for all TB patients.
Barriers to integrated treatment as well as safety concerns on the co-management of HIV and TB infection exist. The obstacles include a separate set of health care providers to treat HIV and TB, lack of proper clinical guidance to detect and manage appropriately the immune reconstitution inflammatory syndrome (IRIS), occurrence of potential drug-drug interactions, additive drug toxicities and tolerability issues besides the adherence challenges associated with high pill burden.
Many HIV TB co-infected people require concomitant ART and anti-TB medication, which increases survival but also introduces certain management issues, such as drug interactions, combined drug toxicities, and TB IRIS which has been reviewed here. In spite of considerable pharmacokinetic interactions between antiretrovirals and antitubercular drugs, when the pharmacological characteristics of drugs are known and appropriate combination regimens, dosing, and timing of initiation are used, adequate clinical response of both infections can be achieved with an acceptable safety profile. To avoid undesirable drug interactions and side effects in patients, anti TB treatment and antiretroviral therapy (ART) must be closely monitored.
Treatment of HIV-TB Co-infection
Drug Sensitive Tuberculosis
Treating TB disease benefits not only the individual patient but also the community as a whole. Patients with TB diseases must be diagnosed promptly, initiated on TB treatment early and complete the full prescribed course of the treatment. New patients with pulmonary TB should receive a regimen containing 6 months of rifampicin (RIF) with the use of fixed dose combination and daily dosing frequency. Patients with drug-susceptible TB and living with HIV also require only 6 months of RIF-containing ATT (4). In all HIV-infected patients with active TB, early diagnosis of TB and prompt initiation of ATT is advised. This should be followed by ART initiation, irrespective of CD4 T lymphocyte cell count. Physicians in developing countries prefer to start ART at least 2-8 weeks after initiation of ATT to avoid developing any IRIS (5–7).
The principles used to treat pulmonary tuberculosis also apply to extrapulmonary forms of the disease in general with the recommendation of 6 month treatment regimen.
Drug-Resistant Tuberculosis
For MDR/RR-TB patients, both shorter and longer treatment regimens are now available based on their previous history of TB treatment. The treatment duration varies from 9-11 months for newly diagnosed MDR-TB patients to 18–20 months long duration for highly drug-resistant TB patients - the treatment period may be extended based on the treatment response of the patient. A shorter MDR-TB regimen of 9–11 months shall be suggested in the place of the longer duration regimens in MDR or RR-TB patients, who were not treated earlier for more than a month with second-line drugs used in the shorter MDR-TB regimen or in those where resistance to fluoroquinolones and second-line injectable agents has been ruled out (8, 9). The anti-TB drugs used to constitute a regimen for MDR-TB management in people living with HIV (PLHIV) is same as for a non-HIV individual.
In the STREAM trial, one-third of the participants were HIV infected and the trial did not restrict participation of PLHIV based on their CD4 T lymphocyte cell count. The cause for the increased death seen among PLHIV in the trial group was not clear - the detailed assessment of the causes of death failed to provide any additional information that the shorter regimen was more detrimental to PLHIV either because of high pill burden or poor adherence to drugs, or drug-drug interactions between ATT and ART (10). Thus the shorter regimen has been suggested for use in PLHIV along with the timely initiation of ART as per World Health Organisation (WHO) guidelines. Besides the response to ART, its safety, tolerability and drug interaction should also be monitored closely at periodic intervals.
Nix-TB trial enrolled HIV-infected patients with a CD4 T lymphocyte cell count of more than 50 cells/µL and were on allowable ART. In the NIX-TB trial, owing to the small sample size it was not possible to perform any adjusted stratified analyses for PLHIV although they represented half of those enrolled. The study showed that in a substantial percentage of patients with extensive drug resistance, the combination of Bedaquiline (BDQ), Pretomanid (Pa), and linezolid (LZD) led to a sustained favorable outcome six months after treatment completion (11). With careful monitoring, few drug-drug interactions may be avoided by referring the website developed by University of Liverpool on HIV drug interactions (12). Thioacetazone (THz), should not be administered to HIV-infected individuals or those whose HIV status is not known because of the danger of Stevens-Johnson syndrome and toxic epidermal necrolysis. Efavirenz (EFV) significantly reduces Pa exposures – hence, an alternative antiretroviral drug must be taken into consideration if Pa or the BPaL regimen is being considered for the management of DR-TB in a PLHIV (13). ART using Zidovudine (AZT) as one of its drug combination should only be used with extreme caution as both AZT and LZD cause peripheral nerve toxicity and combine use may result in additive effect. Both the drugs are also known to cause myelosuppression in both HIV-infected and uninfected individuals.
Choice of ART in HIV TB Co-Infection
Antiretroviral, Integrase inhibitors (INSTIs), which mostly comprise raltegravir (RAL) and dolutegravir (DTG), have been shown to demonstrate good efficacy and safety, and are superior to Protease Inhibitors (PIs) when it comes to longevity (14). They reduce treatment discontinuation and provide a stronger higher genetic barrier to resistance than EFV-based ART (15). DTG, an integrase strand transfer inhibitor, is becoming more used as a first-line ART around the world (16). Preliminary pharmacokinetic data of the trials providing DTG-based ART with BDQ-containing regimens will throw further light on it (16). However, in low- and middle-income countries, where women of reproductive age make up a major proportion of the HIV-positive population, the lack of pregnancy safety evidence has also impeded the use of dolutegravir for all adults but the study done in Botswana found that women who started dolutegravir-based ART during pregnancy had no increased risk of bad birth outcomes, including severe unfavourable birth outcomes (17). Because it is well tolerated and has a low theoretical risk of medication interactions, the DTG fixed-dose combination has been recognized as a core component of ART regimen in the management of DR-TB and HIV co-infection. Due to increasing levels of transmitted Non-nucleoside Reverse Transcriptase Inhibitor (NNRTI) drug resistance in low- and middle-income countries, as well as its high barrier to resistance and improved tolerability compared to EFV, WHO recently recommended DTG as first-line therapy for the initiation of ART (18).
Protease inhibitors with ritonavir (PI/r) should be used in ART regimen in settings or countries where DTG is not available or risk of toxicity is high or there is high level of pre-treatment drug resistance i.e., ≥10 percent (18). The choice of PI/r will depend on the availability of the drug in the country’s programmatic setting. If HIV drug resistance testing is available, then that can also be used to decide on the selection of PI/r and design an ART regimen (18). Unlike EFV, DTG not only has fewer drug-drug interaction (19) but also exhibits higher genetic barrier to develop resistance (20). In addition to these, DTG is also effective against HIV-2 infection, which is resistant to EFV naturally (21–23). Pharmacokinetic studies of DTG with RIF containing ATT in HIV-TB co-infected adults showed the combination to be safe and efficacious (24, 25). As drug-drug interactions was noticed between RIF and DTG, it has been recommended to increase the dose of DTG to 50 mg two times a day. ATT with ART regimens containing the increased dosing of DTG was found to not only effectively suppress viral loads but achieve this faster and in shorter time along with improvement in CD4 T lymphocyte cell counts. Similarly, rifabutin and rifapentine in standard doses can be safely co-administered with DTG in PLHIV (25). Studies to understand the safety, efficacy and drug-drug interaction of other anti-tuberculosis drugs with DTG in PLHIVs with TB diseases are underway.
DTG in comparison with NNRTIs and boosted PIs, seem to have lower risk of drug-drug interaction with other drugs. Still DTG has to be used with caution or cannot be used with few drugs like anticonvulsants (e.g., phenytoin), antiarrhythmic drugs (e.g., dofetilide), antacids containing magnesium or calcium, laxatives and multivitamins. Concomitant use of antacids or laxative may result in sub-therapeutic DTG blood levels and hence if there is a need to combine these medications, then DTG should be administered at least two hours before or six hours after taking these medicines (12). The cations in these agents may act as a chelating agent resulting in sub-therapeutic levels of DTG in blood. In treatment-naive TB/HIV patients, the effects of INSTIs-based ART are equivalent to EFV-based ART, as shown in a meta-analysis of a randomised clinical trial. This supports the recommendation of INIs-based ART as first-line treatment in TB/HIV patients (26). When non-DTG-based ART is not effective in PLHIV, a combination of DTG with nucleoside reverse-transcriptase inhibitors (NRTI) can be recommended. Regimen with boosted protease inhibitors along with optimized NRTI drugs are recommended as preferred second-line regimen for PLHIV in whom DTG-based regimens are failing (18).
Tenofovir alafenamide fumarate (TAF) is the prodrug of Tenofovir (TDF), available as a single-strength tablet. It has been shown that TDF when combined with DTG or other INSTIs has improved renal and bone safety as compared to use of TDF alone. The limitations of taking TAF include an increase in blood cholesterol levels and body weight gain (27, 28). TAF has been shown to interact with anti-TB drugs including RIF. Uncertainity still exists about the exact dose of TAF that can be given along with ATT (29). Hence TDF is the preferred ARV drug for first-line regimens that can be combined with DTG and lamivudine (3TC). However, in special circumstances in adults like bony ailments like osteoporosis or kidney abnormalities like chronic renal disease or concomitant use of nephrotoxic drugs, TAF can be considered as an alternative option (30, 31).
Timing of ART Initiation
Antiretroviral medication should be initiated in all PLHIVs, especially those with TB coinfection. WHO recommends to start ART, regardless of CD4 T lymphocyte count, within two weeks of initiating ATT (27). In persons with CD4 T lymphocyte count ≤50 cells/mm3, a meta-analysis found that starting ART sooner (≤ four weeks) reduced death at one year. There was no evidence that early ART (≤ four weeks) was beneficial or harmful in persons with higher CD4 T lymphocyte counts (27).
Drug toxicity, drug-drug interactions (32), a patient’s perception of high pill burden, and IRIS (33, 34) are all factors to consider for not commencing ART early during TB treatment as all of the above may impair adherence to ART and retention in care (35). Reduced HIV consequences, such as progression to AIDS-defining illness and mortality (36, 37), and a faster HIV viral load reduction, both of which help patients and their partners by reducing HIV transmission, are two of the benefits of starting ART early during TB treatment (38). Early ART initiation may also ease program implementation and minimize unintentional delays caused while waiting for the results of CD4 T lymphocyte cell counts (39). Although IRIS, a hyperinflammatory response against latent infections that happens after the improvement in CD4 T lymphocyte count, was more likely in patients who initiated ART early, IRIS-related death was rare (40). Clinicians should be on the lookout for signs and symptoms of IRIS, and patients should be informed about the risk of acquiring it. Table 1 outlines the summary of the treatment for TB and HIV based on WHO guidelines.
Rifamycins and Antiretrovirals
Rifamycin (RMP) based TB regimens have been demonstrated to be most effective when given throughout TB treatment in HIV-infected individuals. If RMP is given only for the first two months of treatment, high relapse rates have been observed and a minimum of six months of RMP treatment is needed to achieve a cure. When treating HIV co-infected patients, some have recommended even longer treatment regimens of 8 months or more (41). Non-rifamycin-based regimens are thought to be less effective and also they extend the duration of TB therapy to 18–24 months and they are linked to increased toxicity and relapse rates. RIF is the most commonly used and widely available RMP.
In light of clinical relevance, a systematic review had shown that HIV infection increases the risk of low first line drugs exposure, which could have negative effects for treatment results (42). Patients with HIV infection have reduced plasma levels of first-line antitubercular medicines (INH, RIF, EMB, PYZ) when taken orally. Patients with advanced HIV illness and diarrhoea appear to have a higher risk of decreased drug levels (43).
RIF is a cytochrome P450 enzymatic inducer, which implies it would create pharmacokinetic drug-drug interactions. The level of CYP3A4 induction varies depending on the RMP drug taken (RIF > rifapentine > rifabutin) (44). Drug absorption in advanced HIV disease patients and drug-drug interactions are two major pharmacokinetic concerns raised by the use of RMP-based antitubercular regimens for HIV patients.
Interactions between RIF and two types of ARVs: NNRTIs and PIs are expected due to liver enzyme induction. Previously, nevirapine (NVP) was indicated as an NNRTI to be used in conjunction with TB treatment (45), but earlier meta-analysis suggests that NVP-based ART is less efficient than efavirenz-based ART (46, 47). As a result, patients with and without TB coinfection should avoid NVP-based combination therapy whenever possible. If NVP is used to treat HIV the lead-in once-daily dosing phase should be avoided and twice-daily dosing should be used throughout the treatment
If TB is diagnosed in patients on NVP-based ART, Rifabutin should be taken if it is available. If rifabutin is not available, NVP should be replaced with EFV 600 mg. When TB treatment is completed, NVP can be continued (5). Patients with TB who are currently taking many potentially hepatotoxic drugs should be especially concerned about the risk of NVP-related hepatitis (INH, RIF and PYZ). Few drug interaction, like the one between RIF and EFV (which causes a 20–25 percent reduction in area under the concentration-time curve (AUC) (48), donot have published clinical experience. In a thai study, patients on RIF based TB treatment and 600- or 800-mg doses of EFV exhibited equivalent EFV concentrations (49).
Other NNRTIs, such as Rilpivirine (RPV) (50), etravirine (ETR) (51), and doravirine (DOR), are largely metabolized by CYP3A4, and their concentrations are greatly reduced when combined with RIF (52), to the point that they ought not to be used together. DOR exposure is reduced by 50% when given with rifabutin, however, a twice a day DOR dose appears to adequately overcome the induction (53). According to physiologically based pharmacokinetic (PBPK) modeling, combining rifampicin with long-acting intramuscular RPV and cabotegravir could result in sub therapeutic drug concentrations in HIV-infected patients coinfected with TB, therefore these combinations should be avoided (54) Delavirdine (DLV) should not be taken at the same time. Except for zidovudine (ZDV) and enfuvirtide, the nucleoside analogs have no notable interactions with the RIF. NRTIs can be taken together without changing the dose.
When RIF is combined with RAL, RAL exposure is lowered by 40% and DTG AUC is reduced by 54% (55, 56). However, as observed in the Reflate TB trial (57), these medications are widely and efficiently used clinically at a regular dose of 400 mg two times a day or a double dose (800 mg two times a day) for RAL, and a double dose of 50 mg two times a day for DTG (24). Because both elvitegravir/cobicistat and bictegravir are CYP3A4 and UGT1A1 substrates, RIF substantially decreases their levels, making the concomitant usage inappropriate (58–60).
When PIs and RIF are taken together, serum levels of PIs can drop to sub therapeutic levels, while RIF serum levels can rise to dangerous levels. Rifampin significantly lowers the plasma levels of most of the PIs (AUC drops of 80%–95%), making their concurrent administration impossible or contraindicated (48). The drug ritonavir may be an exception to this rule.
(i) The use of RIF in combination with atazanavir (ATV) (300 mg per day) and ritonavir (100 mg per day) should not be used since ATV drug levels become subtherapeutic. RIF reduces the level of unboosted PIs significantly, hence it is not recommended for use with nelfinavir, indinavir, or ATV without ritonavir boosting. With RIF, high dosages of ritonavir can be utilized, albeit at the cost of increased hepatotoxicity. Indinavir, Saquinavir, Amprenavir, fosamprenavir, and Atazanavir/ritonavir should not be taken together. As rifabutin is a less effective enzyme inducer than RIF, it is the preferred medication in patients who are taking PIs.
(ii) Some drug drug interactivity, like the one between RIF and the PI nelfinavir (the outcome of which is an 80 percent–90 percent reduction in the AUC for nelfinavir (48), could very certainly lead to a significant decrease in antiviral activity and the evolution of resistance to nelfinavir and other ART medications.
(iii) For patients receiving both LPV and ritonavir (LPV/r) and RIF, dosages of LPV and ritonavir (LPV/r) must be greatly raised if rifabutin is not available (61). It is important to monitor liver enzymes.
(iv) Darunavir/ritonavir-adjusted dosages with RIF had an unacceptable risk of hepatotoxicity. The daily modified dose significantly reduced darunavir trough concentrations (62).
The appropriate dosing of DTG when used with RIF, a critical medicine used in the treatment of vast number of HIV TB co-infected persons in low middle income countries, is still unknown. RIF increases DTG metabolism and decreases drug levels by inducing the liver enzymes uridine glucuronosyltransferase 1A1 and cytochrome P450 3A4 (63). Although full 48-week results from the INSPIRING trial are pending, preliminary data shows that raising the dose of DTG to 50 mg twice a day when co-administered with RIF may be efficacious, risk- free, and tolerated for individuals co-infected with HIV and TB (24).
Alternative RMP, which produces less enzyme induction, is one way to reduce drug-drug interactions associated with RIF. Rifapentine is presently not suggested for usage in HIV-infected persons due to recurrences of tuberculosis with RMP mono drug-resistant strains when taken once a week during the continuation phase of TB treatment (64). Rifabutin has the lowest potential for improving antiretroviral metabolism of all the RMPs now available. Rifabutin is a CYP3A4 substrate and as a result, combining NNRTIs with PIs lowers rifabutin levels, necessitating rifabutin dose adjustments (48). However it can be given with raltegravir or dolutegravir, integrase strand transfer inhibitors, without requiring dose modifications. Rifabutin should be offered worldwide as a first-line rifamycin for HIV co-infected people and as a switch option for rifampicin-related ADRs (65). Unfortunately, TB control programs in most developing countries cannot employ this technique because rifabutin is now unreasonably costly, and broad adoption could necessitate significant decrease in price and moreover TB is treated with standard treatment regimens in high-burden countries, generally in fixed-dose combinations.
Bedaquiline and Antiretrovirals
BDQ (66), a novel diarylquinoline antimycobacterial, is the first new tuberculosis medicine officially agreed in almost 40 years for the therapy of both multidrug-resistant and extensively drug-resistant tuberculosis (67). Treatment with a BDQ along with LZD-containing regimen was connected to higher treatment outcomes and survival in PLHIV with coinfected MDR-TB (68). BDQ is metabolised to its active M2 metabolite by the cytochrome p450 isoenzyme 3A (CYP3A), that has lesser antimycobacterial activity but may produce a prolongation of QT effect (69). Co-administration of EFV with BDQ, on the other hand, leads in decreased BDQ concentrations because EFV increases CYP3A (67, 69). In a study of 30 healthy volunteers done by the AIDS Clinical Trials Group, co-administration of EFV with a BDQ single dose resulted in an eighteen percent decrease in the BDQ area under the curve (70).
Because NVP has a modest effect on BDQ concentrations, the major WHO recommendation based on these pharmacokinetic and modelling findings is to transition from EFV to NVP when BDQ is started (panel) (71). If switching from an EFV fixed-dose combination to multiple-pill, twice-daily NVP-based regimens results in worse adherence and worse HIV viral control, the overall benefit of BDQ could be endangered. NNRTI resistance may be selected for by poor ART adherence (due to higher pill burden or NVP-related adverse medication effects) (72).
NRTI is unlikely to influence BDQ concentrations (73). RPV is unlikely to have a significant effect on BDQ exposure at a common dose of 25 mg daily. A secondary effect on QT prolongation may be evident, mostly at supratherapeutic RPV dosages (74). RTV is an enzyme inhibitor and when BDQ is used with ritonavir-boosted lopinavir (LPV/r), there is a risk of considerable build-up of BDQ and its metabolites. As a result, this combination is not suggested (75).
As a result, PIs should be used with caution in combination with BDQ and with frequent electrocardiogram (ECG) monitoring, and should be restricted in the presence of alternate ART options availability, particularly true when used along with other QT-prolonging drugs. In DR-TB regimens, fluoroquinolones (especially moxifloxacin), Clofazimine (76), and Delamanid (DLM) (77), all of which cause QT prolongation, are frequently co-administered with BDQ, with the possibility of additive toxicity (78). However, new clinical evidence suggests that combining these drugs is relatively safe (79, 80) if periodic monitoring for QT interval prolongation is done as a routine and no other drug interactions are anticipated. During the use of all the above drugs, patients must be counselled on birth control and safe sexual practices as the safety of these drugs during pregnancy is not certain.
Other II-line Drugs and Antiretrovirals
Pre-XDR and XDR-TB cohorts of patients treated with LZD had a higher rate of culture conversion (81–84) than previously reported in XDR cohorts (81–84), and HIV-infected patients on ART were able to tolerate prolonged LZD exposure, with dose reduction in certain cases. Despite low CD4 T lymphocyte cell counts, HIV infection was not linked to a worse treatment response; however, all HIV-infected patients in this cohort were on first- or second-line ART and the majority had suppressed viral levels, which may have contributed to the positive interim results. All patients using linezolid should be monitored for specific adverse events regularly, and while these occurrences are not rare, they may be handled effectively in HIV-infected and uninfected patients, even on an ambulatory basis (85). There are significant pharmacodynamics interactions between LZD and ART, which may explain the HIV-infected patients experiencing greater LZD toxicity (85). Both LZD and ZDV cause anaemia, which can be worse when taken together; and the use of other NRTIs that inhibit mitochondrial gamma polymerase could increase LZD mitochondrial toxicity (86). Table 2 shows the Possible Drug-drug interactions of Bedaquiline and Linezolid with other drugs (8).
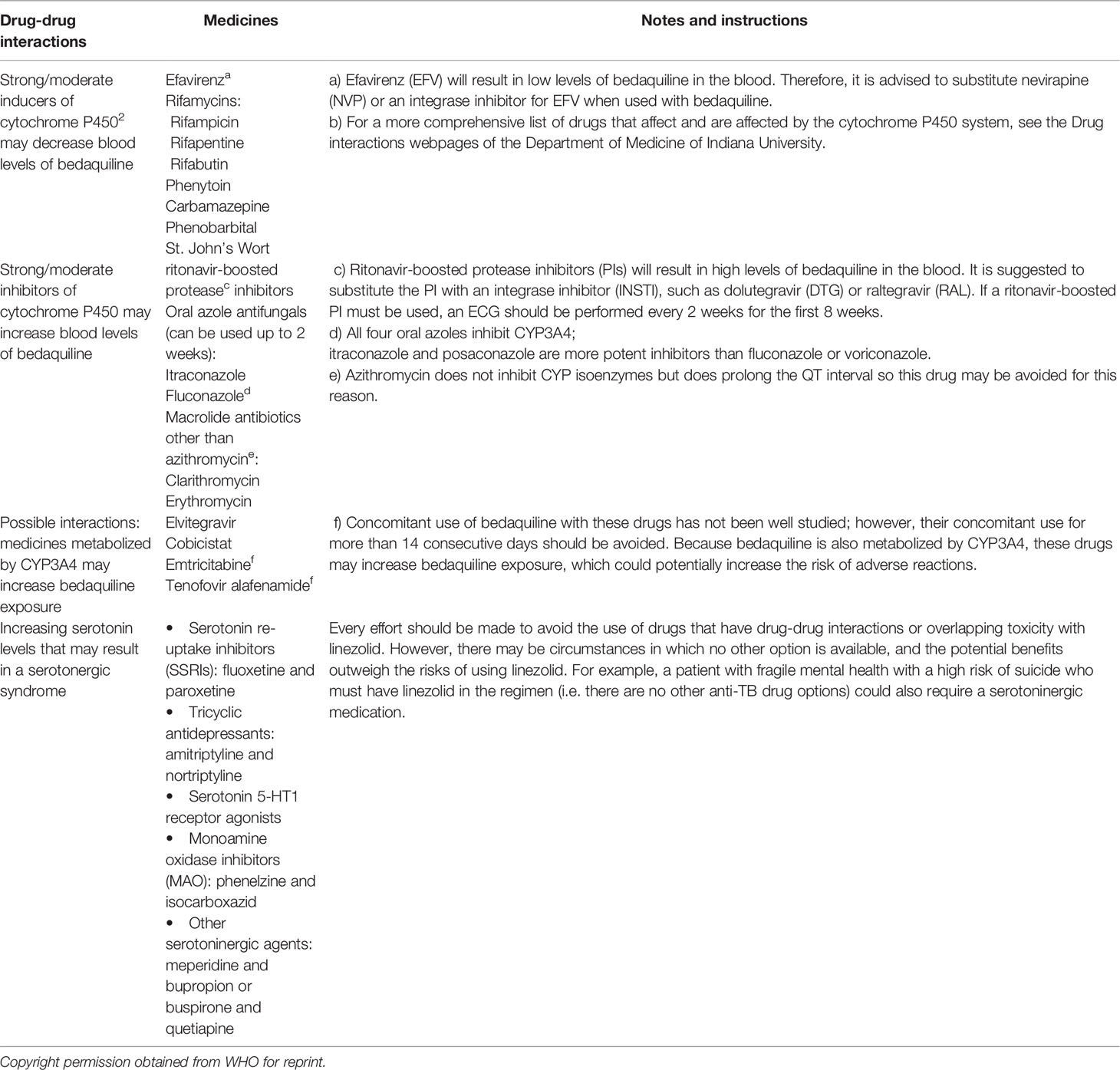
Table 2 Possible Drug-drug interactions of Bedaquiline and Linezolid with other drugs (8).
The DLM and Pretomanid (previously PA-824) nitroimidazoles are the next class of new anti-TB drugs to enter clinical use. DLM, like BDQ, does not affect drug transporters or CYP enzymes (87), and simultaneous use does not affect NNRTI or PI exposures (88). Clofazimine and ART have not been studied for drug-drug interactions, and no dose modifications are currently indicated. Table 3 portrays the adverse effects and overlapping toxicities of ATT and Antiretrovirals.
Adverse Reactions and Tuberculosis Immune Reconstitution Inflammatory Syndrome
Medication-induced liver injury has been linked to all antiretroviral drug classes and some anti-TB treatments such as pyrazinamide, isoniazid, and rifampicin (89, 90). When efavirenz and nevirapine were given together with TB treatment, there was an increase in hepatotoxicity (45). Alcoholism, co-infection with Hepatitis B and C, IRIS hepatitis, and obstruction by nodes at the porta hepatitis all lead to liver injury, as do shared metabolic pathways of anti-TB and antiretroviral medicines. Other side effects include cutaneous and gastrointestinal problems, as well as peripheral neuropathy, which can be severe at times (91). These side effects include drug rashes (which can occur with many TB drugs, as well as co-trimoxazole, nevirapine, and, less frequently, efavirenz), gastro-intestinal intolerance (especially with zidovudine, didanosine, protease inhibitors, pyrazinamide, ethionamide, and para-aminosalicylic acid), and neuropsychiatric side effects (especially with efavirenz, isoniazid, ethionamide and cycloserine) (92). Several factors found to be associated with poor treatment outcomes (default, death, loss to follow up) - smoking, history of hospitalization upon starting treatment, lower TB symptom score, internalized stigma (93), male patients and those with alcoholism (94), older age, HIV co-infection and rural residence (95), diabetes mellitus (96) should be addressed appropriately for better TB cure.
TB-IRIS is the paradoxical worsening of symptoms and signs of TB after starting ART (rarely with ATT itself), despite a favorable immunological recovery and effective virological suppression. TB-IRIS is of two types (1) Paradoxical TB-IRIS that occurs in HIV-TB co-infected patients started on ATT and subsequently started on ART. Paradoxical IRIS is relatively easy to diagnose because of its biphasic pattern of initial improvement with ATT followed by a latter phase “paradoxical” deterioration after ART initiation (2). Unmasking TB-IRIS or ART associated TB occurs in an asymptomatic individual without a prior diagnosis of TB who starts developing symptoms with ART initiation (97). Physicians should keep in mind that the start of this syndrome is associated to ART commencement, ART substitution (from I to II line reducing viremia), and ART cessation followed by re-initiation (91). Initiating ART before CD4 T lymphocyte cell counts drops considerably could protect patients against opportunistic infections and eventual IRIS (98). Anti-inflammatory medicines, particularly steroids, are the mainstay of treatment for TB-IRIS, while non-steroidal anti-inflammatory therapies may be effective as an initial treatment for milder and more localised cases of IRIS (99). A recent review on host directed therapies (HDT) to improve treatment outcome has recommended certain HDT strategies to be most appropriate against active TB and associated forms of TB (such as, TB-IRIS, TB-induced pulmonary diseases and extrapulmonary TB): eicosanoid modulators (NSAID and lipoxygenase inhibitors), inflammatory mediators (corticosteroids and tyrosine kinase inhibitors), metformin, ICIs, cytokine modulating therapy, statins, auranofin, cell-based therapies, miR and autophagy modulating drugs (100).
As novel HIV or TB treatment regimens are developed, the HIV-TB co-infected patient must be taken into account to appropriately examine the drug-drug and drug-disease interactions that influence dose, safety, and response. To reduce TB-related mortality among HIV-TB co-infected patients, ART and ATT outcomes must improve and it is critical to conduct pharmacokinetic (PK) and pharmacodynamic (PD) studies to determine the amount of these drug interactions and their impact on treatment outcomes in these patients including children and women of child bearing age group. Clinical practise should prioritise strategies to promote adherence, such as reducing treatment duration, monitoring and treating adverse events, and improving treatment success rates, to reduce the mortality risk of HIV/TB coinfection.
Author Contributions
NP and CP conceived, designed, conducted literature search, data extraction and manuscript writing. All authors contributed to the article and approved the submitted version.
Conflict of Interest
The authors declare that the research was conducted in the absence of any commercial or financial relationships that could be construed as a potential conflict of interest.
Publisher’s Note
All claims expressed in this article are solely those of the authors and do not necessarily represent those of their affiliated organizations, or those of the publisher, the editors and the reviewers. Any product that may be evaluated in this article, or claim that may be made by its manufacturer, is not guaranteed or endorsed by the publisher.
References
1. Global Tuberculosis Report 2020 (2020). Geneva: World Health Organization. Available at: https://apps.who.int/iris/bitstream/handle/10665/336069/9789240013131-eng.pdf (Accessed on 15/09/2021).
2. Bell LCK, Noursadeghi M. Pathogenesis of HIV-1 and Mycobacterium Tuberculosis Co-Infection. Nat Rev Microbiol (2018) 16(2):80–90. doi: 10.1038/nrmicro.2017.128
3. Chen C, Zhu T, Wang Z, Peng H, Kong W, Zhou Y, et al. High Latent TB Infection Rate and Associated Risk Factors in the Eastern China of Low TB Incidence. PloS One (2015) 10(10):e0141511. doi: 10.1371/journal.pone.0141511
4. Guidelines for Treatment of Drug-Susceptible Tuberculosis and Patient Care, 2017 Update (2017). Geneva: World Health Organization (Accessed on 1/09/2021). Licence: CC BY-NC-SA 3.0 IGO.
5. Abdool Karim SS, Naidoo K, Grobler A, Padayatchi N, Baxter C, Gray AL, et al. Integration of Antiretroviral Therapy With Tuberculosis Treatment. N Engl J Med (2011) 365(16):1492–501. doi: 10.1056/NEJMoa1014181
6. Havlir DV, Kendall MA, Ive P, Kumwenda J, Swindells S, Qasba SS, et al. Timing of Antiretroviral Therapy for HIV-1 Infection and Tuberculosis. N Engl J Med (2011) 365(16):1482–91. doi: 10.1056/NEJMoa1013607
7. Blanc FX, Sok T, Laureillard D, Borand L, Rekacewicz C, Nerrienet E, et al. Earlier Versus Later Start of Antiretroviral Therapy in HIV-Infected Adults With Tuberculosis. N Engl J Med (2011) 365(16):1471–81. doi: 10.1056/NEJMoa1013911
8. WHO Consolidated Guidelines on Tuberculosis. Module 4: Treatment - Drug-Resistant Tuberculosis Treatment. Geneva: World Health Organization (2020). Available at: https://www.who.int/publications-detail-redirect/9789240007048.
9. WHO Consolidated Guidelines on Drug-Resistant Tuberculosis Treatment (2019). Geneva: World Health Organization. Available at: https://apps.who.int/iris/bitstream/handle/10665/311389/9789241550529-eng.pdf?ua=1 (Accessed on 15/09/2021).
10. Position Statement on the Continued Use of the Shorter MDR-TB Regimen Following an Expedited Review of the STREAM Stage 1 Preliminary Results (2018). Geneva: World Health Organization. Available at: https://www.who.int/tb/areas-of-work.drug-resistant-tb/treatment/WHO.PositionStatementShorterRegimensSTREAMStage1.pdf (Accessed 15 September 2021).
11. Conradie F, Diacon AH, Ngubane N, Howell P, Everitt D, Crook AM, et al. Treatment of Highly Drug-Resistant Pulmonary Tuberculosis. N Engl J Med (2020) 382(10):893–902. doi: 10.1056/NEJMoa1901814
12. HIV drug interactions. Liverpool, United Kingdom (2020). University of Liverpool. Available at: https://www.hiv-druginteractions.org/checker (Accessed 15 September 2021).
13. Drug Approval Package: Pretomanid (2019). Maryland, United States of America: US Food and Drug Administration. Available at: https://www.accessdata.fda.gov/drugsatfda_docs/nda/2019/212862Orig1s000TOC.cfm (Accessed 15 September 2021).
14. Stecher M, Schommers P, Kollan C, Stoll M, Kuhlendahl F, Stellbrink HJ, et al. Treatment Modification After Starting cART in People Living With HIV: Retrospective Analysis of the German ClinSurv HIV Cohort 2005–2017. Infection (2020) 48(5):723–33. doi: 10.1007/s15010-020-01469-6
15. Walmsley SL, Antela A, Clumeck N, Duiculescu D, Eberhard A, Gutierrez F, et al. Dolutegravir Plus Abacavir-Lamivudine for the Treatment of HIV-1 Infection. N Engl J Med (2013) 369(19):1807–18. doi: 10.1056/NEJMoa1215541
16. Dorward J, Lessells R, Drain PK, Naidoo K, de Oliveira T, Pillay Y, et al. Dolutegravir for First-Line Antiretroviral Therapy in Low-Income and Middle-Income Countries: Uncertainties and Opportunities for Implementation and Research. Lancet HIV (2018) 5:e400–04. doi: 10.1016/S2352-3018(18)30093-6
17. Zash R, Jacobson DL, Diseko M, Mayondi G, Mmalane M, Essex M, et al. Comparative Safety of Dolutegravir-Based or Efavirenz-Based Antiretroviral Treatment Started During Pregnancy in Botswana: An Observational Study. Lancet Glob Health (2018) 6(7):e804–10. doi: 10.1016/S2214-109X(18)30218-3
18. Consolidated Guidelines on HIV Prevention, Testing, Treatment, Service Delivery, and Monitoring: Recommendations for a Public Health Approach. Geneva: World Health Organization (2021). License: CC BY-NC-SA 3.0 IGO.
19. Cottrell ML, Hadzic T, Kashuba AD. Clinical Pharmacokinetics, Pharmacodynamic and Drug-Interaction Profile of the Integrase Inhibitor Dolutegravir. Clin Pharmacokinet (2013) 52:981–94. doi: 10.1007/s40262-013-0093-2
20. Llibre JM, Pulido F, Garcia F, Garcia Deltoro M, Blanco JL, Delgado R. Genetic Barrier to Resistance for Dolutegravir. AIDS Rev (2015) 17(1):56–64.
21. Descamps D, Peytavin G, Visseaux B, Tubiana R, Damond F, Campa P, et al. Dolutegravir in HIV-2–Infected Patients With Resistant Virus to First-Line Integrase Inhibitors From the French Named Patient Program. Clin Infect Dis (2015) 60:1521–7. doi: 10.1093/cid/civ124
22. Smith RA, Raugi DN, Pan C, Sow PS, Seydi M, Mullins JI, et al. In Vitro Activity of Dolutegravir Against Wild-Type and Integrase Inhibitor-Resistant HIV-2. Retrovirology (2015) 12:10. doi: 10.1186/s12977-015-0146-8
23. Trevino A, Cabezas T, Lozano AB, Garcia-Delgado R, Force L, Fernandez-Montero JM, et al. Dolutegravir for the Treatment of HIV-2 Infection. J Clin Virol (2015) 64:12–5. doi: 10.1016/j.jcv.2015.01.001
24. Dooley KE, Kaplan R, Mwelase N, Grinsztejn B, Ticona E, Lacerda M, et al. Dolutegravir Based Antiretroviral Therapy for Patients Co-Infected With Tuberculosis and HIV: A Multicenter, Non-Comparative, Open-Label, Randomized Trial. Clin Infect Dis (2020) 70:549–56. doi: 10.1093/cid/ciz256.
25. Dooley KE, Sayre P, Borland J, Purdy E, Chen S, Song I, et al. Safety, Tolerability, and Pharmacokinetics of the HIV Integrase Inhibitor Dolutegravir Given Twice Daily With Rifampin or Once Daily With Rifabutin: Results of a Phase 1 Study Among Healthy Subjects. J Acquir Immune Defic Syndr (2013) 62:21–7. doi: 10.1097/QAI.0b013e318276cda9
26. Shu Y, Deng Z, Wang H, Chen Y, Yuan L, Deng Y, et al. Integrase Inhibitors Versus Efavirenz Combination Antiretroviral Therapies for TB/HIV Coinfection: A Meta-Analysis of Randomized Controlled Trials. AIDS Res Ther (2021) 18:25. doi: 10.1186/s12981-021-00348-w
27. Guidelines: Updated Recommendations on HIV Prevention, Infant Diagnosis, Antiretroviral Initiation, and Monitoring (2021). Geneva: World Health Organization. Available at: https://apps.who.int/iris/handle/10665/340190 (Accessed 15 September 2021).
28. Gazzola L, Tagliaferri G, Mondatore D, De Bona A, Borsalino C, Bini T, et al. Increases in Lipid Profile After Switching From TDF to TAF-Based HAART Regimens in a Cohort of HIV-Positive Patients: Is it Clinically Relevant? 2018 International Congress on Drug Therapy in HIV Infection, Glasgow . United Kingdom. Available at: https://hivglasgow.org/wpcontent/uploads/2018/11/P187.pdf (Accessed 15 September 2021).
29. Cerrone M, Alfarisi O, Neary M, Marzinke MA, Parsons TL, Owen A, et al. Rifampicin Effect on Intracellular and Plasma Pharmacokinetics of Tenofovir Alafenamide. J Antimicrob Chemother (2019) 74:1670–8. doi: 10.1093/jac/dkz068
30. Pozniak A, Arribas JR, Gathe J, Gupta SK, Post FA, Bloch M, et al. Switching to Tenofovir Alafenamide, Coformulated With Elvitegravir, Cobicistat, and Emtricitabine, in HIV-Infected Patients With Renal Impairment: 48-Week Results From a Single-Arm, Multicenter, Open-Label Phase 3 Study. J Acquir Immune Defic Syndr (2016) 71:530. doi: 10.1097/QAI.0000000000000908
31. Walti LN, Steinrucken J, Rauch A, Wandeler G. Tenofovir Alafenamide in Multimorbid HIV-Infected Patients With Prior Tenofovir-Associated Renal Toxicity. Open Forum Infect Dis (2018) 5:ofy275. doi: 10.1093/ofid/ofy275
32. Cerrone M, Bracchi M, Wasserman S, Pozniak A, Meintjes G, Cohen K, et al. Safety Implications of Combined Antiretroviral and Anti-Tuberculosis Drugs. Expert Opin Drug Saf (2020) 19(1):23–41. doi: 10.1080/14740338.2020.1694901
33. Müller M, Wandel S, Colebunders R, Attia S, Furrer H, Egger M, et al. Immune Reconstitution Inflammatory Syndrome in Patients Starting Antiretroviral Therapy for HIV Infection: A Systematic Review and Meta-Analysis. Lancet Infect Dis (2010) 10(4):251–61. doi: 10.1016/S1473-3099(10)70026-8
34. Walker NF, Scriven J, Meintjes G, Wilkinson RJ. Immune Reconstitution Inflammatory Syndrome in HIV-Infected Patients. HIV AIDS (2015) 12(7):49–64. doi: 10.2147/HIV.S42328
35. Nachega JB, Morroni C, Chaisson RE, Goliath R, Efron A, Ram M, et al. Impact of Immune Reconstitution Inflammatory Syndrome on Antiretroviral Therapy Adherence. Patient Prefer Adherence (2012) 6(101475748):887–91. doi: 10.2147/PPA.S38897
36. Yan S, Chen L, Wu W, Fu Z, Zhang H, Li Z, et al. Early Versus Delayed Antiretroviral Therapy for HIV and Tuberculosis Co-Infected Patients: A Systematic Review and Meta-Analysis of Randomized Controlled Trials. PloS One (2015) 10(5):e0127645. doi: 10.1371/journal.pone.0127645
37. Uthman OA, Okwundu C, Gbenga K, Volmink J, Dowdy D, Zumla A, et al. Optimal Timing of Antiretroviral Therapy Initiation for HIV-Infected Adults With Newly Diagnosed Pulmonary Tuberculosis: A Systematic Review and Meta-Analysis. Ann Intern Med (2015) 163(1):32–9. doi: 10.7326/M14-2979
38. Cohen MS, Chen YQ, McCauley M, Gamble T, Hosseinipour MC, Kumarasamy N, et al. Antiretroviral Therapy for the Prevention of HIV-1 Transmission. N Engl J Med (2016) 375(9):830–9. doi: 10.1056/NEJMoa1600693
39. Tweya H, Ben-Smith A, Kalulu M, Jahn A, Ng’ambi W, Mkandawire E, et al. Timing of Antiretroviral Therapy and Regimen for HIV-Infected Patients With Tuberculosis: The Effect of Revised HIV Guidelines in Malawi. BMC Public Health (2014) 14(1):183. doi: 10.1186/1471-2458-14-183
40. Burke RM, Rickman HM, Singh V, Corbett EL, Ayles H, Jahn A, et al. What Is the Optimum Time to Start Antiretroviral Therapy in People With HIV and Tuberculosis Coinfection? A Systematic Review and Meta-Analysis. J Int AIDS Soc (2021) 24(7):e25772. doi: 10.1002/jia2.25772
41. Khan FA, Minion J, Pai M, Royce S, Burman W, Harries AD, et al. Treatment of Active Tuberculosis in HIV-Coinfected Patients: A Systematic Review and Meta-Analysis. Clin Infect Dis (2010) 50(9):1288–99. doi: 10.1086/651686
42. Daskapan A, Idrus LR, Postma MJ, Wilffret B, Kosterink JGW, Stienstra Y, et al. A Systematic Review on the Effect of HIV Infection on the Pharmacokinetics of First-Line Tuberculosis Drugs. Clin Pharmacokinet (2019) 58(6):747–66. doi: 10.1007/s40262-018-0716-8
43. Gurumurthy P, Ramachandran G, Hemanth Kumar AK, Rajasekaran S, Padmapriyadarsini C, Swaminathan S, et al. Decreased Bioavailability of Rifampin and Other Antituberculosis Drugs in Patients With Advanced Human Immunodeficiency Virus Disease. Antimicrob Agents Chemother (2004) 48(11):4473–5. doi: 10.1128/AAC.48.11.4473-4475.2004
44. Sahasrabudhe V, Zhu T, Vaz A, Tse S. Drug Metabolism and Drug Interactions: Potential Application to Antituberculosis Drugs. J Infect Dis (2015) 211(Suppl 3):S107–14. doi: 10.1093/infdis/jiv009
45. Shipton LK, Wester CW, Stock S, Ndwapi N, Gaolathe T, Thior I, et al. Safety and Efficacy of Nevirapine- and Efavirenz-Based Antiretroviral Treatment in Adults Treated for TB-HIV Co-Infection in Botswana. Int J Tuberc Lung Dis (2009) 13(3):360–6.
46. Swaminathan S, Padmapriyadarsini C, Venkatesan P, Narendran G, Ramesh Kumar S, Iliayas S, et al. Efficacy and Safety of Once-Daily Nevirapine- or Efavirenz-Based Antiretroviral Therapy in HIV-Associated Tuberculosis: A Randomized Clinical Trial. Clin Infect Dis (2011) 53:716–24. doi: 10.1093/cid/cir447
47. Bhatt NB, Baudin E, Meggi B, da Silva C, Barrail-Tran A, Furlan V, et al. Nevirapine or Efavirenz for Tuberculosis and HIV Coinfected Patients: Exposure and Virological Failure Relationship. J Antimicrob Chemother (2015) 70(1):225–32. doi: 10.1093/jac/dku348
48. Centers for Disease Control and Prevention. Updated Guidelines for the Use of Rifamycins for the Treatment of Tuberculosis Among HIV-Infected Patients Taking Protease Inhibitors or Nonnucleoside Reverse Transcriptase Inhibitors . Available at: http://www.cdc.gov/tb/TB_HIV_Drugs/default.htm (Accessed on 15/09/2021).
49. Manosuthi, Weerawata ,B, Sungkanuparph S, Thakkinstian A, Vibhagool A, Kiertiburanakul S, et al. Efavirenz Levels and 24-Week Efficacy in HIV-Infected Patients With Tuberculosis Receiving Highly Active Antiretroviral Therapy and Rifampicin. AIDS (2005) 19(14):1481–6. doi: 10.1097/01.aids.0000183630.27665.30
50. Crauwels H, van Heeswijk RP, Stevens M, Buelens A, Vanveggel S, Boven K, et al. Clinical Perspective on Drug-Drug Interactions With the Non-Nucleoside Reverse Transcriptase Inhibitor Rilpivirine. AIDS Rev (2013) 15(2):87–101.
51. Kakuda TN, Schöller-Gyüre M, Hoetelmans RMW. Pharmacokinetic Interactions Between Etravirine and Non-Antiretroviral Drugs. Clin Pharmacokinet (2011) 50:25–39. doi: 10.2165/11534740-000000000-00000
52. Yee KL, Khalilieh SG, Sanchez RI, Liu R, Anderson MS, Manthos H, et al. The Effect of Single and Multiple Doses of Rifampin on the Pharmacokinetics of Doravirine in Healthy Subjects. Clin Drug Investig (2017) 37(7):659–67. doi: 10.1007/s40261-017-0513-4
53. Khalilieh SG, Yee KL, Sanchez RI, Liu R, Fan L, Martell M, et al. Multiple Doses of Rifabutin Reduce Exposure of Doravirine in Healthy Subjects. J Clin Pharmacol (2018) 58(8):1044–52. doi: 10.1002/jcph.1103
54. Rajoli RKR, Curley P, Chiong J, Back D, Flexner C, Owen A, et al. Predicting Drug-Drug Interactions Between Rifampicin and Long-Acting Cabotegravir and Rilpivirine Using Physiologically Based Pharmacokinetic Modeling. J Infect Dis (2019) 219(11):1735–42. doi: 10.1093/infdis/jiy726
55. Wenning LA, Hanley WD, Brainard DM, Petry AS, Ghosh K, Jin B, et al. Effect of Rifampin, a Potent Inducer of Drug-Metabolizing Enzymes, on the Pharmacokinetics of Raltegravir. Antimicrob Agents Chemother (2009) 53(7):2852–56. doi: 10.1128/AAC.01468-08
56. ViiV Healthcare UK Ltd. Tivicay, Summary of Product Characteristics. Available at: https://www.medicines.org.uk/emc/product/5248/smpc.
57. Grinsztejn B, De Castro N, Arnold V, Veloso VG, Morgado M, Pilotto JH, et al. Raltegravir for the Treatment of Patients Co-Infected With HIV and Tuberculosis (ANRS 12 180 Reflate TB): A Multicentre, Phase 2, non-Comparative, Open-Label, Randomized Trial. Lancet Infect Dis (2014) 14(6):459–67. doi: 10.1016/S1473-3099(14)70711-X
58. Gilead Sciences Ltd. Stribild - Summary of Product Characteristics (2019). Available at: https://www.medicines.org.uk/emc/product/3154/smpc.
59. Custodio JM, West SK, Collins S, Vu A, Xiao D, Martin H, et al. Pharmacokinetics of Bictegravir Administered Twice Daily in Combination With Rifampin. In 25th Conference Retroviruses Opportunistic Infections. Boston: CROI (2018).
60. Gilead Sciences Ltd. Biktarvy - Summary of Product Characteristics . Available at: https://www.medicines.org.uk/emc/product/9313/smpc.
61. Decloedt EH, McIlleron H, Smith P, Merry C, Orrell C, Maartens G. Pharmacokinetics of Lopinavir in HIV-Infected Adults Receiving Rifampin With Adjusted Doses of Lopinavir-Ritonavir Tablets. Antimicrob Agents Chemother (2011) 55(7):3195–200. doi: 10.1128/AAC.01598-10
62. Ebrahim I, Maartens G, Wiesner L, Orrell C, Smythe W, McIlleron H. Pharmacokinetic Profile and Safety of Adjusted Doses of Darunavir/Ritonavir With Rifampicin in People Living With HIV. J Antimicrob Chemother (2020) 75(4):1019–25. doi: 10.1093/jac/dkz522
63. Maartens G, Boffito M, Flexner CW. Compatibility of Next-Generation First-Line Antiretrovirals With Rifampicin-Based Antituberculosis Therapy in Resource-Limited Settings. Curr Opin HIV AIDS (2017) 12:355–8. doi: 10.1097/COH.0000000000000376
64. Vernon A, Burman W, Benator D, Khan A, Bozeman L. Acquired Rifamycin Monoresistance in Patients With HIV-Related Tuberculosis Treated With Once-Weekly Rifapentine and Isoniazid. Tuberculosis Trials Consortium Lancet (1999) 353(9167):1843–7. doi: 10.1016/s0140-6736(98)11467-8
65. Rockwood N, Cerrone M, Barber M, Hill AM, Pozniak AL. Global Access of Rifabutin for the Treatment of Tuberculosis - Why Should We Prioritize This? J Int AIDS Soc (2019) 22(7):e25333. doi: 10.1002/jia2.25333
66. Diacon AH, Pym A, Grobusch MP, de los Rios JM, Gotuzzo E, Vasilyeva I, et al. Multidrug-Resistant Tuberculosis and Culture Conversion With Bedaquiline. N Engl J Med (2014) 371:723–32. doi: 10.1056/NEJMoa1313865
67. Zumla A, Nahid P, Cole ST. Advances in the Development of New Tuberculosis Drugs and Treatment Regimens. Nat Rev Drug Discov (2013) 12(5):388–404. doi: 10.1038/nrd4001
68. Padayatchi N, Bionghi N, Osman F, Naidu N, Ndjeka N, Master I, et al. Treatment Outcomes in Patients With Drug-Resistant TB-HIV Co-Infection Treated With Bedaquiline and Linezolid. Int J Tuberc Lung Dis (2020) 24(10):1024–31. doi: 10.5588/ijtld.20.0048
69. van Heeswijk RP, Dannemann B, Hoetelmans RM. Bedaquiline: A Review of Human Pharmacokinetics and Drug-Drug Interactions. J Antimicrob Chemother (2014) 69:2310–18. doi: 10.1093/jac/dku171
70. Dooley KE, Park JG, Swindells S, Allen R, Haas DW, Cramer Y, et al. Safety, Tolerability, and Pharmacokinetic Interactions of the Antituberculous Agent TMC207 (Bedaquiline) With Efavirenz in Healthy Volunteers: AIDS Clinical Trials Group Study A5267. J Acquir Immune Defic Syndr (2012) 59(5):455–62. doi: 10.1097/QAI.0b013e3182410503
71. WHO. Companion Handbook to the WHO Guidelines for the Programmatic Management of Drug-Resistant Tuberculosis. Geneva: World Health Organization (2014).
72. Brill MJ, Svensson EM, Pandie M, Maartens G, Karlsson MO. Confirming Model-Predicted Pharmacokinetic Interactions Between Bedaquiline and Lopinavir/Ritonavir or Nevirapine in Patients With HIV and Drug-Resistant Tuberculosis. Int J Antimicrob Agents (2017) 49:212–17. doi: 10.1016/j.ijantimicag.2016.10.020
73. Svensson EM, Aweeka F, Park JG, Marzan F, Dooley KE, Karlsson MO. Model-Based Estimates of the Effects of Efavirenz on Bedaquiline Pharmacokinetics and Suggested Dose Adjustments for Patients Coinfected With HIV and Tuberculosis. Antimicrob Agents Chemother (2013) 57(6):2780–7. doi: 10.1128/AAC.00191-13
74. Sharma M, Saravolatz LD. Rilpivirine: A New non-Nucleoside Reverse Transcriptase Inhibitor. J Antimicrob Chemother (2013) 68:250–56. doi: 10.1093/jac/dks404
75. Pandie M, Wiesner L, McIlleron H, Hughes J, Siwendu S, Conradie F, et al. Drug-Drug Interactions Between Bedaquiline and the Antiretrovirals Lopinavir/Ritonavir and Nevirapine in HIV-Infected Patients With Drug-Resistant TB. J Antimicrob Chemother (2016) 71(4):1037–40. doi: 10.1093/jac/dkv447
76. Diacon AH, Dawson R, von Groote-Bidlingmaier F, Symons G, Venter A, Donald PR, et al. Bactericidal Activity of Pyrazinamide and Clofazimine Alone and in Combinations With Pretomanid and Bedaquiline. Am J Respir Crit Care Med (2015) 191(8):943–53. doi: 10.1164/rccm.201410-1801OC
77. Szumowski JD, Lynch JB. Profile of Delamanid for the Treatment of Multidrug-Resistant Tuberculosis. Drug Des Devel Ther (205) 9: 677.
78. Dannemann B, Bakare N, De Marez T, Lounis N, van Heeswijk R, Meyvisch P. QTcF Prolongation in a Phase II Trial of TMC207 Plus Background Regimen as a Treatment for Multidrug-Resistant Tuberculosis: Effect of Co-Administration With Clofazimine 52nd Intersci. In: 52nd Interscience Conference on Antimicrobial Agents and Chemotherapy. San Fransico, CA, USA (2012). pp. 9–12.
79. Ferlazzo G, Mohr E, Laxmeshwar C, Hewison C, Hughes J, Jonckheere S, et al. Early Safety and Efficacy of the Combination of Bedaquiline and Delamanid for the Treatment of Patients With Drug-Resistant Tuberculosis in Armenia, India, and South Africa: A Retrospective Cohort Study. Lancet Infect Dis (2018) 18:536–44. doi: 10.1016/S1473-3099(18)30100-2
80. Dooley KE, Rosenkranz SL, Conradie F, Moran LE, Hafner R, von Groote-Bidlingmaier F, et al. QT Effects of Bedaquiline, Delamanid or Both in MDR-TB Patients : The Deliberate Trial. In Conference on Retroviruses and Opportunistic Infections (Vol. 5). Seatle: CROI (2019).
81. Dheda K, Shean K, Zumla A, Badri M, Streicher EM, Page-Shipp L, et al. Early Treatment Outcomes and HIV Status of Patients With Extensively Drug-Resistant Tuberculosis in South Africa: A Retrospective Cohort Study. Lancet (2010) 375:1798–807. doi: 10.1016/S0140-6736(10)60492-8
82. Jacobson KR, Tierney DB, Jeon CY, Mitnick CD, Murray MB. Treatment Outcomes Among Patients With Extensively Drug-Resistant Tuberculosis: Systematic Review and Meta-Analysis. Clin Infect Dis (2010) 51(1):6–14. doi: 10.1086/653115
83. Migliori GB, Sotgiu G, Gandhi NR, Falzon D, DeRiemer K, Centis R, et al. Drug Resistance Beyond Extensively Drug-Resistant Tuberculosis: Individual Patient Data Meta-Analysis. Eur Respir J (2013) 42(1):169–79. doi: 10.1183/09031936.00136312
84. Falzon D, Gandhi N, Migliori GB, Sotgiu G, Cox HS, Holtz TH, et al. Resistance to Fluoroquinolones and Second-Line Injectable Drugs: Impact on MDR-TB Outcomes. Eur Respir J (2013) 42(1):156–68. doi: 10.1183/09031936.00134712
85. Hughes J, Isaakidis P, Andries A, Mansoor H, Cox V, Meintjes G, et al. Linezolid for Multidrug-Resistant Tuberculosis in HIV-Infected and -Uninfected Patients. Eur Respir J (2015) 46(1):271–4. doi: 10.1183/09031936.00188114
86. Wasserman S, Meintjes G, Maartens G. Linezolid in the Treatment of Drug-Resistant Tuberculosis: The Challenge of Its Narrow Therapeutic Index. Expert Rev Anti Infect Ther (2016) 14(10):901–15. doi: 10.1080/14787210.2016
87. Shimokawa Y, Sasahara K, Yoda N, Mizuno K, Umehara K. Delamanid Does Not Inhibit or Induce Cytochrome P450 Enzymes In Vitro. Biol Pharm Bull (2014) 37(11):1727–35. doi: 10.1248/bpb.b14-00311
88. Mallikaarjun S, Wells C, Petersen C, Paccaly A, Shoaf SE, Patil S, et al. Delamanid Coadministered With Antiretroviral Drugs or Antituberculosis Drugs Shows No Clinically Relevant Drug-Drug Interactions in Healthy Subjects. Antimicrob Agents Chemother (2016) 60(10):5976–85. doi: 10.1128/AAC.00509-16
89. Nunez M. Clinical Syndromes and Consequences of Antiretroviral-Related Hepatotoxicity. Hepatology (2010) 52:1143–55. doi: 10.1002/hep.23716
90. Schutz C, Ismail Z, Proxenos CJ, Marais S, Burton R, Kenyon C, et al. Burden of Antituberculosis and Antiretroviral Drug-Induced Liver Injury at a Secondary Hospital in South Africa. S Afr Med J (2012) 102(6):506–11. doi: 10.7196/samj.5650
91. Narendran G, Swaminathan S. Tuberculosis Immune Reconstitution Inflammatory Syndrome: Profile of an Enigmatic Condition. Curr Sci (2013) 105(5):657–65.
92. Coyne KM, Pozniak AL, Lamorde M, Boffito M. Pharmacology of Second-Line Antituberculosis Drugs and Potential for Interactions With Antiretroviral Agents. AIDS (2009) 23(4):437–46. doi: 10.1097/qad.0b013e328326ca50
93. Suliman Q, Lim PY, Md Said S, Tan KA, Md Zulkefli NA, et al. Risk Factors for Early TB Treatment Interruption Among Newly Diagnosed Patients in Malaysia. Sci Rep (2022) 12:745. doi: 10.1038/s41598-021-04742-2
94. Ragan EJ, Kleinman MB, Sweigart B, Gnatienko N, Parry CD, Horsburghet CR, et al. The Impact of Alcohol Use on Tuberculosis Treatment Outcomes: A Systematic Review and Meta-Analysis. Int J Tuberc Lung Dis (2020) 24(1):73–82. doi: 10.5588/ijtld.19.0080
95. Ifebunandu NA, Ukwaja KN. Tuberculosis Treatment Default in a Large Tertiary Care Hospital in Urban Nigeria: Prevalence, Trend, Timing and Predictors. J Infect Public Health (2012) 5(5):340–5. doi: 10.1016/j.jiph.2012.06.002
96. Huangfu P, Ugarte-Gil C, Golub J, Pearson F, Critchley J. The Effects of Diabetes on Tuberculosis Treatment Outcomes: An Updated Systematic Review and Meta-Analysis. Int J Tuberc Lung Dis (2019) 23(7):783–96. doi: 10.5588/ijtld.18.0433
97. Meintjes G, Lawn SD, Scano F, Maartens G, French M A, Worodria W, et al. Tuberculosis-Associated Immune Reconstitution Inflammatory Syndrome: Case Definitions for Use in Resource-Limited Settings. Lancet Infect Dis (2008) 8(8):516–23. doi: 10.1016/S1473-3099(08)70184-1
98. Breton G, Duval X, Estellat C, Poaletti X, Bonnet D, Mvondo DM, et al. Determinants of Immune Reconstitution Inflammatory Syndrome in HIV Type 1-Infected Patients With Tuberculosis After Initiation of Antiretroviral Therapy. Clin Infect Dis (2004) 39(11):1709–12. doi: 10.1086/425742
99. Meintjes G, Wilkinson RJ, Morroni C, Pepper DJ, Rebe K, Rangaka MX, et al. Randomized Placebo-Controlled Trial of Prednisone for Paradoxical Tuberculosis-Associated Immune Reconstitution Inflammatory Syndrome. AIDS (2010) 24(15):2381–90. doi: 10.1097/QAD.0b013e32833dfc68
Keywords: antiretroviral therapy, anti tuberculosis treatment, rifamycin, bedaquiline, drug resistant tuberculosis
Citation: Pooranagangadevi N and Padmapriyadarsini C (2022) Treatment of Tuberculosis and the Drug Interactions Associated With HIV-TB Co-Infection Treatment. Front. Trop. Dis 3:834013. doi: 10.3389/fitd.2022.834013
Received: 12 December 2021; Accepted: 07 April 2022;
Published: 13 May 2022.
Edited by:
Carlos Brites, Federal University of Bahia, BrazilReviewed by:
Flávia Sant´Anna, Instituto Nacional de Infectologia Evandro Chagas (INI), BrazilRafael Tiburcio, Gonçalo Moniz Institute (IGM), Brazil
Neesha Rockwood, Imperial College London, United Kingdom
Copyright © 2022 Pooranagangadevi and Padmapriyadarsini. This is an open-access article distributed under the terms of the Creative Commons Attribution License (CC BY). The use, distribution or reproduction in other forums is permitted, provided the original author(s) and the copyright owner(s) are credited and that the original publication in this journal is cited, in accordance with accepted academic practice. No use, distribution or reproduction is permitted which does not comply with these terms.
*Correspondence: Chandrasekaran Padmapriyadarsini, padmapriyadarsinic@nirt.res.in