- 1Clinical Pathology Division, Institute of Pathology, University of Bern, Bern, Switzerland
- 2Translational Research Unit, Institute of Pathology, University of Bern, Bern, Switzerland
In recent years, tumor budding in colorectal cancer has gained much attention as an indicator of lymph node metastasis, distant metastatic disease, local recurrence, worse overall and disease-free survival, and as an independent prognostic factor. Tumor buds, defined as the presence of single tumor cells or small clusters of up to five tumor cells at the peritumoral invasive front (peritumoral buds) or within the main tumor body (intratumoral buds), are thought to represent the morphological correlate of cancer cells having undergone epithelial–mesenchymal transition (EMT), an important mechanism for the progression of epithelial cancers. In contrast to their undisputed prognostic power and potential to influence clinical management, our current understanding of the biological background of tumor buds is less established. Most studies examining tumor buds have attempted to recapitulate findings of mechanistic EMT studies using immunohistochemical markers. The aim of this review is to provide a comprehensive summary of studies examining protein expression profiles of tumor buds and to illustrate the molecular pathways and crosstalk involved in their formation and maintenance.
Introduction
The hallmark of malignant disease, namely the ability of a tumor to disseminate and colonize distant sites, requires an arsenal of cellular characteristics. In colorectal cancer and a growing number of solid tumors, epithelial–mesenchymal transition (EMT) is proposed as a fundamental mechanism for epithelial cells to acquire such a “malignant” phenotype (1, 2). Tumor buds, defined as the presence of single tumor cells or small groups of up to five tumor cells at the invasive tumor front or within the main tumor body [termed intratumoral buds (3)] are thought to be the histomorphological correlate of cells to undergo EMT in colorectal cancer. Indeed, high-grade tumor budding is strongly and independently associated with many adverse features such as vascular invasion, lymph node, and distant metastases and is detrimental to overall and disease-free survival (4–12).
From a morphological point of view, tumor buds tend to appear more atypical than their counterparts in the main tumor body [hence the previous term “tumor dedifferentiation” (13)] Tumor buds may be difficult to detect on H&E stained slides, as they are, per definition, single tumor cells or small clusters of tumor cells that have broken off from the main tumor body and “blend” into the tumor microenvironment, often obscured by peritumoral inflammatory reaction. At high power, it may be difficult to distinguish tumor buds from reactive stromal cells, which may also appear large and atypical. Pancytokeratin immunostains are of great help in the accurate identification of tumor buds (Figures 1A,B), and have been demonstrated to significantly improve interobserver agreement in tumor bud assessments (14).
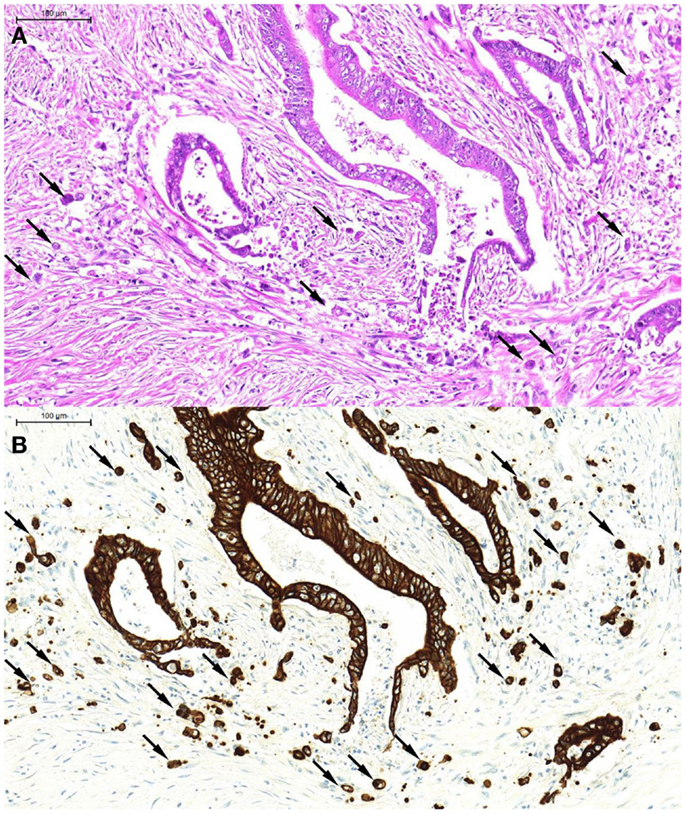
Figure 1. An example of tumor budding (single tumor cells and small clusters of up to five tumor cells detached from the main tumor body). These can be seen on H&E [(A), arrows] but are far more readily recognized on the pancytokeratin immunostain of the corresponding tumor area [(B), arrows].
As tumor buds are visualized in a histological “snapshot,” studies using immunohistochemistry have been pivotal in improving our understanding of tumor buds and their protein expression profiles (15). A distinct heterogeneity in immunohistochemical expression profiles among different tumor compartments (center vs. invasive front and tumor buds) has contributed to our appreciation of the consequences of EMT (15).
Molecular Background of Tumor-Budding Phenotypes
It is well-recognized that “colorectal cancer” encompasses fundamentally different molecular phenotypes following various pathways of carcinogenesis (16). As a consequence, colorectal cancers arising from different pathways differ in terms of biological behavior, histomorphological features, and protein expression (17, 18). One of the major and most well-studied pathways involves mutation of the APC gene, activating the WNT/wingless signaling pathway. Its major downstream effectors, β-catenin, and E-cadherin, are considered integral components of EMT (1). Therefore, it is not surprising that high-grade tumor budding is strongly associated with tumors arising from the tumors with mutation of the APC gene (19). In contrast, tumors with microsatellite instability, another well-studied pathway of colorectal carcinogenesis, are inversely correlated with tumor budding (19, 20). To date, only few studies have systematically assessed differences in tumor bud expression profiles taking into account the molecular background of tumors. In MMR-deficient tumors, reduced β-catenin expression in tumor buds was demonstrated in comparison to MMR-proficient tumors (21), leading to the speculation that mechanisms other than only Wnt signaling may lead to the formation of tumor buds in MMR-deficient cancers. Also, buds arising in MMR-deficient tumors may represent a less aggressive budding phenotype, highlighted by reduced expression of the cell locomotion protein laminin5γ2 in buds and in line with the generally milder clinical course of these tumors (21). On the other hand, adenocarcinomas with “serrated” morphology, which did not display histological features associated with microsatellite instability, were shown to have increased expression of laminin5γ2 and decreased expression of nuclear β-catenin and E-cadherin in tumor-budding cells compared to matched “conventional” adenocarcinomas (22). Unfortunately, the classification of these tumors was made based on morphology alone, hence their true molecular background remains presumptive [serrated morphology without features of MSI-high tumors being most suggestive of BRAF-mutated, CIMP-high, MMR-proficient tumors, which are known to behave aggressively (23, 24)].
Wnt Signaling
Activation of WNT signaling leads to stabilization of membranous/cytoplamasmic β-catenin and its translocation to the nucleus. Located at the cell membrane, β-catenin complexes with E-cadherin and is crucial for maintaining cell–cell adhesion and epithelial cell polarity (24). However, mutations in the APC gene lead to nuclear translocation of β-catenin, where it binds to members of the Tcf/LEF family, and functions as an oncogenic transcription factor. Therefore, preservation of membranous E-cadherin and β-catenin are indicative of an epithelial phenotype, whereas loss of E-Cadherin and nuclear expression of β-catenin are considered hallmarks of EMT. Due to their well-established role in EMT, β-catenin and its transcriptional targets represent the most extensively studied group of proteins in tumor buds. Increased nuclear expression of β-catenin in tumor buds in comparison to the main tumor body has been demonstrated in several studies (21, 22, 25–29) as has loss of E-Cadherin (4, 28, 30, 31) (summarized in Table 1). However, canonical Wnt signaling and β-catenin alone appear not to be the sole driving force behind tumor budding, as nuclear β-catenin at the invasive tumor front did not necessarily predict budding (32, 33) and although up to 90% of all colorectal cancers have dysregulation of Wnt signaling and 60% harbor APC mutations (34), high-grade budding is only seen in a proportion of these (around 40%), depending on case mix and evaluation methods (9, 14, 35–37).
The functions of proteins encoded by WNT target genes confer characteristics of a malignant mesenchymal phenotype. Proteins involved in the degradation of the extracellular matrix, such as MMP-9 and Cathepsin B have been shown to be overexpressed in buds (38). Several studies have demonstrated expression of the cell locomotion protein laminin5γ2 in buds (21, 22, 39, 40).
Other cell adhesion proteins such as EpCAM have been implicated in the budding process, with loss of membranous expression identified in tumor buds (25). EpCAM is activated by proteolysis by tumor-necrosis factor alpha (TNF α) converting enzyme, resulting in release of EpICD into the cytoplasm, which becomes part of the h-catenin and LEF transcriptional complex (41). The neuronal cell adhesion molecule L1 has also been identified as a β-catenin target gene and is preferentially expressed in tumor buds where it is co-regulated with ADAM10, a metalloprotease involved in cleaving and shedding L1s extracellular domain (42). L1 has recently been demonstrated to induce NFκB signaling in colorectal cancer cells (52), NFκB being implicated in EMT (53). These studies demonstrate the degree of crosstalk between Wnt signaling and EMT.
Modulators of Wnt signaling have also been detected in tumor buds, such as the AAA+ protein family member pontin (29), which has been implicated in enhancing the effect of Wnt signaling by binding to the β-catenin/LEF complex.
Tumor Buds, EMT, and “Stemness”
The stem-cell concept is centered on the notion that tumor progression is driven by a primarily undifferentiated population of tumor-initiating cancer cells. After initially being described in acute myeloid cancers, cancer stem cells (CSCs) have been identified in a myriad of solid tumors including colorectal cancers. CSCs display aggressive features such as increased invasiveness, chemoresistance, and the ability to mediate angiogenesis and resist apoptosis, with the ability to re-differentiate at metastatic sites (54). It would therefore stand to reason that tumor buds may represent a population of migrating CSCs (55). Indeed, there is increasing evidence linking CSCs to EMT. For instance, forced expression of the EMT transcription factor snail in CRC cell lines leads to increased expression of the putative stem-cell markers CD133 and CD44 (43). Alleged stem-cell markers in colorectal cancer include EpCAM (alongside its role as a cell adhesion molecule), CD133, CD44, ABCG5, CD90, CD24, CD166, LGR5 (a Wnt pathway target), and ALDH1 (49). Several studies have examined the immunohistochemical expression of stem-cell markers in different compartments of colorectal cancer. CD133 has been reported to be preferentially expressed at the invasive tumor front but not within tumor buds themselves (44). Hostettler et al. (49) found expression of CD133, 166, CD44, and CD90 to be a rare event in tumor buds, but cytoplasmic EpCAM and ABGC5 were frequently expressed in tumor-budding cells. Both of these markers were demonstrated to have a negative effect on survival, and expression of ABCG5 in buds was associated with worse prognosis in node-negative colorectal cancer patients. A study examining the expression of Lgr5 found a small subset of buds to be positive for this putative stem-cell maker but 6- to 11.5-fold higher expression rates in distant metastases were detected (56). Taken together, the above results support the notion that expression of stem-cell markers appears to be heterogeneous among buds and that only small populations of tumor cells (low-frequency subclones) may be perpetrators of metastatic disease.
Cell Cycle-Related Proteins
There is accumulating evidence indicating that the driving force of colorectal cancer progression may not be attributable to tumor cell proliferation alone. Generally, it is thought that EMT-derived tumor cells are hypo-proliferative, underlining the significance of aggressive cellular machinery to exert their malignant properties (57). The cell cycle regulators cyclin D1 and p16 are Wnt signaling targets and their activation is a suggested mechanism of EMT-induced growth arrest. Under normal circumstances, nuclear p16 is a direct inhibitor of cyclin D1, arresting the cell cycle. However, located in the cytoplasm, p16 is thought to bind with CDK4, blocking its transport to the nucleus. CDK4 is required for cyclin D1 activation. Therefore, in the absence of CDK4, cyclin D1 forms an inactive complex with CDK2, accounting for the apparently paradoxical co-upregulation of p16 and cyclin D1 (58). Indeed, tumor buds have been demonstrated to show cytoplasmic expression of p16 (19, 57). As a consequence, several studies have demonstrated the hypo-proliferative nature of the invasive front and tumor buds themselves using Ki-67 immunohistochemistry (30, 39, 45, 59).
As the hypo-proliferative nature of tumor buds is gaining recognition, it may be speculated that in order to survive migration through stroma, tumor buds must confer of essential survival mechanisms. In fact, single epithelial cells detached from the extracellular matrix are programed to undergo a certain form of cell death termed anoikis (60). In addition to their hypo-proliferative state, tumor buds have been demonstrated to be anti-apoptotic by their relative lack of immunoreactivity for caspase 3 (59), suggesting that tumor buds are able to resist anoikis.
EMT-Inducing Pathways Involving RAS/RAF and RAS/MAPK Signaling
Cancer cells frequently exploit growth factor signaling from the surrounding microenvironment (such as insulin growth factor, hepatocyte growth factor, epidermal growth factor, or placental-derived growth factor) to drive tumor progression (61–63). Well-studied downstream pathways include PI3K-, NFκB-, Snail, and RAS–RAF–ERK–ZEB1 (Figure 1). For instance, as Snail controls ZEB1, a transcriptional repressor of E-cadherin (64); it is not surprising that genes involved in growth factor signaling induce EMT.
The tumor suppressor gene RKIP has been linked to EMT on several levels, for one as inhibitor of the Ras–Raf–MEK–ERK signaling cascade at the level of Raf (65). In addition, RKIP modulates other signaling pathways including NFκB–Snail (46, 66). Several studies have demonstrated differential expression of RKIP in zones of colorectal cancer, with gradual loss of expression toward the tumor front (33, 67) and ability of RKIP expression to predict high-grade budding. RKIP was only rarely detected in tumor buds and in line with mechanistic EMT studies, loss of RKIP correlated with E-Cadherin negativity and nuclear translocation of NFκB. However, the prognostic significance of RKIP appears to be restricted to its expression in the tumor center, suggesting that other mechanisms may become increasingly important in the development of tumor-budding cells (67).
The neurotrophic tyrosine kinase receptor TrkB has been linked to EMT via RAS/MAPK-dependent Twist–Snail signaling and has been demonstrated to be a potent and specific suppressor of anoikis (47), which is supported by its overexpression in tumor buds (68). Additionally, KRAS-mutated colorectal cancers also overexpress TrkB, in concordance with the known dependency on MAPK signaling on TrkB-induced EMT.
CXCL12 (sdf-1)/CXCR4 Pathway
Chemokines, integral for cell migration and trafficking, are widely expressed by cells of the lymphatic and hemopoietic systems. The chemokine CXCL12 binds to its receptor CXCL4, activating subsequent intracellular pathways involved in chemotaxis, cell survival, and gene transcription (69). As CXCR4 is expressed in cells in multiple organs including lymph nodes, lungs, and liver, epithelial tumor cells may take advantage of the principle of homing mechanisms to direct the metastasis of CXCL12-positive tumor cells to CXCR4 positive organs (70). CXCL12 can also stimulate the formation of capillary structures (48). CXCL12 expression in tumor buds was found to be correlated with liver metastases and was an independent prognostic marker (71).
Markers of Intestinal Differentiation
The homeobox transcription factor Cdx2 encodes a transcription factor specific to intestinal differentiation, which is essential for development and homeostasis of gut epithelium (72). Recent evidence also suggests that Cdx2 may play a substantial role in Wnt signaling as a tumor suppressor gene and therefore inhibit EMT. For instance, Cdx2 has been found to bind β-catenin, thus disrupting the β-catenin/TCF complex (73). Also, Cdx2 may inhibit the transcriptional activity of β-catenin through interaction with the protocadherin Mucdhl (74). Finally, Cdx2 enhances the function of E-cadherin by trafficking it to the cell membrane, thus restoring cell adhesion (75). As dedifferentiated cancer cells, it is not surprising that tumor buds lack expression of Cdx2 (76). However, the fact that most colorectal cancers that diffusely express Cdx2 also do so in their metastases (as the marker may be used diagnostically for cancers of unknown primary as a marker of intestinal differentiation) supports the notion of tumor redifferentiation and reversibility of EMT at metastatic sites.
Few studies have examined the expression of other markers of epithelial differentiation in tumor buds. For instance, Harbaum et al (30) demonstrated absence of the intestinal-type mucin Muc2 and overexpression of cytokeratin 7, a simple intermediate keratin filament, at the invasive front and strikingly in tumor buds. This finding is intriguing since expression of cytokeratin 7 is relatively infrequent in primary colorectal carcinoma (77), and because intermediate filaments are traditionally known to support cell–cell or cell–matrix adhesions in epithelial cells. However, recent evidence suggests that keratin filaments may contribute to a higher degree of cellular plasticity than originally assumed (78) and it may be postulated that surrounding biochemical and mechanical stimulation in the tumor microenvironment could influence the cytoskeletal protein composition.
Stromal–Epithelial Interaction in the Tumor Microenvironment
It has also been postulated that signals derived from surrounding mesenchymal cells in the tumor microenvironment may play a significant role in facilitating a pro-budding phenotype (50, 79). For instance, immunohistochemical expression of TWIST1 and TWIST2, known activators of EMT, was significantly positively correlated with a tumor-budding phenotype (both low-grade and high-grade budding), yet their expression was virtually restricted to stromal cells in the tumor microenvironment. Moreover, in high-grade budding cancers an inverse correlation between TWIST1 methylation and stromal protein expression was observed, suggesting hypermethylation as a mechanism of TWIST1 regulation (79). TWIST1 has previously been demonstrated to be expressed in neoplastic stromal cells. These cells were shown to be neoplastic, demonstrating the same neoplastic aberrations as the tumor itself, indicating that EMT had indeed taken place with cells having acquired a fully mesenchymal phenotype (80).
The interplay between epithelial and stromal components has also been underlined by studies examining bone morphogenetic protein (BMP) antagonists (51, 81), hypothesizing that CRC cells in the tumor microenvironment can only flourish in a milieu devoid of BMP signaling, this was characterized immunohistochemically by a shift in HTRA3 expression patterns (decreased stromal staining and increased epithelial staining).
Conclusion
Tumor budding is thought to represent the morphological correlate of EMT in colorectal cancers and has been strongly linked to adverse clinicopathological features and poor overall and disease-free patient survival. These consistent associations indicate that tumor budding has a strong value as a prognostic indicator, and it has been proposed that budding should be an integrated category in pathology reports (82).
In an attempt to contribute to our understanding of tumor buds, previous studies have mainly immunohistochemistry to discriminate properties unique to tumor-budding cells. The main reason for this is that immunohistochemistry enables the actual identification of tumor buds for evaluation. To our knowledge, there is currently no method of extracting tumor buds from fresh tumor tissue, presenting huge hurdles for molecular studies specifically geared at tumor buds. Therefore, our understanding of the biology of tumor buds is essentially restricted to protein expresssion profiles (as visualized in Figure 2), and a few studies, which have used mRNA in situ hybridization. Immunohistochemistry as a semi-quantitative method may be especially prone to subjectivity, and staining intensity greatly depends on laboratory methods (83). Such issues may contribute to difficulties in reproducibility and the consistency of results. Not all proteins differentially expressed in tumor buds appear to have significant prognostic relevance, which may be at least in part explained by the timing of certain events in the process of carcinogenesis and the accumulation of different simultaneous molecular occurrences, of which our knowledge is limited.
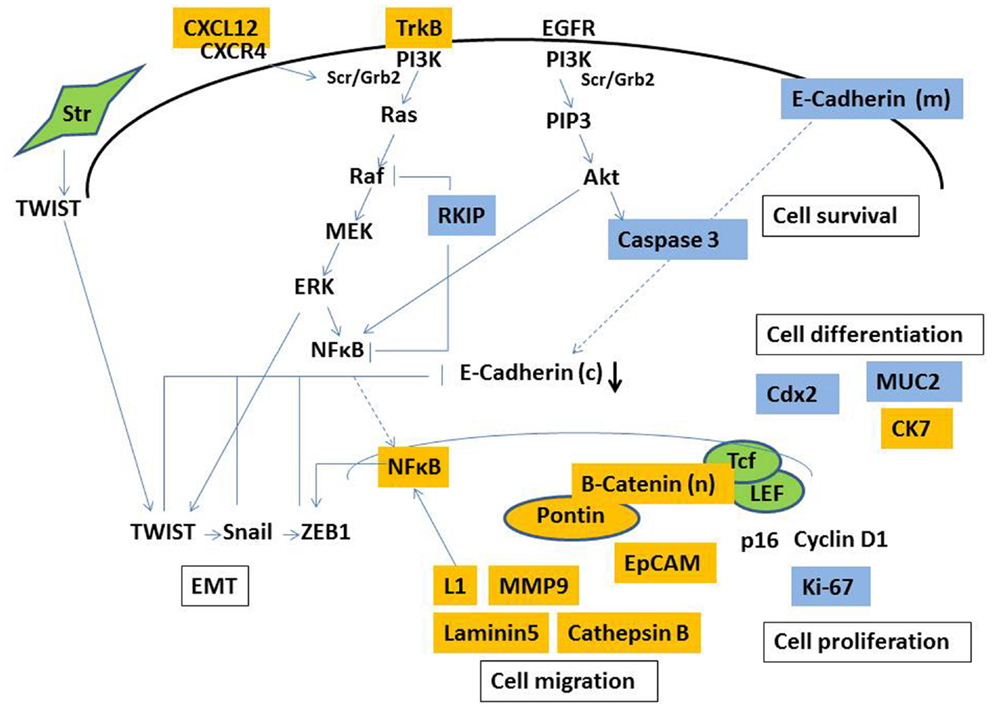
Figure 2. Simplified illustration of molecular pathways involved in the formation of tumor budding. Markers demonstrated to be overexpressed (yellow) and underexpressed (blue) in tumor buds by immunohistochemistry. Str, stromal cell, (c), cytoplasmic, (m) membranous, (n) nuclear.
Therefore, although the molecular background of colorectal cancers appears to play an important role in budding, much remains to be investigated in terms of genetic profiles of tumor buds and how various molecular pathways are taken advantage of by these cells to maintain their malignant phenotype and drive tumor progression. Novel areas of interest include the interaction of tumor buds with cancer-associated fibroblasts and inflammatory cells in the tumor microenvironment (84) and the evasion of anoikis. Taken together, and based on growing evidence that tumor buds may be targetable structures (15), our understanding of these mechanisms will be crucial for the development of future therapies aimed at the destruction of tumor buds.
Conflict of Interest Statement
The authors declare that the research was conducted in the absence of any commercial or financial relationships that could be construed as a potential conflict of interest.
References
1. Kalluri R, Weinberg RA. The basics of epithelial-mesenchymal transition. J Clin Invest (2009) 119:1420–8. doi:10.1172/JCI39104
Pubmed Abstract | Pubmed Full Text | CrossRef Full Text | Google Scholar
2. Thiery JP, Acloque H, Huang RY, Nieto MA. Epithelial-mesenchymal transitions in development and disease. Cell (2009) 139:871–90. doi:10.1016/j.cell.2009.11.007
Pubmed Abstract | Pubmed Full Text | CrossRef Full Text | Google Scholar
3. Zlobec I, Hadrich M, Dawson H, Koelzer VH, Borner M, Mallaev M, et al. Intratumoural budding (ITB) in preoperative biopsies predicts the presence of lymph node and distant metastases in colon and rectal cancer patients. Br J Cancer (2014) 110:1008–13. doi:10.1038/bjc.2013.797
Pubmed Abstract | Pubmed Full Text | CrossRef Full Text | Google Scholar
4. Prall F. Tumour budding in colorectal carcinoma. Histopathology (2007) 50:151–62. doi:10.1111/j.1365-2559.2006.02551.x
5. Mitrovic B, Schaeffer DF, Riddell RH, Kirsch R. Tumor budding in colorectal carcinoma: time to take notice. Mod Pathol (2012) 25:1315–25. doi:10.1038/modpathol.2012.94
Pubmed Abstract | Pubmed Full Text | CrossRef Full Text | Google Scholar
6. Okuyama T, Nakamura T, Yamaguchi M. Budding is useful to select high-risk patients in stage II well-differentiated or moderately differentiated colon adenocarcinoma. Dis Colon Rectum (2003) 46:1400–6. doi:10.1007/s10350-004-6757-0
Pubmed Abstract | Pubmed Full Text | CrossRef Full Text | Google Scholar
7. Tanaka M, Hashiguchi Y, Ueno H, Hase K, Mochizuki H. Tumor budding at the invasive margin can predict patients at high risk of recurrence after curative surgery for stage II, T3 Colon Cancer. Dis Colon Rectum (2003) 46:1054–9. doi:10.1007/s10350-004-7280-z
Pubmed Abstract | Pubmed Full Text | CrossRef Full Text | Google Scholar
8. Hase K, Shatney C, Johnson D, Trollope M, Vierra M. Prognostic value of tumor “budding” in patients with colorectal cancer. Dis Colon Rectum (1993) 36:627–35. doi:10.1007/BF02238588
Pubmed Abstract | Pubmed Full Text | CrossRef Full Text | Google Scholar
9. Ueno H, Murphy J, Jass JR, Mochizuki H, Talbot IC. Tumour ‘budding’ as an index to estimate the potential of aggressiveness in rectal cancer. Histopathology (2002) 40:127–32. doi:10.1046/j.1365-2559.2002.01324.x
Pubmed Abstract | Pubmed Full Text | CrossRef Full Text | Google Scholar
10. Ishikawa Y, Akishima-Fukasawa Y, Ito K, Akasaka Y, Yokoo T, Ishii T, et al. Group for cancer biological, histopathologic determinants of regional lymph node metastasis in early colorectal cancer. Cancer (2008) 112:924–33. doi:10.1002/cncr.23248
Pubmed Abstract | Pubmed Full Text | CrossRef Full Text | Google Scholar
11. Nakamura T, Mitomi H, Kikuchi S, Ohtani Y, Sato K. Evaluation of the usefulness of tumor budding on the prediction of metastasis to the lung and liver after curative excision of colorectal cancer. Hepatogastroenterology (2005) 52:1432–5.
12. Wang LM, Kevans D, Mulcahy H, O’Sullivan J, Fennelly D, Hyland J, et al. Tumor budding is a strong and reproducible prognostic marker in T3N0 colorectal cancer. Am J Surg Pathol (2009) 33:134–41. doi:10.1097/PAS.0b013e318184cd55
Pubmed Abstract | Pubmed Full Text | CrossRef Full Text | Google Scholar
13. Gabbert H, Wagner R, Moll R, Gerharz CD. Tumor dedifferentiation: an important step in tumor invasion. Clin Exp Metastasis (1985) 3:257–79. doi:10.1007/BF01585081
Pubmed Abstract | Pubmed Full Text | CrossRef Full Text | Google Scholar
14. Horcic M, Koelzer VH, Karamitopoulou E, Terracciano L, Puppa G, Zlobec I, et al. Tumor budding score based on 10 high-power fields is a promising basis for a standardized prognostic scoring system in stage II colorectal cancer. Hum Pathol (2013) 44:697–705. doi:10.1016/j.humpath.2012.07.026
Pubmed Abstract | Pubmed Full Text | CrossRef Full Text | Google Scholar
15. Zlobec I, Lugli A. Epithelial mesenchymal transition and tumor budding in aggressive colorectal cancer: tumor budding as oncotarget. Oncotarget (2010) 1:651–61.
16. N. Cancer Genome Atlas. Comprehensive molecular characterization of human colon and rectal cancer. Nature (2012) 487:330–7. doi:10.1038/nature11252
Pubmed Abstract | Pubmed Full Text | CrossRef Full Text | Google Scholar
17. Chen D, Huang JF, Liu K, Zhang LQ, Yang Z, Chuai ZR, et al. BRAFV600E mutation and its association with clinicopathological features of colorectal cancer: a systematic review and meta-analysis. PLoS One (2014) 9:e90607. doi:10.1371/journal.pone.0090607
Pubmed Abstract | Pubmed Full Text | CrossRef Full Text | Google Scholar
18. Morikawa T, Kuchiba A, Qian ZR, Mino-Kenudson M, Hornick JL, Yamauchi M, et al. Prognostic significance and molecular associations of tumor growth pattern in colorectal cancer. Ann Surg Oncol (2012) 19:1944–53. doi:10.1245/s10434-011-2174-5
Pubmed Abstract | Pubmed Full Text | CrossRef Full Text | Google Scholar
19. Jass JR, Barker M, Fraser L, Walsh MD, Whitehall VL, Gabrielli B, et al. APC mutation and tumour budding in colorectal cancer. J Clin Pathol (2003) 56:69–73. doi:10.1136/jcp.56.1.69
20. Zlobec I, Bihl MP, Foerster A, Rufle A, Lugli A. The impact of CpG island methylator phenotype and microsatellite instability on tumour budding in colorectal cancer. Histopathology (2012) 61:777–87. doi:10.1111/j.1365-2559.2012.04273.x
Pubmed Abstract | Pubmed Full Text | CrossRef Full Text | Google Scholar
21. Shinto E, Baker K, Tsuda H, Mochizuki H, Ueno H, Matsubara O, et al. Tumor buds show reduced expression of laminin-5 gamma 2 chain in DNA mismatch repair deficient colorectal cancer. Dis Colon Rectum (2006) 49:1193–202. doi:10.1007/s10350-006-0568-4
Pubmed Abstract | Pubmed Full Text | CrossRef Full Text | Google Scholar
22. Garcia-Solano J, Conesa-Zamora P, Trujillo-Santos J, Torres-Moreno D, Makinen MJ, Perez-Guillermo M. Immunohistochemical expression profile of beta-catenin, E-cadherin, P-cadherin, laminin-5gamma2 chain, and SMAD4 in colorectal serrated adenocarcinoma. Hum Pathol (2012) 43:1094–102. doi:10.1016/j.humpath.2011.08.020
Pubmed Abstract | Pubmed Full Text | CrossRef Full Text | Google Scholar
23. Kang GH. Four molecular subtypes of colorectal cancer and their precursor lesions. Arch Pathol Lab Med (2011) 135:698–703. doi:10.1043/2010-0523-RA.1
Pubmed Abstract | Pubmed Full Text | CrossRef Full Text | Google Scholar
24. Lochhead P, Kuchiba A, Imamura Y, Liao X, Yamauchi M, Nishihara R, et al. Microsatellite instability and BRAF mutation testing in colorectal cancer prognostication. J Natl Cancer Inst (2013) 105:1151–6. doi:10.1093/jnci/djt173
25. Gosens MJ, van Kempen LC, van de Velde CJ, van Krieken JH, Nagtegaal ID. Loss of membranous Ep-CAM in budding colorectal carcinoma cells. Mod Pathol (2007) 20:221–32. doi:10.1038/modpathol.3800733
Pubmed Abstract | Pubmed Full Text | CrossRef Full Text | Google Scholar
26. Brabletz T, Hlubek F, Spaderna S, Schmalhofer O, Hiendlmeyer E, Jung A, et al. Invasion and metastasis in colorectal cancer: epithelial-mesenchymal transition, mesenchymal-epithelial transition, stem cells and beta-catenin. Cells Tissues Organs (2005) 179:56–65. doi:10.1159/000084509
27. Brabletz T, Jung A, Hermann K, Gunther K, Hohenberger W, Kirchner T. Nuclear overexpression of the oncoprotein beta-catenin in colorectal cancer is localized predominantly at the invasion front. Pathol Res Pract (1998) 194:701–4. doi:10.1016/S0344-0338(98)80129-5
Pubmed Abstract | Pubmed Full Text | CrossRef Full Text | Google Scholar
28. El-Bahrawy MA, Poulsom R, Jeffery R, Talbot I, Alison MR. The expression of E-cadherin and catenins in sporadic colorectal carcinoma. Hum Pathol (2001) 32:1216–24. doi:10.1053/hupa.2001.28948
Pubmed Abstract | Pubmed Full Text | CrossRef Full Text | Google Scholar
29. Lauscher JC, Loddenkemper C, Kosel L, Grone J, Buhr HJ, Huber O. Increased pontin expression in human colorectal cancer tissue. Hum Pathol (2007) 38:978–85. doi:10.1016/j.humpath.2007.01.005
Pubmed Abstract | Pubmed Full Text | CrossRef Full Text | Google Scholar
30. Harbaum L, Pollheimer MJ, Kornprat P, Lindtner RA, Schlemmer A, Rehak P, et al. Keratin 7 expression in colorectal cancer – freak of nature or significant finding? Histopathology (2011) 59:225–34. doi:10.1111/j.1365-2559.2011.03694.x
Pubmed Abstract | Pubmed Full Text | CrossRef Full Text | Google Scholar
31. Brabletz T, Jung A, Reu S, Porzner M, Hlubek F, Kunz-Schughart LA, et al. Variable beta-catenin expression in colorectal cancers indicates tumor progression driven by the tumor environment. Proc Natl Acad Sci USA (2001) 98:10356–61. doi:10.1073/pnas.171610498
Pubmed Abstract | Pubmed Full Text | CrossRef Full Text | Google Scholar
32. Horkko TT, Klintrup K, Makinen JM, Napankangas JB, Tuominen HJ, Makela J, et al. Budding invasive margin and prognosis in colorectal cancer – no direct association with beta-catenin expression. Eur J Cancer (2006) 42:964–71. doi:10.1016/j.ejca.2006.01.017
Pubmed Abstract | Pubmed Full Text | CrossRef Full Text | Google Scholar
33. Karamitopoulou E, Zlobec I, Panayiotides I, Patsouris ES, Peros G, Rallis G, et al. Systematic analysis of proteins from different signaling pathways in the tumor center and the invasive front of colorectal cancer. Hum Pathol (2011) 42:1888–96. doi:10.1016/j.humpath.2010.06.020
Pubmed Abstract | Pubmed Full Text | CrossRef Full Text | Google Scholar
34. Jass JR, Young J, Leggett BA. Evolution of colorectal cancer: change of pace and change of direction. J Gastroenterol Hepatol (2002) 17:17–26. doi:10.1046/j.1440-1746.2002.02635.x
Pubmed Abstract | Pubmed Full Text | CrossRef Full Text | Google Scholar
35. Karamitopoulou E, Zlobec I, Kolzer V, Kondi-Pafiti A, Patsouris ES, Gennatas K, et al. Proposal for a 10-high-power-fields scoring method for the assessment of tumor budding in colorectal cancer. Mod Pathol (2013) 26:295–301. doi:10.1038/modpathol.2012.155
Pubmed Abstract | Pubmed Full Text | CrossRef Full Text | Google Scholar
36. Prall F, Nizze H, Barten M. Tumour budding as prognostic factor in stage I/II colorectal carcinoma. Histopathology (2005) 47:17–24. doi:10.1111/j.1365-2559.2005.02161.x
Pubmed Abstract | Pubmed Full Text | CrossRef Full Text | Google Scholar
37. Morodomi T, Isomoto H, Shirouzu K, Kakegawa K, Irie K, Morimatsu M. An index for estimating the probability of lymph node metastasis in rectal cancers. Lymph node metastasis and the histopathology of actively invasive regions of cancer. Cancer (1989) 63:539–43. doi:10.1002/1097-0142(19890201)63:3<539::AID-CNCR2820630323>3.0.CO;2-S
Pubmed Abstract | Pubmed Full Text | CrossRef Full Text | Google Scholar
38. Guzinska-Ustymowicz K. MMP-9 and cathepsin B expression in tumor budding as an indicator of a more aggressive phenotype of colorectal cancer (CRC). Anticancer Res (2006) 26:1589–94.
39. Rubio CA. Arrest of cell proliferation in budding tumor cells ahead of the invading edge of colonic carcinomas. A preliminary report. Anticancer Res (2008) 28:2417–20.
40. Sordat I, Rousselle P, Chaubert P, Petermann O, Aberdam D, Bosman FT, et al. Tumor cell budding and laminin-5 expression in colorectal carcinoma can be modulated by the tissue micro-environment. Int J Cancer (2000) 88:708–17. doi:10.1002/1097-0215(20001201)88:5<708::AID-IJC5>3.0.CO;2-J
Pubmed Abstract | Pubmed Full Text | CrossRef Full Text | Google Scholar
41. Munz M, Baeuerle PA, Gires O. The emerging role of EpCAM in cancer and stem cell signaling. Cancer Res (2009) 69:5627–9. doi:10.1158/0008-5472.CAN-09-0654
Pubmed Abstract | Pubmed Full Text | CrossRef Full Text | Google Scholar
42. Gavert N, Conacci-Sorrell M, Gast D, Schneider A, Altevogt P, Brabletz T, et al. L1, a novel target of beta-catenin signaling, transforms cells and is expressed at the invasive front of colon cancers. J Cell Biol (2005) 168:633–42. doi:10.1083/jcb.200408051
Pubmed Abstract | Pubmed Full Text | CrossRef Full Text | Google Scholar
43. Fan F, Samuel S, Evans KW, Lu J, Xia L, Zhou Y, et al. Overexpression of snail induces epithelial-mesenchymal transition and a cancer stem cell-like phenotype in human colorectal cancer cells. Cancer Med (2012) 1:5–16. doi:10.1002/cam4.4
Pubmed Abstract | Pubmed Full Text | CrossRef Full Text | Google Scholar
44. Horst D, Kriegl L, Engel J, Kirchner T, Jung A. Prognostic significance of the cancer stem cell markers CD133, CD44, and CD166 in colorectal cancer. Cancer Invest (2009) 27:844–50. doi:10.1080/07357900902744502
Pubmed Abstract | Pubmed Full Text | CrossRef Full Text | Google Scholar
45. Jung A, Schrauder M, Oswald U, Knoll C, Sellberg P, Palmqvist R, et al. The invasion front of human colorectal adenocarcinomas shows co-localization of nuclear beta-catenin, cyclin D1, and p16INK4A and is a region of low proliferation. Am J Pathol (2001) 159:1613–7. doi:10.1016/S0002-9440(10)63007-6
Pubmed Abstract | Pubmed Full Text | CrossRef Full Text | Google Scholar
46. Escara-Wilke J, Yeung K, Keller ET. Raf kinase inhibitor protein (RKIP) in cancer. Cancer Metastasis Rev (2012) 31:615–20. doi:10.1007/s10555-012-9365-9
47. Smit MA, Geiger TR, Song JY, Gitelman I, Peeper DS. A Twist-Snail axis critical for TrkB-induced epithelial-mesenchymal transition-like transformation, anoikis resistance, and metastasis. Mol Cell Biol (2009) 29:3722–37. doi:10.1128/MCB.01164-08
Pubmed Abstract | Pubmed Full Text | CrossRef Full Text | Google Scholar
48. Petit I, Jin D, Rafii S. The SDF-1-CXCR4 signaling pathway: a molecular hub modulating neo-angiogenesis. Trends Immunol (2007) 28:299–307. doi:10.1016/j.it.2007.05.007
Pubmed Abstract | Pubmed Full Text | CrossRef Full Text | Google Scholar
49. Hostettler L, Zlobec I, Terracciano L, Lugli A. ABCG5-positivity in tumor buds is an indicator of poor prognosis in node-negative colorectal cancer patients. World J Gastroenterol (2010) 16:732–9. doi:10.3748/wjg.v16.i6.732
Pubmed Abstract | Pubmed Full Text | CrossRef Full Text | Google Scholar
50. Rizzi C, Cataldi P, Iop A, Isola M, Sgarra R, Manfioletti G, et al. The expression of the high-mobility group A2 protein in colorectal cancer and surrounding fibroblasts is linked to tumor invasiveness. Hum Pathol (2013) 44:122–32. doi:10.1016/j.humpath.2012.05.001
Pubmed Abstract | Pubmed Full Text | CrossRef Full Text | Google Scholar
51. Karagiannis GS, Berk A, Dimitromanolakis A, Diamandis EP. Enrichment map profiling of the cancer invasion front suggests regulation of colorectal cancer progression by the bone morphogenetic protein antagonist, gremlin-1. Mol Oncol (2013) 7:826–39. doi:10.1016/j.molonc.2013.04.002
Pubmed Abstract | Pubmed Full Text | CrossRef Full Text | Google Scholar
52. Gavert N, Ben-Shmuel A, Lemmon V, Brabletz T, Ben-Ze’ev A. Nuclear factor-kappaB signaling and ezrin are essential for L1-mediated metastasis of colon cancer cells. J Cell Sci (2010) 123:2135–43. doi:10.1242/jcs.069542
Pubmed Abstract | Pubmed Full Text | CrossRef Full Text | Google Scholar
53. Wu Y, Deng J, Rychahou PG, Qiu S, Evers BM, Zhou BP. Stabilization of snail by NF-kappaB is required for inflammation-induced cell migration and invasion. Cancer Cell (2009) 15:416–28. doi:10.1016/j.ccr.2009.03.016
Pubmed Abstract | Pubmed Full Text | CrossRef Full Text | Google Scholar
54. Zeuner A, Todaro M, Stassi G, De Maria R. Colorectal cancer stem cells: from the crypt to the clinic. Cell Stem Cell (2014) 15:692–705. doi:10.1016/j.stem.2014.11.012
Pubmed Abstract | Pubmed Full Text | CrossRef Full Text | Google Scholar
55. Findlay VJ, Wang C, Watson DK, Camp ER. Epithelial-to-mesenchymal transition and the cancer stem cell phenotype: insights from cancer biology with therapeutic implications for colorectal cancer. Cancer Gene Ther (2014) 21:181–7. doi:10.1038/cgt.2014.15
Pubmed Abstract | Pubmed Full Text | CrossRef Full Text | Google Scholar
56. Kleist B, Xu L, Li G, Kersten C. Expression of the adult intestinal stem cell marker Lgr5 in the metastatic cascade of colorectal cancer. Int J Clin Exp Pathol (2011) 4:327–35.
57. Brabletz T. To differentiate or not – routes towards metastasis. Nat Rev Cancer (2012) 12:425–36. doi:10.1038/nrc3265
Pubmed Abstract | Pubmed Full Text | CrossRef Full Text | Google Scholar
58. Sweeney KJ, Sarcevic B, Sutherland RL, Musgrove EA. Cyclin D2 activates Cdk2 in preference to Cdk4 in human breast epithelial cells. Oncogene (1997) 14:1329–40. doi:10.1038/sj.onc.1200951
Pubmed Abstract | Pubmed Full Text | CrossRef Full Text | Google Scholar
59. Dawson H, Koelzer VH, Karamitopoulou E, Economou M, Hammer C, Muller DE, et al. The apoptotic and proliferation rate of tumour budding cells in colorectal cancer outlines a heterogeneous population of cells with various impacts on clinical outcome. Histopathology (2014) 64:577–84. doi:10.1111/his.12294
Pubmed Abstract | Pubmed Full Text | CrossRef Full Text | Google Scholar
60. Guadamillas MC, Cerezo A, Del Pozo MA. Overcoming anoikis – pathways to anchorage-independent growth in cancer. J Cell Sci (2011) 124:3189–97. doi:10.1242/jcs.072165
Pubmed Abstract | Pubmed Full Text | CrossRef Full Text | Google Scholar
61. Spano JP, Fagard R, Soria JC, Rixe O, Khayat D, Milano G. Epidermal growth factor receptor signaling in colorectal cancer: preclinical data and therapeutic perspectives. Ann Oncol (2005) 16:189–94. doi:10.1093/annonc/mdi057
Pubmed Abstract | Pubmed Full Text | CrossRef Full Text | Google Scholar
62. Capdevila J, Carrato A, Tabernero J, Grande E. What could Nintedanib (BIBF 1120), a triple inhibitor of VEGFR, PDGFR, and FGFR, add to the current treatment options for patients with metastatic colorectal cancer? Crit Rev Oncol Hematol (2014) 92(2):83–106. doi:10.1016/j.critrevonc.2014.05.004
Pubmed Abstract | Pubmed Full Text | CrossRef Full Text | Google Scholar
63. Malaguarnera R, Belfiore A. The emerging role of insulin and insulin-like growth factor signaling in cancer stem cells. Front Endocrinol (Lausanne) (2014) 5:10. doi:10.3389/fendo.2014.00010
Pubmed Abstract | Pubmed Full Text | CrossRef Full Text | Google Scholar
64. Schmalhofer O, Brabletz S, Brabletz T. E-cadherin, beta-catenin, and ZEB1 in malignant progression of cancer. Cancer Metastasis Rev (2009) 28:151–66. doi:10.1007/s10555-008-9179-y
Pubmed Abstract | Pubmed Full Text | CrossRef Full Text | Google Scholar
65. Yeung K, Seitz T, Li S, Janosch P, McFerran B, Kaiser C, et al. Suppression of Raf-1 kinase activity and MAP kinase signalling by RKIP. Nature (1999) 401:173–7. doi:10.1038/43686
Pubmed Abstract | Pubmed Full Text | CrossRef Full Text | Google Scholar
66. Wu K, Bonavida B. The activated NF-kappaB-Snail-RKIP circuitry in cancer regulates both the metastatic cascade and resistance to apoptosis by cytotoxic drugs. Crit Rev Immunol (2009) 29:241–54. doi:10.1615/CritRevImmunol.v29.i3.40
Pubmed Abstract | Pubmed Full Text | CrossRef Full Text | Google Scholar
67. Koelzer VH, Karamitopoulou E, Dawson H, Kondi-Pafiti A, Zlobec I, Lugli A. Geographic analysis of RKIP expression and its clinical relevance in colorectal cancer. Br J Cancer (2013) 108:2088–96. doi:10.1038/bjc.2013.197
Pubmed Abstract | Pubmed Full Text | CrossRef Full Text | Google Scholar
68. Dawson H, Grundmann S, Koelzer VH, Galvan JA, Kirsch R, Karamitopoulou E, et al. Tyrosine kinase receptor B (TrkB) expression in colorectal cancers highlights anoikis resistance as a survival mechanism of tumour budding cells. Histopathology (2014). doi:10.1111/his.12603
Pubmed Abstract | Pubmed Full Text | CrossRef Full Text | Google Scholar
69. Cojoc M, Peitzsch C, Trautmann F, Polishchuk L, Telegeev GD, Dubrovska A. Emerging targets in cancer management: role of the CXCL12/CXCR4 axis. OncoTargets Ther (2013) 6:1347–61. doi:10.2147/OTT.S36109
Pubmed Abstract | Pubmed Full Text | CrossRef Full Text | Google Scholar
70. Teicher BA, Fricker SP. CXCL12 (SDF-1)/CXCR4 pathway in cancer. Clin Cancer Res (2010) 16:2927–31. doi:10.1158/1078-0432.CCR-09-2329
Pubmed Abstract | Pubmed Full Text | CrossRef Full Text | Google Scholar
71. Akishima-Fukasawa Y, Nakanishi Y, Ino Y, Moriya Y, Kanai Y, Hirohashi S. Prognostic significance of CXCL12 expression in patients with colorectal carcinoma. Am J Clin Pathol (2009) 132:202–10. doi:10.1309/AJCPK35VZJEWCUTL
Pubmed Abstract | Pubmed Full Text | CrossRef Full Text | Google Scholar
72. Lorentz O, Duluc I, Arcangelis AD, Simon-Assmann P, Kedinger M, Freund JN. Key role of the Cdx2 homeobox gene in extracellular matrix-mediated intestinal cell differentiation. J Cell Biol (1997) 139:1553–65. doi:10.1083/jcb.139.6.1553
Pubmed Abstract | Pubmed Full Text | CrossRef Full Text | Google Scholar
73. Guo RJ, Funakoshi S, Lee HH, Kong J, Lynch JP. The intestine-specific transcription factor Cdx2 inhibits beta-catenin/TCF transcriptional activity by disrupting the beta-catenin-TCF protein complex. Carcinogenesis (2010) 31:159–66. doi:10.1093/carcin/bgp213
Pubmed Abstract | Pubmed Full Text | CrossRef Full Text | Google Scholar
74. Hinkel I, Duluc I, Martin E, Guenot D, Freund JN, Gross I. Cdx2 controls expression of the protocadherin Mucdhl, an inhibitor of growth and beta-catenin activity in colon cancer cells. Gastroenterology (2012) 142:875–85.e3. doi:10.1053/j.gastro.2011.12.037
Pubmed Abstract | Pubmed Full Text | CrossRef Full Text | Google Scholar
75. Funakoshi S, Kong J, Crissey MA, Dang L, Dang D, Lynch JP. Intestine-specific transcription factor Cdx2 induces E-cadherin function by enhancing the trafficking of E-cadherin to the cell membrane. Am J Physiol Gastrointest Liver Physiol (2010) 299:G1054–67. doi:10.1152/ajpgi.00297.2010
Pubmed Abstract | Pubmed Full Text | CrossRef Full Text | Google Scholar
76. Brabletz T, Spaderna S, Kolb J, Hlubek F, Faller G, Bruns CJ, et al. Down-regulation of the homeodomain factor Cdx2 in colorectal cancer by collagen type I: an active role for the tumor environment in malignant tumor progression. Cancer Res (2004) 64:6973–7. doi:10.1158/0008-5472.CAN-04-1132
Pubmed Abstract | Pubmed Full Text | CrossRef Full Text | Google Scholar
77. Bayrak R, Yenidunya S, Haltas H. Cytokeratin 7 and cytokeratin 20 expression in colorectal adenocarcinomas. Pathol Res Pract (2011) 207:156–60. doi:10.1016/j.prp.2010.12.005
Pubmed Abstract | Pubmed Full Text | CrossRef Full Text | Google Scholar
78. Helfand BT, Chang L, Goldman RD. Intermediate filaments are dynamic and motile elements of cellular architecture. J Cell Sci (2004) 117:133–41. doi:10.1242/jcs.00936
Pubmed Abstract | Pubmed Full Text | CrossRef Full Text | Google Scholar
79. Galvan JA, Helbling M, Koelzer VH, Tschan MP, Berger MD, Hadrich M, et al. TWIST1 and TWIST2 promoter methylation and protein expression in tumor stroma influence the epithelial-mesenchymal transition-like tumor budding phenotype in colorectal cancer. Oncotarget (2015) 20:874–85.
80. Celesti G, Di Caro G, Bianchi P, Grizzi F, Basso G, Marchesi F, et al. Presence of Twist1-positive neoplastic cells in the stroma of chromosome-unstable colorectal tumors. Gastroenterology (2013) 145:647–57.e15. doi:10.1053/j.gastro.2013.05.011
Pubmed Abstract | Pubmed Full Text | CrossRef Full Text | Google Scholar
81. Karagiannis GS, Treacy A, Messenger D, Grin A, Kirsch R, Riddell RH, et al. Expression patterns of bone morphogenetic protein antagonists in colorectal cancer desmoplastic invasion fronts. Mol Oncol (2014) 8:1240–52. doi:10.1016/j.molonc.2014.04.004
Pubmed Abstract | Pubmed Full Text | CrossRef Full Text | Google Scholar
82. Lugli A, Karamitopoulou E, Zlobec I. Tumour budding: a promising parameter in colorectal cancer. Br J Cancer (2012) 106:1713–7. doi:10.1038/bjc.2012.127
83. Zlobec I, Terracciano L, Jass JR, Lugli A. Value of staining intensity in the interpretation of immunohistochemistry for tumor markers in colorectal cancer. Virchows Arch (2007) 451:763–9. doi:10.1007/s00428-007-0466-8
Pubmed Abstract | Pubmed Full Text | CrossRef Full Text | Google Scholar
84. Zlobec I, Lugli A. Invasive front of colorectal cancer: dynamic interface of pro-/anti-tumor factors. World J Gastroenterol (2009) 15:5898–906. doi:10.3748/wjg.15.5898
Pubmed Abstract | Pubmed Full Text | CrossRef Full Text | Google Scholar
Keywords: tumor budding, colorectal cancer, immunohistochemistry, EMT, tumor microenvironment, immunohistochemistry
Citation: Dawson H and Lugli A (2015) Molecular and pathogenetic aspects of tumor budding in colorectal cancer. Front. Med. 2:11. doi: 10.3389/fmed.2015.00011
Received: 12 January 2015; Paper pending published: 04 February 2015;
Accepted: 25 February 2015; Published online: 10 March 2015.
Edited by:
Luigi M. Terracciano, University Hospital Basel, SwitzerlandReviewed by:
Pierlorenzo Pallante, Consiglio Nazionale delle Ricerche (CNR), ItalyVenancio Avancini Alves, University of Sao Paulo School of Medicine, Brazil
Copyright: © 2015 Dawson and Lugli. This is an open-access article distributed under the terms of the Creative Commons Attribution License (CC BY). The use, distribution or reproduction in other forums is permitted, provided the original author(s) or licensor are credited and that the original publication in this journal is cited, in accordance with accepted academic practice. No use, distribution or reproduction is permitted which does not comply with these terms.
*Correspondence: Heather Dawson, Clinical Pathology Division, Institute of Pathology, University of Bern, Murtenstrasse 31, Bern 3010, Switzerland e-mail:aGVhdGhlci5kYXdzb25AcGF0aG9sb2d5LnVuaWJlLmNo