- Centre de Recherche de l’Institut Universitaire de Cardiologie et de Pneumologie de Québec, Faculté de Médecine, Département de Médecine, Université Laval, Québec City, QC, Canada
Asthma is an inflammatory disease usually characterized by increased Type 2 cytokines and by an infiltration of eosinophils to the airways. While the production of Type 2 cytokines has been associated with TH2 lymphocytes, increasing evidence indicates that group 2 innate lymphoid cells (ILC2) play an important role in the production of the Type 2 cytokines interleukin (IL)-5 and IL-13, which likely amplifies the recruitment of eosinophils from the blood to the airways. In that regard, recent asthma treatments have been focusing on blocking Type 2 cytokines, notably IL-4, IL-5, and IL-13. These treatments mainly result in decreased blood or sputum eosinophil counts as well as decreased asthma symptoms. This supports that therapies blocking eosinophil recruitment and activation are valuable tools in the management of asthma and its severity. Herein, we review the mechanisms involved in eosinophil and ILC2 recruitment to the airways, with an emphasis on eotaxins, other chemokines as well as their receptors. We also discuss the involvement of other chemoattractants, notably the bioactive lipids 5-oxo-eicosatetraenoic acid, prostaglandin D2, and 2-arachidonoyl-glycerol. Given that eosinophil biology differs between human and mice, we also highlight and discuss their responsiveness toward the different eosinophil chemoattractants.
Introduction
Asthma is a respiratory disease characterized by inflammation and hyperresponsiveness of the airways and roughly affects 300 million people worldwide (1). Eosinophils play a pivotal role in asthma by generating many mediators inducing bronchoconstriction and/or contributing to inflammation and remodeling (2). Airway eosinophilia is observed in many subjects with asthma and increases with disease severity and exacerbations (3). The anti-inflammatory treatment of asthma is primarily based on inhaled corticosteroids (4). The dose is adjusted to decrease eosinophil counts in the blood and/or in induced sputum, which results in a reduction of asthma exacerbations. However, the chronic use of corticosteroids is linked with significant systemic side effects even at low doses, and some severe asthmatics remain symptomatic and have high sputum eosinophil counts despite the use of high doses of corticosteroids (5). This stresses the need of developing new therapeutics that could limit both bronchoconstriction and inflammation.
Increased eosinophil numbers are observed in many asthmatics, notably those characterized by a Type 2-like inflammation, characterized by an increased production of the cytokines interleukin (IL)-4, IL-5, and IL-13 (6). As such, it is well accepted that the Type 2 cytokines IL-4, IL-5, and IL-13 are linked to increased eosinophil numbers, either by promoting eosinophil survival (IL-5) or by inducing the production of eosinophil chemoattractants (IL-4 and IL-13) (7, 8). While TH2 lymphocytes participate in the release of Type 2 cytokines, group 2 innate lymphoid cells (ILC2) are being increasingly recognized as a significant source of Type 2 cytokines as well (9, 10). Asthma treatments that focused on blocking Type 2 cytokines (IL-4, IL-5, and IL-13) decrease blood or sputum eosinophil counts and asthma symptoms in subjects with severe asthma presenting a high eosinophil count in their induced sputum (11–25). This article reviews the current evidence regarding eosinophil and ILC2 chemoattractants and their involvement in asthma and its severity.
Discovery Timeline of the Main Eosinophil Chemoattractants
The extensive investigation of how eosinophils were recruited really began in the 1970s. Complement component 5a (C5a) has been known to induce guinea pig eosinophil migration since 1970 (26–29), and its impact on human eosinophils was documented in 1973 (26). Histamine was next documented as an eosinophil chemoattractant in 1975 (30) although its effect is limited (31–34).
In 1980s, other eosinophil chemoattractants were characterized, notably platelet-activating factor (PAF), leukotriene (LT) B4, and N-formylmethionyl-leucyl-phenylalanine (fMLP). Numerous reports indicate that PAF induces the migration of eosinophils (29, 35–41). Even if LTB4 is mainly characterized as a neutrophil chemoattractant, it also induces human eosinophil migration (29, 37, 42, 43). fMLP is a weak chemoattractant for eosinophil migration: some studies unraveled a weak migration of eosinophils (29, 37, 44, 45) while others did not find any effect (38, 46).
The expansion of the chemokine field in the 1990s allowed the characterization of additional eosinophil chemoattractants. CCL5 [regulated on activation, normal T cell expressed and secreted (RANTES)] was the first chemokine documented as a human eosinophil chemoattractant in 1992 (47) and was shown to induce both the migration and transmigration of human eosinophils (48–57). The effect of CCL3 (MIP-1α) on human eosinophil migration was also evaluated in 1992 (47). However, the ability of CCL3 as an eosinophil chemoattractant is low, as later reports indicated that at optimal concentration, the CCL3-induced migration of eosinophil corresponded to about 33% of that induced by CCL5 (48, 52, 57). Of note, one study showed that ~20% of individuals responded to CCL3 to the same extent than CCL11, while the others poorly responded to CCL3 and this was linked to CCR1 (58). In mid-1990s, other chemokines were tested for their ability to elicit human eosinophil migration, notably CCL7 (MCP-3), CCL8 (MCP-2), and CCL13 (MCP-4) (34, 48, 50–53, 55–57, 59, 60). However, their impact on human eosinophil migration was limited.
The discovery of eotaxins was a substantial leap forward in understanding how eosinophils were selectively recruited into the tissues. CCL11 (eotaxin-1) was first discovered by Jose et al. in guinea pigs (61, 62). Two years later it was confirmed as a selective chemoattractant of human eosinophils in 1996 (63) and several studies confirmed its potency in several migration models (55, 64–66). A year later, CCL24 (eotaxin-2) was discovered (67) and was confirmed as being as efficient as CCL11 (34, 55–57, 65). Last but not the least, CCL26 (eotaxin-3) was discovered in 1999 (68, 69), and it is the most efficient eotaxin to induce the migration or transmigration of asthmatic eosinophils (65). Of note, CCL26 appears also critical for eosinophil migration/tissue eosinophilia in other human disorders characterized by eosinophil recruitment, notably eosinophilic esophagitis and Churg–Strauss syndrome (70, 71).
It was also in the mid-1990s that additional bioactive lipids from the 5-lipoxygenase pathway were documented as human eosinophil chemoattractants. 5-Oxo-eicosatetraenoic acid (5-KETE) was identified as a potent chemoattractant of eosinophils in 1996 (72, 73). To this date, 5-KETE is the most efficient human eosinophil chemotactic factor in cellulo (41, 43, 65, 66). LTD4 was the first cysteinyl leukotriene (CysLTs) to be defined as a direct chemoattractant of human eosinophils (74) but induces a weak migration (75–78). It was also reported that LTC4 and LTE4 induce an eosinophil migration comparable to LTD4 (79).
The new millennia also expanded our knowledge on how human eosinophils could be recruited into the tissue. In that regard, CXCL12 (SDF-1) was shown to induce the recruitment of eosinophils (65, 80, 81). Furthermore, a 2001 study demonstrated that prostaglandin (PG) D2 selectively induced the migration of eosinophils, Th2 lymphocytes cells, and basophils (82), and increasing evidence support the development of DP2/CRTH2 antagonists for the management of asthma (83). However, PGD2 seems to induce a limited recruitment of eosinophils (66, 84–88). Of note, PGD2 increases CCL11- and 5-KETE-induced-eosinophil migration (87). Finally, in 2004, the endocannabinoid 2-arachidonoyl-glycerol (2-AG) was identified as an eosinophil chemoattractant (89); this effect of 2-AG involves the CB2 receptor and is largely potentiated by IL-3, IL-5, and GM-CSF (66, 90, 91).
Human Eosinophil Recruitment and Asthma
As underscored in the previous section, many soluble mediators and chemokines can induce human eosinophil recruitment and thus participate in asthma pathogenesis. In this section, we review how these chemoattractants contribute to eosinophil recruitment in a context of asthma. A differential eosinophil recruitment could be observed in asthma severity and/or during asthma exacerbations if there is a dysregulation in the release of the different chemoattractants or their receptors, notably by desensitization or internalization. To this end, our data (Figure 1) indicate that with the exception of the CXCR4 and the CB2 receptors, the expression of chemoattractant receptors do not change, at the mRNA level, in human eosinophils isolated from the blood of healthy subjects, mild and severe eosinophilic asthmatics, as defined in Ref. (92). This supports the notion that perhaps the increased recruitment of eosinophils is rather the consequence of increased chemoattractants in the bronchial tissue.
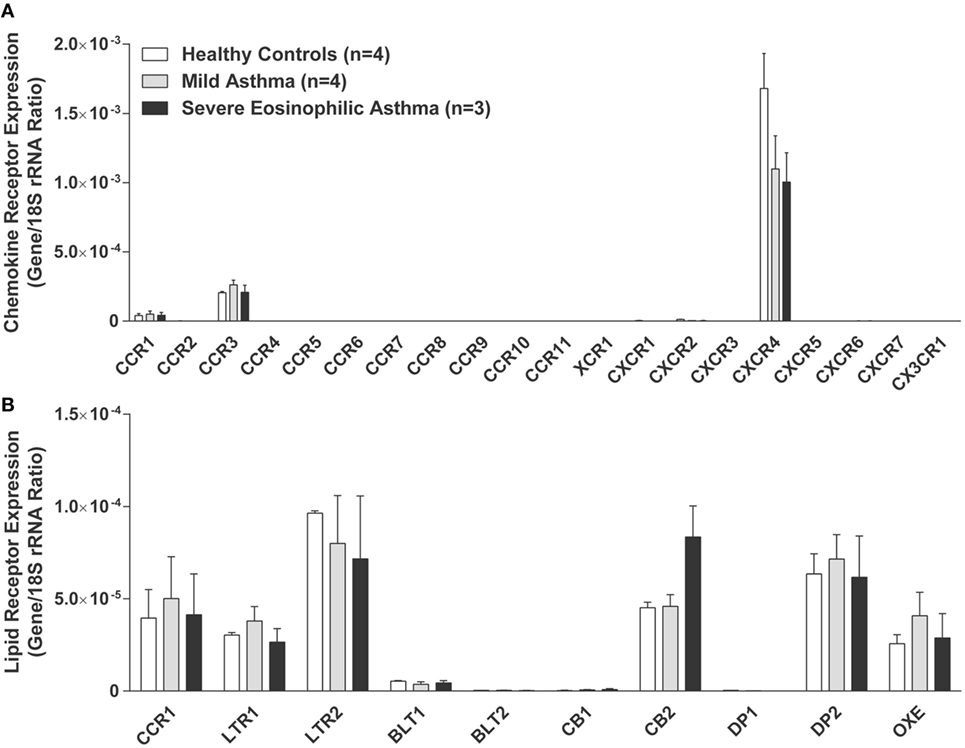
Figure 1. Expression of chemokines and lipid mediator receptors by human eosinophils. Human eosinophils were isolated from the blood of healthy controls, mild asthmatics, and severe eosinophilic asthmatics as defined and described in Ref. (92). mRNAs were quantitated by qPCR array using a custom qPCR array (RT2 Profiler qPCR Multiplex Array Kit, Qiagen, ON, Canada). Chemokine receptor expression (A) and bioactive lipid receptor expression (B) are represented by the ratio between mRNAs and 18S rRNA control. Results are the mean (±SEM) of 3–4 donors for each group. Approval from the local ethics committee was obtained, and all volunteers signed an informed consent form.
Chemokines
The most studied chemokines in asthma are CCL5 and eotaxins, probably because their levels are usually increased in asthmatics compared to healthy controls in all body fluids tested, namely bronchoalveolar lavages (BAL), induced sputum, blood, and bronchial biopsies (92–115). Moreover, these chemokines are linked to poor asthma control and increased eosinophil recruitment to the airways. Indeed, CCL5 levels are greater in induced sputum from poorly controlled asthmatics than from controlled asthmatics (116, 117); subjects undergoing acute exacerbations have higher CCL11 levels in induced sputum and plasma samples than subjects with stable asthma or healthy controls (111, 118–120); and CCL24 and CCL26 expression in airway epithelial cells are associated with lower forced expiratory volume in 1 s (FEV1), more asthma exacerbations, and increased sputum eosinophil counts (92, 121). It is not clear whether one chemokine is more important than the others and if we could target these chemotactic proteins to limit eosinophil recruitment and asthma exacerbation. In that regard, different studies evaluated the expression of these chemokines during allergen challenges, and the obtained data rather indicate that eosinophil-recruiting chemokines are not necessarily present at the same time and might have different as well as overlapping roles. CCL5 levels correlate with eosinophil counts in BAL 4 h after the challenge (122), but not 24 h after the challenge (123). CCL11 levels are increased in BAL, induced sputum and bronchial biopsies of asthmatics, and are associated with eosinophil numbers 4 and 24 h after the challenge (104, 124, 125). That being said, one study reported that CCL11 levels are similar in bronchial biopsies from asthmatics before and 24 h after allergen challenge (103). CCL24 expression is significantly increased in bronchial mucosa from asthmatics 48 h after allergen challenge (126), but is similar before and 24 h after allergen challenge (103). As for CCL26, its expression in bronchial biopsies increases 24 and 48 h after allergen challenge (103, 126), but its expression in bronchial submucosa did not correlate with eosinophil counts 48 h after allergen challenge (126). Additionally, some research groups documented the impact of these chemokines on eosinophil migration in asthma in cellulo. CCL11 and CCL26 induce a greater migration of eosinophils from asthmatics than from healthy subjects (65, 127). Finally, while most evidence reflects an important role of CCL5 and the eotaxins in asthma, some studies reported that there was no increase in CCL5 or eotaxin expression in BAL, airway epithelium brushings, or bronchial biopsies between asthmatics and healthy controls (92, 103, 121, 128, 129).
Studies on CCL3, CCL7, CCL8, CCL13, and CXCL12 in relation with asthma are limited. Among the latter, CCL13 is better associated with eosinophils and asthma. Its expression is higher in BAL, bronchial biopsies, induced sputum, and plasma samples from asthmatics than from healthy controls (99, 100, 105, 130, 131). One study reported increased CCL3 levels in BAL from asthmatics compared to healthy controls (93). Increased CCL7 levels and CCL7-expressing cells are found in bronchial biopsies and BAL from asthmatics compared to healthy controls (94, 95, 100), and serum CCL8 levels are higher in asthmatics compared to healthy controls (132). CXCL12 levels in bronchial mucosa and BAL are greater in asthmatics than in healthy controls (133, 134), and CXCL12 levels in BAL correlate with eosinophil numbers (134).
Lipid Mediators and Others
Other soluble mediators might also participate in the recruitment of eosinophils in asthma. In that regard, CysLT1 receptor blockade usually decreases eosinophil counts, although it is not clear whether this is a direct or indirect effect (135–144). LTB4, histamine, C5a, and PGD2 are all associated with asthma, but their involvement in eosinophil recruitment in asthma is not well defined. Even if LTB4 levels in blood and exhaled breath condensate are increased in asthma (145–147), the LTB4 receptor antagonist, LY293111, decreases neutrophil but not eosinophil counts in BAL from asthmatics (148). As for PGD2, some studies demonstrated similar PGD2 levels in BAL or induced sputum of asthmatics, atopics, and healthy subjects (149–152), but its levels can increase in the BAL after an allergen challenge (149, 153, 154). Of note, the antagonism of the PGD2 receptor 2 (DP2/CRTH2) improves lung function and the quality of life of asthmatics compared to placebo (155, 156). Finally, C5a levels are increased in BAL and in induced sputum from asthmatics compared to healthy controls after an allergen challenge (157, 158), and a haplotype of the C5a gene was identified to be protective against asthma (159).
As for PAF, 5-KETE, fMLP, and 2-AG, their association with asthma is not well documented and this requires further investigations. For example, we have no idea to which extent 2-AG and 5-KETE levels are modulated in asthma and its severity.
Asthma Severity
As underscored with the data from the allergen challenges presented in the previous section, it is not possible to pinpoint one chemoattractant explaining the recruitment of human eosinophils. They rather indicate that they collaborate together and that they might be involved at different times during the asthmatic response. In addition, it is possible that the mediators responsible for eosinophil recruitment might also change as the disease worsens. For example, CCL11 and/or CCL26 levels are greater in induced sputum from severe or moderate asthmatics than from mild asthmatics or healthy controls (92, 160). In plasma samples, CCL11 levels are associated with asthma severity and are not significantly affected by corticosteroid treatment (161). Coleman et al. demonstrated that CCL24 and CCL26, but not CCL11, mRNA expression in bronchial epithelium increases with asthma severity and is associated with sputum eosinophil counts, lower FEV1, and more asthma exacerbations (121). In contrast, subjects with severe eosinophilic asthma have lower CCL24 levels in bronchoalveolar lavage fluids and similar CCL24 levels in bronchial epithelial cells compared to healthy controls (92, 121). For CCL5, Saad-El-Din demonstrated that serum CCL5 levels are greater in subjects with severe or moderate asthma as compared to subjects with mild asthma and are associated with blood eosinophil number (114). As for CXCL12, it induces a greater migration of corticosteroid-treated eosinophils than untreated eosinophils and that the expression of the CXCL12 receptor, CXCR4, increases in corticosteroid-treated eosinophils (80), raising the possibility that CXCL12 plays a more important role in unstable severe eosinophilic asthmatics which are taking large doses of corticosteroids.
In asthma, CysLTs levels in induced sputum are increased in moderate asthmatics compared to severe asthmatics and healthy controls (162). Also, similar sputum CysLTs levels were found in severe eosinophilic and non-eosinophilic asthmatics (162). In contrast, exhaled breath condensate levels of CysLTs correlate with asthma severity (163). In mild-to-moderate asthmatics or eosinophilic asthmatics, the CysLT1 antagonist montelukast, alone or in combination with corticosteroids, decreases sputum or blood eosinophil counts (136, 138, 141, 164). On the other hand, severe eosinophilic asthmatics, severe non-eosinophilic asthmatics, and moderate uncontrolled asthmatics have similar sputum or blood eosinophil counts between montelukast-treated and placebo-treated individuals or between montelukast/corticosteroid-treated and corticosteroid-treated asthmatics (165–167). Of note, PGD2 and DP2/CRTH2 levels are increased in asthma severity in BAL (151, 152), and the DP2/CRTH2 antagonist OC000459 improves FEV1 and the quality of life of subjects with eosinophilic uncontrolled asthma and steroid-free subjects with moderate persistent asthma (155, 156). Finally, C5a receptor expression on bronchial epithelium is greater in subjects with fatal asthma than mild asthmatics and healthy controls (168).
Of Mice and Men
The potential and/or documented roles of multiple chemoattractant involved in eosinophil recruitment in asthma underscore the need to revisit this concept and to establish when and how those actors are involved. The development of experimental asthma models with mice, rats, or guinea pigs has been very helpful to broaden our knowledge about asthma pathogenesis and to identify some eosinophil and ILC2 chemoattractants in allergic asthma. However, eosinophils and their functional responses are very different between species (169). In that regard, some chemoattractants and their receptors in humans are not expressed in mice. For instance, the 5-KETE receptor OXE is not expressed in mice (170, 171), resulting in an absence of 5-KETE-induced eosinophil migration (170). Additionally, CCL26 is not expressed in mice (170) and human CCL26 does not induce the migration of mouse eosinophils (172, 173). Furthermore, CCL5 does not induce the migration of mouse eosinophils (172, 174–176). Globally, three of the most efficient human eosinophil chemoattractants described so far (CCL5, CCL26, and 5-KETE) do not induce the migration of eosinophils from mice, illustrating major differences in eosinophil recruitment between mice and humans and underscoring that transposing eosinophil recruitment data from mice to humans might be hazardous. The impact of the different chemoattractants on the migration of eosinophils from humans and mice is summarized in Table 1 in which the number of migrated eosinophils in different migration assays is compared. It should be kept in mind that the presented data involve different eosinophil migration assays and that a true comparison between the presented chemoattractant is somewhat subjective. This is why we defined the different efficiencies using %migration intervals.
Mediators Promoting ILC2 Recruitment
First identified in 2010, ILC2 are defined as lymphoid cells lacking specific lymphocytes lineage markers and the expression of the DP2/CRTH2 and ST2, the IL-33 receptor (214–218). They produce, in response to IL-25, IL-33 or thymic stromal lymphopoietin (TSLP), large amounts of the TH2 cytokines IL-5, IL-13 and, to a lesser extent, IL-4. Of note, the number of ILC2 correlate with sputum eosinophils in allergic asthma (219). This suggests that ILC2 might play an important role in asthma (220, 221), especially by directly or indirectly modulating eosinophil survival/recruitment. However, the cellular mechanisms by which ILC2 are recruited to the lungs remain poorly defined and few studies addressed the impact of chemokines or bioactive lipids on the migration of ILC2.
Since IL-25, IL-33, and TSLP are potent activators of ILC2, their ability to induce the migration of ILC2 was first evaluated. IL-33 and TSLP induce a weak migration of human ILC2 (218, 222, 223). However, the impact of IL-25 remains a matter of debate, as one study reported a weak IL-25-induced ILC2 migration (223), while another found no effect of IL-25 (218). PGD2 and CysLTs are defined as potent chemoattractants of ILC2. Indeed, PGD2 is almost five times more potent than IL-33 (218, 224), and the PGD2-induced migration is greater in ILC2 from allergic subjects compared to healthy subjects (224). Furthermore, mice lacking DP2/CRTH2 or treated with a DP2/CRTH2 antagonist have lower ILC2 levels in the lungs after intranasal administration of PGD2 (225). As for CysLTs, ILC2 express the receptor CysLTR1 and its expression is increased in atopic subjects (223, 226, 227). Interestingly, a research group recently demonstrated that all CysLTs induce the migration of human ILC2 in vitro, LTE4 ≫ LTD4 > LTC4 ≈ IL-33, indicating that perhaps another CysLT receptor might be involved in this process (223).
Although only IL-33, TSLP, PGD2, and the CysLTs have been identified as chemoattractants of ILC2, some studies reported that human ILC2 express the chemokine receptor CCR4 and mouse ILC2 express the LTB4 receptor BLT1 (222, 227). Furthermore, TGF-β increases the basal migration of murine ILC2, which suggests that it could enhance their response to other chemoattractants (228). Other studies are thus needed to delineate how ILC2 migrate to the bronchial tissue.
Concluding Remarks and Future Directions
This review highlights that many chemokines and soluble mediators are very good to excellent at inducing the migration of eosinophils ex vivo and their recruitment in vivo. This underscores that targeting eosinophil recruitment as a therapeutic approach in asthma might not be readily successful, as suggested with the attempt at blocking the eotaxin receptor CCR3 (229). Additionally, many questions remain unanswered. For instance, it remains unclear when all those chemoattractants actually play a role during the asthmatic response and this needs to be addressed, notably by defining the presence of all eosinophil and ILC2 chemoattractants in the same samples and at different stages of the disease/exacerbation. Experimental restrictions such as specie (mouse vs. humans) or the number of chemoattractants being investigated in a given study make the obtained data a little blurry, sometimes raising more questions than answering them. In addition, the involvement of the different chemoattractants as the disease worsens remains anecdotal. Given that severe asthmatics are frequently older than mild and moderate asthmatics, it is possible that the set of chemoattractant changes with age and perhaps, with gender as well [keeping in mind that aging modulates sex hormones, which could affect the synthesis of the different chemoattractants as it is the case for 5-lipoxygenase derivatives (230)]. Another important aspect of this review is the illustration that some of the best chemoattractants for human eosinophils are not present or are effectless in murine models (Table 1), raising the question that perhaps data obtained from animal models should be taken cautiously until they are validated in humans. Finally, if ILC2 play a prominent role in asthma as it is proposed from mouse data, it will be of crucial importance to rapidly understand the regulation of their recruitment into the airways, by defining which chemokines, lipids, and other chemoattractants are promoting their recruitment both in mice and humans, as well as all the receptors involved in that process.
Author Contributions
All the authors listed have made a substantial, direct, and intellectual contribution to the work and approved it for publication.
Conflict of Interest Statement
The authors declare that the research was conducted in the absence of any commercial or financial relationships that could be construed as a potential conflict of interest.
Acknowledgments
This work was supported by a grant to ML and NF from the J-D-Bégin Research Chair. M-CL is supported by a doctoral training award from the Canadian Institutes of Health Research (CIHR, GSD-141736). A-SA was supported by a doctoral training award the Fond de recherche du Québec—Santé (FRQS). M-CL and A-SA were supported by the CIHR—Québec Respiratory Health Network Training Program. ML and NF are members of the inflammation regroupment of the Respiratory Health Network of the FRQS.
References
1. Kroegel C. Global initiative for asthma (GINA) guidelines: 15 years of application. Expert Rev Clin Immunol (2009) 5:239–49. doi:10.1586/eci.09.1
3. Aleman F, Lim HF, Nair P. Eosinophilic endotype of asthma. Immunol Allergy Clin North Am (2016) 36:559–68. doi:10.1016/j.iac.2016.03.006
4. Directors ABO. Standards for the diagnosis and care of patients with chronic obstructive pulmonary disease (COPD) and asthma. This official statement of the American Thoracic Society was adopted by the ATS board of directors, November 1986. Am Rev Respir Dis (1987) 136:225–44.
5. Walsh GM, Sexton DW, Blaylock MG. Corticosteroids, eosinophils and bronchial epithelial cells: new insights into the resolution of inflammation in asthma. J Endocrinol (2003) 178:37–43. doi:10.1677/joe.0.1780037
6. Fahy JV. Type 2 inflammation in asthma – present in most, absent in many. Nat Rev Immunol (2015) 15:57–65. doi:10.1038/nri3807
7. Busse WW, Lemanske RF Jr. Asthma. N Engl J Med (2001) 344:350–62. doi:10.1056/NEJM200102013440507
8. Lemanske RF Jr, Busse WW. 6. Asthma. J Allergy Clin Immunol (2003) 111:S502–19. doi:10.1067/mai.2003.94
9. Murdoch JR, Lloyd CM. Chronic inflammation and asthma. Mutat Res (2010) 690:24–39. doi:10.1016/j.mrfmmm.2009.09.005
10. Chang YJ, Dekruyff RH, Umetsu DT. The role of type 2 innate lymphoid cells in asthma. J Leukoc Biol (2013) 94:933–40. doi:10.1189/jlb.0313127
11. Haldar P, Brightling CE, Hargadon B, Gupta S, Monteiro W, Sousa A, et al. Mepolizumab and exacerbations of refractory eosinophilic asthma. N Engl J Med (2009) 360:973–84. doi:10.1056/NEJMoa0808991
12. Levine SJ, Wenzel SE. Narrative review: the role of Th2 immune pathway modulation in the treatment of severe asthma and its phenotypes. Ann Intern Med (2010) 152:232–7. doi:10.7326/0003-4819-152-4-201002160-00008
13. Corren J, Lemanske RF, Hanania NA, Korenblat PE, Parsey MV, Arron JR, et al. Lebrikizumab treatment in adults with asthma. N Engl J Med (2011) 365:1088–98. doi:10.1056/NEJMoa1106469
14. Pavord ID, Korn S, Howarth P, Bleecker ER, Buhl R, Keene ON, et al. Mepolizumab for severe eosinophilic asthma (DREAM): a multicentre, double-blind, placebo-controlled trial. Lancet (2012) 380:651–9. doi:10.1016/S0140-6736(12)60988-X
15. Pelaia G, Vatrella A, Maselli R. The potential of biologics for the treatment of asthma. Nat Rev Drug Discov (2012) 11:958–72. doi:10.1038/nrd3792
16. Piper E, Brightling C, Niven R, Oh C, Faggioni R, Poon K, et al. A phase II placebo-controlled study of tralokinumab in moderate-to-severe asthma. Eur Respir J (2013) 41:330–8. doi:10.1183/09031936.00223411
17. Wenzel S, Ford L, Pearlman D, Spector S, Sher L, Skobieranda F, et al. Dupilumab in persistent asthma with elevated eosinophil levels. N Engl J Med (2013) 368:2455–66. doi:10.1056/NEJMoa1304048
18. Brightling CE, Chanez P, Leigh R, O’Byrne PM, Korn S, She D, et al. Efficacy and safety of tralokinumab in patients with severe uncontrolled asthma: a randomised, double-blind, placebo-controlled, phase 2b trial. Lancet Respir Med (2015) 3:692–701. doi:10.1016/S2213-2600(15)00197-6
19. Hanania NA, Noonan M, Corren J, Korenblat P, Zheng Y, Fischer SK, et al. Lebrikizumab in moderate-to-severe asthma: pooled data from two randomised placebo-controlled studies. Thorax (2015) 70:748–56. doi:10.1136/thoraxjnl-2014-206719
20. Hanania NA, Korenblat P, Chapman KR, Bateman ED, Kopecky P, Paggiaro P, et al. Efficacy and safety of lebrikizumab in patients with uncontrolled asthma (LAVOLTA I and LAVOLTA II): replicate, phase 3, randomised, double-blind, placebo-controlled trials. Lancet Respir Med (2016) 4:781–96. doi:10.1016/S2213-2600(16)30265-X
21. Khorasanizadeh M, Eskian M, Assa’ad AH, Camargo CA Jr, Rezaei N. Efficacy and safety of benralizumab, a monoclonal antibody against IL-5Ralpha, in uncontrolled eosinophilic asthma. Int Rev Immunol (2016) 35:294–311. doi:10.3109/08830185.2015.1128901
22. Li J, Lin C, Du J, Xiao B, Du C, Sun J, et al. The efficacy and safety of reslizumab for inadequately controlled asthma with elevated blood eosinophil counts: a systematic review and meta-analysis. J Asthma (2017)54:300–7. doi:10.1080/02770903.2016.1212371
23. Ortega HG, Yancey SW, Mayer B, Gunsoy NB, Keene ON, Bleecker ER, et al. Severe eosinophilic asthma treated with mepolizumab stratified by baseline eosinophil thresholds: a secondary analysis of the DREAM and MENSA studies. Lancet Respir Med (2016) 4(7):549–56. doi:10.1016/S2213-2600(16)30031-5
24. Wenzel S, Castro M, Corren J, Maspero J, Wang L, Zhang B, et al. Dupilumab efficacy and safety in adults with uncontrolled persistent asthma despite use of medium-to-high-dose inhaled corticosteroids plus a long-acting beta2 agonist: a randomised double-blind placebo-controlled pivotal phase 2b dose-ranging trial. Lancet (2016) 388:31–44. doi:10.1016/S0140-6736(16)30307-5
25. Varricchi G, Bagnasco D, Ferrando M, Puggioni F, Passalacqua G, Canonica GW. Mepolizumab in the management of severe eosinophilic asthma in adults: current evidence and practical experience. Ther Adv Respir Dis (2017) 11:40–5. doi:10.1177/1753465816673303
26. Kay AB. Studies on eosinophil leucocyte migration. I. Eosinophil and neutrophil accumulation following antigen-antibody reactions in guinea-pig skin. Clin Exp Immunol (1970) 6:75–86.
27. Kay AB. Studies on eosinophil leucocyte migration. II. Factors specifically chemotactic for eosinophils and neutrophils generated from guinea-pig serum by antigen-antibody complexes. Clin Exp Immunol (1970) 7:723–37.
28. Kay AB, Shin HS, Austen KF. Selective attraction of eosinophils and synergism between eosinophil chemotactic factor of anaphylaxis (ECF-A) and a fragment cleaved from the fifth component of complement (C5a). Immunology (1973) 24:969–76.
29. Morita E, Schroder JM, Christophers E. Differential sensitivities of purified human eosinophils and neutrophils to defined chemotaxins. Scand J Immunol (1989) 29:709–16. doi:10.1111/j.1365-3083.1989.tb01175.x
30. Clark RA, Gallin JI, Kaplan AP. The selective eosinophil chemotactic activity of histamine. J Exp Med (1975) 142:1462–76. doi:10.1084/jem.142.6.1462
31. Turnbull LW, Evans DP, Kay AB. Human eosinophils, acidic tetrapeptides (ECF-A) and histamine. Interactions in vitro and in vivo. Immunology (1977) 32:57–63.
32. Wadee AA, Anderson R, Sher R. In vitro effects of histamine on eosinophil migration. Int Arch Allergy Appl Immunol (1980) 63:322–9. doi:10.1159/000232643
33. O’Reilly M, Alpert R, Jenkinson S, Gladue RP, Foo S, Trim S, et al. Identification of a histamine H4 receptor on human eosinophils – role in eosinophil chemotaxis. J Recept Signal Transduct Res (2002) 22:431–48. doi:10.1081/RRS-120014612
34. Ling P, Ngo K, Nguyen S, Thurmond RL, Edwards JP, Karlsson L, et al. Histamine H4 receptor mediates eosinophil chemotaxis with cell shape change and adhesion molecule upregulation. Br J Pharmacol (2004) 142:161–71. doi:10.1038/sj.bjp.0705899
35. Wardlaw AJ, Moqbel R, Cromwell O, Kay AB. Platelet-activating factor. A potent chemotactic and chemokinetic factor for human eosinophils. J Clin Invest (1986) 78:1701–6. doi:10.1172/JCI112765
36. Hakansson L, Venge P. Inhibition of neutrophil and eosinophil chemotactic responses to PAF by the PAF-antagonists WEB-2086, L-652,731, and SRI-63441. J Leukoc Biol (1990) 47:449–56.
37. Erger RA, Casale TB. Comparative studies indicate that platelet-activating factor is a relatively weak eosinophilotactic mediator. Am J Respir Cell Mol Biol (1995) 12:65–70. doi:10.1165/ajrcmb.12.1.7811471
38. Resnick MB, Colgan SP, Parkos CA, Delp-Archer C, Mcguirk D, Weller PF, et al. Human eosinophils migrate across an intestinal epithelium in response to platelet-activating factor. Gastroenterology (1995) 108:409–16. doi:10.1016/0016-5085(95)90067-5
39. Erger RA, Casale TB. Eosinophil migration in response to three molecular species of platelet activating factor. Inflamm Res (1996) 45:265–7. doi:10.1007/BF02280988
40. Okada S, Kita H, George TJ, Gleich GJ, Leiferman KM. Transmigration of eosinophils through basement membrane components in vitro: synergistic effects of platelet-activating factor and eosinophil-active cytokines. Am J Respir Cell Mol Biol (1997) 16:455–63. doi:10.1165/ajrcmb.16.4.9115757
41. Guilbert M, Ferland C, Bosse M, Flamand N, Lavigne S, Laviolette M. 5-Oxo-6,8,11,14-eicosatetraenoic acid induces important eosinophil transmigration through basement membrane components: comparison of normal and asthmatic eosinophils. Am J Respir Cell Mol Biol (1999) 21:97–104. doi:10.1165/ajrcmb.21.1.3517
42. Casale TB, Erger RA, Little MM. Platelet-activating factor-induced human eosinophil transendothelial migration: evidence for a dynamic role of the endothelium. Am J Respir Cell Mol Biol (1993) 8:77–82. doi:10.1165/ajrcmb/8.1.77
43. O’Flaherty JT, Kuroki M, Nixon AB, Wijkander J, Yee E, Lee SL, et al. 5-Oxo-eicosatetraenoate is a broadly active, eosinophil-selective stimulus for human granulocytes. J Immunol (1996) 157:336–42.
44. Warringa RA, Koenderman L, Kok PT, Kreukniet J, Bruijnzeel PL. Modulation and induction of eosinophil chemotaxis by granulocyte-macrophage colony-stimulating factor and interleukin-3. Blood (1991) 77:2694–700.
45. Warringa RA, Mengelers HJ, Raaijmakers JA, Bruijnzeel PL, Koenderman L. Upregulation of formyl-peptide and interleukin-8-induced eosinophil chemotaxis in patients with allergic asthma. J Allergy Clin Immunol (1993) 91:1198–205. doi:10.1016/0091-6749(93)90323-8
46. Roberts RL, Gallin JI. Rapid method for isolation of normal human peripheral blood eosinophils on discontinuous Percoll gradients and comparison with neutrophils. Blood (1985) 65:433–40.
47. Rot A, Krieger M, Brunner T, Bischoff SC, Schall TJ, Dahinden CA. RANTES and macrophage inflammatory protein 1 alpha induce the migration and activation of normal human eosinophil granulocytes. J Exp Med (1992) 176:1489–95. doi:10.1084/jem.176.6.1489
48. Dahinden CA, Geiser T, Brunner T, von Tscharner V, Caput D, Ferrara P, et al. Monocyte chemotactic protein 3 is a most effective basophil- and eosinophil-activating chemokine. J Exp Med (1994) 179:751–6. doi:10.1084/jem.179.2.751
49. Ebisawa M, Yamada T, Bickel C, Klunk D, Schleimer RP. Eosinophil transendothelial migration induced by cytokines. III. Effect of the chemokine RANTES. J Immunol (1994) 153:2153–60.
50. Noso N, Proost P, Van DJ, Schroder JM. Human monocyte chemotactic proteins-2 and 3 (MCP-2 and MCP-3) attract human eosinophils and desensitize the chemotactic responses towards RANTES. Biochem Biophys Res Commun (1994) 200:1470–6. doi:10.1006/bbrc.1994.1616
51. Weber M, Uguccioni M, Ochensberger B, Baggiolini M, Clark-Lewis I, Dahinden CA. Monocyte chemotactic protein MCP-2 activates human basophil and eosinophil leukocytes similar to MCP-3. J Immunol (1995) 154:4166–72.
52. Kitayama J, Carr MW, Roth SJ, Buccola J, Springer TA. Contrasting responses to multiple chemotactic stimuli in transendothelial migration: heterologous desensitization in neutrophils and augmentation of migration in eosinophils. J Immunol (1997) 158:2340–9.
53. Stellato C, Collins P, Ponath PD, Soler D, Newman W, La RG, et al. Production of the novel C-C chemokine MCP-4 by airway cells and comparison of its biological activity to other C-C chemokines. J Clin Invest (1997) 99:926–36. doi:10.1172/JCI119257
54. Liu L, Zuurbier AE, Mul FP, Verhoeven AJ, Lutter R, Knol EF, et al. Triple role of platelet-activating factor in eosinophil migration across monolayers of lung epithelial cells: eosinophil chemoattractant and priming agent and epithelial cell activator. J Immunol (1998) 161:3064–70.
55. Nagase H, Yamaguchi M, Jibiki S, Yamada H, Ohta K, Kawasaki H, et al. Eosinophil chemotaxis by chemokines: a study by a simple photometric assay. Allergy (1999) 54:944–50. doi:10.1034/j.1398-9995.1999.00184.x
56. Shahabuddin S, Ponath P, Schleimer RP. Migration of eosinophils across endothelial cell monolayers: interactions among IL-5, endothelial-activating cytokines, and C-C chemokines. J Immunol (2000) 164:3847–54. doi:10.4049/jimmunol.164.7.3847
57. Umland SP, Wan Y, Shortall J, Shah H, Jakway J, Garlisi CG, et al. Receptor reserve analysis of the human CCR3 receptor in eosinophils and CCR3-transfected cells. J Leukoc Biol (2000) 67:441–7.
58. Phillips RM, Stubbs VE, Henson MR, Williams TJ, Pease JE, Sabroe I. Variations in eosinophil chemokine responses: an investigation of CCR1 and CCR3 function, expression in atopy, and identification of a functional CCR1 promoter. J Immunol (2003) 170:6190–201. doi:10.4049/jimmunol.170.12.6190
59. Uguccioni M, Loetscher P, Forssmann U, Dewald B, Li H, Lima SH, et al. Monocyte chemotactic protein 4 (MCP-4), a novel structural and functional analogue of MCP-3 and eotaxin. J Exp Med (1996) 183:2379–84. doi:10.1084/jem.183.5.2379
60. Luster AD. Chemokines – chemotactic cytokines that mediate inflammation. N Engl J Med (1998) 338:436–45. doi:10.1056/NEJM199802123380706
61. Jose PJ, Adcock IM, Griffiths-Johnson DA, Berkman N, Wells TN, Williams TJ, et al. Eotaxin: cloning of an eosinophil chemoattractant cytokine and increased mRNA expression in allergen-challenged guinea-pig lungs. Biochem Biophys Res Commun (1994) 205:788–94. doi:10.1006/bbrc.1994.2734
62. Jose PJ, Griffiths-Johnson DA, Collins PD, Walsh DT, Moqbel R, Totty NF, et al. Eotaxin: a potent eosinophil chemoattractant cytokine detected in a guinea pig model of allergic airways inflammation. J Exp Med (1994) 179:881–7. doi:10.1084/jem.179.3.881
63. Garcia-Zepeda EA, Rothenberg ME, Ownbey RT, Celestin J, Leder P, Luster AD. Human eotaxin is a specific chemoattractant for eosinophil cells and provides a new mechanism to explain tissue eosinophilia. Nat Med (1996) 2:449–56. doi:10.1038/nm0496-449
64. Kikuchi I, Kikuchi S, Kobayashi T, Hagiwara K, Sakamoto Y, Kanazawa M, et al. Eosinophil trans-basement membrane migration induced by interleukin-8 and neutrophils. Am J Respir Cell Mol Biol (2006) 34:760–5. doi:10.1165/rcmb.2005-0303OC
65. Provost V, Larose MC, Langlois A, Rola-Pleszczynski M, Flamand N, Laviolette M. CCL26/eotaxin-3 is more effective to induce the migration of eosinophils of asthmatics than CCL11/eotaxin-1 and CCL24/eotaxin-2. J Leukoc Biol (2013) 94:213–22. doi:10.1189/jlb.0212074
66. Larose MC, Turcotte C, Chouinard F, Ferland C, Martin C, Provost V, et al. Mechanisms of human eosinophil migration induced by the combination of IL-5 and the endocannabinoid 2-arachidonoyl-glycerol. J Allergy Clin Immunol (2014) 133:1480–2.e1481–1483. doi:10.1016/j.jaci.2013.12.1081
67. Forssmann U, Uguccioni M, Loetscher P, Dahinden CA, Langen H, Thelen M, et al. Eotaxin-2, a novel CC chemokine that is selective for the chemokine receptor CCR3, and acts like eotaxin on human eosinophil and basophil leukocytes. J Exp Med (1997) 185:2171–6. doi:10.1084/jem.185.12.2171
68. Kitaura M, Suzuki N, Imai T, Takagi S, Suzuki R, Nakajima T, et al. Molecular cloning of a novel human CC chemokine (Eotaxin-3) that is a functional ligand of CC chemokine receptor 3. J Biol Chem (1999) 274:27975–80. doi:10.1074/jbc.274.39.27975
69. Shinkai A, Yoshisue H, Koike M, Shoji E, Nakagawa S, Saito A, et al. A novel human CC chemokine, eotaxin-3, which is expressed in IL-4-stimulated vascular endothelial cells, exhibits potent activity toward eosinophils. J Immunol (1999) 163:1602–10.
70. Blanchard C, Wang N, Stringer KF, Mishra A, Fulkerson PC, Abonia JP, et al. Eotaxin-3 and a uniquely conserved gene-expression profile in eosinophilic esophagitis. J Clin Invest (2006) 116:536–47. doi:10.1172/JCI26679
71. Polzer K, Karonitsch T, Neumann T, Eger G, Haberler C, Soleiman A, et al. Eotaxin-3 is involved in Churg-Strauss syndrome – a serum marker closely correlating with disease activity. Rheumatology (Oxford) (2008) 47:804–8. doi:10.1093/rheumatology/ken033
72. Powell WS, Chung D, Gravel S. 5-Oxo-6,8,11,14-eicosatetraenoic acid is a potent stimulator of human eosinophil migration. J Immunol (1995) 154:4123–32.
73. Powell WS, Gravel S, Gravelle F. Formation of a 5-oxo metabolite of 5,8,11,14,17-eicosapentaenoic acid and its effects on human neutrophils and eosinophils. J Lipid Res (1995) 36:2590–8.
74. Spada CS, Krauss AH, Nieves AL, Woodward DF. Effects of leukotrienes B4 (LTB4) and D4 (LTD4) on motility of isolated normodense human eosinophils and neutrophils. Adv Exp Med Biol (1997) 400B:699–706.
75. Ohshima N, Nagase H, Koshino T, Miyamasu M, Yamaguchi M, Hirai K, et al. A functional study on CysLT(1) receptors in human eosinophils. Int Arch Allergy Immunol (2002) 129:67–75. doi:10.1159/000065175
76. Saito K, Nagata M, Kikuchi I, Sakamoto Y. Leukotriene D4 and eosinophil transendothelial migration, superoxide generation, and degranulation via beta2 integrin. Ann Allergy Asthma Immunol (2004) 93:594–600. doi:10.1016/S1081-1206(10)61269-0
77. Nagata M, Saito K, Kikuchi I, Hagiwara K, Kanazawa M. Effect of the cysteinyl leukotriene antagonist pranlukast on transendothelial migration of eosinophils. Int Arch Allergy Immunol (2005) 137(Suppl 1):2–6. doi:10.1159/000085424
78. Hemelaers L, Henket M, Sele J, Bureau F, Louis R. Cysteinyl-leukotrienes contribute to sputum eosinophil chemotactic activity in asthmatics. Allergy (2006) 61:136–9. doi:10.1111/j.1398-9995.2006.00993.x
79. Fregonese L, Silvestri M, Sabatini F, Rossi GA. Cysteinyl leukotrienes induce human eosinophil locomotion and adhesion molecule expression via a CysLT1 receptor-mediated mechanism. Clin Exp Allergy (2002) 32:745–50. doi:10.1046/j.1365-2222.2002.01384.x
80. Nagase H, Miyamasu M, Yamaguchi M, Fujisawa T, Ohta K, Yamamoto K, et al. Expression of CXCR4 in eosinophils: functional analyses and cytokine-mediated regulation. J Immunol (2000) 164:5935–43. doi:10.4049/jimmunol.164.11.5935
81. Smith SG, Imaoka H, Punia N, Irshad A, Janssen LL, Sehmi R, et al. The effect of PPAR agonists on the migration of mature and immature eosinophils. PPAR Res (2012) 2012:235231. doi:10.1155/2012/235231
82. Hirai H, Tanaka K, Yoshie O, Ogawa K, Kenmotsu K, Takamori Y, et al. Prostaglandin D2 selectively induces chemotaxis in T helper type 2 cells, eosinophils, and basophils via seven-transmembrane receptor CRTH2. J Exp Med (2001) 193:255–61. doi:10.1084/jem.193.2.255
83. Kupczyk M, Kuna P. Targeting the PGD2/CRTH2/DP1 signaling pathway in asthma and allergic disease: current status and future perspectives. Drugs (2017) 77(12):1281–94. doi:10.1007/s40265-017-0777-2
84. Gervais FG, Cruz RP, Chateauneuf A, Gale S, Sawyer N, Nantel F, et al. Selective modulation of chemokinesis, degranulation, and apoptosis in eosinophils through the PGD2 receptors CRTH2 and DP. J Allergy Clin Immunol (2001) 108:982–8. doi:10.1067/mai.2001.119919
85. Heinemann A, Schuligoi R, Sabroe I, Hartnell A, Peskar BA. Delta 12-prostaglandin J2, a plasma metabolite of prostaglandin D2, causes eosinophil mobilization from the bone marrow and primes eosinophils for chemotaxis. J Immunol (2003) 170:4752–8. doi:10.4049/jimmunol.170.9.4752
86. Bohm E, Sturm GJ, Weiglhofer I, Sandig H, Shichijo M, Mcnamee A, et al. 11-Dehydro-thromboxane B2, a stable thromboxane metabolite, is a full agonist of chemoattractant receptor-homologous molecule expressed on TH2 cells (CRTH2) in human eosinophils and basophils. J Biol Chem (2004) 279:7663–70. doi:10.1074/jbc.M310270200
87. Schratl P, Sturm EM, Royer JF, Sturm GJ, Lippe IT, Peskar BA, et al. Hierarchy of eosinophil chemoattractants: role of p38 mitogen-activated protein kinase. Eur J Immunol (2006) 36:2401–9. doi:10.1002/eji.200535672
88. Chiba T, Ueki S, Ito W, Kato H, Kamada R, Takeda M, et al. The opposing role of two prostaglandin D2 receptors, DP and CRTH2, in human eosinophil migration. Ann Allergy Asthma Immunol (2011) 106:511–7. doi:10.1016/j.anai.2011.01.027
89. Oka S, Ikeda S, Kishimoto S, Gokoh M, Yanagimoto S, Waku K, et al. 2-arachidonoylglycerol, an endogenous cannabinoid receptor ligand, induces the migration of EoL-1 human eosinophilic leukemia cells and human peripheral blood eosinophils. J Leukoc Biol (2004) 76:1002–9. doi:10.1189/jlb.0404252
90. Kishimoto S, Oka S, Gokoh M, Sugiura T. Chemotaxis of human peripheral blood eosinophils to 2-arachidonoylglycerol: comparison with other eosinophil chemoattractants. Int Arch Allergy Immunol (2006) 140(Suppl 1):3–7. doi:10.1159/000092704
91. Frei RB, Luschnig P, Parzmair GP, Peinhaupt M, Schranz S, Fauland A, et al. Cannabinoid receptor 2 augments eosinophil responsiveness and aggravates allergen-induced pulmonary inflammation in mice. Allergy (2016) 71:944–56. doi:10.1111/all.12858
92. Larose MC, Chakir J, Archambault AS, Joubert P, Provost V, Laviolette M, et al. Correlation between CCL26 production by human bronchial epithelial cells and airway eosinophils: involvement in patients with severe eosinophilic asthma. J Allergy Clin Immunol (2015) 136:904–13. doi:10.1016/j.jaci.2015.02.039
93. Alam R, York J, Boyars M, Stafford S, Grant JA, Lee J, et al. Increased MCP-1, RANTES, and MIP-1alpha in bronchoalveolar lavage fluid of allergic asthmatic patients. Am J Respir Crit Care Med (1996) 153:1398–404. doi:10.1164/ajrccm.153.4.8616572
94. Powell N, Humbert M, Durham SR, Assoufi B, Kay AB, Corrigan CJ. Increased expression of mRNA encoding RANTES and MCP-3 in the bronchial mucosa in atopic asthma. Eur Respir J (1996) 9:2454–60. doi:10.1183/09031936.96.09122454
95. Humbert M, Ying S, Corrigan C, Menz G, Barkans J, Pfister R, et al. Bronchial mucosal expression of the genes encoding chemokines RANTES and MCP-3 in symptomatic atopic and nonatopic asthmatics: relationship to the eosinophil-active cytokines interleukin (IL)-5, granulocyte macrophage-colony-stimulating factor, and IL-3. Am J Respir Cell Mol Biol (1997) 16:1–8. doi:10.1165/ajrcmb.16.1.8998072
96. Lamkhioued B, Renzi PM, Abi-Younes S, Garcia-Zepada EA, Allakhverdi Z, Ghaffar O, et al. Increased expression of eotaxin in bronchoalveolar lavage and airways of asthmatics contributes to the chemotaxis of eosinophils to the site of inflammation. J Immunol (1997) 159:4593–601.
97. Mattoli S, Stacey MA, Sun G, Bellini A, Marini M. Eotaxin expression and eosinophilic inflammation in asthma. Biochem Biophys Res Commun (1997) 236:299–301. doi:10.1006/bbrc.1997.6958
98. Nakamura H, Weiss ST, Israel E, Luster AD, Drazen JM, Lilly CM. Eotaxin and impaired lung function in asthma. Am J Respir Crit Care Med (1999) 160:1952–6. doi:10.1164/ajrccm.160.6.9811089
99. Taha RA, Minshall EM, Miotto D, Shimbara A, Luster A, Hogg JC, et al. Eotaxin and monocyte chemotactic protein-4 mRNA expression in small airways of asthmatic and nonasthmatic individuals. J Allergy Clin Immunol (1999) 103:476–83. doi:10.1016/S0091-6749(99)70474-4
100. Ying S, Meng Q, Zeibecoglou K, Robinson DS, Macfarlane A, Humbert M, et al. Eosinophil chemotactic chemokines (eotaxin, eotaxin-2, RANTES, monocyte chemoattractant protein-3 (MCP-3), and MCP-4), and C-C chemokine receptor 3 expression in bronchial biopsies from atopic and nonatopic (Intrinsic) asthmatics. J Immunol (1999) 163:6321–9.
101. Jahnz-Ro YK, Plusa T, Mierzejewska J. Eotaxin in serum of patients with asthma or chronic obstructive pulmonary disease: relationship with eosinophil cationic protein and lung function. Mediators Inflamm (2000) 9:175–9. doi:10.1080/09629350020008691
102. Yamada H, Yamaguchi M, Yamamoto K, Nakajima T, Hirai K, Morita Y, et al. Eotaxin in induced sputum of asthmatics: relationship with eosinophils and eosinophil cationic protein in sputum. Allergy (2000) 55:392–7. doi:10.1034/j.1398-9995.2000.00474.x
103. Berkman N, Ohnona S, Chung FK, Breuer R. Eotaxin-3 but not eotaxin gene expression is upregulated in asthmatics 24 hours after allergen challenge. Am J Respir Cell Mol Biol (2001) 24:682–7. doi:10.1165/ajrcmb.24.6.4301
104. Lilly CM, Nakamura H, Belostotsky OI, Haley KJ, Garcia-Zepeda EA, Luster AD, et al. Eotaxin expression after segmental allergen challenge in subjects with atopic asthma. Am J Respir Crit Care Med (2001) 163:1669–75. doi:10.1164/ajrccm.163.7.9812044
105. Taha RA, Laberge S, Hamid Q, Olivenstein R. Increased expression of the chemoattractant cytokines eotaxin, monocyte chemotactic protein-4, and interleukin-16 in induced sputum in asthmatic patients. Chest (2001) 120:595–601. doi:10.1378/chest.120.2.595
106. Komiya A, Nagase H, Yamada H, Sekiya T, Yamaguchi M, Sano Y, et al. Concerted expression of eotaxin-1, eotaxin-2, and eotaxin-3 in human bronchial epithelial cells. Cell Immunol (2003) 225:91–100. doi:10.1016/j.cellimm.2003.10.001
107. Feltis BN, Reid DW, Ward C, Walters EH. BAL eotaxin and IL-5 in asthma, and the effects of inhaled corticosteroid and beta2 agonist. Respirology (2004) 9:507–13. doi:10.1111/j.1440-1843.2004.00624.x
108. Hadjicharalambous C, Dent G, May RD, Handy RL, Anderson IK, Davies DE, et al. Measurement of eotaxin (CCL11) in induced sputum supernatants: validation and detection in asthma. J Allergy Clin Immunol (2004) 113:657–62. doi:10.1016/j.jaci.2004.01.757
109. Azazi EA, Bakir SM, Mohtady HA, Almonem AA. Circulating chemokine eotaxin and chemokine receptor CCR3 in allergic patients. Egypt J Immunol (2007) 14:73–82.
110. Scheicher ME, Teixeira MM, Cunha FQ, Teixeira AL Jr, Filho JT, Vianna EO. Eotaxin-2 in sputum cell culture to evaluate asthma inflammation. Eur Respir J (2007) 29:489–95. doi:10.1183/09031936.00060205
111. Xu J, Jiang F, Nayeri F, Zetterstrom O. Apoptotic eosinophils in sputum from asthmatic patients correlate negatively with levels of IL-5 and eotaxin. Respir Med (2007) 101:1447–54. doi:10.1016/j.rmed.2007.01.026
112. Kim HB, Kim CK, Iijima K, Kobayashi T, Kita H. Protein microarray analysis in patients with asthma: elevation of the chemokine PARC/CCL18 in sputum. Chest (2009) 135:295–302. doi:10.1378/chest.08-0962
113. Kim CK, Choi J, Callaway Z, Iijima K, Volcheck G, Kita H. Increases in airway eosinophilia and a th1 cytokine during the chronic asymptomatic phase of asthma. Respir Med (2010) 104:1436–43. doi:10.1016/j.rmed.2010.03.023
114. Saad-El-Din BS, Abo El-Magd GH, Mabrouk MM. Serum chemokines RANTES and monocyte chemoattractant protein-1 in Egyptian patients with atopic asthma: relationship to disease severity. Arch Med Res (2012) 43:36–41. doi:10.1016/j.arcmed.2012.01.009
115. Hosoki K, Ying S, Corrigan C, Qi H, Kurosky A, Jennings K, et al. Analysis of a panel of 48 cytokines in BAL fluids specifically identifies IL-8 levels as the only cytokine that distinguishes controlled asthma from uncontrolled asthma, and correlates inversely with FEV1. PLoS One (2015) 10:e0126035. doi:10.1371/journal.pone.0126035
116. Konno S, Gonokami Y, Kurokawa M, Kawazu K, Asano K, Okamoto K, et al. Cytokine concentrations in sputum of asthmatic patients. Int Arch Allergy Immunol (1996) 109:73–8. doi:10.1159/000237234
117. Romagnoli M, Vachier I, Tarodo DLF, Meziane H, Chavis C, Bousquet J, et al. Eosinophilic inflammation in sputum of poorly controlled asthmatics. Eur Respir J (2002) 20:1370–7. doi:10.1183/09031936.02.00029202
118. Lilly CM, Woodruff PG, Camargo CA Jr, Nakamura H, Drazen JM, Nadel ES, et al. Elevated plasma eotaxin levels in patients with acute asthma. J Allergy Clin Immunol (1999) 104:786–90. doi:10.1016/S0091-6749(99)70288-5
119. Park SW, Kim DJ, Chang HS, Park SJ, Lee YM, Park JS, et al. Association of interleukin-5 and eotaxin with acute exacerbation of asthma. Int Arch Allergy Immunol (2003) 131:283–90. doi:10.1159/000072140
120. Daldegan MB, Teixeira MM, Talvani A. Concentration of CCL11, CXCL8 and TNF-alpha in sputum and plasma of patients undergoing asthma or chronic obstructive pulmonary disease exacerbation. Braz J Med Biol Res (2005) 38:1359–65. doi:10.1590/S0100-879X2005000900010
121. Coleman JM, Naik C, Holguin F, Ray A, Ray P, Trudeau JB, et al. Epithelial eotaxin-2 and eotaxin-3 expression: relation to asthma severity, luminal eosinophilia and age at onset. Thorax (2012) 67:1061–6. doi:10.1136/thoraxjnl-2012-201634
122. Teran LM, Noso N, Carroll M, Davies DE, Holgate S, Schroder JM. Eosinophil recruitment following allergen challenge is associated with the release of the chemokine RANTES into asthmatic airways. J Immunol (1996) 157:1806–12.
123. Sur S, Kita H, Gleich GJ, Chenier TC, Hunt LW. Eosinophil recruitment is associated with IL-5, but not with RANTES, twenty-four hours after allergen challenge. J Allergy Clin Immunol (1996) 97:1272–8. doi:10.1016/S0091-6749(96)70195-1
124. Brown JR, Kleimberg J, Marini M, Sun G, Bellini A, Mattoli S. Kinetics of eotaxin expression and its relationship to eosinophil accumulation and activation in bronchial biopsies and bronchoalveolar lavage (BAL) of asthmatic patients after allergen inhalation. Clin Exp Immunol (1998) 114:137–46. doi:10.1046/j.1365-2249.1998.00688.x
125. Zeibecoglou K, Macfarlane AJ, Ying S, Meng Q, Pavord I, Barnes NC, et al. Increases in eotaxin-positive cells in induced sputum from atopic asthmatic subjects after inhalational allergen challenge. Allergy (1999) 54:730–5. doi:10.1034/j.1398-9995.1999.00058.x
126. Ravensberg AJ, Ricciardolo FL, Van SA, Rabe KF, Sterk PJ, Hiemstra PS, et al. Eotaxin-2 and eotaxin-3 expression is associated with persistent eosinophilic bronchial inflammation in patients with asthma after allergen challenge. J Allergy Clin Immunol (2005) 115:779–85. doi:10.1016/j.jaci.2004.11.045
127. Ferland C, Guilbert M, Davoine F, Flamand N, Chakir J, Laviolette M. Eotaxin promotes eosinophil transmigration via the activation of the plasminogen-plasmin system. J Leukoc Biol (2001) 69:772–8.
128. Berkman N, Krishnan VL, Gilbey T, Newton R, O’Connor B, Barnes PJ, et al. Expression of RANTES mRNA and protein in airways of patients with mild asthma. Am J Respir Crit Care Med (1996) 154:1804–11. doi:10.1164/ajrccm.154.6.8970374
129. Fahy JV, Figueroa DJ, Wong HH, Liu JT, Abrams JS. Similar RANTES levels in healthy and asthmatic airways by immunoassay and in situ hybridization. Am J Respir Crit Care Med (1997) 155:1095–100. doi:10.1164/ajrccm.155.3.9116993
130. Lamkhioued B, Garcia-Zepeda EA, Abi-Younes S, Nakamura H, Jedrzkiewicz S, Wagner L, et al. Monocyte chemoattractant protein (MCP)-4 expression in the airways of patients with asthma. Induction in epithelial cells and mononuclear cells by proinflammatory cytokines. Am J Respir Crit Care Med (2000) 162:723–32. doi:10.1164/ajrccm.162.2.9901080
131. Kalayci O, Sonna LA, Woodruff PG, Camargo CA Jr, Luster AD, Lilly CM. Monocyte chemotactic protein-4 (MCP-4; CCL-13): a biomarker of asthma. J Asthma (2004) 41:27–33. doi:10.1081/JAS-120024590
132. Dajani R, Al-Haj Ali E, Dajani B. Macrophage colony stimulating factor and monocyte chemoattractant protein 2 are elevated in intrinsic asthmatics. Cytokine (2011) 56:641–7. doi:10.1016/j.cyto.2011.08.040
133. Hoshino M, Aoike N, Takahashi M, Nakamura Y, Nakagawa T. Increased immunoreactivity of stromal cell-derived factor-1 and angiogenesis in asthma. Eur Respir J (2003) 21:804–9. doi:10.1183/09031936.03.00082002
134. Negrete-Garcia MC, Velazquez JR, Popoca-Coyotl A, Montes-Vizuet AR, Juarez-Carvajal E, Teran LM. Chemokine (C-X-C motif) ligand 12/stromal cell-derived factor-1 is associated with leukocyte recruitment in asthma. Chest (2010) 138:100–6. doi:10.1378/chest.09-2104
135. Nakamura Y, Hoshino M, Sim JJ, Ishii K, Hosaka K, Sakamoto T. Effect of the leukotriene receptor antagonist pranlukast on cellular infiltration in the bronchial mucosa of patients with asthma. Thorax (1998) 53:835–41. doi:10.1136/thx.53.10.835
136. Reiss TF, Chervinsky P, Dockhorn RJ, Shingo S, Seidenberg B, Edwards TB. Montelukast, a once-daily leukotriene receptor antagonist, in the treatment of chronic asthma: a multicenter, randomized, double-blind trial. Montelukast clinical research study group. Arch Intern Med (1998) 158:1213–20. doi:10.1001/archinte.158.11.1213
137. Diamant Z, Grootendorst DC, Veselic-Charvat M, Timmers MC, De SM, Leff JA, et al. The effect of montelukast (MK-0476), a cysteinyl leukotriene receptor antagonist, on allergen-induced airway responses and sputum cell counts in asthma. Clin Exp Allergy (1999) 29:42–51. doi:10.1046/j.1365-2222.1999.00447.x
138. Pizzichini E, Leff JA, Reiss TF, Hendeles L, Boulet LP, Wei LX, et al. Montelukast reduces airway eosinophilic inflammation in asthma: a randomized, controlled trial. Eur Respir J (1999) 14:12–8. doi:10.1034/j.1399-3003.1999.14a04.x
139. Yoshida S, Ishizaki Y, Shoji T, Onuma K, Nakagawa H, Nakabayashi M, et al. Effect of pranlukast on bronchial inflammation in patients with asthma. Clin Exp Allergy (2000) 30:1008–14. doi:10.1046/j.1365-2222.2000.00834.x
140. Yamauchi K, Tanifuji Y, Pan LH, Yoshida T, Sakurai S, Goto S, et al. Effects of pranlukast, a leukotriene receptor antagonist, on airway inflammation in mild asthmatics. J Asthma (2001) 38:51–7. doi:10.1081/JAS-100000021
141. Minoguchi K, Kohno Y, Minoguchi H, Kihara N, Sano Y, Yasuhara H, et al. Reduction of eosinophilic inflammation in the airways of patients with asthma using montelukast. Chest (2002) 121:732–8. doi:10.1378/chest.121.3.732
142. Horiguchi T, Tachikawa S, Kondo R, Miyazaki J, Shiga M, Hirose M, et al. Comparative evaluation of the leukotriene receptor antagonist pranlukast versus the steroid inhalant fluticasone in the therapy of aged patients with mild bronchial asthma. Arzneimittelforschung (2007) 57:87–91. doi:10.1055/s-0031-1296588
143. Tamaoki J, Isono K, Taira M, Tagaya E, Nakata J, Kawatani K, et al. Role of regular treatment with inhaled corticosteroid or leukotriene receptor antagonist in mild intermittent asthma. Allergy Asthma Proc (2008) 29:189–96. doi:10.2500/aap.2008.29.3100
144. Ramsay CF, Sullivan P, Gizycki M, Wang D, Swern AS, Barnes NC, et al. Montelukast and bronchial inflammation in asthma: a randomised, double-blind placebo-controlled trial. Respir Med (2009) 103:995–1003. doi:10.1016/j.rmed.2009.01.019
145. Shindo K, Matsumoto Y, Hirai Y, Sumitomo M, Amano T, Miyakawa K, et al. Measurement of leukotriene B4 in arterial blood of asthmatic patients during wheezing attacks. J Intern Med (1990) 228:91–6. doi:10.1111/j.1365-2796.1990.tb00200.x
146. Sampson AP, Castling DP, Green CP, Price JF. Persistent increase in plasma and urinary leukotrienes after acute asthma. Arch Dis Child (1995) 73:221–5. doi:10.1136/adc.73.3.221
147. Kazani S, Planaguma A, Ono E, Bonini M, Zahid M, Marigowda G, et al. Exhaled breath condensate eicosanoid levels associate with asthma and its severity. J Allergy Clin Immunol (2013) 132:547–53. doi:10.1016/j.jaci.2013.01.058
148. Evans DJ, Barnes PJ, Spaethe SM, Van Alstyne EL, Mitchell MI, O’Connor BJ. Effect of a leukotriene B4 receptor antagonist, LY293111, on allergen induced responses in asthma. Thorax (1996) 51:1178–84. doi:10.1136/thx.51.12.1178
149. Wenzel SE, Westcott JY, Larsen GL. Bronchoalveolar lavage fluid mediator levels 5 minutes after allergen challenge in atopic subjects with asthma: relationship to the development of late asthmatic responses. J Allergy Clin Immunol (1991) 87:540–8. doi:10.1016/0091-6749(91)90013-E
150. Pavord ID, Ward R, Woltmann G, Wardlaw AJ, Sheller JR, Dworski R. Induced sputum eicosanoid concentrations in asthma. Am J Respir Crit Care Med (1999) 160:1905–9. doi:10.1164/ajrccm.160.6.9903114
151. Balzar S, Fajt ML, Comhair SA, Erzurum SC, Bleecker E, Busse WW, et al. Mast cell phenotype, location, and activation in severe asthma. Data from the severe asthma research program. Am J Respir Crit Care Med (2011) 183:299–309. doi:10.1164/rccm.201002-0295OC
152. Fajt ML, Gelhaus SL, Freeman B, Uvalle CE, Trudeau JB, Holguin F, et al. Prostaglandin D(2) pathway upregulation: relation to asthma severity, control, and TH2 inflammation. J Allergy Clin Immunol (2013) 131:1504–12. doi:10.1016/j.jaci.2013.01.035
153. Liu MC, Hubbard WC, Proud D, Stealey BA, Galli SJ, Kagey-Sobotka A, et al. Immediate and late inflammatory responses to ragweed antigen challenge of the peripheral airways in allergic asthmatics. Cellular, mediator, and permeability changes. Am Rev Respir Dis (1991) 144:51–8. doi:10.1164/ajrccm/144.1.51
154. Nowak D, Grimminger F, Jorres R, Oldigs M, Rabe KF, Seeger W, et al. Increased LTB4 metabolites and PGD2 in BAL fluid after methacholine challenge in asthmatic subjects. Eur Respir J (1993) 6:405–12.
155. Barnes N, Pavord I, Chuchalin A, Bell J, Hunter M, Lewis T, et al. A randomized, double-blind, placebo-controlled study of the CRTH2 antagonist OC000459 in moderate persistent asthma. Clin Exp Allergy (2012) 42:38–48. doi:10.1111/j.1365-2222.2011.03813.x
156. Pettipher R, Hunter MG, Perkins CM, Collins LP, Lewis T, Baillet M, et al. Heightened response of eosinophilic asthmatic patients to the CRTH2 antagonist OC000459. Allergy (2014) 69:1223–32. doi:10.1111/all.12451
157. Krug N, Tschernig T, Erpenbeck VJ, Hohlfeld JM, Kohl J. Complement factors C3a and C5a are increased in bronchoalveolar lavage fluid after segmental allergen provocation in subjects with asthma. Am J Respir Crit Care Med (2001) 164:1841–3. doi:10.1164/ajrccm.164.10.2010096
158. Marc MM, Korosec P, Kosnik M, Kern I, Flezar M, Suskovic S, et al. Complement factors c3a, c4a, and c5a in chronic obstructive pulmonary disease and asthma. Am J Respir Cell Mol Biol (2004) 31:216–9. doi:10.1165/rcmb.2003-0394OC
159. Hasegawa K, Tamari M, Shao C, Shimizu M, Takahashi N, Mao XQ, et al. Variations in the C3, C3a receptor, and C5 genes affect susceptibility to bronchial asthma. Hum Genet (2004) 115:295–301. doi:10.1007/s00439-004-1157-z
160. Dent G, Hadjicharalambous C, Yoshikawa T, Handy RL, Powell J, Anderson IK, et al. Contribution of eotaxin-1 to eosinophil chemotactic activity of moderate and severe asthmatic sputum. Am J Respir Crit Care Med (2004) 169:1110–7. doi:10.1164/rccm.200306-855OC
161. Tateno H, Nakamura H, Minematsu N, Nakajima T, Takahashi S, Nakamura M, et al. Plasma eotaxin level and severity of asthma treated with corticosteroid. Respir Med (2004) 98:782–90. doi:10.1016/j.rmed.2004.01.005
162. Aggarwal S, Moodley YP, Thompson PJ, Misso NL. Prostaglandin E2 and cysteinyl leukotriene concentrations in sputum: association with asthma severity and eosinophilic inflammation. Clin Exp Allergy (2010) 40:85–93. doi:10.1111/j.1365-2222.2009.03386.x
163. Samitas K, Chorianopoulos D, Vittorakis S, Zervas E, Economidou E, Papatheodorou G, et al. Exhaled cysteinyl-leukotrienes and 8-isoprostane in patients with asthma and their relation to clinical severity. Respir Med (2009) 103:750–6. doi:10.1016/j.rmed.2008.11.009
164. Philip G, Villaran C, Shah SR, Vandormael K, Smugar SS, Reiss TF. The efficacy and tolerability of inhaled montelukast plus inhaled mometasone compared with mometasone alone in patients with chronic asthma. J Asthma (2011) 48:495–502. doi:10.3109/02770903.2011.573042
165. Jayaram L, Duong M, Pizzichini MM, Pizzichini E, Kamada D, Efthimiadis A, et al. Failure of montelukast to reduce sputum eosinophilia in high-dose corticosteroid-dependent asthma. Eur Respir J (2005) 25:41–6. doi:10.1183/09031936.04.00008104
166. Green RH, Brightling CE, Mckenna S, Hargadon B, Neale N, Parker D, et al. Comparison of asthma treatment given in addition to inhaled corticosteroids on airway inflammation and responsiveness. Eur Respir J (2006) 27:1144–51. doi:10.1183/09031936.06.00102605
167. Barnes N, Laviolette M, Allen D, Flood-Page P, Hargreave F, Corris P, et al. Effects of montelukast compared to double dose budesonide on airway inflammation and asthma control. Respir Med (2007) 101:1652–8. doi:10.1016/j.rmed.2007.03.007
168. Fregonese L, Swan FJ, Van Schadewijk A, Dolhnikoff M, Santos MA, Daha MR, et al. Expression of the anaphylatoxin receptors C3aR and C5aR is increased in fatal asthma. J Allergy Clin Immunol (2005) 115:1148–54. doi:10.1016/j.jaci.2005.01.068
169. Kita H. Eosinophils: multifaceted biological properties and roles in health and disease. Immunol Rev (2011) 242:161–77. doi:10.1111/j.1600-065X.2011.01026.x
170. Lee JJ, Jacobsen EA, Ochkur SI, Mcgarry MP, Condjella RM, Doyle AD, et al. Human versus mouse eosinophils: “that which we call an eosinophil, by any other name would stain as red”. J Allergy Clin Immunol (2012) 130:572–84. doi:10.1016/j.jaci.2012.07.025
171. Powell WS, Rokach J. The eosinophil chemoattractant 5-oxo-ETE and the OXE receptor. Prog Lipid Res (2013) 52:651–65. doi:10.1016/j.plipres.2013.09.001
172. Borchers MT, Ansay T, Desalle R, Daugherty BL, Shen H, Metzger M, et al. In vitro assessment of chemokine receptor-ligand interactions mediating mouse eosinophil migration. J Leukoc Biol (2002) 71:1033–41.
173. Heller NM, Gwinn WM, Donnelly RP, Constant SL, Keegan AD. IL-4 engagement of the type I IL-4 receptor complex enhances mouse eosinophil migration to eotaxin-1 in vitro. PLoS One (2012) 7:e39673. doi:10.1371/journal.pone.0039673
174. Campbell EM, Proudfoot AE, Yoshimura T, Allet B, Wells TN, White AM, et al. Recombinant guinea pig and human RANTES activate macrophages but not eosinophils in the guinea pig. J Immunol (1997) 159:1482–9.
175. Nabe T, Yamamura H, Kohno S. Eosinophil chemotaxis induced by several biologically active substances and the effects of apafant on it in vitro. Arzneimittelforschung (1997) 47:1112–6.
176. Teixeira MM, Williams TJ, Hellewell PG. Description of an in vivo model for the assessment of eosinophil chemoattractants in the mouse. Mem Inst Oswaldo Cruz (1997) 92(Suppl 2):211–4. doi:10.1590/S0074-02761997000800029
177. Ponath PD, Qin S, Ringler DJ, Clark-Lewis I, Wang J, Kassam N, et al. Cloning of the human eosinophil chemoattractant, eotaxin. Expression, receptor binding, and functional properties suggest a mechanism for the selective recruitment of eosinophils. J Clin Invest (1996) 97:604–12. doi:10.1172/JCI118456
178. Uguccioni M, Mackay CR, Ochensberger B, Loetscher P, Rhis S, Larosa GJ, et al. High expression of the chemokine receptor CCR3 in human blood basophils. Role in activation by eotaxin, MCP-4, and other chemokines. J Clin Invest (1997) 100:1137–43. doi:10.1172/JCI119624
179. White JR, Imburgia C, Dul E, Appelbaum E, O’Donnell K, O’Shannessy DJ, et al. Cloning and functional characterization of a novel human CC chemokine that binds to the CCR3 receptor and activates human eosinophils. J Leukoc Biol (1997) 62:667–75.
180. Oliveira SH, Lira S, Martinez A, Wiekowski M, Sullivan L, Lukacs NW. Increased responsiveness of murine eosinophils to MIP-1beta (CCL4) and TCA-3 (CCL1) is mediated by their specific receptors, CCR5 and CCR8. J Leukoc Biol (2002) 71:1019–25.
181. Rothenberg ME, Luster AD, Leder P. Murine eotaxin: an eosinophil chemoattractant inducible in endothelial cells and in interleukin 4-induced tumor suppression. Proc Natl Acad Sci U S A (1995) 92:8960–4. doi:10.1073/pnas.92.19.8960
182. Patel VP, Kreider BL, Li Y, Li H, Leung K, Salcedo T, et al. Molecular and functional characterization of two novel human C-C chemokines as inhibitors of two distinct classes of myeloid progenitors. J Exp Med (1997) 185:1163–72. doi:10.1084/jem.185.7.1163
183. Daugherty BL, Siciliano SJ, Demartino JA, Malkowitz L, Sirotina A, Springer MS. Cloning, expression, and characterization of the human eosinophil eotaxin receptor. J Exp Med (1996) 183:2349–54. doi:10.1084/jem.183.5.2349
184. Chou CC, Fine JS, Pugliese-Sivo C, Gonsiorek W, Davies L, Deno G, et al. Pharmacological characterization of the chemokine receptor, hCCR1 in a stable transfectant and differentiated HL-60 cells: antagonism of hCCR1 activation by MIP-1beta. Br J Pharmacol (2002) 137:663–75. doi:10.1038/sj.bjp.0704907
185. Agrawal DK, Ali N, Numao T. PAF receptors and G-proteins in human blood eosinophils and neutrophils. J Lipid Mediat (1992) 5:101–4.
186. Korth RM. Specific high affinity binding of platelet activating factor to intact human blood neutrophils and eosinophils. Int Arch Allergy Immunol (1996) 110:124–31. doi:10.1159/000237276
187. Ukena D, Krogel C, Dent G, Yukawa T, Sybrecht G, Barnes PJ. PAF-receptors on eosinophils: identification with a novel ligand, [3H]WEB 2086. Biochem Pharmacol (1989) 38:1702–5. doi:10.1016/0006-2952(89)90322-5
188. Oppermann M, Raedt U, Hebell T, Schmidt B, Zimmermann B, Gotze O. Probing the human receptor for C5a anaphylatoxin with site-directed antibodies. Identification of a potential ligand binding site on the NH2-terminal domain. J Immunol (1993) 151:3785–94.
189. Elsner J, Oppermann M, Kapp A. Detection of C5a receptors on human eosinophils and inhibition of eosinophil effector functions by anti-C5a receptor (CD88) antibodies. Eur J Immunol (1996) 26:1560–4. doi:10.1002/eji.1830260723
190. Czech W, Dichmann S, Herouy Y, Rheinen H, Elsner J, Kapp A, et al. Distinct amplification of the C5a-receptor pathways in normodense and hypodense eosinophils of patients with atopic dermatitis. Scand J Immunol (2001) 53:235–9. doi:10.1046/j.1365-3083.2001.00860.x
191. Baelder R, Fuchs B, Bautsch W, Zwirner J, Kohl J, Hoymann HG, et al. Pharmacological targeting of anaphylatoxin receptors during the effector phase of allergic asthma suppresses airway hyperresponsiveness and airway inflammation. J Immunol (2005) 174:783–9. doi:10.4049/jimmunol.174.2.783
192. Karsten CM, Laumonnier Y, Eurich B, Ender F, Broker K, Roy S, et al. Monitoring and cell-specific deletion of C5aR1 using a novel floxed GFP-C5aR1 reporter knock-in mouse. J Immunol (2015) 194:1841–55. doi:10.4049/jimmunol.1401401
193. Ogawa H, Kunkel SL, Fantone JC, Ward PA. Comparative study of eosinophil and neutrophil chemotaxis and enzyme release. Am J Pathol (1981) 105:149–55.
194. Chouinard F, Lefebvre JS, Navarro P, Bouchard L, Ferland C, Lalancette-Hebert M, et al. The endocannabinoid 2-arachidonoyl-glycerol activates human neutrophils: critical role of its hydrolysis and de novo leukotriene B4 biosynthesis. J Immunol (2011) 186:3188–96. doi:10.4049/jimmunol.1002853
195. Hosoi T, Koguchi Y, Sugikawa E, Chikada A, Ogawa K, Tsuda N, et al. Identification of a novel human eicosanoid receptor coupled to G(i/o). J Biol Chem (2002) 277:31459–65. doi:10.1074/jbc.M203194200
196. Jones CE, Holden S, Tenaillon L, Bhatia U, Seuwen K, Tranter P, et al. Expression and characterization of a 5-oxo-6E,8Z,11Z,14Z-eicosatetraenoic acid receptor highly expressed on human eosinophils and neutrophils. Mol Pharmacol (2003) 63:471–7. doi:10.1124/mol.63.3.471
197. Huang WW, Garcia-Zepeda EA, Sauty A, Oettgen HC, Rothenberg ME, Luster AD. Molecular and biological characterization of the murine leukotriene B4 receptor expressed on eosinophils. J Exp Med (1998) 188:1063–74. doi:10.1084/jem.188.6.1063
198. Kamohara M, Takasaki J, Matsumoto M, Saito T, Ohishi T, Ishii H, et al. Molecular cloning and characterization of another leukotriene B4 receptor. J Biol Chem (2000) 275:27000–4. doi:10.1074/jbc.C000382200
199. Patnode ML, Bando JK, Krummel MF, Locksley RM, Rosen SD. Leukotriene B4 amplifies eosinophil accumulation in response to nematodes. J Exp Med (2014) 211:1281–8. doi:10.1084/jem.20132336
200. Matsuoka T, Hirata M, Tanaka H, Takahashi Y, Murata T, Kabashima K, et al. Prostaglandin D2 as a mediator of allergic asthma. Science (2000) 287:2013–7. doi:10.1126/science.287.5460.2013
201. Hirai H, Abe H, Tanaka K, Takatsu K, Sugamura K, Nakamura M, et al. Gene structure and functional properties of mouse CRTH2, a prostaglandin D2 receptor. Biochem Biophys Res Commun (2003) 307:797–802. doi:10.1016/S0006-291X(03)01266-X
202. Spik I, Brenuchon C, Angeli V, Staumont D, Fleury S, Capron M, et al. Activation of the prostaglandin D2 receptor DP2/CRTH2 increases allergic inflammation in mouse. J Immunol (2005) 174:3703–8. doi:10.4049/jimmunol.174.6.3703
203. Royer JF, Schratl P, Lorenz S, Kostenis E, Ulven T, Schuligoi R, et al. A novel antagonist of CRTH2 blocks eosinophil release from bone marrow, chemotaxis and respiratory burst. Allergy (2007) 62:1401–9. doi:10.1111/j.1398-9995.2007.01452.x
204. Devosse T, Guillabert A, D’Haene N, Berton A, De NP, Noel S, et al. Formyl peptide receptor-like 2 is expressed and functional in plasmacytoid dendritic cells, tissue-specific macrophage subpopulations, and eosinophils. J Immunol (2009) 182:4974–84. doi:10.4049/jimmunol.0803128
205. Svensson L, Redvall E, Johnsson M, Stenfeldt AL, Dahlgren C, Wenneras C. Interplay between signaling via the formyl peptide receptor (FPR) and chemokine receptor 3 (CCR3) in human eosinophils. J Leukoc Biol (2009) 86:327–36. doi:10.1189/jlb.0908514
206. Prevete N, Rossi FW, Rivellese F, Lamacchia D, Pelosi C, Lobasso A, et al. Helicobacter pylori HP(2-20) induces eosinophil activation and accumulation in superficial gastric mucosa and stimulates VEGF-alpha and TGF-beta release by interacting with formyl-peptide receptors. Int J Immunopathol Pharmacol (2013) 26:647–62. doi:10.1177/039463201302600308
207. Sun FF, Crittenden NJ, Czuk CI, Taylor BM, Stout BK, Johnson HG. Biochemical and functional differences between eosinophils from animal species and man. J Leukoc Biol (1991) 50:140–50.
208. Lukacs NW, Standiford TJ, Chensue SW, Kunkel RG, Strieter RM, Kunkel SL. C-C chemokine-induced eosinophil chemotaxis during allergic airway inflammation. J Leukoc Biol (1996) 60:573–8.
209. Dunzendorfer S, Kaneider NC, Kaser A, Woell E, Frade JM, Mellado M, et al. Functional expression of chemokine receptor 2 by normal human eosinophils. J Allergy Clin Immunol (2001) 108:581–7. doi:10.1067/mai.2001.118518
210. Liu LY, Jarjour NN, Busse WW, Kelly EA. Chemokine receptor expression on human eosinophils from peripheral blood and bronchoalveolar lavage fluid after segmental antigen challenge. J Allergy Clin Immunol (2003) 112:556–62. doi:10.1016/S0091-6749(03)01798-6
211. Thivierge M, Doty M, Johnson J, Stankova J, Rola-Pleszczynski M. IL-5 up-regulates cysteinyl leukotriene 1 receptor expression in HL-60 cells differentiated into eosinophils. J Immunol (2000) 165:5221–6. doi:10.4049/jimmunol.165.9.5221
212. Fujii M, Tanaka H, Abe S. Interferon-gamma up-regulates expression of cysteinyl leukotriene type 2 receptors on eosinophils in asthmatic patients. Chest (2005) 128:3148–55. doi:10.1378/chest.128.5.3148
213. Zhang YJ, Zhang L, Wang SB, Shen HH, Wei EQ. Montelukast modulates lung CysLT(1) receptor expression and eosinophilic inflammation in asthmatic mice. Acta Pharmacol Sin (2004) 25:1341–6.
214. Moro K, Yamada T, Tanabe M, Takeuchi T, Ikawa T, Kawamoto H, et al. Innate production of T(H)2 cytokines by adipose tissue-associated c-Kit(+)Sca-1(+) lymphoid cells. Nature (2010) 463:540–4. doi:10.1038/nature08636
215. Neill DR, Wong SH, Bellosi A, Flynn RJ, Daly M, Langford TK, et al. Nuocytes represent a new innate effector leukocyte that mediates type-2 immunity. Nature (2010) 464:1367–70. doi:10.1038/nature08900
216. Price AE, Liang HE, Sullivan BM, Reinhardt RL, Eisley CJ, Erle DJ, et al. Systemically dispersed innate IL-13-expressing cells in type 2 immunity. Proc Natl Acad Sci U S A (2010) 107:11489–94. doi:10.1073/pnas.1003988107
217. Spits H, Artis D, Colonna M, Diefenbach A, Di Santo JP, Eberl G, et al. Innate lymphoid cells – a proposal for uniform nomenclature. Nat Rev Immunol (2013) 13:145–9. doi:10.1038/nri3365
218. Xue L, Salimi M, Panse I, Mjosberg JM, Mckenzie AN, Spits H, et al. Prostaglandin D2 activates group 2 innate lymphoid cells through chemoattractant receptor-homologous molecule expressed on TH2 cells. J Allergy Clin Immunol (2014) 133:1184–94. doi:10.1016/j.jaci.2013.10.056
219. Chen R, Smith SG, Salter B, El-Gammal A, Oliveria JP, Obminski C, et al. Allergen-induced increases in sputum levels of group 2 innate lymphoid cells in asthmatic subjects. Am J Respir Crit Care Med (2017). doi:10.1164/rccm.201612-2427OC
220. Kabata H, Moro K, Koyasu S, Asano K. Group 2 innate lymphoid cells and asthma. Allergol Int (2015) 64:227–34. doi:10.1016/j.alit.2015.03.004
221. Karta MR, Broide DH, Doherty TA. Insights into group 2 innate lymphoid cells in human airway disease. Curr Allergy Asthma Rep (2016) 16:8. doi:10.1007/s11882-015-0581-6
222. Salimi M, Barlow JL, Saunders SP, Xue L, Gutowska-Owsiak D, Wang X, et al. A role for IL-25 and IL-33-driven type-2 innate lymphoid cells in atopic dermatitis. J Exp Med (2013) 210:2939–50. doi:10.1084/jem.20130351
223. Salimi M, Stoger L, Liu W, Go S, Pavord I, Klenerman P, et al. Cysteinyl leukotriene E4 activates human ILC2s and enhances the effect of prostaglandin D2 and epithelial cytokines. J Allergy Clin Immunol (2017). doi:10.1016/j.jaci.2016.12.958
224. Chang JE, Doherty TA, Baum R, Broide D. Prostaglandin D2 regulates human type 2 innate lymphoid cell chemotaxis. J Allergy Clin Immunol (2014) 133:899–901.e893. doi:10.1016/j.jaci.2013.09.020
225. Wojno ED, Monticelli LA, Tran SV, Alenghat T, Osborne LC, Thome JJ, et al. The prostaglandin D(2) receptor CRTH2 regulates accumulation of group 2 innate lymphoid cells in the inflamed lung. Mucosal Immunol (2015) 8:1313–23. doi:10.1038/mi.2015.21
226. Doherty TA, Khorram N, Lund S, Mehta AK, Croft M, Broide DH. Lung type 2 innate lymphoid cells express cysteinyl leukotriene receptor 1, which regulates TH2 cytokine production. J Allergy Clin Immunol (2013) 132:205–13. doi:10.1016/j.jaci.2013.03.048
227. von Moltke J, O’Leary CE, Barrett NA, Kanaoka Y, Austen KF, Locksley RM. Leukotrienes provide an NFAT-dependent signal that synergizes with IL-33 to activate ILC2s. J Exp Med (2017) 214:27–37. doi:10.1084/jem.20161274
228. Denney L, Byrne AJ, Shea TJ, Buckley JS, Pease JE, Herledan GM, et al. Pulmonary epithelial cell-derived cytokine TGF-beta1 is a critical cofactor for enhanced innate lymphoid cell function. Immunity (2015) 43:945–58. doi:10.1016/j.immuni.2015.10.012
229. Neighbour H, Boulet LP, Lemiere C, Sehmi R, Leigh R, Sousa AR, et al. Safety and efficacy of an oral CCR3 antagonist in patients with asthma and eosinophilic bronchitis: a randomized, placebo-controlled clinical trial. Clin Exp Allergy (2014) 44:508–16. doi:10.1111/cea.12244
Keywords: eosinophil, group 2 innate lymphoid cells, 2-arachidonoyl-glycerol, chemokine, eotaxin, asthma
Citation: Larose M-C, Archambault A-S, Provost V, Laviolette M and Flamand N (2017) Regulation of Eosinophil and Group 2 Innate Lymphoid Cell Trafficking in Asthma. Front. Med. 4:136. doi: 10.3389/fmed.2017.00136
Received: 08 May 2017; Accepted: 27 July 2017;
Published: 11 August 2017
Edited by:
Mats W. Johansson, University of Wisconsin-Madison, United StatesReviewed by:
James Edward Pease, Imperial College London, United KingdomTing Wen, Cincinnati Children’s Hospital Medical Center, United States
Copyright: © 2017 Larose, Archambault, Provost, Laviolette and Flamand. This is an open-access article distributed under the terms of the Creative Commons Attribution License (CC BY). The use, distribution or reproduction in other forums is permitted, provided the original author(s) or licensor are credited and that the original publication in this journal is cited, in accordance with accepted academic practice. No use, distribution or reproduction is permitted which does not comply with these terms.
*Correspondence: Nicolas Flamand, bmljb2xhcy5mbGFtYW5kQGNyaXVjcHEudWxhdmFsLmNh