- Department of Anaesthesiology and Intensive Therapy, University of Szeged, Szeged, Hungary
Purpose of review: The aim of this article is to study the overview of pathophysiology and clinical application of central venous oxygen saturation monitoring in critically ill patients and during the perioperative period.
Recent findings: There are several clinical studies and animal experiments evaluating the effects of goal-directed hemodynamic stabilization on critically ill patients. Recent systematic reviews and meta-analyses found that advanced hemodynamic endpoints-targeted management has a positive effect on outcome in high-risk surgical patients. As all interventions aim to improve tissue oxygenation, it is of utmost importance to monitor the balance between oxygen delivery and consumption. For this purpose, central venous blood gas analysis provides an easily available tool in the everyday clinical practice. The adequate interpretation of central venous oxygen saturation renders the need of careful evaluation of several physiological and pathophysiological circumstances. When appropriately evaluated, central venous oxygen saturation can be a valuable component of a multimodal individualized approach, in which components of oxygen delivery are put in the context of the patients’ individual oxygen consumption. In addition to guide therapy, central venous oxygen saturation may also serve as an early warning sign of inadequate oxygen delivery, which would otherwise remain hidden from the attending physician.
Summary: With the incorporation of central venous oxygen saturation in the everyday clinical routine, treatment could be better tailored for the patients’ actual needs; hence, it may also improve outcome.
Introduction
Interventions to improve oxygen delivery and decrease oxygen consumption are the cornerstone of resuscitation in the critically ill patients and during the perioperative period of high-risk patients. Early recognition of the patients at risks and the implementation of adequate monitoring-guided interventions can have a profound effect on outcome. On the contrary, delaying adequate interventions will inevitably lead to hypoperfusion, tissue hypoxia, and multiple-organ failure affecting both outcome and wasting of resources and costs (1). Therefore, the use of appropriate indices, which are able to detect the imbalance between oxygen delivery (DO2) and consumption (VO2), is mandatory for adequate management (2). Conventional parameters such as heart rate, mean arterial blood pressure, mental status, and urine output are robust warning signs of inadequate tissue perfusion, but for fine tuning of therapy detailed hemodynamic monitoring is warranted (3). The recent FENICE (Fluid Challenges In Intensive Care) trial indicate that there is a considerable gap between the accumulating knowledge about the benefits of advanced hemodynamic monitoring based optimization and the actual clinical practice. In more than 2,000 patients, fluid challenges were evaluated. The main indicator of administering fluid boluses was hypotension in 57%, and in 43% of cases, no hemodynamic variable was used to predict fluid responsiveness (4). Detailed assessment of global hemodynamic indices such as cardiac output (CO) and derived variables and also the measures of oxygen delivery and uptake should be taken into account to provide appropriate therapy for these patients (5, 6). Furthermore, in addition to the optimization of global hemodynamic parameters, indicators of tissue perfusion should also be monitored to verify the effectiveness of our interventions (7). To monitor changes in tissue oxygenation, central or mixed venous blood gas measurements can give more detailed information, which should be incorporated into a multimodal approach that can lead to a better, individualized, patient-centered care. The goal of this review is to highlight the importance of central venous oxygen saturation in this multimodal, individualized hemodynamic management in the context of the pathophysiological background and the results of recent clinical and experimental studies.
Physiological Issues
Tissue oxygenation is the net product of oxygen delivery and oxygen consumption, which can be described by the following formulae (8):
If SaO2 is taken as 1, as under normal circumstances, the hemoglobin is almost fully saturated with oxygen, and the other hemodynamic variables are kept constant, then:
DO2, oxygen delivery; CO, cardiac output; Hb, hemoglobin; SaO2, arterial oxygen saturation; PaO2, partial pressure of oxygen in the arterial blood; CaO2, arterial oxygen content; VO2, oxygen consumption; ScvO2, central venous oxygen saturation; CcvO2, central venous oxygen content; O2ER, oxygen extraction; PcvO2, central venous partial pressure of oxygen.
Taking a 75-kg healthy adult man when resting, the relationship between DO2 and VO2 can be estimated as:
Oxygen delivery:
Oxygen consumption:
Oxygen extraction:
The main difference between the equations of DO2 and VO2 is the oxygen content (CaO2 versus CcvO2), especially the central venous oxygen saturation (ScvO2). Therefore, it can be useful to assess the imbalance between DO2 and VO2 in the critically ill.
When the arterial oxygen content (CaO2) and/or CO becomes impaired, DO2 decreases, which is often accompanied by a parallel decrease in VO2. The most frequently occurring scenarios are represented in Figure 1. In the early phase of decreasing DO2, the circulation can compensate to some extent, and VO2 remains stable. However, beyond a critical point, any further drop in DO2 will result in a decrease in VO2. From this point, VO2 becomes dependent on DO2, and aerobic metabolism will have to be switched to anaerobic metabolism, leading to low ScvO2, hyperlactatemia, metabolic acidosis, and oxygen debt (9).
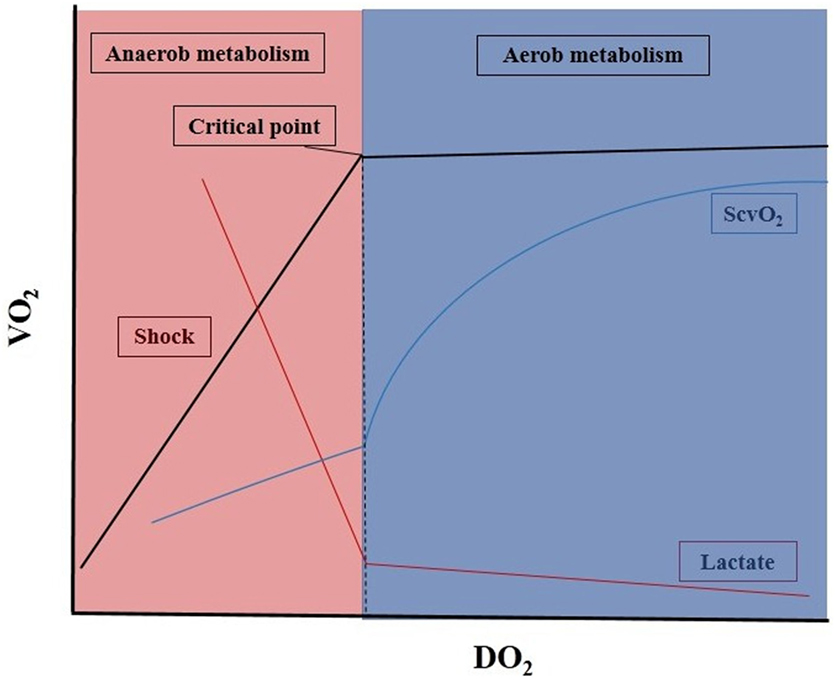
Figure 1. Oxygen delivery and consumption in critically ill patients. DO2, oxygen delivery; VO2, oxygen consumption; ScvO2, central venous oxygen saturation ratio. For details, see main text.
The principle task of early resuscitation is to regain balance by optimizing the VO2/DO2 ratio. However, it is also important to define the endpoints of resuscitation to avoid overresuscitation. In the case of fluid resuscitation, for example, unnecessary administration of fluids will lead to hypervolemia, which increases morbidity and mortality to a similar extent to that of hypovolemia (10, 11). Unjustified blood transfusions also carry the risk of hypervolemia and transmission of infections (12) or allergic reactions (13). There is evidence that prolonged use of catecholamines is associated with poor outcome (14). Therefore, it is important to recognize the point when tissue perfusion has been normalized, oxygen debt has been resolved, and resuscitation has been terminated.
Individualized Goal-Directed Hemodynamic Therapy
The multimodal concept in hemodynamic monitoring enables us to appreciate that each patient is different, hence the so-called normal values, which are more or less appropriate for a given population may be inadequate for the given patient. Therefore, this concept can be translated into the individualized or personalized use of target endpoints to avoid underresuscitation or overresuscitation.
Parameters for Assessment of Tissue Metabolism
Mixed Venous and Central Venous Oxygen Saturation
Mixed venous oxygen saturation (SvO2) measured in the pulmonary artery via a pulmonary artery catheter, and its surrogate, central venous oxygen saturation (ScvO2) measured in the superior vena cava are the most commonly used parameters to assess global oxygen extraction (VO2/DO2). As central venous catheters are frequently applied in most critically ill patients, ScvO2 is more readily available compared to SvO2. Although the absolute values of ScvO2 are 5% higher than SvO2 on average, but changes usually occur in a parallel manner (15), therefore ScvO2 is regarded as a surrogate marker in the clinical setting (16, 17).
The main factors, which influence ScvO2, are hemoglobin, arterial oxygen saturation of hemoglobin, CO, and oxygen consumption. There are multiple physiologic, pathophysiologic, and therapeutic factors that influence venous oxygen saturation such as anemia, hypovolemia, contractility, bleeding, sedation, fever, and pain (18).
ScvO2 in Intensive Care Patients
During sepsis, organ dysfunction is most likely the result of inadequate tissue perfusion causing cellular hypoxia. Interventions improving the balance between DO2 and VO2 may prevent the development of tissue hypoperfusion, organ dysfunction syndrome, and thus improve the outcome of septic patients. In patients with early phase of severe sepsis, septic shock, early goal-directed intervention guided by continuous monitoring of ScvO2, central venous pressure and mean arterial pressure (MAP), with target values of CVP 8 to 12 mmHg, MAP > 65 mmHg and ScvO2 > 70%, reduced mortality from 46.5 to 30.5% at the 28th day (19).
Although this study has been criticized for several reasons and these results could never be repeated, there is international consensus that that low ScvO2 values are very important warning signs of inadequate DO2 and can prognosticate complications and poor outcome. However, recent data suggest that high ScvO2 values may also have adverse outcomes in septic patients (20). Due to deranged microcirculation when shunting is present on the level of capillaries, impaired oxygen utilization can lead to normal or supraphysiological ScvO2 values, which represent an inability of the cells to extract oxygen in sepsis (21). In patients with ScvO2 > 70% complementary blood gas parameters, such as elevated venous-to-arterial CO2 gap (dCO2) (>6 mmHg), increased or persistently elevated serum lactate levels could help the clinicians to identify tissue hypoxia. In a retrospective analysis, septic patients with physiological ScvO2 and abnormal dCO2 mortality was significantly higher as compared to patients with normal dCO2 values (22).
In patients treated on intensive care units, heart failure is often present resulting impaired CO, hence decreased oxygen delivery (23), and resulting oxygen extraction imbalance that could be detected by low ScvO2 (24). In a clinical study after myocardial infarction in patients with heart failure and cardiogenic shock, SvO2 was 43%, while in patients with heart failure without shock, it was 56% compared to patients without heart failure with an SvO2 of 70% (25). It may also be useful in patients with cardiogenic shock requiring the support by intraaortic balloon counter pulsation. In a study during weaning period, intraaortic balloon pump assist ratio was decreased from 1:1 to 1:3. In the weaning failure group, decreased support was accompanied by a drop in ScvO2, while it remained constant in the successful group (26). In patients with chronic heart failure, ScvO2 can be chronically low. However, during acute decompensation, major cardiac events were observed in 81% of patients with ScvO2 ≤ 60% at 24 h after ICU admission, while it was only 13% in patients with higher ScvO2 (27).
ScvO2 and Blood Transfusion
In addition to heart failure, anemia is another frequent cause of impaired DO2 in critically ill patients, and almost 40–45% of patients will receive blood transfusion during the treatment period (28). As large multicenter trials (TRICC and TRISS) suggest that patients with hemoglobin levels above 10 mg/dl usually do not require transfusion, while red blood cell administration is usually beneficial if the hemoglobin level is below 7 mg/dl (29, 30). Between these values, physicians have to make decisions according to clinical signs like mental status, tachycardia, tachypnea, blood pressure, and diuresis. To be able to give additional objective data about oxygen debt of organs, ScvO2 may offer an easily obtainable tool to detect a low hemoglobin-related altered O2ER and hence may serve as a physiological trigger for blood transfusion (30). In human studies, both on volunteers and retrospective data in critically ill patients suggest that lower levels of hemoglobin compared to that of recommended by international guidelines were well tolerated and did not produce hemodynamic instability, and when oxygen imbalance occurred, it was accompanied by a significant drop in SvO2 (30–32). In our recent animal experiment on isovolemic anemia, we have found that anemia-induced change in VO2/DO2 showed significant correlation with changes of ScvO2 (33); hence, ScvO2 may be used as a “physiologic transfusion trigger” in otherwise hemodynamically stable patients.
ScvO2 and High-Risk Surgery
High-risk surgical patients are at an increased risk of developing imbalance between VO2 and DO2 in the perioperative period; therefore, monitoring ScvO2 may have a rationale during both the intraoperative and postoperative managements.
It has been shown that patients with low ScvO2 values preoperatively, intraoperatively, or postoperatively are at an increased risk for complications and poor prognosis (34). Therefore, it seems to be logical to maintain ScvO2 in normal range during the perioperative care. We reported in a small, single-center prospective randomized study about continuously measured ScvO2-assisted intraoperative hemodynamic optimization (CeVOX Maquet® Munich Germany) during major abdominal surgery. In the conventional group, patients were treated according to mean arterial and central venous pressure, while in the ScvO2 group, additionally venous oxygen saturation was also measured via fiberoptic catheter placed in the superior vena cava. ScvO2 monitorization resulted in more interventions, more fluid boluses and more blood transfusion compared to the conventional group. These intervention resulted in better organ functions, less complication rate, and better 28 days of survival (35). These results are in accord with the results of an earlier single-center study, where ScvO2 over 73% directed group had fewer postoperative complications and had shorter length of hospital stay compared to patients in whom hemodynamic stabilization was guided according to MAP and central venous pressure (36). However, it is important to considerate that in anesthetized, mechanically ventilated patients, “physiological” values of ScvO2 are 5–10% higher (i.e., 75–80%) because of the decreased oxygen extraction of the brain. Second, when bleeding is present and blood loss is replaced by crystalloids, considerable hemodilution can take place. In our experimental stroke volume-guided hemorrhage and fluid resuscitation animal model, ScvO2 normalized at the end of resuscitation, but returned to a significantly lower level (with a mean of 5%) as the hemodilution caused significant drop in hemoglobin levels (37). In a clinical study performed on patients with esophagectomy, ScvO2 could indicate decreased DO2 caused by low hemoglobin levels; therefore, the authors suggest to use ScvO2 as complementary transfusion trigger to hemoglobin in the perioperative period (32).
High-risk patient with major surgery benefits most from goal-directed therapy with significant reduction in mortality and morbidity compared to patients with low-risk interventions (38). ScvO2 is an important element of this complex perioperative multimodal monitoring-based concept, including advanced hemodynamic monitoring and assessment of VO2/DO2, what we call the individualized, multimodal approach (39).
Complementary Blood Gas Parameters
Venous-to-Arterial CO2 Gap (dCO2)
Mixed-, or central venous-to-arterial carbon dioxide gap is an easily attainable parameter when patients has arterial and central venous lines in situ. The physiological value is ≤6 mmHg, and this holds true for both mixed- (Pv-aCO2) and central venous-to-arterial (Pcv-aCO2) CO2 gap values. Therefore, the central venous Pcv-aCO2-gap can be useful surrogate of Pv-aCO2 in the everyday practice.
Increased CO2 gap of >40 mmHg was described 30 years ago during cardiac arrest in patients who were monitored with pulmonary artery catheters and also in an animal experiment on cardiopulmonary resuscitation (40). After these landmark studies, increased dCO2 was detected in several low-flow states (41–43). During anaerobic metabolism, increased production of hydrogen ions are buffered by bicarbonate presented in the cells, and this process will generate CO2 production (44). When the Fick principle is applied for carbon dioxide, there is an inverse relationship between the CO and dCO2 (45); in other words, increased levels of dCO2 should reflect low-flow states. Indeed, it has been shown that in sepsis, heart failure, and severe hypovolemia, its value can be elevated (46, 47).
In the perioperative setting, dCO2 also has a strong predictive value. Patients with high dCO2 had significantly higher mortality compared to patients with normal values (36.4 versus 4.5%) (48). High-risk surgical patients admitted to intensive care unit postoperatively with high dCO2 also developed more complications. The cutoff value was 5.8 mmHg (49), and in a different clinical study, a dCO2 > 5 mmHg had 96% sensitivity to predict the occurrence of postoperative complications in patients with physiological (≥71%) ScvO2 (50). In critically ill patients, the dCO2 shows good inverse correlation with the CO (42), and it has also been shown to be a good predictor for bad outcome in patients with septic shock (41). In cases like septic shock, when due to microcirculatory or mitochondrial defects oxygen uptake is insufficient, ScvO2 can be supranormal. Previous studies have suggested that under such circumstances the increased value of dCO2 (>5 mmHg) and increased lactate level can help the physician in detecting inadequate flow to the tissues; hence, the complementary use of ScvO2 and dCO2 is recommended (50, 51).
Conclusion
Early and adequate interventions to improve hemodynamics, oxygen delivery, and reducing oxygen needs have a significant effect on outcome. Protocolized care with predefined values of certain physiological indices, such as blood pressure, CO, may benefit the majority of the population, but these values may be inadequate for the rest; hence, they will remain either underresuscitated or overresuscitated. Therefore, individualizing treatment should be desirable. For this purpose, additional physiological parameters like central venous oxygen saturation, lactate, and venous-to-arterial CO2 gap should be assessed together with other hemodynamic variables to get a detailed picture about the hemodynamic status of our patients. Putting the pieces of the puzzle together in context is what we define as multimodal, individualized hemodynamic support, in which ScvO2 has a pivotal role.
Author Contributions
All authors listed have made a substantial, direct and intellectual contribution to the work, and approved it for publication.
Conflict of Interest Statement
ZM receives regular honoraria for being in the Medical Advisory Board of PULSION Maquet, for lectures from Biotest, ThermoFisher Scientific, and CytoSorbents. The remaining author declares that the research was conducted in the absence of any commercial or financial relationships that could be construed as a potential conflict of interest.
References
1. Shoemaker WC, Appel PL, Kram HB. Role of oxygen debt in the development of organ failure sepsis, and death in high-risk surgical patients. Chest (1992) 102:208–15. doi:10.1378/chest.102.1.208
2. Shoemaker WC, Appel PL, Kram HB. Tissue oxygen debt as determinant of lethal and nonlethal postoperative organ failure. Crit Care Med (1988) 16:1117–20. doi:10.1097/00003246-198811000-00007
3. Goodrich C. Endpoints of resuscitation: what should we be monitoring? AACN Adv Crit Care (2006) 17:306–16. doi:10.4037/15597768-2006-3008
4. Cecconi M, Hofer C, Teboul JL, Pettila V, Wilkman E, Molnar Z, et al. Fluid challenges in intensive care: the FENICE study: a global inception cohort study. Intensive Care Med (2015) 41:1529–37. doi:10.1007/s00134-015-3850-x
5. Donati A, Pelaia P, Pietropaoli P, Preiser JC. Do use ScvO2 and O2ER as therapeutical goals. Minerva Anestesiol (2011) 7:483–4.
6. Marik PE, Desai H. Goal directed fluid therapy. Curr Pharm Des (2012) 18:6215–24. doi:10.2174/138161212803832399
7. Benes J, Pradl R, Chyrta I. Perioperative hemodynamic optimization: a way to individual goals. In: Vincent JL, editor. Annual Update in Intensive Care and Emergency Medicine 2012. New York: Springer (2012). p. 357–67.
8. Vallet B, Tavernier B, Lund N. Assessment of tissue oxygenation in the critically ill. Eur J Anaesthesiol (2000) 17:221–9. doi:10.1097/00003643-200004000-00003
9. Vincent JL. The relationship between oxygen demand, oxygen uptake, and oxygen supply. Intensive Care Med (1990) 16:145–8. doi:10.1007/BF01785244
10. Holte K, Sharrock NE, Kehlet H. Pathophysiology and clinical implications of perioperative fluid excess. Br J Anaesth (2002) 89:622–32. doi:10.1093/bja/aef220
11. Acheampong A, Vincent JL. A positive fluid balance is an independent prognostic factor in patients with sepsis. Crit Care (2015) 19:251. doi:10.1186/s13054-015-0970-1
12. Rohde JM, Dimcheff DE, Blumberg N, Saint S, Langa KM, Kuhn L, et al. Health care-associated infection after red blood cell transfusion: a systematic review and meta-analysis. JAMA (2014) 311:1317–26. doi:10.1001/jama.2014.2726
13. Yeh SP, Chang CW, Chen JC, Yeh WC, Chen PC, Chuang SJ, et al. A well-designed online transfusion reaction reporting system improves the estimation of transfusion reaction incidence and quality of care in transfusion practice. Am J Clin Pathol (2011) 136:842–7. doi:10.1309/AJCPOQNBKCDXFWU3
14. Kastrup M, Braun J, Kaffarnik M, von Dossow-Hanfstingl V, Ahlborn R, Wernecke KD, et al. Catecholamine dosing and survival in adult intensive care unit patients. World J Surg (2013) 37:766–73. doi:10.1007/s00268-013-1926-8
15. Rivers E. Mixed versus central venous oxygen saturation may be not numerically equal, but both are still clinically useful. Chest (2006) 129:507–8. doi:10.1378/chest.129.3.507
16. Chawla LS, Zia H, Gutierrez G, Katz NM, Seneff MG, Shah M. Lack of equivalence between central and mixed venous oxygen saturation. Chest (2004) 126:1891–6. doi:10.1378/chest.126.6.1891
17. Reinhart K, Kuhn HJ, Hartog C, Bredle DL. Continuous central venous and pulmonary artery oxygen saturation monitoring in the critically ill. Intensive Care Med (2004) 30:1572–8. doi:10.1007/s00134-004-2337-y
18. van Beest P, Wietasch G, Scheeren T, Spronk P, Kuiper M. Clinical review: use of venous oxygen saturations as a goal – a yet unfinished puzzle. Crit Care (2011) 15:232. doi:10.1186/cc10351
19. Rivers E, Nguyen B, Havstad S, Ressler J, Muzzin A, Knoblich B, et al. Early goal-directed therapy in the treatment of severe sepsis and septic shock. N Engl J Med (2001) 345:1368–77. doi:10.1056/NEJMoa010307
20. Pope JV, Jones AE, Gaieski DF, Arnold RC, Trzeciak S, Shapiro NI, et al. Multicenter study of central venous oxygen saturation (ScvO2) as a predictor of mortality in patients with sepsis. Ann Emerg Med (2010) 55:40–6. doi:10.1016/j.annemergmed.2009.08.014
21. Ince C, Sinaasappel M. Microcirculatory oxygenation and shunting in sepsis and shock. Crit Care Med (1999) 27:1369–77. doi:10.1097/00003246-199907000-00031
22. Du W, Liu DW, Wang XT, Long Y, Chai WZ, Zhou X, et al. Combining central venous-to-arterial partial pressure of carbon dioxide difference and central venous oxygen saturation to guide resuscitation in septic shock. J Crit Care (2013) 28:1110. doi:10.1016/j.jcrc.2013.07.049
23. Dar O, Cowie MR. Acute heart failure in the intensive care unit: epidemiology. Crit Care Med (2008) 36:S3–8. doi:10.1097/01.CCM.0000296264.41365.80
24. Muir AL, Kirby BJ, King AJ, Miller HC. Mixed venous oxygen saturation in relation to cardiac output in myocardial infarction. Br Med J (1970) 4:276–8. doi:10.1136/bmj.4.5730.276
25. Goldman RH, Braniff B, Harrison DC, Spivack AP. The use of central venous oxygen sturation measurements in a coronary care unit. Ann Intern Med (1968) 68:1280–7. doi:10.7326/0003-4819-68-6-1280
26. Hsin HT, Chen LY, Lin PC, Shieh JS, Ao CV. Central venous oxygen saturation (ScVO2) facilitates the weaning of intra-aortic balloon pump in acute heart failure related to acute myocardial infarction. Int J Cardiol (2013) 168:4568–70. doi:10.1016/j.ijcard.2013.06.077
27. Gallet R, Lellouche N, Mitchell-Heggs L, Bouhemad B, Bensaid A, Dubois-Randé JL, et al. Prognosis value of central venous oxygen saturation in acute decompensated heart failure. Arch Cardiovasc Dis (2012) 105:5–12. doi:10.1016/j.acvd.2011.10.005
28. Vincent JL, Baron JF, Reinhart K, Gattinoni L, Thijs L, Webb A, et al. Anemia and blood transfusion in critically ill patients. JAMA (2002) 288:1499–507. doi:10.1001/jama.288.12.1499
29. Hébert PC, Wells G, Blajchman MA, Marshall J, Martin C, Pagliarello G, et al. A multicenter, randomized, controlled clinical trial of transfusion requirements in critical care. Transfusion Requirements in Critical Care Investigators, Canadian Critical Care Trials Group. N Engl J Med (1999) 340:409–17. doi:10.1056/NEJM199902113400601
30. Holst LB, Haase N, Wetterslev J, Wernerman J, Guttormsen AB, Karlsson S, et al. Lower versus higher hemoglobin threshold for transfusion in septic shock. N Engl J Med (2014) 371:1381–91. doi:10.1056/NEJMoa1406617
31. Weiskopf RB, Viele MK, Feiner J, Kelley S, Lieberman J, Noorani M, et al. Human cardiovascular and metabolic response to acute, severe isovolemic anemia. JAMA (1998) 279:217–21. doi:10.1001/jama.279.3.217
32. Kobayashi M, Ko M, Irinoda T, Meguro E, Hayakawa Y, Akijama Y, et al. Clinical usefulness of continuous central venous oxygen saturation measurement for postoperative management of patients following transthoracic esophagectomy for carcinoma. Esophagus (2011) 8:53–8. doi:10.1007/s10388-011-0260-1
33. Kocsi S, Demeter G, Fogas J, Erces D, Kaszaki J, Molnar Z. Central venous oxygen saturation is a good indicator of altered oxygen balance in isovolemic anemia. Acta Anaesthesiol Scand (2012) 56:291–7. doi:10.1111/j.1399-6576.2011.02622.x
34. Collaborative Study Group on Perioperative ScvO2 Monitoring. Multicentre study on peri- and postoperative central venous oxygen saturation in high-risk surgical patients. Crit Care (2006) 10:R158. doi:10.1186/cc5018
35. Mikor A, Trasy D, Nemeth MF, Osztroluczki A, Kocsi S, Kovacs I, et al. Continuous central venous oxygen saturation assisted intraoperative hemodynamic management during major abdominal surgery: a randomized, controlled trial. BMC Anesthesiol (2015) 15:82. doi:10.1186/s12871-015-0064-2
36. Donati A, Loggi S, Preiser JC, Orsetti G, Münch C, Gabbanelli V, et al. Goal-directed intraoperative therapy reduces morbidity and length of hospital stay in high-risk surgical patients. Chest (2007) 132:1817–24. doi:10.1378/chest.07-0621
37. Nemeth M, Tanczos K, Demeter G, Erces D, Kaszaki J, Mikor A, et al. Central venous oxygen saturation and carbon dioxide gap as resuscitation targets in a hemorrhagic shock. Acta Anaesthesiol Scand (2014) 58:611–9. doi:10.1111/aas.12312
38. Cecconi M, Corredor C, Arulkumaran N, Abuella G, Ball J, Grounds RM, et al. Clinical review: goal-directed therapy-what is the evidence in surgical patients? The effect on different risk groups. Crit Care (2013) 17:209. doi:10.1186/cc11823
39. Molnar Z, Szabo Z, Nemeth M. Multimodal individualized concept of hemodynamic monitoring. Curr Opin Anaesthesiol (2017) 30:171–7. doi:10.1097/ACO.0000000000000440
40. Grundler W, Weil MH, Rackow EC. Arteriovenous carbon dioxide and pH gradients during cardiac arrest. Circulation (1986) 74:1071–4. doi:10.1161/01.CIR.74.5.1071
41. Bakker J, Vincent JL, Gris P, Leon M, Coffernils M, Kahn RJ. Veno-arterial carbon dioxide gradient in human septic shock. Chest (1992) 101:509–15. doi:10.1378/chest.101.2.509
42. Cuschieri J, Rivers EP, Donnino MW, Katilius M, Jacobsen G, Nguyen HB, et al. Central venous-arterial carbon dioxide difference as an indicator of cardiac index. Intensive Care Med (2005) 31:818–22. doi:10.1007/s00134-005-2602-8
43. Benjamin E, Paluch TA, Berger SR, Premus G, Wu C, Iberti TJ. Venous hypercarbia in canine hemorrhagic shock. Crit Care Med (1987) 15:516–8. doi:10.1097/00003246-198705000-00013
44. Vallet B, Teboul JL, Cain S, Curtis S. Venoarterial CO(2) difference during regional ischemic or hypoxic hypoxia. J Appl Physiol (2000) 89:1317–21. doi:10.1152/jappl.2000.89.4.1317
45. Lamia B, Monnet X, Teboul JL. Meaning of arterio-venous PCO2 difference in circulatory shock. Minerva Anestesiol (2006) 72:597–604.
46. Mecher CE, Rackow EC, Astiz ME, Weil MH. Venous hypercarbia associated with severe sepsis and systemic hypoperfusion. Crit Care Med (1990) 18:585–9. doi:10.1097/00003246-199006000-00001
47. Adrogué HJ, Rashad MN, Gorin AB, Yacoub J, Madias NE. Assessing acid-base status in circulatory failure. Differences between arterial and central venous blood. N Engl J Med (1989) 320:1312–6. doi:10.1056/NEJM198905183202004
48. Silva JM Jr, Oliveira AM, Segura JL, Ribeiro MH, Sposito CN, Toledo DO, et al. A large venous-arterial PCO(2) is associated with poor outcomes in surgical patients. Anesthesiol Res Pract (2011) 2011:759792. doi:10.1155/2011/759792
49. Robin E, Futier E, Pires O, Fleyfel M, Tavernier B, Lebuffe G, et al. Central venous-to-arterial carbon dioxide difference as a prognostic tool in high-risk surgical patients. Crit Care (2015) 19:227. doi:10.1186/s13054-015-0917-6
50. Futier E, Robin E, Jabaudon M, Guerin R, Petit A, Bazin JE, et al. Central venous O2 saturation and venous-to-arterial CO2 difference as complementary tools for goal-directed therapy during high-risk surgery. Crit Care (2010) 14:R193. doi:10.1186/cc9310
Keywords: venous oxygen saturation, central venous oxygen saturation, oxygen debt, hemodynamic monitoring, oxygen delivery, oxygen consumption, goal-directed therapy
Citation: Molnar Z and Nemeth M (2018) Monitoring of Tissue Oxygenation: an Everyday Clinical Challenge. Front. Med. 4:247. doi: 10.3389/fmed.2017.00247
Received: 31 October 2017; Accepted: 19 December 2017;
Published: 16 January 2018
Edited by:
Samir G. Sakka, Witten/Herdecke University, GermanyReviewed by:
Evgenia V. Fot, Northern State Medical University, RussiaInge Bauer, University Hospital Duesseldorf, Germany
Copyright: © 2018 Molnar and Nemeth. This is an open-access article distributed under the terms of the Creative Commons Attribution License (CC BY). The use, distribution or reproduction in other forums is permitted, provided the original author(s) or licensor are credited and that the original publication in this journal is cited, in accordance with accepted academic practice. No use, distribution or reproduction is permitted which does not comply with these terms.
*Correspondence: Zsolt Molnar, enNvbHRtb2xuYUBnbWFpbC5jb20=