- 1St. Boniface Research Centre, Winnipeg, MB, Canada
- 2Department of Medical Microbiology, University of Manitoba, Winnipeg, MB, Canada
- 3Deer Lodge Centre, Winnipeg, MB, Canada
- 4Cardiac Sciences Program, I.H. Asper Clinical Research Institute, Winnipeg, MB, Canada
Introduction: Type 2 diabetes (T2D) has reached epidemic proportions in North America. Recent evidence suggests that prebiotics can modulate the gut microbiome, which then plays an important role in regulating lipid metabolism, blood glucose, and insulin sensitivity. As such, prebiotics are appealing potential therapeutic strategies for prediabetes and T2D. The key objectives of this study were to determine the tolerability as well as the glucose and insulin modulating ability of MSPrebiotic® digestion resistant starch (DRS) in healthy mid-age (MID) and elderly (ELD) adults.
Materials and methods: This was a prospective, blinded, placebo-controlled study. Prediabetes and diabetes were among the exclusion factors. ELD (>70 years) and MID (30–50 years) Canadian adults were recruited and, after 2 weeks of consuming placebo, they were randomized to consume 30 g of either MSPrebiotic® or placebo per day for 12 weeks. In total, 42 ELD and 42 MID participants completed the study. Blood samples were collected over the 14-week study and analyzed for glucose, lipid profile, and CRP, lipid particles, TNF-α, IL-10, insulin, and insulin resistance (IR).
Results: At baseline, the ELD population had a significantly higher percentage (p < 0.01) with elevated glucose and significantly higher TNF-α (p < 0.01) compared to MID adults. MSPrebiotic® DRS was well tolerated in both MID and ELD adults. There was a significant difference over time in blood glucose (p = 0.0301) and insulin levels (p = 0.009), as well as IR (HOMA-IR; p = 0.009) in ELD adults who consumed MSPrebiotic® compared to placebo. No significant changes were found in MID adults.
Conclusion: Our results suggest that dietary supplementation with prebiotics such as MSPrebiotic® may be part of an effective strategy to reduce IR, a major risk factor for developing T2D, in the ELD.
Clinical Trial Registration: NCT01977183 listed on NIH website: ClinicalTrials.gov, The metadata generated in this study have been submitted to the NCBI Sequence Read Archive (http://www.ncbi.nlm.nih.gov/bioproject/381931).
Introduction
A prebiotic substance has been recently defined as “a substrate that is selectively utilized by host microorganisms conferring a health benefit.” (1). In view of the emerging link between particular gut microbiota ecosystems and the development of obesity and type 2 diabetes (T2D), prebiotics, along with probiotics, have received much attention in recent years (2–23).
Most of the published data on prebiotics relate to the effects of inulin, fructooligosaccharides (FOS), galactooligosaccharides (GOS), xylooligosaccharides (XOS), and lactulose (3, 4, 12, 24–26). These ingredients have been well tested in vitro, in animal studies, and occasionally in human studies with relatively consistent data. The characterization of these commercially available prebiotics is now reasonably well established. In contrast, recent publications (13, 27, 28) indicate that there are very few studies involving various categories of digestion resistant starch (DRS) in humans. The applicability of findings from animal studies, particularly those done in rodents, to human lipid metabolism has also been questioned (4, 13, 22). According to various authors (29–33), the pig is considered a better model than rodents, especially for studying age-related disorders in humans (31). Recent findings emanating from studies in swine suggest that DRS improves glycemic control and modifies blood lipoprotein profiles even at a relatively modest daily dose of 15 g (10.5 g of active ingredient) (33).
Reviews of recent evidence regarding the role of the gut microbiome in obesity, T2D, and metabolic disease in humans found several reports of gut dysbiosis (imbalance in the diversity or abundance of gut microbes) in patients with T2D compared to those without T2D (9, 13, 22). However, there were conflicting conclusions regarding the basis of the dysbiosis as data interpretation is confounded by different study methods, genetics, subject ethnic origins, diet, and geography (9). Furthermore, it is unclear whether the use of probiotics or prebiotics could modify this gut microbiome dysbiosis. There are substantial data to suggest that prebiotics can improve fasting blood glucose levels (6, 9, 13, 18, 34–36). However, human clinical studies often combine probiotic and prebiotic consumption making it difficult to assess the impact of prebiotics alone, and none of these published studies included elderly (ELD) adults (70 years or older).
Although many regulatory bodies around the world recognize the value of adequate dietary fiber, there are no dietary recommendations specifically for prebiotic consumption (7). Verbeke et al. (37) and Bindels et al. (12) have highlighted the value of fermentation metabolites as markers for prebiotic health benefits. However, this is difficult when there are no defined “healthy” levels of such metabolites, and current testing methods do not take into consideration the rapid absorption of such metabolites (e.g., short chain fatty acids). Although many of the health effects of probiotics and prebiotics have been linked to modulation of the gut microbiome, a limited number of clinical trials have been conducted in humans investigating the tolerability or function of DRS as a prebiotic (4, 11, 13, 15, 17, 28, 35, 38). Our recent publication focused on the microbiome changes in both mid-age (MID) and ELD adult groups after 3 months of consuming MSPrebiotic® DRS. Our results demonstrated that no changes were observed in MID or ELD groups consuming the placebo (38). However, the MID and ELD groups consuming MSPrebiotic® experienced significant increases in Bifidobacteria, and the ELD group consuming MSPrebiotic® experienced a reduction in the abundance of Proteobacteria. Both of these microbiome changes are thought to provide health benefits. However, there are still many gaps in our knowledge regarding the health benefits of DRS (13). It is particularly unclear if DRS is well tolerated in MID or ELD humans, and whether this type of prebiotic alone can influence inflammation, lipid metabolism, blood glucose levels, or insulin resistance (IR).
The primary objectives of this aspect of the clinical study were to extend the microbiome findings of Alfa et al. (38) to determine if consumption of 30 g of MSPrebiotic® DRS per day for 12 weeks was well tolerated compared to placebo and whether it could alter systemic health markers such as the lipid profile, inflammatory markers, glucose, insulin levels, or IR in MID or ELD groups.
Materials and Methods
Power Analysis and Sample Size
For the prospective study design and using the outcome measures described in the study protocol, the statistical power analysis indicated that a total sample of n = 20 in each of four groups (i.e., 20 ELD on placebo and 20 ELD on MSP as well as 20 MID on placebo and 20 MID on MSP) would have power = 0.80 to detect a Cohen’s F effect size = 0.33. This effect size was chosen based on the hypothesis that there would be moderate differences (0.50 SD) between the placebo and treatment groups for the ELD participants and that there would be small differences (0.20 SD) between the placebo and treatment groups for the MID participants. Effect sizes were estimated from published studies for other types of prebiotics using similar outcome measures (gastrointestinal tolerance, e.g., flatulence, bloating, and abdominal pain, in addition to serum glucose, lipid parameters, and inflammatory markers, such as C-reactive protein and TNF-α). A nominal α = 0.05 and two-tailed tests were used in the power calculations.
Clinical Study
This was a prospective, randomized, blinded, placebo-controlled study. Research and ethics approval was obtained from the University of Manitoba Research Ethics Board prior to implementation. This study protocol was reviewed and approved by Health Canada (Submission #188517; “Notice of Authorization” dated June 5, 2013) and was also listed on the NIH ClinicalTrials.gov website (Identifier: NCT01977183). The clinical study was carried out in accordance with the ethical standards of the University of Manitoba and Health Canada. All modifications of the protocol were reported to Health Canada and the University of Manitoba Research Ethics Board for approval prior to implementation. The allocation sequence to placebo or study product was based on computer-generated random numbers. For the institutionalized ELD, the facility study nurse contacted residents (with permission from the facility physician) to determine if they would agree to the research study nurse contacting them about this clinical trial. For all other participants, a commercial recruitment agency provided a list of participants that they had permission from to contact for clinical trials. Participants who met enrollment criteria and gave informed consent in accordance with the Declaration of Helsinki were enrolled by the study coordinator or study nurse who also sequentially assigned participants to placebo or study product based on the list of computer-generated randomized numbers. Care providers, trial participants, laboratory testing personnel, and data analysts were blinded to which arm participants were assigned. All information collected (hard copy reports and electronic databases) for the purpose of the study was kept in a locked and secured area, and participant identifiers were treated in accordance with the Personal Health Information Act of Manitoba. All information sent for statistical analyses was “de-identified” and had only a study number but no participant identifiers. The University of Manitoba Office, Research Quality Management unit performed a voluntary audit of the study in a process that was independent from the investigators and the study sponsors.
All participants in this study were recruited in Winnipeg, MB, Canada. The ELD cohort was recruited from a long-term care (LTC) facility (11 completed the study), as well as from the community (31 completed the study), and consisted of adults >70 years old. The MID participants were recruited from the community and aged 30–50 years old (42 completed the study). The details of recruitment and dropout are shown in Figure 1. All participants (or authorized third party) provided written informed consent in compliance with the University of Manitoba informed consent guidelines. Participants were informed that they could request to withdraw from the clinical study at any time without any impact on their clinical care. To reduce confounding underlying factors unrelated to consumption of DRS, the exclusion criteria included the following: pregnancy, Crohn’s disease or any other inflammatory bowel disease, individuals with systemic lupus erythematosus, on cancer chemotherapy, prediabetes or diabetes, thyroid disease, renal disease, hepatic disease, previous gastrointestinal surgery (intestinal resection, gastric bypass, colorectal surgery), individuals on probiotics (e.g., probiotic yogurt), individuals on antibiotics at the time of recruitment or on antibiotics within the previous 5 weeks, individuals experiencing dysphagia, subjects using additional fiber supplements, and individuals on digestants, emetics, anti-emetics, medications for acid peptic disease, or taking antacids.
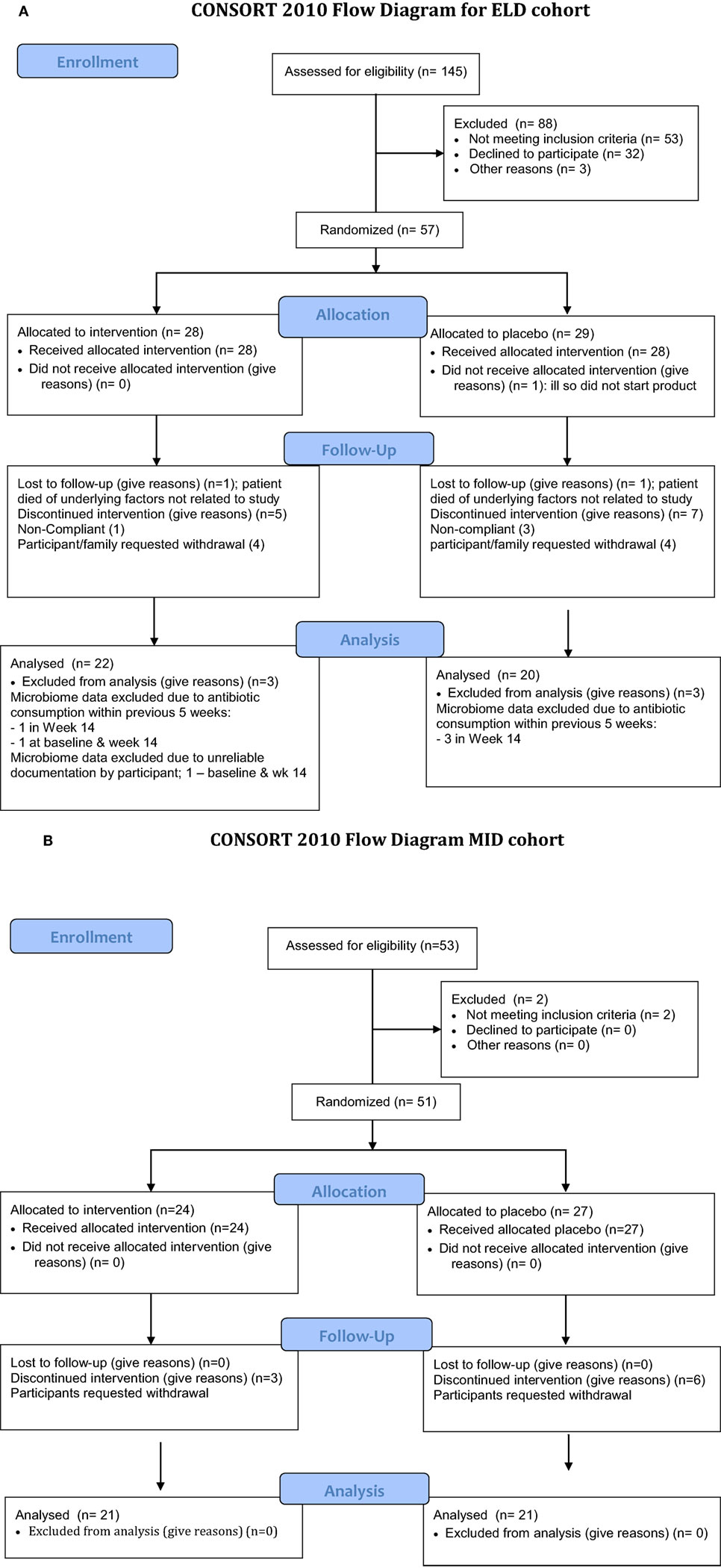
Figure 1. Flow chart of enrollment for the study. The elderly (ELD) enrollment is shown in (A) and the mid-age (MID) enrollment is shown in (B). (A) Consort 2010 flow diagram for ELD cohort. (B) Consort 2010 flow diagram MID cohort.
MSPrebiotic® (MSPrebiotics Inc., Carberry, MB, Canada) DRS was used in this study. MSPrebiotic® is to be consumed in unheated fluid or food products. This product is an unmodified natural DRS from potatoes, and the active ingredient is Solanum tuberosum extract, which is classified as a Natural Health Product on the Health Canada (39). It is composed of granules (15–100 µm in diameter) consisting of 80% amylopectin (branched glucose polymer with alpha-1,4 and alpha-1,6 linkages) and ~20% amylose (linear glucose polymer with mostly alpha-1,4 linkages). These DRS granules reach the colon relatively intact as they are not digested in the upper portions of the digestive tract. The DRS granules were analyzed using standardized validated methods by MSPrebiotic Inc. and demonstrated that MSPrebiotic® contained 70% DRS.
Amioca TF (Ingredion™, Brampton, ON, Canada), a food-grade corn starch that is readily digestible, was the placebo in this study. The same standardized validated methods were used by MSPrebiotic Inc. to show that Amioca TF does not contain any DRS. All participants consumed 30 g of placebo daily for 2 weeks and then they were randomly assigned to either MSPrebiotic® or placebo (30 g/day) for the remaining 12 weeks of the study. Participants were instructed to consume the study products at any time of the day providing it was taken 2 h before or after any other medication. In the LTC facility, MSPrebiotic® or placebo was administered following the standard administration of medication protocol (2 h before or after receiving any other medication) using documented observed consumption as well as documentation in the daily health-log forms. The general population participants also documented product consumption by completing the daily health-log forms. In addition, the amount of returned product was documented by the study coordinator at monthly visits.
Both study products were provided to participants in identical sealed foil pouches labeled only with the information identifying that the product was part of a clinical trial. There was nothing regarding the packaging or labeling that would allow participants to know which product they were getting.
Participants completed a daily health-log to track excessive flatulence, changes in bowel movements, abdominal pain, and bloating. These parameters were used to assess tolerance of consuming MSPrebiotic® versus placebo. The daily health log was also used to document consumption of the study product as well as the use of stool softeners and antibiotics. There were no changes to the normal daily diet consumed by participants other than the requirement that they did not consume probiotic-containing products.
Blood samples (fasting) were collected at enrollment (week 0), after 2 weeks of consuming placebo (participants were randomized to receive either MSPrebiotic® or placebo at this time), and then at weeks 6, 10, and 14 (total of five samples collected). Blood samples were submitted to the laboratory for analysis on the day of collection. Additional blood samples collected at the same five time points were centrifuged, the serum was dispensed into aliquots, and stored at −70°C until sent for additional testing.
Analysis Performed
Blood Analysis
Blood samples (fasting) at the five collection times indicated previously were analyzed by Diagnostic Services Manitoba, Winnipeg, MB, Canada, for C-reactive protein, glucose, and lipid profile using the standardized methods validated by the testing laboratory. In addition, aliquots of frozen serum (fasting serum) were sent to LipoScience Inc. (Raleigh, NC, USA) for lipid particle size analysis using NMR (40, 41), as well as tests to determine lipid profile, glucose level, insulin level, and IR. Samples were collected, transported, and analyzed as per the company instructions and protocols (www.labcorp.com). The frozen serum aliquots were also tested in-house for human TNF-α and IL-10 using commercial assay kits (Invitrogen, Frederick, MD, USA) as per the manufacturer’s instructions. Each frozen aliquot was thawed once for analysis and any unused portion of the aliquot was discarded.
Statistical Analysis
Baseline characteristics were compared between the MID and ELD cohorts using a Chi square test or Fisher’s exact test for categorical variables, and a Student’s t-test or Mann–Whitney test where appropriate. The difference in the change (baseline to 14 weeks) experienced in the control and MSPrebiotic® groups were compared for several systematic markers, lipid measures, glucose, and IR via a non-parametric Mann–Whitney test. In addition, a repeated measures analysis of variance was also performed as a supplementary analysis on variables that were measured at more than two time points to obtain a group, time, and group/time effect. Data collected in the weekly health log were assessed via mixed-effects models containing random slopes and intercepts. All p-values calculated were two-tailed, and a p-value less than 0.05 was considered statistically significant. All statistical analyses were performed using SAS version 9.3.
Results
Enrollment for the clinical study was started in September 2013 and completed in May 2015. Figure 1 outlines the recruitment, enrollment, and attrition of participants in the ELD and MID groups. In the ELD group, there were 31 participants from the community and 11 participants who were residents of LTC that completed the clinical trial. The data for non-institutionalized ELD participants and institutionalized ELD participants showed similar trends for all the parameters we evaluated, so the data from both ELD groups were combined for all subsequent analyses. All the MID participants enrolled were from the community (42 completed the clinical trial). The baseline (at the time of enrollment) levels of blood glucose, cholesterol parameters, and inflammatory markers of the ELD and MID groups are shown in Table 1. TNF-α levels were significantly higher (p < 0.01) in ELD versus MID at the time of enrollment, while C-reactive protein was elevated in ELD participants, but not significantly (p = 0.052). Furthermore, the percentage of participants with elevated blood glucose levels was significantly higher (p = 0.03) in the ELD group (10/42) compared to the MID group (2/42) at baseline despite all participants meeting enrollment criteria that excluded diabetics and prediabetics.
The compliance with consuming the study product was: MID-placebo; 93.5% (range: 25.0–100.0%; median: 93.5%), MID-MSPrebiotic®; 91.7% (range: 0.0–100.0%; median: 99.0%), ELD-placebo; 95.4% (range: 66.3–100.0%; median: 99.0%) and ELD-MSPrebiotic®; 97.2% (range: 80.6–100.0%; median: 99.0%). MSPrebiotic® was well tolerated in both groups, as shown in Figure 2. Levels of abdominal pain, bloating, and flatulence were all low (2 or less on a scale of 1 = none to 5 = excessive) for both groups taking either placebo or MSPrebiotic®, and there were no significant differences over the study period. The mean number of bowel movements for the MID and ELD group on placebo versus MSPrebiotic® did not change over the duration of the study, and at all times the mean number of bowel movements was less than two per day for both groups.
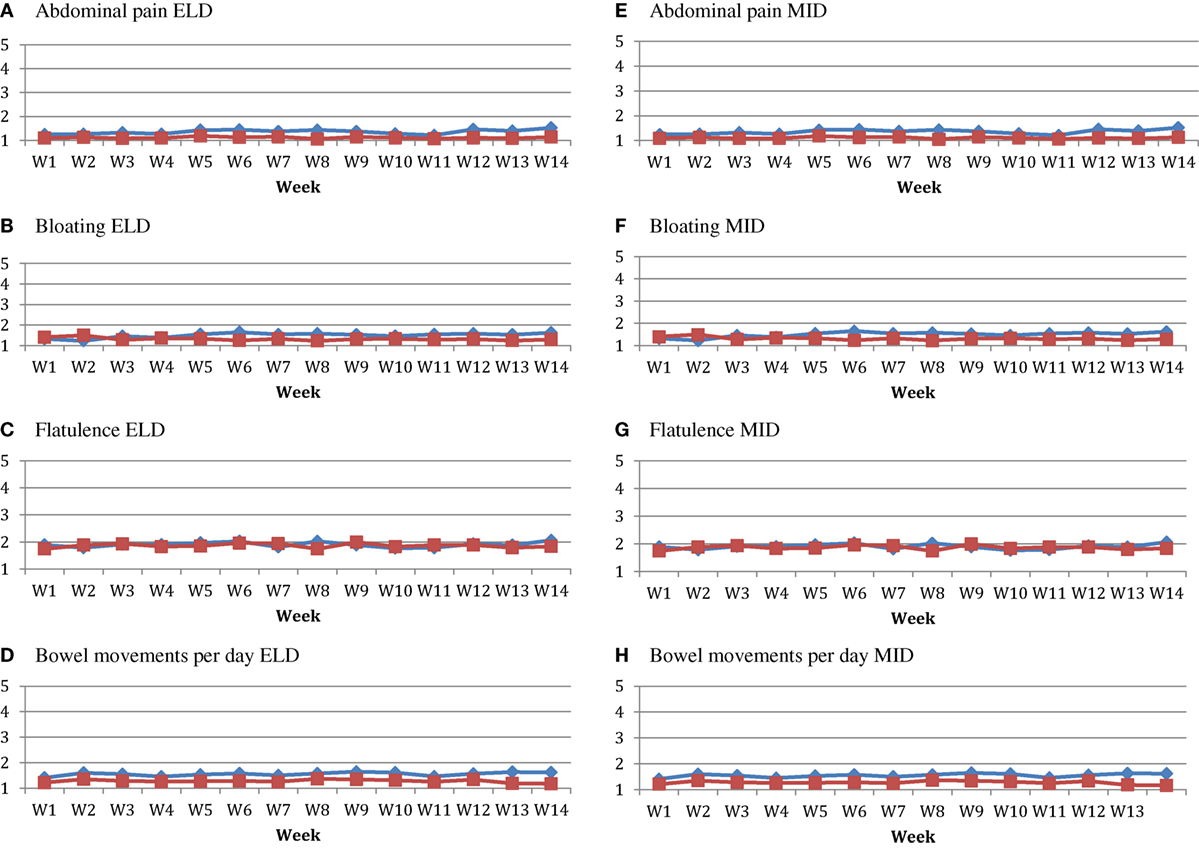
Figure 2. Tolerability of consuming MSPrebiotic® versus Placebo over time in elderly (ELD) and mid-age (MID) adults. All ELD (A–D) and MID (E–H) participants consumed 30 g placebo/day for 2 weeks and then were randomized to continue placebo or consume 30 g MSPrebiotic®/day for the remaining 12 weeks of the study. For abdominal pain, bloating and flatulence the scale was from 1 (none) to 5 (extreme). The red square symbols represent the placebo group, and the blue diamond symbols represent the MSPrebiotic® group. There was no statistically significant difference between groups for any of these parameters. (A) Abdominal pain ELD. (B) Bloating ELD. (C) Flatulence ELD. (D) Bowel movements per day ELD. (E) Abdominal pain MID. (F) Bloating MID. (G) Flatulence MID. (H) Bowel movements per day MID.
Supplementation with MSPrebiotic® or placebo had no effect on systemic cholesterol transport or IL-10 for ELD or MID groups (Table 2). Lipid profile and particle size profile were also largely unaffected after consumption of MSPrebiotic® versus placebo for ELD and MID (Tables 3 and 4, respectively). Only in the MID group consuming MSPrebiotic® was there a significant increase in large VLDL and chylomicron particles (Table 4).
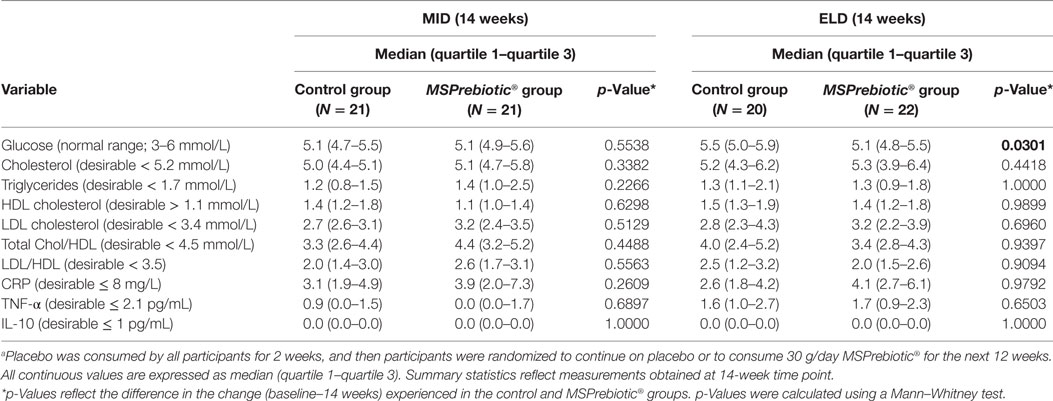
Table 2. Impact of consuming MSPrebiotic® versus placeboa on systemic markers in mid-age (MID) and elderly (ELD) adults.
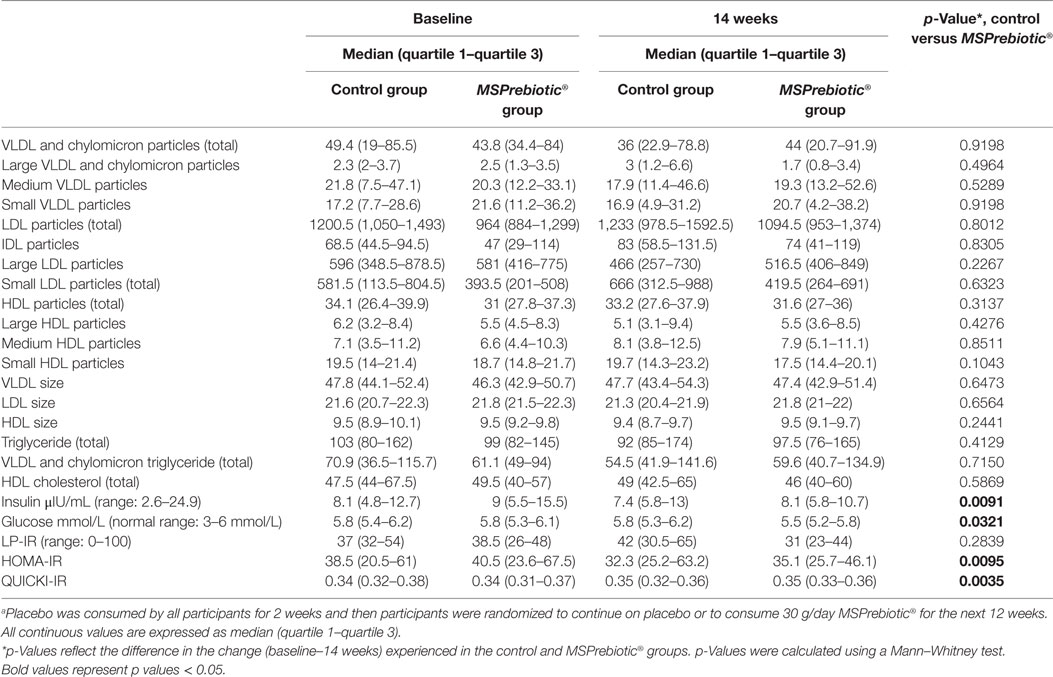
Table 3. Lipid particle and insulin resistance (IR) profiles in elderly after consumption of MSPrebiotic or placeboa.
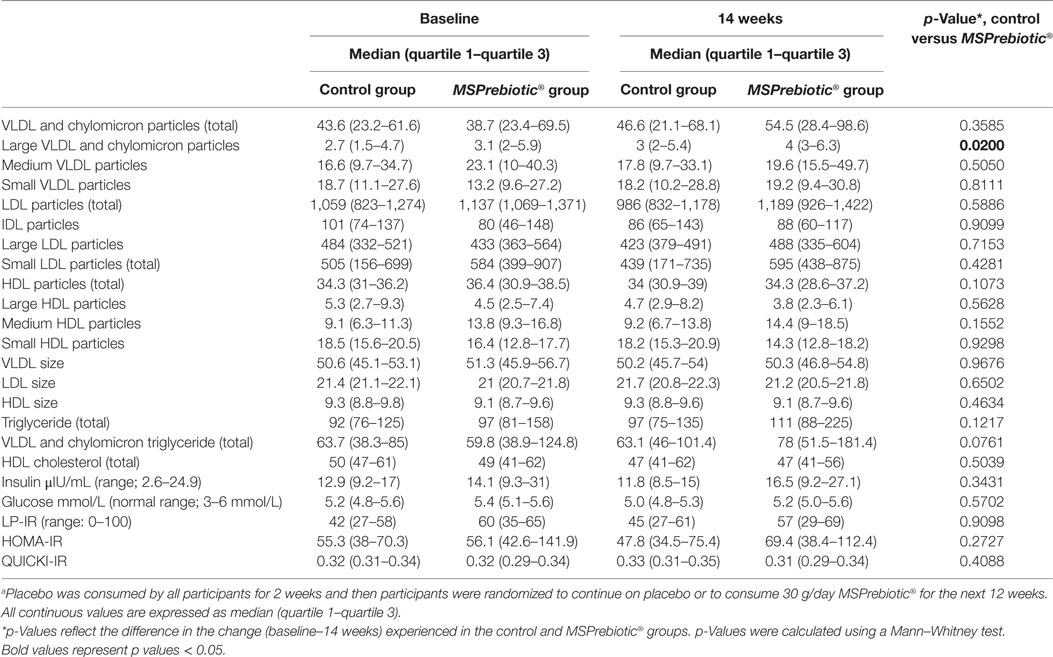
Table 4. Lipid particle and insulin resistance (IR) profiles in mid-age after consumption of MSPrebiotic or placeboa.
Despite all participants being screened against exclusion criteria for diabetes or prediabetes, the ELD group had significantly more individuals with elevated blood glucose levels at baseline (Table 1). Blood glucose levels of ELD participants assigned to take MSPrebiotic® were not significantly different from ELD participants assigned to take placebo at baseline (p = 0.515). Blood glucose levels significantly differed over time (p = 0.0301) in ELD participants taking MSPrebiotic® for 12 weeks compared to those consuming the placebo (Table 2). The change over the 14-week study is shown in Figure 3. Retesting of the samples at a second independent laboratory confirmed these results (Tables 3 and 4). Furthermore, the group/time interaction in the ELD population taking MSPrebiotic® versus placebo (Figure 3) showed there was a significant reduction (p = 0.045) in glucose levels as early as week 10 (i.e., after 2 weeks of placebo and 8 weeks of MSPrebiotic®).
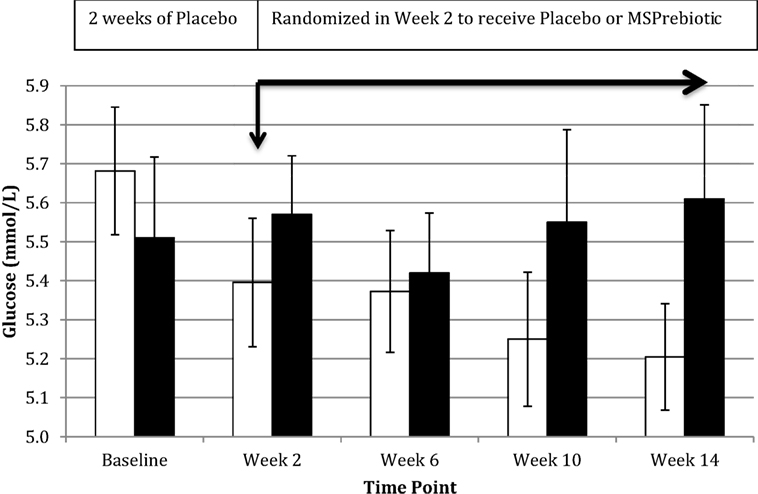
Figure 3. Impact of MSPrebiotic® versus placebo on mean blood glucose levels in the elderly (ELD) group. Placebo (30 g/day) was consumed by all participants for 2 weeks and then participants were randomized to continue on placebo (30 g/day) or to consume 30 g/day MSPrebiotic® for the next 12 weeks. Serum samples over the course of the study for the ELD group were analyzed on the day of collection for mean glucose levels. The black bars represent the placebo group and the white bars represent the MSPrebiotic® group. There was a significant group/time interaction for placebo versus MSPrebiotic® for the samples taken in week 0 compared to week 10 (8 weeks of consuming MSPrebiotic®) or week 14 (12 weeks of consuming MSPrebiotic®), p = 0.045 and p = 0.0124, respectively.
Blood insulin levels also significantly differed over time in ELD participants consuming MSPrebiotic® compared to the placebo group (Table 3). IR was calculated using three methods: LP-IR (calculated by LipoScience), HOMA-IR (40, 41), and QUICKI-IR (40) (Tables 3 and 4). The LP-IR IR was not significantly different between MSPrebiotic® and placebo groups over time in either ELD or MID categories (Tables 3 and 4). However, HOMA-IR and QUICKI-IR values showed significant improvement over time in IR for participants on MSPrebiotic® compared to placebo (p = 0.009 and 0.004, respectively).
Discussion
There is a strong link between gut microbiota dysbiosis, where pro-inflammatory Proteobacteria abundance increases, and the endotoxin from these bacteria stimulates TLR4 to cause an increase in inflammatory markers (e.g., TNF-α) and an increase in IR, which over a prolonged period can lead to hyperglycemia and ultimately T2D (5). We previously demonstrated that MSPrebiotic® promotes the growth of Bifidobacteria and corrects the Proteobacteria dysbiosis in the ELD (38), meeting recent prebiotic classification criteria (1). Here, we extend our analysis of our 2017 clinical trial by demonstrating that MSPrebiotic® is well-tolerated in MID and ELD adults (Figure 2), and by further characterizing the physiological consequences of the “bifidogenic effect.” Importantly, we found that MSPrebiotic® significantly reduced blood glucose and insulin levels by 7 and 41%, respectively, in otherwise healthy ELD individuals (Table 3). Taken together, the results from our clinical study suggest that MSPrebiotic® may be an effective tool in promoting the growth of Bifidobacteria and improving blood glucose management, especially in the ELD.
Previous studies have examined the blood glucose-lowering and insulin-sensitizing potential of DRS prebiotics (11, 13, 15, 21, 34). These studies examined the role of DRS as a carbohydrate replacement in food rather than as a supplement, where at least some of the effects on blood glucose level can be attributed to differences in the amount of digestible starch. While connections between DRS consumption and improved insulin sensitivity have been reported, they do not necessarily translate to lower blood glucose levels (15, 18, 19). In healthy people, this should not be surprising, given that the body will adapt by generating glucose via gluconeogenesis to prevent hypoglycemia in those with proper glycemic control (11). Such is likely the case in the MID population, where we observed no effect of MSPrebiotic® on blood glucose, insulin levels, or IR (Table 4). However, in the ELD population, where the baseline level of glucose was elevated toward the high end of the normal range (Table 1), consumption of MSPrebiotic® lowered the blood glucose levels within 8 weeks of supplementation (Figure 3). Gower et al. (18) reported that there was no impact of maize-derived DRS2 consumption on IR in women with adequate glucose homeostasis, whereas there was a significant reduction in IR in women with elevated IR. Our data are therefore consistent with those of Gower et al. (18), extending these findings to both men and women who are MID or ELD. In combination with other published data (2, 10, 13, 22, 33), our data suggest that various types of DRS prebiotics, just like other types of oligosaccharide prebiotics (i.e., FOS, GOS, XOS, etc.), have differing abilities to modulate lipids, glucose, and IR. Furthermore, the ability of prebiotics to modulate these parameters appears to be dependent on the extent of elevated blood glucose and IR (13, 18). For example, DRS can improve IR in humans who are prediabetic (18), but not after T2D has been established (13). Although the mean reduction of glucose in the ELD on MSPrebiotic® was small (7%), this is likely due to the limited room for blood glucose improvement in this group. For this reason, our findings support the value of future clinical studies on MSPrebiotic® in prediabetics.
Our data showed that MSPrebiotic® significantly reduced blood glucose and insulin levels in the ELD population and reduced IR as measured by HOMA-IR and QUICKI-IR (Table 3). However, LP-IR, which includes lipid particle analysis to determine IR in prediabetics with abnormal lipid profiles (40), was not significantly affected, suggesting that lower blood glucose levels were due to improved use of endogenous insulin rather than improved lipid metabolism. Given that the ELD and MID populations had neither prediabetes nor abnormal lipid profiles, it is not surprising that the HOMA-IR and QUICKI-IR (measures of IR based solely on fasting blood glucose and insulin) showed significant improvements in IR for those consuming MSPrebiotic® compared to placebo but LP-IR was not different. The microbiome changes and increases in butyrate (38), combined with lower blood glucose, insulin, and IR, were all benefits achieved without the use of probiotic supplementation. As suggested by others (5, 9, 13, 20, 22, 26, 28), our data support the concept that it is possible for the gut microbiome to be modulated via the consumption of specific types of prebiotics, leading to better glycemic control especially in ELD adults. Further studies are needed to clarify if the glucose and IR changes that were achieved by MSPrebiotic® consumption by the ELD and MID as described in this study are linked to or are independent from the bifidogenic effect also achieved by MSPrebiotic® in this same group of participants (38).
A recent animal study evaluated the effect of MSPrebiotic® supplementation on blood glucose, insulin, and IR in a swine model fed a Western diet (33). Of note, the swine were fed one half of the daily amount (15 g) of MSPrebiotic® that was consumed by our participants (30 g). While insulin levels were not significantly affected, blood glucose levels and IR were both reduced in swine consuming MSPrebiotic®. Furthermore, the authors also found a 141% increase in glucagon-like peptide-1 (GLP-1) in swine supplemented with MSPrebiotic®. GLP-1 is an incretin secreted by enteroendocrine cells (L-cells) in the colon in response to bacteria metabolites, which improves insulin utilization (8, 13), and increased GLP-1 could explain improved insulin sensitivity in response to fermentation of MSPrebiotic® in swine.
While determining the mechanisms by which blood glucose and IR decreased in the ELD group consuming MSPrebiotic® is beyond the scope of our investigation, it is appealing to speculate that increased GLP-1 in the MSPrebiotic® ELD group contributes to the improved glycemic response, which is consistent with recent publications (13, 23, 33). Alternatively, fermentation of MSPrebiotic® may have improved IR by stimulating production of short-chain fatty acids such as butyrate that have been shown to improve glucose homeostasis through intestinal gluconeogenesis (16). Such an explanation would also be consistent with our previous microbiome publication demonstrating that consumption of MSPrebiotic® led to a significant increase in the relative abundance of butyrate in ELD individuals (38). Other currently unknown mechanisms may also be at work (5, 8). Future studies examining the mechanisms by which MSPrebiotic® improves glycemic response in animals and humans are warranted.
Rideout et al. (33) also examined markers of cholesterol metabolism in their Western diet swine model, where they found that supplementation with MSPrebiotic® led to significant increases in total HDL particles, driven largely by an increase in the small HDL subclass of particles. Other cholesterol parameters were unchanged. The effect of DRS on cholesterol has been examined elsewhere, for example, in prediabetic obese individuals, where DRS improved these parameters (17). The consumption of wheat-based chemically modified resistant starch was correlated with a significant reduction in total cholesterol, HDL, and non-HDL (including LDL), but had no significant effect on triglycerides (2). Other published data (2, 10, 13) suggest that different types of DRS have differing abilities to modulate lipids, glucose, and IR. MSPrebiotic® had no significant impact on total cholesterol, HDL, or LDL, in either MID or ELD participants. However, there was a significant increase in the number of large VLDL/chylomicron particles in the MID group. To our knowledge, this is the first time that the consumption of DRS has been associated with such an effect, and the clinical significance of this is unclear.
Our data extend the published literature with respect to the impact of prebiotic consumption on the understudied ELD demographic (42, 43), which represents a large proportion of the Canadian population. Others have reported that ELD adults have an increased prevalence of non-specific inflammatory markers compared to younger adults, and this is thought to be due to increased permeability of the gut mucosa (5, 11, 14, 36, 42). Our data support this concept, as there were more ELD participants with elevated baseline CRP (trend toward statistical significance; p = 0.08) and TNF-α (p < 0.01) compared to the MID participants. This is likely linked to the microbiome dysbiosis in our ELD group, who at baseline had significantly increased levels of pro-inflammatory Proteobacteria (Escherichia coli/Shigella) (38). Of interest, the elevated inflammatory levels in the ELD group were not reduced by the end of the study in either the placebo or MSPrebiotic® groups. This suggests that the colonocyte apoptosis or reduced mucus production (36, 44) leading to increased bowel permeability (thought to be the basis of increased inflammatory response) could be irreversible or take longer than the 3-month period evaluated in our study. It may be that earlier addition of prebiotics to the diet (i.e., before 70 years old) is needed to prevent the bowel damage, permeability, and the associated increase in non-specific inflammatory markers.
A limitation of our study was the relatively small sample size (N = 21 or 22 for each group). However, we offset this limitation by collecting samples at multiple time points over 14 weeks for each participant (i.e., not just a one-time sampling). Despite a small sample size, we were able to demonstrate significant changes in glucose, insulin, and IR, supporting the adequacy of our power calculations. Furthermore, we would recommend future studies that have less extensive exclusion criteria than the current study undertaken in the ELD population to determine the impact of DRS in a wider cross-section of this demographic. This type of clinical study is needed to more extensively assess the clinical impact of MSPrebiotic® in the ELD population.
In conclusion, our data demonstrated that MSPrebiotic® is a prebiotic as defined by Gibson et al. (1) that is well-tolerated in Canadian adults and that this DRS effectively lowers blood glucose and insulin levels and reduces IR in ELD adults. Future studies in subjects with prediabetes and T2D are warranted given that dietary supplementation is an understudied avenue for the amelioration of risk factors linked to the development and progression of these conditions. While MSPrebiotic® had a minimal impact on cholesterol metabolism, further studies in dyslipidemic individuals are required to fully evaluate the effect(s) of this DRS on these measures. Consumption of MSPrebiotic® for 3 months was not sufficient to reduce the elevated CRP and TNF-α levels in the ELD group, suggesting that earlier intervention (i.e., before age 70) may be necessary to prevent the development of gut barrier damage or that these inflammatory markers are due to other underlying factors such as vascular comorbidities (45).
Ethics Statement
This study was carried out in accordance with the recommendations of University of Manitoba Research Ethics Board and Health Canada with written informed consent from all subjects. All subjects gave written informed consent in accordance with the Declaration of Helsinki. The protocol was approved by the University of Manitoba Research Ethics Board and Health Canada. No animal subjects were used in this study.
Author Contributions
All the authors provided substantial contribution to the design of the work as well as the acquisition, analysis, and interpretation of the work. MA drafted the manuscript, and all the other authors contributed to revising and critical assessment of intellectual content. All the authors have given final approval of this manuscript and agree to be accountable for all aspects of the work and to ensuring that all questions related to the accuracy or integrity of any part of the work are properly investigated and resolved.
Conflict of Interest Statement
MSPrebiotics Inc. paid for printing of a poster and for flight, accommodation, and speaker honorarium for MA to attend 4th Microbiome R&D and Business Collaboration Forum: USA October 3–4, 2016, San Diego, CA, USA, for poster presentation of the microbiome data from this clinical study (i.e., data presented in this current manuscript were not presented at the conference). There are no disclaimers or conflicts of interest for any of the other authors.
Acknowledgments
The outstanding support and assistance from all the Deer Lodge staff (particularly Michael Kaan, Daryl Dyck, Jo-Ann Lapointe-Mckenzie, and Jean Helps) for oversight of the initial participant screening, product administration, and stool sample collection is acknowledged. The key role of Nila McFarlane and Betty Smith in completing the informed consent and enrollment of participants from Deer Lodge is acknowledged. We thank all the study support staff and participants for their enthusiasm. We would like to acknowledge and thank Jason Bush and Diane Clayton for their help with reviewing and suggesting edits for this manuscript.
Funding
The funding for this study was provided by the National Research Council of Canada and by MSPrebiotics Inc. The Natural Health Products-quality packages of MSPrebiotic® and Amioca were provided by MSPrebiotics Inc.
References
1. Gibson GR, Hutkins R, Sanders ME, Prescott SL, Reimer RA, Salminen SJ, et al. Expert consensus document: the International Scientific Association for Probiotics and Prebiotics (ISAPP) consensus statement on the definition and scope of prebiotics. Nat Rev Gastroenterol Hepatol (2017). 14(8):491–502. doi:10.1038/nrgastro.2017.75
2. Haub MD, Hubach KL, Al-tamimi EK, Ornelas S, Seib PA. Different types of resistant starch elicit different glucose responses in humans. J Nutr Metab (2010) 2010:1–4. doi:10.1155/2010/230501
3. Delzenne NM, Neyrinck AM, Cani PD. Modulation of the gut microbiota by nutrients with prebiotic properties: consequences for host health in the context of obesity and metabolic syndrome. Microb Cell Fact (2011) 10(Suppl 1):S10. doi:10.1186/1475-2859-10-S1-S10
4. Holmes E, Kinross J, Gibson GR, Burcelin R, Jia W, Pettersson S, et al. Therapeutic modulation of microbiota-host metabolic interactions. Sci Transl Med (2012) 4:137. doi:10.1126/scitranslmed.3004244
5. Carvalho BM, Abdalla Saad MJ. Influence of gut microbiota on subclinical inflammation and insulin resistance. Mediators Inflamm (2013) 2013:986734. doi:10.1155/2013/986734
6. MacNeil S, Rebry RM, Tetlow IJ, Emes MJ, McKeown B, Graham TE. Resistant starch intake at breakfast affects postprandial responses in type 2 diabetics and enhances the glucose-dependent insulinotropic polypeptide-insulin relationship following a second meal. Appl Physiol Nutr Metab (2013) 38:1187–95. doi:10.1139/apnm-2013-0023
7. Slavin S. Fiber and prebiotics: mechanisms and health benefits. Nutrients (2013) 5:1417–35. doi:10.3390/nu5041417
8. Everard A, Cani PD. Gut microbiota and GLP-1. Rev Endocr Metab Disord (2014) 15:189–96. doi:10.1007/s11154-014-9288-6
9. Han JL, Lin HL. Intestinal microbiota and type 2 diabetes: from mechanism insights to therapeutic perspective. World J Gastroenterol (2014) 20:17737–45. doi:10.3748/wjg.v20.i47.17737
10. Nichenametla SN, Weidauer LA, Wey HE, Beare TM, Specker BL, Dey M. Resistant starch type 4-enriched diet lowered blood cholesterols and improved body composition in a double-blind controlled crossover intervention. Mol Nutr Food Res (2014) 58:1365–9. doi:10.1002/mnfr.201300829
11. Keenan M, Zhou J, Hegsted M, Pelkman C, Durham HA, Coulon DB, et al. Role of resistant starch in improving gut health, adiposity, and insulin resistance. Adv Nutr (2015) 6:198–205. doi:10.3945/an.114.007419
12. Bindels LB, Delzenne NM, Cani PD, Walter J. Towards a more comprehensive concept for prebiotics. Nat Rev Gastroenterol. Hepatol (2015) 12:303–10. doi:10.1038/nrgastro.2015.47
13. Bindels LB, Walter J, Ramer-Tait AE. Resistant starches for the management of metabolic diseases. Curr Opin Clin Nutr Metab Care (2015) 18:559–65. doi:10.1097/MCO.0000000000000223
14. Arboleya S, Watkins C, Stanton C, Ross RP. Gut bifidobacteria populations in human health and aging. Front Microbiol (2016) 7:1204. doi:10.3389/fmicb.2016.01204
15. Dainty SA, Klingel SL, Pilkey SE, McDonald E, McKeown B, Emes MJ, et al. Resistant starch bagels reduce fasting and postprandial insulin in adults at risk of type 2 diabetes. J Nutr (2016) 146:2252–9. doi:10.3945/jn.116.239418
16. De Vadder F, Kovatcheva-Datchary P, Goncalves D, Vinera J, Zitoun C, Duchampt A, et al. Microbiota-generated metabolites promote metabolic benefits via gut-brain neural circuits. Cell (2014) 156(1–2):84–96. doi:10.1016/j.cell.2013.12.016
17. Dodevska MS, Sobajic SS, Djordevic PB, Simitrijvec-Sreckovic VS, Spasojevic-Kalimanovska VV, Djordjevic BI. Effects of total fibre or resistant starch-rich diets within lifestyle intervention in obese prediabetic adults. Eur J Nutr (2016) 55(1):127–37. doi:10.1007/s00394-015-0831-3
18. Gower BA, Bergman R, Stefanovski D, Darnell B, Ovalle F, Fisher G, et al. Baseline insulin sensitivity affects response to high-amylose maize resistant starch in women: a randomized, controlled trial. Nutr Metab (2016) 13:2. doi:10.1186/s12986-016-0062-5
19. Karimi P, Farhangi MA, Saramadi B, Gargari BP, Zare JA, Pouraghae M, et al. The therapeutic potential of resistant starch in modulation of insulin resistance, endotoxemia, oxidative stress and antioxidant biomarkers in women with type 2 diabetes: a randomized controlled clinical trial. Ann Nutr Metab (2016) 68:85–93. doi:10.1159/000441683
20. Neyrinck AM, Pachikian B, Taminiau B, Daube G, Frédérick R, Cani PD, et al. Intestinal sucrase as a novel target contributing to the regulation of glycemia by prebiotics. PLoS One (2016) 11:e0160488. doi:10.1371/journal.pone.0160488
21. O’Connor LE, Campbell WW. A novel fiber composite ingredient incorporated into a beverage and bar blunts postprandial serum glucose and insulin responses: a randomized controlled trial. Nutr Res (2016) 36:253. doi:10.1016/j.nutres.2015.11.015
22. Scheithauer TPM, Dallinga-Thie GM, de Vos WM, Nieuwdorp M, van Raalte DH. Causality of small and large intestinal microbiota in weight regulation and insulin resistance. Mol Metab (2016) 5:759–70. doi:10.1016/j.molmet.2016.06.002
23. Zietec T, Roth E. Inflammation meets metabolic disease: gut feeling mediate by GLP-1. Front Immunol (2016) 7:154. doi:10.3389/fimmu.2016.00154
24. Kleesen B, Dykura B, Aunft H-J, Blaut M. Effects of inulin and lactose on fecal microflora, microbial activity and bowel habit in elderly constipated persons. Am J Clin Nutr (1997) 65:1397–402.
25. Toward RE, Montandon SL, Walton GE, Gibson GR. Effect of prebiotics on the human gut microbiota of elderly persons. Gut Microbes (2012) 3:57–60. doi:10.4161/gmic.19411
26. Patel R, DuPont HL. New approaches for bacteriotherapy: prebiotics, new-generation probiotics and synbiotics. Clin Infect Dis (2015) 60:S108–21. doi:10.1093/cid/civ177
27. Marchesi JR, Adams DH, Fava F, Hermes GDA, Hirschfield GM, Hold G, et al. The gut microbiota and host health: a new clinical frontier. Gut (2016) 65:330–9. doi:10.1136/gutjnl-2015-309990
28. Costabile A, Deaville ER, Morales AM, Gibson GR. Prebiotic potential of a maize-based soluble fibre and impact of dose on the human gut microbiota. PLoS One (2016) 11(1):e0144457. doi:10.1371/journal.pone.0144457
29. Wang M, Donovan SM. Human microbiota-associated swine: current progress and future opportunities. ILAR J (2015) 56:63–73. doi:10.1093/ilar/ilv006
30. Gutierrez K, Dicks N, Glanzner WG, Agellon LB, Bordignon V. Efficacy of the porcine species in biomedical research. Front Genet (2015) 6:293. doi:10.3389/fgene.2015.00293
31. Santulli G, Borras C, Bousquet J, Calza L, Cano A, Illario M, et al. Models for preclinical studies in ageing-related disorders: one is not for all. Transl Med UniSa (2015) 13:4–12.
32. Heinritz SN, Weiss E, Eklund M, Aumiller T, Louis S, Rings A, et al. Intestinal microbiota and microbial metabolites are changed in a pig model fed a high-fat/low-fiber or a low-fat/high-fiber diet. PLoS One (2016) 11:e0154329. doi:10.1371/journal.pone.0154329
33. Rideout TC, Harding SV, Raslawsky A, Rempel C. Dietary resistant starch supplementation increases HDL particle number in pigs fed a western diet. J Diet Suppl (2017) 14(3):334–345. doi:10.1080/19390211.2016.1229371
34. Edwards CH, Grundy MM, Grassby T, Vasilopoulou D, Frost GS, Butterworth PJ, et al. Manipulation of starch bioaccessibility in wheat endosperm to regulate starch digestion, postprandial glycemia, insulinemia, and gut hormone responses: a randomized controlled trial in healthy ileostomy participants. Am J Clin Nutr (2015) 102:791–800. doi:10.3945/ajcn.114.106203
35. Boll EV, Ekstrom LM, Courtin CM, Delcour JA, Nilsson AC, Bjorck IM, et al. Effects of wheat bran extract rich in arabinoxylan oligosaccharides and resistant starch on overnight glucose tolerance and markers of gut fermentation in healthy young adults. Eur J Nutr (2016) 55:1661–70. doi:10.1007/s00394-015-0985-z
36. Desai MS, Seekatz AM, Koropatkin NM, Kamada N, Hickey CA, Wolter M, et al. A dietary fiber-deprived gut microbiota degrades the colonic mucus barrier and enhances pathogen susceptibility. Cell (2016) 167:1339–53. doi:10.1016/j.cell.2016.10.043
37. Verbeke KA, Boobis AR, Chiodini A, Edwards CA, Fanck A, Kleerebezem M, et al. Towards microbial fermentation metabolites as markers for health benefits of prebiotics. Nutr Res Rev (2015) 28:42–66. doi:10.1017/S0954422415000037
38. Alfa MJ, Strang D, Tappia PS, Graham M, Van Domselaar G, Forbes JD, et al. A randomized trial to determine the impact of the digestion resistant starch MSPrebiotic® versus placebo on the gut microbiome in elderly compared to mid-age adults. Eur J Clin Nutr (2017). doi:10.1016/j.clnu.2017.03.025
39. Health Canada. Natural Health Product. (2016). Available from: http://webprod.hc-sc.gc.ca/nhpid-bdipsn/ingredReq.do?id=6482&lang=eng, Solanum Tuberosum Extract
40. Shalaurova I, Connelly MA, Garvey WT, Otvos JD. Lipoprotein insulin resistance index: a lipoprotein particle-derived measure of insulin resistance. Metab Syndr Relat Disord (2014) 12:422–9. doi:10.1089/met.2014.0050
41. Matyus SP, Brauna PJ, Wolak-Dinsmore J, Saenger AK, Jeyarajah EJ, Shalaurova I, et al. HDL particle number measured on the Vantera®, the first clinical NMR analyzer. Clin Biochem (2015) 48:148–55. doi:10.1016/j.clinbiochem.2014.11.017
42. Biagi C, Candela M, Fairweather-Tait C, Ostan R, Bucci L, Pini E, et al. Ageing of the human metaorganism: the microbial counterpart. Age (2012) 34:247–67. doi:10.1007/s11357-011-9217-5
43. Kolanowski A, Mulhall P, Yevchak A, Hill N, Fick D. The triple challenge of recruiting older adults with dementia and high medical acuity in skilled nursing facilities. J Nurs Scholarsh (2013) 45:397–404. doi:10.1111/jnu.12042
44. Sun J, Shen X, Li Y, Guo Z, Zhu W, Zuo L, et al. Therapeutic potential to modify the mucus barrier in inflammatory bowel disease. Nutrients (2016) 8(1):44. doi:10.3390/nu8010044
Keywords: digestion resistant starch, elderly, diabetes, glucose, insulin-resistance, gut microbiome, prebiotics
Citation: Alfa MJ, Strang D, Tappia PS, Olson N, DeGagne P, Bray D, Murray B-L and Hiebert B (2018) A Randomized Placebo Controlled Clinical Trial to Determine the Impact of Digestion Resistant Starch MSPrebiotic® on Glucose, Insulin, and Insulin Resistance in Elderly and Mid-Age Adults. Front. Med. 4:260. doi: 10.3389/fmed.2017.00260
Received: 17 August 2017; Accepted: 26 December 2017;
Published: 22 January 2018
Edited by:
Tzvi Dwolatzky, Rambam Health Care Campus, IsraelReviewed by:
Mario Ulises Pérez-Zepeda, Instituto Nacional de Geriatría, MexicoRenato Gorga Bandeira De Mello, Federal University of Rio Grande do Sul (UFRGS), Brazil
Copyright: © 2018 Alfa, Strang, Tappia, Olson, DeGagne, Bray, Murray and Hiebert. This is an open-access article distributed under the terms of the Creative Commons Attribution License (CC BY). The use, distribution or reproduction in other forums is permitted, provided the original author(s) or licensor are credited and that the original publication in this journal is cited, in accordance with accepted academic practice. No use, distribution or reproduction is permitted which does not comply with these terms.
*Correspondence: Michelle J. Alfa, bWFsZmFAc2JyYy5jYQ==