- Department of Immunohematology and Blood Transfusion, Leiden University Medical Center, Leiden, Netherlands
In allogeneic transplantation, genetic disparities between patient and donor may lead to cellular and humoral immune responses mediated by both naïve and memory alloreactive cells of the adaptive immune system. This review will focus on alloreactive T and B cells with emphasis on the memory compartment, their role in relation to kidney rejection, and in vitro assays to detect these alloreactive cells. Finally, the potential additional value of utilizing donor-specific memory T and B cell assays supplementary to current routine pre-transplant risk assessment of kidney transplant recipients will be discussed.
Introduction
Immunological memory, the capacity to mount a rapid and robust immune response when a given antigen is re-encountered, protects individuals against a wide range of pathogens following infections or vaccinations (1). Both cellular and humoral adaptive immune responses contribute to this long-lasting protection, making long-lived memory T and B cells the central players in immunological memory. These cells are generated by clonal expansion of a subset of antigen-specific naïve cells during primary immune responses, and can persist over decades after the pathogen has been eliminated (2–4). Although recent evidence suggests that some features of immunological memory exist in innate immune cells, memory T and B cells of the adaptive immune system will be the focus of this review (5).
While being extremely effective in protecting individuals against pathogens, memory T and B cells can sabotage successful allogeneic transplantations by eliciting vigorous alloimmune responses to donor antigens. Alloreactive memory T and B cells may arise as a result of exposure to allogeneic human leukocyte antigens (HLA) through blood transfusions, pregnancies or previous transplantations. Interestingly, individuals who have never been exposed to alloantigens may as well harbor alloreactive memory as a result of heterologous immunity (6, 7).
Advances in immunosuppressive drugs have led to a dramatic reduction in acute rejection rates resulting in significant improvements in short term allograft survival. However, lack of improvement of long-term graft survival remains a major problem in kidney transplantation. Furthermore, repeat transplantation candidates, as well as those with a history of pregnancy and multiple blood transfusions who have broad HLA sensitization tend to accumulate on transplant waitlists. These patients are not only difficult to pair with a crossmatch negative donor in order to be transplanted but are also more susceptible to develop T cell and/or antibody-mediated acute and chronic rejection unless special allocation algorithms or desensitization treatments are used (8–12).
In the setting of kidney transplantation, the current practice of immunological pre-transplant risk assessment mainly focuses on the presence or absence of plasma cell-derived donor HLA directed antibodies (DSA) in the serum of patients, in addition to HLA matching between the patient and donor (13, 14). This strategy, however, ignores the potential contribution of alloreactive memory cells to graft rejection (15–19). In this review, we will focus on alloreactive (memory) cells and their possible contribution to rejection episodes primarily in the setting of kidney transplantation, and provide an overview of in vitro assays to detect alloreactive memory T and B cells. Furthermore, we will elaborate on the potential use of these assays in pre-transplant immunological risk assessment of kidney transplant recipients.
Contribution of Alloreactive T and B cells to Graft Rejection
Alloreactive T Cells
Alloreactive T cells are considered to be the central players in mediating allograft rejection. They contribute to both acute and chronic rejection depending on the pathway utilized to recognize donor antigens (both major and minor histocompatibility antigens). T cells recognize alloantigens through the direct, indirect or semi-direct pathway (Figure 1). The direct pathway of T cell recognition is unique to allogeneic transplantation, and involves both CD4 and CD8 T cells of the recipient recognizing intact allogeneic major histocompatibility complex (MHC) antigens class II and I, respectively, expressed on the surface of donor cells (Figure 1A). This pathway of allorecognition is considered to be short-lived, especially for HLA class II, due to the limited life-span of donor dendritic cells migrating to lymphoid tissues of the recipient to initiate the immune response. Therefore, the direct pathway T cells are considered to be the predominant mediators of acute cellular rejections in the early post-transplantation period, although MHC expressed on graft parenchyma may as well directly activate T cells at any time after transplantation, contributing to long term injury (20–23).
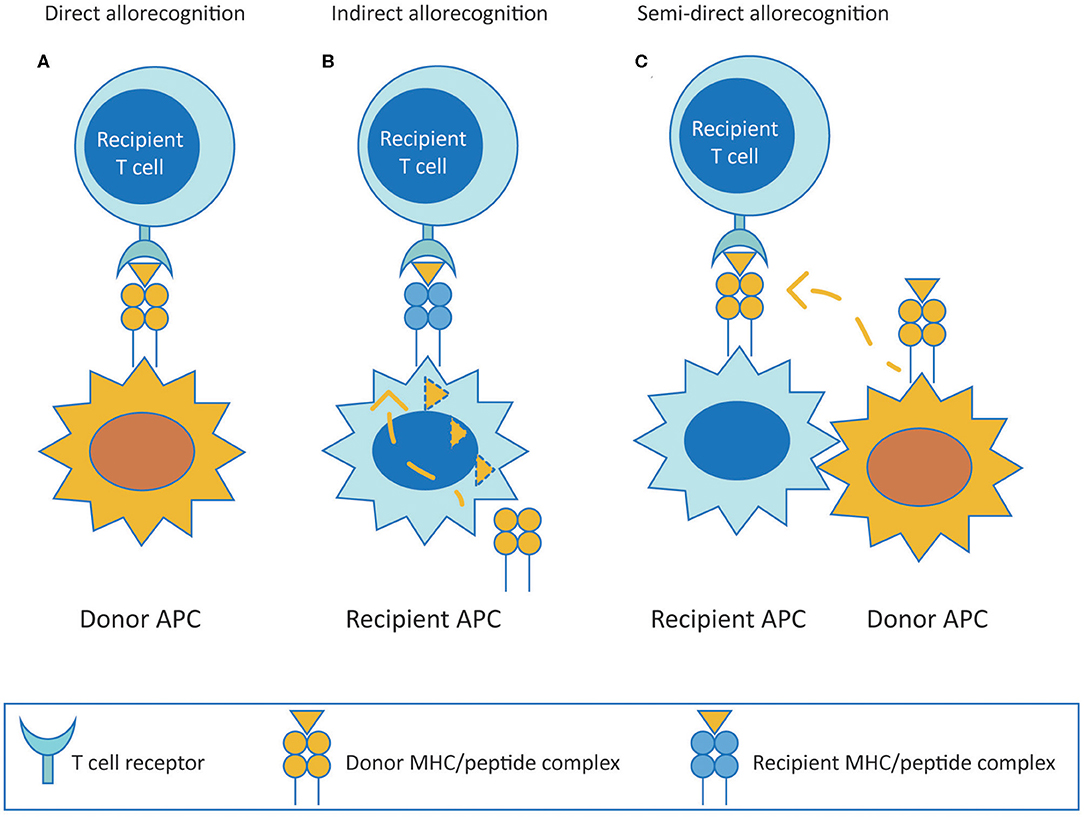
Figure 1. T cell allorecognition pathways. (A) (Direct pathway) Recipient T cells recognize intact donor alloantigens on the surface of donor APC. (B) (Indirect pathway) Recipient T cells recognize processed donor allogeneic peptides presented on the context of self MHC antigen by recipient APC. (C) (Semi-direct pathway) Recipient T cells recognize intact donor MHC acquired by recipient APC. MHC, major histocompatibility complex; APC, antigen presenting cell.
In comparison to conventional T cell responses to protein antigens, the direct pathway alloimmune response is stronger, likely due to the high frequency of direct pathway alloreactive T cells (24). This allows for measurement of direct pathway alloimmune responses in vitro without the need for priming in mixed lymphocyte reactions (MLR). T cell alloimmune responses measured in vitro involves CD4 and CD8 T cells with contributions both from naïve and memory T cell fractions (25, 26). Between 1-10% of circulating T cells in humans are known to be alloreactive as tested by traditional assays (27, 28). Recently, using high throughput sequencing in combination with MLR in healthy individuals, Emerson et al. observed an average of 14,000 alloreactive T cell clones in each experiment they performed. Strikingly, antigen-experienced memory T cell clones made up to 60% of the alloreactive T cell repertoire (29). In addition, the alloreactive memory T cell repertoire could be detected at similar clonal frequencies in a later time point sample when the same allogeneic donor was used for stimulation in MLR, indicating their persistence in circulation. Presence of alloreactive memory T cells in individuals who have never been exposed to alloantigens is supportive for a role of heterologous immunity by which T cells generated in response to infectious or environmental antigens can cross-react with allogeneic MHC antigens (30). Indeed, cross reactivity of virus-induced memory T cells with allogeneic HLA has been shown to be common (7). A classic example of cross reactivity of virus-induced memory T cells with alloantigens is that of HLA-B*08:01 bearing patients who have been exposed to Epstein-Barr virus (EBV) infection showing cross-reactivity to allogeneic HLA-B*44:02 (6, 31). Cross-reactivity of virus-induced T cell receptors (TCR) with alloantigens could be of clinical relevance because they have been shown to directly recognize donor MHC and cause allograft rejection in murine studies. However, a significant impact on transplantation outcome in humans has not been shown so far (32, 33).
The indirect pathway is analogous to adaptive T cell responses mounted to common protein antigens, and involves alloreactive T cells of the recipient recognizing allogeneic MHC class I or class II as processed peptides presented in the context of self MHC class II (Figure 1B). Indirect pathway alloreactive CD4 T cells can provide help to induce cytotoxic CD8 T cells and are known to be the only cells that can provide help to alloreactive B cells (34–36). The indirect pathway of T cell allorecognition is considered to be long-lasting and particularly important in the development of chronic allograft rejection because of exclusive cognate help provided by indirect CD4 T cells to alloreactive B cells leading to alloantibody production. Indirect allorecognition can also apply to alloreactive CD8 T cells through cross-priming whereby antigen presenting cells (APC) present alloantigenic peptides in the context of class I molecules (37). However, indirect pathway CD8 T cells have been shown to have no role on alloimmune response to vascularized cardiac allografts in murine models, possibly because CD8 T cells require direct contact with target cells to exert their cytolytic effects (38). Additionally, indirect alloresponses against minor histocompatibility antigens may also occur. However, in comparison to the enormous polymorphism of MHC, indirect allorecognition of minor antigens appears to be less relevant (39).
Finally, in the semi-direct pathway, recipient T cells recognize intact allo-MHC similar to direct way of allorecognition but on the surface of self APC that have acquired allo-MHC by various means including cell to cell contact or exosomes, suggesting a role for both direct and indirect allorecognition pathways in chronic alloimmune responses (Figure 1C) (40, 41).
Alloreactive B Cells
Humoral alloimmunity can lead to antibody mediated rejection (ABMR), of which the chronic form is the leading cause of graft loss in kidney transplantation (42). Interaction between alloreactive T and B cells plays a key role in the generation of full-blown humoral alloimmune responses. Help from indirect pathway CD4 T cells is essential for generating antibodies against HLA, as with conventional protein antigens. Typically, when a naïve B cell ligates a protein antigen via its B cell receptor (BCR) in secondary lymphoid organs, it internalizes the antigen by receptor mediated endocytosis and then migrates to the interface between the B cell follicles and the T cell zone of the lymphoid tissue. At the T-B cell border of the lymphoid tissue, activated B cells present the processed peptide in the context of self MHC class II to cognate CD4 T cells in the presence of CD40-CD154 ligation (43). Activated B cells receiving help from CD4 T cells at the T-B cell border can either form an extrafollicular response and become short-lived plasma cells or go back to B cell follicles and initiate germinal center reactions (44). In the pre-germinal center period, class switching to IgG isotype may occur whereas somatic hypermutations are not observed and antibodies of the extrafollicular foci are known to be low/moderate affinity antibodies (45). Some of the activated B cells in the extrafollicular foci may give rise to germinal center independent memory B cells. Within germinal centers, B cells undergo somatic hypermutation to increase the affinity of their BCRs for the antigen. B cells that recognize the antigen presented by follicular dendritic cells in germinal centers internalize, process and present the peptides to follicular T helper cells (Tfh) (46). In germinal centers, B cells compete for limited availability of Tfh interactions of which are critical for high affinity B cells to be selected further (47). Those B cells that have an improved affinity for the antigen are selected to leave the germinal centers either as isotype switched memory B cells or plasma cells producing high affinity isotype switched antibodies (IgG, IgA, and IgE). While B cells with highest affinity antigen receptors are preferentially recruited to the plasma cell pool, B cells with less high BCR affinity may be selected for the memory B cell pools. Less stringent selection criteria for memory B cells generates a more diverse memory B cell repertoire compared to long-lived plasma cell pools (48, 49). Some germinal center B cells leave germinal centers before the class switch recombination occurs and may give rise to IgM memory B cells which have the capacity to re-enter germinal centers and give rise to new IgG memory B cells (45).
Following germinal center reactions, plasma cells home to the bone marrow and mucosal tissues to become long-lived plasma cells, whereas memory B cells circulate in their quiescent form until a reencounter with the antigen takes place. Despite the fact that the fate of B cells following germinal centers is extensively investigated in studies focusing on responses against pathogenic or vaccine antigens, it is still unknown what proportion of B cells going through germinal center reactions is committed to become plasma cells or memory B cells. For some viral and vaccine antigens, correlations of memory B cells with serum antibodies are known (3) but it is not clear whether immunization against a certain HLA antigen will always result in generation of both memory and plasma cells and whether the ratio of these cells is different in individuals, per route of immunization, and even per HLA antigen encountered. Likewise, while more diversity has been shown for the memory B cell repertoire in comparison to serum antibodies using high throughput heavy chain variable region sequencing of BCR and serum IgG in the setting of tetanus toxoid vaccination in humans (49), knowledge on memory B cell derived vs. serum HLA antibody profiles is newly emerging with indications suggesting that they are not identical (50–52). The latter is particularly important considering the property of memory B cells to rapidly differentiate into antibody producing cells and to give rise to donor-specific antibody (DSA) responses in patients previously known to have no serum DSA (53). In addition to antigen-specific re-encounter, anamnestic responses can also derive from bystander activation of HLA-specific memory B cells upon infection or vaccination (54). In this context, given that exposure to viral infections shapes the alloreactive T cell repertoire of an individual, we wondered whether this would hold true for B cells as well. However, by testing several virus-specific monoclonal antibodies against HLA and many HLA-specific monoclonal antibodies against viral antigens, we did not detect such a cross-reactivity between viral antigens and HLA for B cells at least at the level of monoclonal antibodies (55).
T-B Cell Interactions
Interactions between alloreactive T and B cells in secondary lymphoid organs may have implications on the strength and specificity of alloantibody produced. A recent study in a mice heart transplant model, designed to investigate solely the contribution of indirect allorecognition to ABMR in the absence of direct pathway CD8 cytotoxic T cells, showed that when a high number of alloreactive CD4 T cells are present to provide help to B cells, this may lead to extrafollicular alloantibody responses with moderate affinity yet still capable of binding to endothelial cells and activating complement and causing acute ABMR, regardless of the number of allospecific B cells (56). On the contrary, germinal center activity leading to high affinity antibody production and progression to chronic allograft injury was more profoundly influenced by the number of antigen-specific B cells provided that they receive help from Tfh cells (57). Differences in the nature of interactions between alloreactive CD4 T cells and B cells shown in these studies suggest that some de novo DSA that disappear early after transplantation may be a product of extrafollicular foci, whereas those that persist may be high affinity DSA produced by long-lived plasma cells and both with the capacity to mediate ABMR.
Conventionally, CD4 T cells and B cells that are specific for different epitopes of the same antigen cooperate through linked recognition in order to generate long-lasting humoral immunity specific for a protein antigen. Interestingly, studies in mice have shown evidence that B cells recognizing one donor MHC could receive help from T cells specific for another allogeneic MHC on the donor organ although the alloantibody levels generated were relatively lower compared to the conventional way of receiving help in this “unlinked help condition” (58). Interestingly, when naïve CD4 T cell help was abolished by co-stimulation blockade targeting CD40/CD154 interactions, interferon-gamma (IFN-γ) producing memory CD4 T cells could still provide help to B cells (59, 60). Hypothetically, in a setting where there are multiple HLA mismatches between the patient and donor and alloreactive T cell activation is independent of alloreactive B cell activation, memory CD4 T cells generated in response to a previous alloantigen may provide help to a naïve B cell leading to the production of a different alloantibody specificity and isotype at least through extrafollicular immune responses (58). This type of immune response in humans could also occur when high frequency alloreactive memory CD4 T cells are present before transplantation as a result of previous alloantigen exposure or heterologous immunity.
In vitro Methods to Detect Alloreactive T and B cells
The development of in vitro methods allowing for accurate, sensitive and reproducible detection and quantification of donor-specific alloimmune responses has long been a challenge in the field of transplantation immunology. Such assays are required not only to detect the sensitization against a potential donor before transplantation, and thus select the patient-donor pair with the lowest risk of alloimmune responses, but also to be able to monitor the ongoing alloimmune response post-transplant with non-invasive methods in order to adjust the immunosuppressive treatments. Currently available methods to detect alloreactive T and B cells are outlined in Figures 2, 3, respectively, and in Table 1.
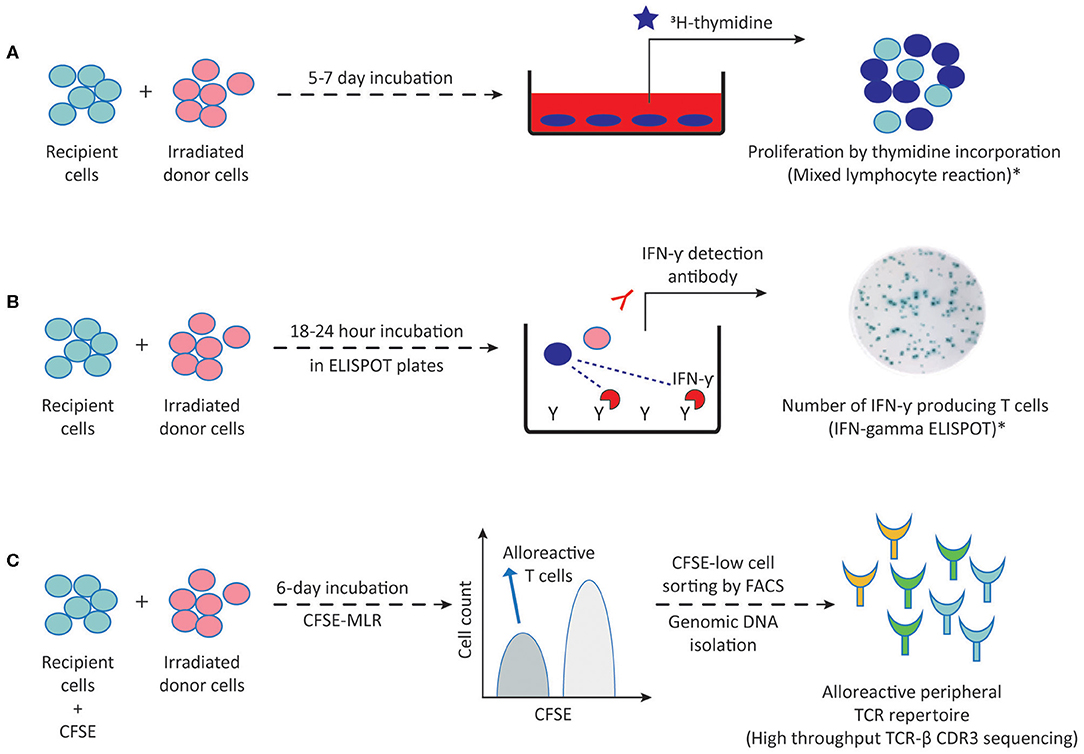
Figure 2. Methods to detect alloreactive T cells. (A) Proliferation of direct pathway alloreactive T cells following 5–7 days incubation of recipient cells (responder) with irradiated donor cells (stimulator) can be measured by 3H-thymidine incorporation into proliferating cells in MLR. (B) Following a 18–24 h MLR in ELISPOT plates, IFN-γ producing direct pathway primed/memory T cells can be visualized by addition of the IFN-γ detection antibody. (C) Following a 6-day MLR, CFSE-low dividing direct pathway alloreactive T cells are sorted by FACS and genomic DNA is isolated for high throughput TCR-β CDR3 sequencing. This generates an alloreactive TCR repertoire which can then be compared to the TCR repertoire of the unstimulated patient sample. 3H-thymidine, tritiated thymidine; CFSE, carboxyfluorescein succinimidyl ester; MLR, mixed lymphocyte reaction; TCR-β, T cell receptor beta chain; FACS, fluorescence activated cell sorting; CDR3, complementarity determining region-3. Dark blue circles represent alloreactive T cells. *These methods are also being used for detecting indirect pathway alloreactive T cells by replacing irradiated donor cells with donor cell fragments, synthetic peptides or synthetic HLA molecules.
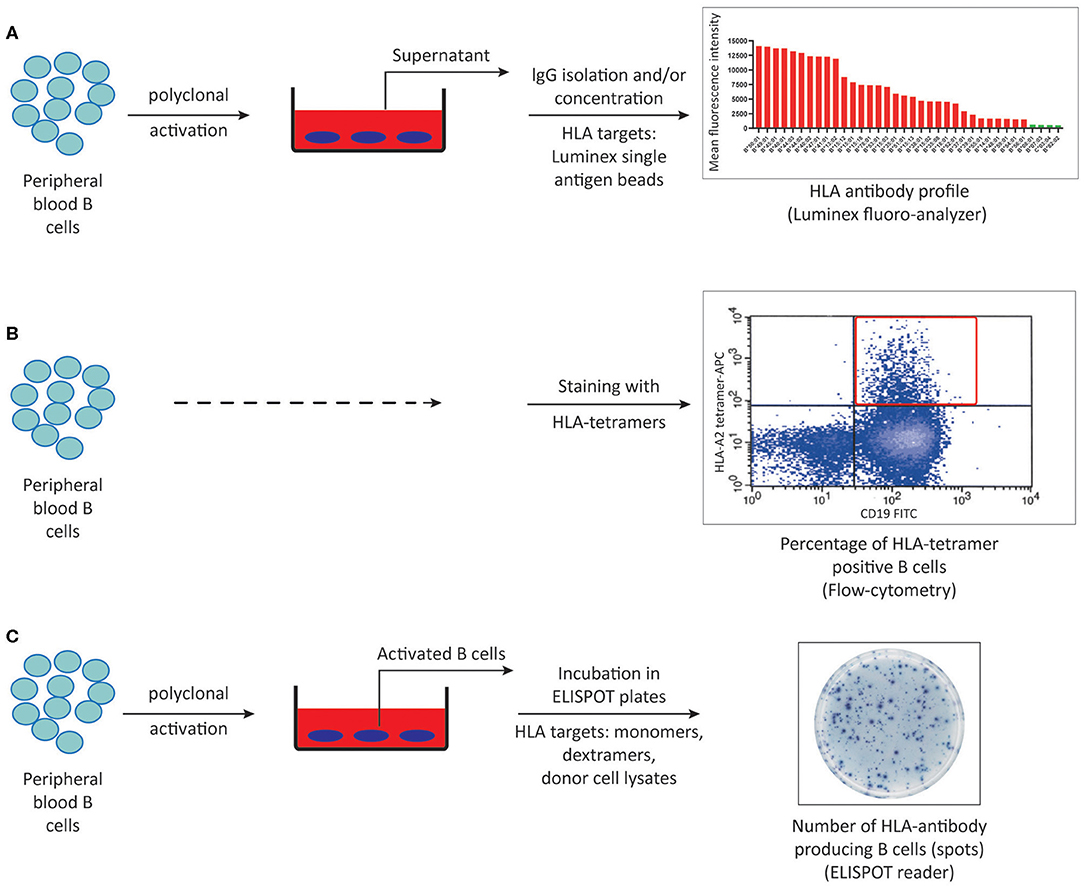
Figure 3. Methods to detect HLA-specific memory B cells. (A) Peripheral blood B cells can be polyclonally activated and IgG isolated culture supernatants can be screened for memory B cell derived-HLA antibodies using luminex single antigen bead assays. (B) Peripheral blood B cells can be stained with HLA-tetramers using flow cytometry. (C) HLA-specific memory B cells can be quantified by ELISPOT assay using either synthetic or donor-derived HLA molecules following in vitro polyclonal activation. ELISPOT, Enzyme-linked immunosorbent spot.
Direct Pathway T Cells
T cells with direct alloreactive capacity have been shown to play a predominant role in acute rejection occurring early after transplantation. Mixed lymphocyte reaction (MLR) stands as the traditional and most commonly used method to measure donor HLA class II specific T cell alloreactivity and forms the basis for complementary approaches to further assess the frequency and diversity of the alloreactive T cell repertoire. The MLR is based on culturing patient peripheral blood mononuclear cells (PBMC) or T cells as responder cells with irradiated allogeneic donor cells (stimulator) for 5–7 days (Figure 2A). For an accurate donor-specific response calculation, autologous cells of the patient against irradiated autologous targets, fully HLA-mismatched third-party cells as stimulators, and a non-specific stimulation of patient T cells (e.g., PHA or Concavalin-A) as a positive control should preferentially be included. Approximately 18–24 h before the end of the MLR, cells are pulsed with radioactive thymidine to determine the level of proliferating patient T cells. Early studies have defined the relative donor-specific response rate either as hypo-responsiveness or as hyper-responsiveness in kidney transplant recipients and related these responses to good graft function or acute rejection within the first year after transplantation, respectively (61, 62). However, limited predictive value of the MLR for acute rejection has been reported since (63, 64).
Measuring proliferation alone in MLRs does not give accurate information on the effector functions or the frequency of donor-specific alloreactive cells. While tedious limiting dilution assays can be used to quantify both cytotoxic T lymphocyte precursors and IL-2 producing helper T cells (65, 66), a carboxyfluorescein succinimidyl ester (CFSE)-based MLR can easily help to dissect precursor frequencies of dividing alloreactive CD4 and CD8 T cells. Measuring CFSE dilutions in MLR using flow cytometry provides a better estimation of precursor frequencies, since CFSE dilution indicates the number of times the cells have divided throughout the entire MLR rather than giving a snapshot of proliferation over a period of time in culture as thymidine incorporation does. Macedo et al. showed that CD4 and CD8 T cells proliferated equally in response to allo-stimulation with average frequencies of 4% of total T cells by using a CFSE-MLR in combination with additional cell markers (25). However, precursor frequency calculations should be interpreted carefully because of the potential bystander proliferation of non-alloreactive clones in MLRs.
Both naïve and memory T cells contribute to alloimmune responses (25, 26, 30). Given that memory T cells are primed to respond to alloantigens more rapidly in comparison to their naïve counterparts, Heeger and colleagues developed an enzyme-linked immunosorbent spot (ELISPOT) assay in which IFN-γ producing alloreactive primed/memory T cells could be detected and quantified upon 18–24-h MLRs (Figure 2B) (67, 68). Using the IFN-γ ELISPOT assay, several researchers found a correlation between pre-transplant alloreactive memory T cell frequencies and subsequent acute rejection within the first year of kidney transplantations, independent of HLA antibodies or HLA matching (16, 67–69). These results, in combination with efforts to bypass the need for donor cells inspired its utilization as a screening tool against a panel of allogeneic stimulator cells (panel of reactive T cells-PRT) in pre-transplant risk assessment of kidney transplant recipients, analogous to panel reactive antibody testing (70–72). Using this PRT approach, Poggio et al. showed that 63% of pre-transplant PRT-positive patients experienced acute cellular rejection in a small cohort of 30 transplant recipients (72). However, a recent study performed on 168 consecutive kidney transplant recipients concluded that pre-transplant IFN-γ ELISPOT positivity correlated with biopsy proven acute rejection only when performed in a donor-specific manner and not against a panel of surrogate donors (17). While a clear correlation between donor-specific pre-transplant IFN-γ ELISPOT and acute rejection risk could not be established in a multicenter clinical trial of 176 kidney transplant recipients (73), Crespo et al. found a strong correlation between IFN-γ ELISPOT positive patients and early acute cellular rejections only in a subset of patients not receiving T lymphocyte depleting induction therapy (74). The latter finding is particularly interesting considering that after T lymphocyte depleting therapies, homeostatic proliferation favors memory T cell population. Yet, lower memory T cell frequencies may be due to the effect of other immunosuppressive agents used in combination with T cell depleting agents such as calcineurin inhibitors (75, 76). Moreover, although a harmony on standardization and validation of IFN-γ ELISPOT assays between different laboratories has been shown (77, 78), the IFN-γ ELISPOT approach suffers from predicting outcomes for individual patients despite correlations with outcome at the population level (79).
CD30 is considered to be a marker to identify a subset of alloreactive T cells producing IFN-γ in vitro (80). Several studies showed the pre- and post-transplant prognostic value of serum soluble CD30 (sCD30) levels in predicting graft survival and efficacy of immunosuppressive treatments in kidney transplant recipients (81, 82). More recently, Velasquez et al. have shown the predominant dependence of sCD30 release on memory CD4 T cells in MLR culture supernatants, suggesting that high serum sCD30 levels in kidney waitlist patients could be attributed to active memory T cell responses (83). While this may be an explanation for the significantly lower 3-year graft survival rate in patients with simultaneous pre-transplant DSA and sCD30 positivity in comparison to those with DSA only (84), lack of direct biological link between deleterious DSA and sCD30 as well as the absence of evidence on whether sCD30 release is correlated with donor-specific T cell proliferation render sCD30 to be a non-specific marker of immune activation.
Recently, with the advent of T cell receptor deep sequencing in combination with CFSE-MLR, the size and diversity of the donor-specific T cell repertoire of a particular patient can be monitored before and after transplantation (29, 85). This can be achieved by fluorescence activated cell sorting (FACS) of alloreactive CD4 and CD8 T cells of the patient based on CFSE-MLR, isolating genomic DNA from the divided alloreactive populations as well as from unstimulated patient cells and comparing TCR-beta chain complementarity determining region 3 (CDR3) using high throughput sequencing (Figure 2C). Since each individual T cell clone has a distinct CDR3 sequence, such an approach allows for generation of a “catalog” demonstrating alloreactive clone size and diversity for a patient. Using this approach in a cohort of combined kidney-bone marrow transplant patients, Morris et al. found a decrease in circulating pre-transplant donor-reactive CD4 and CD8 T cell clones in tolerant patients, whereas such a reduction was not observed in non-tolerant patients on immunosuppression (85). The same approach can be used for monitoring changes in frequencies of alloreactive naïve and memory T cells by mapping MLR-expanded alloreactive T cell fractions to the sorted naïve or memory fractions of the unstimulated population (26). However, cell numbers, as well as low frequency clones may be a limiting factor in practice. Even so, TCR-beta CDR3 sequencing stands out to be a highly promising method by which post-transplantation fate of donor-specific direct pathway alloreactive T cell clones can be monitored in comparison to pre-transplant “catalog” of a patient without the need for further functional assays.
Indirect Pathway T Cells
Several studies suggested a role for indirect pathway alloreactive T cells in both acute and chronic allograft rejection of kidney, heart and lung transplant patients using the MLR-based methods originally applied for direct allorecognition (20, 21, 23, 86–90). Since indirect allorecognition is caused by the activation of T cells by processed alloantigens presented as peptides in the context of self MHC class II, properly fractionated cells can theoretically be used as the source of antigens, given the assumption that no intact HLA molecules are remaining (21, 23). Increased indirect pathway responses in kidney transplant recipients experiencing chronic rejection were found when cytoplasmic membrane proteins from donor cells were used to stimulate patient T cells in MLR (21). Using a similar approach in IFN-γ ELISPOT assays, a significant correlation between proteinuria and indirectly activated IFN-γ secreting primed/memory T cells in patients >2 years after kidney transplantation was shown (23). While cellular fragments likely provide a proper representation of the donor alloantigen repertoire, controls should be taken along in order to exclude involvement of direct/semidirect pathway recognition which may activate patient T cells due to residual intact antigens in these donor cell fragment preparations. On the other hand, lack of standardization in preparation of cellular fragments may lead to poor reproducibility. To overcome this issue, synthetic peptides produced in a standardized way, corresponding to polymorphic domains of mismatched donor MHC class I and II as well as peptides deriving from oligomorphic domains can be used. However, one should be aware of the possibility of the recognition of neo-epitopes which do not exist in vivo on peptides by recipient T cells and include peptides from self HLA as controls (91–94). Recently, HLA monomers were suggested as tools to study indirect alloreactivity, but the requirement for very high concentrations of these monomers in order to induce a reproducible indirect T cell response limited their use as the antigen source in this setting (95, 96).
Methods to detect indirect pathway are particularly important since only indirect pathway CD4 T cells can provide help for alloreactive B cells to produce alloantibodies, as shown in animal models (35, 36). On the other hand, currently there is no reliable in vitro assay to detect indirect pathway CD4 T cells mainly due to the problems related to the antigen source used to activate these T cells (specificity) and the low frequency of indirectly recognizing T cells (sensitivity) (97).
HLA-Specific Memory B Cells
Humoral alloimmune responses to mismatched donor HLA can be detected as circulating HLA antibodies or as HLA-specific memory B cells. Serum antibodies are produced by long-lived plasma cells residing in the bone marrow, whereas memory B cells are quiescent cells that continuously circulate between the secondary lymphoid organs and peripheral blood. In kidney transplantation, pre-existence or de novo development of IgG isotype DSA has been clearly shown to be associated with both acute and chronic ABMR and poor graft survival (11, 12, 98). Given that memory B cells may replenish the pool of long-lived plasma cells and may harbor a more diverse repertoire than serum (99), current immunological risk assessment based on detection of circulating HLA antibodies may be incomplete due to the lack of information on the possible presence of donor-reactive memory B cells (53).
Unlike plasma cells that spontaneously produce antibodies, memory B cells need to be stimulated either by re-encountering the same antigen they have seen in primary responses or via bystander activation in a non-antigen specific manner to become antibody producers (54, 100). In order to be detected as antibody secreting cells in vitro, memory B cells need to be polyclonally activated (101). While several protocols exist to activate B cells in vitro, it is critical that the polyclonal activation cocktail does not induce isotype switching in the naïve B cell population allowing for accurate detection of antibodies deriving from only pre-existing memory B cells. These cocktails stimulate B cells either by ligation to the BCR or Toll-like receptors and/or costimulatory molecules in combination with B cell cytokines and growth factors (102, 103). The ability of in vitro activated B cells from alloantigen exposed individuals to secrete HLA-specific antibodies was first demonstrated by our group, paving the way to the development of HLA-specific memory B cell assays (104). In a few studies screening for HLA antibodies in culture supernatants, neat culture supernatants were 10-fold concentrated in order to increase the detectability of the HLA antibodies. A recent method developed by our group in which IgG isotype of antibodies are isolated from culture supernatants resulted in ~20% increase in detectability of HLA-specific memory in alloantigen exposed individuals in comparison to 10-fold concentration (Figure 3A) (52). In a small cohort of 13 alloantigen exposed individuals, we showed that HLA antibody profiles derived from serum and memory B cells were not identical. Unlike the sequencing of BCR and serum IgG in the setting of tetanus toxoid vaccination (49), a broader serum repertoire was the most commonly observed profile, followed by a complete overlap of HLA antibody specificities in serum and culture supernatants. Interestingly, 10% of the HLA antibody specificities were only found in the memory B cell compartment, which may be of clinical relevance. In a cohort of 20 kidney patients transplanted across a DSA barrier we found that concurrent presence of donor-specific memory and serum DSA pre-transplantation was associated with higher 1-year incidence of ABMR and more severe microvascular inflammation in allograft biopsies (19). While supernatant analysis of IgG isolated cultures is an easy and sensitive way of profiling memory B cell derived HLA antibodies in addition to its potential in clinical use, it does not allow for quantification of HLA-specific memory B cells.
One way to quantify HLA-specific memory B cells is based on the ability of BCR to bind to synthetic HLA molecules and can be achieved by flow cytometric analysis upon staining B cells with HLA-tetramers (Figure 3B) (105, 106). Tetramer staining by flow cytometry requires a second step of sorting and culturing to confirm the antibody production capacity of these tetramer positive cells. Considering that not all tetramer positive cells will turn out to be antibody producers, in combination with non-specific binding of BCRs to HLA tetramers, quantification of HLA-specific memory B cells by tetramer positivity alone should be interpreted cautiously.
HLA-ELISPOT assays combine the ability of B cells to produce antibodies upon in vitro polyclonal activation with BCR's ability to bind to synthetic HLA molecules, thereby enables detection and quantification HLA-specific memory B cells (Figure 3C). Importantly, since ELISPOT assays purely rely on the capacity of activated B cells to produce antibodies in vitro, viability of the stimulated cells at the end of pre-culture phase plays an extremely important role in the quality and accuracy of the ELISPOT results. Hence, in contrast to supernatant analysis favoring longer culture periods up to 10–12 days to achieve the highest IgG concentrations in the culture supernatants, pre-culture phase of ELISPOT assays are preferred to be 6–7 days in order to have highest number of viable cells producing antibodies. Following 6–7 days of culturing, these polyclonally activated B cells are transferred to special ELISPOT plates coated with anti-IgG antibodies to capture the IgG isotype of antibodies produced by polyclonally activated memory B cells. In the second phase of the assay (visualization), antibody fingerprints captured on the filter of ELISPOT plates can be visualized as a single spot representative of one HLA antibody producing cell, using either synthetic (monomeric or multimeric) or donor-lysate derived HLA molecules serving as detection matrix (18, 107–110). Since HLA-specific memory B cells assays are not standardized assays such as the commercially available HLA-antibody detection kits, it is critical to include positive and negative controls to assure a certain standard in each phase of ELISPOT assay. In this regard, performing total IgG ELISPOT assays with every HLA-specific memory B cell ELISPOT is necessary in order to confirm that polyclonal activation indeed gave rise to antibody producing cells at the end of the pre-culture phase. Regarding the visualization phase, one such control would be including HLA-specific hybridoma cells to assure that the HLA target used as the detection matrix, regardless of its source, can give rise to a positive signal (111, 112). Similarly, since no HLA-specific antibody production is expected to occur against self, autologous controls using self HLA as the detection matrix can serve as a negative control in ELISPOT assays. Furthermore, when analyzing memory B cell assays, one should take into account the frequency of spots in autologous controls, if any, as well as the total IgG production capacity of the polyclonally activated cells in order to accurately define the frequency HLA-specific memory B cells per IgG producing cells (110). Using ELISPOT methods, increased frequencies of HLA-specific memory B cells in the absence of serum DSA pre-transplantation and at the time of ABMR in kidney transplant patients have been reported (18, 113). While these results demonstrate the clinical relevance of HLA-specific memory B cell testing in addition to serum DSA analysis, complexity of the ELISPOT method in addition to the labor intensity necessary for accurate performance makes clinical utility of HLA-ELISPOT assays difficult. It is important to note that inherent to all antigen specific B cell detection methods performed on peripheral blood samples, HLA-specific memory B cells may not be circulating at the time of sampling and instead can be residing in secondary lymphoid organs, precluding their detectability.
A place for HLA-Specific Memory T and B cell Assays in Pre-transplant Risk Assesment?
Alloreactive immune memory contributes to early allograft rejection that is difficult to block or inhibit, rendering a subset of kidney transplant recipients at risk for developing ABMR or T cell mediated rejections (TCMR) (14). To further improve pre-transplant risk estimation in these patients, it would be appropriate to implement T and B cell memory assays as additional tools.
Donor-specific alloreactive T cells are the central players in allograft rejection and a relatively large fraction of this repertoire consists of memory T cells (29). In this regard, IFN-γ producing alloreactive T cell frequencies of the direct pathway as measured by ELISPOT could be a good assay candidate to be used in practice. However, the actual predictive value per individual patient needs to be determined by well-characterized large scale studies, such as the BIO-DrIM consortium (114). Moreover, the need for donor cells in addition to the fact that IFN-γ ELISPOT can only be used to screen patients for living-donor transplantations due to the time consumed to perform the assay are the obstacles in the way of its use.
Currently, high throughput TCRβ CDR3 sequencing of alloreactive direct pathway T cells selected upon MLR offers the possibility to monitor the changes in the frequency of donor-specific T cell clones after transplantation. Application of this approach in larger cohorts may identify the dominant T cell clones leading to rejection or tolerance, yet still requirement for pre-transplant MLR is still a limitation for clinical use. Nonetheless, modification of the TCRβ CDR3 sequencing for indirect pathway T cells is desired to improve our understanding of the mechanisms involved in chronic allograft rejection. Since indirect allorecognition plays a central role in graft rejection and tolerance, the ability to measure indirect allorecognition accurately is an absolute requirement to understand the evolution of alloimmune responses and aid-in application of tailor-made immunosuppressive approaches.
Recently, high throughput sequencing technology has been applied to monitor changes in the B cell repertoire before and after desensitization treatments in highly sensitized individuals with the aim to be able to distinguish responders to treatment from non-responders however no dominant B cell clone that may influence the response to treatment was found (115). In a recent study involving a small cohort of pediatric kidney transplant recipients, B cell repertoire was longitudinally assessed using high throughput BCR sequencing. Patients who experienced rejection had higher B cell diversity before transplantation which decreased post transplantation in comparison to those who did not progress to rejection or chronic injury (116). While BCR heavy chain variable region CDR3 analyses in these studies provide information on the bulk B cell repertoire, HLA-specific IgG and memory B cell repertoires can also be delineated using a similar approach in combination with HLA-specific B cell and antibody sorting. An ideal HLA-specific memory B cell assay, however, should be easily applicable in all HLA laboratories in a standardized way and should preferentially be compatible to current practice of serum HLA antibody analysis.
Conclusion
Currently, no single assay is capable of capturing all aspects of alloreactive cellular and humoral immune responses. Therefore, combinations of different assays in addition to current practice should be used to have a complete picture of the alloimmune response. Inherent to all the above-mentioned assays sampling peripheral blood, responses from circulating lymphocytes detected in these assays may not be reflective of those infiltrating the donor organs or some responses may be undetected because donor-specific cells might be residing within the graft or in secondary lymphoid organs. Nevertheless, in order to better understand the predictive values of these assays for individual patients, studies with larger cohorts are warranted.
Author Contributions
GK, FC, and SH wrote the manuscript. All authors contributed to the article and approved the submitted version.
Funding
GK was funded by the Foundation National Reference Center for Histocompatibility Testing.
Conflict of Interest
The authors declare that the research was conducted in the absence of any commercial or financial relationships that could be construed as a potential conflict of interest.
References
1. Crotty S, Ahmed R. Immunological memory in humans. Semin Immunol. (2004) 16:197–203. doi: 10.1016/j.smim.2004.02.008
2. Gray D. A role for antigen in the maintenance of immunological memory. Nat Rev Immunol. (2002) 2:60–5. doi: 10.1038/nri706
3. Amanna IJ, Carlson NE, Slifka MK. Duration of humoral immunity to common viral and vaccine antigens. N Engl J Med. (2007) 357:1903–15. doi: 10.1056/NEJMoa066092
4. Hammarlund E, Lewis MW, Hansen SG, Strelow LI, Nelson JA, Sexton GJ, et al. Duration of antiviral immunity after smallpox vaccination. Nat Med. (2003) 9:1131–7. doi: 10.1038/nm917
5. Netea MG, Joosten LA, Latz E, Mills KH, Natoli G, Stunnenberg HG, et al. Trained immunity: a program of innate immune memory in health and disease. Science. (2016) 352:aaf1098. doi: 10.1126/science.aaf1098
6. Burrows SR, Khanna R, Silins SL, Moss DJ. The influence of antiviral T-cell responses on the alloreactive repertoire. Immunol Today. (1999) 20:203–7. doi: 10.1016/S0167-5699(98)01429-7
7. Amir AL, D'Orsogna LJ, Roelen DL, van Loenen MM, Hagedoorn RS, de Boer R, et al. Allo-HLA reactivity of virus-specific memory T cells is common. Blood. (2010) 115:3146–57. doi: 10.1182/blood-2009-07-234906
8. Zhu L, Fu C, Lin K, Wang Z, Guo H, Chen S, et al. Patterns of early rejection in renal retransplantation: a single-center experience. J Immunol Res. (2016) 2016:2697860. doi: 10.1155/2016/2697860
9. Sethi S, Choi J, Toyoda M, Vo A, Peng A, Jordan SC. Desensitization: overcoming the immunologic barriers to transplantation. J Immunol Res. (2017) 2017:6804678. doi: 10.1155/2017/6804678
10. Heidt S, Haasnoot GW, Witvliet MD, van der Linden-van Oevelen MJH, Kamburova EG, Wisse BW, et al. Allocation to highly sensitized patients based on acceptable mismatches results in low rejection rates comparable to nonsensitized patients. Am J Transplant. (2019) 19:2926–33. doi: 10.1111/ajt.15486
11. Lefaucheur C, Loupy A, Hill GS, Andrade J, Nochy D, Antoine C, et al. Preexisting donor-specific HLA antibodies predict outcome in kidney transplantation. J Am Soc Nephrol. (2010) 21:1398–406. doi: 10.1681/ASN.2009101065
12. Wehmeier C, Honger G, Cun H, Amico P, Hirt-Minkowski P, Georgalis A, et al. Donor specificity but not broadness of sensitization is associated with antibody-mediated rejection and graft loss in renal allograft recipients. Am J Transplant. (2017) 17:2092–102. doi: 10.1111/ajt.14247
13. Tait BD, Susal C, Gebel HM, Nickerson PW, Zachary AA, Claas FH, et al. Consensus guidelines on the testing and clinical management issues associated with HLA and non-HLA antibodies in transplantation. Transplantation. (2013) 95:19–47. doi: 10.1097/TP.0b013e31827a19cc
14. Tambur AR, Campbell P, Claas FH, Feng S, Gebel HM, Jackson AM, et al. Sensitization in Transplantation: Assessment of Risk (STAR) 2017. Working group meeting report. Am J Transplant. (2018) 18:1604–14. doi: 10.1111/ajt.14752
15. Hricik DE, Rodriguez V, Riley J, Bryan K, Tary-Lehmann M, Greenspan N, et al. Enzyme linked immunosorbent spot (ELISPOT) assay for interferon-gamma independently predicts renal function in kidney transplant recipients. Am J Transplant. (2003) 3:878–84. doi: 10.1034/j.1600-6143.2003.00132.x
16. Augustine JJ, Siu DS, Clemente MJ, Schulak JA, Heeger PS, Hricik DE. Pre-transplant IFN-gamma ELISPOTs are associated with post-transplant renal function in African American renal transplant recipients. Am J Transplant. (2005) 5:1971–5. doi: 10.1111/j.1600-6143.2005.00958.x
17. Gandolfini I, Crespo E, Baweja M, Jarque M, Donadei C, Luque S, et al. Impact of preformed T-cell alloreactivity by means of donor-specific and panel of reactive T cells (PRT) ELISPOT in kidney transplantation. PLoS ONE. (2018) 13:e0200696. doi: 10.1371/journal.pone.0200696
18. Lucia M, Luque S, Crespo E, Melilli E, Cruzado JM, Martorell J, et al. Preformed circulating HLA-specific memory B cells predict high risk of humoral rejection in kidney transplantation. Kidney Int. (2015) 88:874–87. doi: 10.1038/ki.2015.205
19. Wehmeier C, Karahan GE, Krop J, de Vaal Y, Langerak-Langerak J, Binet I, et al. Donor-specific B cell memory in alloimmunized kidney transplant recipients - first clinical application of a novel method. Transplantation. (2019) 70:123–8. doi: 10.1097/TP.0000000000002909
20. Hornick PI, Mason PD, Baker RJ, Hernandez-Fuentes M, Frasca L, Lombardi G, et al. Significant frequencies of T cells with indirect anti-donor specificity in heart graft recipients with chronic rejection. Circulation. (2000) 101:2405–10. doi: 10.1161/01.CIR.101.20.2405
21. Baker RJ, Hernandez-Fuentes MP, Brookes PA, Chaudhry AN, Cook HT, Lechler RI. Loss of direct and maintenance of indirect alloresponses in renal allograft recipients: implications for the pathogenesis of chronic allograft nephropathy. J Immunol. (2001) 167:7199–206. doi: 10.4049/jimmunol.167.12.7199
22. Ali JM, Bolton EM, Bradley JA, Pettigrew GJ. Allorecognition pathways in transplant rejection and tolerance. Transplantation. (2013) 96:681–8. doi: 10.1097/TP.0b013e31829853ce
23. Bestard O, Nickel P, Cruzado JM, Schoenemann C, Boenisch O, Sefrin A, et al. Circulating alloreactive T cells correlate with graft function in longstanding renal transplant recipients. J Am Soc Nephrol. (2008) 19:1419–29. doi: 10.1681/ASN.2007050539
24. Baker RJ, Hernandez-Fuentes MP, Brookes PA, Chaudhry AN, Lechler RI. The role of the allograft in the induction of donor-specific T cell hyporesponsiveness. Transplantation. (2001) 72:480–5. doi: 10.1097/00007890-200108150-00020
25. Macedo C, Orkis EA, Popescu I, Elinoff BD, Zeevi A, Shapiro R, et al. Contribution of naive and memory T-cell populations to the human alloimmune response. Am J Transplant. (2009) 9:2057–66. doi: 10.1111/j.1600-6143.2009.02742.x
26. Podesta MA, Binder C, Sellberg F, DeWolf S, Shonts B, Ho SH, et al. Siplizumab selectively depletes effector memory T cells and promotes a relative expansion of alloreactive regulatory T cells in vitro. Am J Transplant. (2020) 20:88–100. doi: 10.1111/ajt.15533
27. Suchin EJ, Langmuir PB, Palmer E, Sayegh MH, Wells AD, Turka LA. Quantifying the frequency of alloreactive T cells in vivo: new answers to an old question. J Immunol. (2001) 166:973–81. doi: 10.4049/jimmunol.166.2.973
28. Welsh RM, Selin LK. No one is naive: the significance of heterologous T-cell immunity. Nat Rev Immunol. (2002) 2:417–26. doi: 10.1038/nri820
29. Emerson RO, Mathew JM, Konieczna IM, Robins HS, Leventhal JR. Defining the alloreactive T cell repertoire using high-throughput sequencing of mixed lymphocyte reaction culture. PLoS ONE. (2014) 9:e111943. doi: 10.1371/journal.pone.0111943
30. Lombardi G, Sidhu S, Daly M, Batchelor JR, Makgoba W, Lechler RI. Are primary alloresponses truly primary? Int Immunol. (1990) 2:9–13. doi: 10.1093/intimm/2.1.9
31. Burrows SR, Khanna R, Burrows JM, Moss DJ. An alloresponse in humans is dominated by cytotoxic T lymphocytes (CTL) cross-reactive with a single Epstein-Barr virus CTL epitope: implications for graft-versus-host disease. J Exp Med. (1994) 179:1155–61. doi: 10.1084/jem.179.4.1155
32. Heutinck KM, Yong SL, Tonneijck L, van den Heuvel H, van der Weerd NC, van der Pant KA, et al. Virus-specific CD8(+) T cells cross-reactive to donor-alloantigen are transiently present in the circulation of kidney transplant recipients infected with CMV and/or EBV. Am J Transplant. (2016) 16:1480–91. doi: 10.1111/ajt.13618
33. Mifsud NA, Nguyen TH, Tait BD, Kotsimbos TC. Quantitative and functional diversity of cross-reactive EBV-specific CD8+ T cells in a longitudinal study cohort of lung transplant recipients. Transplantation. (2010) 90:1439–49. doi: 10.1097/TP.0b013e3181ff4ff3
34. Steele DJ, Laufer TM, Smiley ST, Ando Y, Grusby MJ, Glimcher LH, et al. Two levels of help for B cell alloantibody production. J Exp Med. (1996) 183:699–703. doi: 10.1084/jem.183.2.699
35. Conlon TM, Saeb-Parsy K, Cole JL, Motallebzadeh R, Qureshi MS, Rehakova S, et al. Germinal center alloantibody responses are mediated exclusively by indirect-pathway CD4 T follicular helper cells. J Immunol. (2012) 188:2643–52. doi: 10.4049/jimmunol.1102830
36. Taylor AL, Negus SL, Negus M, Bolton EM, Bradley JA, Pettigrew GJ. Pathways of helper CD4 T cell allorecognition in generating alloantibody and CD8 T cell alloimmunity. Transplantation. (2007) 83:931–7. doi: 10.1097/01.tp.0000257960.07783.e3
37. Valujskikh A, Lantz O, Celli S, Matzinger P, Heeger PS. Cross-primed CD8(+) T cells mediate graft rejection via a distinct effector pathway. Nat Immunol. (2002) 3:844–51. doi: 10.1038/ni831
38. Valujskikh A, Zhang Q, Heeger PS. CD8 T cells specific for a donor-derived, self-restricted transplant antigen are nonpathogenic bystanders after vascularized heart transplantation in mice. J Immunol. (2006) 176:2190–6. doi: 10.4049/jimmunol.176.4.2190
39. Gould DS, Auchincloss H Jr. Direct and indirect recognition: the role of MHC antigens in graft rejection. Immunol Today. (1999) 20:77–82. doi: 10.1016/S0167-5699(98)01394-2
40. Russo V, Zhou D, Sartirana C, Rovere P, Villa A, Rossini S, et al. Acquisition of intact allogeneic human leukocyte antigen molecules by human dendritic cells. Blood. (2000) 95:3473–7. doi: 10.1182/blood.V95.11.3473.011k06_3473_3477
41. Marino J, Babiker-Mohamed MH, Crosby-Bertorini P, Paster JT, LeGuern C, Germana S, et al. Donor exosomes rather than passenger leukocytes initiate alloreactive T cell responses after transplantation. Sci Immunol. (2016) 1:aaf8759. doi: 10.1126/sciimmunol.aaf8759
42. Lefaucheur C, Loupy A. Antibody-mediated rejection of solid-organ allografts. N Engl J Med. (2018) 379:2580–2. doi: 10.1056/NEJMc1813976
43. Noelle RJ, Snow EC. Cognate interactions between helper T cells and B cells. Immunol Today. (1990) 11:361–8. doi: 10.1016/0167-5699(90)90142-V
44. MacLennan IC, Toellner KM, Cunningham AF, Serre K, Sze DM, Zuniga E, et al. Extrafollicular antibody responses. Immunol Rev. (2003) 194:8–18. doi: 10.1034/j.1600-065X.2003.00058.x
45. Kurosaki T, Kometani K, Ise W. Memory B cells. Nat Rev Immunol. (2015) 15:149–59. doi: 10.1038/nri3802
46. Crotty S. Follicular helper CD4 T cells (TFH). Annu Rev Immunol. (2011) 29:621–63. doi: 10.1146/annurev-immunol-031210-101400
47. Victora GD, Nussenzweig MC. Germinal centers. Annu Rev Immunol. (2012) 30:429–57. doi: 10.1146/annurev-immunol-020711-075032
48. Purtha WE, Tedder TF, Johnson S, Bhattacharya D, Diamond MS. Memory B cells, but not long-lived plasma cells, possess antigen specificities for viral escape mutants. J Exp Med. (2011) 208:2599–606. doi: 10.1084/jem.20110740
49. Lavinder JJ, Wine Y, Giesecke C, Ippolito GC, Horton AP, Lungu OI, et al. Identification and characterization of the constituent human serum antibodies elicited by vaccination. Proc Natl Acad Sci USA. (2014) 111:2259–64. doi: 10.1073/pnas.1317793111
50. Han M, Rogers J, Lavingia B, Stastny P. Peripheral blood B cells producing donor-specific HLA antibodies in vitro. Hum Immunol. (2009) 70:29–34. doi: 10.1016/j.humimm.2008.10.013
51. Snanoudj R, Claas FH, Heidt S, Legendre C, Chatenoud L, Candon S. Restricted specificity of peripheral alloreactive memory B cells in HLA-sensitized patients awaiting a kidney transplant. Kidney Int. (2015) 87:1230–40. doi: 10.1038/ki.2014.390
52. Karahan GE, Krop J, Wehmeier C, de Vaal YJH, Langerak-Langerak J, Roelen DL, et al. An easy and sensitive method to profile the antibody specificities of HLA-specific memory B cells. Transplantation. (2019) 103:716–23. doi: 10.1097/TP.0000000000002516
53. Karahan GE, Claas FH, Heidt S. Detecting the humoral alloimmune response: we need more than serum antibody screening. Transplantation. (2015) 99:908–15. doi: 10.1097/TP.0000000000000724
54. D'Orsogna L, van den Heuvel H, van Kooten C, Heidt S, Claas FHJ. Infectious pathogens may trigger specific allo-HLA reactivity via multiple mechanisms. Immunogenetics. (2017) 69:631–41. doi: 10.1007/s00251-017-0989-3
55. Heidt S, Feltkamp MC, Karahan GE, de Brouwer CS, Langerak-Langerak J, Mulder A, et al. No evidence for cross-reactivity of virus-specific antibodies with HLA allo-antigens. Transplantation. (2018) 102:1844–9. doi: 10.1097/TP.0000000000002369
56. Alsughayyir J, Chhabra M, Qureshi MS, Mallik M, Ali JM, Gamper I, et al. Relative frequencies of alloantigen-specific helper CD4 T cells and B cells determine mode of antibody-mediated allograft rejection. Front Immunol. (2018) 9:3039. doi: 10.3389/fimmu.2018.03039
57. Chhabra M, Alsughayyir J, Qureshi MS, Mallik M, Ali JM, Gamper I, et al. Germinal center alloantibody responses mediate progression of chronic allograft injury. Front Immunol. (2018) 9:3038. doi: 10.3389/fimmu.2018.03038
58. Conlon TM, Cole JL, Motallebzadeh R, Harper I, Callaghan CJ, Bolton EM, et al. Unlinked memory helper responses promote long-lasting humoral alloimmunity. J Immunol. (2012) 189:5703–12. doi: 10.4049/jimmunol.1202257
59. Rabant M, Gorbacheva V, Fan R, Yu H, Valujskikh A. CD40-independent help by memory CD4 T cells induces pathogenic alloantibody but does not lead to long-lasting humoral immunity. Am J Transplant. (2013) 13:2831–41. doi: 10.1111/ajt.12432
60. Gorbacheva V, Fan R, Wang X, Baldwin WM 3rd, Fairchild RL, Valujskikh A. IFN-gamma production by memory helper T cells is required for CD40-independent alloantibody responses. J Immunol. (2015) 194:1347–56. doi: 10.4049/jimmunol.1401573
61. Ghobrial II, Morris AG, Booth LJ. Clinical significance of in vitro donor-specific hyporesponsiveness in renal allograft recipients as demonstrated by the MLR. Transpl Int. (1994) 7:420–7. doi: 10.1111/j.1432-2277.1994.tb01261.x
62. Harmon WE, Parkman R, Lavin PT, Grupe WE, Ingelfinger JR, Yunis EJ, et al. Comparison of cell-mediated lympholysis and mixed lymphocyte culture in the immunologic evaluation for renal transplantation. J Immunol. (1982) 129:1573–7.
63. van Besouw NM, van der Mast BJ, de Kuiper P, Smak Gregoor PJ, Vaessen LM, IJzermans JN, et al. Donor-specific T-cell reactivity identifies kidney transplant patients in whom immunosuppressive therapy can be safely reduced. Transplantation. (2000) 70:136–43.
64. van der Mast BJ, van Besouw NM, de Kuiper P, Vaessen LM, Gregoor PJ, IJzermans JN, et al. Pretransplant donor-specific helper T cell reactivity as a tool for tailoring the individual need for immunosuppression. Transplantation. (2001) 72:873–80. doi: 10.1097/00007890-200109150-00023
65. Roelen DL, Stobbe I, Young NT, van Bree SP, Doxiadis II, Oudshoorn M, et al. Permissible and immunogenic HLA-A mismatches: cytotoxic T-cell precursor frequencies reflect graft survival data. Hum Immunol. (2001) 62:661–7. doi: 10.1016/S0198-8859(01)00263-4
66. Beik AI, Higgins RM, Lam FT, Morris AG. Clinical significance of selective decline of donor-reactive IL-2-producing T lymphocytes after renal transplantation. Transpl Immunol. (1997) 5:89–96. doi: 10.1016/S0966-3274(97)80048-2
67. Heeger PS, Greenspan NS, Kuhlenschmidt S, Dejelo C, Hricik DE, Schulak JA, et al. Pretransplant frequency of donor-specific, IFN-gamma-producing lymphocytes is a manifestation of immunologic memory and correlates with the risk of posttransplant rejection episodes. J Immunol. (1999) 163:2267–75.
68. Gebauer BS, Hricik DE, Atallah A, Bryan K, Riley J, Tary-Lehmann M, et al. Evolution of the enzyme-linked immunosorbent spot assay for post-transplant alloreactivity as a potentially useful immune monitoring tool. Am J Transplant. (2002) 2:857–66. doi: 10.1034/j.1600-6143.2002.20908.x
69. Nickel P, Presber F, Bold G, Biti D, Schonemann C, Tullius SG, et al. Enzyme-linked immunosorbent spot assay for donor-reactive interferon-gamma-producing cells identifies T-cell presensitization and correlates with graft function at 6 and 12 months in renal-transplant recipients. Transplantation. (2004) 78:1640–6. doi: 10.1097/01.TP.0000144057.31799.6A
70. Poggio ED, Clemente M, Hricik DE, Heeger PS. Panel of reactive T cells as a measurement of primed cellular alloimmunity in kidney transplant candidates. J Am Soc Nephrol. (2006) 17:564–72. doi: 10.1681/ASN.2005030293
71. Andree H, Nickel P, Nasiadko C, Hammer MH, Schonemann C, Pruss A, et al. Identification of dialysis patients with panel-reactive memory T cells before kidney transplantation using an allogeneic cell bank. J Am Soc Nephrol. (2006) 17:573–80. doi: 10.1681/ASN.2005030299
72. Poggio ED, Augustine JJ, Clemente M, Danzig JM, Volokh N, Zand MS, et al. Pretransplant cellular alloimmunity as assessed by a panel of reactive T cells assay correlates with acute renal graft rejection. Transplantation. (2007) 83:847–52. doi: 10.1097/01.tp.0000258730.75137.39
73. Hricik DE, Augustine J, Nickerson P, Formica RN, Poggio ED, Rush D, et al. Interferon gamma ELISPOT testing as a risk-stratifying biomarker for kidney transplant injury: results from the CTOT-01 multicenter study. Am J Transplant. (2015) 15:3166–73. doi: 10.1111/ajt.13401
74. Crespo E, Lucia M, Cruzado JM, Luque S, Melilli E, Manonelles A, et al. Pre-transplant donor-specific T-cell alloreactivity is strongly associated with early acute cellular rejection in kidney transplant recipients not receiving T-cell depleting induction therapy. PLoS ONE. (2015) 10:e0117618. doi: 10.1371/journal.pone.0117618
75. Pearl JP, Parris J, Hale DA, Hoffmann SC, Bernstein WB, McCoy KL, et al. Immunocompetent T-cells with a memory-like phenotype are the dominant cell type following antibody-mediated T-cell depletion. Am J Transplant. (2005) 5:465–74. doi: 10.1111/j.1600-6143.2005.00759.x
76. Cherkassky L, Lanning M, Lalli PN, Czerr J, Siegel H, Danziger-Isakov L, et al. Evaluation of alloreactivity in kidney transplant recipients treated with antithymocyte globulin versus IL-2 receptor blocker. Am J Transplant. (2011) 11:1388–96. doi: 10.1111/j.1600-6143.2011.03540.x
77. Bestard O, Crespo E, Stein M, Lucia M, Roelen DL, de Vaal YJ, et al. Cross-validation of IFN-gamma Elispot assay for measuring alloreactive memory/effector T cell responses in renal transplant recipients. Am J Transplant. (2013) 13:1880–90. doi: 10.1111/ajt.12285
78. Ashoor I, Najafian N, Korin Y, Reed EF, Mohanakumar T, Ikle D, et al. Standardization and cross validation of alloreactive IFNgamma ELISPOT assays within the clinical trials in organ transplantation consortium. Am J Transplant. (2013) 13:1871–9. doi: 10.1111/ajt.12286
79. Montero N, Farouk S, Gandolfini I, Crespo E, Jarque M, Meneghini M, et al. Pretransplant donor-specific IFNgamma ELISPOT as a predictor of graft rejection: a diagnostic test accuracy meta-analysis. Transplant Direct. (2019) 5:e451. doi: 10.1097/TXD.0000000000000886
80. Martinez OM, Villanueva J, Abtahi S, Beatty PR, Esquivel CO, Krams SM. CD30 expression identifies a functional alloreactive human T-lymphocyte subset. Transplantation. (1998) 65:1240–7. doi: 10.1097/00007890-199805150-00016
81. Süsal C, Pelzl S, Dohler B, Opelz G. Identification of highly responsive kidney transplant recipients using pretransplant soluble CD30. J Am Soc Nephrol. (2002) 13:1650–6. doi: 10.1097/01.ASN.0000014256.75920.5B
82. Weimer R, Susal C, Yildiz S, Staak A, Pelzl S, Renner F, et al. Post-transplant sCD30 and neopterin as predictors of chronic allograft nephropathy: impact of different immunosuppressive regimens. Am J Transplant. (2006) 6:1865–74. doi: 10.1111/j.1600-6143.2006.01407.x
83. Velasquez SY, Garcia LF, Opelz G, Alvarez CM, Susal C. Release of soluble CD30 after allogeneic stimulation is mediated by memory T cells and regulated by IFN-gamma and IL-2. Transplantation. (2013) 96:154–61. doi: 10.1097/TP.0b013e318296fd69
84. Susal C, Dohler B, Ruhenstroth A, Morath C, Slavcev A, Fehr T, et al. Donor-specific antibodies require preactivated immune system to harm renal transplant. EBioMedicine. (2016) 9:366–71. doi: 10.1016/j.ebiom.2016.06.006
85. Morris H, DeWolf S, Robins H, Sprangers B, LoCascio SA, Shonts BA, et al. Tracking donor-reactive T cells: evidence for clonal deletion in tolerant kidney transplant patients. Sci Transl Med. (2015) 7:272ra10. doi: 10.1126/scitranslmed.3010760
86. Ciubotariu R, Liu Z, Colovai AI, Ho E, Itescu S, Ravalli S, et al. Persistent allopeptide reactivity and epitope spreading in chronic rejection of organ allografts. J Clin Invest. (1998) 101:398–405. doi: 10.1172/JCI1117
87. Stanford RE, Ahmed S, Hodson M, Banner NR, Rose ML. A role for indirect allorecognition in lung transplant recipients with obliterative bronchiolitis. Am J Transplant. (2003) 3:736–42. doi: 10.1034/j.1600-6143.2003.00142.x
88. Liu Z, Colovai AI, Tugulea S, Reed EF, Fisher PE, Mancini D, et al. Indirect recognition of donor HLA-DR peptides in organ allograft rejection. J Clin Invest. (1996) 98:1150–7. doi: 10.1172/JCI118898
89. Vella JP, Spadafora-Ferreira M, Murphy B, Alexander SI, Harmon W, Carpenter CB, et al. Indirect allorecognition of major histocompatibility complex allopeptides in human renal transplant recipients with chronic graft dysfunction. Transplantation. (1997) 64:795–800. doi: 10.1097/00007890-199709270-00001
90. Reznik SI, Jaramillo A, SivaSai KS, Womer KL, Sayegh MH, Trulock EP, et al. Indirect allorecognition of mismatched donor HLA class II peptides in lung transplant recipients with bronchiolitis obliterans syndrome. Am J Transplant. (2001) 1:228–35. doi: 10.1034/j.1600-6143.2001.001003228.x
91. Hanvesakul R, Maillere B, Briggs D, Baker R, Larche M, Ball S. Indirect recognition of T-cell epitopes derived from the alpha 3 and transmembrane domain of HLA-A2. Am J Transplant. (2007) 7:1148–57. doi: 10.1111/j.1600-6143.2007.01743.x
92. Stegmann S, Muller A, Zavazava N. Synthetic HLA-A2 derived peptides are recognized and presented in renal graft recipients. Hum Immunol. (2000) 61:1363–9. doi: 10.1016/S0198-8859(00)00215-9
93. Najafian N, Salama AD, Fedoseyeva EV, Benichou G, Sayegh MH. Enzyme-linked immunosorbent spot assay analysis of peripheral blood lymphocyte reactivity to donor HLA-DR peptides: potential novel assay for prediction of outcomes for renal transplant recipients. J Am Soc Nephrol. (2002) 13:252–9.
94. Smith HJ, Hanvesakul R, Bentall A, Shabir S, Morgan MD, Briggs D, et al. T lymphocyte responses to nonpolymorphic HLA-derived peptides are associated with chronic renal allograft dysfunction. Transplantation. (2011) 91:279–86. doi: 10.1097/TP.0b013e318203862d
95. Breman E, Ruben JM, Franken KL, Heemskerk MH, Roelen DL, Claas FH, et al. Uptake of HLA alloantigens via CD89 and CD206 does not enhance antigen presentation by indirect allorecognition. J Immunol Res. (2016) 2016:4215684. doi: 10.1155/2016/4215684
96. Breman E, van Miert PP, van der Steen DM, Heemskerk MH, Doxiadis II, Roelen D, et al. HLA monomers as a tool to monitor indirect allorecognition. Transplantation. (2014) 97:1119–27. doi: 10.1097/TP.0000000000000113
97. Waanders MM, Heidt S, Koekkoek KM, Zoet YM, Doxiadis II, Amir A, et al. Monitoring of indirect allorecognition: wishful thinking or solid data? Tissue Antigens. (2008) 71:1–15. doi: 10.1111/j.1399-0039.2007.00979.x
98. Mohan S, Palanisamy A, Tsapepas D, Tanriover B, Crew RJ, Dube G, et al. Donor-specific antibodies adversely affect kidney allograft outcomes. J Am Soc Nephrol. (2012) 23:2061–71. doi: 10.1681/ASN.2012070664
99. Lavinder JJ, Horton AP, Georgiou G, Ippolito GC. Next-generation sequencing and protein mass spectrometry for the comprehensive analysis of human cellular and serum antibody repertoires. Curr Opin Chem Biol. (2015) 24:112–20. doi: 10.1016/j.cbpa.2014.11.007
100. Amanna IJ, Slifka MK. Mechanisms that determine plasma cell lifespan and the duration of humoral immunity. Immunol Rev. (2010) 236:125–38. doi: 10.1111/j.1600-065X.2010.00912.x
101. Bernasconi NL, Traggiai E, Lanzavecchia A. Maintenance of serological memory by polyclonal activation of human memory B cells. Science. (2002) 298:2199–202. doi: 10.1126/science.1076071
102. Heidt S, Roelen DL, Eijsink C, van Kooten C, Claas FH, Mulder A. Effects of immunosuppressive drugs on purified human B cells: evidence supporting the use of MMF and rapamycin. Transplantation. (2008) 86:1292–300. doi: 10.1097/TP.0b013e3181874a36
103. Karahan GE, Eikmans M, Anholts JD, Claas FH, Heidt S. Polyclonal B cell activation for accurate analysis of pre-existing antigen-specific memory B cells. Clin Exp Immunol. (2014) 177:333–40. doi: 10.1111/cei.12305
104. Mulder A, Kardol MJ, Kamp J, Uit Het Broek C, Schreuder GM, Doxiadis, et al. Determination of the frequency of HLA antibody secreting B-lymphocytes in alloantigen sensitized individuals. Clin Expe Immunol. (2001) 124:9–15. doi: 10.1046/j.1365-2249.2001.01497.x
105. Mulder A, Eijsink C, Kardol MJ, Franke-van Dijk ME, van der Burg SH, Kester M, et al. Identification, isolation, and culture of HLA-A2-specific B lymphocytes using MHC class I tetramers. J Immunol. (2003) 171:6599–603. doi: 10.4049/jimmunol.171.12.6599
106. Zachary AA, Kopchaliiska D, Montgomery RA, Leffell MS. HLA-specific B cells: I. A method for their detection, quantification, and isolation using HLA tetramers. Transplantation. (2007) 83:982–8. doi: 10.1097/01.tp.0000259017.32857.99
107. Heidt S, Roelen DL, de Vaal YJ, Kester MG, Eijsink C, Thomas S, et al. A novel ELISPOT assay to quantify HLA-specific B cells in HLA-immunized individuals. Am J Transplant. (2012) 12:1469–78. doi: 10.1111/j.1600-6143.2011.03982.x
108. Karahan GE, de Vaal YJ, Roelen DL, Buchli R, Claas FH, Heidt S. Quantification of HLA class II-specific memory B cells in HLA-sensitized individuals. Hum Immunol. (2015) 76:129–36. doi: 10.1016/j.humimm.2015.01.014
109. Luque S, Lucia M, Crespo E, Jarque M, Grinyo JM, Bestard O. A multicolour HLA-specific B-cell FluoroSpot assay to functionally track circulating HLA-specific memory B cells. J Immunol Methods. (2018) 462:23–33. doi: 10.1016/j.jim.2018.07.011
110. Karahan GE, de Vaal YJ, Krop J, Wehmeier C, Roelen DL, Claas FH, et al. A memory B cell crossmatch assay for quantification of donor-specific memory B cells in the peripheral blood of HLA-immunized individuals. Am J Transplant. (2017) 17:2617–26. doi: 10.1111/ajt.14293
111. Duquesnoy RJ, Marrari M, Tambur AR, Mulder A, Sousa LC, da Silva AS, et al. First report on the antibody verification of HLA-DR, HLA-DQ and HLA-DP epitopes recorded in the HLA Epitope Registry. Hum Immunol. (2014) 75:1097–103. doi: 10.1016/j.humimm.2014.09.012
112. Mulder A, Kardol MJ, Arn JS, Eijsink C, Franke ME, Schreuder GM, et al. Human monoclonal HLA antibodies reveal interspecies crossreactive swine MHC class I epitopes relevant for xenotransplantation. Mol Immunol. (2010) 47:809–15. doi: 10.1016/j.molimm.2009.10.004
113. Luque S, Lucia M, Melilli E, Lefaucheur C, Crespo M, Loupy A, et al. Value of monitoring circulating donor-reactive memory B cells to characterize antibody-mediated rejection after kidney transplantation. Am J Transplant. (2018) 19:368–80. doi: 10.1111/ajt.15055
114. Weber S, Pietzsch M, Bestard O, Grinyo J, Viklicky O, Reinke P. Investigating the health-economic profiles of biomarker-driven immunosuppresion (BIO-DrIM) following solid organ transplantation. Advanc Precis Med. (2016) 1:66–77. doi: 10.18063/APM.2016.01.004
115. Beausang JF, Fan HC, Sit R, Hutchins MU, Jirage K, Curtis R, et al. B cell repertoires in HLA-sensitized kidney transplant candidates undergoing desensitization therapy. J Transl Med. (2017) 15:9. doi: 10.1186/s12967-017-1118-7
Keywords: HLA-specific memory, alloantibodies, alloimmune response, T cell-mediated rejection (TCMR), antibody-mediated rejection (ABMR)
Citation: Karahan GE, Claas FHJ and Heidt S (2020) Pre-existing Alloreactive T and B Cells and Their Possible Relevance for Pre-transplant Risk Estimation in Kidney Transplant Recipients. Front. Med. 7:340. doi: 10.3389/fmed.2020.00340
Received: 14 April 2020; Accepted: 08 June 2020;
Published: 21 July 2020.
Edited by:
Gurvinder Kaur, All India Institute of Medical Sciences, IndiaReviewed by:
Burkhard Tönshoff, Heidelberg University Hospital, GermanyFlorian Grahammer, University Medical Center Hamburg-Eppendorf, Germany
Copyright © 2020 Karahan, Claas and Heidt. This is an open-access article distributed under the terms of the Creative Commons Attribution License (CC BY). The use, distribution or reproduction in other forums is permitted, provided the original author(s) and the copyright owner(s) are credited and that the original publication in this journal is cited, in accordance with accepted academic practice. No use, distribution or reproduction is permitted which does not comply with these terms.
*Correspondence: Gonca E. Karahan, Zy5lLmthcmFoYW5AbHVtYy5ubA==