- 1Division of Experimental Allergy and Immunodermatology, Faculty of Medicine and Health Sciences, University of Oldenburg, Oldenburg, Germany
- 2Division of Immunology, Faculty of Medicine and Health Sciences, University of Oldenburg, Oldenburg, Germany
- 3Department of Medicine 1, University Hospital Erlangen and Friedrich-Alexander-University Erlangen-Nürnberg, Erlangen, Germany
- 4Department of Dermatology, Heinrich-Heine-University of Düsseldorf, Düsseldorf, Germany
- 5University Clinic of Dermatology and Allergy, Oldenburg Clinic, Oldenburg, Germany
Pruritus is a common, but very challenging symptom with a wide diversity of underlying causes like dermatological, systemic, neurological and psychiatric diseases. In dermatology, pruritus is the most frequent symptom both in its acute and chronic form (over 6 weeks in duration). Treatment of chronic pruritus often remains challenging. Affected patients who suffer from moderate to severe pruritus have a significantly reduced quality of life. The underlying physiology of pruritus is very complex, involving a diverse network of components in the skin including resident cells such as keratinocytes and sensory neurons as well as transiently infiltrating cells such as certain immune cells. Previous research has established that there is a significant crosstalk among the stratum corneum, nerve fibers and various immune cells, such as keratinocytes, T cells, basophils, eosinophils and mast cells. In this regard, interactions between receptors on cutaneous and spinal neurons or on different immune cells play an important role in the processing of signals which are important for the transmission of pruritus. In this review, we discuss the role of various receptors involved in pruritus and inflammation, such as TRPV1 and TRPA1, IL-31RA and OSMR, TSLPR, PAR-2, NK1R, H1R and H4R, MRGPRs as well as TrkA, with a focus on interaction between nerve fibers and different immune cells. Emerging evidence shows that neuro-immune interactions play a pivotal role in mediating pruritus-associated inflammatory skin diseases such as atopic dermatitis, psoriasis or chronic spontaneous urticaria. Targeting these bidirectional neuro-immune interactions and the involved pruritus-specific receptors is likely to contribute to novel insights into the underlying pathogenesis and targeted treatment options of pruritus.
Introduction
The complex symptom of pruritus shows up in several diseases which ranges from numerous inflammatory skin diseases, metabolic disorders, liver and kidney diseases, or lymphoproliferative and myeloproliferative disorders (1). The most common chronic inflammatory skin diseases include atopic dermatitis (AD), psoriasis and chronic spontaneous urticaria (CSU). These patients often suffer from moderate to severe pruritus and experience a reduced quality of life (2, 3). Chronic pruritus in these patients remains a challenge regarding effective anti-pruritic treatments (4). The physiology of pruritus is transmitted by a complex interaction network of cutaneous and neuronal cells (5–7). Thus, it is very important to understand this network and dynamic processes to identify novel signaling pathways and pruritus mediators. Particularly, immune and neuronal systems are not acting separately, but interact rather closely with each other. Neurons modulate the function of immune cells by releasing neurotransmitters and neuropeptides leading to the transmission of pruritus and inflammation. In turn, activation of immune cells leads to the production and release of proinflammatory mediators including several cytokines, chemokines and neuropeptides that trigger neuronal pruritus response and inflammation in the skin (8, 9). These neuro-immune interactions arise not only from an intense biochemical crosstalk between immune cells and neurons, but also from sharing many properties, including receptor and ligand expression, which enables efficient communication between these two systems (10, 11). Thus, linking immune and neuronal systems provides a powerful way to gain insight into complex interactions associated with the neuro-immune interaction mechanism in pruritus. In this review, we highlight recent discoveries and approaches concerning interaction of pruritus receptors and channels in a neuro-immune manner in the field of pruritus research. We set our focus on transient receptor potential (TRP) channels, such as TRP vanilloid 1 (TRPV1) and ankyrin 1 (TRPA1), the heterodimer IL-31 receptor A (IL-31RA) and oncostatin-M receptor (OSMR), thymic stromal lymphopoietin receptor (TSLPR) and different G protein-coupled receptors (GPCR). These GPCRs comprise protease-activated receptor-2 (PAR-2), neurokinin-1 receptor (NK1R), histamine receptors H1 and H4 (H1R/H4R) and mas-related G-protein coupled receptors (MRGPRs) as well as tropomyosin receptor kinase A (TrkA) receptor (Figure 1, Table 1). These receptors and channels have been found on sensory neurons and play a crucial role in pruritus and neuro-immune pathways as well as pruritus associated inflammatory skin diseases (4, 12, 13). Here, we put emphasis on inflammatory skin diseases including AD, psoriasis and CSU that are highly in context with symptom of pruritus. In previous research, several treatment options for patients suffering from these pruritus-associated disorders were described (14–17). Additionally, we outline current therapeutic options in correspondence with these pruritus-associated receptors and channels (Table 2). Targeting neuro-immune pathways may open up new perspectives in terms of the development of more effective pharmacological treatment options for patients suffering from chronic pruritus.
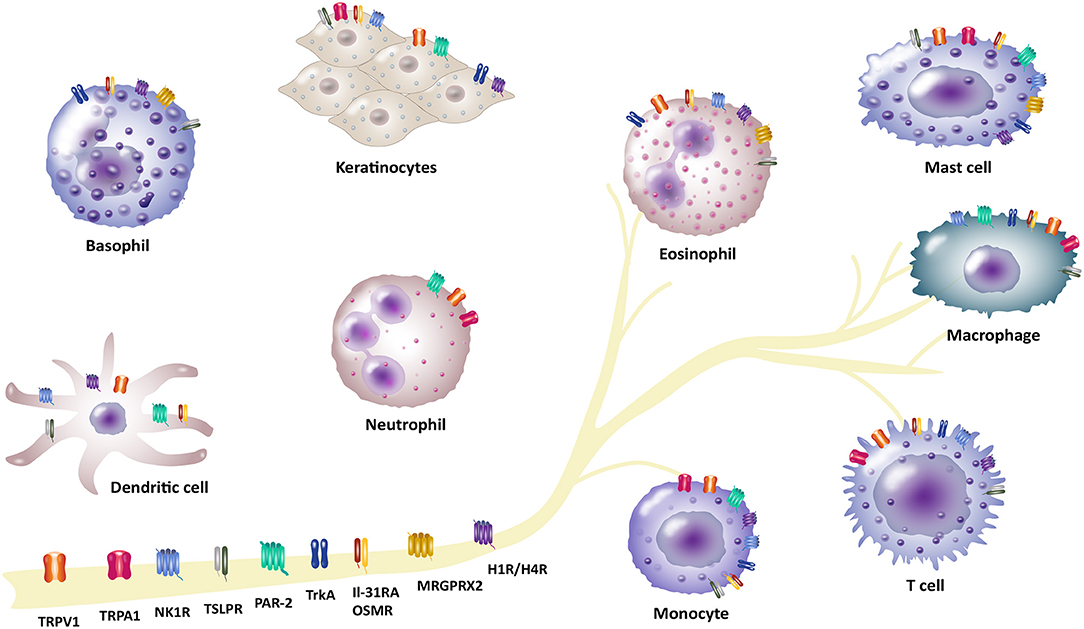
Figure 1. Involvement of different receptors/channels in neuro-immune interactions in pruritus. There is a complex interplay between neurons and immune cells in transmission of pruritus and inflammation. Several receptors act as a bridge between the neuronal and immune network and function as pruritus mediators. These receptors are located on neurons, but also expressed by different non-neuronal cells (e.g., basophils, dendritic cells, eosinophils, keratinocytes, mast cells, macrophages and monocytes, neutrophils or T cells): Transient receptor potential vanilloid 1 (TRPV1) and ankyrin 1 (TRPA1), IL-31 receptor A (IL-31RA) and the oncostatin-M receptor (OSMR), thymic stromal lymphopoietin receptor (TSLPR), protease-activated receptor 2 (PAR-2), neurokinin-1 receptor (NK1R), histamine receptors H1/H4 (H1R/H4R), mas-related G-protein coupled receptor X2 (MRGPRX2), tropomyosin receptor kinase A (TrkA).
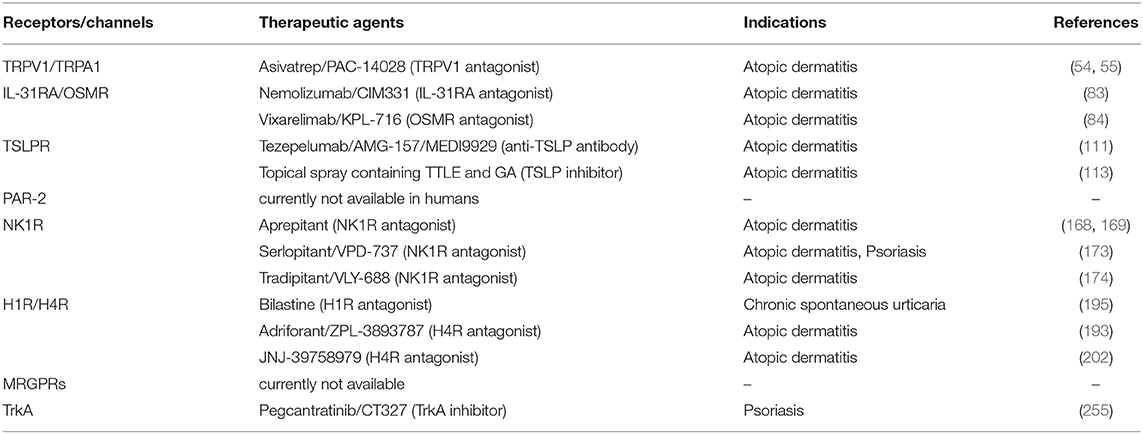
Table 2. Emerging therapeutic targets for treatment of pruritus associated inflammatory skin diseases in humans.
Receptors In Neuro-Immune Interactions
Transient Receptor Potential Channels TRPV1 and TRPA1
TRP channels are non-selective calcium-permeable cation channels comprising 28 members in mammals that can be categorized in six related protein families including TRPA, TRPC, TRPM, TRPML, TRPP, and TRPV (18, 19). TRP channels are involved in various sensory functions, such as mechanosensation, olfaction, osmolarity, pain, taste and thermoception (20–22). Several studies presented evidence showing that TRPV1 and TRPA1 play crucial roles in pruritus transmission (23–27). TRPA1 is essential in the signaling pathways that promote histamine-independent pruritus (22, 24), whereas TRPV1 is presumed to be required for both histaminergic and non-histaminergic pruritus (23, 28–31). These recent studies used knockout (KO) mice models and corresponding inhibitors and led to the conclusion that TRP channels are necessary in the pruritus pathways initiated by GPCR agonists like chloroquine and histamine (24, 29, 31). On the other hand, Ru et al. (32) demonstrated that TRPV1 and TRPA1 channels are not required for chloroquine activation of nerves by using dorsal skin-nerve preparation of healthy mice. This indicated that these TRP channels could affect other than primary afferent terminals (32). However, both ion channels are well-expressed in primary afferent sensory neurons, but also in non-neuronal cells like keratinocytes (33, 34), monocytes and macrophages (35–38), mast cells (39, 40), neutrophils (41, 42) and T cells (25, 43–45). TRPV1 is additionally expressed in dendritic cells (46, 47) and eosinophils (48). Besides neuro-immune interactions, crosstalk between the channels TRPV1 and TRPA1 and other receptors has been established in previous studies (5, 24, 47, 49, 50). An experimental study of Oh et al. (40) has described a case of complex interactions among nerve fibers and mast cells with the TRPA1 channel. The study demonstrated a neural TRPA1 dependent mechanism comprising interactions between TRPA1+ dermal mast cells and TRPA1+ dermal afferent nerves in a TH2-dominated inflammatory environment, which is responsible for the pruritogenesis of chronic pruritus in AD (40). Another example of neuro-immune interactions highlights the involvement of TRPV1 in the crosstalk between neurons and T-lymphocytes (25). Experiments revealed that IL-31 induces pruritus by binding to IL-31RA that is exclusively expressed on TRPV1+/TRPA1+ DRG neurons indicating TRP channels as key mediators of T-cell mediated IL-31-induced pruritus. Interestingly, only around 4% of DRG neurons were observed to be IL-31RA+ (25, 51). Surprisingly, IL-31 was shown to be a potential pruritogen, since injection of IL-31 into the cheek of mice induced profound pruritus, but not pain. This implicates that pruritus and pain may be induced by different subsets of unmyelinated afferents and pruritus specific afferents might exist (25). Interestingly, several studies reported a delayed pruritus after IL-31 injection in mice (52) as well as in patients with AD and healthy volunteers (53). These studies have led to great interest in targeting TRPV1 and developing potential drugs to treat pruritus, especially in AD. In that regard, a topical TRPV1 antagonist termed asivatrep, has shown to significantly improve symptoms (e.g., pruritus, sleep disturbance) of patients with mild-to-moderate AD (54, 55). Further investigations will be needed to unravel the neuro-immune axis involving TRP channels TRPA1 and TRPV1, neurons and different immune cells for new anti-pruritic therapeutic options.
IL-31 Receptor A and Oncostatin-M Receptor
The novel cytokine IL-31 signals through a heterodimeric receptor composed of IL-31RA and the OSMR. IL-31 is a TH2-cell-derived cytokine and the only known ligand for IL-31RA (56–58). It has previously been observed by Cevikbas et al. (25) that TH2 cells are main producers of IL-31. Besides TH2 cells, other immune cells like basophils, eosinophils or mast cells can produce and release IL-31 (59–62). The IL-31 receptor complex is not only expressed by DRG neurons (63), but also located on non-neuronal cells, such as basophils (62), eosinophils (61, 64), monocytes and macrophages (65–67), mast cells (13), dendritic cells (68), keratinocytes (69, 70), and T cells (25, 57, 71). IL-31/IL-31RA interaction activates signal transduction pathways leading to expression and release of various chemokines, proinflammatory cytokines, regulation of cell proliferation and stimulation of DRG neurons that play important role in pruritus induction and inflammatory diseases (72–74). A number of researchers observed an association between IL-31 and inflammatory skin diseases with severe pruritus including AD (61, 75), bullous pemphigoid (76), cutaneous T-cell lymphoma (77), CSU (78) and psoriasis (79). Regarding treatment approaches, a successful therapy of urticaria using omalizumab led to decreased serum levels of IL-31 (80). Previous research has established a neuro-immune crosstalk between IL-31 receptor, T cells and sensory nerves in pruritus (25). Cevikbas et al. (25) have shown that TH2-derived IL-31 is able to activate IL-31RA on TRPV1+/TRPA1+ sensory nerves in the skin causing the pruritus associated with AD. Furthermore, it was shown that the TH2-related and atopy-associated cytokine IL-31 directly induces nerve fiber elongation in vitro and in vivo in mice, suggesting that IL-31-associated nerve fiber elongation could be involved in skin hypersensitivity of AD patients (57). In this regard, IL-31 has been shown to correlate with disease severity and pruritus in AD patients (75). More recent findings have demonstrated that nemolizumab, an anti-IL-31RA antibody that binds to IL-31RA with subsequent inhibition of IL-31 signaling effectively relieves AD-associated pruritus (81, 82). The first clinical study revealed a statistically significant reduced pruritus visual analog scale (VAS) score to about 50% at week 4 compared with 20% with placebo in patients with AD (81). In a subsequent phase II study 264 adults with moderate to severe AD were treated every 4 weeks with nemolizumab in doses of 0.1, 0.5, or 2.0 mg/kg. Treatment led to decrease of pruritus VAS by 43.7% to 63.1% in a dose-dependent manner over a 12-week period compared with a 20.9% decrease with placebo (82). Further, a long-term extension study based on phase II trial resulted in reduced pruritus up to 90%, whereby it was limited by a placebo group (83). Another approach is provided by a human monoclonal antibody KPL-716, which specifically targets the OSMRβ chain and simultaneously inhibits both IL-31 and OSM signaling. Therefore, blocking OSMRβ with KPL-716 may be a potential treatment option of inflammatory skin diseases (e.g., AD) and needs to be clarified in further experiments (84). These studies indicate that IL-31 is an important cytokine for regulating pruritus and AD disease activity.
Thymic Stromal Lymphopoietin Receptor
TSLP is a four-helix bundle, IL-7-like cytokine, and a member of the IL-2 cytokine family that contributes to the initiation of type-2 inflammation. It is primarily produced by epithelial cells including keratinocytes, fibroblasts and stromal cells, but also by dendritic cells and mast cells (85, 86). TSLP signaling requires a heterodimeric receptor complex that consists of the IL-7 receptor α-chain (IL-7Rα) and the TSLP receptor chain (TSLPR) (87, 88). TSLP receptor is expressed by a variety of cell populations including non-neuronal cells, such as basophils (89), eosinophils (90), dendritic cells (91), keratinocytes (92), mast cells (93), macrophages and monocytes (94, 95), B and T cells (96, 97), but also by neurons (11, 98). The expression of TSLP from these different target cells can be triggered by various stimuli comprising respiratory viruses (99), cigarette smoke extracts (100) as well as several cytokines, such as TNF-α and IL-1β (101). TSLP is known to be involved in various allergic diseases such as AD (102, 103), bronchial asthma (104) and eosinophilic esophagitis (105). There is a growing evidence indicating that TSLP may also play role in other diseases including autoimmune, chronic inflammatory disorders and cancer (86, 106, 107). In terms of AD several studies show that TSLP serum level as well as TSLP level in the skin of AD patients is elevated (102, 103, 108). An overexpression of TSLP in mice models resulted in the development of AD (109, 110). Wilson et al. (98) have demonstrated that intradermal injection of TSLP led to scratching behavior in mice. Additionally, their data confirmed that TSLP released from keratinocytes acts directly on sensory neurons to induce itch-evoked scratching that was depended on TSLPR. Further it was evidenced that both functional TSLPRs and TRPA1 channels are required for TSLP-induced pruritus. A crosstalk between TSLP and PAR-2 was also observed. PAR-2 activation by its agonists SLIGLR and tryptase induced scratching behavior and Ca2+-dependent release of TSLP (98). However, the mechanism behind the TSLP-induced pruritus remains to be elucidated in further experimental studies. Targeting TSLP-TSLPR signaling via anti-TSLP therapy like with tezepelumab, a human monoclonal antibody targeting circulating TSLP, might be a promising tool to prevent and treat several diseases associated with elevated TSLP such as AD (111, 112). Contrarily, a phase II clinical trial tezepelumab treatment of patient with moderate to severe AD showed limited efficacy and insignificant pruritus reduction (111). More recently, Fitoussi et al. (113) demonstrated that a topical spray containing Tambourissa trichophylla leaf extract (TTLE) and 18β-glycyrrhetinic acid (GA), which inhibits TSLP secretion, efficiently decreases pruritus in AD patients and improves their quality of life.
Protease-Activated Receptor-2
The PAR family consists of four members, PAR-1, PAR-2, PAR-3, and PAR-4. All together they belong to G-protein coupled receptors activated by proteolytic cleavage of amino-terminal exodomain (114–117). Furthermore, an activation by different proteases generated by endogenous (e.g., proteases from endothelium, epithelium, fibroblast or immune cells) or exogenous sources (e.g., allergens, dust mite and various plants) is possible (118, 119). Existing research recognizes the critical role played by PAR-2 in skin neurogenic inflammation and in pruritic skin diseases such as AD (119–123). PAR-2 is expressed by various cell types including endothelial cells and keratinocytes (124), dendritic cells, monocytes and macrophages (125–127), mast cells (128, 129), neutrophils (130) and sensory nerve fibers (123, 131, 132). Steinhoff et al. (120) reported an increased signaling through PAR-2 that comprises an increased release of endogenous PAR-2 agonist mast cell tryptase followed by a higher occurrence of PAR-2+ nerve fibers in AD patients (120, 133). In addition to the crosstalk between nerve fibers, mast cells and PAR-2, it was shown that PAR-2 synergistically interact with TRPV1 channel resulting in pruritus sensation (134, 135). A key role of TRPV1 channel in PAR2-evoked Ca2+ release in differentiated human primary keratinocytes was shown by Gouin et al. (136). They demonstrated that TRPV1 independently regulate the production of inflammatory mediators, such as IL-1β, TNF-α, and TSLP via Ca2+ and NF-kB signaling (136). Overexpression of these inflammatory mediators is in connection with inflammatory skin diseases, such as AD or psoriasis (137–141). In a very recent follow-up study Buhl et al. (119) found that PAR-2 regulates neuro-epidermal communication in AD using a mouse model with epidermal overexpression of PAR-2. The research results indicate that PAR-2 signaling in keratinocytes causes epidermal responses leading to neuronal sensory and inflammatory responses in their AD model (119). A promising therapeutic approach presents a PAR-2 pepducin, termed PZ-235. Barr et al. (142) examined the capacity of PZ-235 to suppress skin lesion thickening, inflammation, and pruritus in acute and chronic models of AD. For this, MA-1, a mast cell-degranulating peptide from wasp venom, was utilized to induce severe scratching in mice. Subsequent PZ-235 treatment significantly reduced scratching behavior in mice up to 50%. Further results demonstrated that targeting PAR-2 via PZ-235 application attenuated production of inflammatory factors, leukocyte infiltration, skin thickening as well as severity of skin lesions. Therefore, PZ-235 may have potential in the effective treatment of patients with AD (142). More studies and clinical trials in humans are currently lacking and needs to be investigated.
Neurokinin-1 Receptor
Neurokinin receptors belong to G protein-coupled receptors and consists of three members, neurokinin-1-3 receptors (NK1-3R) that are implicated in afferent neuronal signal transduction. There are various ligands for these receptors like neurokinin A (NKA), neurokinin B (NKB), neuropeptide K (NPK), neuropeptide-γ (NKγ), endokinin, hemokinin 1 as well as substance P (SP), belonging to tachykinin family, whereas SP binds with high affinity to the NK1R (143–146). Especially, NK1R is known to mainly contribute to transmission of pruritus (4, 12, 147). NK1R is widely expressed by different immune cells, such as dendritic cells (148), eosinophils (149), mast cells (150), macrophages and monocytes (151) and T and B cells (152, 153), but also by keratinocytes (154, 155) and sensory nerve endings (11, 156, 157). Activation of NK1R via SP leads to multiple signaling cascades involving mast cell degranulation and release of proinflammatory mediators, such as histamine, nerve growth factor expression and leukotriene B4 in keratinocytes and neurogenic inflammation resulting in induction of inflammation and pruritus (145, 146, 158). Several studies investigated the role of SP and NK1R in the pathogenesis of pruritus in various diseases like AD, psoriasis and CSU (7, 159–163). Recently, it was reported that SP and its receptor NK1R are overexpressed in pruritic AD and psoriatic lesional skin (164). A previous study demonstrated that increased serum levels of SP in AD patients correlate with pruritus intensity (165, 166). Interestingly, oral treatment with the NK1R antagonist aprepitant led to reduced serum levels of immunoglobulin E (IgE) and SP levels in tissue as well as decreased cutaneous infiltration of regulatory T cells in an NC/Nga mouse model (167). In contrast, clinical studies revealed no significant differences between aprepitant treatment and placebo concerning reduction in pruritus, improvement in pruriginous lesions or quality of life (168, 169). However, another clinical study has shown that the NK1R antagonist serlopitant has potential as a therapeutic agent for the treatment of patients with chronic pruritus by significantly reducing the pruritus symptom (170–172). A phase II clinical study concluded that serlopitant reduced pruritus in patients with mild to moderate psoriasis (173). Another NK1R antagonist, tradipitant, was examined in terms of reduction of pruritus associated with AD through inhibition of SP-mediated itch signaling. Tradipitant treatment improved pruritus and sleep in mild AD (174). Several NK1R antagonists that potentially reduce pruritus activity in dermatological diseases are reviewed by Pojawa-Goła et al. (146) and Reszke et al. (172). Thus, targeting SP and/or NK1R with regard to neuro-immune crosstalk seems to be a promising approach in the treatment of pruritus. In a previous research it was established that Mas-related GPCR X2, which is also activated by SP, induced inflammation (175). Further, it was suggested that SP-induced pruritus may be mediated by MRGPRs rather than NK1R, since SP-induced pruritus was not decreased in Nk1r KO mice. Co-injection of QWF and SP in both Nk1r KO and wild-type mice led to significantly decreased SP-induced pruritus. Interestingly, an NK1R antagonist termed QWF was shown to have a dual action on MRGPRX2 (176). However, not only the crosstalk between different immune cells, neurons and NK1R, but also the interaction of NK1R with other receptors is an interesting approach for a better understanding of the pathogenesis of pruritic diseases.
Histamine Receptors H1 and H4
One of the well-characterized pruritogens is histamine. Histamine is released from mast cells and basophils via activation of histamine receptors, which belong to the G protein-coupled receptor superfamily. While four histamine receptor subtypes (H1–H4) exist, notably histamine receptors H1 and H4 are known to modulate pruritus (13, 177–181). Both histamine receptors (H1R and H4R) are extensively expressed in a wide range of cell types involving sensory neurons (182), epithelial cells like keratinocytes (183, 184), but also immune cells, such as basophils (185), dendritic cells (186, 187), eosinophils (13), monocytes (187), mast cells (188) and T cells (28, 189–191). Especially, the H4R is predominantly expressed by immune cells and is in conjunction with lots of functional histamine-mediated inflammatory responses like modulation of cytokine and chemokine release, chemotaxis and cell recruitment as well as upregulation of adhesion molecule expression (192, 193). However, both the H1 and the H4 histamine receptors play pivotal roles in various pruritic skin diseases, such as AD or CSU (188, 194–196). Various H1R antihistamines like ebastine, cetirizine, and levocetirizine were shown to decrease pruritus symptom of patients with CSU by 60–70% (197). A very recent clinical study presented a switch to bilastine, a H1R antagonist, as an optional treatment for patients with CSU, who are unresponsive to H1R antihistamines at the licensed doses (195). Although H1R antihistamines demonstrated convincing anti-pruritic effects in urticaria, they show limited efficiency in other pruritic skin diseases such as AD (11, 197, 198). In the study of Gutzmer et al. (190), it was demonstrated that AD patients express increased levels of H4R on T cells. Upon stimulation of the H4 receptor pruritogenic IL-31 is up-regulated leading to pruritic response (190). H4R antagonists were shown to reduce TH2 cytokine production, pruritus and skin inflammation in AD-associated animal models (199, 200). Therefore, new clinical trials using novel H4R antagonists might a promising treatment for patients with AD such as the H4R antagonist JNJ-39758979, which led to an improvement of inflammatory skin lesions in AD patients (193, 201). In addition, marked effects against pruritus in Japanese patients with AD could be observed in a phase II clinical trial, but the development of agranulocytosis by 2 subjects resulted in early trial termination (202). More recently, H4R antagonist adriforant was shown to improve inflammatory skin lesions in patients with AD. Although adriforant treatment cause a 3-point reduction (scale, 1–10) in pruritus, there was no significant difference in comparison to reduced pruritus with placebo (193). Interestingly, a combined treatment of both H1R and H4R antagonists demonstrated an anti-inflammatory effect in an AD mice model that might be a good strategy to treat patients with AD (203).
Mas-Related G-Protein Coupled Receptors
MRGPRs are G-protein coupled receptors that comprise at least 50 family members in mice, divided into subgroups MRGPRA-H and 8 members in humans named MRGPRX1-X4, D, E, F, and G. Several members of MRGPRs have emerged as critically important receptors in histamine-independent pruritus. They are mainly expressed by sensory neurons and some also by mast cells (5, 8, 204, 205). Recently, human basophils and eosinophils were reported to express MRGPRX2 (206). However, the MRGPRs can be activated by various endogenous and exogenous peptides or molecules, such as antimicrobial host defense or opioid peptides, SP or eosinophilic granules, but also by drugs like vancomycin or chloroquine (CQ) (12, 207). Particularly, MRGPRA3 and MRGPRC11 in mice as well as the human ortholog MRGPRX1 got into the focus of pruritus researchers over the past decade (12, 208). It was shown that CQ activated MRGPRA3 leading to a pruritus signal via the activation of TRPA1 (24). Furthermore, it was demonstrated that expression of MRGPRA3 establishes a subset of nociceptors that specifically mediate pruritus, but not pain in a mouse model. In addition, a deletion of MRGPRA3+ sensory neurons significantly inhibits scratching behavior (209, 210). A recent study by Lee et al. (211) determined that Korean Red Ginseng water extract (KRGE) inhibits CQ-induced pruritus by blocking the MRGPRA3/TRPA1 pathway. Interestingly, KRGE has also anti-pruritic effects on the histamine-dependent H1R/TRPV1 pathway, which might provide a dual anti-pruritic candidate agent for the treatment of pruritus patients (211, 212). Moreover, KRGE treatment significantly decreased hyperplasia and hyperkeratosis in the epidermis, infiltration of inflammatory cells and suppressed the overexpression of cytokines in the AD-like skin lesions of AD mice model (167). MRGPRC11 is in addition to MRGPRA3 co-localized and expressed in a subset of TRPV1+ afferents and mediates pruritus induced by BAM8-22 (24, 209, 213). Liu et al. (204) has proven that activation through MRGPRC11-specific agonist BAM8-22 induces scratching in murine models. In a following clinical study, BAM8-22 triggered pruritus and nociceptive sensations in humans in a histamine-independent manner as topical antihistamine-containing cream did not attenuate scratching behavior (214). This indicates BAM8-22 as an endogenous pruritus mediator and MRGPRX1 antagonists may present potential anti-pruritic therapies. The synthetic peptide SLIGRL was long believed to mediate scratching behavior via the PAR-2. However, intradermal injected SLIGRL caused scratching behavior in PAR-2 KO mice similar to that of wild-type mice. Liu and colleagues (215) proved that the pruritus induction of SLIGRL was mediated by MRGPRC11 while its hyperalgesic mode of action was derived from PAR-2 (2, 215). Furthermore, MRGPRX1 is responsible for neuronal activation and scratching behavior induced by both CQ and BAM8-22 (204, 213, 216). To date, there is a lack of knowledge about the involvement of MRGPRX1 in the pathology in chronic pruritic diseases such as AD and the potential role of MRGPRX1 antagonists in affected patients. Recently, researchers have shown an increased interest in MRGPRX2 in terms of pruriceptive receptor and its involvement in pruritic diseases like AD or psoriasis (164, 176, 217, 218). MRGPRX2 is expressed in mast cells and an activation of MRGPRX2 by peptides such as SP results in mast cell degranulation leading to release of proinflammatory factors as well as modulation of neurogenic inflammation and pruritus (219, 220). Previous research has established that both the percentage of MRGPRX2+ mast cells and MRGPRX2+ skin mast cells of patients with CSU were significantly higher in comparison to non-chronic urticaria subjects. It was further shown that SP-induced histamine release from human skin mast cells through MRGPRX2 contributing to neurogenic inflammation (221). Interestingly, Green et al. (222) found out that SP-mediated inflammatory responses were independent of its canonical receptor NK1R and identified MRGPRX2 and its mouse homolog MRGPRB2 as an important neuro-immune modulator and a potential target for treating inflammatory pain. Involvement in pruritus transmission and anti-pruritic treatment therapies remain elusive and needs to be clarified in further studies (222). In a recent study, increased MRGPRX2 mRNA expression in pruritic skin of patients with AD and psoriasis was demonstrated as well (164). However, research has consistently shown that only few endogenous agonists for most of these receptors are known so far and their role in the pathogenesis chronic pruritus diseases such as AD remains still unclear.
Tropomyosin Receptor Kinase A
Trk receptors were firstly described in 1986 and three members of the tyrosine kinase receptor family, TrkA, TrkB, and TrkC, have been identified so far. Trk receptors are activated by various neurotrophins including nerve-growth factor (NGF), brain-derived neurotrophic factor (BDNF), neurotrophin-3 (NT-3) and neurotrophin-4 (NT-4) (223–226). The main source of NGF are keratinocytes in the skin (227, 228), but it is also expressed and secreted by other immune cells, such as basophils (229), monocytes and macrophages (230), mast cells (231, 232) and eosinophils (233) as well as by neurons (234, 235) during inflammation. NGF binds with high affinity to its receptor TrkA as well as the low-affinity neurotrophin receptor p75NTR. TrkA is widely expressed across the airway smooth muscles, the lung epithelium and sensory neurons (236, 237), but also located on various non-neuronal cells like basophils (238), eosinophils (233), keratinocytes (228), monocytes and macrophages (239), mast cells (231, 232) as well as B and T cells (240, 241). Both NGF and its receptor TrkA are suggested to play important roles in pruritus and allergic inflammation. Several studies reported that NGF in the skin and NGF serum levels of AD and psoriatic patients as well as serum levels of patients with asthma are increased (227, 242–245). Additionally, an increased TrkA expression in keratinocytes of patients with AD has been observed during inflammation (228). In AD it was shown, that increased peripheral serum levels of BDNF significantly correlate with disease severity and pruritus (246, 247). Also scratching activities were significantly correlated to increased levels of BDNF as shown by Hon and colleagues (248) which used a DigiTrac model to assess scratching activities in children with AD. In this regard, it has been shown that eosinophils are a source of BDNF and release BDNF and are functionally activated by BDNF with induction of chemotaxis (246–248). Thus, the question arises if BDNF which is released by eosinophils of AD patients is also capable to stimulate nerves. This has recently been shown in a study by us in which we could see that BDNF released by peripheral blood eosinophils of patients with AD led to a significant sprouting of peripheral nerves derived from spinal neurons of mice (247). Thus, also BDNF seems to have an important impact in neuro-immune interaction mechanisms and pruritus. However, NGF affects neurite outgrowth and neuronal survival (236, 249). Interestingly, sprouting of itch-sensitive nerve fibers, promoted by increased NGF levels, has been observed in the skin of patients with AD (242) and in AD-associated mice models (250, 251). Since NGF is known to increase cutaneous innervation in AD models and might contribute to the development of chronic pruritus, NGF and its receptor TrkA could be targets for future treatment of pruritus and allergic inflammation in pruritic diseases like AD or psoriasis. A clinical study demonstrated a promising treatment of AD by neutralizing antibodies against NGF that inhibited the development of skin lesions and epidermal innervation as well as scratching behavior in AD mice model (252). In human sensory neurons, NGF up-regulated the expression and sensitivity of TRPV1 channels by activating TrkA (253, 254). Interestingly, TrkA inhibitor CT327 was shown to significantly reduce chronic pruritus in patients with psoriasis as measured by VAS in a phase II clinical study. The results demonstrated that 62, 46, and 61% of patients treated with CT327 0.05, 0.1, and 0.5%, respectively, had at least a 50% decrease in pruritus VAS in comparison to 32% on vehicle (255). Further trials are necessary to prove the anti-pruritic effects of CT327 in AD. There is growing evidence that cutaneous NGF-TrkA-TRPV1 signaling might be a key mechanism contributing to neurogenic inflammation and pruritus in different dermatological diseases (147, 255).
Conclusion
Our understanding of the pathogenesis of pruritus has significantly evolved in recent years. There is a growing body of literature on the complex crosstalk between neuronal and immune cells that are involved in the development of acute and chronic pruritus. Neurons directly communicate with and regulate the function of various immune cells, such as mast cells, dendritic cells, eosinophils and T cells in pruritus transmission and inflammation. Immune cells release proinflammatory mediators including cytokines, chemokines, neurotrophins, and neuropeptides that activate sensory neurons to mediate pruritus. Activation of these neurons leads to a release of neurotransmitters and neuropeptides that vice versa have a direct impact on the functional activity of immune cells. The literature on neuro-immune crosstalk has emphasized several key mediators and neuronal pathways involved in the transmission of pruritus. Potential mediator and promising receptor therapeutic targets in the skin as well as in peripheral nerves comprises TRPV1, TRPA1, IL-31RA, TSLPR, PAR-2, NK1R, H1R and H4R, MRGPRs and TrkA, which are highlighted in this review (Figure 1, Tables 1, 2). Future studies targeting neuro-immune interactions will help to unravel the underlying mechanisms of pruritus and to develop specific therapies.
Author Contributions
AR has performed the literature research and wrote the manuscript including the tables. MML designed the figure and revised the manuscript. UR, KL, AEK, and BH critically revised the manuscript. All authors read and approved the final version of the submitted manuscript.
Funding
This work was kindly supported by the Deutsche Forschungsgemeinschaft-funded consortium FOR 2690: Translationale Pruritusforschung (RA-1026/3-1, KR-3618/3-1, HO 2092/7-1).
Conflict of Interest
The authors declare that the research was conducted in the absence of any commercial or financial relationships that could be construed as a potential conflict of interest.
References
1. Raap U, Ständer S, Metz M. Pathophysiology of itch and new treatments. Curr Opin Allergy Clin Immunol. (2011) 11:420–7. doi: 10.1097/ACI.0b013e32834a41c2
2. Han L, Dong X. Itch mechanisms and circuits. Annu Rev Biophys. (2014) 43:331–55. doi: 10.1146/annurev-biophys-051013-022826
3. Silverberg JI, Hinami K, Trick WE, Cella D. Itch in the general internal medicine setting: a cross-sectional study of prevalence and quality-of-life effects. Am J Clin Dermatol. (2016) 17:681–90. doi: 10.1007/s40257-016-0215-3
4. Mack MR, Kim BS. The itch-scratch cycle: a neuroimmune perspective. Trends Immunol. (2018) 39:980–91. doi: 10.1016/j.it.2018.10.001
5. Mollanazar NK, Smith PK, Yosipovitch G. Mediators of chronic pruritus in atopic dermatitis: getting the itch out? Clin Rev Allergy Immunol. (2016) 51:263–92. doi: 10.1007/s12016-015-8488-5
6. Pereira MP, Agelopoulos K, Kremer AE, Schmelz M. [New findings regarding the neurobiology of pruritus]. Hautarzt. (2018) 69:620–5. doi: 10.1007/s00105-018-4210-x
7. Yosipovitch G, Berger T, Fassett MS. Neuroimmune interactions in chronic itch of atopic dermatitis. J Eur Acad Dermatol Venereol. (2020) 34:239–50. doi: 10.1111/jdv.15973
8. Voisin T, Bouvier A, Chiu IM. Neuro-immune interactions in allergic diseases: novel targets for therapeutics. Int Immunol. (2017) 29:247–61. doi: 10.1093/intimm/dxx040
9. Kabata H, Artis D. Neuro-immune crosstalk and allergic inflammation. J Clin Invest. (2019) 129:1475–82. doi: 10.1172/JCI124609
10. Lopez-Requena A, Boonen B, Van Gerven L, Hellings PW, Alpizar YA, Talavera K. Roles of neuronal TRP channels in neuroimmune interactions. In: Emir TLR, editor. Neurobiology of TRP Channels. Boca Raton, FL: Frontiers in Neuroscience. (2017). p. 277–94. doi: 10.4324/9781315152837-15
11. Oetjen LK, Kim BS. Interactions of the immune and sensory nervous systems in atopy. FEBS J. (2018) 285:3138–51. doi: 10.1111/febs.14465
12. Ständer S, Raap U, Weisshaar E, Schmelz M, Mettang T, Handwerker H, et al. Pathogenesis of pruritus. J Dtsch Dermatol Ges. (2011) 9:456–63. doi: 10.1111/j.1610-0387.2011.07585.x
13. Nakashima C, Ishida Y, Kitoh A, Otsuka A, Kabashima K. Interaction of peripheral nerves and mast cells, eosinophils, and basophils in the development of pruritus. Exp Dermatol. (2019) 28:1405–11. doi: 10.1111/exd.14014
14. Misery L, Huet F, Gouin O, Ständer S, Deleuran M. Current pharmaceutical developments in atopic dermatitis. Curr Opin Pharmacol. (2019) 46:7–13. doi: 10.1016/j.coph.2018.12.003
15. McEwen MW, Fite EM, Yosipovitch G, Patel T. Drugs on the horizon for chronic pruritus. Dermatol Clin. (2018) 36:335–44. doi: 10.1016/j.det.2018.02.016
16. Pereira MP, Ständer S. Chronic pruritus: current and emerging treatment options. Drugs. (2017) 77:999–1007. doi: 10.1007/s40265-017-0746-9
17. Golpanian RS, Yosipovitch G. Current and emerging systemic treatments targeting the neural system for chronic pruritus. Expert Opin Pharmacother. (2020) 21:1629–36. doi: 10.1080/14656566.2020.1775815
18. Clapham DE. TRP channels as cellular sensors. Nature. (2003) 426:517–24. doi: 10.1038/nature02196
19. Montell C. The TRP superfamily of cation channels. Sci STKE. (2005) 2005:re3. doi: 10.1126/stke.2722005re3
20. Sun S, Dong X. Trp channels and itch. Semin Immunopathol. (2016) 38:293–307. doi: 10.1007/s00281-015-0530-4
21. Kittaka H, Tominaga M. The molecular and cellular mechanisms of itch and the involvement of TRP channels in the peripheral sensory nervous system and skin. Allergol Int. (2017) 66:22–30. doi: 10.1016/j.alit.2016.10.003
22. Xie Z, Hu H. TRP channels as drug targets to relieve itch. Pharmaceuticals. (2018) 11:4. doi: 10.3390/ph11040100
23. Shim WS, Tak MH, Lee MH, Kim M, Kim M, Koo JY, et al. TRPV1 mediates histamine-induced itching via the activation of phospholipase A2 and 12-lipoxygenase. J Neurosci. (2007) 27:2331–7. doi: 10.1523/JNEUROSCI.4643-06.2007
24. Wilson SR, Gerhold KA, Bifolck-Fisher A, Liu Q, Patel KN, Dong X, et al. TRPA1 is required for histamine-independent, Mas-related G protein-coupled receptor-mediated itch. Nat Neurosci. (2011) 14:595–602. doi: 10.1038/nn.2789
25. Cevikbas F, Wang X, Akiyama T, Kempkes C, Savinko T, Antal A, et al. A sensory neuron-expressed IL-31 receptor mediates T helper cell-dependent itch: Involvement of TRPV1 and TRPA1. J Allergy Clin Immunol. (2014) 133:448–60. doi: 10.1016/j.jaci.2013.10.048
26. Hojland CR, Andersen HH, Poulsen JN, Arendt-Nielsen L, Gazerani P. A human surrogate model of itch utilizing the TRPA1 agonist trans-cinnamaldehyde. Acta Derm Venereol. (2015) 95:798–803. doi: 10.2340/00015555-2103
27. Jian T, Yang N, Yang Y, Zhu C, Yuan X, Yu G, et al. TRPV1 and PLC participate in histamine H4 receptor-induced itch. Neural Plast. (2016) 2016:1682972. doi: 10.1155/2016/1682972
28. Brennan F. The pathophysiology of pruritus – a review for clinicians. Progress in Palliative Care. (2016) 24:133–46. doi: 10.1179/1743291X14Y.0000000110
29. Imamachi N, Park GH, Lee H, Anderson DJ, Simon MI, Basbaum AI, et al. TRPV1-expressing primary afferents generate behavioral responses to pruritogens via multiple mechanisms. Proc Natl Acad Sci USA. (2009) 106:11330–5. doi: 10.1073/pnas.0905605106
30. Kim SJ, Park GH, Kim D, Lee J, Min H, Wall E, et al. Analysis of cellular and behavioral responses to imiquimod reveals a unique itch pathway in transient receptor potential vanilloid 1 (TRPV1)-expressing neurons. Proc Natl Acad Sci USA. (2011) 108:3371–6. doi: 10.1073/pnas.1019755108
31. Wilson SR, Bautista DM. Role of transient receptor potential channels in acute and chronic itch. In: Carstens E, Akiyama T, editors. Itch: Mechanisms and Treatment. Boca Raton, FL: Frontiers in Neuroscience (2014).
32. Ru F, Sun H, Jurcakova D, Herbstsomer RA, Meixong J, Dong X, et al. Mechanisms of pruritogen-induced activation of itch nerves in isolated mouse skin. J Physiol. (2017) 595:3651–66. doi: 10.1113/JP273795
33. Denda M, Fuziwara S, Inoue K, Denda S, Akamatsu H, Tomitaka A, et al. Immunoreactivity of VR1 on epidermal keratinocyte of human skin. Biochem Biophys Res Commun. (2001) 285:1250–2. doi: 10.1006/bbrc.2001.5299
34. Biro T, Kovacs L. An “ice-cold” TR(i)P to skin biology: the role of TRPA1 in human epidermal keratinocytes. J Invest Dermatol. (2009) 129:2096–9. doi: 10.1038/jid.2009.179
35. Billeter AT, Galbraith N, Walker S, Lawson C, Gardner SA, Sarojini H, et al. TRPA1 mediates the effects of hypothermia on the monocyte inflammatory response. Surgery. (2015) 158:646–54. doi: 10.1016/j.surg.2015.03.065
36. Parenti A, De Logu F, Geppetti P, Benemei S. What is the evidence for the role of TRP channels in inflammatory and immune cells? Br J Pharmacol. (2016) 173:953–69. doi: 10.1111/bph.13392
37. Omari SA, Adams MJ, Geraghty DP. TRPV1 channels in immune cells and hematological malignancies. Adv Pharmacol. (2017) 79:173–98. doi: 10.1016/bs.apha.2017.01.002
38. Khalil M, Alliger K, Weidinger C, Yerinde C, Wirtz S, Becker C, et al. Functional role of transient receptor potential channels in immune cells and epithelia. Front Immunol. (2018) 9:174. doi: 10.3389/fimmu.2018.00174
39. Ständer S, Moormann C, Schumacher M, Buddenkotte J, Artuc M, Shpacovitch V, et al. Expression of vanilloid receptor subtype 1 in cutaneous sensory nerve fibers, mast cells, and epithelial cells of appendage structures. Exp Dermatol. (2004) 13:129–39. doi: 10.1111/j.0906-6705.2004.0178.x
40. Oh MH, Oh SY, Lu J, Lou H, Myers AC, Zhu Z, et al. TRPA1-dependent pruritus in IL-13-induced chronic atopic dermatitis. J Immunol. (2013) 191:5371–82. doi: 10.4049/jimmunol.1300300
41. Heiner I, Eisfeld J, Luckhoff A. Role and regulation of TRP channels in neutrophil granulocytes. Cell Calcium. (2003) 33:533–40. doi: 10.1016/S0143-4160(03)00058-7
42. Zhou Y, Han D, Follansbee T, Wu X, Yu S, Wang B, et al. Transient receptor potential ankyrin 1 (TRPA1) positively regulates imiquimod-induced, psoriasiform dermal inflammation in mice. J Cell Mol Med. (2019) 23:4819–28. doi: 10.1111/jcmm.14392
43. Bertin S, Aoki-Nonaka Y, de Jong PR, Nohara LL, Xu H, Stanwood SR, et al. The ion channel TRPV1 regulates the activation and proinflammatory properties of CD4(+) T cells. Nat Immunol. (2014) 15:1055–63. doi: 10.1038/ni.3009
44. Bertin S, Aoki-Nonaka Y, Lee J, de Jong PR, Kim P, Han T, et al. The TRPA1 ion channel is expressed in CD4+ T cells and restrains T-cell-mediated colitis through inhibition of TRPV1. Gut. (2017) 66:1584–96. doi: 10.1136/gutjnl-2015-310710
45. Sahoo SS, Majhi RK, Tiwari A, Acharya T, Kumar PS, Saha S, et al. Transient receptor potential ankyrin1 channel is endogenously expressed in T cells and is involved in immune functions. Biosci Rep. (2019) 39:BSR20191437. doi: 10.1042/BSR20191437
46. Toth BI, Benko S, Szollosi AG, Kovacs L, Rajnavolgyi E, Biro T. Transient receptor potential vanilloid-1 signaling inhibits differentiation and activation of human dendritic cells. FEBS Lett. (2009) 583:1619–24. doi: 10.1016/j.febslet.2009.04.031
47. Gouin O, L'Herondelle K, Lebonvallet N, Le Gall-Ianotto C, Sakka M, Buhe V, et al. TRPV1 and TRPA1 in cutaneous neurogenic and chronic inflammation: pro-inflammatory response induced by their activation and their sensitization. Protein Cell. (2017) 8:644–61. doi: 10.1007/s13238-017-0395-5
48. Zhu X, Learoyd J, Butt S, Zhu L, Usatyuk PV, Natarajan V, et al. Regulation of eosinophil adhesion by lysophosphatidylcholine via a non-store-operated Ca2+ channel. Am J Respir Cell Mol Biol. (2007) 36:585–93. doi: 10.1165/rcmb.2006-0391OC
49. Patricio ES, Costa R, Figueiredo CP, Gers-Barlag K, Bicca MA, Manjavachi MN, et al. Mechanisms underlying the scratching behavior induced by the activation of proteinase-activated receptor-4 in mice. J Invest Dermatol. (2015) 135:2484–91. doi: 10.1038/jid.2015.183
50. Zhang X. Targeting TRP ion channels for itch relief. Naunyn Schmiedebergs Arch Pharmacol. (2015) 388:389–99. doi: 10.1007/s00210-014-1068-z
51. Li YR, Gupta P. Immune aspects of the bi-directional neuroimmune facilitator TRPV1. Mol Biol Rep. (2019) 46:1499–510. doi: 10.1007/s11033-018-4560-6
52. Kasutani K, Fujii E, Ohyama S, Adachi H, Hasegawa M, Kitamura H, et al. Anti-IL-31 receptor antibody is shown to be a potential therapeutic option for treating itch and dermatitis in mice. Br J Pharmacol. (2014) 171:5049–58. doi: 10.1111/bph.12823
53. Hawro T, Saluja R, Weller K, Altrichter S, Metz M, Maurer M. Interleukin-31 does not induce immediate itch in atopic dermatitis patients and healthy controls after skin challenge. Allergy. (2014) 69:113–7. doi: 10.1111/all.12316
54. Lim KM, Park YH. Development of PAC-14028, a novel transient receptor potential vanilloid type 1 (TRPV1) channel antagonist as a new drug for refractory skin diseases. Arch Pharm Res. (2012) 35:393–6. doi: 10.1007/s12272-012-0321-6
55. Lee YW, Won CH, Jung K, Nam HJ, Choi G, Park YH, et al. Efficacy and safety of PAC-14028 cream - a novel, topical, nonsteroidal, selective TRPV1 antagonist in patients with mild-to-moderate atopic dermatitis: a phase IIb randomized trial. Br J Dermatol. (2019) 180:1030–8. doi: 10.1111/bjd.17455
56. Dillon SR, Sprecher C, Hammond A, Bilsborough J, Rosenfeld-Franklin M, Presnell SR, et al. Interleukin 31, a cytokine produced by activated T cells, induces dermatitis in mice. Nat Immunol. (2004) 5:752–60. doi: 10.1038/ni1084
57. Feld M, Garcia R, Buddenkotte J, Katayama S, Lewis K, Muirhead G, et al. The pruritus- and TH2-associated cytokine IL-31 promotes growth of sensory nerves. J Allergy Clin Immunol. (2016) 138:500–8.e24. doi: 10.1016/j.jaci.2016.02.020
58. Nakashima C, Otsuka A, Kabashima K. Interleukin-31 and interleukin-31 receptor: New therapeutic targets for atopic dermatitis. Exp Dermatol. (2018) 27:327–31. doi: 10.1111/exd.13533
59. Ishii T, Wang J, Zhang W, Mascarenhas J, Hoffman R, Dai Y, et al. Pivotal role of mast cells in pruritogenesis in patients with myeloproliferative disorders. Blood. (2009) 113:5942–50. doi: 10.1182/blood-2008-09-179416
60. Niyonsaba F, Ushio H, Hara M, Yokoi H, Tominaga M, Takamori K, et al. Antimicrobial peptides human beta-defensins and cathelicidin LL-37 induce the secretion of a pruritogenic cytokine IL-31 by human mast cells. J Immunol. (2010) 184:3526–34. doi: 10.4049/jimmunol.0900712
61. Kunsleben N, Rudrich U, Gehring M, Novak N, Kapp A, Raap U. IL-31 Induces Chemotaxis, Calcium Mobilization, Release of Reactive Oxygen Species, and CCL26 in Eosinophils, Which Are Capable to Release IL-31. J Invest Dermatol. (2015) 135:1908–11. doi: 10.1038/jid.2015.106
62. Raap U, Gehring M, Kleiner S, Rudrich U, Eiz-Vesper B, Haas H, et al. Human basophils are a source of - and are differentially activated by - IL-31. Clin Exp Allergy. (2017) 47:499–508. doi: 10.1111/cea.12875
63. Bando T, Morikawa Y, Komori T, Senba E. Complete overlap of interleukin-31 receptor A and oncostatin M receptor beta in the adult dorsal root ganglia with distinct developmental expression patterns. Neuroscience. (2006) 142:1263–71. doi: 10.1016/j.neuroscience.2006.07.009
64. Cheung PF, Wong CK, Ho AW, Hu S, Chen DP, Lam CW. Activation of human eosinophils and epidermal keratinocytes by Th2 cytokine IL-31: implication for the immunopathogenesis of atopic dermatitis. Int Immunol. (2010) 22:453–67. doi: 10.1093/intimm/dxq027
65. Bilsborough J, Leung DY, Maurer M, Howell M, Boguniewicz M, Yao L, et al. IL-31 is associated with cutaneous lymphocyte antigen-positive skin homing T cells in patients with atopic dermatitis. J Allergy Clin Immunol. (2006) 117:418–25. doi: 10.1016/j.jaci.2005.10.046
66. Kasraie S, Niebuhr M, Werfel T. Interleukin (IL)-31 induces pro-inflammatory cytokines in human monocytes and macrophages following stimulation with staphylococcal exotoxins. Allergy. (2010) 65:712–21. doi: 10.1111/j.1398-9995.2009.02255.x
67. Edukulla R, Singh B, Jegga AG, Sontake V, Dillon SR, Madala SK. Th2 cytokines augment IL-31/IL-31RA Interactions via STAT6-dependent IL-31RA expression. J Biol Chem. (2015) 290:13510–20. doi: 10.1074/jbc.M114.622126
68. Horejs-Hoeck J, Schwarz H, Lamprecht S, Maier E, Hainzl S, Schmittner M, et al. Dendritic cells activated by IFN-gamma/STAT1 express IL-31 receptor and release proinflammatory mediators upon IL-31 treatment. J Immunol. (2012) 188:5319–26. doi: 10.4049/jimmunol.1101044
69. Heise R, Neis MM, Marquardt Y, Joussen S, Heinrich PC, Merk HF, et al. IL-31 receptor alpha expression in epidermal keratinocytes is modulated by cell differentiation and interferon gamma. J Invest Dermatol. (2009) 129:240–3. doi: 10.1038/jid.2008.183
70. Kasraie S, Niebuhr M, Baumert K, Werfel T. Functional effects of interleukin 31 in human primary keratinocytes. Allergy. (2011) 66:845–52. doi: 10.1111/j.1398-9995.2011.02545.x
71. Cevikbas F, Kempkes C, Buhl T, Mess C, Buddenkotte J, Steinhoff M. Role of interleukin-31 and oncostatin M in itch and neuroimmune communication. In: Carstens E, Akiyama T, editors. Itch: Mechanisms and Treatment. Boca Raton, FL: Frontiers in Neuroscience (2014).
72. Sonkoly E, Muller A, Lauerma AI, Pivarcsi A, Soto H, Kemeny L, et al. IL-31: a new link between T cells and pruritus in atopic skin inflammation. J Allergy Clin Immunol. (2006) 117:411–7. doi: 10.1016/j.jaci.2005.10.033
73. Stott B, Lavender P, Lehmann S, Pennino D, Durham S, Schmidt-Weber CB. Human IL-31 is induced by IL-4 and promotes TH2-driven inflammation. J Allergy Clin Immunol. (2013) 132:446–54.e5. doi: 10.1016/j.jaci.2013.03.050
74. Gibbs BF, Patsinakidis N, Raap U. Role of the pruritic cytokine IL-31 in autoimmune skin diseases. Front Immunol. (2019) 10:1383. doi: 10.3389/fimmu.2019.01383
75. Raap U, Wichmann K, Bruder M, Ständer S, Wedi B, Kapp A, et al. Correlation of IL-31 serum levels with severity of atopic dermatitis. J Allergy Clin Immunol. (2008) 122:421–3. doi: 10.1016/j.jaci.2008.05.047
76. Salz M, Haeberle S, Hoffmann J, Enk AH, Hadaschik EN. Elevated IL-31 serum levels in bullous pemphigoid patients correlate with eosinophil numbers and are associated with BP180-IgE. J Dermatol Sci. (2017) 87:309–11. doi: 10.1016/j.jdermsci.2017.07.019
77. Ohmatsu H, Sugaya M, Suga H, Morimura S, Miyagaki T, Kai H, et al. Serum IL-31 levels are increased in patients with cutaneous T-cell lymphoma. Acta Derm Venereol. (2012) 92:282–3. doi: 10.2340/00015555-1345
78. Raap U, Wieczorek D, Gehring M, Pauls I, Ständer S, Kapp A, et al. Increased levels of serum IL-31 in chronic spontaneous urticaria. Exp Dermatol. (2010) 19:464–6. doi: 10.1111/j.1600-0625.2010.01067.x
79. Bodoor K, Al-Qarqaz F, Heis LA, Alfaqih MA, Oweis AO, Almomani R, et al. IL-33/13 axis and IL-4/31 axis play distinct roles in inflammatory process and itch in psoriasis and atopic dermatitis. Clin Cosmet Investig Dermatol. (2020) 13:419–24. doi: 10.2147/CCID.S257647
80. Altrichter S, Hawro T, Hanel K, Czaja K, Luscher B, Maurer M, et al. Successful omalizumab treatment in chronic spontaneous urticaria is associated with lowering of serum IL-31 levels. J Eur Acad Dermatol Venereol. (2016) 30:454–5. doi: 10.1111/jdv.12831
81. Nemoto O, Furue M, Nakagawa H, Shiramoto M, Hanada R, Matsuki S, et al. The first trial of CIM331, a humanized antihuman interleukin-31 receptor A antibody, in healthy volunteers and patients with atopic dermatitis to evaluate safety, tolerability and pharmacokinetics of a single dose in a randomized, double-blind, placebo-controlled study. Br J Dermatol. (2016) 174:296–304. doi: 10.1111/bjd.14207
82. Ruzicka T, Mihara R. Anti-interleukin-31 receptor A antibody for atopic dermatitis. N Engl J Med. (2017) 376:2093. doi: 10.1056/NEJMoa1606490
83. Kabashima K, Furue M, Hanifin JM, Pulka G, Wollenberg A, Galus R, et al. Nemolizumab in patients with moderate-to-severe atopic dermatitis: randomized, phase II, long-term extension study. J Allergy Clin Immunol. (2018) 142:1121–30.e7. doi: 10.1016/j.jaci.2018.03.018
84. Richards CD, Gandhi R, Botelho F, Ho L, Paolini JF. Oncostatin M induction of monocyte chemoattractant protein 1 is inhibited by anti-oncostatin M receptor beta monoclonal antibody KPL-716. Acta Derm Venereol. (2020) 100:adv00197. doi: 10.2340/00015555-3505
85. He R, Geha RS. Thymic stromal lymphopoietin. Ann N Y Acad Sci. (2010) 1183:13–24. doi: 10.1111/j.1749-6632.2009.05128.x
86. Ziegler SF, Roan F, Bell BD, Stoklasek TA, Kitajima M, Han H. The biology of thymic stromal lymphopoietin (TSLP). Adv Pharmacol. (2013) 66:129–55. doi: 10.1016/B978-0-12-404717-4.00004-4
87. Zhong J, Sharma J, Raju R, Palapetta SM, Prasad TS, Huang TC, et al. TSLP signaling pathway map: a platform for analysis of TSLP-mediated signaling. Database. (2014) 2014:bau007. doi: 10.1093/database/bau007
88. Park LS, Martin U, Garka K, Gliniak B, Di Santo JP, Muller W, et al. Cloning of the murine thymic stromal lymphopoietin (TSLP) receptor: Formation of a functional heteromeric complex requires interleukin 7 receptor. J Exp Med. (2000) 192:659–70. doi: 10.1084/jem.192.5.659
89. Sokol CL, Barton GM, Farr AG, Medzhitov R. A mechanism for the initiation of allergen-induced T helper type 2 responses. Nat Immunol. (2008) 9:310–8. doi: 10.1038/ni1558
90. Cook EB, Stahl JL, Schwantes EA, Fox KE, Mathur SK. IL-3 and TNFalpha increase thymic stromal lymphopoietin receptor (TSLPR) expression on eosinophils and enhance TSLP-stimulated degranulation. Clin Mol Allergy. (2012) 10:8. doi: 10.1186/1476-7961-10-8
91. Kashyap M, Rochman Y, Spolski R, Samsel L, Leonard WJ. Thymic stromal lymphopoietin is produced by dendritic cells. J Immunol. (2011) 187:1207–11. doi: 10.4049/jimmunol.1100355
92. Kubo T, Kamekura R, Kumagai A, Kawata K, Yamashita K, Mitsuhashi Y, et al. DeltaNp63 controls a TLR3-mediated mechanism that abundantly provides thymic stromal lymphopoietin in atopic dermatitis. PLoS ONE. (2014) 9:e105498. doi: 10.1371/journal.pone.0105498
93. Allakhverdi Z, Comeau MR, Jessup HK, Yoon BR, Brewer A, Chartier S, et al. Thymic stromal lymphopoietin is released by human epithelial cells in response to microbes, trauma, or inflammation and potently activates mast cells. J Exp Med. (2007) 204:253–8. doi: 10.1084/jem.20062211
94. Hirano R, Hasegawa S, Hashimoto K, Haneda Y, Ohsaki A, Ichiyama T. Human thymic stromal lymphopoietin enhances expression of CD80 in human CD14+ monocytes/macrophages. Inflamm Res. (2011) 60:605–10. doi: 10.1007/s00011-011-0310-0
95. Ying S, O'Connor B, Ratoff J, Meng Q, Fang C, Cousins D, et al. Expression and cellular provenance of thymic stromal lymphopoietin and chemokines in patients with severe asthma and chronic obstructive pulmonary disease. J Immunol. (2008) 181:2790–8. doi: 10.4049/jimmunol.181.4.2790
96. Tatsuno K, Fujiyama T, Yamaguchi H, Waki M, Tokura Y. TSLP directly interacts with skin-homing Th2 cells highly expressing its receptor to enhance IL-4 production in atopic dermatitis. J Invest Dermatol. (2015) 135:3017–24. doi: 10.1038/jid.2015.318
97. Astrakhan A, Omori M, Nguyen T, Becker-Herman S, Iseki M, Aye T, et al. Local increase in thymic stromal lymphopoietin induces systemic alterations in B cell development. Nat Immunol. (2007) 8:522–31. doi: 10.1038/ni1452
98. Wilson SR, The L, Batia LM, Beattie K, Katibah GE, McClain SP, et al. The epithelial cell-derived atopic dermatitis cytokine TSLP activates neurons to induce itch. Cell. (2013) 155:285–95. doi: 10.1016/j.cell.2013.08.057
99. Tu HY, Chen X, Li J. [Signal transduction in respiratory syncytial virus infection-induced thymic stromal lymphopoietin expression in human epithelial cells]. Nan Fang Yi Ke Da Xue Xue Bao. (2007) 27:1581–3.
100. Nakamura Y, Miyata M, Ohba T, Ando T, Hatsushika K, Suenaga F, et al. Cigarette smoke extract induces thymic stromal lymphopoietin expression, leading to T(H)2-type immune responses and airway inflammation. J Allergy Clin Immunol. (2008) 122:1208–14. doi: 10.1016/j.jaci.2008.09.022
101. Lee HC, Ziegler SF. Inducible expression of the proallergic cytokine thymic stromal lymphopoietin in airway epithelial cells is controlled by NFkappaB. Proc Natl Acad Sci USA. (2007) 104:914–9. doi: 10.1073/pnas.0607305104
102. Lee EB, Kim KW, Hong JY, Jee HM, Sohn MH, Kim KE. Increased serum thymic stromal lymphopoietin in children with atopic dermatitis. Pediatr Allergy Immunol. (2010) 21:e457–60. doi: 10.1111/j.1399-3038.2009.00919.x
103. Nygaard U, Hvid M, Johansen C, Buchner M, Folster-Holst R, Deleuran M, et al. TSLP, IL-31, IL-33 and sST2 are new biomarkers in endophenotypic profiling of adult and childhood atopic dermatitis. J Eur Acad Dermatol Venereol. (2016) 30:1930–8. doi: 10.1111/jdv.13679
104. Uller L, Leino M, Bedke N, Sammut D, Green B, Lau L, et al. Double-stranded RNA induces disproportionate expression of thymic stromal lymphopoietin versus interferon-beta in bronchial epithelial cells from donors with asthma. Thorax. (2010) 65:626–32. doi: 10.1136/thx.2009.125930
105. Sherrill JD, Gao PS, Stucke EM, Blanchard C, Collins MH, Putnam PE, et al. Variants of thymic stromal lymphopoietin and its receptor associate with eosinophilic esophagitis. J Allergy Clin Immunol. (2010) 126:160–5.e3. doi: 10.1016/j.jaci.2010.04.037
106. Fang C, Siew LQ, Corrigan CJ, Ying S. The role of thymic stromal lymphopoietin in allergic inflammation and chronic obstructive pulmonary disease. Arch Immunol Ther Exp. (2010) 58:81–90. doi: 10.1007/s00005-010-0064-3
107. Varricchi G, Pecoraro A, Marone G, Criscuolo G, Spadaro G, Genovese A, et al. Thymic stromal lymphopoietin isoforms, inflammatory disorders, and cancer. Front Immunol. (2018) 9:1595. doi: 10.3389/fimmu.2018.01595
108. Alysandratos KD, Angelidou A, Vasiadi M, Zhang B, Kalogeromitros D, Katsarou-Katsari A, et al. Increased affected skin gene expression and serum levels of thymic stromal lymphopoietin in atopic dermatitis. Ann Allergy Asthma Immunol. (2010) 105:403–4. doi: 10.1016/j.anai.2010.09.017
109. Yoo J, Omori M, Gyarmati D, Zhou B, Aye T, Brewer A, et al. Spontaneous atopic dermatitis in mice expressing an inducible thymic stromal lymphopoietin transgene specifically in the skin. J Exp Med. (2005) 202:541–9. doi: 10.1084/jem.20041503
110. Li M, Messaddeq N, Teletin M, Pasquali JL, Metzger D, Chambon P. Retinoid X receptor ablation in adult mouse keratinocytes generates an atopic dermatitis triggered by thymic stromal lymphopoietin. Proc Natl Acad Sci USA. (2005) 102:14795–800. doi: 10.1073/pnas.0507385102
111. Simpson EL, Parnes JR, She D, Crouch S, Rees W, Mo M, et al. Tezepelumab, an anti-thymic stromal lymphopoietin monoclonal antibody, in the treatment of moderate to severe atopic dermatitis: A randomized phase 2a clinical trial. J Am Acad Dermatol. (2019) 80:1013–21. doi: 10.1016/j.jaad.2018.11.059
112. Nakajima S, Kabata H, Kabashima K, Asano K. Anti-TSLP antibodies: targeting a master regulator of type 2 immune responses. Allergol Int. (2020) 69:197–203. doi: 10.1016/j.alit.2020.01.001
113. Fitoussi J, Virassamynaik S, Callejon S, Weber S, Collet E, Scalia J, et al. Inhibition of thymic stromal lymphopoietin production to improve pruritus and quality of life in infants and children with atopic dermatitis. J Cosmet Dermatol. (2020) 19:2061–9. doi: 10.1111/jocd.13515
114. Kawabata A, Kuroda R, Hollenberg MD. [Physiology of protease-activated receptors (PARs): involvement of PARs in digestive functions]. Nihon Yakurigaku Zasshi. (1999) 114(Suppl. 1):173P−9. doi: 10.1254/fpj.114.supplement_173
115. Compton SJ, Renaux B, Wijesuriya SJ, Hollenberg MD. Glycosylation and the activation of proteinase-activated receptor 2 [PAR(2)] by human mast cell tryptase. Br J Pharmacol. (2001) 134:705–18. doi: 10.1038/sj.bjp.0704303
116. Adams MN, Ramachandran R, Yau MK, Suen JY, Fairlie DP, Hollenberg MD, et al. Structure, function and pathophysiology of protease activated receptors. Pharmacol Ther. (2011) 130:248–82. doi: 10.1016/j.pharmthera.2011.01.003
117. Akiyama T, Lerner EA, Carstens E. Protease-activated receptors and itch. Handb Exp Pharmacol. (2015) 226:219–35. doi: 10.1007/978-3-662-44605-8_13
118. Gieseler F, Ungefroren H, Settmacher U, Hollenberg MD, Kaufmann R. Proteinase-activated receptors (PARs) - focus on receptor-receptor-interactions and their physiological and pathophysiological impact. Cell Commun Signal. (2013) 11:86. doi: 10.1186/1478-811X-11-86
119. Buhl T, Ikoma A, Kempkes C, Cevikbas F, Sulk M, Buddenkotte J, et al. Protease-activated receptor-2 regulates neuro-epidermal communication in atopic dermatitis. Front Immunol. (2020) 11:1740. doi: 10.3389/fimmu.2020.01740
120. Steinhoff M, Neisius U, Ikoma A, Fartasch M, Heyer G, Skov PS, et al. Proteinase-activated receptor-2 mediates itch: a novel pathway for pruritus in human skin. J Neurosci. (2003) 23:6176–80. doi: 10.1523/JNEUROSCI.23-15-06176.2003
121. Steinhoff M, Buddenkotte J, Shpacovitch V, Rattenholl A, Moormann C, Vergnolle N, et al. Proteinase-activated receptors: transducers of proteinase-mediated signaling in inflammation and immune response. Endocr Rev. (2005) 26:1–43. doi: 10.1210/er.2003-0025
122. Lee SE, Jeong SK, Lee SH. Protease and protease-activated receptor-2 signaling in the pathogenesis of atopic dermatitis. Yonsei Med J. (2010) 51:808–22. doi: 10.3349/ymj.2010.51.6.808
123. Kempkes C, Buddenkotte J, Cevikbas F, Buhl T, Steinhoff M. Role of PAR-2 in neuroimmune communication and itch. In: Carstens E, Akiyama T, editors. Itch: Mechanisms and Treatment. Boca Raton, FL: Frontiers in Neuroscience (2014).
124. Steinhoff M, Corvera CU, Thoma MS, Kong W, McAlpine BE, Caughey GH, et al. Proteinase-activated receptor-2 in human skin: tissue distribution and activation of keratinocytes by mast cell tryptase. Exp Dermatol. (1999) 8:282–94. doi: 10.1111/j.1600-0625.1999.tb00383.x
125. Colognato R, Slupsky JR, Jendrach M, Burysek L, Syrovets T, Simmet T. Differential expression and regulation of protease-activated receptors in human peripheral monocytes and monocyte-derived antigen-presenting cells. Blood. (2003) 102:2645–52. doi: 10.1182/blood-2002-08-2497
126. Shrestha Palikhe N, Nahirney D, Laratta C, Gandhi VD, Vethanayagam D, Bhutani M, et al. Increased Protease-activated receptor-2 (PAR-2) expression on CD14++CD16+ peripheral blood monocytes of patients with severe asthma. PLoS ONE. (2015) 10:e0144500. doi: 10.1371/journal.pone.0144500
127. Ge S, Li T, Yao Q, Yan H, Huiyun Z, Zheng Y, et al. Expression of proteinase-activated receptor (PAR)-2 in monocytes from allergic patients and potential molecular mechanism. Cell Biol Toxicol. (2016) 32:529–42. doi: 10.1007/s10565-016-9353-x
128. D'Andrea MR, Rogahn CJ, Andrade-Gordon P. Localization of protease-activated receptors-1 and−2 in human mast cells: indications for an amplified mast cell degranulation cascade. Biotech Histochem. (2000) 75:85–90. doi: 10.3109/10520290009064152
129. He SH, Xie H, He YS. [Effect of a proteinase-activated receptor-2 (PAR-2) agonist on tryptase release from human mast cells]. Sheng Li Xue Bao. (2002) 54:531–4.
130. Wang H, He S. Induction of lactoferrin and IL-8 release from human neutrophils by tryptic enzymes via proteinase activated receptor-2. Cell Biol Int. (2006) 30:688–97. doi: 10.1016/j.cellbi.2006.04.007
131. Steinhoff M, Vergnolle N, Young SH, Tognetto M, Amadesi S, Ennes HS, et al. Agonists of proteinase-activated receptor 2 induce inflammation by a neurogenic mechanism. Nat Med. (2000) 6:151–8. doi: 10.1038/72247
132. Shpacovitch V, Feld M, Bunnett NW, Steinhoff M. Protease-activated receptors: novel PARtners in innate immunity. Trends Immunol. (2007) 28:541–50. doi: 10.1016/j.it.2007.09.001
133. Steinhoff M, Ständer S, Seeliger S, Ansel JC, Schmelz M, Luger T. Modern aspects of cutaneous neurogenic inflammation. Arch Dermatol. (2003) 139:1479–88. doi: 10.1001/archderm.139.11.1479
134. Amadesi S, Nie J, Vergnolle N, Cottrell GS, Grady EF, Trevisani M, et al. Protease-activated receptor 2 sensitizes the capsaicin receptor transient receptor potential vanilloid receptor 1 to induce hyperalgesia. J Neurosci. (2004) 24:4300–12. doi: 10.1523/JNEUROSCI.5679-03.2004
135. Davidson S, Giesler GJ. The multiple pathways for itch and their interactions with pain. Trends Neurosci. (2010) 33:550–8. doi: 10.1016/j.tins.2010.09.002
136. Gouin O, L'Herondelle K, Buscaglia P, Le Gall-Ianotto C, Philippe R, Legoux N, et al. Major role for TRPV1 and InsP3R in PAR2-elicited inflammatory mediator production in differentiated human keratinocytes. J Invest Dermatol. (2018) 138:1564–72. doi: 10.1016/j.jid.2018.01.034
137. Schön M, Behmenburg C, Denzer D, Schon MP. Pathogenic function of IL-1 beta in psoriasiform skin lesions of flaky skin (fsn/fsn) mice. Clin Exp Immunol. (2001) 123:505–10. doi: 10.1046/j.1365-2249.2001.01421.x
138. Sereflican B, Goksugur N, Bugdayci G, Polat M, Haydar Parlak A. Serum visfatin, adiponectin, and tumor necrosis factor alpha (TNF-alpha) levels in patients with psoriasis and their correlation with disease severity. Acta Dermatovenerol Croat. (2016) 24:13–9.
139. Hebert HL, Bowes J, Smith RL, McHugh NJ, Barker J, Griffiths CEM, et al. Polymorphisms in IL-1B distinguish between psoriasis of early and late onset. J Invest Dermatol. (2014) 134:1459–62. doi: 10.1038/jid.2013.485
140. Moniaga CS, Jeong SK, Egawa G, Nakajima S, Hara-Chikuma M, Jeon JE, et al. Protease activity enhances production of thymic stromal lymphopoietin and basophil accumulation in flaky tail mice. Am J Pathol. (2013) 182:841–51. doi: 10.1016/j.ajpath.2012.11.039
141. Indra AK. Epidermal TSLP: a trigger factor for pathogenesis of atopic dermatitis. Expert Rev Proteomics. (2013) 10:309–11. doi: 10.1586/14789450.2013.814881
142. Barr TP, Garzia C, Guha S, Fletcher EK, Nguyen N, Wieschhaus AJ, et al. PAR2 pepducin-based suppression of inflammation and itch in atopic dermatitis models. J Invest Dermatol. (2019) 139:412–21. doi: 10.1016/j.jid.2018.08.019
143. Pennefather JN, Lecci A, Candenas ML, Patak E, Pinto FM, Maggi CA. Tachykinins and tachykinin receptors: a growing family. Life Sci. (2004) 74:1445–63. doi: 10.1016/j.lfs.2003.09.039
144. Steinhoff MS, von Mentzer B, Geppetti P, Pothoulakis C, Bunnett NW. Tachykinins and their receptors: contributions to physiological control and the mechanisms of disease. Physiol Rev. (2014) 94:265–301. doi: 10.1152/physrev.00031.2013
145. Ständer S, Luger TA. NK-1 antagonists and itch. Handb Exp Pharmacol. (2015) 226:237–55. doi: 10.1007/978-3-662-44605-8_14
146. Pojawa-Golab M, Jaworecka K, Reich A. NK-1 receptor antagonists and pruritus: review of current literature. Dermatol Ther. (2019) 9:391–405. doi: 10.1007/s13555-019-0305-2
147. Yosipovitch G, Rosen JD, Hashimoto T. Itch: From mechanism to (novel) therapeutic approaches. J Allergy Clin Immunol. (2018) 142:1375–90. doi: 10.1016/j.jaci.2018.09.005
148. Marriott I, Bost KL. Expression of authentic substance P receptors in murine and human dendritic cells. J Neuroimmunol. (2001) 114:131–41. doi: 10.1016/S0165-5728(00)00466-5
149. Raap M, Rudrich U, Ständer S, Gehring M, Kapp A, Raap U. Substance P activates human eosinophils. Exp Dermatol. (2015) 24:557–9. doi: 10.1111/exd.12717
150. van der Kleij HP, Ma D, Redegeld FA, Kraneveld AD, Nijkamp FP, Bienenstock J. Functional expression of neurokinin 1 receptors on mast cells induced by IL-4 and stem cell factor. J Immunol. (2003) 171:2074–9. doi: 10.4049/jimmunol.171.4.2074
151. Ho WZ, Lai JP, Zhu XH, Uvaydova M, Douglas SD. Human monocytes and macrophages express substance P and neurokinin-1 receptor. J Immunol. (1997) 159:5654–60.
152. Lai JP, Douglas SD, Ho WZ. Human lymphocytes express substance P and its receptor. J Neuroimmunol. (1998) 86:80–6. doi: 10.1016/S0165-5728(98)00025-3
153. Morelli AE, Sumpter TL, Rojas-Canales DM, Bandyopadhyay M, Chen Z, Tkacheva O, et al. Neurokinin-1 receptor signaling is required for efficient Ca(2+) flux in T-cell-receptor-activated T cells. Cell Rep. (2020) 30:3448–65.e8. doi: 10.1016/j.celrep.2020.02.054
154. Liu JY, Hu JH, Zhu QG, Li FQ, Sun HJ. Substance P receptor expression in human skin keratinocytes and fibroblasts. Br J Dermatol. (2006) 155:657–62. doi: 10.1111/j.1365-2133.2006.07408.x
155. Roggenkamp D, Kopnick S, Stab F, Wenck H, Schmelz M, Neufang G. Epidermal nerve fibers modulate keratinocyte growth via neuropeptide signaling in an innervated skin model. J Invest Dermatol. (2013) 133:1620–8. doi: 10.1038/jid.2012.464
156. Schmelz M, Schmidt R, Bickel A, Handwerker HO, Torebjork HE. Specific C-receptors for itch in human skin. J Neurosci. (1997) 17:8003–8. doi: 10.1523/JNEUROSCI.17-20-08003.1997
157. Scholzen T, Armstrong CA, Bunnett NW, Luger TA, Olerud JE, Ansel JC. Neuropeptides in the skin: interactions between the neuroendocrine and the skin immune systems. Exp Dermatol. (1998) 7:81–96. doi: 10.1111/j.1600-0625.1998.tb00307.x
158. Ständer S, Yosipovitch G. Substance P and neurokinin 1 receptor are new targets for the treatment of chronic pruritus. Br J Dermatol. (2019) 181:932–8. doi: 10.1111/bjd.18025
159. Chang SE, Han SS, Jung HJ, Choi JH. Neuropeptides and their receptors in psoriatic skin in relation to pruritus. Br J Dermatol. (2007) 156:1272–7. doi: 10.1111/j.1365-2133.2007.07935.x
160. Amatya B, El-Nour H, Holst M, Theodorsson E, Nordlind K. Expression of tachykinins and their receptors in plaque psoriasis with pruritus. Br J Dermatol. (2011) 164:1023–9. doi: 10.1111/j.1365-2133.2011.10241.x
161. Akiyama T, Nguyen T, Curtis E, Nishida K, Devireddy J, Delahanty J, et al. A central role for spinal dorsal horn neurons that express neurokinin-1 receptors in chronic itch. Pain. (2015) 156:1240–6. doi: 10.1097/j.pain.0000000000000172
162. Zheng W, Wang J, Zhu W, Xu C, He S. Upregulated expression of substance P in basophils of the patients with chronic spontaneous urticaria: induction of histamine release and basophil accumulation by substance P. Cell Biol Toxicol. (2016) 32:217–28. doi: 10.1007/s10565-016-9330-4
163. Zhang Z, Zheng W, Xie H, Chai R, Wang J, Zhang H, et al. Up-regulated expression of substance P in CD8(+) T cells and NK1R on monocytes of atopic dermatitis. J Transl Med. (2017) 15:93. doi: 10.1186/s12967-017-1196-6
164. Nattkemper LA, Tey HL, Valdes-Rodriguez R, Lee H, Mollanazar NK, Albornoz C, et al. The genetics of chronic itch: gene expression in the skin of patients with atopic dermatitis and psoriasis with severe itch. J Invest Dermatol. (2018) 138:1311–7. doi: 10.1016/j.jid.2017.12.029
165. Salomon J, Baran E. The role of selected neuropeptides in pathogenesis of atopic dermatitis. J Eur Acad Dermatol Venereol. (2008) 22:223–8. doi: 10.1111/j.1468-3083.2007.02399.x
166. Hosokawa C, Takeuchi S, Furue M. Severity scores, itch scores and plasma substance P levels in atopic dermatitis treated with standard topical therapy with oral olopatadine hydrochloride. J Dermatol. (2009) 36:185–90. doi: 10.1111/j.1346-8138.2009.00621.x
167. Lee JH, Cho SH. Korean red ginseng extract ameliorates skin lesions in NC/Nga mice: an atopic dermatitis model. J Ethnopharmacol. (2011) 133:810–7. doi: 10.1016/j.jep.2010.11.020
168. Tsianakas A, Zeidler C, Riepe C, Borowski M, Forner C, Gerss J, et al. Aprepitant in anti-histamine-refractory chronic nodular prurigo: a multicentre, randomized, double-blind, placebo-controlled, cross-over, phase-II trial (APREPRU). Acta Derm Venereol. (2019) 99:379–85. doi: 10.2340/00015555-3120
169. Lönndahl L, Holst M, Bradley M, Killasli H, Heilborn J, Hall MA, et al. Substance P Antagonist Aprepitant Shows No Additive Effect Compared With Standardized Topical Treatment Alone In Patients With Atopic Dermatitis. Acta Derm Venereol. (2018) 98:324–8. doi: 10.2340/00015555-2852
170. Yosipovitch G, Ständer S, Kerby MB, Larrick JW, Perlman AJ, Schnipper EF, et al. Serlopitant for the treatment of chronic pruritus: results of a randomized, multicenter, placebo-controlled phase 2 clinical trial. J Am Acad Dermatol. (2018) 78:882–91.e10. doi: 10.1016/j.jaad.2018.02.030
171. Ständer S, Spellman MC, Kwon P, Yosipovitch G. The NK1 receptor antagonist serlopitant for treatment of chronic pruritus. Expert Opin Investig Drugs. (2019) 28:659–66. doi: 10.1080/13543784.2019.1638910
172. Reszke R, Krajewski P, Szepietowski JC. Emerging therapeutic options for chronic pruritus. Am J Clin Dermatol. (2020) 21:601–18. doi: 10.1007/s40257-020-00534-y
173. Pariser DM, Bagel J, Lebwohl M, Yosipovitch G, Chien E, Spellman MC. Serlopitant for psoriatic pruritus: a phase 2 randomized, double-blind, placebo-controlled clinical trial. J Am Acad Dermatol. (2020) 82:1314–20. doi: 10.1016/j.jaad.2020.01.056
174. Welsh SE, Xiao C, Kaden AR, Brzezynski JL, Mohrman MA, Wang J, et al. Neurokinin-1 receptor antagonist tradipitant has mixed effects on itch in atopic dermatitis: results from EPIONE, a randomized clinical trial. J Eur Acad Dermatol Venereol. (2020). doi: 10.1101/2020.06.21.20136911. [Epub ahead of print].
175. McNeil BD, Pundir P, Meeker S, Han L, Undem BJ, Kulka M, et al. Identification of a mast-cell-specific receptor crucial for pseudo-allergic drug reactions. Nature. (2015) 519:237–41. doi: 10.1038/nature14022
176. Azimi E, Reddy VB, Shade KC, Anthony RM, Talbot S, Pereira PJS, et al. Dual action of neurokinin-1 antagonists on Mas-related GPCRs. JCI Insight. (2016) 1:e89362. doi: 10.1172/jci.insight.89362
177. Bischoff SC, Zwahlen R, Stucki M, Mullner G, de Weck AL, Stadler BM, et al. Basophil histamine release and leukotriene production in response to anti-IgE and anti-IgE receptor antibodies. Comparison of normal subjects and patients with urticaria, atopic dermatitis or bronchial asthma. Int Arch Allergy Immunol. (1996) 110:261–71. doi: 10.1159/000237297
178. Ikoma A, Rukwied R, Ständer S, Steinhoff M, Miyachi Y, Schmelz M. Neuronal sensitization for histamine-induced itch in lesional skin of patients with atopic dermatitis. Arch Dermatol. (2003) 139:1455–8. doi: 10.1001/archderm.139.11.1455
179. Parsons ME, Ganellin CR. Histamine and its receptors. Br J Pharmacol. (2006) 147(Suppl. 1):S127–35. doi: 10.1038/sj.bjp.0706440
180. Rossbach K, Nassenstein C, Gschwandtner M, Schnell D, Sander K, Seifert R, et al. Histamine H1, H3 and H4 receptors are involved in pruritus. Neuroscience. (2011) 190:89–102. doi: 10.1016/j.neuroscience.2011.06.002
181. Toth BI, Szallasi A, Biro T. Transient receptor potential channels and itch: how deep should we scratch? Handb Exp Pharmacol. (2015) 226:89–133. doi: 10.1007/978-3-662-44605-8_6
182. Connelly WM, Shenton FC, Lethbridge N, Leurs R, Waldvogel HJ, Faull RL, et al. The histamine H4 receptor is functionally expressed on neurons in the mammalian CNS. Br J Pharmacol. (2009) 157:55–63. doi: 10.1111/j.1476-5381.2009.00227.x
183. Giustizieri ML, Albanesi C, Fluhr J, Gisondi P, Norgauer J, Girolomoni G. H1 histamine receptor mediates inflammatory responses in human keratinocytes. J Allergy Clin Immunol. (2004) 114:1176–82. doi: 10.1016/j.jaci.2004.07.054
184. Glatzer F, Gschwandtner M, Ehling S, Rossbach K, Janik K, Klos A, et al. Histamine induces proliferation in keratinocytes from patients with atopic dermatitis through the histamine 4 receptor. J Allergy Clin Immunol. (2013) 132:1358–67. doi: 10.1016/j.jaci.2013.06.023
185. Mommert S, Kleiner S, Gehring M, Eiz-Vesper B, Stark H, Gutzmer R, et al. Human basophil chemotaxis and activation are regulated via the histamine H4 receptor. Allergy. (2016) 71:1264–73. doi: 10.1111/all.12875
186. Idzko M, la Sala A, Ferrari D, Panther E, Herouy Y, Dichmann S, et al. Expression and function of histamine receptors in human monocyte-derived dendritic cells. J Allergy Clin Immunol. (2002) 109:839–46. doi: 10.1067/mai.2002.124044
187. Damaj BB, Becerra CB, Esber HJ, Wen Y, Maghazachi AA. Functional expression of H4 histamine receptor in human natural killer cells, monocytes, and dendritic cells. J Immunol. (2007) 179:7907–15. doi: 10.4049/jimmunol.179.11.7907
188. Thangam EB, Jemima EA, Singh H, Baig MS, Khan M, Mathias CB, et al. The role of histamine and histamine receptors in mast cell-mediated allergy and inflammation: the hunt for new therapeutic targets. Front Immunol. (2018) 9:1873. doi: 10.3389/fimmu.2018.01873
189. Jutel M, Watanabe T, Klunker S, Akdis M, Thomet OA, Malolepszy J, et al. Histamine regulates T-cell and antibody responses by differential expression of H1 and H2 receptors. Nature. (2001) 413:420–5. doi: 10.1038/35096564
190. Gutzmer R, Mommert S, Gschwandtner M, Zwingmann K, Stark H, Werfel T. The histamine H4 receptor is functionally expressed on T(H)2 cells. J Allergy Clin Immunol. (2009) 123:619–25. doi: 10.1016/j.jaci.2008.12.1110
191. Mahapatra S, Albrecht M, Behrens B, Jirmo A, Behrens G, Hartwig C, et al. Delineating the role of histamine-1- and−4-receptors in a mouse model of Th2-dependent antigen-specific skin inflammation. PLoS ONE. (2014) 9:e87296. doi: 10.1371/journal.pone.0087296
192. Panula P, Chazot PL, Cowart M, Gutzmer R, Leurs R, Liu WL, et al. International Union of basic and clinical pharmacology. XCVIII. Histamine receptors. Pharmacol Rev. (2015) 67:601–55. doi: 10.1124/pr.114.010249
193. Werfel T, Layton G, Yeadon M, Whitlock L, Osterloh I, Jimenez P, et al. Efficacy and safety of the histamine H4 receptor antagonist ZPL-3893787 in patients with atopic dermatitis. J Allergy Clin Immunol. (2019) 143:1830–7 e4. doi: 10.1016/j.jaci.2018.07.047
194. Novak N, Peng WM, Bieber T, Akdis C. FcepsilonRI stimulation promotes the differentiation of histamine receptor 1-expressing inflammatory macrophages. Allergy. (2013) 68:454–61. doi: 10.1111/all.12109
195. Fukunaga A, Oda Y, Washio K, Omori T, Kakei Y, Hide M, et al. Efficacy of switching to bilastine, a histamine H1 receptor antagonist, in patients with chronic spontaneous urticaria (H1-SWITCH): study protocol for a randomized controlled trial. Trials. (2020) 21:23. doi: 10.1186/s13063-019-3878-2
196. Schaper-Gerhardt K, Rossbach K, Nikolouli E, Werfel T, Gutzmer R, Mommert S. The role of the histamine H4 receptor in atopic dermatitis and psoriasis. Br J Pharmacol. (2020) 177:490–502. doi: 10.1111/bph.14550
197. Thurmond RL, Kazerouni K, Chaplan SR, Greenspan AJ. Antihistamines and itch. Handb Exp Pharmacol. (2015) 226:257–90. doi: 10.1007/978-3-662-44605-8_15
198. Yamaura K, Oda M, Suwa E, Suzuki M, Sato H, Ueno K. Expression of histamine H4 receptor in human epidermal tissues and attenuation of experimental pruritus using H4 receptor antagonist. J Toxicol Sci. (2009) 34:427–31. doi: 10.2131/jts.34.427
199. Suwa E, Yamaura K, Oda M, Namiki T, Ueno K. Histamine H(4) receptor antagonist reduces dermal inflammation and pruritus in a hapten-induced experimental model. Eur J Pharmacol. (2011) 667:383–8. doi: 10.1016/j.ejphar.2011.05.037
200. Rossbach K, Schaper K, Kloth C, Gutzmer R, Werfel T, Kietzmann M, et al. Histamine H4 receptor knockout mice display reduced inflammation in a chronic model of atopic dermatitis. Allergy. (2016) 71:189–97. doi: 10.1111/all.12779
201. Ohsawa Y, Hirasawa N. The role of histamine H1 and H4 receptors in atopic dermatitis: from basic research to clinical study. Allergol Int. (2014) 63:533–42. doi: 10.2332/allergolint.13-RA-0675
202. Murata Y, Song M, Kikuchi H, Hisamichi K, Xu XL, Greenspan A, et al. Phase 2a, randomized, double-blind, placebo-controlled, multicenter, parallel-group study of a H4 R-antagonist (JNJ-39758979) in Japanese adults with moderate atopic dermatitis. J Dermatol. (2015) 42:129–39. doi: 10.1111/1346-8138.12726
203. Ohsawa Y, Hirasawa N. The antagonism of histamine H1 and H4 receptors ameliorates chronic allergic dermatitis via anti-pruritic and anti-inflammatory effects in NC/Nga mice. Allergy. (2012) 67:1014–22. doi: 10.1111/j.1398-9995.2012.02854.x
204. Liu Q, Tang Z, Surdenikova L, Kim S, Patel KN, Kim A, et al. Sensory neuron-specific GPCR Mrgprs are itch receptors mediating chloroquine-induced pruritus. Cell. (2009) 139:1353–65. doi: 10.1016/j.cell.2009.11.034
205. Bader M, Alenina N, Andrade-Navarro MA, Santos RA. MAS and its related G protein-coupled receptors, Mrgprs. Pharmacol Rev. (2014) 66:1080–105. doi: 10.1124/pr.113.008136
206. Wedi B, Gehring M, Kapp A. The pseudoallergen receptor MRGPRX2 on peripheral blood basophils and eosinophils: expression and function. Allergy. (2020) 75:2229–42. doi: 10.1111/all.14213
207. Steinhoff M, Buddenkotte J, Lerner EA. Role of mast cells and basophils in pruritus. Immunol Rev. (2018) 282:248–64. doi: 10.1111/imr.12635
209. Han L, Ma C, Liu Q, Weng HJ, Cui Y, Tang Z, et al. A subpopulation of nociceptors specifically linked to itch. Nat Neurosci. (2013) 16:174–82. doi: 10.1038/nn.3289
210. Qu L, Fan N, Ma C, Wang T, Han L, Fu K, et al. Enhanced excitability of MRGPRA3- and MRGPRD-positive nociceptors in a model of inflammatory itch and pain. Brain. (2014) 137:1039–50. doi: 10.1093/brain/awu007
211. Lee WJ, Kim YS, Shim WS. Korean Red Ginseng extract and ginsenoside Rg3 have anti-pruritic effects on chloroquine-induced itch by inhibition of MrgprA3/TRPA1-mediated pathway. J Ginseng Res. (2018) 42:470–5. doi: 10.1016/j.jgr.2017.05.004
212. Jang Y, Lee WJ, Hong GS, Shim WS. Red ginseng extract blocks histamine-dependent itch by inhibition of H1R/TRPV1 pathway in sensory neurons. J Ginseng Res. (2015) 39:257–64. doi: 10.1016/j.jgr.2015.01.004
213. Zhu Y, Hanson CE, Liu Q, Han L. Mrgprs activation is required for chronic itch conditions in mice. Itch. (2017) 2:e9. doi: 10.1097/itx.0000000000000009
214. Sikand P, Dong X, LaMotte RH. BAM8-22 peptide produces itch and nociceptive sensations in humans independent of histamine release. J Neurosci. (2011) 31:7563–7. doi: 10.1523/JNEUROSCI.1192-11.2011
215. Liu Q, Weng HJ, Patel KN, Tang Z, Bai H, Steinhoff M, et al. The distinct roles of two GPCRs, MrgprC11 and PAR2, in itch and hyperalgesia. Sci Signal. (2011) 4:ra45. doi: 10.1126/scisignal.2001925
216. Liu Q, Dong X. The role of the Mrgpr receptor family in itch. Handb Exp Pharmacol. (2015) 226:71–88. doi: 10.1007/978-3-662-44605-8_5
217. Kühn H, Kolkhir P, Babina M, Dull M, Frischbutter S, Fok JS, et al. Mas-related G protein-coupled receptor X2 and its activators in dermatologic allergies. J Allergy Clin Immunol. (2020). doi: 10.1016/j.jaci.2020.08.027. [Epub ahead of print].
218. Corbiere A, Loste A, Gaudenzio N. MRGPRX2 sensing of cationic compounds - a bridge between nociception and skin diseases? Exp Dermatol. (2020) 1–8. doi: 10.1111/exd.14222
219. Wu H, Zeng M, Cho EY, Jiang W, Sha O. The origin, expression, function and future research focus of a G protein-coupled receptor, mas-related gene X2 (MrgX2). Prog Histochem Cytochem. (2015) 50:11–7. doi: 10.1016/j.proghi.2015.06.001
220. Subramanian H, Gupta K, Ali H. Roles of Mas-related G protein-coupled receptor X2 on mast cell-mediated host defense, pseudoallergic drug reactions, and chronic inflammatory diseases. J Allergy Clin Immunol. (2016) 138:700–10. doi: 10.1016/j.jaci.2016.04.051
221. Fujisawa D, Kashiwakura J, Kita H, Kikukawa Y, Fujitani Y, Sasaki-Sakamoto T, et al. Expression of Mas-related gene X2 on mast cells is upregulated in the skin of patients with severe chronic urticaria. J Allergy Clin Immunol. (2014) 134:622–33.e9. doi: 10.1016/j.jaci.2014.05.004
222. Green DP, Limjunyawong N, Gour N, Pundir P, Dong X. A mast-cell-specific receptor mediates neurogenic inflammation and pain. Neuron. (2019) 101:412–20.e3. doi: 10.1016/j.neuron.2019.01.012
223. Martin-Zanca D, Hughes SH, Barbacid M. A human oncogene formed by the fusion of truncated tropomyosin and protein tyrosine kinase sequences. Nature. (1986) 319:743–8. doi: 10.1038/319743a0
224. Martin-Zanca D, Oskam R, Mitra G, Copeland T, Barbacid M. Molecular and biochemical characterization of the human trk proto-oncogene. Mol Cell Biol. (1989) 9:24–33. doi: 10.1128/MCB.9.1.24
225. Barbacid M. Structural and functional properties of the TRK family of neurotrophin receptors. Ann N Y Acad Sci. (1995) 766:442–58. doi: 10.1111/j.1749-6632.1995.tb26693.x
226. Skaper SD. The neurotrophin family of neurotrophic factors: an overview. Methods Mol Biol. (2012) 846:1–12. doi: 10.1007/978-1-61779-536-7_1
227. Nockher WA, Renz H. Neurotrophins in allergic diseases: from neuronal growth factors to intercellular signaling molecules. J Allergy Clin Immunol. (2006) 117:583–9. doi: 10.1016/j.jaci.2005.11.049
228. Matsumura S, Terao M, Murota H, Katayama I. Th2 cytokines enhance TrkA expression, upregulate proliferation, and downregulate differentiation of keratinocytes. J Dermatol Sci. (2015) 78:215–23. doi: 10.1016/j.jdermsci.2015.02.021
229. Sin AZ, Roche EM, Togias A, Lichtenstein LM, Schroeder JT. Nerve growth factor or IL-3 induces more IL-13 production from basophils of allergic subjects than from basophils of nonallergic subjects. J Allergy Clin Immunol. (2001) 108:387–93. doi: 10.1067/mai.2001.117459
230. Caroleo MC, Costa N, Bracci-Laudiero L, Aloe L. Human monocyte/macrophages activate by exposure to LPS overexpress NGF and NGF receptors. J Neuroimmunol. (2001) 113:193–201. doi: 10.1016/S0165-5728(00)00441-0
231. Nilsson G, Forsberg-Nilsson K, Xiang Z, Hallbook F, Nilsson K, Metcalfe DD. Human mast cells express functional TrkA and are a source of nerve growth factor. Eur J Immunol. (1997) 27:2295–301. doi: 10.1002/eji.1830270925
232. Groneberg DA, Serowka F, Peckenschneider N, Artuc M, Grutzkau A, Fischer A, et al. Gene expression and regulation of nerve growth factor in atopic dermatitis mast cells and the human mast cell line-1. J Neuroimmunol. (2005) 161:87–92. doi: 10.1016/j.jneuroim.2004.12.019
233. Noga O, Englmann C, Hanf G, Grutzkau A, Guhl S, Kunkel G. Activation of the specific neurotrophin receptors TrkA, TrkB and TrkC influences the function of eosinophils. Clin Exp Allergy. (2002) 32:1348–54. doi: 10.1046/j.1365-2745.2002.01442.x
234. Lindsay RM, Shooter EM, Radeke MJ, Misko TP, Dechant G, Thoenen H, et al. Nerve growth factor regulates expression of the nerve growth factor receptor gene in adult sensory neurons. Eur J Neurosci. (1990) 2:389–96. doi: 10.1111/j.1460-9568.1990.tb00431.x
235. Ma L, Merenmies J, Parada LF. Molecular characterization of the TrkA/NGF receptor minimal enhancer reveals regulation by multiple cis elements to drive embryonic neuron expression. Development. (2000) 127:3777–88.
236. Freund-Michel V, Frossard N. The nerve growth factor and its receptors in airway inflammatory diseases. Pharmacol Ther. (2008) 117:52–76. doi: 10.1016/j.pharmthera.2007.07.003
237. Manti S, Brown P, Perez MK, Piedimonte G. The role of neurotrophins in inflammation and allergy. Vitam Horm. (2017) 104:313–41. doi: 10.1016/bs.vh.2016.10.010
238. Bürgi B, Otten UH, Ochensberger B, Rihs S, Heese K, Ehrhard PB, et al. Basophil priming by neurotrophic factors. Activation through the trk receptor. J Immunol. (1996) 157:5582–8.
239. Ehrhard PB, Ganter U, Stalder A, Bauer J, Otten U. Expression of functional trk protooncogene in human monocytes. Proc Natl Acad Sci USA. (1993) 90:5423–7. doi: 10.1073/pnas.90.12.5423
240. Ehrhard PB, Erb P, Graumann U, Otten U. Expression of nerve growth factor and nerve growth factor receptor tyrosine kinase Trk in activated CD4-positive T-cell clones. Proc Natl Acad Sci USA. (1993) 90:10984–8. doi: 10.1073/pnas.90.23.10984
241. Torcia M, Bracci-Laudiero L, Lucibello M, Nencioni L, Labardi D, Rubartelli A, et al. Nerve growth factor is an autocrine survival factor for memory B lymphocytes. Cell. (1996) 85:345–56. doi: 10.1016/S0092-8674(00)81113-7
242. Dou YC, Hagstromer L, Emtestam L, Johansson O. Increased nerve growth factor and its receptors in atopic dermatitis: an immunohistochemical study. Arch Dermatol Res. (2006) 298:31–7. doi: 10.1007/s00403-006-0657-1
243. Bonini S, Lambiase A, Bonini S, Angelucci F, Magrini L, Manni L, et al. Circulating nerve growth factor levels are increased in humans with allergic diseases and asthma. Proc Natl Acad Sci USA. (1996) 93:10955–60. doi: 10.1073/pnas.93.20.10955
244. Hodeib A, El-Samad ZA, Hanafy H, El-Latief AA, El-Bendary A, Abu-Raya A. Nerve growth factor, neuropeptides and cutaneous nerves in atopic dermatitis. Indian J Dermatol. (2010) 55:135–9. doi: 10.4103/0019-5154.62735
245. Kubanov AA, Katunina OR, Chikin VV. Expression of neuropeptides, neurotrophins, and neurotransmitters in the skin of patients with atopic dermatitis and psoriasis. Bull Exp Biol Med. (2015) 159:318–22. doi: 10.1007/s10517-015-2951-4
246. Folster-Holst R, Papakonstantinou E, Rudrich U, Buchner M, Pite H, Gehring M, et al. Childhood atopic dermatitis-brain-derived neurotrophic factor correlates with serum eosinophil cationic protein and disease severity. Allergy. (2016) 71:1062–5. doi: 10.1111/all.12916
247. Guseva D, Rudrich U, Kotnik N, Gehring M, Patsinakidis N, Agelopoulos K, et al. Neuronal branching of sensory neurons is associated with BDNF-positive eosinophils in atopic dermatitis. Clin Exp Allergy. (2020) 50:577–84. doi: 10.1111/cea.13560
248. Hon KL, Lam MC, Leung TF, Kam WY, Lee KC, Li MC, et al. Nocturnal wrist movements are correlated with objective clinical scores and plasma chemokine levels in children with atopic dermatitis. Br J Dermatol. (2006) 154:629–35. doi: 10.1111/j.1365-2133.2006.07213.x
249. Di Marco E, Mathor M, Bondanza S, Cutuli N, Marchisio PC, Cancedda R, et al. Nerve growth factor binds to normal human keratinocytes through high and low affinity receptors and stimulates their growth by a novel autocrine loop. J Biol Chem. (1993) 268:22838–46. doi: 10.1016/S0021-9258(18)41604-3
250. Horiuchi Y, Bae S, Katayama I. Nerve growth factor (NGF) and epidermal nerve fibers in atopic dermatitis model NC/Nga mice. J Dermatol Sci. (2005) 39:56–8. doi: 10.1016/j.jdermsci.2005.03.012
251. Tominaga M, Ozawa S, Ogawa H, Takamori K. A hypothetical mechanism of intraepidermal neurite formation in NC/Nga mice with atopic dermatitis. J Dermatol Sci. (2007) 46:199–210. doi: 10.1016/j.jdermsci.2007.02.002
252. Takano N, Sakurai T, Kurachi M. Effects of anti-nerve growth factor antibody on symptoms in the NC/Nga mouse, an atopic dermatitis model. J Pharmacol Sci. (2005) 99:277–86. doi: 10.1254/jphs.FP0050564
253. Stein AT, Ufret-Vincenty CA, Hua L, Santana LF, Gordon SE. Phosphoinositide 3-kinase binds to TRPV1 and mediates NGF-stimulated TRPV1 trafficking to the plasma membrane. J Gen Physiol. (2006) 128:509–22. doi: 10.1085/jgp.200609576
254. Anand U, Otto WR, Casula MA, Day NC, Davis JB, Bountra C, et al. The effect of neurotrophic factors on morphology, TRPV1 expression and capsaicin responses of cultured human DRG sensory neurons. Neurosci Lett. (2006) 399:51–6. doi: 10.1016/j.neulet.2006.01.046
255. Roblin D, Yosipovitch G, Boyce B, Robinson J, Sandy J, Mainero V, et al. Topical TrkA kinase inhibitor CT327 is an effective, novel therapy for the treatment of pruritus due to psoriasis: results from experimental studies, and efficacy and safety of CT327 in a phase 2b Clinical trial in patients with psoriasis. Acta Derm Venereol. (2015) 95:542–8. doi: 10.2340/00015555-2047
Keywords: pruritus, inflammation, neuro-immune, sensory neurons, skin disease, atopic dermatitis (AD), psoriasis, chronic spontaneous urticaria (CSU)
Citation: Ruppenstein A, Limberg MM, Loser K, Kremer AE, Homey B and Raap U (2021) Involvement of Neuro-Immune Interactions in Pruritus With Special Focus on Receptor Expressions. Front. Med. 8:627985. doi: 10.3389/fmed.2021.627985
Received: 10 November 2020; Accepted: 27 January 2021;
Published: 18 February 2021.
Edited by:
Sonja Ständer, University Hospital Münster, GermanyReviewed by:
Ethan Lerner, Massachusetts General Hospital, United StatesLaurent Misery, Université de Bretagne Occidentale, France
Simone Garcovich, Catholic University of the Sacred Heart, Italy
Copyright © 2021 Ruppenstein, Limberg, Loser, Kremer, Homey and Raap. This is an open-access article distributed under the terms of the Creative Commons Attribution License (CC BY). The use, distribution or reproduction in other forums is permitted, provided the original author(s) and the copyright owner(s) are credited and that the original publication in this journal is cited, in accordance with accepted academic practice. No use, distribution or reproduction is permitted which does not comply with these terms.
*Correspondence: Ulrike Raap, cmFhcC51bHJpa2VAa2xpbmlrdW0tb2xkZW5idXJnLmRl