- 1Alameen Hospital, Taif, Saudi Arabia
- 2Mansoura Nephrology and Dialysis Unit, Internal Medicine Department, College of Medicine, Mansoura University, Mansoura, Egypt
- 3College of Medicine, Taif University, Taif, Saudi Arabia
- 4Internal Medicine Department, King Abdulaziz Hospital, Jeddah, Saudi Arabia
- 5General Department of Medical Services, Security Forces Hospital, Mecca, Saudi Arabia
- 6Anesthesiology Department, Faculty of Medicine, Ain Shams University, Cairo, Egypt
- 7Radiology Department, Faculty of Medicine, Cairo University, Giza, Egypt
- 8Albbassia Chest Hospital, Cairo, Egypt
- 9Department of Physiology, Faculty of Veterinary Medicine, Kafrelsheikh University, Kafrelsheikh, Egypt
Background: SARS-CoV-2, the causative agent of COVID-19, continues to cause a worldwide pandemic, with more than 147 million being affected globally as of this writing. People's responses to COVID-19 range from asymptomatic to severe, and the disease is sometimes fatal. Its severity is affected by different factors and comorbidities of the infected patients. Living at a high altitude could be another factor that affects the severity of the disease in infected patients.
Methods: In the present study, we have analyzed the clinical, laboratory, and radiological findings of COVID-19-infected patients in Taif, a high-altitude region of Saudi Arabia. In addition, we compared matched diseased subjects to those living at sea level. We hypothesized that people living in high-altitude locations are prone to develop a more severe form of COVID-19 than those living at sea level.
Results: Age and a high Charlson comorbidity score were associated with increased numbers of intensive care unit (ICU) admissions and mortality among COVID-19 patients. These ICU admissions and fatalities were found mainly in patients with comorbidities. Rates of leukocytosis, neutrophilia, higher D-dimer, ferritin, and highly sensitive C-reactive protein (CRP) were significantly higher in ICU patients. CRP was the most independent of the laboratory biomarkers found to be potential predictors of death. COVID-19 patients who live at higher altitude developed a less severe form of the disease and had a lower mortality rate, in comparison to matched subjects living at sea level.
Conclusion: CRP and Charlson comorbidity scores can be considered predictive of disease severity. People living at higher altitudes developed less severe forms of COVID-19 disease than those living at sea level, due to a not-yet-known mechanism.
Introduction
Humans worldwide have been besieged by the relentless spread of the SARS-CoV-2 pandemic. As of this writing, SARS-CoV-2 laboratory-confirmed cases have exceeded 147 million globally. Patients infected with this newly discovered coronavirus can be affected by either asymptomatic or symptomatic forms of the disease. The latter includes fever, sore throat, cough, shortness of breath, and a loss of smell and taste sensation. Some patients develop severe pneumonia and acute respiratory distress syndrome with fatal consequences (1). Older people and those with other health problems such as diabetes, cancer, lung diseases, and heart disease are the most vulnerable groups afflicted by the severe form of COVID-19. Early diagnosis and treatment of infected patients are important to avoid a complicated form of the disease and to prevent further spread of the virus to uninfected subjects (2).
Lymphopenia, elevated inflammatory markers such as high C-reactive protein (CRP), ferritin, and elevated D-dimer, in association with abnormal radiological findings in both chest X-rays and CT scans, are suggestive of COVID-19 (3). The COVID-19 Reporting and Data System (CO-RADS) is used as an indicator of the pulmonary engagement of COVID-19 (4). However, nucleic acid testing on respiratory tract specimens is the only laboratory confirmation of SARS-CoV-2. The angiotensin-converting enzyme 2 (ACE2) receptor has been recognized as the binding site of SARS-CoV-2 in the target cells (5). ACE2 plays a major role in patients with hypertension, CVD, obesity, and type 2 diabetes by opposing the harmful effects of the renin–angiotensin–aldosterone system (RAAS); therefore, the ACE/ACE2 ratio could be a good indicator of SARS-CoV-2 disease progression. There is a direct proportional relationship between COVID-19 infectivity and virulence that depends on the level of ACE2 expression on target cells (6, 7).
High-altitude-linked hypoxia has been reported to cause systemic inflammation associated with hypercoagulability and promotes venous thromboembolism development (8–10). Exposure to high altitudes has been reported to predispose patients to a series of thromboembolic events, due to increased blood hypercoagulability (11, 12). Platelet counts have been observed to rise within 1 week of exposure to high altitudes (13). Moreover, exposure to high altitudes for 1 year has been shown to increase plasma fibrinogen concentration by as much as 61% (14). In addition, a recent study showed that the mean platelet count is higher among high-altitude dwellers compared to low-altitude dwellers in Saudi Arabia, predisposing them for hypercoagulability (15).
The development of coagulation test abnormalities seen in COVID-19-infected patients is most likely a result of the profound inflammatory response caused by the virus and its associated coagulopathy (16). Based on these data, the combined causes of hypoxia and increased platelet counts in high-altitude dwellers may leave these people prone to more severe attacks of disseminated intravascular coagulation if they develop a COVID-19 infection. However, a recent study has reported less severe manifestations of the disease in people living in high-altitude regions (17).
The current study aimed to analyze the clinical characteristics, laboratory findings, risk factors, associated comorbidities, radiological findings, and outcomes in patients admitted to the hospital with a confirmed COVID-19 infection in Taif, Saudi Arabia. In addition, we also aimed to investigate the effect of high altitude on the severity of the disease, based on the clinical outcomes and disease severity in the hospitalized COVID-19 patients from one high-altitude city, Taif, and a sea-level city, Jeddah.
Methods
Patients and Time Frame
An observational retrospective study was conducted on a total of 760 COVID-19 laboratory-confirmed cases between April and September 2020 in Taif, Saudi Arabia. All patients were at least 18 years old and had confirmed positive polymerase chain reaction (PCR) results for COVID-19. Data from subjects were collected from the Alameen and King Faisal Hospitals, in Taif. An additional 208 subjects were recruited from Jeddah (which is at sea level), from patients at King Abdulaziz Hospital. The Jeddah data (n = 208) were compared to matched patients from Taif (n = 272/760). Matching patients' data from Taif were selected from the original 760 subjects based on patients' ages and comorbidities.
Clinical and Laboratory Investigations
Initial investigations included complete blood count, highly sensitive CRP, and chest X-rays. CT chest scans were used as a routine investigation for most of the Taif patients during the early months of the epidemic. X-rays were performed on all patients, while the CT chest scans were only applied to 722/760 (95%) of the patients. Other investigations, such as D-dimer, ferritin, alanine aminotransferase, aspartate aminotransferase (AST), creatinine, international normalized ratio, prothrombin time, and partial thromboplastin time were also conducted. Supplemental oxygen therapy was used if patients' oxygen levels were <90%, to keep these levels between 92 and 96%. Patients who were admitted to an intensive care unit (ICU) were closely monitored for adequate hemodynamic support and fluid therapy, depending on both dynamic and static parameters. Patients who required supplemental oxygen were also given dexamethasone (6 mg daily), either orally or via intravenous injection.
The Charlson Comorbidity Index
The relationships among the severity of COVID-19, ICU admission, disease outcome, and associated comorbidities were assessed in patients from Taif. Twenty-nine points were assigned to different comorbidities: a history of myocardial infarction (one point), congestive heart failure (one point), peripheral vascular disease (one point), dementia (one point), chronic pulmonary disease (one point), rheumatologic disease (one point), peptic ulcer disease (one point), liver disease (three points), diabetes (two points), hemiplegia or paraplegia (two points), renal disease (two points), malignancy (six points), and HIV/AIDS (six points). The Charlson comorbidity index was used as previously described (18–20).
CO-RADS Classification
CO-RADS classification was used to classify patients from Taif according to their CT results (4). Those in the CO-RADS 1 group had a normal CT or showed non-infectious radiological changes, while those in CO-RADS 2 had a CT that showed changes relevant to non-COVID-19 infectious diseases. Those described as CO-RADS 3 showed an intermediate suspension of COVID-19, including widespread bronchopneumonia, lobar pneumonia, and ground-glass opacities (GGO) or septic emboli. Patients in CO-RADS 4 were cases in which COVID-19 was highly suspected. Unilateral GGO and multifocal consolidations without any other typical findings may be present in these patients' CT scans. A CO-RADS 5 classification is highly indicative for COVID-19, with CT showing multifocal GGO and consolidation. CO-RADS 6 was used to describe patients with a positive PCR with bilateral GGO.
Statistical Analysis
Data are reported as means and standard error of mean (SEM) or counts and percentages, as appropriate. Comparisons among groups were made using t tests, the Mann–Whitney U test, chi-square, or Fisher exact tests, as dictated by data type and distribution. A multiple regression analysis using independent variables determined the predictors of ICU admission. One-way analysis of variance (ANOVA) was used to test differences among more than two groups. Pearson's or Spearman's correlations were used to test the correlations among variables. A p < 0.05 was considered significant for all statistical analyses in this study. All analyses were performed using the Statistical Package for the Social Sciences (SPSS) version 24 for Windows (SPSS, Inc., Chicago, IL, USA).
Results
Demographic Data of Patients From Taif
Healthcare workers accounted for 6.4% of all patients. The detected comorbidities included diabetes (16.2%), hypertension (12%), chronic obstructive pulmonary disease (3%), chronic kidney disease (2.6%), peripheral vascular disease (2.6%), coronary artery disease (1.8%), congestive heart failure (1.4%), and pulmonary embolism (PE) (3.8%). The symptoms included fever (84.7%), cough (58.2%), shortness of breath (45.3%), GIT symptoms (25.4%), sore throat (10.8%), anosmia (20.9%), and loss of taste sensation (15.7%). Sixty-four (8.5%) patients were admitted to the ICU, and 13 (1.7%) patients died. Patients were classified into three groups: (i) recovered patients without ICU admission, 695 (91.5%); (ii) recovered patients after ICU admission 52 (6.8%) patients; and (iii) deceased patients, 13 (1.7%) patients (Table 1).
Age is a major factor in the severity of COVID-19, as greater ages were associated with increased ICU admission and mortality rates, with p < 0.0001. We found no significant differences between the patient subgroups for gender and nationality. Patients who were admitted to the ICU and/or who died showed significant histories of hypertension, diabetes, chronic kidney disease, peripheral vascular disease, and coronary artery disease, with a p value of 0.0001 for all. We observed no significant differences in most of the initial clinical presentations among the patient subgroups, though patients with a history of ICU admission had a longer duration of hospital length of stay, with a p value of < 0.0001. Charlson's comorbidity scores were significantly higher in patients admitted to the ICU and/or who died, with a p < 0.0001 (Table 1).
Patients' mean oxygen saturation percentage at the time of admission was 94.6%, and 45.1% of our patients showed absolute lymphocytopenia. The mean level of high-sensitivity CRP was 23.6 (3.8) mg/L, the mean ferritin level was 540 (47.7) ng/ml, and the mean D-dimer level was 0.7 (0.1) mcg/ml. Patients with a history of ICU admission and/or death showed significant hypoxia, with a p < 0.001. Leukocytosis and neutrophilia were found to be significant among patients admitted to the ICU and/or who died, in addition to significantly higher D-dimer, ferritin, and highly sensitive CRP levels, with a p < 0.0001 for all patients (Table 2).
A logistic regression analysis for patients admitted to an ICU showed that the level of highly sensitive CRP was the most independent predictor for death, with a p value of 0.003 (Table 3). Radiological investigations of our confirmed cases showed that 74.5% had positive results in a chest x-ray. By contrast, 95% of our patients had CT chest affections and were classified according to the CO-RADS classification system (Figure 1); 18.2% were CO-RADS 1, 3.8% were CO-RADS 2, 1.6% were CO-RADS 3, 2.9% were CO-RADS 4, and 68.6% were CO-RADS 5. There was a significant difference among patient subgroups regarding the chest CT and chest X-ray results, with p-values of 0.03 and 0.0001, respectively (Table 4).
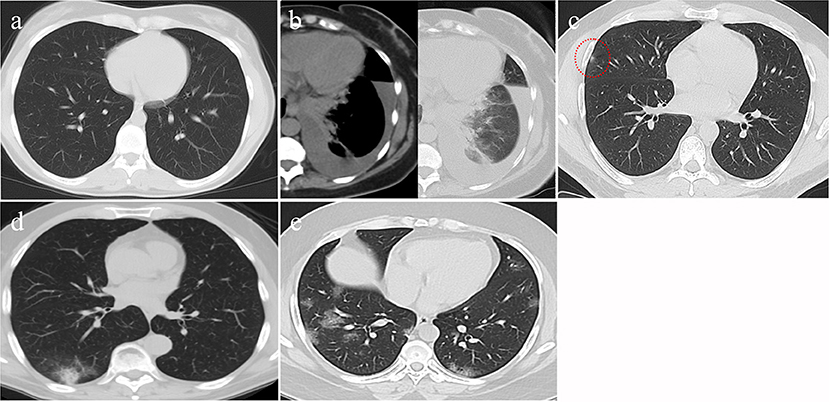
Figure 1. CT findings of different CO-RAD stages. (a) Normal CT findings, in keeping with CO-RAD1. (b) CT showed a left lower lobar patchy area of consolidation associated with moderate pleural effusion. This picture is suggestive of bacterial pneumonia (in keeping with CO-RAD2). Note that pleural effusion is very unlikely to occur with COVID-19 pneumonia. (c) A very tiny right middle lobar patchy area of faint ground glass opacity (red dashed circle), in keeping with CO-RAD3. (d) CT chest showed right lower lobar patchy area of ground glass veiling, mounting to consolidation, in keeping with CO-RADS 4. (e) Bilateral, mainly peripheral and lower lobar, small patchy areas of ground glass opacity, in keeping with CO-RADS 5.
Pearson's correlation was conducted between the different parameters with disease severity and radiological findings. Items that only showed significant correlation was presented in Table 5. Age, AST, D-dimer, ferritin, and CRP showed highly significant correlation (P > 0.01) with both disease severity and radiological findings.
Comparison of Matched Patients in High Altitude and Sea Level
Upon comparing the clinical data of matched patients from Taif and Jeddah, we noted that SARS-CoV-2 infection rates were found to be significantly higher in males than in females, and among subjects between the ages of 21–40 years and 41–60 years in both the high-altitude (Taif) and sea-level (Jeddah) regions (Table 6).
Interestingly, we detected a significantly higher percentage of recovery in the high-altitude region, in comparison with those living at sea level. Importantly, the death rate in the sea level region was nearly threefold that reported in the high-altitude region (Table 7).
Contrary to the above results, significantly higher rates of deep vein thrombosis, PE, MI, and acute large vessel occlusion were reported in the high-altitude region, compared to the sea-level region. However, the incidence of fever, cough, shortness of breath, and acute respiratory distress syndrome (ARDS) during the course of the disease were higher in Jeddah than in Taif. Importantly, the incidence of comorbidities such as diabetes mellitus, hypertension, asthma, ischemic stroke, and coronary disease showed no significant differences between the high-altitude and sea-level regions (Table 7).
Discussion
In our study, as reported in other studies, patient age was a critical risk factor for ICU admission, with elderly patients being the most frequently admitted. In addition, comorbidities such as hypertension, diabetes mellitus, chronic kidney disease, ischemic heart disease, peripheral vascular disease, and malignancies were found to correlate with an increased probability of ICU admission and fatal consequences. Our findings also agree with previously available studies in this respect (21–25).
Patients who were admitted to the ICU and those who died showed significantly higher Charlson comorbidity scores than other patients did. These results were similar to previously published data from Denmark (26). Our results indicated that the Charlson comorbidity index is a valid indicator for COVID-19 patients with poor outcomes, in concordance with previous studies (27, 28).
In this study, we found no significant relationship between the presence of fever, cough, shortness of breath, GIT symptoms, and being a healthcare worker or with ICU admission or mortality. Our results did not match other studies (29, 30) that found a high fever to be associated with COVID-19 progression; we attribute this distance to differences in the studies' designs.
In contrast to the above, sore throat and a loss of smell and taste sensation were associated with a good prognosis among confirmed cases of COVID-19. Our results agree with a previous study that reported a correlation between smell loss and decreased hospitalization, intubation, and acute respiratory distress syndrome (31). In addition, another study also found that patients with a sore throat had better outcomes than others (32). However, there is no available explanation for these correlations.
Our study showed that low oxygen saturation at the time of admission was associated with poor outcomes. Hypoxia was found to be a powerful independent predictor of mortality in COVID-19 patients (33). Previous studies showed a strong relationship between hypoxia and both increasing the inflammatory response (34) and induction of viral replication (35, 36). It might also play a role in the pathogenesis of hypercoagulation (37).
In our study, we found that the presence of leukocytosis, neutrophilia, high levels of highly sensitive CRP, ferritin, D-dimer, and AST at the time of admission were associated with patients' deterioration, ICU admission, and death. Additionally, a significant correlation was found between such parameters and both the disease severity. These results were in line with previous studies that reported a significant association between disease progression and poor outcomes, with increased levels of inflammatory biomarkers and leukocytosis (38–40).
Logistic regression analysis showed that highly sensitive CRP was the most significant independent predictor of death among COVID-19 patients who were admitted to an ICU. This finding corresponds with previous reports that confirmed that CRP is the major determinant of disease severity in COVID-19 patients (41, 42). It may also highlight the role of secondary infection in the deterioration of patients' health status. Interestingly, the use of steroids was reported to be associated with secondary infection (43, 44).
In all, 18.2% of our patients showed normal chest CT appearance. Chest CTs were conducted early for most of our patients, and it is well-known that chest CTs continue to be normal in the first 4–5 days of infection (45). Meanwhile, among those who were admitted to the ICU and who later died, the chest CT results were significantly positive. At present, chest CT is not routinely ordered, and its use is limited to specific cases, especially in patients with false negative PCR (46, 47). However, we found a significant correlation of the radiological findings and the severity of the disease. This finding was also in accordance with recent findings that found similar correlation (48, 49).
We observed a lower mortality rate among people living at a high altitude, in comparison to those living at sea level. Another study has suggested a reduced severity of SARS-CoV-2 in high-altitude-dwelling patients (17); however, there are no current data explaining the mechanism involved in such an effect. The number of ACE2 receptors in target cells affects the susceptibility of such cells to SARS-CoV-2 infection. One speculation is the assumption that high-altitude inhabitants possess hypoxia-induced downregulation of ACE2. The RAAS is the expression of both ACE1 and ACE2 in negative feedback manner. During hypoxia, ACE2 is downregulated in the concomitant upregulation of ACE1 (50). Accordingly, decreased expressions of ACE2 in the pulmonary endothelial lining among high-altitude inhabitants is assumed to reduce the amplitude of virus replication (17). This speculation was argued strongly by Pun et al. (51), who presented conflicting results regarding ACE2. Their argument was augmented by many previous studies that reported the upregulation of ACE2 via hypoxia (52–54). Furthermore, deciphering hypoxia as a potential factor that alters the ACE2 expression is still obscure and needs further investigation. It is possible that some other, still-unknown mechanism renders high-altitude inhabitants more resistant to the severe consequences of COVID-19, compared to their sea-level-dwelling contemporaries.
Conclusion
Fever, cough, and shortness of breath are the main clinical presentations in COVID-19 patients. Age, multiple comorbidities, a high Charlson comorbidity score, leukocytosis, neutrophilia, higher levels of highly sensitive CRP, ferritin, D-dimer, and AST are associated with poor outcomes among COVID-19 patients. High-altitude inhabitants showed reduced disease severity, in comparison with sea-level-dwelling patients.
Data Availability Statement
The original contributions presented in the study are included in the article/supplementary material, further inquiries can be directed to the corresponding author/s.
Ethics Statement
The studies involving human participants were reviewed and approved by Research and Studies Department, Directorate of Health Affairs, Taif, Saudi Arabia (NO. 397 on 23/07/2020). The patients/participants provided their written informed consent to participate in this study.
Author Contributions
AF, AR, MR, RMMA, and AAA collected and analyzed the data from Alamin hospital. RMA, SAA, TA, and SMA collected and analyzed data from Jeddah. MA, ZI, DN, ANA, and DA analyzed the data and prepared the initial draft. DN, DA, ASA-M and AAA conceived the study protocol and critically revised the manuscript. All authors shared in data analysis and discussing and approved the final form of the manuscript.
Conflict of Interest
The authors declare that the research was conducted in the absence of any commercial or financial relationships that could be construed as a potential conflict of interest.
Acknowledgments
The researchers gratefully acknowledge the Taif University Researchers Supporting Program (TURSP-2020/233) of Taif University, Taif, Saudi Arabia.
References
1. McIntosh K, Hirsch M, Bloom A. Coronavirus Disease 2019 (COVID-19). Up-to-Date. (2020). Available online at: https://www.uptodate.com/contents/middle-east-respiratory-syndrome-coronavirus-treatment-and-preventionUpdatedFebruary24,2020. (accessed February 22, 2020).
2. Carlos WG, Dela Cruz CS, Cao B, Pasnick S, Jamil S. Novel Wuhan (2019-nCoV) coronavirus. Am J Respir Crit Care Med. (2020) 201:P7–P8. doi: 10.1164/rccm.2014P7
3. Guan W-J, Ni Z-Y, Hu Y, Liang W-H, Ou C-Q, He J-X, et al. Clinical characteristics of coronavirus disease 2019 in China. N Engl J Med. (2020) 382:1708–20. doi: 10.1056/NEJMoa2002032
4. Prokop M, Van Everdingen W, Van Rees Vellinga T, Quarles Van Ufford H, Stöger L, Beenen L, et al. CO-RADS: a categorical CT assessment scheme for patients suspected of having COVID-19-definition and evaluation. Radiology. (2020) 296:E97–104. doi: 10.1148/radiol.2020201473
5. Wan Y, Shang J, Graham R, Baric RS, Li F. Receptor recognition by the novel coronavirus from Wuhan: an analysis based on decade-long structural studies of SARS coronavirus. J Virol. (2020) 94:e00127–20. doi: 10.1128/JVI.00127-20
6. Ferreira AJ, Shenoy V, Qi Y, Fraga-Silva RA, Santos RA, Katovich MJ, et al. Angiotensin-converting enzyme 2 activation protects against hypertension-induced cardiac fibrosis involving extracellular signal-regulated kinases. Exp Physiol. (2011) 96:287–94. doi: 10.1113/expphysiol.2010.055277
7. Patel VB, Zhong JC, Grant MB, Oudit GY. Role of the ACE2/angiotensin 1-7 axis of the renin-angiotensin system in heart failure. Circ Res. (2016) 118:1313–26. doi: 10.1161/CIRCRESAHA.116.307708
8. Van Veen JJ, Makris M. Altitude and coagulation activation: does going high provoke thrombosis? Acta Haematol. (2008) 119:156–57. doi: 10.1159/000128045
9. Sabit R, Thomas P, Shale DJ, Collins P, Linnane SJ. The effects of hypoxia on markers of coagulation and systemic inflammation in patients with COPD. Chest. (2010) 138:47–51. doi: 10.1378/chest.09-2764
10. Brill A, Suidan GL, Wagner DD. Hypoxia, such as encountered at high altitude, promotes deep vein thrombosis in mice. J Thromb Haemost. (2013) 11:1773–75. doi: 10.1111/jth.12310
11. Schreijer AJ, Cannegieter SC, Rosendaal FR, Helmerhorst FM. A case of thrombosis at high altitude. Thromb Haem. (2005) 94:1104–5. doi: 10.1160/TH05-05-1104
12. Gupta N, Ashraf MZ. Exposure to high altitude: a risk factor for venous thromboembolism? Semin Thromb Hemost. (2012) 38:156–63. doi: 10.1055/s-0032-1301413
13. Hudson JG, Bowen AL, Navia P, Rios-Dalenz J, Pollard AJ, Williams D, et al. The effect of high altitude on platelet counts, thrombopoietin and erythropoietin levels in young Bolivian airmen visiting the Andes. Int J Biometeorol. (1999) 43:85–90. doi: 10.1007/s004840050120
14. Vij AG. Effect of prolonged stay at high altitude on platelet aggregation and fibrinogen levels. Platelets. (2009) 20:421–7. doi: 10.1080/09537100903116516
15. Algahtani FH, Alqahtany FS, Al-Shehri A, Abdelgader AM. Features and incidence of thromboembolic disease: a comparative study between high and low altitude dwellers in Saudi Arabia. Saudi J Biol Sci. (2020) 27:1632–6. doi: 10.1016/j.sjbs.2020.03.004
16. Connors JM, Levy JH. COVID-19 and its implications for thrombosis and anticoagulation. Blood. (2020) 135:2033–40. doi: 10.1182/blood.2020006000
17. Arias-Reyes C, Zubieta-Deurioste N, Poma-Machicao L, Aliaga-Raduan F, Carvajal-Rodriguez F, Dutschmann M, et al. Does the pathogenesis of SARS-CoV-2 virus decrease at high-altitude? Respir Physiol Neurobiol. (2020) 277:103443. doi: 10.1016/j.resp.2020.103443
18. Charlson ME, Pompei P, Ales KL, Mackenzie CR. A new method of classifying prognostic comorbidity in longitudinal studies: development and validation. J Chronic Dis. (1987) 40:373–83. doi: 10.1016/0021-9681(87)90171-8
19. Charlson M, Szatrowski TP, Peterson J, Gold J. Validation of a combined comorbidity index. J Clin Epidemiol. (1994) 47:1245–51. doi: 10.1016/0895-4356(94)90129-5
20. Quan H, Li B, Couris CM, Fushimi K, Graham P, Hider P, et al. Updating and validating the Charlson comorbidity index and score for risk adjustment in hospital discharge abstracts using data from 6 countries. Am J Epidemiol. (2011) 173:676–682. doi: 10.1093/aje/kwq433
21. Zheng Z, Peng F, Xu B, Zhao J, Liu H, Peng J, et al. Risk factors of critical & mortal COVID-19 cases: A systematic literature review and meta-analysis. J Infect 81, e16–e25. doi: 10.1016/j.jinf.2020.04.021
22. Huang C, Wang Y, Li X, Ren L, Zhao J, Hu Y, et al. Clinical features of patients infected with 2019 novel coronavirus in Wuhan, China. Lancet. (2020) 395:497–506. doi: 10.1016/S0140-6736(20)30183-5
23. Liu W, Tao ZW, Wang L, Yuan ML, Liu K, Zhou L, et al. Analysis of factors associated with disease outcomes in hospitalized patients with 2019 novel coronavirus disease. Chin Med J (Engl). (2020) 133:1032–8. doi: 10.1097/CM9.0000000000000775
24. Wang D, Hu B, Hu C, Zhu F, Liu X, Zhang J, et al. Clinical Characteristics of 138 Hospitalized Patients With 2019 Novel Coronavirus-Infected Pneumonia in Wuhan, China. Jama 323, 1061–1069. doi: 10.1001/jama.2020.1585
25. Zhou F, Yu T, Du R, Fan G, Liu Y, Liu Z, et al. Clinical course and risk factors for mortality of adult inpatients with COVID-19 in Wuhan, China: a retrospective cohort study. Lancet 395, 1054–1062. doi: 10.1016/S0140-6736(20)30566-3
26. Christensen DM, Strange JE, Gislason G, Torp-Pedersen C, Gerds T, Fosbøl E, et al. Charlson comorbidity index score and risk of severe outcome and death in danish COVID-19 patients. J Gen Intern Med 35, 2801–2803. doi: 10.1007/s11606-020-05991-z
27. Tuty Kuswardhani RA, Henrina J, Pranata R, Anthonius Lim M, Lawrensia S, Suastika K. Charlson comorbidity index and a composite of poor outcomes in COVID-19 patients: A systematic review and meta-analysis. Diabetes Metab Syndr 14, 2103–2109. doi: 10.1016/j.dsx.2020.10.022
28. Zhou W, Qin X, Hu X, Lu Y, Pan J. Prognosis models for severe and critical COVID-19 based on the Charlson and Elixhauser comorbidity indices. Int J Med Sci 17, 2257–2263. doi: 10.7150/ijms.50007
29. Chang MC, Park YK, Kim BO, Park D. Risk factors for disease progression in COVID-19 patients. BMC Infect Dis. (2020) 20:445. doi: 10.1186/s12879-020-05144-x
30. Rod JE, Oviedo-Trespalacios O, Cortes-Ramirez J. A brief-review of the risk factors for covid-19 severity. Rev Saude Publica. (2020) 54:60. doi: 10.11606/s1518-8787.2020054002481
31. Foster KJ, Jauregui E, Tajudeen B, Bishehsari F, Mahdavinia M. Smell loss is a prognostic factor for lower severity of coronavirus disease 2019. Ann Allergy Asthma Immunol. (2020) 125:481–3. doi: 10.1016/j.anai.2020.07.023
32. Kim SR, Nam SH, Kim YR. Risk factors on the progression to clinical outcomes of COVID-19 patients in south korea: using national data. Int J Environ Res Public Health. (2020) 17:1723884. doi: 10.3390/ijerph17238847
33. Somers VK, Kara T, Xie J. Progressive hypoxia: A pivotal pathophysiologic mechanism of COVID-19 pneumonia. Mayo Clin Proc 95, 2339–2342. doi: 10.1016/j.mayocp.2020.09.015
34. Eltzschig HK, Carmeliet P. Hypoxia and inflammation. N Engl J Med. (2011) 364:656–65. doi: 10.1056/NEJMra0910283
35. Vassilaki N, Kalliampakou KI, Kotta-Loizou I, Befani C, Liakos P, Simos G, et al. Low oxygen tension enhances hepatitis C virus replication. J Virol. (2013) 87:2935–48. doi: 10.1128/JVI.02534-12
36. López-Rodríguez DM, Kirillov V, Krug LT, Mesri EA, Andreansky S. A role of hypoxia-inducible factor 1 alpha in Murine Gammaherpesvirus 68 (MHV68) lytic replication and reactivation from latency. PLoS Pathog. (2019) 15:e1008192. doi: 10.1371/journal.ppat.1008192
37. Pilli VS, Datta A, Afreen S, Catalano D, Szabo G, Majumder R. Hypoxia downregulates protein S expression. Blood. (2018) 132:452–5. doi: 10.1182/blood-2018-04-841585
38. Cao G, Li P, Chen Y, Fang K, Chen B, Wang S, et al. A risk prediction model for evaluating the disease progression of COVID-19 pneumonia. Front Med (Lausanne). (2020) 7:556886. doi: 10.3389/fmed.2020.556886
39. Liu F, Li L, Xu M, Wu J, Luo D, Zhu Y, et al. Prognostic value of interleukin-6, C-reactive protein, and procalcitonin in patients with COVID-19. J Clin Virol. (2020) 127:104370. doi: 10.1016/j.jcv.2020.104370
40. Tan C, Huang Y, Shi F, Tan K, Ma Q, Chen Y, et al. C-reactive protein correlates with computed tomographic findings and predicts severe COVID-19 early. J Med Virol. (2020) 92:856–62. doi: 10.1002/jmv.25871
41. Malik P, Patel U, Mehta D, Patel N, Kelkar R, Akrmah M, et al. Biomarkers and outcomes of COVID-19 hospitalisations: systematic review and meta-analysis. BMJ Evid Based Med. (2020) bmjebm-2020-111536. doi: 10.1136/bmjebm-2020-111536
42. Sharifpour M, Rangaraju S, Liu M, Alabyad D, Nahab FB, Creel-Bulos CM, et al. C-Reactive protein as a prognostic indicator in hospitalized patients with COVID-19. PLoS One. (2020) 15:e0242400. doi: 10.1371/journal.pone.0242400
43. Obata R, Maeda T, Do DR, Kuno T. Increased secondary infection in COVID-19 patients treated with steroids in New York City. Jpn J Infect Dis. (2020). doi: 10.7883/yoken.JJID.2020.884
44. Ripa M, Galli L, Poli A, Oltolini C, Spagnuolo V, Mastrangelo A, et al. Secondary infections in patients hospitalized with COVID-19: incidence and predictive factors. Clin Microbiol Infect. (2020) 27:451–7. doi: 10.1016/j.cmi.2020.10.021
45. Adams HJA, Kwee TC, Yakar D, Hope MD, Kwee RM. Chest CT imaging signature of coronavirus disease 2019 infection: in pursuit of the scientific evidence. Chest. (2020) 158, 1885–95. doi: 10.1016/j.chest.2020.06.025
46. Pan F, Ye T, Sun P, Gui S, Liang B, Li L, et al. Time course of lung changes at chest ct during recovery from coronavirus disease 2019 (COVID-19). Radiology. (2020) 295:715–21. doi: 10.1148/radiol.2020200370
47. Rubin GD, Ryerson CJ, Haramati LB, Sverzellati N, Kanne JP, Raoof S, et al. The role of chest imaging in patient management during the COVID-19 pandemic: a multinational consensus statement from the fleischner society. Chest. (2020) 158:106–16. doi: 10.1016/j.chest.2020.04.003
48. Francone M, Iafrate F, Masci GM, Coco S, Cilia F, Manganaro L, et al. Chest CT score in COVID-19 patients: correlation with disease severity and short-term prognosis. Eur Radiol. (2020) 30:6808–17. doi: 10.1007/s00330-020-07033-y
49. Al-Smadi AS, Bhatnagar A, Ali R, Lewis N, Johnson S. Correlation of chest radiography findings with the severity and progression of COVID-19 pneumonia. Clin Imag. (2021) 71:17–23. doi: 10.1016/j.clinimag.2020.11.004
50. Zhang R, Wu Y, Zhao M, Liu C, Zhou L, Shen S, et al. Role of HIF-1alpha in the regulation ACE and ACE2 expression in hypoxic human pulmonary artery smooth muscle cells. Am J Physiol Lung Cell Mol Physiol. (2009) 297:L631–40. doi: 10.1152/ajplung.90415.2008
51. Pun M, Turner R, Strapazzon G, Brugger H, Swenson ER. Lower incidence of COVID-19 at high altitude: facts and confounders. High Alt Med Biol. (2020) 21:217–22. doi: 10.1089/ham.2020.0114
52. Hampl V, Herget J, Bíbová J, Banasová A, Husková Z, Vanourková Z, et al. Intrapulmonary activation of the angiotensin-converting enzyme type 2/angiotensin 1-7/G-protein-coupled Mas receptor axis attenuates pulmonary hypertension in Ren-2 transgenic rats exposed to chronic hypoxia. Physiol Res. (2015) 64:25–38. doi: 10.33549/physiolres.932861
53. Oarhe CI, Dang V, Dang M, Nguyen H, Gopallawa I, Gewolb IH, et al. (2015). Hyperoxia downregulates angiotensin-converting enzyme-2 in human fetal lung fibroblasts. Pediatric Res. (2015) 77:656–62. doi: 10.1038/pr.2015.27
Keywords: COVID–19, SARS-CoV-2, high-altitude, crp, CO-RADS classification, d-dimer
Citation: Abdelsalam M, Althaqafi RMM, Assiri SA, Althagafi TM, Althagafi SM, Fouda AY, Ramadan A, Rabah M, Ahmed RM, Ibrahim ZS, Nemenqani DM, Alghamdi AN, Al Aboud D, Abdel-Moneim AS and Alsulaimani AA (2021) Clinical and Laboratory Findings of COVID-19 in High-Altitude Inhabitants of Saudi Arabia. Front. Med. 8:670195. doi: 10.3389/fmed.2021.670195
Received: 20 February 2021; Accepted: 23 March 2021;
Published: 12 May 2021.
Edited by:
Reza Lashgari, Institute for Research in Fundamental Sciences, IranReviewed by:
Jawhar Gharbi, King Faisal University, Saudi ArabiaMajid Marjani, National Research Institute of Tuberculosis and Lung Diseases (NRITLD), Iran
Copyright © 2021 Abdelsalam, Althaqafi, Assiri, Althagafi, Althagafi, Fouda, Ramadan, Rabah, Ahmed, Ibrahim, Nemenqani, Alghamdi, Al Aboud, Abdel-Moneim and Alsulaimani. This is an open-access article distributed under the terms of the Creative Commons Attribution License (CC BY). The use, distribution or reproduction in other forums is permitted, provided the original author(s) and the copyright owner(s) are credited and that the original publication in this journal is cited, in accordance with accepted academic practice. No use, distribution or reproduction is permitted which does not comply with these terms.
*Correspondence: Ahmed S. Abdel-Moneim, YXNhQHR1LmVkdS5zYQ==