- Department of Nuclear Medicine, The First Hospital of Jilin University, Changchun, China
Background: To optimize [18F] 9-fluoropropyl-(+)-dihydrotetrabenazine (18F-FP-(+)-DTBZ) purification via solid-phase extraction (SPE) with combined cartridges to facilitate its widespread clinical application.
Methods: A modified SPE purification method, employing Sep-Pak PS-2 and Sep-Pak C18 cartridges, was used for the preparation of 18F-FP-(+)-DTBZ. This method was compared to the purification method of high-pressure liquid chromatography (HPLC) and SPE with one cartridge, following quality control test and positron emission tomography (PET) imaging in healthy volunteers and patients with parkinsn's disease (PD).
Results: A SPE purification method integrating Sep-Pak PS-2 and Sep-Pak C18 cartridges was implemented successfully. The retention time of 18F-FP-(+)-DTBZ purified by HPLC, SPE with Sep-Pak PS-2, SPE with Sep-Pak C18, and SPE with combined use of Sep-Pak PS-2 and Sep-Pak C18 cartridges was 8.7, 8.8, 8.7, and 8.9 min, respectively. Fewest impurity peak was detected in 18F-FP-(+)-DTBZ purified by the SPE with combined use of Sep-Pak PS-2 and Sep-Pak C18 cartridges. This modified SPE purification method provided a satisfactory radiochemical yield of 29 ± 1.8% with radiochemical purity >99% and shortened synthesis time to 27 min. The brain uptake of 18F-FP-(+)-DTBZ purified by the modified SPE was comparable to that purified by HPLC in both healthy volunteers and PD patients.
Conclusions: A SPE method integrating Sep-Pak PS-2 and Sep-Pak C18 cartridges for purification of 18F-FP-(+)-DTBZ may be highly suited to automatic synthesis for routine clinical applications, as it provides excellent radiochemical purity, high yield as well as operational simplicity.
Introduction
Vesicular monoamine transporter type 2 (VMAT2) plays critical role in the mechanism of packaging and transporting neurotransmitters from the cytosol into synaptic vesicle, verifying its status as an objective marker of nigrostriatal terminal integrity (1, 2). VMAT2 located on vesicle membranes in neurons and its activity largely affects the scale of dopamine release (3). The activity of the presynaptic monoaminergic binding site is less likely influenced by the medication or compensatory mechanisms, as it is active only when VMAT2-containing neurons are active (4). Therefore, it is a reliable and objective biomarker for exploring neurological and psychiatric disorders such as Parkinson disease (PD) (5), Alzheimer's disease and dementia with Lewy bodies (6), as well as endocrine system disease such as diabetes (7–9).
The imaging of VMAT2 has potential advantages such as better image quality and quantification, shorter time between tracer administration and scanning, shorter scan duration, and no requirement for prior blockade of the thyroid to prevent radioactive iodine uptake (10–15). To date, different kinds of agents for VMAT2 have been investigated to map VMAT2 distribution, and some of them have been tested in humans and achieved favorable results (16–18). For example, as early as in 1996, Frey et al. (19) found that PD patients had reduction in specific [11C]dihydrotetrabenazine (DTBZ) distribution volume in the putamen (−61%) and in the caudate nucleus (−43%). Similarly, regional specific uptake ratio of [18F] 9-fluoropropyl-(+)- DTBZ (18F-FP-(+)-DTBZ) of caudate, putamen, substantia nigra, and globus pallidus in the moderate and advanced PD patients were significantly lower than those in the healthy human subjects (16). More encouragingly, Li et al. (8) provided evidence that dysfunction of streptozotocin-diabetic retinas was detected by 18F-FP-(+)-DTBZ imaging at least 4 weeks earlier than other examinations such as electroretinogram, color fundus photography, and angiography. Generally, widespread use of this agent could facilitate the disease diagnosis and monitoring treatment response.
However, the published methods of purification of this agent cost a long preparation time, which limits its routine application for VMAT2 tracing. High-pressure liquid chromatography (HPLC) is a conventional method used for purification of 11C-labeled or 18F-labeled DTBZ to eliminate the pseudo-carrier. The procedure of this purification method was relatively complex, and the preparation of clinically useful radiotracer using HPLC lasted over 1 h. Subsequently, solid phase extraction (SPE) were adopted and the shortest synthesis time for 18F-FP-(+)-DTBZ using SPE was 40 min in the published studies (20, 21). Therefore, purification of 18F-FP-(+)-DTBZ is in need to improve in order to increase the application of this tracer. In the present study, we employed a SPE method with combined use of Sep-Pak PS-2 and Sep-Pak C18 cartridges to purify 18F-FP-(+)-DTBZ to maximize the final yield and simplify the purification process. With this method, 18F-FP-(+)-DTBZ can be expanded into the clinical setting to test the usefulness of 18F-FP-(+)-DTBZ in the diagnosis and monitoring of diseases.
Materials and Methods
Chemicals and Reagents
All reagents were commercially acquired and utilized without further purification unless otherwise stated. 4,7,13,16,21,24-Hexaoxa-1,10-diazabicyclo [8.8.8] hexacosane (K222, 98.0 %) was purchased from ABX (Germany). Anhydrous acetonitrile and potassium carbonate (K2CO3) were purchased from Sigma (America). All SPE cartridges were purchased from Waters Corporation (USA). To activate Sep-Pak QMA, Sep-Pak C18, and Sep-Pak PS-2, we flushed them with 5 mL absolute ethanol followed by 10 mL water.
Radiosynthesis of 18F-FP-(+)-DTBZ
Precursor of 18F-FP-(+)-DTBZ was synthesized in the College of Chemistry, Jilin University (Figure 1). 18F was produced in a cyclotron by 18O (p, n)18F nuclear reaction. 18F was passed into the CFN-MPS200 multi-functional synthesis module through the target transmission system and captured by the Sep-Pak QMA anion exchange column. The residual liquid was put into the oxygen water recovery unit. QMA was eluted with a 0.9mL K2.2.2 / K2CO3 mixture. Then, 18F was rinsed into the reaction bottle. The eluent was dried through successive evaporation with nitrogen (N2) flow (30 ml/min) at 100°C for 3 min to remove water and acetonitrile. Next, the residue was redried under the same N2 flow at 110°C for 2 min. A total of 1.0 mg TsOP-(+)-DTBZ precursor solution in anhydrous was introduced into the reaction tube. Then, radiofluorination was performed in the reaction bottle at 120°C for 12 min and cooled to 40°C. We transferred the mixture from the reaction bottle to the transfer bottle. The reaction bottle was cleaned using 5 mL 5% ethanol solution, which was then poured into the transfer bottle. The crude product solution purification by HPLC or SPE and the final solution in the collection bottle was passed through a Millex GV for sterilization and diluted to 10 mL with saline in a sterile bottle. The synthesis route and diagram of the automated system of 18F-FP-(+)-DTBZ are outlined in Figure 2.
HPLC Purification of 18F-FP-(+)-DTBZ
After radiofluorination, we transferred the crude product to preparative HPLC (mobile phase of acetonitrile: 50 mM ammonium acetate = 57:43; flow 4 ml/min, velocity; 280 nm, ultraviolet absorption wavelength; ultraviolet absorption peak in 12 + 2 min, plus or minus 2 min 14 radiation peak, collect radiation peak), and then absorbed on C18 column after dilution with saline solution. Exactly 1.5 ml of ethanol was to elute the C18 column. Ethanol was diluted to 10% concentration using 0.9% sodium chloride. The final products of 18F-FP-(+)-DTBZ were obtained following aseptic filtration.
SPE Purification of 18F-FP-(+)-DTBZ
The crude product solution was passed through the small column of Sep-Pak PS-2 and/or Sep-Pak C18 to eliminate unreacted [18F] fluoride and water-soluble impurities, which finally was channeled to the waste liquid bottle. To determine the optimum concentration of elusion ethanol, we used 10 mL ethanol with different concentrations from 5 to 70% to wash out the impurities, and found that the lost rate was in acceptable range when the ethanol concentration was below 30% (Figure 3A). Similarly, to determine the optimum volume of elusion ethanol, we used 30% ethanol with different volumes from 4 to 20 mL of ethanol to wash out impurities, and found the loss rate was in acceptable range when using 10 mL ethanol or below 10 mL. The best final condition for elution ethanol was as follows: for the first time, 7 mL 5% ethanol solution was used to wash out impurities to the waste vial; for the second time, 8 mL 5% ethanol solution was used to wash out excess impurities to the waste vial; for the third time, 8 mL 30% ethanol solution was used for further removal of impurities to the waste vial. Then, the final product was eluted with 3 mL 70% ethanol solution to the product vial as 70% ethanol was enough to elute the all product (Figure 3B).
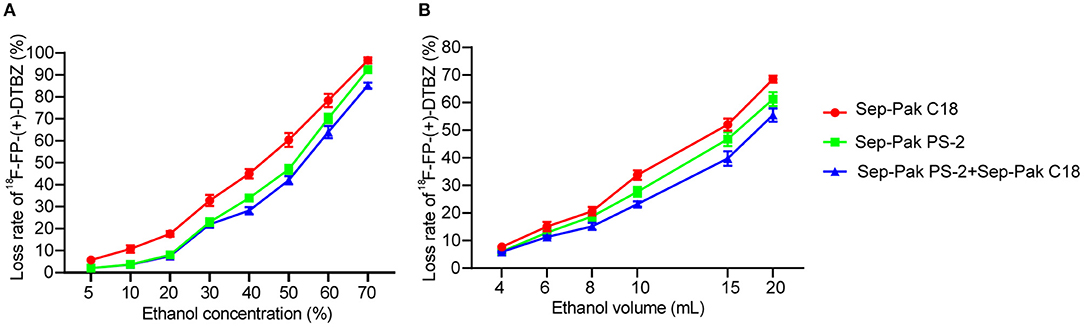
Figure 3. Loss rate of 18F-FP-(+)-DTBZ using Sep-Pak PS-2 cartridge and/or Sep-Pak C18 cartridges under different concentration of 10 mL ethanol elusion (A) and different volume of 30% ethanol elusion (B).
Quality Control of 18F-FP-(+)-DTBZ
Visual Inspection
The 18F-FP-(+)-DTBZ product solution was visually examined in the labeling hot cell through the leading glass window under bright light.
pH
We employed the pH indicator paper (pH 2–9) referring to the attached indicator chart to estimate the pH value of the purified 18F-FP-(+)-DTBZ product.
Radiochemical Purity
Before purity analysis, a reference substance solution (non-radioactive FP-(+)-DTBZ; 20 μl, 2.7 mmol/L) was injected into the analytical HPLC system. The chemical purity of 18F-FP-(+)-DTBZ after purification was determined by HPLC with UV detection at 280 nm and radiation purity of the product was monitored by HPLC with radiation detector. Simultaneously, thin layer chromatography (TLC) was also applied to the determine the radiation purity.
Bacterial Endotoxin Test
Limulus Amebocyte Lysate (LAL) assay was applied to test for bacterial endotoxins.
Imaging Quality
Two healthy volunteers and two PD patients underwent PET/CT scan to test the imaging quality of 18F-FP-(+)-DTBZ from HPLC and SPE with combined use of Sep-Pak PS-2 and Sep-Pak C18 cartridges. This study was approved by the Ethics Committee of the First Hospital of Jilin University (20K070-001) and conducted in accordance with the 1964 Declaration of Helsinki and its later amendments or comparable ethical standards. Patients were recruited for enrollment in this study from December 2020 through January 2021 at our institute, with agreement from the oncologists and on determination of the patients' eligibility.
Results
The loss rate of SPE with combined use of Sep-Pak PS-2 and Sep-Pak C18 cartridges was lower than that of SPE with one cartridge at the same concentration of ethanol and same volume of ethanol elusion (Figure 3).
The identity of 18F- FP-(+)-DTBZ was confirmed by comparing the retention time of the radioactive product with that of FP-(+)-DTB. The retention time of 18F- FP-(+)-DTBZ purified by HPLC, SPE with Sep-Pak PS-2, SPE with Sep-Pak C18, and SPE with combined use of Sep-Pak PS-2 and Sep-Pak C18 cartridges was 8.7, 8.8, 8.7, and 8.9 min, respectively. Either 18F-FP-(+)-DTBZ purified by HPLC or SPE matched well with that of FP-(+)-DTBZ within an admissible error. Fewest impurity peak was detected in 18F-FP-(+)-DTBZ purified by SPE with combined use of Sep-Pak PS-2 and Sep-Pak C18 cartridges in all purification methods (Figure 4).
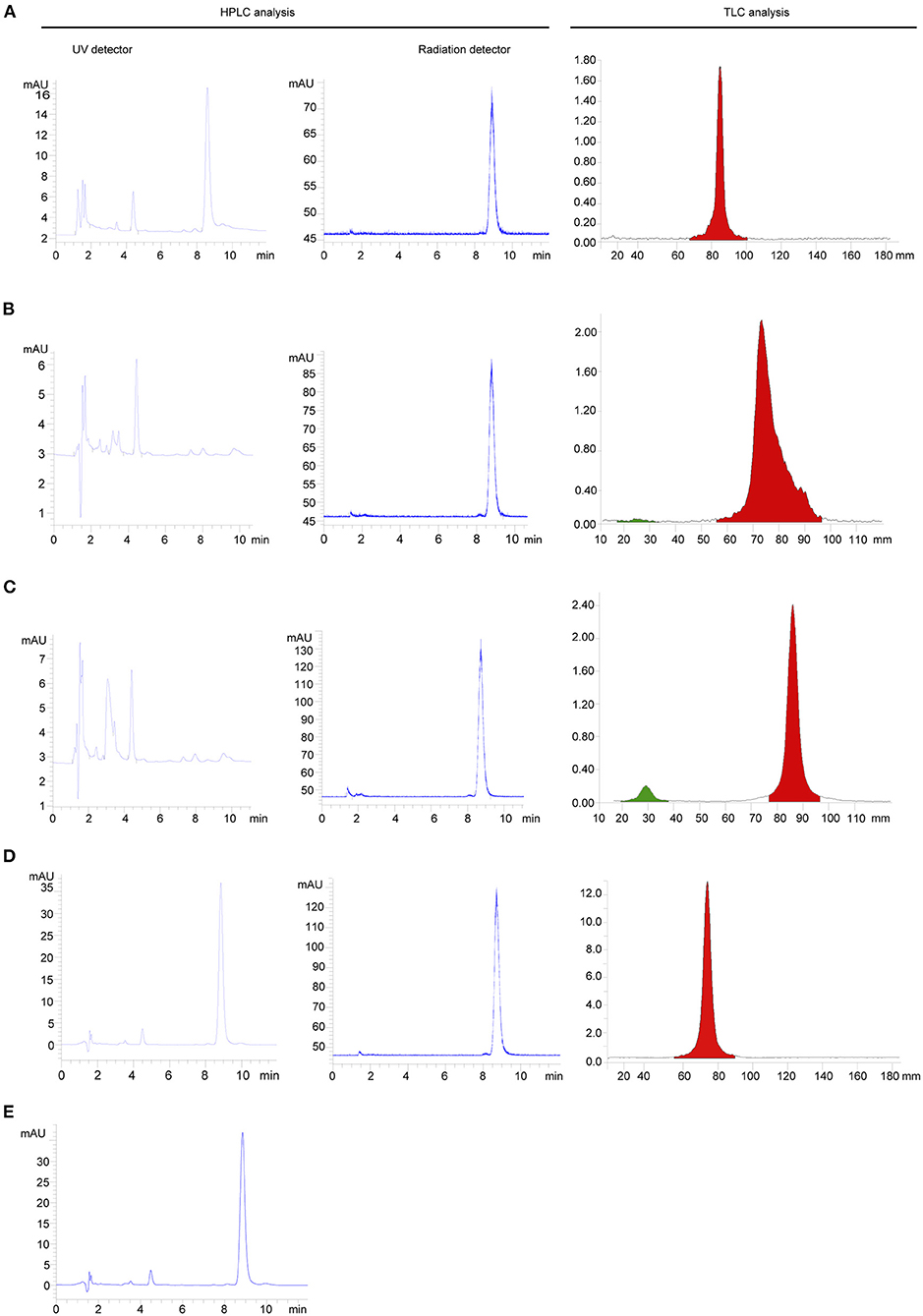
Figure 4. HPLC and TLC analysis of radiochemical purity of 18F-FP-(+)-DTBZ purified by HPLC (A), SPE with Sep-Pak PS-2 (B), SPE with Sep-Pak C18 (C) SPE with combined use of Sep-Pak PS-2 and Sep-Pak C18 (D), and radiochemical purity of FP-(+)-DTBZ (E).
Comparison of HPLC and SPE purification method of 18F-FP-(+)-DTBZ is presented in Table 1. The radiochemical purity exceeded 99%, and the product was radiochemically stable for at least 3 h for the tracers purified by HPLC and SPE with combined use of Sep-Pak PS-2 and Sep-Pak C18 cartridges. The synthesis time of 18F-FP-(+)-DTBZ with SPE purification was significantly shorter than that with HPLC purification. Brain uptake of 18F-FP-(+)-DTBZ purified by SPE with combined use of Sep-Pak PS-2 and Sep-Pak C18 cartridges was comparable to that purified by HPLC in both healthy volunteers and PD patients (Figure 5). In healthy volunteers, a symmetric distribution pattern of 18F-FP-(+)-DTBZ was shown in caudate, putamen, globus pallidus, substantia nigra. In PD patients, 18F-FP-(+)-DTBZ avidity in nigrostriatal regions reduced obviously (Figure 5).
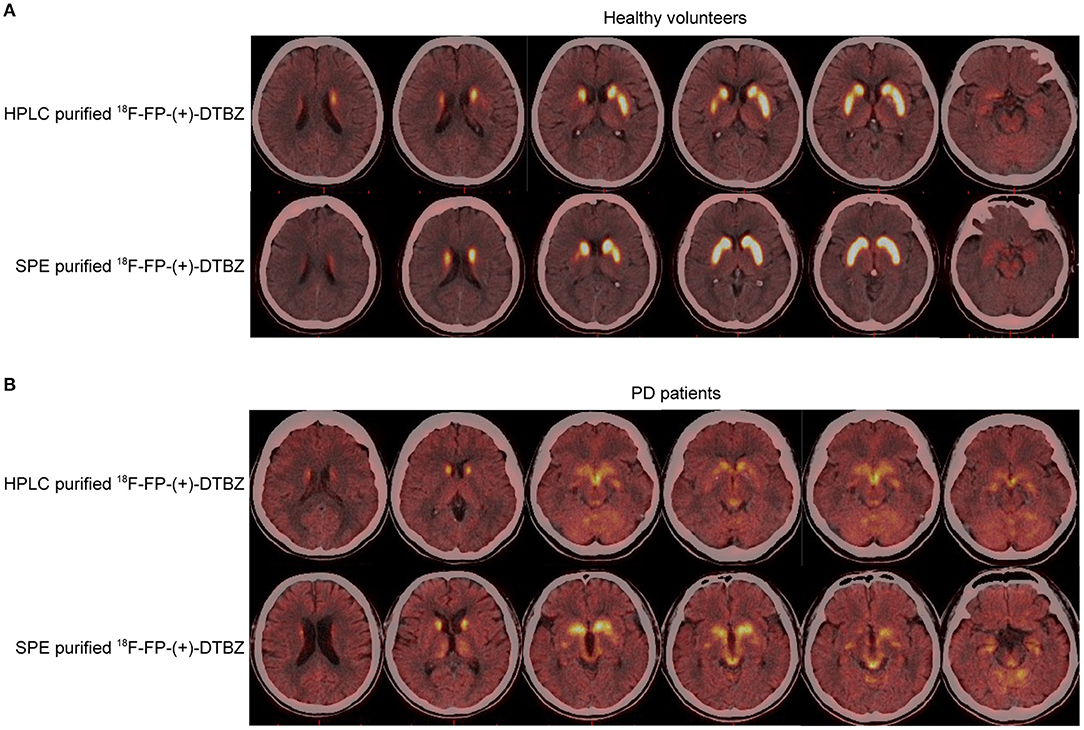
Figure 5. PET/CT performance of HPLC- and SPE- purified 18F-FP-(+)-DTBZ in healthy volunteers (A), and PD patients (B).
Discussion
In this study, we optimized SPE purification of 18F-FP-(+)-DTBZ via combining Sep-Pak PS-2 and Sep-Pak C18 cartridges. The radiochemical purity from this modified SPE purification method was much more ideal for clinical use than those purified by HPLC or SPE with one cartridge. By testing the imaging quality of 18F-FP-(+)-DTBZ in healthy volunteers and PD patients, we further elucidated that the imaging quality of 18F-FP-(+)-DTBZ from the modified SPE was comparable to that from HPLC. Additionally, the preparation time of 18F-FP-(+)-DTBZ synthesis was shortened to 27 minutes via use of the modified SPE purification.
In the early 1990s, 11C labeled tetrabenazine (TBZ), dihydrotetrabenazine (DTBZ), and methoxytetrabenazine (MTBZ) were continuously investigated as tracers for imaging of VMAT2 (22, 23). At that time, silica gel semi-preparative HPLC was mainly adopted in the purification of different kinds of tracers. 11C-TBZOMe was purified using a silica gel semi-preparative HPLC column, by passing the HPLC solvent (CH2CI2: hexane: (isopropanol: diethylamine 24:l) 37:62:1; 6 mL/min) through the extraction column and onto the HPLC column (24). Similarly, in the preparation of 11C-TBZ and 11C-DTBZ, the radiotracer was also purified using semipreparative silica gel chromatography and prepared for injection by evaporation of the HPLC solvent (23, 25). Considering the half time of radionuclide, 18F-labeled DTBZ analogs (T1/2 = 110 min) will be more practical to increase the application in clinics equipped with PET scanners but not with sufficient resources to make 11C (T1/2 = 20 min) (26, 27). Radiosynthesis of 18F-FP-(+)-DTBZ was firstly reported in 2006 (26). It is prepared with 18F fluoride as a substitute for the O-tosylate leaving group of precursors, catalyzed by K222/K2CO3 following the purification of the reaction mixture via semipreparative HPLC column. The preparation of 18F labeled compound took about 50–55 min at the end of synthesis.
Since HPLC is a long and laborious process, accompanied by considerable loss of radioactive product, multiple sequential solid-phase cartridges were, therefore, adopted to optimize the preparation of different radiotracers in the early 2010s (20, 21, 28). It is worth noting that the preparation time distinguishes HPLC- from SPE-purified 18F-FP-(+)-DTBZ. For instance, in 2010, Zhu et al. employed different precursors (-OTs or -Br as the leaving group at the 9-propoxy position), reagents [K222/K(2)CO(3) vs. tributylammonium bicarbonate] and solvents (acetonitrile vs. DMSO), reaction temperature, reaction time, and purification method with SPE (Oasis HLB) to improve 18F-FP-(+)-DTBZ radiosynthesis. This method generated a satisfactory radiochemical yield of 21–41% (n = 10) with radiochemical purity > 95% and shortened the synthesis time to 40 min (20). In 2014, a new 11C-labeled radiotracer, 10-(11) C-dihydrotetrabenazine (10-11C-DTBZ) was successfully synthesized and purified by SPE using an alumina Sep-Pak cartridge, the final 10-11C-DTBZ product was obtained. The overall synthesis time was only 20 min from bombardment to release of the product for quality control (29). In 2019, SPE with Sep-Pak C18 cartridge was implemented into the purification of the 18F-FP-(+)-DTBZ (30). In this study, they have tested purification effect of SPE equipped with different cartridges including Sep-Pak Oasis, tC2, C8, tC18, CN, Chromafix C18 Hydrox, Chromafix C18, and Chromfix C4, and finally focused on the Sep-Pak tC18 with suitable ethanol-water elution as this system showed the smallest loss in activity. The desired product was obtained in 35 min. In 2020, Zhao et al. (31) successfully obtained a radiolabeling method of 18F-FP-(+)-DTBZ under the optimized conditions (P/K2CO3 = 1:8, heating at 120°C for 3 min in dimethyl sulfoxide). The automated synthesis gave a high activity yield of 30–55% in about 40 min with a >99.0% radiochemical purity. Taken together, using SPE instead of HPLC for purification is the trend for different kinds of radiotracers.
Herein, we modified the SPE purification equipped with two different cartridges to obtain four goals: eliminate the residual chemical impurities, achieve excellent radiochemical purity, maximize the final product, and simplified purification method. In the previous studies, SPE with one cartridge was used for the purification of 18F-FP-(+)-DTBZ. However, SPE with two cartridges have not been reported. In our study, we have compared the loss rate and radiochemical purity of 18F-FP-(+)-DTBZ from SPE with two cartridges to those from SPE with one cartridge. Of note, SPE with two cartridges showed lower loss rate than SPE with one cartridge under the same concentration or same volume of ethanal elution. Whereas, under the same elution condition, although radiation purity of product from SPE with one cartridge was comparable to that from SPE with combined cartridges in the analysis of analytic HPLC with radiation detector, both HPLC analysis with UV detector and TLC analysis showed fewer impurity peaks in 18F-FP-(+)-DTBZ from SPE with two cartridges comparing to that from SPE with one cartridge. Therefore, considering the loss rate, radiochemical purity and final yield, 18F-FP-(+)-DTBZ from SPE with two cartridges was more likely to obtain favorable products than SPE with one cartridge. In addition, we have compared radiochemical purity, final yield, imaging quality and synthesis time of products purified from SPE with two cartridges to those from HPLC. Radiochemical purity, final yield and imaging quality of SPE with two cartridges were comparable to those from HPLC, while synthesis time of radiotracer from SPE with two cartridges was much shorter than that from HPLC, which indicated that the modified SPE purification was suitable for the clinic use.
In conclusion, the present study demonstrated an improved purification approach for 18F-FP-(+)-DTBZ, using SPE with two different cartridges (Sep-Pak PS-2 and Sep-Pak C18). The desired product was prepared in 27 min with a 29 ± 1.8% yield rate. This SPE purification method is highly suited to automatic synthesis for routine clinical applications.
Data Availability Statement
The raw data supporting the conclusions of this article will be made available by the authors, without undue reservation.
Ethics Statement
The studies involving human participants were reviewed and approved by the Ethics Committee of The First Hospital of Jilin University. The patients/participants provided their written informed consent to participate in this study. Written informed consent was obtained from the individual(s) for the publication of any potentially identifiable images or data included in this article.
Author Contributions
YL and HZ designed the study. YD, RS, FG, and YL participated radiosynthesis of tracer. QW and HZ analyzed scans and data. YD and RS wrote and revised the draft. All authors contributed to the article and approved the submitted version.
Funding
This study was funded by the Jilin Scientific and Technological Development Program (No. 20200201334JC).
Conflict of Interest
The authors declare that the research was conducted in the absence of any commercial or financial relationships that could be construed as a potential conflict of interest.
Acknowledgments
We thank the College of Chemistry, Jilin University for their excellent technical assistance.
References
1. Kong Y, Zhou H, Feng H, Zhuang J, Wen T, Zhang C, et al. Elucidating the relationship between diabetes mellitus and Parkinson's Disease using (18)F-FP-(+)-DTBZ, a positron-emission tomography probe for vesicular monoamine transporter 2. Front Neurosci. (2020) 14:682. doi: 10.3389/fnins.2020.00682
2. Shi X, Zhang Y, Xu S, Kung HF, Qiao H, Jiang L, et al. Decreased striatal vesicular monoamine transporter type 2 correlates with the nonmotor symptoms in Parkinson disease. Clin Nucl Med. (2019) 44:707–13. doi: 10.1097/RLU.0000000000002664
3. Eiden LE, Weihe E. VMAT2: a dynamic regulator of brain monoaminergic neuronal function interacting with drugs of abuse. Ann N Y Acad Sci. (2011) 1216:86–98. doi: 10.1111/j.1749-6632.2010.05906.x
4. Wilson JM, Kish SJ. The vesicular monoamine transporter, in contrast to the dopamine transporter, is not altered by chronic cocaine self-administration in the rat. J Neurosci. (1996) 16:3507–10. doi: 10.1523/JNEUROSCI.16-10-03507.1996
5. Sanchez-Catasus CA, Bohnen NI, Yeh FC, D'Cruz N, Kanel P, Müller M. Dopaminergic nigrostriatal connectivity in early Parkinson disease: in vivo neuroimaging study of (11)C-DTBZ PET combined with correlational tractography. J Nucl Med. (2021) 62:545–52. doi: 10.2967/jnumed.120.248500
6. Villemagne VL, Okamura N, Pejoska S, Drago J, Mulligan RS, Chételat G, et al. Differential diagnosis in Alzheimer's disease and dementia with Lewy bodies via VMAT2 and amyloid imaging. Neurodegener Dis. (2012) 10:161–5. doi: 10.1159/000334535
7. Naganawa M, Lim K, Nabulsi NB, Lin SF, Labaree D, Ropchan J, et al. Evaluation of pancreatic VMAT2 binding with active and inactive enantiomers of [(18)F]FP-DTBZ in healthy subjects and patients with type 1 diabetes. Mol Imaging Biol. (2018) 20:835–45. doi: 10.1007/s11307-018-1170-6
8. Li J, Chen P, Bao Y, Sun Y, He J, Liu X. PET imaging of vesicular monoamine transporter 2 in early diabetic retinopathy using [(18)F]FP-(+)-DTBZ. Mol Imaging Biol. (2020) 22:1161–9. doi: 10.1007/s11307-019-01443-1
9. Normandin MD, Petersen KF, Ding YS, Lin SF, Naik S, Fowles K, et al. In vivo imaging of endogenous pancreatic β-cell mass in healthy and type 1 diabetic subjects using 18F-fluoropropyl-dihydrotetrabenazine and PET. J Nucl Med. (2012) 53:908–16. doi: 10.2967/jnumed.111.100545
10. Okamura N, Villemagne VL, Drago J, Pejoska S, Dhamija RK, Mulligan RS, et al. In vivo measurement of vesicular monoamine transporter type 2 density in Parkinson disease with (18)F-AV-133. J Nucl Med. (2010) 51:223–8. doi: 10.2967/jnumed.109.070094
11. Lin KJ, Lin WY, Hsieh CJ, Weng YH, Wey SP, Lu CS, et al. Optimal scanning time window for 18F-FP-(+)-DTBZ (18F-AV-133) summed uptake measurements. Nucl Med Biol. (2011) 38:1149–55. doi: 10.1016/j.nucmedbio.2011.05.010
12. Chang CC, Hsiao IT, Huang SH, Lui CC, Yen TC, Chang WN. 8F-FP-(+)-DTBZ positron emission tomography detection of monoaminergic deficient network in patients with carbon monoxide related parkinsonism. Eur J Neurol. (2015) 22:845–52, e59–60. doi: 10.1111/ene.12672
13. Lin KJ, Weng YH, Wey SP, Hsiao IT, Lu CS, Skovronsky D. Whole-body biodistribution and radiation dosimetry of 18F-FP-(+)-DTBZ (18F-AV-133): a novel vesicular monoamine transporter 2 imaging agent. J Nucl Med. (2010) 51:1480–5. doi: 10.2967/jnumed.110.078196
14. Alexander PK, Lie Y, Jones G, Sivaratnam C, Bozinvski S, Mulligan RS, et al. Management impact of imaging brain vesicular monoamine transporter type 2 in clinically uncertain Parkinsonian Syndrome with (18)F-AV133 and PET. J Nucl Med. (2017) 58:1815–20. doi: 10.2967/jnumed.116.189019
15. Boileau I, Rusjan P, Houle S, Wilkins D, Tong J, Selby P, et al. Increased vesicular monoamine transporter binding during early abstinence in human methamphetamine users: Is VMAT2 a stable dopamine neuron biomarker? J Neurosci. (2008) 28:9850–6. doi: 10.1523/JNEUROSCI.3008-08.2008
16. Hsiao IT, Weng YH, Hsieh CJ, Lin WY, Wey SP, Kung MP. Correlation of Parkinson disease severity and 18F-DTBZ positron emission tomography. JAMA Neurol. (2014) 71:758–66. doi: 10.1001/jamaneurol.2014.290
17. Bohnen NI, Koeppe RA, Meyer P, Ficaro E, Wernette K, Kilbourn MR, et al. Decreased striatal monoaminergic terminals in Huntington disease. Neurology. (2000) 54:1753–9. doi: 10.1212/WNL.54.9.1753
18. Bohnen NI, Albin RL, Koeppe RA, Wernette KA, Kilbourn MR, Minoshima S, et al. Positron emission tomography of monoaminergic vesicular binding in aging and Parkinson disease. J Cereb Blood Flow Metab. (2006) 26:1198–212. doi: 10.1038/sj.jcbfm.9600276
19. Frey KA, Koeppe RA, Kilbourn MR, Vander Borght TM, Albin RL, Gilman S, et al. Presynaptic monoaminergic vesicles in Parkinson's disease and normal aging. Ann Neurol. (1996) 40:873–84. doi: 10.1002/ana.410400609
20. Zhu L, Liu Y, Plössl K, Lieberman B, Liu J, Kung HF. An improved radiosynthesis of [18F]AV-133: a PET imaging agent for vesicular monoamine transporter 2. Nucl Med Biol. (2010) 37:133–41. doi: 10.1016/j.nucmedbio.2009.10.005
21. Wu X, Zhou X, Zhang S, Zhang Y, Deng A, Han J, et al. Brain uptake of a non-radioactive pseudo-carrier and its effect on the biodistribution of [(18)F]AV-133 in mouse brain. Nucl Med Biol. (2015) 42:630–6. doi: 10.1016/j.nucmedbio.2015.03.009
22. Vander Borght TM, Kilbourn MR, Koeppe RA, DaSilva JN, Carey JE, Kuhl DE, et al. In vivo imaging of the brain vesicular monoamine transporter. J Nucl Med. (1995) 36:2252–60.
23. DaSilva JN, Kilbourn MR, Domino EF. In vivo imaging of monoaminergic nerve terminals in normal and MPTP-lesioned primate brain using positron emission tomography (PET) and [11C]tetrabenazine. Synapse. (1993) 14:128–31. doi: 10.1002/syn.890140205
24. DaSilva JN, Kilbourn MR, Mangner TJ. Synthesis of a [11C]methoxy derivative of alpha-dihydrotetrabenazine: a radioligand for studying the vesicular monoamine transporter. Appl Radiat Isot. (1993) 44:1487–9. doi: 10.1016/0969-8043(93)90103-H
25. Kilbourn M, Lee L, Vander Borght T, Jewett D, Frey K. Binding of alpha-dihydrotetrabenazine to the vesicular monoamine transporter is stereospecific. Eur J Pharmacol. (1995) 278:249–52. doi: 10.1016/0014-2999(95)00162-E
26. Goswami R, Ponde DE, Kung MP, Hou C, Kilbourn MR, Kung HF. Fluoroalkyl derivatives of dihydrotetrabenazine as positron emission tomography imaging agents targeting vesicular monoamine transporters. Nucl Med Biol. (2006) 33:685–94. doi: 10.1016/j.nucmedbio.2006.05.006
27. Kung MP, Hou C, Goswami R, Ponde DE, Kilbourn MR, Kung HF. Characterization of optically resolved 9-fluoropropyl-dihydrotetrabenazine as a potential PET imaging agent targeting vesicular monoamine transporters. Nucl Med Biol. (2007) 34:239–46. doi: 10.1016/j.nucmedbio.2006.12.005
28. Zhu L, Qiao H, Lieberman BP, Wu J, Liu Y, Pan Z, et al. Imaging of VMAT2 binding sites in the brain by (18)F-AV-133: the effect of a pseudo-carrier. Nucl Med Biol. (2012) 39:897–904. doi: 10.1016/j.nucmedbio.2012.05.002
29. Li X, Chen Z, Tang J, Liu C, Zou P, Huang H, et al. Synthesis and biological evaluation of 10-(11) C-dihydrotetrabenazine as a vesicular monoamine transporter 2 radioligand. Arch Pharm. (2014) 347:313–9. doi: 10.1002/ardp.201300307
30. Zhao R, Zha Z, Yao X, Ploessl K, Choi SR, Liu F, et al. VMAT2 imaging agent, D6-[(18)F]FP-(+)-DTBZ: improved radiosynthesis, purification by solid-phase extraction and characterization. Nucl Med Biol. (2019) 72–3:26–35. doi: 10.1016/j.nucmedbio.2019.07.002
Keywords: 18F-FP-(+)-DTBZ, 18F-FDG, PET/CT, solid phase extraction, Parkinson disease
Citation: Dai Y, Sa R, Guan F, Wang Q, Li Y and Zhao H (2021) A Purification Method of 18F-FP-(+)-DTBZ via Solid-Phase Extraction With Combined Cartridges. Front. Med. 8:693632. doi: 10.3389/fmed.2021.693632
Received: 11 April 2021; Accepted: 17 June 2021;
Published: 09 July 2021.
Edited by:
Laura Mezzanotte, Erasmus Medical Center, NetherlandsReviewed by:
Kun Zheng, Peking Union Medical College Hospital (CAMS), ChinaKritika Subramanian, Weill Cornell Medicine, United States
Copyright © 2021 Dai, Sa, Guan, Wang, Li and Zhao. This is an open-access article distributed under the terms of the Creative Commons Attribution License (CC BY). The use, distribution or reproduction in other forums is permitted, provided the original author(s) and the copyright owner(s) are credited and that the original publication in this journal is cited, in accordance with accepted academic practice. No use, distribution or reproduction is permitted which does not comply with these terms.
*Correspondence: Yinghua Li, bGl5aW5naEBqbHUuZWR1LmNu; Hongguang Zhao, emhhb2hnQGpsdS5lZHUuY24=
†These authors share first authorship