- 1Department of Trauma Surgery, University Medical Center Utrecht, Utrecht, Netherlands
- 2Department of Surgery, Sint Antonius Hospital, Nieuwegein, Netherlands
- 3Department of Respiratory Medicine, University Medical Center Utrecht, Utrecht, Netherlands
- 4Center for Translational Immunology (CTI), University Medical Center Utrecht, Utrecht, Netherlands
Infections in trauma patients are an increasing and substantial cause of morbidity, contributing to a mortality rate of 5–8% after trauma. With increased early survival rates, up to 30–50% of multitrauma patients develop an infectious complication. Trauma leads to a complex inflammatory cascade, in which neutrophils play a key role. Understanding the functions and characteristics of these cells is important for the understanding of their involvement in the development of infectious complications. Recently, analysis of neutrophil phenotype and function as complex biomarkers, has become accessible for point-of-care decision making after trauma. There is an intriguing relation between the neutrophil functional phenotype on admission, and the clinical course (e.g., infectious complications) of trauma patients. Potential neutrophil based cellular diagnostics include subsets based on neutrophil receptor expression, responsiveness of neutrophils to formyl-peptides and FcγRI (CD64) expression representing the infectious state of a patient. It is now possible to recognize patients at risk for infectious complications when presented at the trauma bay. These patients display increased numbers of neutrophil subsets, decreased responsiveness to fMLF and/or increased CD64 expression. The next step is to measure these biomarkers over time in trauma patients at risk for infectious complications, to guide decision making regarding timing and extent of surgery and administration of (preventive) antibiotics.
Outline
Trauma leads to a complex inflammatory cascade that can cause an acquired immunodeficiency, which makes patients prone to develop infectious complications (1). Neutrophils play a key role in these processes (2). Distinct neutrophil subtypes with different functions exist, and these cells participate in the development and/or recovery of infectious complications after trauma (3–5). Recently, analysis of neutrophil phenotype and function as complex biomarkers has become accessible for point-of-care decision-making in the management of trauma patients (6). The aim of this narrative review is to discuss the potential use of longitudinal assessment of the neutrophil compartment by point-of-care flow cytometry. The goal of these sequential measurements over time is to guide clinical decision-making regarding end goals of resuscitation, indication and timing of immune protective surgery in a damage control setting as well as definitive surgery and the administration of (preventive) antibiotics.
The immunological response to trauma is initiated at the moment of injury. At this point, two inflammatory responses are induced in parallel. The systemic pro-inflammatory response to tissue damage makes patients susceptible to multi organ dysfunction syndrome (MODS), while the simultaneous immune paralysis makes patients susceptible to late infectious complications (7). Additional trauma caused by (operative) interventions can worsen this inflammatory response, thereby, increasing the risk of inflammatory and infectious complications (1). From a clinical perspective, these processes can complicate the course of trauma patients by early pro-inflammatory complications during the first 4 days after trauma and by late-onset (>5 days) sepsis, which is suggested to have a relation with the magnitude of the inflammatory response in the beginning (8–11).
This relation between extent of the inflammatory response and the activation of the immune system can be measured by multiple neutrophil biomarkers for both phenotype and function (12). The next step is to measure neutrophil biomarkers at different time points after trauma to guide decision making regarding timing and burden (i.e., approach and extent) of surgery and administration of (preventive) antibiotics.
Clinical relevance
Injury is the leading cause of death in young individuals (13). Due to advances in the organization of trauma care, hemorrhage control, surgical approaches and resuscitation, overall mortality after trauma has declined up to 75% in recent decades (14–17). With increased early survival rates, infections are the most common complications affecting trauma patients (18, 19). Thus, infections are an increasing and substantial cause of morbidity, contributing to a mortality rate of 5–8% after trauma (17, 20). A large number of trauma patients are able to survive the first critical phase after trauma, which creates new challenges in terms of diagnostics and treatment in longitudinal management of trauma patients.
Injury induces an immediate innate immune response, leading to a complex inflammatory cascade that induces both immune activation and a refractory immune state in parallel (21). Neutrophils are the first immune cells responding to tissue damage and invading pathogens and they are known to play a key role in regulating the inflammatory response after trauma (2). Recently, analysis of neutrophil phenotype and function (e.g., neutrophil size, neutrophil subsets, neutrophil activation markers, responsiveness of these markers to bacterial stimuli) has become accessible to such extent that they can be used for point-of-care (POC) decision making after trauma (6).
Until now, studies mainly focused on the assessment of the neutrophil functional phenotype on admission at the trauma bay. In this setting, a relation between neutrophil phenotype on admission and the clinical course of trauma patients (e.g., inflammatory, and infectious complications) was demonstrated (8–11). However, the course of the inflammatory response is dynamic in time and is driven by both patient characteristics, injury type and severity, timing and burden (i.e., extent) of (operative) interventions. Further determination of these changes over time will increasingly facilitate personalized care of injured patients. As it is now possible to measure the neutrophil functional phenotype at any time point after trauma in a fast and non-labor intensive manner (12), the longitudinal course of the inflammatory response and the impact of additional interventions can be measured.
Inflammatory responses after trauma
The innate immune response is initiated by injury-related tissue damage, coined as “the first hit” (22). The amplitude of this response is thought to be determined by the severity and type of injury (6, 9, 11, 23). The response is characterized by two responses developing in parallel. Firstly, a pro-inflammatory response is mediated by a systemic inflammatory response to tissue damage, in reaction to damage-associated molecular patterns (DAMPs) and microbe-associated molecular patterns (MAMPs) (24). A dysregulated pro-inflammatory response can increase susceptibility to (multi) organ failure such as ARDS or MODS (9). Neutrophils might also help to resolve tissue damage by the release DNA structures that are formed like a web, namely neutrophil extracellular traps (NETs). NETs could contribute to preventing dissemination of (larger) pathogens (25). However, when neutrophils produce too many NETs as a reaction to trauma, these toxic structures further amplify the pro-inflammatory response, contributing to an imbalanced immune reaction (26). Secondly, an anti-inflammatory response is mediated by a refractory immune state, which leads to immune paralysis and susceptibility to infectious complications e.g., sepsis. Recently, it became clear that although these responses appear clinically distinct, the underlying immunological mechanisms, in terms of the appearance of neutrophil subsets and pro-inflammatory cytokines e.g., IL-6 and IL-10, start simultaneously and immediately after the injury (8, 27–30).
Additional trauma caused by surgical intervention, generally referred to as “a second hit,” can contribute to a further dysregulation of the immune response, thereby increasing the risk of inflammatory/infectious complications (31). Over the past decade surgical procedures were shortened to minimize the effect of such a second hit. This physiological and immune protective surgery is becoming more and more recognized as important in the early phases of treatment after trauma. Over time, different strategies have been practiced for treating multitrauma patients that are considered too unstable for early total care. The concept of damage control surgery (DCS) has come up since the 1990s, focusing on improving survival by rapidly controlling hemorrhage and contamination by a brief initial operation. Only when a patient becomes hemodynamically stable, definitive surgery is performed (22). Nowadays, a strategy that focusses on early fixation of bones, combined with a continual reassessment of the reaction and response to injury and surgery, has gained popularity. When physiology (e.g., in terms of physiological or immunological response) deteriorates, fixation should be delayed until the patient stabilizes (32). This strategy is coined early appropriate care.
Over the past decades, due to advanced hemorrhage control (damage control surgery and damage control orthopedics) and (damage control) resuscitation, a decrease in mortality and a significant lowering of the incidence of inflammatory complications (e.g., ARDS, MODS) was observed (33–35). This had consequences for studies before 2010 and after 2012, during which the inflammatory marker interleukin-6 (IL-6) was measured (11, 36, 37). In the earlier period 2006–2010 higher injury severity scores (ISS) and higher concentrations of IL-6 were found to correlate with inflammatory complications. Interestingly, this correlation between IL-6 and ISS was lost in studies published after 2012, where a lower incidence of inflammatory complications was observed (see Table 1) (11, 36–38). Thus, despite a pronounced initial inflammatory response, the systemic inflammation was lower leading to a decrease in inflammatory complications most likely due to ongoing better management of trauma care (39).
In addition, in a cohort study with thirty-three patients with acute burn patients, a clear dose response relation was found between the degree of skin injury and the percentage of banded neutrophils. Furthermore, patients with additional thermal inhalation injuries were characterized by a decreased responsiveness of fNLF of neutrophils in peripheral blood. This indicates that burn injuries cause a mild activation of systemic cellular inflammation, and that additional thermal inhalation injury induces refractory systemic neutrophils, possibly leading to an increased susceptibility to infectious complications. These results are not published.
Currently, the mortality after trauma is increasingly caused by neurotrauma (33, 40, 41). This illustrates that the pro-inflammatory response, that used to be causing hyper-inflammatory systemic conditions, seems more under control (33). This leads now to an anti-inflammatory imbalance with new clinical challenges, such as recognition and treatment of late (>5 days) infections mainly resulting in increased morbidity. A dysregulation of the immune response, in which neutrophils play a pivotal role, predisposes patients to these infectious complications (11). Understanding the functions and characteristics of neutrophils is important to understand their involvement in the development of infectious complications.
Potential neutrophil biomarkers for treatment of trauma patients
The immediate immune response directly after trauma is regulated by many humoral and cellular mediators including leukocytes, coagulation, systemic cytokine release and complement cascades (42). Neutrophils are the most abundant innate effector cells and belong to a final common pathway causing tissue damage upon hyper activation of these cells (2). Therefore, research over the past decades has focused on identification of complex activation of neutrophils to identify trauma patients with high risk on adverse outcome. An overview of the investigated markers is described below.
Cell size and granularity of neutrophils
White blood cell count (WBC) and differentiation into leukocyte subsets have been extensively investigated in trauma patients. A marked increase of WBC and especially neutrophilic granulocytes has been noted after trauma, but this increase in cell numbers per se was found to be of no prognostic value (43, 44). In addition, WBC morphological parameters have been assessed to identify patients at risk. An increase in neutrophil cell size was observed in non-survivors after severe trauma, implying an increased cell size to be a predictor for mortality (45). Thereafter, in another cohort, a rise in neutrophil cell size preceded the clinical manifestation of organ dysfunction in every patient with a complication (46). Unfortunately, it remains to be determined which precise mechanisms underlie the increase in neutrophil cell size.
A partial explanation for an increased cell-size could be the influx of immature neutrophils in the peripheral blood (47, 48). It is known that immature neutrophils appear in the bloodstream as a result of the inflammatory reaction immediately after trauma; generally referred to as a left shift (49, 50). These young neutrophils with a larger cell size are known to have a C shaped (“band”) nucleus instead of a lobulated nucleus. The neutrophil left shifts have proven their diagnostic value in patients with a bacterial infection (51). However, routine analyzers are not able to recognize a left shift of banded neutrophils in trauma patients, while they are able to do so in infectious patient groups (52). So, the question remains if banded neutrophils in trauma patients are different in function and phenotype from banded neutrophils in patients suffering from an infection. Further research has focused on phenotyping neutrophils based on receptor expression (CD16/CD62L), as this is a more accurate method to identify different phenotypes of neutrophils in trauma patients (6).
Subsets of neutrophils
Under homeostatic conditions, only a homogeneous population of mature neutrophils identified by CD16bright/CD62Lbright cells circulate in the peripheral blood. However, during acute inflammation, neutrophils in the peripheral blood can be divided into different subsets based on the expression of specific surface proteins (CD16/FcγRIII and CD62L/L-selectin) (3). Immediately after trauma, large numbers of immature neutrophils with a banded shaped nucleus (CD16dim/CD62Lbright) enter the circulation and after several days also hypersegmented neutrophils (CD16bright/CD62Ldim) can be observed (Figure 1) (50, 53). Interestingly, these neutrophil subsets based on the expression of CD16 and CD62L have different functions (3, 54). Landmark studies in healthy controls, who received lipopolysaccharide (LPS) inducing systemic inflammation, showed that banded (immature) neutrophils display a higher killing capacity than segmented neutrophils and that hypersegmented neutrophils are associated with almost immediate bacterial outgrowth, due to impaired intracellular killing after adequate phagocytosis (3). In addition, polytrauma patients who developed infectious complications had significantly higher percentage CD16dim/CD62Lbright (banded) neutrophils in the blood immediately after trauma compared with polytrauma patients who did not develop infectious complications (6).
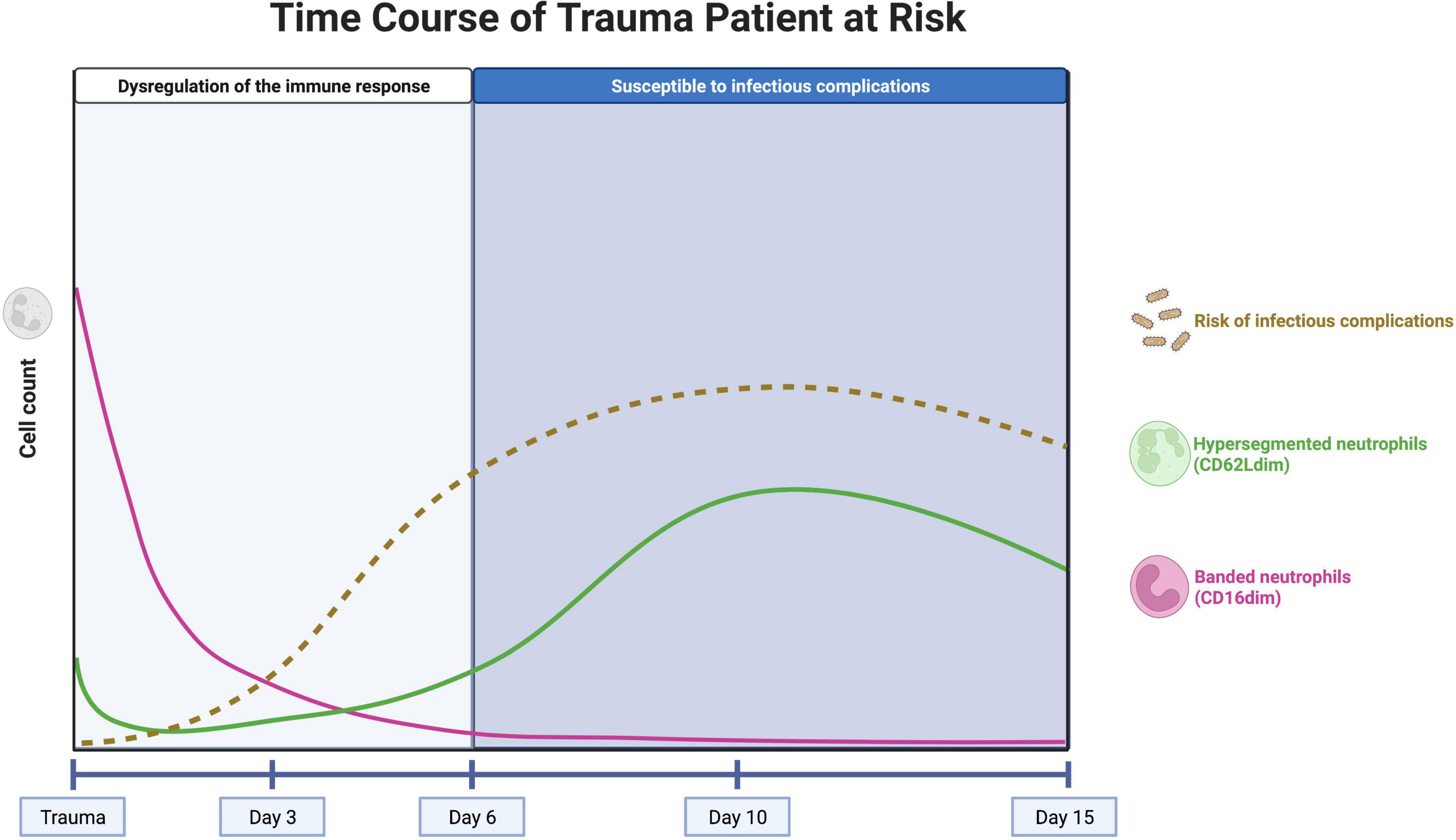
Figure 1. Concept of the neutrophil subset response over time in a trauma patient at risk for infectious complications. The pink line represents the course of well-functioning banded (CD16dim) neutrophils, entering the circulation immediately after trauma. The influx of these cells stops after approximately 1 day and the neutrophils have an average lifetime of 5 days. The green line represents the course of hypersegmented (CD62Ldim) neutrophils, with impaired killing capacity. These cells appear after several days, with a peak after 10 days. The brown, dashed line represents the risk of infectious complications over time. The risk increases after 3–5 days, when there are no banded neutrophils left in the circulation. After 10 days, the peak of hypersegmented neutrophils coincides with the first systemic infectious complications. Created with BioRender.com.
Neutrophil left shifts (influx of immature CD16dim (banded) neutrophils into the blood) are observed immediately after trauma but quickly disappear after approximately 1 day (5). This well-known phenomenon is explained by a massive mobilization of neutrophils from the bone marrow (50). This can lead to a subsequent imbalance of the neutrophil compartment and the bone marrow might be unable to fully compensate in time for the loss of these well-functioning neutrophils that can live up to 5 days under homeostatic conditions (55, 56). This phenomenon might be the driving force for the clinical observation that the trauma patient becomes highly sensitive to infectious complications after 5–6 days post trauma (Figure 1) (57).
On the other hand, CD62Ldim (hypersegmented) neutrophils appear in the peripheral blood several days (>3 days) after trauma with a peak after 10 days (5). These CD62Ldim neutrophils were shown to have impaired killing capacity and a reduced acidification capacity, but they have immunoregulatory characteristics that could be important for keeping the balance in the immune response (54). The presence of high numbers of CD62Ldim neutrophils during the days to weeks following severe trauma might play a significant role in the risk of infectious complications in trauma patients but this concept needs more experimental support. It is, however, known that the first systemic infectious complications that have consequences for the clinical course of the patient arise at the end of the first week after trauma (58). This coincides with the rising counts of CD62Llow cells during the first week after trauma, so it is tempting to hypothesize that high numbers of CD62Llow neutrophils can lead to an increased risk of infectious complications (Figure 1) (10).
Trauma patients with high numbers of banded neutrophils directly after trauma and high numbers of hypersegmented neutrophils after 10 days in the peripheral blood seem also at risk for infectious complications (Figure 1). In these patients, longitudinal measurement of the neutrophil compartment and the amount of CD16dim and CD62Ldim neutrophils could guide the clinician regarding decisions on the timing and extent (burden) of surgery. A patient that is not at risk could benefit from early total care, while a patient displaying extensive neutrophil subsets could benefit from damage control immune protective surgery.
Responsiveness to inflammatory mediators (e.g., formyl-peptides/fMLF)
In addition to the assessment of neutrophil subsets by receptor expression, the functionality of neutrophils can be investigated in terms of responsiveness of neutrophil to formyl peptides (e.g., fMLF). fMLF activates neutrophils by interactions with their formyl peptide receptors (FPR) (59–63). A decreased responsiveness of neutrophils to fMLF at initial presentation was demonstrated in trauma patients who later developed septic shock (10). In addition, low responsiveness of neutrophils to fMLF immediately after trauma preceded by almost a week the development of septic complications after > 5 days (11). Thus, responsiveness to formyl-peptides appears to be a sensitive marker as it is involved in an essential part of the immune system that is activated during the pathogenesis of sepsis and septic shock. Modulation of this responsiveness can aid the clinician in identifying patients at risk. No longitudinal data has been published yet about the responsiveness of neutrophils in trauma patients. These patients might also benefit from longitudinal monitoring to guide decision making.
Expression of FCγRI (CD64) on neutrophils
Specific expression of neutrophil receptors could be used as an extra tool to measure markers associated with specific components of the immune response. Neutrophil CD64 is a receptor that is upregulated on neutrophils within 1–6 h after administration of pro-inflammatory cytokines or bacterial cell wall components such as LPS (64). In the absence of a pro-inflammatory stimulus, CD64 levels on neutrophils start to decrease after 48 h and are return to baseline within 7 days (65). Thus, neutrophil CD64 is a relatively specific marker for bacterial infections (66). This property of CD64 has been utilized as a diagnostic marker of infection particularly for sepsis in intensive care patients (67). This could potentially also be a marker to aid the decision to early antibiotics in patients at risk for bacterial infections. Longitudinal measurement of CD64 expression in patients admitted at the hospital could aid the clinician with early diagnosis and follow up of bacterial infections (64, 68–71).
Burden of surgery: Timing + intensity
There are different strategies for treating severely injured trauma patients. Damage control surgery (DCS) is characterized by a brief initial operation to stabilize the patient, followed by several short interventions (Figure 2) (22). This approach of physiological and immune protective surgery is commonly chosen when a patient is too unstable to survive definitive fixation in the acute moment (1). The alternative to damage control surgery is early total care (ETC) with definitive fixation within the first 24–36 h (Figure 2) (32). Recently, there has been a trend toward early appropriate care (EAC), which means providing a plan of action based on continuous assessment and reaction to the response to injury and surgery (31, 72). It is based on fixation of the fractured bones at an early stage, unless physiology deteriorates and definitive fixation should be postponed (32). However, the challenge is to correctly assess the response to injury and surgery to select the patients that benefit from delayed definitive fixation of bones.
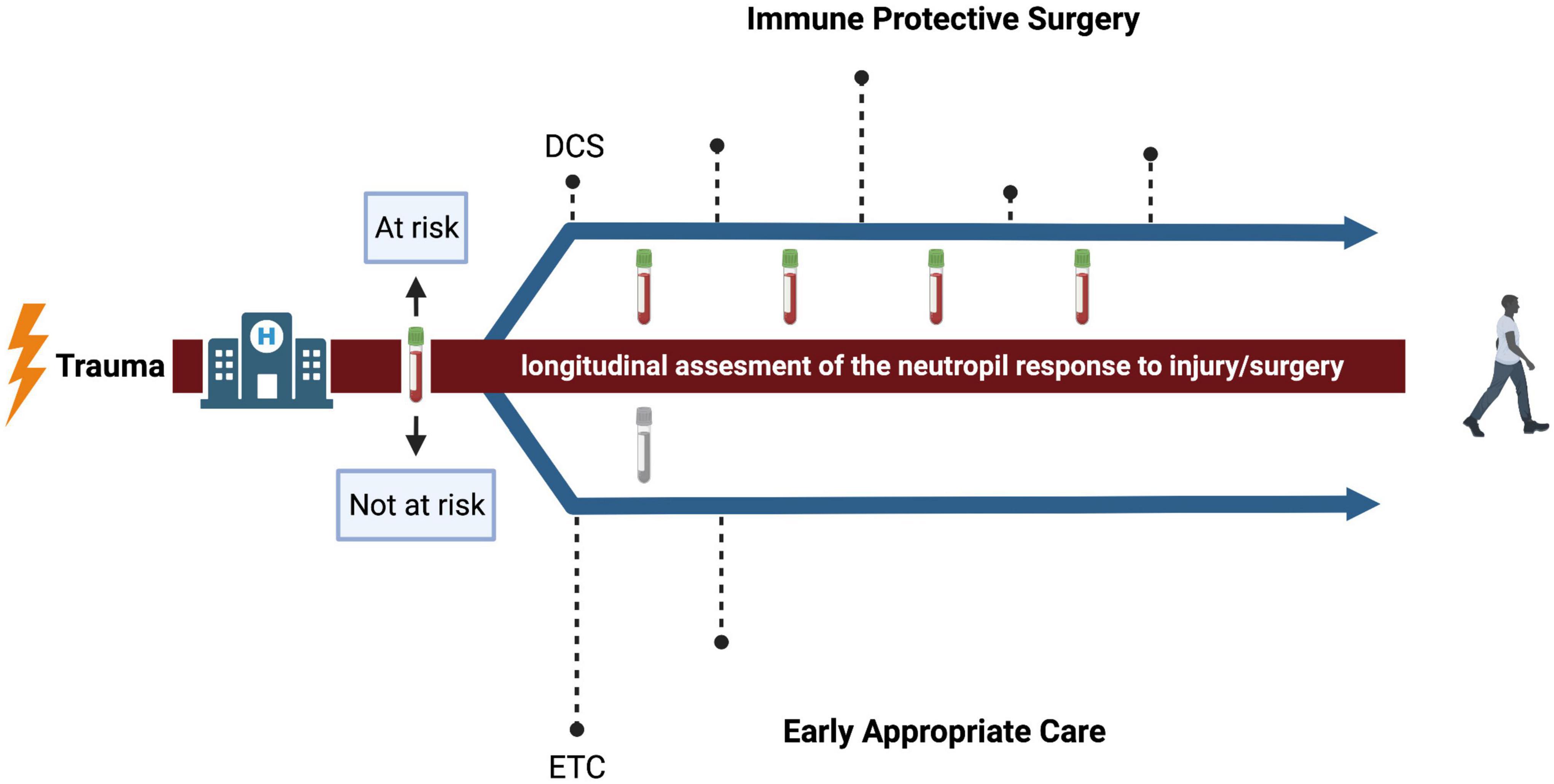
Figure 2. Conceptual representation of surgical strategies after trauma. The dashed lines represent the timing of surgical interventions and the height of the dashed lines represent the intensity. The upper arrow represents a proposed strategy for patients that, after flow cytometric measurement of the neutrophil compartment, are expected to be at risk for infectious complications. The impact of surgery is minimized and adjusted over time to reassessment of the neutrophil response. The lower arrow represents a proposed strategy for patients that are expected to be not at risk. Here, the approach of early appropriate care is suggested. DCS, damage control surgery; ETC, early total care. Created with BioRender.com.
Currently, there is no adequate method to estimate the physiological status of an injured patient over time. As shown in Figure 1, the trauma patient at risk of infectious complications is characterized by the presence of prominent neutrophil subsets in the peripheral blood directly after trauma (6). The change in the occurrence of these subsets over time might be associated with the risk of developing infectious complications (10). The impact of extensive surgery on the amount of neutrophil subsets in the blood and neutrophil responsiveness, has not yet been described. The next essential step is to investigate if the imbalance in the immune response further deteriorates, in terms of e.g., influx of banded/hypersegmented neutrophils of a further decreased responsiveness to fMLF, due to (extensive) surgery. Possibly, this could possibly aid decision making regarding timing and extent of surgery. Assessment of neutrophil subsets and responsiveness before and after surgery in patients that are deemed “at risk” directly after trauma is likely to give information about the impact of surgery and might give the opportunity to compare different surgical strategies. Thus, longitudinal assessment of changes in the neutrophil compartment might be the promising next step in personalized medicine in trauma patients, because it quantifies the inflammatory response caused by the first hit and subsequent hits.
Antibiotic interventions
Infectious complications in trauma patients are treated with antibiotics. However, not all antibiotics may be useful at every moment after trauma. Patients with increased CD62Ldim subsets and decreased fMLF responses, could suffer from invading bacteria that reside inside the neutrophils for up to 5 days after trauma (3, 55, 73, 74). Staphylococcus aureus (S. aureus) is a common causative pathogen of infections after trauma and it is known that it has the ability to survive and proliferate inside neutrophils, as in a Trojan horse (3, 75). Treatment with antibiotics that could kill bacteria that are contained inside the neutrophil would protect vulnerable patients against severe infections that typically occur >5 days after trauma. The antibiotics quinolones, rifamycins and sulfamethoxazole-trimethoprim seem effective against S. Aureus inside a human neutrophil (74). Moreover, the infectious state of a patient should be monitored by measuring the neutrophil receptor CD64. In patients with a suspected infection, high levels of CD64 are of diagnostic value (66). Moreover, when the infection is diagnosed and antibiotics are administered, sequential measurement of CD64 gives early information on the effect of the antibiotics (76). If the expression of CD64 remains high, the bacteria could be resistant, or the antibiotic is not able to reach the bacteria. Later, a low expression of CD64 after cessation of antibiotic treatment, confirms a successfully treated infection. Thus, sequentially analyzing neutrophil subsets, estimating the killing capacity and monitoring the infectious status by CD64 on neutrophils may individualize both indication, timing and type of antibiotic used in severely injured trauma patients.
Conclusion
The extent of the inflammatory response after trauma can be measured with an automatic point-of-care flow cytometry approach. It is known that there is a relation between the neutrophil functional phenotype on admission, and the clinical course (e.g., infectious complications) of the trauma patient. Potential neutrophil associated biomarkers include determination of subsets based on neutrophil receptor expression, responsiveness of neutrophils to formyl-peptides (fMLF) and CD64 expression representing the infectious state of a patient. By this technology it is likely possible to identify patients at risk for infectious complications when presented at the trauma bay. These patients display large extents of banded neutrophils directly after trauma and high numbers of hypersegmented neutrophils after 10 days, decreased responsiveness to fMLF and/or increased CD64 expression. The next step is to measure these biomarkers over time to guide decision making regarding timing and extent of surgery to achieve early appropriate care and determine the administration of (preventive) antibiotics.
Author contributions
EF wrote the first draft of the manuscript. LK, LL, NV, KW, and FH critically reviewed the manuscript. All authors contributed to the article and approved the submitted version.
Conflict of interest
The authors declare that the research was conducted in the absence of any commercial or financial relationships that could be construed as a potential conflict of interest.
Publisher’s note
All claims expressed in this article are solely those of the authors and do not necessarily represent those of their affiliated organizations, or those of the publisher, the editors and the reviewers. Any product that may be evaluated in this article, or claim that may be made by its manufacturer, is not guaranteed or endorsed by the publisher.
References
1. Lord JM, Midwinter MJ, Chen YF, Belli A, Brohi K, Kovacs EJ, et al. The systemic immune response to trauma: an overview of pathophysiology and treatment. Lancet. (2014) 384:1455–65. doi: 10.1016/S0140-6736(14)60687-5
2. Kubes P. The enigmatic neutrophil: what we do not know. Cell Tissue Res. (2018) 371:399–406. doi: 10.1007/s00441-018-2790-5
3. Leliefeld PHC, Pillay J, Vrisekoop N, Heeres M, Tak T, Kox M, et al. Differential antibacterial control by neutrophil subsets. Blood Adv. (2018) 2:1344–55. doi: 10.1182/bloodadvances.2017015578
4. Pillay J, Ramakers BP, Kamp VM, Loi ALT, Lam SW, Hietbrink F, et al. Functional heterogeneity and differential priming of circulating neutrophils in human experimental endotoxemia. J Leukoc Biol. (2010) 88:211–20. doi: 10.1189/jlb.1209793
5. Bongers SH, Chen N, van Grinsven E, van Staveren S, Hassani M, Spijkerman R, et al. Kinetics of neutrophil subsets in acute, subacute, and chronic inflammation. Front Immunol. (2021) 12:674079. doi: 10.3389/fimmu.2021.674079
6. Spijkerman R, Hesselink L, Bongers S, van Wessem KJP, Vrisekoop N, Hietbrink F, et al. Point-of-care analysis of neutrophil phenotypes: a first step toward immuno-based precision medicine in the trauma ICU. Crit Care Explor. (2020) 2:e0158. doi: 10.1097/cce.0000000000000158
7. Hesselink L, Spijkerman R, Van Wessem KJP, Koenderman L, Leenen LPH, Huber-Lang M, et al. Neutrophil heterogeneity and its role in infectious complications after severe trauma. World J Emerg Surg. (2019) 14:1–11. doi: 10.1186/s13017-019-0244-3
8. Hietbrink F, Oudijk EJ, Braams R, Koenderman L, Leenen L. Aberrant regulation of polymorphonuclear phagocyte responsiveness in multitrauma patients. Shock. (2006) 26:558–64. doi: 10.1097/01.shk.0000233196.40989.78
9. Hietbrink F, Koenderman L, Althuizen M, Leenen LPH. Modulation of the innate immune response after trauma visualised by a change in functional PMN phenotype. Injury. (2009) 40:851–5. doi: 10.1016/j.injury.2008.11.002
10. Hietbrink F, Koenderman L, Althuizen M, Pillay J, Kamp V, Leenen LPH. Kinetics of the innate immune response after trauma: implications for the development of late onset sepsis. Shock. (2013) 40:21–7. doi: 10.1097/SHK.0b013e318295a40a
11. Groeneveld KM, Koenderman L, Warren BL, Jol S, Leenen LPH, Hietbrink F. Early decreased neutrophil responsiveness is related to late onset sepsis in multitrauma patients: an international cohort study. PLoS One. (2017) 12:e0180145. doi: 10.1371/journal.pone.0180145
12. Spijkerman R, Hesselink L, Hellebrekers P, Vrisekoop N, Hietbrink F, Leenen LPH, et al. Automated flow cytometry enables high performance point-of-care analysis of leukocyte phenotypes. J Immunol Methods. (2019) 474:112646. doi: 10.1016/j.jim.2019.112646
13. CDC. Top Ten Leading Causes of Death in the U.S. for Ages 1-44 from 1981-2020. Atlanta, GA: CDC (2020).
14. Murray CJL, Lopez AD. Global mortality, disability, and the contribution of risk factors: global burden of disease study. Lancet. (1997) 349:1436–42. doi: 10.1016/S0140-6736(96)07495-8
15. GBD 2017 DALYS and Hale Collaborators. The global burden of disease. Lancet. (2018) 392:1859–922.
17. Haagsma JA, Graetz N, Bolliger I, Naghavi M, Higashi H, Mullany EC, et al. The global burden of injury: incidence, mortality, disability-adjusted life years and time trends from the global burden of disease study 2013. Inj Prev. (2016) 22:3–18. doi: 10.1136/injuryprev-2015-041616
18. Komori A, Iriyama H, Kainoh T, Aoki M, Naito T, Abe T. The impact of infection complications after trauma differs according to trauma severity. Sci Rep. (2021) 11:1–8. doi: 10.1038/s41598-021-93314-5
19. Eguia E, Bunn C, Kulshrestha S, Markossian T, Durazo-Arvizu R, Baker MS, et al. Trends, cost, and mortality from sepsis after trauma in the United States: an evaluation of the national inpatient sample of hospitalizations, 2012–2016. Crit Care Med. (2020) 48:1296–303. doi: 10.1097/ccm.0000000000004451
20. Wafaisade A, Lefering R, Bouillon B, Sakka SG, Thamm OC, Paffrath T, et al. Epidemiology and risk factors of sepsis after multiple trauma: an analysis of 29,829 patients from the trauma registry of the German society for trauma surgery. Crit Care Med. (2011) 39:621–8. doi: 10.1097/CCM.0b013e318206d3df
21. Hietbrink F, Koenderman L, Rijkers GT, Leenen LPH. Trauma: the role of the innate immune system. World J Emerg Surg. (2006) 1:1–11. doi: 10.1186/1749-7922-1-15
22. Rotondo MF, Schwab CW, McGonigal MD, Phillips GR, Fruchterman TM, Kauder DR, et al. ‘Damage control’: an approach for improved survival in exsanguinating penetrating abdominal injury. J Trauma Inj Infect Crit Care. (1993) 35:375–83. doi: 10.1097/00005373-199309000-00008
23. Visser T, Pillay J, Pickkers P, Leenen LPH, Koenderman L. Homology in systemic neutrophil response induced by human experimental endotoxemia and by trauma. Shock. (2012) 37:145–51. doi: 10.1097/SHK.0b013e31823f14a4
24. Hazeldine J, Hampson P, Lord JM. The impact of trauma on neutrophil function. Injury. (2014) 45:1824–33. doi: 10.1016/j.injury.2014.06.021
25. Hidalgo A, Libby P, Soehnlein O, Aramburu I, Papayannopoulos V, Silvestre-Roig C. Neutrophil extracellular traps.: from physiology to pathology. Cardiovasc Res. (2021) cvab329. doi: 10.1093/cvr/cvab329 [Epub ahead of print].
26. Wang J. Neutrophils in tissue injury and repair. Cell Tissue Res. (2018) 371:531–9. doi: 10.1007/s00441-017-2785-7
27. Visser T, Pillay J, Koenderman L, Leenen LPH. Postinjury immune monitoring: can multiple organ failure be predicted? Curr Opin Crit Care. (2008) 14:666–72. doi: 10.1097/MCC.0B013E3283196522
28. Xiao W, Mindrinos MN, Seok J, Cuschieri J, Cuenca AG, Gao H, et al. A genomic storm in critically injured humans. J Exp Med. (2011) 208:2581–90. doi: 10.1084/JEM.20111354
29. Hazeldine J, Naumann DN, Toman E, Davies D, Bishop JRB, Su Z, et al. Prehospital immune responses and development of multiple organ dysfunction syndrome following traumatic injury: a prospective cohort study. PLoS Med. (2017) 14:1–29. doi: 10.1371/journal.pmed.1002338
30. Timmermans K, Kox M, Vaneker M, van den Berg M, John A, van Laarhoven A, et al. Plasma levels of danger-associated molecular patterns are associated with immune suppression in trauma patients. Intensive Care Med. (2016) 42:551–61. doi: 10.1007/S00134-015-4205-3
31. Pape HC, Giannoudis P, Krettek C. The timing of fracture treatment in polytrauma patients: relevance of damage control orthopedic surgery. Am J Surg. (2002) 183:622–9. doi: 10.1016/S0002-9610(02)00865-6
32. Berwin JT, Pearce O, Harries L, Kelly M. Managing polytrauma patients. Injury. (2020) 51:2091–6. doi: 10.1016/j.injury.2020.07.051
33. Van Wessem KJP, Leenen LPH. Reduction in mortality rates of postinjury multiple organ dysfunction syndrome: a shifting paradigm? A prospective population-based cohort study. Shock. (2018) 49:33–8. doi: 10.1097/SHK.0000000000000938
34. van Wessem KJP, Leenen LPH. Incidence of acute respiratory distress syndrome and associated mortality in a polytrauma population. Trauma Surg Acute Care Open. (2018) 3:e000232. doi: 10.1136/tsaco-2018-000232
35. Sauaia A, Moore EE, Johnson JL, Chin TL, Banerjee A, Sperry JL, et al. Temporal trends of postinjury multiple-organ failure: still resource intensive, morbid, and lethal. J Trauma Acute Care Surg. (2014) 76:582–93. doi: 10.1097/TA.0000000000000147
36. Hietbrink F, Koenderman L, Leenen LPH. Intramedullary nailing of the femur and the systemic activation of monocytes and neutrophils. World J Emerg Surg. (2011) 6:1–6. doi: 10.1186/1749-7922-6-34
37. Hietbrink F, Koenderman L, Van Wessem KJP, Leenen LPH. The Impact of intramedullary nailing of tibia fractures on the innate immune system. Shock. (2015) 44:209–14. doi: 10.1097/SHK.0000000000000405
38. Heeres M, Visser T, van Wessem KJP, Koenderman AHL, Strengers PFW, Koenderman L, et al. The effect of C1-esterase inhibitor on systemic inflammation in trauma patients with a femur fracture – the CAESAR study: study protocol for a randomized controlled trial. Trials. (2011) 12:223. doi: 10.1186/1745-6215-12-223
39. van Wessem KJP, Leenen LPH, Hietbrink F. Physiology dictated treatment after severe trauma: timing is everything. Eur J Trauma Emerg Surg. (2022) doi: 10.1007/s00068-022-01916-z [Epub ahead of print].
40. Evans JA, Van Wessem KJP, McDougall D, Lee KA, Lyons T, Balogh ZJ. Epidemiology of traumatic deaths: comprehensive population-based assessment. World J Surg. (2010) 34:158–63. doi: 10.1007/S00268-009-0266-1
41. Pfeifer R, Tarkin IS, Rocos B, Pape HC. Patterns of mortality and causes of death in polytrauma patients–has anything changed? Injury. (2009) 40:907–11. doi: 10.1016/J.INJURY.2009.05.006
42. Huber-Lang M, Lambris JD, Ward PA. Innate immune responses to trauma review-article. Nat Immunol. (2018) 19:327–41. doi: 10.1038/s41590-018-0064-8
43. Chang DC, Cornwell EE, Phillips J, Paradise J, Campbell K. Early leukocytosis in trauma patients: what difference does it make? Curr Surg. (2003) 60:632–5. doi: 10.1016/j.cursur.2003.07.011
44. Lam SW, Leenen LPH, Van Solinge WW, Hietbrink F, Huisman A. Evaluation of hematological parameters on admission for the prediction of 7-day in-hospital mortality in a large trauma cohort. Clin Chem Lab Med. (2011) 49:493–9. doi: 10.1515/CCLM.2011.069
45. Lam SW, Leenen LPH, Van Solinge WW, Hietbrink F, Huisman A. Comparison between the prognostic value of the white blood cell differential count and morphological parameters of neutrophils and lymphocytes in severely injured patients for 7-day in-hospital mortality. Biomarkers. (2012) 17:642–7. doi: 10.3109/1354750X.2012.712161
46. Hesselink L, Heeres M, Paraschiakos F, Ten Berg M, Huisman A, Hoefer IE, et al. A rise in neutrophil cell size precedes organ dysfunction after trauma. Shock. (2019) 51:439–46. doi: 10.1097/SHK.0000000000001200
47. Botha AJ, Moore FA, Moore EE, Sauaia A, Banerjee A, Peterson VM. Early neutrophil sequestration after injury: a pathogenic mechanism for multiple organ failure. J Trauma. (1995) 39:411–7. doi: 10.1097/00005373-199509000-00003
48. van Hout GPJ, van Solinge WW, Gijsberts CM, Teuben MPJ, Leliefeld PHC, Heeres M, et al. Elevated mean neutrophil volume represents altered neutrophil composition and reflects damage after myocardial infarction. Basic Res Cardiol. (2015) 110:58. doi: 10.1007/S00395-015-0513-6
49. Köller M, Wick M, Muhr G. Decreased leukotriene release from neutrophils after severe trauma: role of immature cells. Inflammation. (2001) 25:53–9. doi: 10.1023/A:1007027712387
50. Pillay J, Kamp VM, Van Hoffen E, Visser T, Tak T, Lammers JW, et al. A subset of neutrophils in human systemic inflammation inhibits T cell responses through Mac-1. J Clin Invest. (2012) 122:327–36. doi: 10.1172/JCI57990
51. Chung HN, Jong WC, Lee J. Delta neutrophil index in automated immature granulocyte counts for assessing disease severity of patients with sepsis. Ann Clin Lab Sci. (2008) 38:241–6.
52. Hellebrekers P, Hesselink L, Huisman A, ten Berg M, Koenderman L, Leenen LPH, et al. Recognizing the mobilization of neutrophils with banded nuclei early after trauma. Int J Lab Hematol. (2020) 42:e224–7. doi: 10.1111/ijlh.13272
53. Hampson P, Dinsdale RJ, Wearn CM, Bamford AL, Bishop JRB, Hazeldine J, et al. Neutrophil dysfunction, immature granulocytes, and cell-free DNA are early biomarkers of sepsis in burn-injured patients: a prospective observational cohort study. Ann Surg. (2017) 265:1241–9. doi: 10.1097/SLA.0000000000001807
54. Tak T, Wijten P, Heeres M, Pickkers P, Scholten A, Heck AJR, et al. Human CD62Ldim neutrophils identified as a separate subset by proteome profiling and in vivo pulse-chase labeling. Blood. (2017) 129:3476–85. doi: 10.1182/blood-2016-07-727669
55. Pillay J, Den Braber I, Vrisekoop N, Kwast LM, De Boer RJ, Borghans JAM, et al. In vivo labeling with 2H2O reveals a human neutrophil lifespan of 5.4 days. Blood. (2010) 116:625–7. doi: 10.1182/blood-2010-01-259028
56. Overbeeke C, Tak T, Koenderman L. The journey of neutropoiesis : how complex landscapes in bone marrow guide continuous neutrophil lineage determination. Blood. (2021) 39:2285–93. doi: 10.1182/blood.2021012835
57. Livingston DH, Anjaria D, Wu J, Hauser CJ, Chang V, Deitch EA, et al. Bone marrow failure following severe injury in humans. Ann Surg. (2003) 238:748–53. doi: 10.1097/01.sla.0000094441.38807.09
58. Cole E, Davenport R, Willett K, Brohi K. The burden of infection in severely injured trauma patients and the relationship with admission shock severity. J Trauma Acute Care Surg. (2014) 76:730–5. doi: 10.1097/TA.0b013e31829fdbd7
59. Carp H. Mitochondrial N-formylmethionyl proteins as chemoattractants for neutrophils. J Exp Med. (1982) 155:264–75. doi: 10.1084/JEM.155.1.264
60. Marasco WA, Phan SH, Krutzsch H, Showell HJ, Feltner DE, Nairn R, et al. Purification and identification of formyl-methionyl-leucyl-phenylalanine as the major peptide neutrophil chemotactic factor produced by Escherichia coli. J Biol Chem. (1984) 259:5430–9. doi: 10.1016/S0021-9258(18)91029-X
61. Chatham WW, Turkiewicz A, Blackburn WD. Determinants of neutrophil HOCl generation: ligand-dependent responses and the role of surface adhesion. J Leukoc Biol. (1994) 56:654–60. doi: 10.1002/JLB.56.5.654
62. Ye RD, Boulay F, Ji MW, Dahlgren C, Gerard C, Parmentier M, et al. International union of basic and clinical pharmacology. LXXIII. Nomenclature for the formyl peptide receptor (FPR) family. Pharmacol Rev. (2009) 61:119–61. doi: 10.1124/PR.109.001578
63. Nauseef WM. How human neutrophils kill and degrade microbes: an integrated view. Immunol Rev. (2007) 219:88–102. doi: 10.1111/J.1600-065X.2007.00550.X
64. Li S, Huang X, Chen Z, Zhong H, Peng Q, Deng Y, et al. Neutrophil CD64 expression as a biomarker in the early diagnosis of bacterial infection: a meta-analysis. Int J Infect Dis. (2013) 17:e902–13. doi: 10.1016/j.ijid.2012.07.017
65. Schiff DE, Rae J, Martin TR, Davis BH, Curnutte JT. Increased phagocyte FcγRI expression and improved Fcγ-receptor- mediated phagocytosis after in vivo recombinant human interferon-γ treatment of normal human subjects. Blood. (1997) 90:3187–94. doi: 10.1182/blood.v90.8.3187
66. Fjaertoft G, Håkansson LD, Pauksens K, Sisask G, Venge P. Neutrophil CD64 (FcgammaRI) expression is a specific marker of bacterial infection: a study on the kinetics and the impact of major surgery. Scand J Infect Dis. (2007) 39:525–35. doi: 10.1080/00365540601113693
67. Mahmoodpoor A, Paknezhad S, Shadvar K, Hamishehkar H, Movassaghpour AA, Sanaie S, et al. Flow cytometry of CD64, HLA-DR, CD25, and TLRs for diagnosis and prognosis of sepsis in critically Ill Patients admitted to the intensive care unit: a review article. Anesthesiol pain Med. (2018) 8:e83128. doi: 10.5812/AAPM.83128
68. Mahmoodpoor A, Movassaghpour A, Talebi M, Shadvar K, Soleimanpour H. Value of flow cytometry (HLA-DR, CD14, CD25, CD13, CD64) in prediction of prognosis in critically ill septic patients admitted to ICU: a pilot study. J Clin Anesth. (2020) 61:109646. doi: 10.1016/J.JCLINANE.2019.109646
69. Cong S, Ma T, Di X, Tian C, Zhao M, Wang K. Diagnostic value of neutrophil CD64, procalcitonin, and interleukin-6 in sepsis: a meta-analysis. BMC Infect Dis. (2021) 21:384. doi: 10.1186/s12879-021-06064-0
70. Thiriet C, Mahjoub K, Courte G, Labroca P, Cravoisy A, Lemarie J, et al. Automated measurement of neutrophil CD64 expression for diagnosing sepsis in critically ill patients. Minerva Anestesiol. (2019) 85:943–50. doi: 10.23736/S0375-9393.19.13420-7
71. Ye Z, Zou H, Liu S, Mei C, Chang X, Hu Z, et al. Diagnostic performance of neutrophil CD64 index in patients with sepsis in the intensive care unit. J Int Med Res. (2019) 47:4304–11. doi: 10.1177/0300060519860677
72. Pfeifer R, Pape HC. Diagnostik und versorgungsstrategien beim polytraumatisierten patienten. Der Chir. (2016) 87:165–75. doi: 10.1007/s00104-015-0139-0
73. Kolaczkowska E, Kubes P. Neutrophil recruitment and function in health and inflammation. Nat Rev Immunol. (2013) 13:159–75. doi: 10.1038/nri3399
74. Bongers S, Hellebrekers P, Leenen LPH, Koenderman L, Hietbrink F. Intracellular penetration and effects of antibiotics on staphylococcus aureus inside human neutrophils: a comprehensive review. Antibiotics. (2019) 8:1–22. doi: 10.3390/antibiotics8020054
75. Fraunholz M, Sinha B. Intracellular Staphylococcus aureus: live-in and let die. Front Cell Infect Microbiol. (2012) 2:43. doi: 10.3389/fcimb.2012.00043
Keywords: trauma, inflammatory, neutrophil, infection, longitudinal
Citation: de Fraiture EJ, Vrisekoop N, Leenen LPH, van Wessem KJP, Koenderman L and Hietbrink F (2022) Longitudinal assessment of the inflammatory response: The next step in personalized medicine after severe trauma. Front. Med. 9:983259. doi: 10.3389/fmed.2022.983259
Received: 30 June 2022; Accepted: 01 September 2022;
Published: 20 September 2022.
Edited by:
Antoine Roquilly, Université de Nantes, FranceReviewed by:
Marco Carbonara, IRCCS Ca ‘Granda Foundation Maggiore Policlinico Hospital, ItalyR. Clinton Webb, University of South Carolina, United States
Copyright © 2022 de Fraiture, Vrisekoop, Leenen, van Wessem, Koenderman and Hietbrink. This is an open-access article distributed under the terms of the Creative Commons Attribution License (CC BY). The use, distribution or reproduction in other forums is permitted, provided the original author(s) and the copyright owner(s) are credited and that the original publication in this journal is cited, in accordance with accepted academic practice. No use, distribution or reproduction is permitted which does not comply with these terms.
*Correspondence: E. J. de Fraiture, ZS5kZS5mcmFpdHVyZUBhbnRvbml1c3ppZWtlbmh1aXMubmw=