- 1Division of Nephrology, Nanfang Hospital, Southern Medical University, Guangzhou, China
- 2Key Laboratory of Molecular Epidemiology of Hunan Province, Changsha, China
- 3Department of Nephrology, Center of Kidney and Urology, The Seventh Affiliated Hospital, Sun Yat-sen University, Shenzhen, China
- 4Fifth Department of Medicine (Nephrology/Endocrinology/Rheumatology/Pneumonology), University Medical Centre Mannheim, University of Heidelberg, Heidelberg, Germany
- 5Klinik für Innere Medizin, Bundeswehrkrankenhaus Berlin, Berlin, Germany
- 6Department of Nephrology, The First Affiliated Hospital of Hainan Medical University, Haikou, China
- 7European Center for Angioscience (ECAS), Medical Faculty Mannheim of the University of Heidelberg, Mannheim, Germany
- 8Reproductive and Genetic Hospital of CITIC-Xiangya, Changsha, China
- 9Key Laboratory of Study and Discovery of Small Targeted Molecules of Hunan Province, School of Medicine, Hunan Normal University, Changsha, China
- 10Institute of Medical Diagnostics, IMD, Berlin, Germany
Background: Vitamin D deficiency (VDD) or vitamin D insufficiency is common in kidney transplant recipients (KTRs). The impact of VDD on clinical outcomes in KTRs remain poorly defined and the most suitable marker for assessing vitamin D nutritional status in KTRs is unknown so far.
Methods: We conducted a prospective study including 600 stable KTRs (367 men, 233 women) and a meta-analysis to pool existing evidence to determine whether 25(OH)D or 1,25(OH)2D predicted graft failure and all-cause mortality in stable KTRs.
Results: Compared with a higher 25(OH)D concentration, a low concentration of 25(OH)D was a risk factor for graft failure (HR 0.946, 95% CI 0.912−0.981, p = 0.003), whereas 1,25 (OH)2D was not associated with the study end-point graft loss (HR 0.993, 95% CI 0.977−1.009, p = 0.402). No association was found between either 25(OH)D or 1,25 (OH)2D and all-cause mortality. We furthermore conducted a meta-analysis including 8 studies regarding the association between 25(OH)D or 1,25(OH)2D and graft failure or mortality, including our study. The meta-analysis results were consistent with our study in finding that lower 25(OH)D levels were significantly associated with the risk of graft failure (OR = 1.04, 95% CI: 1.01−1.07), but not associated with mortality (OR = 1.00, 95% CI: 0.98−1.03). Lower 1,25(OH)2D levels were not associated with the risk of graft failure (OR = 1.01, 95% CI: 0.99−1.02) and mortality (OR = 1.01, 95% CI: 0.99−1.02).
Conclusion: Baseline 25(OH)D concentrations but not 1,25(OH)2D concentrations were independently and inversely associated with graft loss in adult KTRs.
Introduction
Graft and patient survival rates after kidney transplantation (KT) have improved over the past decade. The death-censored graft survival rate has increased steadily in adults and pediatric recipients (1). Although sequential improvements in short-term graft patency were achieved, the advances were not accompanied by similar progress in long-term graft survival (2). Thus, there is an urgent yet unmet medical need to implement comprehensive strategies to improve long-term outcomes.
Vitamin D deficiency (VDD) or vitamin D insufficiency is common and conflicting results concerning VDD-associated graft failure and mortality were described in kidney transplant recipients (KTRs). Many studies showed that VDD, measured as 25-hydroxyvitamin D [25(OH)D] or 1,25-dihydroxyvitamin D [1,25(OH)2D], relates to kidney dysfunction in renal disease and graft loss in KTRs. An animal study demonstrated that VDD reduces renal function, worsens renovascular morphological features, and aggravates moderate chronic kidney disease (CKD) (3). Epidemiologic research observed that VDD is associated with an increased risk of CKD progression (4–7), while vitamin D analogs in two small clinical trials provided some indication of renoprotective effects by reducing proteinuria (8–10).
Moreover, other clinical studies revealed that when comparing KTR patients with deficient and insufficient vitamin D levels to KTR patients with sufficient vitamin D levels, the latter had better graft survival and overall survival outcomes (11–13).
However, some clinical studies reported conflicting results that VDD, whether measured as 25(OH)D or 1,25(OH)2D, is not associated with patient survival and graft loss in KTRs (14–17). Also, a recent meta-analysis (18) showed that early vitamin D deficiency was associated with a higher mortality rate after KT; graft loss was unaffected. However, the vitamin D status of the studies included was assessed by 25(OH)D only, focused on vitamin D status right after transplantation, and these studies were not corrected for the varying methods of vitamin D measurement.
Given the increasing knowledge of widespread deficiency of vitamin D with 10% of cases in North America and>80% in part of Asia (18) and the lack of clarity as to whether VDD is associated with clinical outcomes in KTRs and the most suitable marker for assessing vitamin D nutritional status, we conducted a prospective study and a meta-analysis to pool existing evidence and the current observational study to determine whether 25(OH)D or 1,25(OH)2D predicted graft failure and all-cause mortality in stable KTRs.
Materials and methods
Study population and design
This prospective cohort study comprises 600 KTRs who received a deceased kidney donation, which received a kidney transplant before October 15th, 2012, at the transplant clinic Charité-Mitte, Berlin, Germany. The clinical and research activities being reported are consistent with the Principles of the Declaration of Istanbul as outlined in the “Declaration of Istanbul on Organ Trafficking and Transplant Tourism.” Exclusion criteria: patients with an acute infection, malignancy, acute rejection, acute myocardial infarction, pulmonary edema, or heart failure at blood sampling. Patients were followed up for graft loss and all-cause mortality for 3 years. Loss of graft function was defined as the need for renal replacement therapy based on the judgment of the treating physicians. The protocol was approved by the ethics committee of Charité University Hospital (approval number 2012−327) under the Declaration of Helsinki. After the patients’ consent, clinical and laboratory data were collected. Patients’ blood was collected at the beginning of the study.
Data sources and assays
Demographic data for recipients and donors (cold ischemia time, HLA mismatches, donor’s age, panel reactive antibodies, recipient’s age, sex, transplant survival, underlying kidney disease) were extracted from hospital records and the Euro-transplant records of the patients. Blood samples were collected from 600 KTRs during September and October 2012. EDTA was added to blood samples followed by centrifugation (4,500 rpm) for 20 min at 4°C, then plasma was collected and stored at −20°C until analysis. Laboratory parameters (including plasma 25(OH)D, 1,25(OH)2D, calcium, phosphorus, albumin, cholesterol, and creatinine) were measured by standardized laboratory techniques in the central clinical laboratory of the Charité Universitaetsmedizin Berlin, Germany.
Statistical analysis
Data are presented as median (interquartile range) and number (percentage) for normally distributed and nominal data. A p-value of less than 0.05 (two-tailed) was considered statistically significant. Statistical analyses were performed using SPSS 20.0 for Windows (SPSS Inc.) and STATA 14 (StataCorp, College Station, TX, USA).
The blood levels of 25(OH)D and 1,25(OH)2D were firstly analyzed using the area under the receiver operating characteristic (ROC) curve to obtain the optimal cut-off values, respectively (Supplementary Figure 1). Since the histograms of all parameters do not suggest multimodal distribution and the normality tests indicate normal distributions, plasma levels of all parameters in patients with 25(OH)D above or below the cutoff-value were compared using the independent T-test in Table 1. Furthermore, using these cut-off values, time-to-event analyses were performed with the log-rank test using the Kaplan-Meier method (Figure 1). In addition, multivariable-adjusted graft failure analyses were performed using Cox proportional hazards regression models (Tables 2, 3). Based on the following confounding factors: patient age, sex, donor age, cold ischemia time, urine protein, time on dialysis, eGFR, plasma calcium, phosphate, iPTH, hemoglobin, immunosuppressants and HLA mismatches, several models were built by adding 25(OH)D, 1,25(OH)2D and then both, respectively, to analyze which is the independent risk factor for graft loss in KTRs. Pearson’s correlation analysis was conducted to illustrate the correlation between the two forms of vitamin D and eGFR (Supplementary Figure 2). Furthermore, the 25(OH)D curves with hazard ratio were fitted for graft loss in the generalized additive model (GAM) (Figure 2).
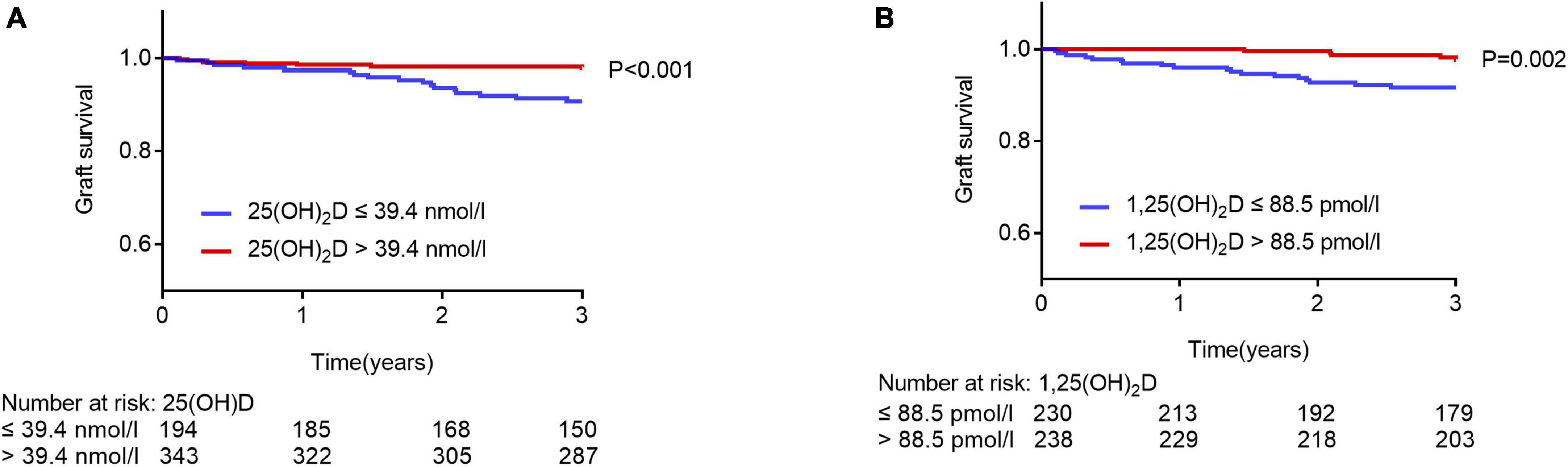
Figure 1. Graft survival rates in patients with higher and lower levels of plasma 25(OH)D or 1,25(OH)2D. Kaplan-Meier curves, according to plasma 25(OH)D and 1,25 (OH)2D for graft failure. Survival rates were compared with the log-rank test. (A) Blue lines, patients with plasma 25(OH)D levels of ≤ 39.4 nmol/l; red lines, patients with plasma 25(OH)D levels of > 39.4 nmol/l. (B) Blue lines, patients with plasma 1,25(OH)2D levels of ≤ 88.5 pmol/l; red lines, patients with plasma 1,25(OH)2D levels of > 88.5 pmol/l.
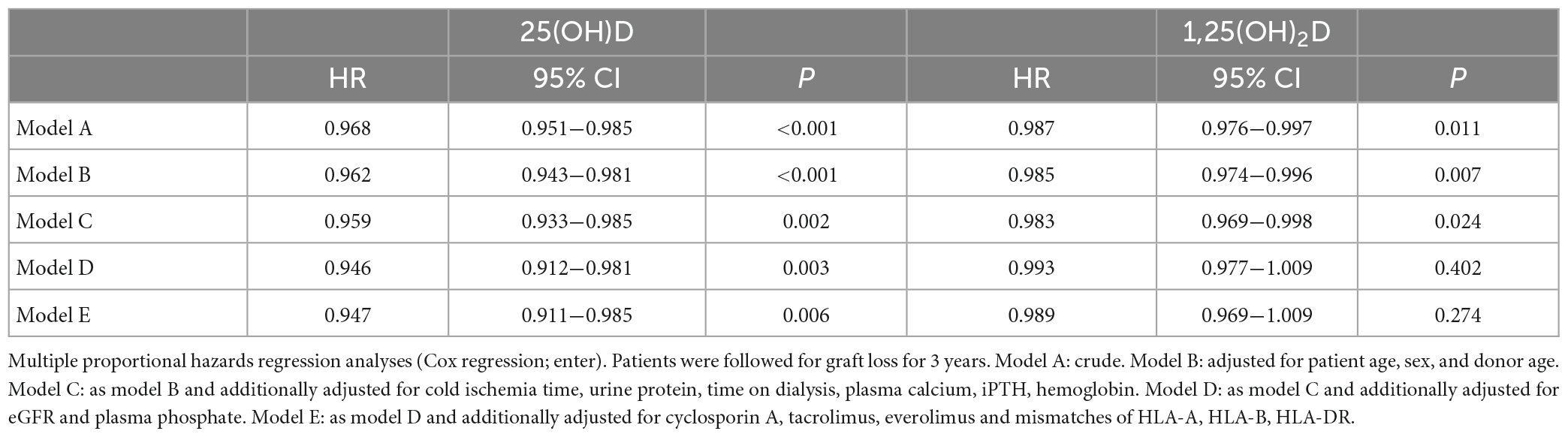
Table 2. Cox proportional hazards analysis of 25(OH)D or 1,25(OH)2D and the relevant factors for graft loss in renal transplant recipients.
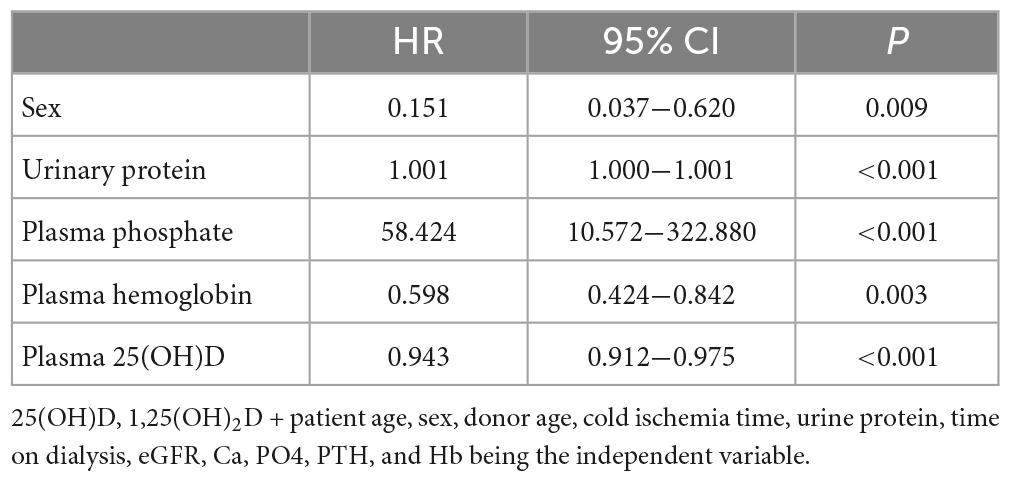
Table 3. Cox proportional hazards analysis of potential bio-marker predicted graft failure of renal transplant patients forward likelihood ratio model (n = 600).
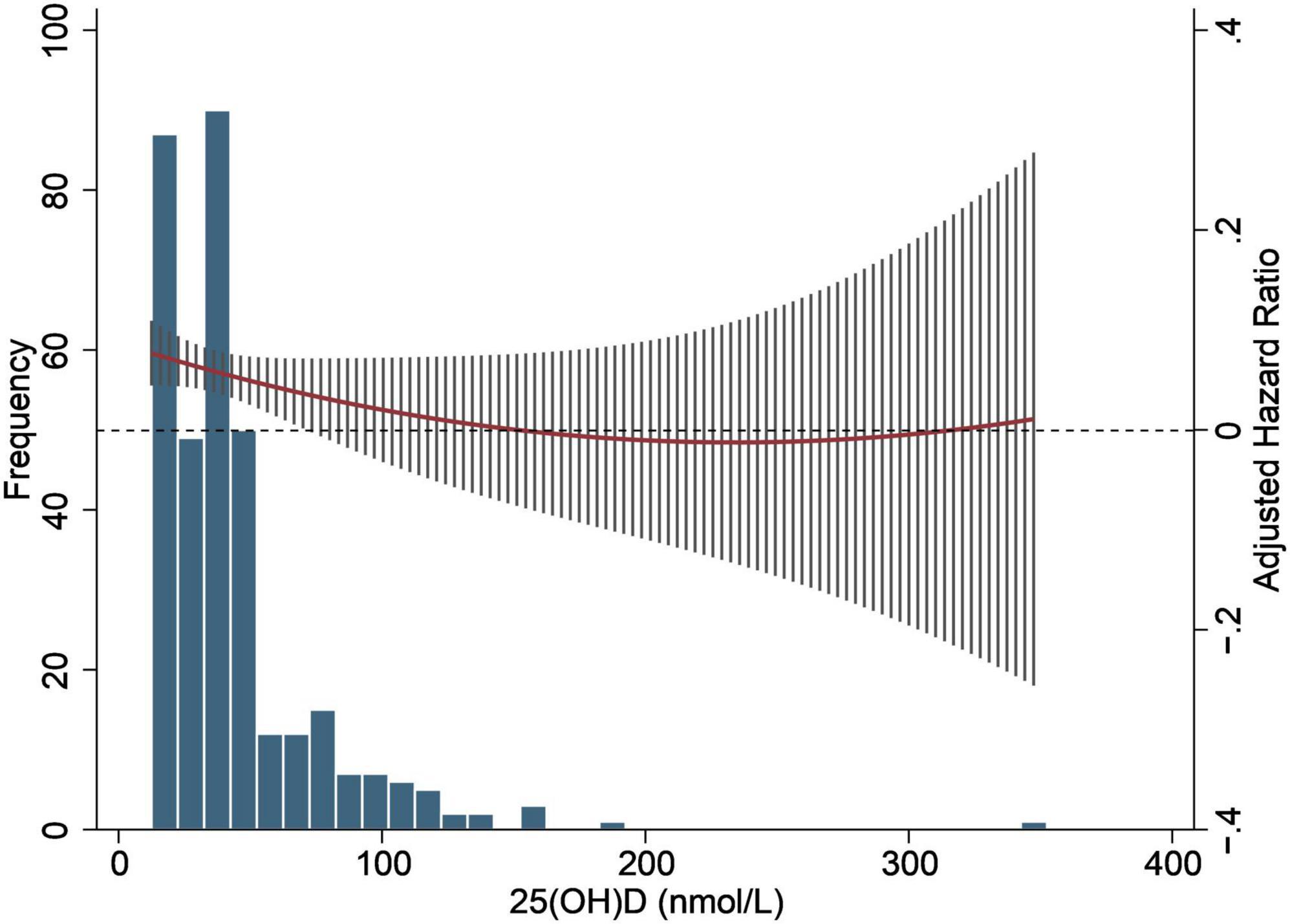
Figure 2. Estimated spline for the hazard ratio of graft failure with 25(OH)D in renal transplant recipients. Y axis represents spline smooth functions. Tick marks in X axis indicate distribution of observations. Dashed lines designate 95% confidence interval for smoothing functions.
For the association between 25(OH)D, or 1,25(OH)2D and the risk of graft failure or mortality, we further conducted a meta-analysis to estimate the pooled estimates (HR). First, we searched PubMed electronically, using terms relating to vitamin D [e.g., vitamin D or 25-hydroxyvitamin D or 1,25-dihydroxyvitamin D or 1,25(OH)2D or 25(OH)D], kidney transplantation (e.g., Kidney transplantation or renal transplantation or kidney transplant or renal transplant) and (graft failure or graft loss). The inclusion criteria of this meta-analysis were: original paper; follow-up studies with 1,25(OH)2D or 25(OH)D data available; outcome included graft loss or mortality; published before December 31st, 2021; the language of the study was English. Exclusion criteria were: animal study or review paper.
After screening the papers, we included seven studies that met the inclusion criteria for our meta-analysis to calculate the pooled HR of the association between 1,25(OH)2D or 25(OH)D and the risk of graft failure or mortality. Fixed effects models were used for studies without significant heterogeneity, while random-effects models were used for studies with significant heterogeneity. RevMan Manager version 5.4 software1 was used for the present meta-analysis.
Results
Study population
The baseline characteristics for 600 KTRs consisting of 233 men and 367 women aged 20–87 years are shown in Table 1, which contains both clinical and laboratory parameters. After 3 years of follow-up, 38 patients showed graft failure, and 65 died in this observation period.
Association of vitamin D status and all-cause mortality
In order to separate the study population, 1,25(OH)2D and 25(OH)D were firstly analyzed using ROC analysis for all-cause mortality to obtain the cut-off values [1,25(OH)2D 83.5 pmol/l; 25(OH)D 52.35 nmol/l]. No association was found between two forms of vitamin D and all-cause mortality using the Kaplan-Meier survival curve [1,25(OH)2D, p = 0.385; 25(OH)D, p = 0.616; data do not show]. Furthermore, univariable Cox regression analysis indicated that none of the two forms of vitamin D is associated with all-cause mortality [1,25(OH)2D, HR 1.001, 95% CI 0.996−1.006, p = 0.688; 25(OH)D, HR 1.004, 95% CI 0.998−1.011, p = 0.154; data do not show].
Association of vitamin D status and graft loss, and eGFR
We separated the study population with optimal cut-off values of 1,25(OH)2D and 25(OH)D, respectively, using a ROC analysis for graft loss (Supplementary Figure 1). In a Kaplan-Meier survival curve in patients after kidney transplantation, 25(OH)D concentrations above 39.4 nmol/l were significantly associated with graft loss (Figure 1, p < 0.001, log-rank test), as well as 1,25(OH)2D concentrations above 88.5 pmol/l were associated with graft loss (p = 0.002, log-rank test). On the contrary, the ratio of 1,25 (OH)2D and 25(OH)D was not associated with graft loss (p = 0.531, log-rank test, data do not show).
We analyzed the impact of 1,25(OH)2D, as well as 25(OH)D in KTRs using Cox regression models by gradually adding confounding factors (Table 2). In univariable Cox analyses, both 1,25(OH)2D and 25(OH)D were significantly associated with graft loss (Model A, HR 0.987, 95% CI 0.976−0.997, p = 0.011; HR 0.968, 95% CI 0.951−0.985, p < 0.001). The association between 25(OH)D and graft loss remained significant after adjustment for known confounders factors (Model D, HR 0.946, 95% CI 0.912−0.981, p = 0.003). However, 1,25 (OH)2D was no longer significantly correlated to graft loss after adding an adjustment for eGFR and plasma phosphate (Model D, HR 0.993, 95% CI 0.977−1.009, p = 0.402). In addition, we build model E based on model D and additionally adjusted for cyclosporin A, tacrolimus, everolimus and mismatches of HLA-A, HLA-B, HLA-DR, and the association between 25(OH)D and graft loss remained significant (model E, HR 0.947, 95% CI 0.911−0.985, p = 0.006). Moreover, we also performed Cox regression models using the forward likelihood ratio method. This approach likewise showed that a low concentration of plasma 25(OH)D (Table 3, HR 0.943, 95% CI 0.912−0.975, p < 0.001) was a risk factor for graft failure, whereas again, 1,25 (OH)2D concentrations were not associated with the study end-point graft loss.
Supplementary Figure 2 showed that eGFR was positively correlated with 1,25(OH)2D plasma concentration but not with 25(OH)D plasma concentration in KTRs. Furthermore, Figure 2 reveals that graft loss hazard ratios (HRs) were inversely associated with 25(OH)D in a non-linear model.
Meta-analysis
We performed a meta-analysis to assess the association between 1,25(OH)2D or 25(OH)D and graft failure or mortality. Lower 25(OH)D levels were significantly associated with the risk of graft failure (OR = 1.04, 95% CI: 1.01−1.07) but not associated with mortality (OR = 1.00, 95% CI: 0.98−1.03) (Figure 3). Lower 1,25(OH)2D levels were not associated with the risk of graft failure (OR = 1.01, 95% CI: 0.99−1.02) and mortality (OR = 1.01, 95% CI: 0.99−1.02) (Figure 3).
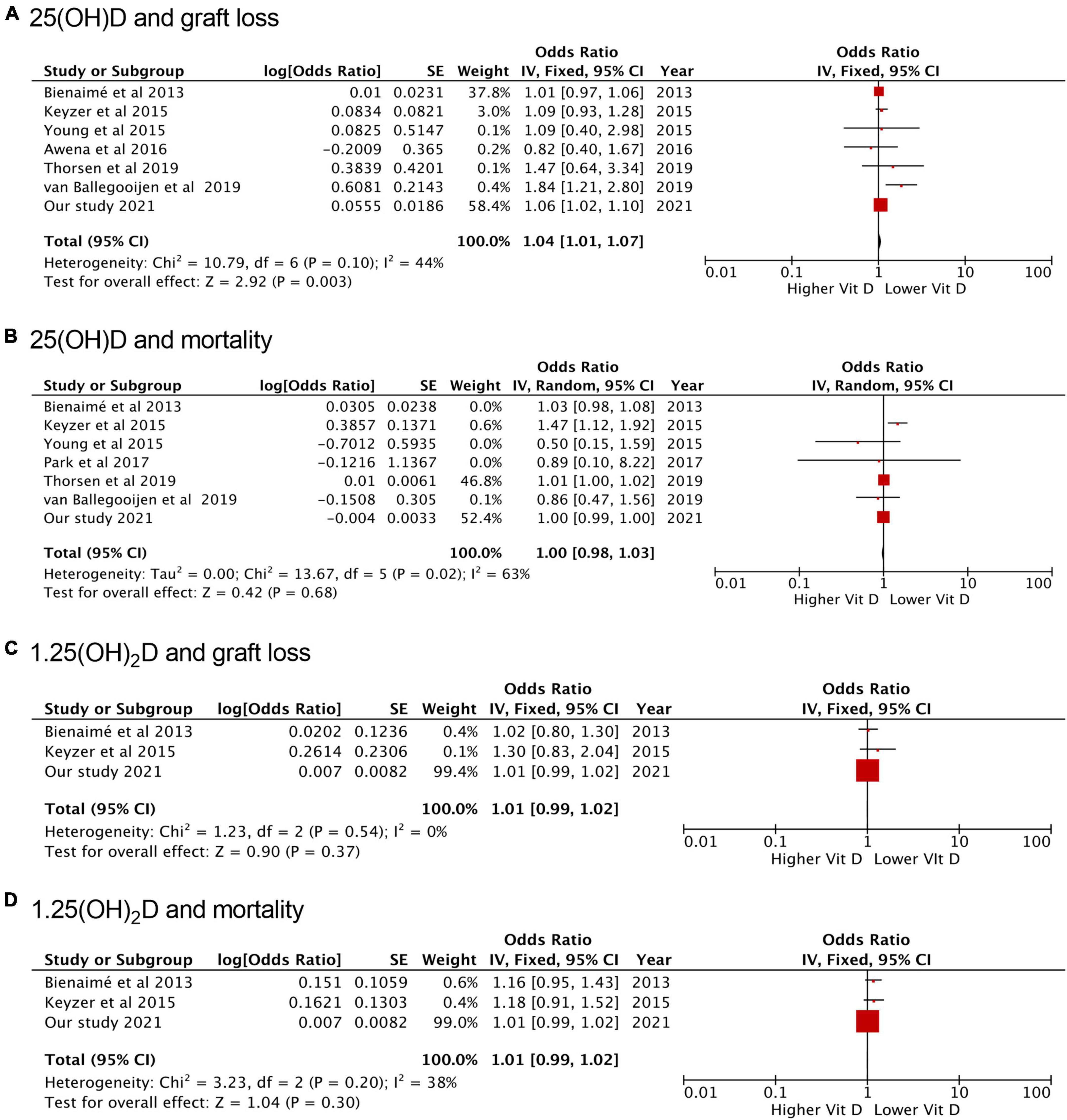
Figure 3. Forest plots of the included studies evaluating the association between vitamin D and death and graft loss. (A) 25(OH)D and graft loss; (B) 25(OH)D and mortality; (C) 1,25(OH)2D and graft loss; (D) 1,25(OH)2D and mortality. SE, standard error; IV, inverse-variance; CI, confidence interval.
Discussion
We performed an observational cohort study and meta-analysis to investigate the effect of low 25(OH)D and 1,25(OH)2D levels measured in stable KTRs on graft failure and all-cause mortality. This meta-analysis is the first to summarize the available evidence on the long-term outcomes of 25(OH)D and 1,25(OH)2D in KTRs head-to-head in one study. The cohort study and meta-analysis results were consistent in finding that baseline 25(OH)D concentrations were independently and inversely associated with graft loss regardless of known confounding factors, whereas 25(OH)D concentrations were not associated with mortality in adult KTRs. In addition, no association of 1,25(OH)2D with mortality and graft failure was found in adult KTRs.
The patient characteristics indicated that the cohort study population was representative of a typical European post-transplant cohort (19). We observed that the incidence rate of renal graft loss was 6.33% (38/600) after a follow-up of 3 years, in line with the average in other centers (2). Therefore, our results are generally applicable. In addition to our cohort study, the meta-analysis included seven clinical studies published on the prognostic impact of vitamin D levels in KTRs with comparable patient characteristics to the study population in our study (Table 4) (11–17).
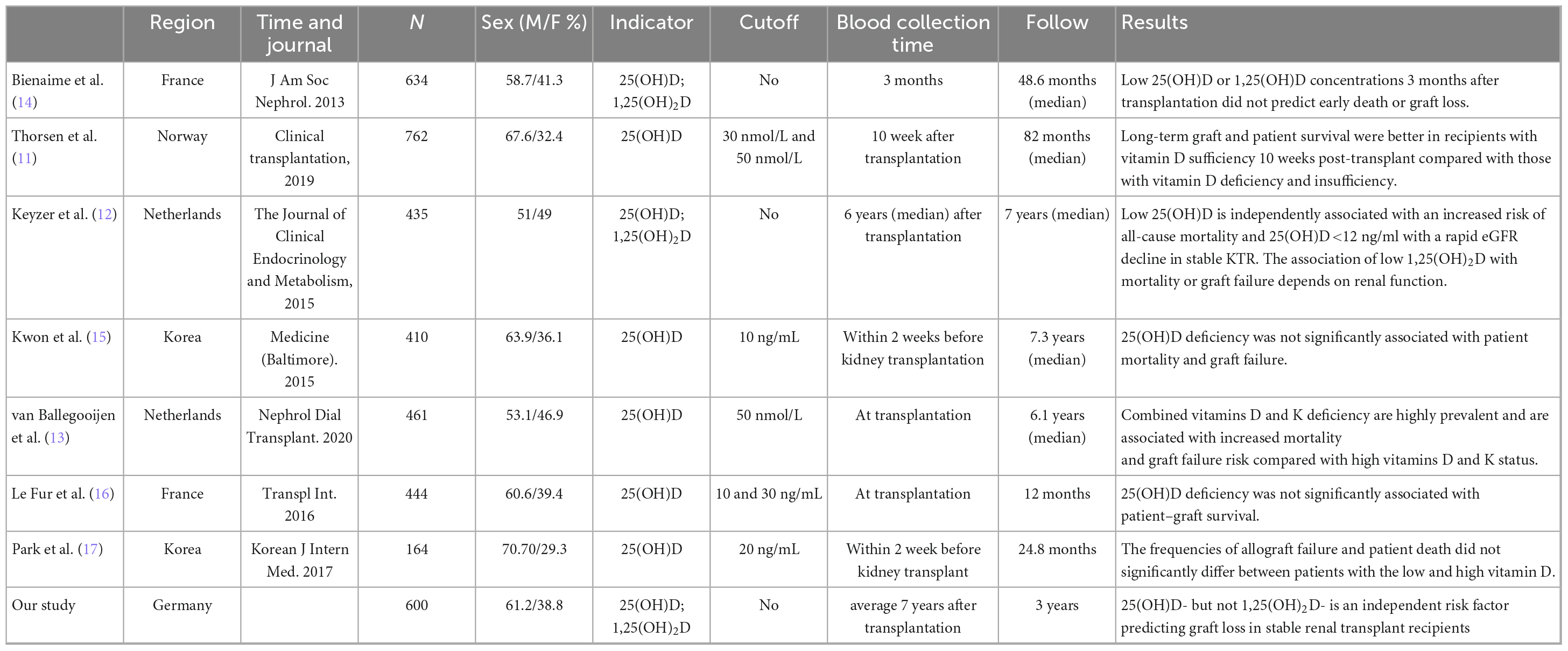
Table 4. Information clinical studies analyzing the correlation between vitamin D levels and survival in renal transplant recipients.
Our cohort study and meta-analysis consistently showed that 25(OH)D concentrations were associated with increased graft loss rates but not mortality. Although some of the previous clinical studies (11–13) suggested a relationship between vitamin D levels and all-cause mortality, we did not find any benefit in patient survival from higher concentrations of 25(OH). This discrepancy may be due to the small sample size of previous studies. They were most likely underpowered for this end-point. Hence, our metanalysis is helpful by combining the evidence from all published studies, including our data, and indicates no mortality effect of either 25(OH)D or 1,25(OH)2D.
Our finding that 25(OH)D is associated with graft loss fits very well with published preclinical studies in the field. VDD deficiency was linked to an impaired kidney function in a CKD animal model (3) and associated with increased graft failure risk in experimental KTRs (11–13). It has been confirmed that vitamin D analogs inhibit kidney fibrosis with potential renoprotective activity in a cyclosporine-induced rat model of CKD (20). Renal vitamin D receptor binding to nuclear response elements is reduced in rats with incipient renal failure (21).
Moreover, the administration of vitamin D is beneficial in experimental transplantation models. 1,25(OH)2D administration prevented acute rejection and prolonged survival in the ACI to Lewis rat renal transplant model (22) and also prevented chronic allograft nephropathy in the Fisher 344 to Lewis rat renal transplantation model (23). A close association between VDD and graft loss was also reported in liver transplant recipients. Martucci et al. (24) showed that after orthotopic liver transplantation, incomplete graft recovery was associated with lower vitamin D on postoperative day (POD) 28 (OR: 0.84; CI 95%: 0.73−0.97; P = 0.014), indicating that the value of vitamin D on POD28 had a strong association with graft function.
The deficiency of 25(OH)D, but not 1,25(OH)2D, in blood circulation is an independent risk factor for diminished allograft survival in our cohort study and meta-analysis. It may be that the stability of 25(OH)D in the circulation makes it more representative of the patient’s vitamin D status. It is known that 25(OH)D has a higher affinity for vitamin D’s transport protein than 1,25(OH)2D but a lower affinity for specific VDR (25). Because of these features, 25(OH)D is considered a transfer form rather than a biological effector in vivo. Compared to 1,25(OH)2D, 25(OH)D in the peripheral circulation usually has a higher concentration, lower affinity, and a longer half-life (26) describing better the average vitamin status and or vitamin D substitution than 1,25(OH)2D and may thus be better linked to outcomes such as graft loss.
The association between low 1,25(OH)2D and graft failure was seen in our cohort and was dependent on renal function. This association is not unexpected since the 1α-hydroxylase of the kidney, i.e., the enzyme that converts 25(OH)D into 1,25(OH)2D, is damaged simultaneously with the deterioration of renal function.
1,25(OH)2D is identified as a VD form with relatively short half-life that is also influenced by adrenocorticotropic hormone (27) and sex hormone (28). Furthermore, 1,25(OH)2D has been shown to reduce glomerulosclerosis and urinary albumin excretion in progressive glomerular damage (25). Furthermore, it has been demonstrated that 1,25(OH)2D has anti-proliferative properties for glomeruli (29) and has a renal protective effect by targeting podocytes (30, 31). Therefore, in the case of chronic kidney damage, it makes little sense to correlate graft loss with 1,25(OH)2D concentrations alone; instead, it is more reasonable to adjust the association of low 1,25(OH)2D concentrations with graft failure and mortality for impaired renal function (12). This could be why 25(OH)D, rather than 1,25(OH)2D, is a better marker of VD status in this patients cohort and can independently predict graft loss.
Given the high prevalence of vitamin D deficiency (VDD) in renal transplant patients and that VDD is treatable, a target concentration of 25(OH)D needs to be determined. The generalized additive model (GAM) (see Figure 2) shows that in the patients with 25(OH)D levels below 150 nmol/L, the hazard ratio decreases with an increase of 25 (OH)D levels in stable KTRs. A recent guideline suggested that vitamin D exceeding 250 nmol/L is deemed a “risk” of vitamin D toxicity (32). In Figure 2, the curve of 25(OH)D with hazard ratio in generalized additive model (GAM), the levels of 310 nmol/L is above zero, and the curve’s trend increases with a numerical increase of 25(OH)D. Since only a very small number of patients had levels of 25(OH)D that exceed the level of possible adverse effects, we cannot make any firm conclusions if an unlimited increase in 25(OH)D may increase the risk ratio of graft failure and at what precise concentration of 25(OH)D this may occur. Nowadays, no clear criteria have been applied to define the deficiency/insufficiency status (33). The bone centered guidelines recommend a target 25(OH)D concentration of at least 20 ng/mL (50 nmol/L), while the guidelines that focus on the pleiotropic effect of vitamin D recommend a higher threshold of 25(OH)D concentration of 30 ng/mL (75 nmol/L). Despite that, it seems evident that most KTRs have moderately or even severely decreased levels of native vitamin D (34, 35). Vitamin D deficiency is a widespread global health problem (36). Since 74% of KTR’s sample was below 30 ng/mL (75 nmol/L) in our cohort, 25(OH)D Vitamin D deficiency/insufficiency in KTRs is more frequent and severe than in the general population. Most KTRs require an additional replenishment of vitamin D. The optimal target interval of vitamin D levels in the healthy general population may not be appropriate for KTRs, and the 25 (OH)D level must be much higher than 50 nmol/mL effectively improve the clinical outcome of KTRs.
Several limitations of our cohort study warrant consideration:
1. Although we have eliminated several potential known confounding factors, we cannot exclude the possibility of remaining confounding, as our study had an observational character.
2. Since barely any patients with the levels of 25(OH)D exceed the level of possible adverse effects [i.e., 25(OH)D levels>250 nmol/L], we do not know what concentration of 25 (OH) D may have an adverse effect.
This meta-analysis has several strengths and limitations:
1. All included studies were observational studies, and 25% were retrospective.
2. The studies assessed 25(OH)D levels at different time points, from 2 weeks before transplant to 6 months after. In addition, the use of different cut-off values may increase heterogeneity among studies.
3. Although early VDD was confirmed as a risk factor for inferior outcomes, studies focusing on the effect of vitamin D supplements on transplant outcomes are lacking.
4. Finally, just total but not the bioactive free form of 25(OH)D was measured. This, however, might be especially important in patients with CKD (33, 37–40).
In conclusion, 25(OH)D was independently associated with graft loss in adult KTRs. The lower optimal range of 25(OH)D to prevent renal graft loss in KTRs seems to be 50 nmol/L. The association of low 1,25(OH)2D with graft failure depends on renal function. Vitamin D deficiency/insufficiency in KTRs is widespread and might be a preventable risk factor for graft loss.
Data availability statement
The raw data supporting the conclusions of this article will be made available by the authors, without undue reservation.
Ethics statement
The protocol was approved by the Ethics Committee of Charité University Hospital (approval number 2012−327) under the Declaration of Helsinki. The patients/participants provided their written informed consent to participate in this study.
Author contributions
BH initiated this study, finalized and critically reviewed the manuscript, and approved the final version. SZ wrote the manuscript and conducted the literature search of the meta-analysis. SL drafted the Figure 2. YY drafted the Figure 3, performed the data extraction, quality assessment, and statistical analysis of the meta-analysis. SL, C-FH, CC, and ZW edited the manuscript and collected data. ZZ, BK, and BH reviewed the manuscript. All authors contributed to the article and approved the submitted version.
Funding
This research was supported by the Hainan Provincial Natural Science Foundation of China (821QN406).
Acknowledgments
We thank the China Scholarship Council supports SZ and CC.
Conflict of interest
The authors declare that the research was conducted in the absence of any commercial or financial relationships that could be construed as a potential conflict of interest.
Publisher’s note
All claims expressed in this article are solely those of the authors and do not necessarily represent those of their affiliated organizations, or those of the publisher, the editors and the reviewers. Any product that may be evaluated in this article, or claim that may be made by its manufacturer, is not guaranteed or endorsed by the publisher.
Supplementary material
The Supplementary Material for this article can be found online at: https://www.frontiersin.org/articles/10.3389/fmed.2023.1141646/full#supplementary-material
Footnotes
References
1. Hart A, Smith J, Skeans M, Gustafson S, Wilk A, Castro S, et al. Optn/Srtr 2017 annual data report: kidney. Am J Trans Offi J Am Soc Trans Am Soc Trans Surg. (2019) 19:19–123. doi: 10.1111/ajt.15274
2. Wekerle T, Segev D, Lechler R, Oberbauer R. Strategies for long-term preservation of kidney graft function. Lancet. (2017) 389:2152–62. doi: 10.1016/s0140-673631283-7
3. de Braganca A, Canale D, Goncalves J, Shimizu M, Seguro A, Volpini R. Vitamin D deficiency aggravates the renal features of moderate chronic kidney disease in 5/6 nephrectomized rats. Front Med. (2018) 5:282. doi: 10.3389/fmed.2018.00282
4. de Boer I, Katz R, Chonchol M, Ix J, Sarnak M, Shlipak M, et al. Serum 25-hydroxyvitamin d and change in estimated glomerular filtration rate. Clin. J Am Soc Nephrol. (2011) 6:2141–9. doi: 10.2215/CJN.02640311
5. Ravani P, Malberti F, Tripepi G, Pecchini P, Cutrupi S, Pizzini P, et al. Vitamin D levels and patient outcome in chronic kidney disease. Kidney Int. (2009) 75:88–95. doi: 10.1038/ki.2008.501
6. Kendrick J, Cheung A, Kaufman J, Greene T, Roberts W, Smits G, et al. Associations of plasma 25-hydroxyvitamin d and 1,25-dihydroxyvitamin d concentrations with death and progression to maintenance dialysis in patients with advanced kidney disease. Am J Kidney Dis Offi J Nat Kidney Found. (2012) 60:567–75. doi: 10.1053/j.ajkd.2012.04.014
7. Rebholz C, Grams M, Lutsey P, Hoofnagle A, Misialek J, Inker L, et al. Biomarkers of vitamin D status and risk of esrd. Am J Kidney Dis Offi J Nat Kidney Found. (2016) 67:235–42. doi: 10.1053/j.ajkd.2015.08.026
8. de Borst M, Vervloet M, ter Wee P, Navis G. Cross Talk between the renin-angiotensin-aldosterone system and vitamin D-Fgf-23-klotho in chronic kidney disease. J Am Soc Nephrol. (2011) 22:1603–9. doi: 10.1681/ASN.2010121251
9. Fishbane S, Chittineni H, Packman M, Dutka P, Ali N, Durie N. Oral paricalcitol in the treatment of patients with CKD and proteinuria: a randomized trial. Am J Kidney Dis Offi J Nat Kidney Found. (2009) 54:647–52. doi: 10.1053/j.ajkd.2009.04.036
10. Keyzer C, van Breda G, Vervloet M, de Jong M, Laverman G, Hemmelder M, et al. Effects of vitamin D receptor activation and dietary sodium restriction on residual albuminuria in Ckd: the virtue-Ckd trial. J Am Soc Nephrol. (2017) 28:1296–305. doi: 10.1681/ASN.2016040407
11. Thorsen I, Bleskestad I, Asberg A, Hartmann A, Skadberg O, Brede C, et al. Vitamin D as a risk factor for patient survival after kidney transplantation: a prospective observational cohort study. Clin Trans. (2019) 33:e13517. doi: 10.1111/ctr.13517
12. Keyzer C, Riphagen I, Joosten M, Navis G, Muller Kobold A, Kema I, et al. Associations of 25(Oh) and 1,25(Oh)2 vitamin D with long-term outcomes in stable renal transplant recipients. J Clin Endocrinol Metab. (2015) 100:81–9. doi: 10.1210/jc.2014-3012
13. van Ballegooijen A, Beulens J, Keyzer C, Navis G, Berger S, de Borst M, et al. Joint association of vitamins d and k status with long-term outcomes in stable kidney transplant recipients. Nephrol Dial Trans. (2020) 35:706–14. doi: 10.1093/ndt/gfy399
14. Bienaime F, Girard D, Anglicheau D, Canaud G, Souberbielle J, Kreis H, et al. Vitamin D status and outcomes after renal transplantation. J Am Soc Nephrol. (2013) 24:831–41. doi: 10.1681/ASN.2012060614
15. Kwon Y, Kim H, Oh H, Park J, Han S, Ryu D, et al. Vitamin D deficiency is an independent risk factor for urinary tract infections after renal transplants. Medicine. (2015) 94:e594. doi: 10.1097/MD.0000000000000594
16. Le Fur A, Fournier M, Gillaizeau F, Masson D, Giral M, Cariou B, et al. Vitamin D deficiency is an independent risk factor for ptdm after kidney transplantation. Transpl Int. (2016) 29:207–15. doi: 10.1111/tri.12697
17. Park Y, Kim S, Lee K, Lee J, Kwon E, Jung H, et al. Vitamin D deficiency is associated with increased risk of bacterial infections after kidney transplantation. Korean J Int Med. (2017) 32:505–13. doi: 10.3904/kjim.2015.214
18. Yin S, Wang X, Li L, Huang Z, Fan Y, Song T, et al. Prevalence of vitamin D deficiency and impact on clinical outcomes after kidney transplantation: a systematic review and meta-analysis. Nutr Rev. (2022) 80:950–61. doi: 10.1093/nutrit/nuab058
19. Kramer A, Pippias M, Stel V, Bonthuis M, Abad Diez J, Afentakis N, et al. Renal replacement therapy in europe: a summary of the 2013 era-edta registry annual report with a focus on diabetes mellitus. Clin Kidney J. (2016) 9:457–69. doi: 10.1093/ckj/sfv151
20. Tomasini-Johansson B, O’Brien C, Larson-Osborne A, Toraason I, Hullett D, Plum L, et al. Effects of the vitamin D analog 2amd in cyclosporine-induced nephrotoxicity: dose-response and antifibrotic activity. Exp Clin Trans. (2017) 15: 641–7.
21. Sawaya B, Koszewski N, Qi Q, Langub M, Monier-Faugere M, Malluche H. Secondary hyperparathyroidism and vitamin d receptor binding to vitamin d response elements in rats with incipient renal failure. J Am Soc Nephrol. (1997) 8:271–8. doi: 10.1681/ASN.V82271
22. Redaelli C, Wagner M, Gunter-Duwe D, Tian Y, Stahel P, Mazzucchelli L, et al. 1alpha,25-dihydroxyvitamin D3 shows strong and additive immunomodulatory effects with cyclosporine a in rat renal allotransplants. Kidney Int. (2002) 61:288–96. doi: 10.1046/j.1523-1755.2002.00101.x
23. Hullett D, Laeseke P, Malin G, Nessel R, Sollinger H, Becker B. Prevention of chronic allograft nephropathy with vitamin D. Transpl Int. (2005) 18:1175–86. doi: 10.1111/j.1432-2277.2005.00187.x
24. Martucci G, Volpes R, Panarello G, Tuzzolino F, Di Carlo D, Ricotta C, et al. Vitamin D levels in liver transplantation recipients and early postoperative outcomes: prospective observational dliverx study. Clin Nutr. (2021) 40:2355–63. doi: 10.1016/j.clnu.2020.10.027
25. Mazzaferro S, Goldsmith D, Larsson T, Massy Z, Cozzolino M. Vitamin D metabolites and/or analogs: which D for which patient? Curr Vasc Pharmacol. (2014) 12:339–49. doi: 10.2174/15701611113119990024
26. Lips P. Relative value of 25(Oh)D and 1,25(Oh)2d measurements. J Bone Miner Res. (2007) 22:1668–71. doi: 10.1359/jbmr.070716
27. Tourkova I, Liu L, Sutjarit N, Larrouture Q, Luo J, Robinson L, et al. Adrenocorticotropic hormone and 1,25-dihydroxyvitamin D enhance human osteogenesis in vitro by synergistically accelerating the expression of bone-specific genes. Lab Invest. (2017) 97:1072–83. doi: 10.1038/labinvest.2017.62
28. Grzesiak M, Waszkiewicz E, Wojtas M, Kowalik K, Franczak A. Expression of vitamin D receptor in the porcine uterus and effect of 1,25(Oh)D on progesterone and estradiol-17β secretion by uterine tissues in vitro. Theriogenology. (2019) 125:102–8. doi: 10.1016/j.theriogenology.2018.10.026
29. Schwarz U, Amann K, Orth S, Simonaviciene A, Wessels S, Ritz E. Effect of 1,25 (Oh)2 vitamin D3 on glomerulosclerosis in subtotally nephrectomized rats. Kidney Int. (1998) 53:1696–705. doi: 10.1046/j.1523-1755.1998.00951.x
30. Kuhlmann A, Haas C, Gross M, Reulbach U, Holzinger M, Schwarz U, et al. 1,25-dihydroxyvitamin D3 decreases podocyte loss and podocyte hypertrophy in the subtotally nephrectomized rat. Am J Physiol Renal Physiol. (2004) 286:F526–33. doi: 10.1152/ajprenal.00316.2003
31. Migliori M, Giovannini L, Panichi V, Filippi C, Taccola D, Origlia N, et al. Treatment with 1,25-dihydroxyvitamin D3 preserves glomerular slit diaphragm-associated protein expression in experimental glomerulonephritis. Int J Immunopathol Pharmacol. (2005) 18:779–90. doi: 10.1177/039463200501800422
32. Munns C, Shaw N, Kiely M, Specker B, Thacher T, Ozono K, et al. Global consensus recommendations on prevention and management of nutritional rickets. J Clin Endocrinol Metab. (2016) 101:394–415. doi: 10.1210/jc.2015-2175
33. Zeng S, Chu C, Doebis C, von Baehr V, Hocher B. Reference values for free 25-hydroxy-vitamin d based on established total 25-hydroxy-vitamin d reference values. J Steroid Biochem Mol Biol. (2021) 210:105877. doi: 10.1016/j.jsbmb.2021.105877
34. Battaglia Y, Cojocaru E, Fiorini F, Granata A, Esposito P, Russo L, et al. Vitamin D in kidney transplant recipients. Clin Nephrol. (2020) 93:57–64. doi: 10.5414/CN109735
35. Messa P, Regalia A, Alfieri C. Nutritional vitamin D in renal transplant patients: speculations and reality. Nutrients. (2017) 9:550. doi: 10.3390/nu9060550
36. Hossein-nezhad A, Holick M. Vitamin D for health: a global perspective. Mayo Clin Proc. (2013) 88:720–55. doi: 10.1016/j.mayocp.2013.05.011
37. Zeng S, Bachert D, Pavkovic M, Sandner P, Hocher C, Tsuprykov O, et al. Free vitamin D is independently associated with systolic blood pressure in diabetic patients with impaired kidney function. Clin Nephrol. (2022) 97:63–9. doi: 10.5414/CN110549
38. Chen X, Lu Y, Luo T, Wu H, Cai S, Tian M, et al. Free 25-vitamin D is correlated with cardiovascular events in prevalent hemodialysis patients but not with markers of renal mineral bone disease. Kidney Blood Press Res. (2019) 44:344–53. doi: 10.1159/000499878
39. Tsuprykov O, Chen X, Hocher C, Skoblo R, Lianghong Y, Hocher B. Why should we measure free 25(Oh) vitamin D J Steroid Biochem Mol Biol. (2018) 180:87–104. doi: 10.1016/j.jsbmb.2017.11.014
Keywords: kidney transplantation, all-cause mortality, graft loss, 25(OH)D, 1, 25(OH)2D
Citation: Zeng S, Yang Y, Li S, Hocher C-F, Chu C, Wang Z, Zheng Z, Krämer BK and Hocher B (2023) 25(OH)D-but not 1,25(OH)2D–Is an independent risk factor predicting graft loss in stable kidney transplant recipients. Front. Med. 10:1141646. doi: 10.3389/fmed.2023.1141646
Received: 14 February 2023; Accepted: 03 April 2023;
Published: 20 April 2023.
Edited by:
Sing-Chung Li, Taipei Medical University, TaiwanReviewed by:
Chiao-Ming Chen, Shih Chien University, TaiwanPetru Cianga, Grigore T. Popa University of Medicine and Pharmacy, Romania
Copyright © 2023 Zeng, Yang, Li, Hocher, Chu, Wang, Zheng, Krämer and Hocher. This is an open-access article distributed under the terms of the Creative Commons Attribution License (CC BY). The use, distribution or reproduction in other forums is permitted, provided the original author(s) and the copyright owner(s) are credited and that the original publication in this journal is cited, in accordance with accepted academic practice. No use, distribution or reproduction is permitted which does not comply with these terms.
*Correspondence: Berthold Hocher, YmVydGhvbGQuaG9jaGVyQG1lZG1hLnVuaS1oZWlkZWxiZXJnLmRl
†These authors share first authorship