- Department of Medical Microbiology, University Medical Center Groningen, University of Groningen, Groningen, Netherlands
Torquetenovirus (TTV), a small, single stranded anellovirus, is currently being explored as a marker of immunocompetence in patients with immunological impairment and inflammatory disorders. TTV has an extremely high prevalence and is regarded as a part of the human virome, the replication of which is controlled by a functioning immune system. The viral load of TTV in plasma of individuals is thought to reflect the degree of immunosuppression. Measuring and quantifying this viral load is especially promising in organ transplantation, as many studies have shown a strong correlation between high TTV loads and increased risk of infection on one side, and low TTV loads and an increased risk of rejection on the other side. As clinical studies are underway, investigating if TTV viral load measurement is superior for gauging antirejection therapy compared to medication-levels, some aspects nevertheless have to be considered. In contrast with medication levels, TTV loads have to be interpreted bearing in mind that viruses have properties including transmission, tropism, genotypes and mutations. This narrative review describes the potential pitfalls of TTV measurement in the follow-up of solid organ transplant recipients and addresses the questions which remain to be answered.
Introduction
Torquetenovirus (TTV) is currently being explored as a functional marker of the immune system in patients with immunological impairment and inflammatory disorders. The discovery of TTV in 1997, and subsequently other members of the anelloviridae family, have led to the concept of “commensal viruses”(1), defined as constituents of the human virome, not known to cause pathology in humans. TTV has an extremely high prevalence and has been detected in various conditions involving immune dysfunction and immune activation, including congenital and iatrogenic immunodeficiency, chronic viral infections and aging (2–6). The replication of TTV, similar to all the viruses constituting the virome, is controlled by a functioning immune system. And quantifying the virus load in blood is thought to be a potential read-out of this functionality. Nearly all studies investigating TTV in infectious and inflammatory processes show a correlation between unfavorable outcome or disease progression, and increasing or higher TTV loads (Tables 1, 2).
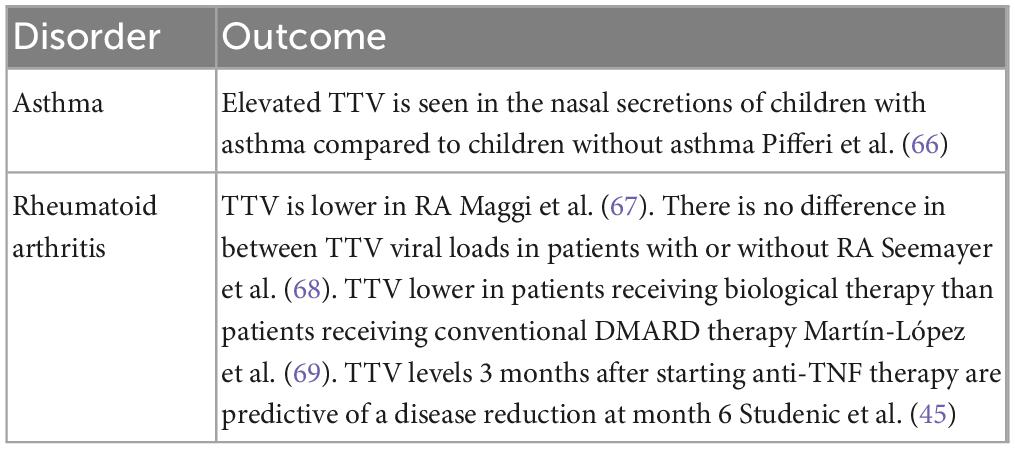
Table 2. Examples of inflammatory diseases in which TTV has been investigated as a prognostic marker.
A biomarker of the immune status is especially promising in solid organ transplantation, where it could improve the lives of recipients (7). Antirejection therapy, which is essential for maintaining a transplanted organ, has side-effects and increase the recipient’s risk of developing an infection (8). Currently, antirejection medication is adjusted in response to trough levels of these drugs in blood. However, trough levels do not reliably predict rejection or infection in patients (9). A biomarker of the immune status would provide greater insight into the biological effects of the immunosuppressive drugs, and reduce the dangers of suboptimal dosing. Several potential biomarkers have been investigated in recent years, including T-cell subsets, T-cell proliferation assays and soluble CD30 (10–12). TTV is the most investigated biomarker in this context, with most studies showing that high TTV loads are associated with an increased risk of infection, and low TTV loads are associated with an increased risk of rejection (Table 3) (3). TTV viral load measurement in blood has advantages as a PCR can be done in a few hours, is affordable, and easy to perform. Moreover, stored blood samples can be tested, meaning that TTV measurements may also be used to obtain data retrospectively (13).
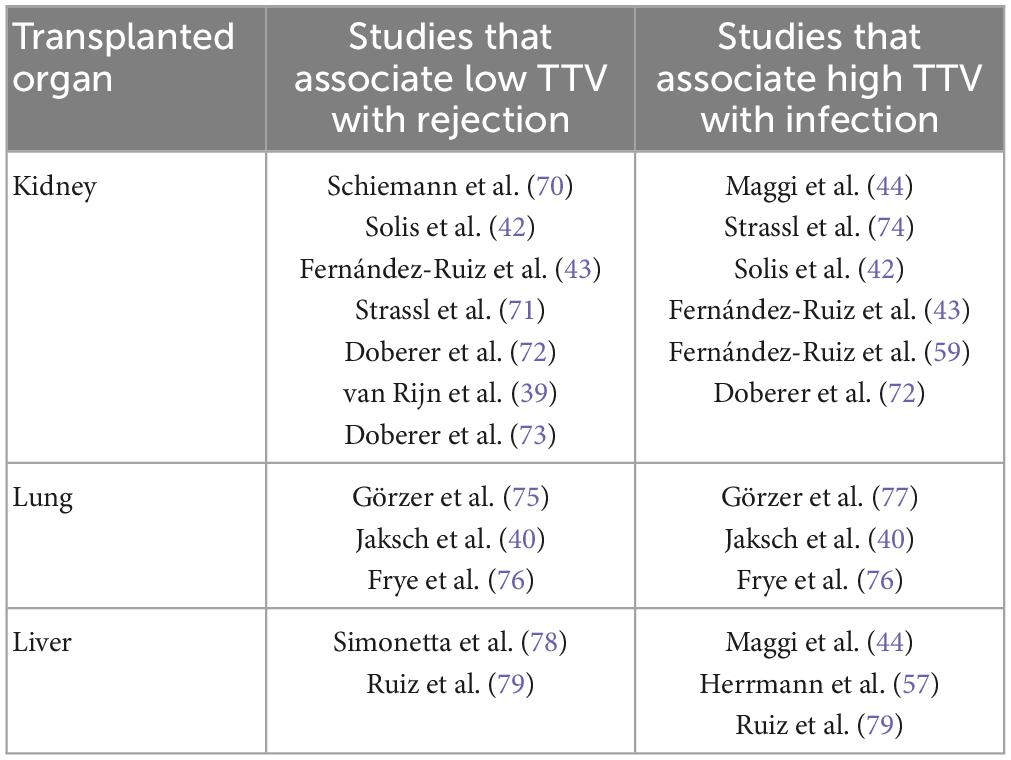
Table 3. Examples of studies which have shown that TTV is an immune marker in solid organ transplantation.
Two important clinical trials are currently being conducted, examining whether TTV viral load is superior to trough level measurements of the calcineurin inhibitor tacrolimus, when adjusting the dose of antirejection medication. The VIGILung Trial (Clinical trials number NCT04198506) is a multicenter study initiated in Germany and Austria in lung transplantation patients. This study was set up to investigate if a reduction in nephrotoxicity in the first year after transplantation can be achieved. 144 patients are randomized to undergo tacrolimus dose adjustments either based on TTV levels, or based on trough levels. The VIGILung trial is due to end in 2025 (14).
The second clinical trial is the TTV-GUIDE IT study, which is a multicenter European study in sixteen transplantation centers. This study was designed to explore if TTV can be safely used to adjust the dose of tacrolimus in kidney transplantation patients (EU CT-Number 2022-500024-30-00). A total of 260 patients are be randomized in to two groups. One group will have their tacrolimus dose adjusted based on TTV viral load, while the other group will have the tacrolimus adjusted based on tacrolimus trough levels. Patients will be followed for 13 months after transplantation with infection, allograft rejection, graft-loss and death as the primary outcomes. The TTV GUIDE IT study is due to end in August 2025 (15).
However, before TTV quantification can be implemented as a more reliable alternative to trough level measurements, there are some aspects that have to be considered. After all, in contrast with medication levels, when TTV loads are interpreted, virological features including transmission, tropism, mutations, viral variations and epidemiology, should be taken into account, as these may be relevant in the follow-up of solid organ transplant recipients (SOTR).
Virology of TTV
Torquetenovirus is a small, non-enveloped, circular, negative-sense single stranded DNA anellovirus. The virus features an enormous sequence diversity, with so far five identified genogroups and over 50 genotypes. Sequence divergence within the family is high, as these five genogroups display at least 50% nucleotide divergence, and the different genotypes up to 30% divergence. The coding region of the TTV genome contains at least four open reading frames (ORFs), of which one contains three hypervariable regions. The untranslated region (UTR) is well preserved between the genotypes and represents a target for detection PCRs. TTV was the first identified member of the anellovirus family, however, more recently, torque teno minivirus (TTMV) and torque teno midivirus (TTMDV) have been identified in 2000 and 2007, respectively. In addition, several anelloviruses have been described in other mammals (Figure 1) (16, 17).
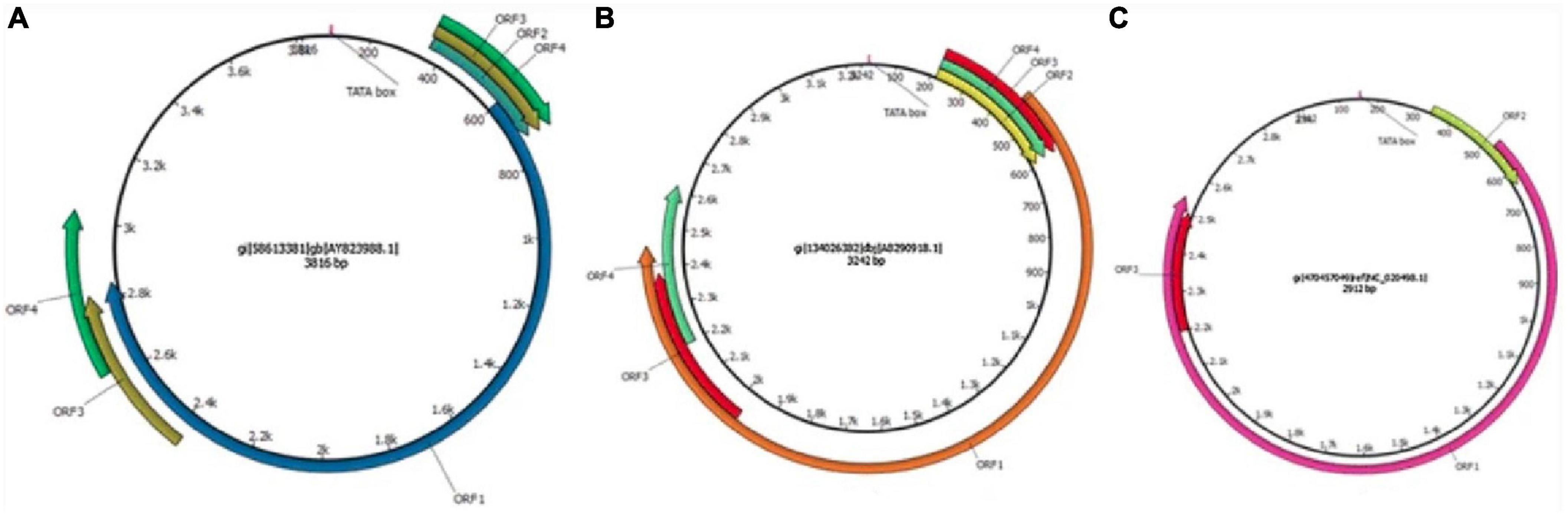
Figure 1. Schematic illustration of the genome organization of torque teno viruses. (A) TTV isolate 2 h (GenBank accession no. AY823988), (B) TTMDV isolate MD1-073 (GenBank accession no. AB290918), (C) TTMV isolate TTMV_LY1 (GenBank accession no. NC_020498.1). The arrows represent open reading frames. Used with permission (16).
Torquetenovirus is thought to induce long term or chronic infections, with no known negative effects. The virus is found in many clinical syndromes where inflammation is part of the process, but also in the general population TTV can be detected in 2–90% of individuals (17). Studies in infants and children have found that transmission occurs early in life, likely occurring via breastmilk, saliva, the fecal-oral route, or through respiratory and droplet transmission. In addition, transmission in a healthcare setting is conceivable, as blood transfusions are a recognized transmission route. Longitudinal studies in infants showed that nearly all were infected with TTV before the age of 4 (18–21). Moreover, coinfections with different genotypes are common. Two different genotypes can be detected in most individuals and some individuals are co-infected with multiple genotypes, belonging to up to four different genogroups (18, 21).
Replication of TTV
As with most viruses with a circular genomes it is believed that TTV replicates using rolling circle replication and escapes from infected cells through cell lysis (22, 23) It is as yet unclear precisely which cells are responsible for TTV replication. It was first reported from in vitro experiments in 2002, that activated PBMCs produce TTV (24). Longitudinal studies in hematology and transplantation patients showed subsequently that T- lymphocytes are the main source of TTV in peripheral blood (25–28). It was observed that cellular depletion resulting from conditioning for stem cell transplantation (SCT), resulted in a temporary loss of nearly all TTV in peripheral blood (25, 26). As lymphocytes start to reappear at first engraftment, TTV increases rapidly until reaching a peak at 60 days after SCT, after which a gradual decline is observed paralleling immune-reconstitution. In SOTR, many of whom have detectable TTV prior to transplantation, TTV loads decrease significantly in the first few days after induction immunosuppression therapy with T-lymphocyte inhibitors. Focosi et al. reported that T-cell depletion with Anti Thymocyte Globulin (ATG) in kidney transplant recipients induced a stronger decrease in TTV load than the less potent T-cell inhibitor basiliximab (27). Also, a higher ATG dose had a greater effect on the TTV load than a lower dose. Görzer et al. described a similar early decrease of the TTV load in lung transplant recipients (LTR) after induction with alemtuzumab (28). In this very early post-transplantation period, low or negative TTV loads should clearly not be regarded as a reflection of a functional immune system, but rather an effect of profound T-cell lymphopenia. After the initial period, TTV loads increased rapidly in all these cases, reflecting an immunosuppressive status.
TTV DNA prevalence in materials and populations
Besides blood, TTV can be detected in many tissues and fluids, to such an extent that it is thought to be pantropic. Some researchers have reported fairly high viral loads in breastmilk, respiratory secretions, and saliva (29, 30). Some caution has to be taken in interpreting these sometimes conflicting results as many of these studies were carried out with different PCR assays resulting in higher or lower detection limit. There is no official standard yet to calibrate quantitative tests, and until recently, there was no commercial assay (31). Some research teams developed their own tests to detect and quantify the virus, with different sensitivities (29). In light of the enormous genotypic diversity of TTV and the various sensitivities of the PCR tests used to detect the virus, quantitative results are difficult to compare, not only between materials, but also populations. As such, healthy blood donors were found to have detectable TTV loads varying between 4% of cases in Iran to 96% of cases in Jordan. Within Europe, where many TTV-related studies are conducted, prevalence among healthy donors ranged between 46% in Italy and 76% in neighboring Austria, when tested with laboratory developed PCR tests (6, 32–34). A large virome study using whole genome sequencing, which is known to be less sensitive, showed that 14% of individuals from the USA had detectable TTV (35). There is not only a difference in the prevalence of TTV worldwide but the prevalence of certain genotypes also appears to change depending on the population studied (13, 16). The development of a commercial PCR test will make comparisons between studies, and thereby between populations and even different patient materials, much easier in future. Newly developed external quality control schemes, developed by QCMD (Quality Control in Molecular Diagnostics, Glasgow, Scotland, UK) as well as controls used in studies, should enable more standardization in viral load measurements within clinical studies.
The immune response and TTV
Investigations into both the replication cycle of TTV as well as the immune response inhibiting replication, have undoubtedly been hampered by the fact that culturing this virus has been extremely difficult with few groups reporting success (22, 36). The almost ubiquitous nature of the virus also does not allow for comparisons between individuals who are carriers of active or dormant TTV replication in blood. Therefore the consequences of the antigenic pressures of this virus in infected individuals, or the absence of antigenic pressure in uninfected individuals has not been explored. Rocchi et al. showed that unmethylated CpG DNA of TTV is capable of inducing an inflammatory response through activation of TLR-9 in vitro (37). No studies have confirmed this finding in vivo, but longitudinal studies in which the acquisition of primary TTV infections and acquisition of new genotypes have been followed, do not seem to point toward a systemic inflammatory response to these infections (18, 21). As more evidence is accumulating that rejection and some infections after SOT are correlated with the TTV load measured in blood, the thought that the immunological pathways involved in these processes may overlap with the immune response against TTV is appealing. More research into these immunological pathways is urgently needed. In addition, greater understanding of how the various immunosuppressants impact the TTV load, is essential for any study investigating the use of the virus in the follow-up of SOTR.
In lung and kidney transplantation, the standard antirejection regimen is a combination of three drugs, consisting of a calcineurin inhibitor [mostly tacrolimus (TAC) or cyclosporine], a proliferation inhibitor [usually mycophenolate mofetil (MMF) or azathioprine (AZA), and prednisolone] (38). Alternative regimens may include mammalian target of rapamycin inhibitors (mTOR inhibitors) and belatacept. Immediately after transplantation, induction of immunosuppression may incorporate T-lymphocyte inhibitors such as ATG, alemtuzumab and basiliximab. Despite the above recommendations following SOT there is much variation in the regimes chosen by transplantation centers due to the availability of medication and inter-center culture. Further changes in the regimen during the follow-up period are usually motivated by side effects or other adverse events experienced by the patient. These practices explain the absence of large studies investigating TTV loads in patients groups of similar time after transplantation, in which different antirejection regimens are given in equal proportions to different study cohorts. Comparisons between different agents and their separate effects on the TTV load, have to be made by comparing either different transplantation centers with different standard regimens, or by comparing patient cohorts spanning a longer period of time, during which the standard regimen changed. This is exemplified by Görzer et al. who investigated the TTV load during the immediate post-transplantation period, in which all of the 46 lung transplantation recipients received alemtuzumab conditioning (28). Focosi et al. compared induction with basiliximab and ATG in kidney and pancreas transplantation, but in this study no one received alemtuzumab (27). van Rijn et al. reported the TTV load in a series of kidney transplantation patients in which the regime changed from cyclosporin-based to tacrolimus-based, during the study period (39). Jaksch et al. followed 143 LTRs, of which most patients (n = 133) received alemtuzumab conditioning (40). And because many of these studies were conducted with different TTV detection PCRs, the differential effect of the antirejection drugs are difficult to assess, even more so in the long term. To date, some studies have shown that TTV loads are relatively higher in tacrolimus-based regimens, as opposed to those based on cyclosporine, whereas others could not confirm this (41–44). Belatacept is not frequently used in any of the studies, but transplant patients receiving this agent seem to have lower TTV loads than those with TAC-based regimens (45). The same could be true for patients receiving mTOR inhibitors (46). Future studies aiming to use TTV to gauge the antirejection therapy should answer the question how much which agent has to be increased or decrease for the TTV load to move toward a desired level.
The significance of a negative TTV PCR
There are multiple ways for a SOTR to become infected with TTV. The patient comes into contact with multiple sources of TTV, through the donation of the organ and through blood products if they are given. Finding a SOTR with no detectable TTV in blood therefore should be an extremely rare event, considering the immunosuppression induced by the antirejection therapy. Some cross-sectional and longitudinal studies nevertheless show that considerable numbers of SOTR may have a negative PCR at various timepoints during the follow up period (39, 47). The interpretation of a single negative sample or a series of samples from a particular patient is difficult, as there are several possible explanations. It could be the case that most of these patients have suppression of TTV replication due to a highly functional immune system. But there are several reasons, other than immunological control, why TTV may be undetectable. Extreme lymphopenia, the most obvious cause for TTV-negativity, can easily be excluded. When a reasonable number of circulating lymphocytes is present in the blood, several other explanations remain for a not detecting TTV. Firstly: the test in use fails to detect the genotypes infecting this individual, this is a theoretical possibility. With five genogroups known, and 29 genotypes of TTV found, it is conceivable that assays are not able to detect all genotypes. The commercial assay from Biomerieux is able to detect eight genotypes (31). Secondly, a PCR test may be negative simply because a patient has failed to become infected with TTV. Studies such as done by Görzer et al. show that all 143 LTR included in the study develop positive TTV loads within weeks after transplantation (28). Yet, even the most prevalent viruses are unable to infect the entire population. If a study were conducted to investigate the use of TTV after SOT, these rare individuals, along with the ones that are negative because their genotypes are not detected, could easily be identified and excluded from this type of follow-up. Knowing that nearly all SOTR will become TTV positive after induction of antirejection treatment, after the initial lymphopenic phase, TTV could be used as a marker of immunosuppression used in the majority of SOTR.
The third reason for a negative TTV test not implying immunological control, is the possibility that the immune system could clear a TTV infection. If it is possible that an individual clears TTV, than a TTV load would also remain negative, even after increasing the immunosuppression. The viral load would no longer be suitable as a marker for immunosuppression. One case reports details the appearance and disappearance of a TTV genogroup 2 infection, which could be an example of an infection which was subsequently cleared (48). Since there is no way to distinguish these virus-clearing patients from elite controllers, this would represent a conceivable risk.
Significance of a high TTV load
Just as being TTV DNA -negative may not always mean someone is an elite TTV controller, there are reasons why a high TTV load may not always reflect a bad immune response. The main uncertainty is that it is not at all clear how new infections with different genotypes are dealt with by the immune system. A longitudinal study in children showed that the acquisition of new genotypes resulted in recurrent high viral loads (18). Moreover, Maggi et al. showed that individuals carrying more TTV genogroups had higher viral loads in general (20). These studies also suggest that it is a relatively common occurrence to become infected with new genotypes. The study in children shows that five out of five children acquired new genotypes during the study period. Maggi et al. showed that three out of three stem cell transplantation recipients were infected with new genogroups after SCT (21).
The question of the ideal TTV load
Despite the potential for both highly positive and negative TTV loads not necessarily representing immunological control, multiple studies have shown a strong link between high TTV load and the risk of infection, as well as a relationship between low TTV load and the risk of rejection (Table 3). These studies were recently summarized by van Rijn et al., who conducted a meta-analysis (3). The definition of what constitutes a high and low TTV load varies depending on the method used, type of organ transplantation and period of observation. Nearly all the research on this subject was carried out by a limited number of research groups which, for a long time, used their own test, with their own definitions of a high or al low viral load. The correlation between low TTV levels and rejection seems to be stronger than the correlation between high TTV levels and infection risk (3). A major drawback in the studies is that the follow-up period is restricted to, in most cases, one or two years after transplantation. When observing the data, it is important to reflect on the type of infections that are usually seen during this very early time frame after transplantation and whether these infections are the result of the immunosuppression (8). Studies investigating TTV viral load versus infection risk, frequently investigate infections which are prominent in particular SOT populations, but that are only in part due to the immunosuppression. CMV infections are bound to occur when an organ from a seropositive donor is transplanted into a seronegative recipient. CMV reactivations in SOTR who were seropositive prior to transplantation may be caused by the degree of immunosuppression, but not all authors have concluded this (3, 39, 44). Urinary tract infections are particularly common after kidney transplantation because of urological problems resulting from new urinary tract connections made during the operation. Also, BK virus, which is found to correlate with immunosuppression in kidney transplant recipients (KTR) (49), is very uncommon in thoracic organ recipients, in spite of a higher dosing of immunosuppressants and median TTV load found in the latter group (50, 51). Infections which occur in all types of SOTR are more likely the result of immunosuppression, as is the case in chronic norovirus infections (52). These infections are nevertheless too infrequent to be statistically relevant, and they may occur at any time after transplantation, when recipients are no longer followed by the transplantation center.
A rapidly increasing TTV load after transplantation leading to a peak in the first months, is universally seen in studies. Furthermore, observations during the first years after transplantation imply that the TTV peak is followed by a plateau and thereafter a slow reduction in TTV (Figure 2) (47). In contrast, cross-sectional studies suggest that SOTR who are further away from the transplantation date have lower TTV loads than those who were transplanted more recently. This was shown in research by our team in KTR with a follow-up period of up to 30 years after transplantation. The TTV load was significantly lower in KTR longer after transplantation. The study also showed that infections and the mortality rate due to an infectious cause were higher in patients with high TTV load, whereas we were not able to show a correlation between low TTV load and the risk for rejection (47). Likewise, a study in lung transplant recipients (LTR) showed that the TTV load was lower in recipients who were long after the date of transplantation. These patients also had a better response to the COVID-19 vaccine (53). The conclusion that TTV is lower in patients who were transplanted longer ago, was also drawn by others in both KTR and LTR, confirming that TTV load predicted the response to the COVID-19 vaccine (54, 55). These cross sectional studies suggest that a plateau phase lasting for several years is debatable, but equally support the hypothesis that a high TTV load in the long term still reflects over-immunosuppression. A low TTV load nevertheless may over years become less predictive for the risk of rejections (47).
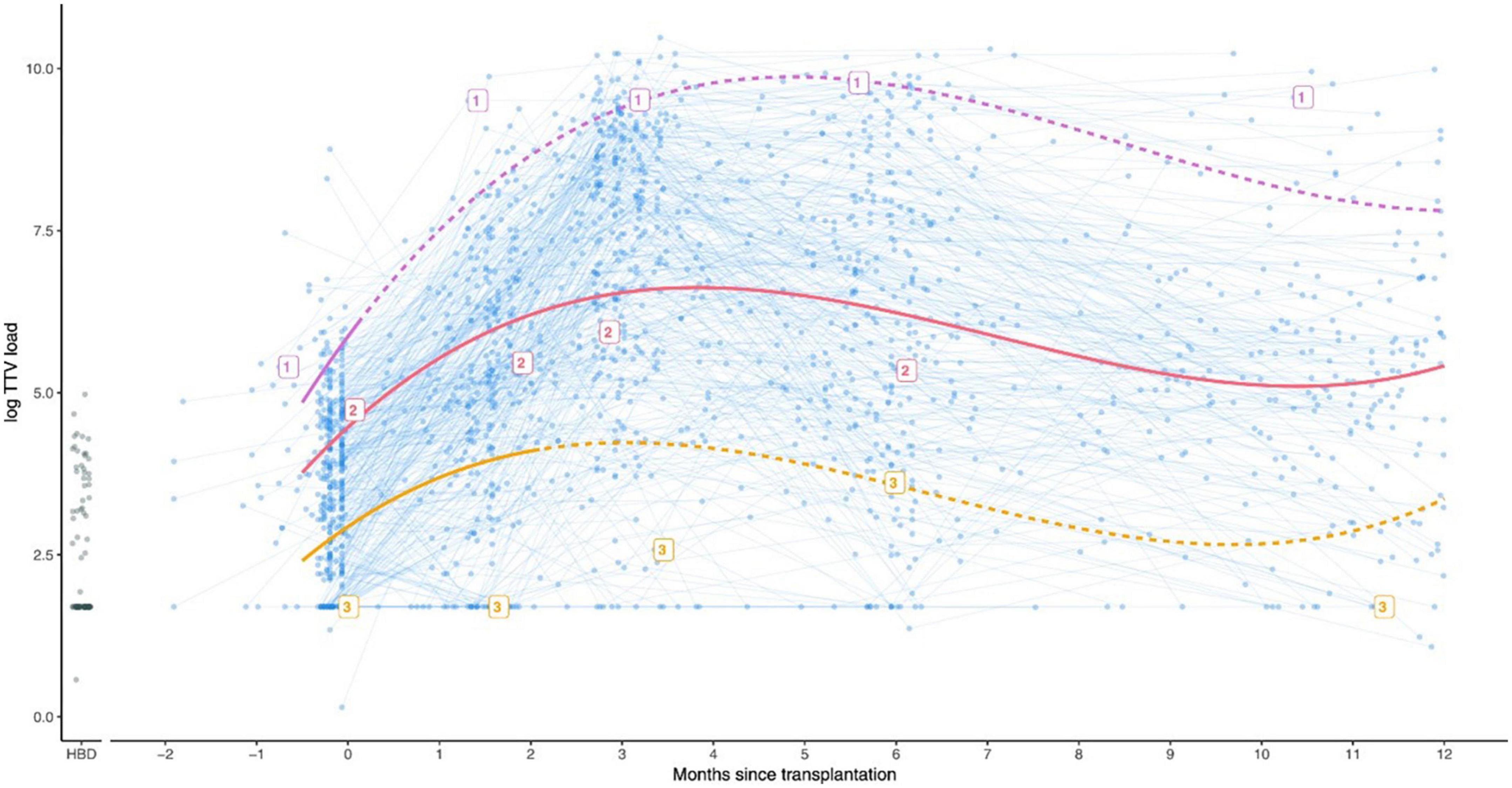
Figure 2. Graph showing the observed TTV loads for kidney transplant recipients (blue), and a group of 88 healthy blood donors for comparison (HBD, shown in black circles). In addition, three example patients indicated with the labels 1, 2, and 3 are shown. Patient 1 (purple) developed rejection 5 days after transplantation and was treated accordingly with a course of strong immunosuppressants. Patient 2 (pink) did not develop rejection. Patient 3 (orange) developed rejection 64 days after transplantation. Used with permission (39).
Discussion and outlook
Two decades after the discovery of TTV as a first representative of the human virome, insights on how this virus can be used to assess the function of the immune system have evolved rapidly. Accumulating evidence points at the potential use for TTV in immunocompromised patients. SOTR may benefit most from a marker of immunosuppression, as in this population the degree of immunosuppression is amendable and can be adjusted to a desired level. As to what constitutes a desired level will have to be answered by clinical studies, such as the ones which are currently conducted. Both these two clinical studies use the commercially available TTV-quantitative PCR test in their protocols, and exclude participants with consistently negative TTV loads after transplantation, thereby overcoming some of the limitations detailed above. While the VIGILung trial focusses on TTV as a predictor of toxicity associated with high dosages of tacrolimus, the TTV GUIDE IT study aims to examine the immunosuppressive status of the SOTR during the first few months after transplantation, similar to most of the longitudinal studies which have been published to date. The currently available data indicates a significant correlation between rejection and low TTV loads during precisely this period (Table 3). In contrast, the relationship between high TTV loads and risk for infection is less clear from existing evidence (3). Although there are several reports showing a correlation, infections after transplantation are not always caused by over-immunosuppression alone and their incidence can sometimes be decreased by use of prophylactic medication, as is the case for CMV infections (3, 7, 8). In the long term, over-immunosuppression may well represent the highest risk to the majority of transplant recipients (7). Dose-dependent side effects of antirejection drugs, as well as infections have a huge impact on the quality of life of transplant recipients and continuing graft survival (7, 49, 56). It is conceivable that TTV load could also be used as a marker to adjust immunosuppression 5, 10, or 20 years after transplantation, but there is as yet not enough evidence supporting that hypothesis. Barring a small number of cross-sectional studies, there are no studies investigating the use of TTV load measurement for longer than three years after transplantation. Such studies are still needed to investigate if a particular TTV load signifies the same risks several years after transplantation, or whether the ideal TTV load is changes in time. Aside from research in the organ transplant population which seems to be gaining momentum, more research is in general needed to answer remaining questions regarding the prevalence of TTV, the meaning of a negative TTV load, genotypic variation and detection of these genotypes by different assays, as well as replication of TTV and the immune response to this virus. Nevertheless, the two clinical studies into the use of TTV in the follow-up of SOTR represent an exciting new way of thinking in which the virome is used as a tool to assess the immune system.
Author contributions
EG contributed to collecting articles, appraising references, and correcting the first draft of the manuscript. LG contributed to writing paragraphs with molecular data and critically reading and correcting the manuscript. HN contributed to critically reading the manuscript and editing. CV contributed to drafting the first manuscript and appraising references. All authors contributed to the article and approved the submitted version.
Conflict of interest
The authors declare that the research was conducted in the absence of any commercial or financial relationships that could be construed as a potential conflict of interest.
Publisher’s note
All claims expressed in this article are solely those of the authors and do not necessarily represent those of their affiliated organizations, or those of the publisher, the editors and the reviewers. Any product that may be evaluated in this article, or claim that may be made by its manufacturer, is not guaranteed or endorsed by the publisher.
References
1. Griffiths P. Time to consider the concept of a commensal virus? Rev Med Virol. (1999) 9:73–4. doi: 10.1002/(SICI)1099-1654(199904/06)9:2<73::AID-RMV254>3.0.CO;2-5
2. Maggi F, Pifferi M, Michelucci A, Albani M, Sbranti S, Lanini L, et al. Torque teno virus viremia load size in patients with selected congenital defects of innate immunity. Clin Vaccine Immunol. (2011) 18:692–4. doi: 10.1128/CVI.00466-10
3. van Rijn A, Roos R, Dekker F, Rotmans J, Feltkamp M. Torque teno virus load as marker of rejection and infection in solid organ transplantation – a systematic review and meta-analysis. Rev Med Virol. (2023) 33:e2393. doi: 10.1002/rmv.2393
4. Al-Qahtani A, Al-Anazi M, Abdo A, Sanai F, Al-Hamoudi W, Alswat K, et al. Correlation between genetic variations and serum level of interleukin 28B with virus genotypes and disease progression in chronic hepatitis C virus infection. J Immunol Res. (2015) 2015:768470. doi: 10.1155/2015/768470
5. AbuOdeh R, Al-Mawlawi N, Al-Qahtani A, Bohol M, Al-Ahdal M, Hasan H, et al. Detection and genotyping of torque teno virus (TTV) in healthy blood donors and patients infected with HBV or HCV in Qatar. J Med Virol. (2015) 87:1184–91. doi: 10.1002/jmv.24146
6. Haloschan M, Bettesch R, Görzer I, Weseslindtner L, Kundi M, Puchhammer-Stöckl E. TTV DNA plasma load and its association with age, gender, and HCMV IgG serostatus in healthy adults. Age (2014) 36:9716. doi: 10.1007/s11357-014-9716-2
7. Bamoulid J, Staeck O, Halleck F, Khadzhynov D, Brakemeier S, Dürr M, et al. The need for minimization strategies: current problems of immunosuppression. Transpl Int. (2015) 28:891–900. doi: 10.1111/tri.12553
8. Cippà P, Schiesser M, Ekberg H, van Gelder T, Mueller N, Cao C, et al. Risk stratification for rejection and infection after kidney transplantation. Clin J Am Soc Nephrol. (2015) 10:2213–20. doi: 10.2215/CJN.01790215
9. Dendle C, Mulley W, Holdsworth S. Can immune biomarkers predict infections in solid organ transplant recipients? a review of current evidence. Transplant Rev. (2019) 33:87–98. doi: 10.1016/j.trre.2018.10.001
10. Calarota S, Zelini P, De Silvestri A, Chiesa A, Comolli G, Sarchi E, et al. Kinetics of T-lymphocyte subsets and posttransplant opportunistic infections in heart and kidney transplant recipients. Transplantation (2012) 93:112–9. doi: 10.1097/TP.0b013e318239e90c
11. Wang D, Wu W, Chen J, Yang S, Wang Q, Zeng Z, et al. Pre-transplant soluble CD30 level as a predictor of not only acute rejection and graft loss but pneumonia in renal transplant recipients. Transpl Immunol. (2010) 22:115–20. doi: 10.1016/j.trim.2009.12.004
12. Valor L, Sarmiento E, Navarro J, Gallego A, Fernandez-Yañez J, Fernandez-Cruz E, et al. Evaluation of lymphoproliferative responses by carboxy fluorescein succinimidyl ester assay in heart recipients with infections. Transplant Proc. (2012) 44:2649–52. doi: 10.1016/j.transproceed.2012.09.054
13. Macera L, Spezia P, Medici C, Rofi E, Del RM, Focosi D, et al. Comparative evaluation of molecular methods for the quantitative measure of torquetenovirus viremia, the new surrogate marker of immune competence. J Med Virol. (2022) 94:491–8. doi: 10.1002/jmv.25488
14. Gottlieb J, Reuss A, Mayer K, Weide K, Schade-Brittinger C, Hoyer S, et al. Viral load-guided immunosuppression after lung transplantation (VIGILung)—study protocol for a randomized controlled trial. Trials (2021) 22:48. doi: 10.1186/s13063-020-04985-w
15. Haupenthal F, Rahn J, Maggi F, Gelas F, Bourgeois P, Hugo C, et al. A multicentre, patient- and assessor-blinded, non-inferiority, randomised and controlled phase II trial to compare standard and torque teno virus-guided immunosuppression in kidney transplant recipients in the first year after transplantation: TTVguideIT. Trials (2023) 24:213. doi: 10.1186/s13063-023-07216-0
16. Spandole S, Cimponeriu D, Berca L, Mihaescu G. Human anelloviruses: an update of molecular, epidemiological and clinical aspects. Arch Virol. (2015) 160:893–908. doi: 10.1007/s00705-015-2363-9
17. Redondo N, Navarro D, Aguado J, Fernández-Ruiz M. Viruses, friends, and foes: the case of Torque teno virus and the net state of immunosuppression. Transpl Infect Dis. (2022) 24:e13778. doi: 10.1111/tid.13778
18. Väisänen E, Kuisma I, Mäkinen M, Ilonen J, Veijola R, Toppari J, et al. Torque teno virus primary infection kinetics in early childhood. Viruses. (2022) 14:1277. doi: 10.3390/v14061277
19. Tyschik E, Rasskazova A, Degtyareva AV, Rebrikov DV, Sukhikh G. Torque teno virus dynamics during the first year of life. Virol J. (2018) 15:94–6. doi: 10.1186/s12985-018-1007-6
20. Hu Y, Al-Moslih M, Al Ali M, Uzicanin S, Perkins H, Yi Q, et al. Clinical outcome of frequent exposure to torque teno virus (TTV) through blood transfusion in thalassemia patients with or without hepatitis C virus (HCV) infection. J Med Virol. (2008) 80:365–71. doi: 10.1002/jmv.21070
21. Maggi F, Focosi D, Albani M, Lanini L, Vatteroni M, Petrini M, et al. Role of hematopoietic cells in the maintenance of chronic human torquetenovirus plasma viremia. J Virol. (2010) 84:6891–3. doi: 10.1128/JVI.00273-10
22. de Villiers E, Borkosky S, Kimmel R, Gunst K, Fei J. The diversity of Torque teno viruses: In Vitro replication leads to the formation of additional replication-competent subviral molecules. J Virol. (2011) 85:7284–95. doi: 10.1128/JVI.02472-10
23. Bird S, Kirkegaard K. Escape of non-enveloped virus from intact cells. Virology. (2015) 479:444–9. doi: 10.1016/j.virol.2015.03.044
24. Mariscal L, López-Alcorocho J, Rodríguez-Iñigo E, Ortiz-Movilla N, de Lucas S, Bartolomé J, et al. TT virus replicates in stimulated but not in non-stimulated peripheral blood mononuclear cells. Virology. (2002) 301:121–9. doi: 10.1006/viro.2002.1545
25. Schmitz J, Kobbe G, Kondakci M, Schuler E, Magorsch M, Adams O. The value of Torque teno virus (TTV) as a marker for the degree of immunosuppression in adult patients after hematopoietic stem cell transplantation (HSCT). Biol Blood Marrow Transplantat. (2020) 26:643–50. doi: 10.1016/j.bbmt.2019.11.002
26. Albert E, Solano C, Pascual T, Torres I, Macera L, Focosi D, et al. Dynamics of Torque teno virus plasma DNAemia in allogeneic stem cell transplant recipients. J Clin Virol. (2017) 94:22–8. doi: 10.1016/j.jcv.2017.07.001
27. Focosi D, Macera L, Boggi U, Nelli L, Maggi F. Short-term kinetics of torque teno virus viraemia after induction immunosuppression confirm T lymphocytes as the main replication-competent cells. J General Virol. (2015) 96:115–7. doi: 10.1099/vir.0.070094-0
28. Görzer I, Jaksch P, Kundi M, Seitz T, Klepetko W, Puchhammer-Stöckl E. Pre-transplant plasma Torque teno virus load and increase dynamics after lung transplantation. PLoS One. (2015) 10:e0122975. doi: 10.1371/journal.pone.0122975
29. Goto K, Sugiyama K, Ando T, Mizutani F, Terabe K, Tanaka K, et al. Detection rates of TT virus DNA in serum of umbilical cord blood, breast milk and saliva. Tohoku J Exp Med. (2000) 191:203–7. doi: 10.1620/tjem.191.203
30. Chikasue K, Kimura M, Ikeda K, Ohnishi T, Kawanishi S, Iio T, et al. Detection of Torque teno virus DNA in exhaled breath by polymerase chain reaction. Acta Med Okayama. (2012) 66:387–97.
31. Kulifaj D, Durgueil-Lariviere B, Meynier F, Munteanu E, Pichon N, Dubé M, et al. Development of a standardized real time PCR for Torque teno viruses (TTV) viral load detection and quantification: a new tool for immune monitoring. J Clin Virol. (2018) 105:118–27. doi: 10.1016/j.jcv.2018.06.010
32. Focosi D, Spezia P, Macera L, Salvadori S, Navarro D, Lanza M, et al. Assessment of prevalence and load of torquetenovirus viraemia in a large cohort of healthy blood donors. Clin Microbiol Infect. (2020) 26:1406–10. doi: 10.1016/j.cmi.2020.01.011
33. Moghimi M, Shayestehpour M, Doosti M, Ahmadi Vasmehjani A, Seyed Khorrami S, Ahmadi A, et al. Prevalence of torque teno virus in healthy individuals and those infected with hepatitis C virus living in Yazd, Iran. Caspian J Intern Med. (2020) 11:199–204.
34. Sarairah H, Bdour S, Gharaibeh W. The molecular epidemiology and phylogeny of Torque teno virus (TTV) in Jordan. Viruses. (2020) 12:165. doi: 10.3390/v12020165
35. Moustafa A, Xie C, Kirkness E, Biggs W, Wong E, Turpaz Y, et al. The blood DNA virome in 8,000 humans. PLoS Pathog. (2017) 13:e1006292. doi: 10.1371/journal.ppat.1006292
36. Focosi D, Antonelli G, Pistello M, Maggi F. Torquetenovirus: the human virome from bench to bedside. Clin Microbiol Infect. (2016) 22:589–93. doi: 10.1016/j.cmi.2016.04.007
37. Rocchi J, Ricci V, Albani M, Lanini L, Andreoli E, Macera L, et al. Torquetenovirus DNA drives proinflammatory cytokines production and secretion by immune cells via toll-like receptor 9. Virology. (2009) 394:235–42. doi: 10.1016/j.virol.2009.08.036
38. Nelson J, Alvey N, Bowman L, Schulte J, Segovia M, McDermott J, et al. Consensus recommendations for use of maintenance immunosuppression in solid organ transplantation: endorsed by the American college of clinical pharmacy, American society of transplantation, and the international society for heart and lung transplantation. Pharmacotherapy. (2022) 42:599–633. doi: 10.1002/phar.2716
39. van Rijn A, Wunderink H, Sidorov I, de Brouwer C, Kroes A, Putter H, et al. Torque teno virus loads after kidney transplantation predict allograft rejection but not viral infection. J Clin Virol. (2021) 140:104871. doi: 10.1016/j.jcv.2021.104871
40. Jaksch P, Kundi M, Görzer I, Muraközy G, Lambers C, Benazzo A, et al. Torque teno virus as a novel biomarker targeting the efficacy of immunosuppression after lung transplantation. J Infect Dis. (2018) 218:1922–8. doi: 10.1093/infdis/jiy452
41. Nordén R, Magnusson J, Lundin A, Tang K, Nilsson S, Lindh M, et al. Quantification of Torque teno virus and Epstein-Barr virus is of limited value for predicting the net state of immunosuppression after lung transplantation. Open Forum Infect Dis. (2018) 5:ofy050. doi: 10.1093/ofid/ofy050
42. Solis M, Velay A, Gantner P, Bausson J, Filiputti A, Freitag R, et al. Torquetenovirus viremia for early prediction of graft rejection after kidney transplantation. J Infect. (2019) 79:56–60. doi: 10.1016/j.jinf.2019.05.010
43. Fernández-Ruiz M, Albert E, Giménez E, Ruiz-Merlo T, Parra P, López-Medrano F, et al. Monitoring of alphatorquevirus DNA levels for the prediction of immunosuppression-related complications after kidney transplantation. Am J Transpl. (2019) 19:1139–49. doi: 10.1111/ajt.15145
44. Maggi F, Focosi D, Statzu M, Bianco G, Costa C, Macera L, et al. Early post-transplant Torquetenovirus viremia predicts cytomegalovirus reactivations in solid organ transplant recipients. Sci Rep. (2018) 8:15490–8. doi: 10.1038/s41598-018-33909-7
45. Studenic P, Bond G, Kerschbaumer A, Bécède M, Pavelka K, Karateev D, et al. Torque teno virus quantification for monitoring of immunomodulation with biologic compounds in the treatment of rheumatoid arthritis. Rheumatology. (2022) 61:2815–25. doi: 10.1093/rheumatology/keab839
46. Uhl P, Heilos A, Bond G, Meyer E, Böhm M, Puchhammer-Stöckl E, et al. Torque teno viral load reflects immunosuppression in paediatric kidney-transplanted patients—a pilot study. Pediatric Nephrol. (2020) 36:153–62. doi: 10.1007/s00467-020-04606-3
47. Gore E, Gomes-Neto A, Wang L, Bakker S, Niesters H, de Joode A, et al. Torquetenovirus serum load and long-term outcomes in renal transplant recipients. J Clin Med. (2020) 9:440. doi: 10.3390/jcm9020440
48. Maggi F, Andreoli E, Lanini L, Meschi S, Rocchi J, Fornai C, et al. Rapid increase in Total Torquetenovirus (TTV) Plasma Viremia load reveals an apparently transient superinfection by a TTV of a novel group 2 genotype. J Clin Microbiol. (2006) 44:2571–4. doi: 10.1128/JCM.00233-06
49. Masutani K. Viral infections directly involved in kidney allograft function. Nephrology. (2018) 23(Suppl. 2):31–7. doi: 10.1111/nep.13285
50. Jaksch P, Görzer I, Puchhammer-Stöckl E, Bond G. Integrated immunologic monitoring in solid organ transplantation: the road toward Torque teno virus-guided immunosuppression. Transplantation. (2022) 106:1940–51. doi: 10.1097/TP.0000000000004153
51. Crowhurst T, Nolan J, Faull R, Holmes M, Holmes-Liew CL. virus-associated nephropathy in a lung transplant patient: case report and literature review. BMC Infect Dis. (2020) 20:600. doi: 10.1186/s12879-020-05292-0
52. van Beek J, van der Eijk A, Fraaij P, Caliskan K, Cransberg K, Dalinghaus M, et al. Chronic norovirus infection among solid organ recipients in a tertiary care hospital, the Netherlands, 2006-2014. Clin Microbiol Infect. (2017) 23:265.e9–265.e13 doi: 10.1016/j.cmi.2016.12.010
53. Hoek R, Verschuuren E, de Vries R, Vonk J, van Baarle D, van der Heiden M, et al. High torque tenovirus (TTV) load before first vaccine dose is associated with poor serological response to COVID-19 vaccination in lung transplant recipients. J Heart Lung Transpl. (2022) 41:765. doi: 10.1016/j.healun.2022.03.006
54. Gallais F, Renaud-Picard B, Solis M, Laugel E, Soulier E, Caillard S, et al. Torque teno virus DNA load as a predictive marker of antibody response to a three-dose regimen of COVID-19 mRNA-based vaccine in lung transplant recipients. J Heart Lung Transplant. (2022) 41:1429–39. doi: 10.1016/j.healun.2022.07.008
55. Roberto P, Cinti L, Napoli A, Paesani D, Riveros Cabral R, Maggi F, et al. Torque teno virus (TTV): a gentle spy virus of immune status, predictive marker of seroconversion to COVID-19 vaccine in kidney and lung transplant recipients. J Med Virol. (2023) 95:e28512. doi: 10.1002/jmv.28512
56. de Zwart A, Riezebos-Brilman A, Lunter G, Neerken E, van Leer-Buter C, Alffenaar J, et al. Impact of COVID-19 social distancing measures on lung transplant recipients: decline in overall respiratory virus infections is associated with stabilisation of lung function. Eur Respir J. (2022) 60:2200085. doi: 10.1183/13993003.00085-2022
57. Herrmann A, Sandmann L, Adams O, Herrmann D, Dirks M, Widera M, et al. Role of BK polyomavirus (BKV) and Torque teno virus (TTV) in liver transplant recipients with renal impairment. J Med Microbiol. (2018) 67:1496–508. doi: 10.1099/jmm.0.000823
58. Handala L, Descamps V, Morel V, Castelain S, François C, Duverlie G, et al. No correlation between Torque teno virus viral load and BK virus replication after kidney transplantation. J Clin Virol. (2019) 116:4–6. doi: 10.1016/j.jcv.2019.03.018
59. Fernández-Ruiz M, Albert E, Giménez E, Rodríguez-Goncer I, Andrés A, Navarro D, et al. Early kinetics of Torque teno virus DNA load and BK polyomavirus viremia after kidney transplantation. Transplant Infect Dis. (2020) 22:e13240. doi: 10.1111/tid.13240
60. Garbuglia A, Iezzi T, Capobianchi M, Pignoloni P, Pulsoni A, Sourdis J, et al. Detection of TT virus in lymph node biopsies of B-cell lymphoma and Hodgkin’s disease, and its association with EBV infection. Int J Immunopathol Pharmacol. (2003) 16:109–18. doi: 10.1177/039463200301600204
61. Borkosky S, Whitley C, Kopp-Schneider A, Zur HH, de Villiers E. Epstein-Barr virus stimulates Torque teno virus replication: a possible relationship to multiple sclerosis. PLoS One. (2012) 7:e32160. doi: 10.1371/journal.pone.0032160
62. Mallet F, Perret M, Tran T, Meunier B, Guichard A, Tabone O, et al. Early herpes and TTV DNAemia in septic shock patients: a pilot study. Intensive Care Med Exp. (2019) 7:13–28. doi: 10.1186/s40635-019-0256-z
63. Albert E, Solano C, Giménez E, Focosi D, Pérez A, Macera L, et al. The kinetics of torque teno virus plasma DNA load shortly after engraftment predicts the risk of high-level CMV DNAemia in allogeneic hematopoietic stem cell transplant recipients. Bone Marrow Transplant. (2018) 53:180–7. doi: 10.1038/bmt.2017.235
64. Schmidt L, Jensen B, Walker A, Keitel-Anselmino V, di Cristanziano V, Böhm M, et al. Torque teno virus plasma level as novel biomarker of retained immunocompetence in HIV-infected patients. Infection. (2021) 49:501–9. doi: 10.1007/s15010-020-01573-7
65. Lapa D, Del Porto P, Minosse C, D’Offizi G, Antinori A, Capobianchi M, et al. Clinical relevance of Torque teno virus (TTV) in HIV/HCV coinfected and HCV monoinfected patients treated with direct-acting antiviral therapy. J Clin Med. (2021) 10:2092. doi: 10.3390/jcm10102092
66. Pifferi M, Maggi F, Andreoli E, Lanini L, Marco E, Fornai C, et al. Associations between Nasal Torquetenovirus load and spirometric indices in children with asthma. J Infect Dis. (2005) 192:1141–8. doi: 10.1086/444389
67. Maggi F, Andreoli E, Riente L, Meschi S, Rocchi J, Delle Sedie A, et al. Torquetenovirus in patients with arthritis. Rheumatology. (2007) 46:885–6. doi: 10.1093/rheumatology/kem032
68. Seemayer C. Prevalence of TTV DNA and GBV-C RNA in patients with systemic sclerosis, rheumatoid arthritis, and osteoarthritis does not differ from that in healthy blood donors. Ann Rheum Dis. (2001) 60:806–9. doi: 10.1136/ard.60.8.806
69. Martín-López M, Albert E, Fernández-Ruiz M, González-Álvaro I, Rodríguez E, Aguado J, et al. Torque teno virus viremia in patients with chronic arthritis: influence of biologic therapies. Semin Arthritis Rheum. (2019) 50:166–71. doi: 10.1016/j.semarthrit.2019.06.019
70. Schiemann M, Puchhammer-Stöckl E, Eskandary F, Kohlbeck P, Rasoul-Rockenschaub S, Heilos A, et al. Torque teno virus load-inverse association with antibody-mediated rejection after kidney transplantation. Transplantation. (2017) 101:360–7. doi: 10.1097/TP.0000000000001455
71. Strassl R, Doberer K, Rasoul-Rockenschaub S, Herkner H, Görzer I, Kläger J, et al. Torque teno virus for risk stratification of acute biopsyproven alloreactivity in kidney transplant recipients. J Infect Dis. (2019) 219:1934–9. doi: 10.1093/infdis/jiz039
72. Doberer K, Schiemann M, Strassl R, Haupenthal F, Dermuth F, Görzer I, et al. Torque teno virus for risk stratification of graft rejection and infection in kidney transplant recipients—a prospective observational trial. Am J Transpl. (2020) 20:2081–90. doi: 10.1111/ajt.15810
73. Doberer K, Haupenthal F, Nackenhorst M, Bauernfeind F, Dermuth F, Eigenschink M, et al. Torque teno virus load is associated with subclinical alloreactivity in kidney transplant recipients: a prospective observational trial. Transplantation. (2021) 105:2112–8. doi: 10.1097/TP.0000000000003619
74. Strassl R, Schiemann M, Doberer K, Görzer I, Puchhammer-Stöckl E, Eskandary F, et al. Quantification of Torque teno Virus viremia as a prospective biomarker for infectious disease in kidney allograft recipients. J Infect Dis. (2018) 218:1191–9. doi: 10.1093/infdis/jiy306
75. Görzer I, Jaksch P, Strassl R, Klepetko W, Puchhammer-Stöckl E. Association between plasma Torque teno virus level and chronic lung allograft dysfunction after lung transplantation. J. Heart Lung Transpl. (2017) 36:366–8. doi: 10.1016/j.healun.2016.10.011
76. Frye B, Bierbaum S, Falcone V, Köhler T, Gasplmayr M, Hettich I, et al. Kinetics of Torque teno virus-DNA plasma load predict rejection in lung transplant recipients. Transplantation. (2019) 103:815–22. doi: 10.1097/TP.0000000000002436
77. Görzer I, Haloschan M, Jaksch P, Klepetko W, Puchhammer-Stöckl E. Plasma DNA levels of Torque teno virus and immunosuppression after lung transplantation. J Heart Lung Transpl. (2014) 33:320–3. doi: 10.1016/j.healun.2013.12.007
78. Simonetta F, Pradier A, Masouridi-Levrat S, Van Delden C, Giostra E, Morard I, et al. Torque teno virus load and acute rejection after orthotopic liver transplantation. Transplantation. (2017) 101:e219–21. doi: 10.1097/TP.0000000000001723
Keywords: torquetenovirus, transplantation, TTV, immune marker, functional immunity, pitfalls
Citation: Gore EJ, Gard L, Niesters HGM and Van Leer Buter CC (2023) Understanding torquetenovirus (TTV) as an immune marker. Front. Med. 10:1168400. doi: 10.3389/fmed.2023.1168400
Received: 17 February 2023; Accepted: 02 May 2023;
Published: 13 June 2023.
Edited by:
Paulo Henrique Braz-Silva, Faculdade de Odontologia da Universidade de São Paulo, BrazilReviewed by:
Malik Aydin, Witten/Herdecke University, GermanyTania Regina Tozetto-Mendoza, University of São Paulo, Brazil
Copyright © 2023 Gore, Gard, Niesters and Van Leer Buter. This is an open-access article distributed under the terms of the Creative Commons Attribution License (CC BY). The use, distribution or reproduction in other forums is permitted, provided the original author(s) and the copyright owner(s) are credited and that the original publication in this journal is cited, in accordance with accepted academic practice. No use, distribution or reproduction is permitted which does not comply with these terms.
*Correspondence: Coretta C. Van Leer Buter, Yy52YW4ubGVlckB1bWNnLm5s