- 1The State Key Laboratory for Biology of Plant Diseases and Insect Pests, Institute of Plant Protection, Chinese Academy of Agricultural Sciences, Beijing, China
- 2College of Plant Science and Technology, Huazhong Agricultural University, Wuhan, China
Bacterial symbionts associated with insects are often involved in host development and ecological fitness. In aphids, the role of these symbionts is variable and not fully understood across different host species. Here, we investigated the symbiont diversity of the grain aphid, Sitobion miscanthi (Takahashi), from 17 different geographical areas. Of these, two strains with the same symbiont profile, except for the presence of Hamiltonella defensa, were selected using PCR. The Hamiltonella-infected strain, YX, was collected from a Yuxi wheat field in Yunnan Province, China. The Hamiltonella-free strain, DZ, was collected from a Dezhou wheat field in Shandong Province, China. Using artificial infection with H. defensa and antibiotic treatment, a Hamiltonella-re-infected strain (DZ-H) and Hamiltonella-significantly decreased strain (DZ-HT) were established and compared to the Hamiltonella-free DZ strain in terms of ecological fitness. Infection with the DZ-H strain increased the fitness of S. miscanthi, which led to increases in adult weight, percent of wingless individuals, and number of offspring. Meanwhile, decreased abundance of H. defensa (DZ-HT strain) resulted in a lower adult weight and wingless aphid rate compared to the DZ-H strain. However, the indices of longevity in both the DZ-H and DZ-HT strains decreased slightly, but were not significantly different, compared to the DZ strain. Furthermore, quantitative PCR showed that the relative abundance of the primary symbiont Buchnera aphidicola in the DZ-H strain was significantly higher than in the DZ strain in all but the first developmental stage. These results indicate that H. defensa may indirectly improve the fitness of S. miscanthi by stimulating the proliferation of B. aphidicola.
Introduction
Many insects harbor various types of maternally inherited microbial symbionts (Baumann, 2005). A classic model for heritable symbiosis is the association of aphid and its primary (P-) symbiont Buchnera aphidicola, many aphid species first colonized an aphid ancestor 150 million years ago and which persists in almost all of the 5,000-aphid species (Baumann et al., 1995; Clark et al., 2000). B. aphidicola plays a prominent role in insect nutritional ecology by providing essential nutrients that are not obtained in sufficient amounts from a restricted diet of plant phloem (Baumann et al., 1995). Previous studies have shown that in addition to B. aphidicola, a wide range of heritable secondary (S-) symbionts are found in aphids (Buchner, 1965; Oliver et al., 2006; Vorburger and Gouskov, 2011). In contrast to B. aphidicola, which is strictly housed in bacteriocytes, S-symbionts are scattered in sheath cells and aphid hemolymph. They are not thought to be essential to host survival (Douglas, 1989). However, recent studies have revealed the important role of S-symbionts. These symbionts may confer conditional adaptive advantages to their host, such as protecting the insect host against pathogens and natural enemies (Piel, 2002; Oliver et al., 2003, 2005; Guay et al., 2009), ameliorating the detrimental effects of heat (Douglas, 1989, 1998; Ohtaka and Ishikawa, 1991; Montllor et al., 2002), enhancing insecticide resistance, aiding in adaptation to the plant (Leonardo and Muiru, 2003; Tsuchida et al., 2004; Guidolin and Cônsoli, 2017), and mediating insect host metabolism and biosynthesis (Akman and Douglas, 2009; Benoit et al., 2017). With the continuous development of molecular biology and omics technologies, greater knowledge of the evolutionary relationships between symbionts and host insects has been revealed (Degnan et al., 2009, 2010; Manzanomarín and Latorre, 2014; Cassone et al., 2015).
There are many S-symbionts that have been identified in aphids, including Serratia symbiotica, Hamiltonella defensa, Regiella insecticola, Rickettsia, Wolbachia, Spiroplasma, Arsenophonus (Tsuchida et al., 2002; Oliver et al., 2010), and SMLS (Sitobion miscanthi L type symbiont) (Li et al., 2016). H. defensa is a well-studied S-symbiont of insects and a gammaproteobacterium informally known as the pea aphid Bemisia-like symbiont or T-type (Darby et al., 2001), which is widely, but not universally, distributed in natural populations of aphid, especially in the grain aphid, Sitobion miscanthi Takahashi.
Sitobion miscanthi, a dominant grain aphid species in China, is a major wheat pest that frequently harbors S-symbionts. H. defensa has been comprehensively studied in Acyrthosiphon pisum (Nyabuga et al., 2010; Łukasik et al., 2013; Mclean and Godfray, 2015), but most of these studies focused on the role of H. defensa in helping the insect during pathogen or parasite invasion via the presence of a lysogenic lambdoid bacteriophage designated APSE (Moran et al., 2005a; Degnan and Moran, 2008). Hamiltonella-induced tolerance to high temperature has also been reported (Russell and Moran, 2006). Sporadic reports have noted the positive effect of H. defensa on aphid fecundity (Łukasik et al., 2013), but there is still relatively little information available on the ecological adaptation of H. defensa to the aphid host, especially in the wheat aphid S. miscanthi.
In general, artificial infection and antibiotic elimination studies offer a powerful opportunity to study the influence of symbionts on the host. Thus, in the case of presented study system, H. defensa was transferred by microinjection and selectively reduced by antibiotic treatment to provide a host line for the experimental assays. Different geographical populations of aphids naturally harbor various symbionts and provide a source of symbiont diversity for aphid-symbiont interaction research (Tsuchida et al., 2002; Zhao et al., 2016). Aphids with a single strain of S-symbiont are scarce in nature and hence the collection and identification of secondary symbionts in different geographical isofemale strains of S. miscanthi were the foundation for the target symbiont screening.
This study was conducted to assess the diversity of S-symbionts in 17 different geographical populations of S. miscanthi in China, from which the Hamiltonella-infected and Hamiltonella-free strains were obtained. A new Hamiltonella-re-infected strain and a Hamiltonella-significantly decreased strain with the identical genetic backgrounds was generated to evaluate the impact of Hamiltonella on host ecological fitness. Furthermore, the relative abundance of the B. aphidicola in each development stage was measured in Hamiltonella-free and Hamiltonella-re-infected strains. Our study clearly identifies the diversity of S-symbionts in S. miscanthi and reveals the effect of H. defensa on host aphid ecology adaptation and the correlation with B. aphidicola.
Materials and Methods
Aphid Rearing
The strains of S. miscanthi used in this study are shown in Figure 1 and Table 1. These samples were collected from 17 locations covering a wide range of the main wheat-producing areas in mainland China. All of these isofemale strains were established from different collections, feeding separately on aphid-susceptible wheat seedlings (Triticum aestivum L) in the culture room at 20 ± 1°C with a 75% relative humidity and a light: dark photoperiod of 16:8 h. After 10 generations, the aphids were used for the following experiments.
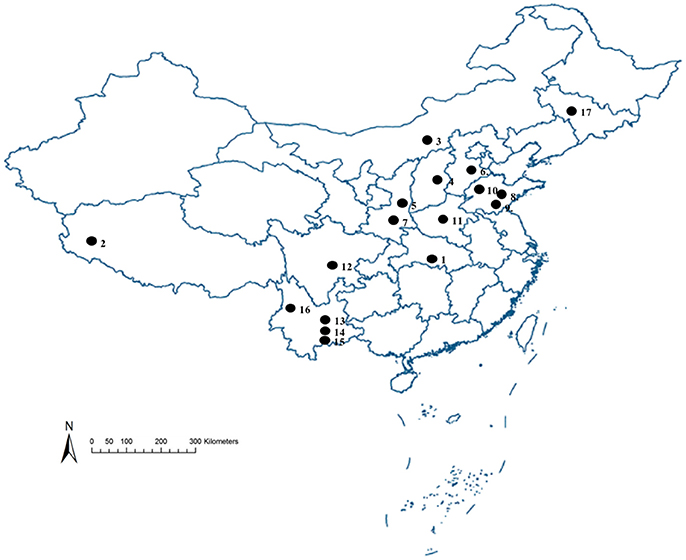
Figure 1. Sampling sites for 17 geographical populations collection to investigate the diversity of secondary symbionts in different geographical S. miscanthi strains in China Numbers on the map correspond to locality numbers in Table 1.
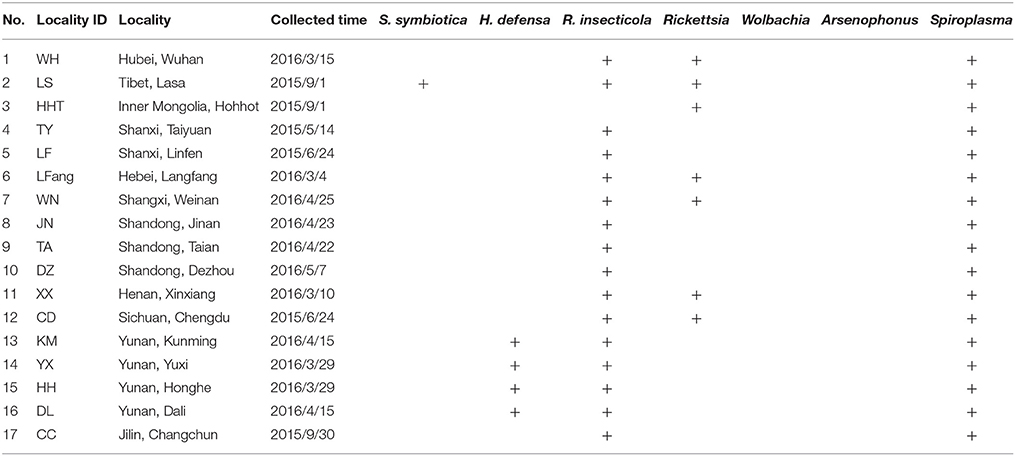
Table 1. Locality information and diversity of secondary symbionts infection in the different geographic isofemale strains of S. miscanthi.
Aphid DNA Extraction, Sequencing, and Secondary Symbiont Identification
Total aphid DNA was extracted from a single adult aphid with the DNeasy Blood & Tissue Kit (Qiagen, Valencia, CA) following the manufacturer's protocol. DNA was extracted from a total of 20 replicates for each geographical strain. DNA quality was evaluated using a NanoDrop-1000 spectrophotometer (Nano-Drop Technologies, Wilmington, DE). These samples were then screened for the seven known S-symbionts of pea aphids, S. symbiotica, H. defensa, R. insecticola, Rickettsia, Wolbachia, Spiroplasma, and Arsenophonus (Tsuchida et al., 2002; Oliver et al., 2010) with PCR using the specific symbiont primers listed in Table 2. Cycling conditions were 94°C for 4 min, followed by 35 cycles at 94°C for 30 s, 60°C for 45 s, 72°C for 1 min, and 4°C for the final elongation. The reaction products were analyzed with a model 3500 ABI PRISM DNA sequencer (Perkin-Elmer, New York, NY). The nucleotide sequence of the H. defensa 16S rRNA partial gene from S. miscanthi described in this paper has been deposited in GenBank under accession number MG721025.
Artificial Infection of H. defensa by Hemolymph Injection
The injection of H. defensa followed a previously described method (Koga et al., 2003). Briefly, 0.5 μl of hemolymph was extracted from the YX strain and diluted with 0.5 μl of 0.01 M PBS. A 1 μl dilution was injected into the body of third-instar nymphs of the DZ strains. We detected the infection of H. defensa in the newborn nymphs from the offspring of the treated aphids 2 weeks after injection. Presence of H. defensa was confirmed again after three generations and before the start of the fitness experiment.
H. defensa Elimination by Antibiotic Treatment
To verify the fitness effect of H. defensa on S. miscanthi, a Hamiltonella-significantly decreased strain was established via antibiotic treatment. With a litter modified by the previous treatment, a mixture of the antibiotics ampicillin and gentamycin (Dykstra et al., 2014), each at 100 μg/ml, was added to a 20% sucrose solution as the artificial diet, with the control treatment containing antibiotic-free sucrose solution, for two days. The aphids that fed on the antibiotic artificial solution are referred to as Hamiltonella-significantly decreased aphids. Following antibiotic treatment, all of the adult aphids were placed on wheat seedlings to produce new nymphs. After rearing for 10 generations, the offspring of aphids were screened for abundance of B. aphidicola and S-symbionts by quantitative PCR. The feeding apparatus was prepared according to Chen et al. (2000) with 250 μl of solution diet sandwiched between two layers of parafilm membrane and stretched to a glass tube of 21-mm diameter under sterile conditions.
Fitness Measurement
The nymphs of DZ (collected from Dezhou wheat filed), DZ-H (a new strain created by DZ strain with H. defensa artificial infection) and DZ-HT (a new strain created by DZ-H strain with antibiotic treatment) were collected over 24 h from adult aphids. Thirty nymphs from strains were selected at random and individually placed in petri dishes containing wheat seedlings whose roots were inserted into water in 1.5 ml tubes and kept at 20°C under a long-day (16 h) light cycle. These nymphs were allowed to develop to adults in the petri dishes, and the fitness indices wingless aphid rate, total number of offspring and longevity were checked daily until all nymphs completed their whole lifecycle. We measured the weight of 30 newly emerged adults collected from each strain and performed 6 replications. To eliminate any adverse effects of the injection or antibiotics on aphids, the DZ-H and DZ-HT strains were reared for 10 generations before performing the fitness experiment.
Quantitative PCR
DNA was extracted from different developmental stages of aphids of the DZ-H and DZ-HT strains within 24 h. To examine whether H. defensa infection in aphids influences the relative abundance of the primary symbiont B. aphidicola, symbiont relative abundance was quantified by the SYBR Green ROX mix (Takara, Dalian, China) and ABI Prism 7,500 Sequence Detection System (Thermo Fisher Scientific, Waltham, MA, USA). B. aphidicola, H. defensa, R. insecticola, and Spiroplasma were quantified in terms of 16S rRNA gene using the primers in the Table 2. The S. miscanthi actin gene with primers ß-actin was used as an internal standard for data normalization. Three replicates were performed. The amplification efficiency amplified with primers were 103.4, 99.1, 95.5, 97.2, and 101.9% for B. aphidicola, H. defensa, R. insecticola, and Spiroplasma and actin, respectively.
Quantitative PCR (QPCR) was carried out in 20-μl volumes containing 10 μl of SYBR Green PCR Mix (Takara Bio, Shiga, Japan), 0.4 μl of 50x ROX Dye II, 1 μl of each primer, and 1 μl of DNA. Cycling conditions were 95°C for 15 min, then 40 cycles of 15 s at 95°C, 30 s at the annealing temperature at 60°C, and 30 s at 72°C. The relative abundance of aphid B. aphidicola was normalized to the aphid housekeeping gene ß-actin and calculated using the comparative Ct method 2−ΔΔctmethod (Vandesompele et al., 2002).
Statistical Analyses
The treatment effects were analyzed by nonparametric tests. Kruskal-Wallis was used for multiple comparisons and Mann-Whitney was used for two sample comparisons at α = 0.05. All of the data analyses were performed using the IBM SPSS statistics Version 21 (ver. 21, SPSS Inc., Chicago, IL, USA) software.
Results
Diversity of Secondary Symbiont Infection in Different Geographical Isofemale Strains of S. miscanthi
The diversity of S-symbiont infections of S. miscanthi was investigated from 17 isofemale strains by specific PCR detection. S. symbiotica, H. defensa, R. insecticola, Rickettsia, Arsenophonus, and Spiroplasma exhibited partial infections in different geographical strains. However, Wolbachia was not detected from any of the Chinese strains of S. miscanthi (Table 1). When an S-symbiont was detected in a population, infection rate was close to 100%. In these isofemale strains, no single S-symbiont infected strain was detected.
H. defensa Artificial Reinfection and Antibiotic Elimination
Because no H. defensa single infected strains were found in different isofemale strains and given the negative impact of using multiple antibiotics to remove different symbionts, we performed the following experiments using the two strains with the same symbionts background, except H. defensa. Twenty third-instar nymphs of the Hamiltonella-free DZ strain of S. miscanthi were injected with hemolymph obtained from the YX strain of S. miscanthi, and the Hamiltonella-re-infected strain DZ-H with the identical background of DZ strain was stably established. To evaluate whether H. defensa had been transferred into the aphids, DNA samples obtained from sets of 10 first-instar nymphs were subjected to PCR detection based on the H. defensa 16S rRNA gene with two replications (data not shown). The sequence of H. defensa in our study, 1380-bp in length, exhibited 99.8% similarity to the sequence of H. defensa from A. pisum. After antibiotic treatment, DZ-HT strains were reared for 10 generations and, then, we estimated the relative abundance of symbionts in DZ-HT strains to ensure that the antibiotic did not affect the other symbionts, except H. defensa. Compared to the DZ-H strain, the abundance of H. defensa diminished by approximately 4.15-fold (P < 0.05; Figure 2A), while B. aphidicola, R. insecticola, and Spiroplasma showed almost no change (Figures 2B–D). These results showed that a moderate concentration of mixed antibiotics could specifically decrease targeted symbionts without affecting the other symbionts.
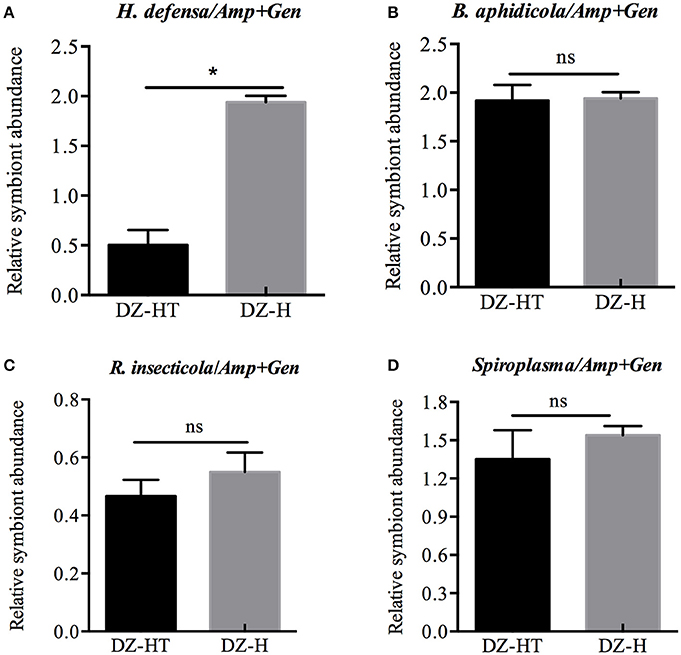
Figure 2. Relative symbiont abundance change with antibiotic treatment designed to specifically eliminate H. defensa QPCR analysis of DNA extracted from antibiotic-treated aphids and controls. (A) The abundance of H. defensa significantly declined in DZ-HT strains treated with antibiotics compared to the control DZ-H strains (P < 0.05). Antibiotic treatment had no effect on the abundance of B. aphidicola, R. insecticola, and Spiroplasma (B–D). The asterisk indicates significant differences based on the Mann-Whitney U-test for two sample comparison at P < 0.05 and ns indicates no significant difference.
Role of H. defensa on Host Aphid Fitness
Aphid demographic parameters, including adult aphid weight, percentage of wingless aphids, total number of offspring, and longevity were compared among the DZ-H (Hamiltonella-re-infected), DZ (Hamiltonella-free), and DZ-HT strains (Hamiltonella-decreased). The DZ-H strain had a significant positive effect on partial fitness indices compared to the DZ and DZ-HT strains (Figure 3). The total number of offspring per five adults of the DZ-H strain was 112.3, which was significantly higher than the DZ strain (89.3) (t = 3.326, P < 0.05), but it was not significantly different from the DZ-HT strain (Figure 3A). For the DZ-H strain, the adult aphid weight was 1.93 g, which was significantly higher than the DZ (1.68 g) (t = 5.677, P < 0.05) and DZ-HT strains (1.808 g) (t = 2.814, P < 0.05) (Figure 3B). The wingless aphid rate in the DZ-H strain was 67%, which was significantly higher than in the DZ (42%) (t = 3.727, P < 0.05) and DZ-HT strains (46%) (t = 3.316, P < 0.05) (Figure 3C). However, the longevity of the DZ-H strain was 22.9 days, which was not significantly different from the DZ (26.4 days) (t = 1.705, P = 0.09) and DZ-HT strains (22.4 d) (t = 0.278, P = 0.79) (Figure 3D).
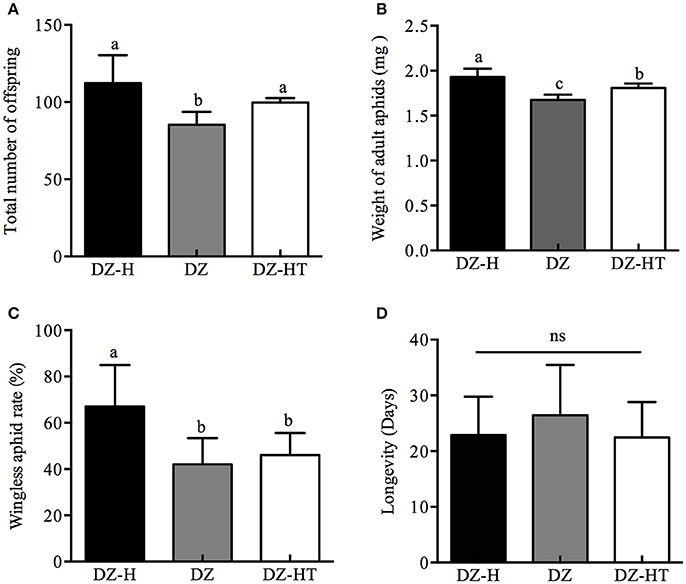
Figure 3. Fitness indices of Hamiltonella-infected, Hamiltonella-free, and Hamiltonella-decreased S. miscanthi strains. (A) Total number of offspring. (B) Weight of adult aphids. (C) Percentage of wingless aphids. (D) Longevity. Bar represents standard errors of means and different letters above the bars indicate significant differences based on Kruskal-Wallis for multiple comparisons at P < 0.05, while ns indicates no significant difference.
Relative Abundance Difference in B. aphidicola Between DZ-H and DZ Strains
The relative abundance of B. aphidicola was measured by quantitative PCR. The abundance of B. aphidicola in the DZ-H strains was significantly higher than in the DZ strain during the entire development stage, except for the first instar nymphs. The comparison of the abundance ratios of B. aphidicola between DZ-H and DZ in different developmental stages of S. miscanthi are listed as follows: 1.139-fold (t = 0.519, P = 0.631), 3.415-fold (t = 10.645, P < 0.05), 4.712-fold (t = 31.191, P < 0.05), 6.381-fold (t = 50.261, P < 0.05) for the first-fourth instar nymph stage, respectively (Figure 4A), and 3.281-fold (t = 19.345, P < 0.05) and 9.671-fold (t = 21.363, P < 0.05) in winged and wingless adult aphids, respectively (Figure 4B). These results further confirmed that the relative abundance of B. aphidicola was affected by H. defensa infection.
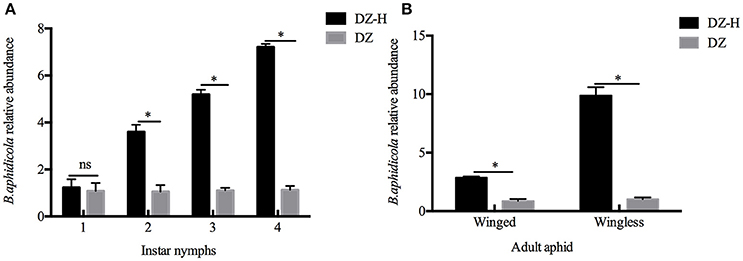
Figure 4. Relative abundance of B. aphidicola in the DZ-H and DZ strains. (A) Relative abundance of B. aphidicola in different instar nymphs. (B) Relative abundance of B. aphidicola in winged and wingless adult aphids. The asterisk indicates significant differences based on the Mann-Whitney U-test for two sample comparison at P < 0.05 and ns indicates no significant difference.
Discussion
Research on the role of S-symbionts in the aphid S. miscanthi, one of the most important pests of gramineous crops in China, is still incomplete. In order to obtain target S-symbionts and understand their functions, stable geographical populations with different symbiotic infections are needed. We screened the diversity of S-symbiont infections from established laboratory populations of 17 geographical clones. A remarkably variable composition of S-symbiont infection was evident in S. miscanthi. Interestingly, the infection of S-symbionts among the different wheat-producing provinces differed somewhat, but samples from different places in the same province showed a high degree of consistency. It is noteworthy that H. defensa has only been detected in Yunnan province with an average elevation of 2,000 m, and S. symbiotic has only been detected in the Tibet autonomous region with an average elevation of 4,000 m. Interestingly, both areas belong to the plateau region of southwest China that features complex geological and topographical conditions. Our geographic sampling data suggest elevation and topography may contribute to the symbiotic diversity. As the mechanism of symbiont distribution in S. miscanthi are unknown, however, biotic and/or abiotic factors could produce the regional differentiation, as proposed by Oliver et al. (2014) and Tsuchida et al. (2002). Indeed, other factors that are not mediated by natural selection such as genetic drift, founder effects (Russell et al., 2013) and host plant (Guidolin and Cônsoli, 2017) can also affect the diversity of S-symbionts between geographical populations of S. miscanthi in different locations in China. Although we did not investigate the infection rate of symbionts in natural populations, our results, combined with information reported in the literature (Sepúlveda et al., 2016; Zytynska and Weisser, 2016), show that multiple S-symbionts coexist within local populations of S. miscanthi in different geographical strains.
Hamiltonella defensa is a well-known S-symbiont that confers protection to the aphid against parasitoid wasps (Oliver et al., 2005; Degnan and Moran, 2008; Łukasik et al., 2013; Vorburger and Rouchet, 2016), with a high infection rate of 31.3% (5/16) in US strains (Sandström et al., 2001) and 39.5% (15/38) in UK strains of A. pisum (Darby et al., 2001). However, it was not detected (0/119) in Japanese strains of A. pisum (Tsuchida et al., 2002; Rothacher et al., 2016). In our study, H. defensa was also not widely distributed in S. miscanthi from the screened area. It was only present in four clones collected in Yunnan Province. Even though we did not obtain a single Hamiltonella-infected S. miscanthi strain, two interesting strains (YX and DZ) were collected from Yuxi and Dezhou wheat fields in China. Both strains had the same S-symbiont infection profiles, except for the presence of H. defensa (YX: Hamiltonella-infected and DZ: Hamiltonella-free). By artificial infection, Hamiltonella-free strain (DZ) and Hamiltonella-re-infected strain (DZ-H) with the same genetic background were generated. After antibiotic treatment, a Hamiltonella-decreased strain (DZ-HT) was also obtained.
Fitness measurements revealed that the Hamiltonella-re-infected treatment increased the fitness of S. miscanthi, as evidenced by greater numbers of offspring, increased weight, and higher wingless aphid rate. Meanwhile, decreasing the abundance of H. defensa with antibiotics resulted in lowered adult aphid weight and percentage of wingless aphids. Similar results have been reported for the whitefly (Su et al., 2013), while Oliver et al. (2008) reported that H. defensa shortened the mean generation time of A. pisum. However, in S. miscanthi, research on the influence of H. defensa in improving aphid fecundity is sporadic (Łukasik et al., 2013) and other S-symbiont infections were not identified. In our research, we used four fitness indices (number of offspring, weight of adult aphids, percentage of wingless aphids, and longevity) to evaluate the effect of H. defensa on the ecological fitness of S. miscanthi strains. Surprisingly, the difference in longevity found between DZ and DZ-H strains were not significant, but was only slightly decreased. A close relationship with symbionts can be costly for the host (Polin et al., 2014), with these direct costs caused by a trade-off between allocating resources to symbiosis and other adaptive functions. A previous study found that aphids exhibited many behavioral defenses against enemies and these behaviors had some associated costs, leading to a reduction in aphid reproduction (Dion et al., 2011). We hypothesize that the benefit of H. defensa infection in terms of number of offspring, weight, and wingless aphid rate represents a trade-off against the cost of longevity, with an overall benefit of H. defensa infection.
Buchnera aphidicola, a primary symbiont in almost all aphids, appears to benefit their hosts primarily through the provision of nutrients that are limiting for growth and reproduction (Clark et al., 2000; Oliver et al., 2010). In this study, the relative abundance of B. aphidicola in the DZ-H strain was significantly higher than the DZ strain in all but the first developmental stage. This phenomenon implies a linkage of B. aphidicola with H. defensa, and the difference abundance of B. aphidicola in aphid offspring might not be caused by microinjection. The increased in B. aphidicola could result from increased nutritional requirements of the whole system. B. aphidicola may be “feeding” H. defensa since the latter does not seem to be able to manufacture many essential amino acids (Degnan et al., 2009). Therefore, we speculate that infection with H. defensa may indirectly improve the fitness of S. miscanthi by stimulating the abundance of B. aphidicola. In addition, based on the results of the current study and combined with the viewpoint that H. defensa inhabits secondary bacteriocytes intercalated between primary bacteriocytes that harbor B. aphidicola (Moran et al., 2005b), it can be hypothesized that B. aphidicola and H. defensa may perform complementary functions in the aphid host and facilitate biological interactions between them. More interestingly, several reports using high-throughput sequencing methods have suggested that S. symbiotica and Wolbachia, which are facultative symbionts in many aphid species, have evolved to form a deep and co-obligate association with B. aphidicola in different aphids (Manzanomarín and Latorre, 2014; De et al., 2015). Although H. defensa is not necessary for S. miscanthi, it affects the host aphid ecological adaptation to some extent. Further studies employing metagenomics and proteomics techniques will be needed to reveal the underlying mechanism of how H. defensa improves the fitness of S. miscanthi, as well as to examine the interactions between H. defensa and B. aphidicola and the interactions among the symbionts themselves.
In summary, we established a feasible method to obtain aphid strains with and without a target symbiont under the same other S-symbiont infection profiles and genetic background by screening different geographical populations and microinjection. In addition, infection with H. defensa increased the fitness of S. miscanthi and enhanced the relative abundance of B. aphidicola. Our results establish a basis for functional studies by revealing the effect of H. defensa on host aphid ecology adaptation and the correlation with B. aphidicola. A better understanding of the insect and endosymbionts interaction will supply information on the potential value of manipulating microbiota to control insect pests. The diversity and ecological function of symbionts may provide important guidance to field arrangements of wheat varieties in different wheat production areas.
Author Contributions
LQ, FJ, CJ, and WM conceived and designed the experiments. LQ and SJ performed the experiments. LQ analyzed the data. LQ and CJ wrote the paper. All of the authors read and approved the final version of the manuscript.
Funding
This study was financially supported by the National Key R & D Plan of China (2016YFD0300700, 2017YFD0201700) and the Opening Fund of the State Key Laboratory for the Biology of Plant Diseases and Insect Pests (SKLOF201601).
Conflict of Interest Statement
The authors declare that the research was conducted in the absence of any commercial or financial relationships that could be construed as a potential conflict of interest.
Acknowledgments
We would like to thank Dr. Aid Ali from the University of Agriculture in Faisalabad, Pakistan for providing comments on an earlier version of the manuscript.
References
Akman, G. E., and Douglas, A. E. (2009). Symbiotic bacterium enable insect to use a nutritionally inadequate diet. Proc. Biol. Sci. 276, 987–991. doi: 10.1098/rspb.2008.1476kfo
Baumann, P. (2005). Biology bacteriocyte-associated endosymbionts of plant sap-sucking insects. Annu. Rev. Microbiol. 59, 155–189. doi: 10.1146/annurev.micro.59.030804.121041
Baumann, P., Baumann, L., Lai, C. Y., Rouhbakhsh, D., Moran, N. A., and Clark, M. A. (1995). Genetics physiology and evolutionary relationships of the genus Buchnera: intracellular symbionts of aphids. Annu. Rev. Microbiol. 49, 55–94. doi: 10.1146/annurev.mi.49.100195.000415
Benoit, J. B., Vigneron, A., Broderick, N. A., Wu, Y. N., Sun, J. S., Carlson, J. R., et al. (2017). Symbiont-induced odorant binding proteins mediate insect host hematopoiesis. Elife 6, 1–24. doi: 10.7554/eLife.19535
Cassone, B. J., Wenger, J. A., and Michel, A. P. (2015). Whole genome sequence of the soybean aphid endosymbiont Buchnera aphidicola and genetic differentiation among biotype-specific strains. J. Genom. 3, 85–94. doi: 10.7150/jgen.12975
Chen, J. L., Ni, H. X., Ding, J. H., and Sun, J. R. (2000). Studies on a chemically defined diet of English grain aphid. Sci. Agric. Sin. 33, 54–59.
Clark, M. A., Moran, N. A., Baumann, P., and Wernegreen, J. J. (2000). Cospeciation between bacterial endosymbionts (Buchnera) and a recent radiation of aphids (Uroleucon) and pitfalls of testing for phylogenetic congruence. Evolution 2, 517–525. doi: 10.1111/j.0014-3820.2000.tb00054.x
Darby, A. C., Birkle, L. M., Turner, S. L., and Douglas, A. E. (2001). An aphid-borne bacterium allied to the secondary symbionts of whitefly. FEMS Microb. Ecol. 36, 43–50. doi: 10.1111/j.1574-6941.2001.tb00824.x
De, C. C., Fujiwara, A., Joncour, P., Simon, L., Felix, M. L., Frederic, F., et al. (2015). A metagenomic approach from aphid's hemolymph sheds light on the potential roles of co-existing endosymbionts. Microbiome 3, 63–74. doi: 10.1186/s40168-015-0130-5
Degnan, P. H., Leonardo, T. E., Cass, B. N., Hurwitz, B., Stern, D., Gibbs, R. A., et al. (2010). Dynamics of genome evolution in facultative symbionts of aphids. Environ. Microbiol. 12, 2060–2069. doi: 10.1111/j.1462-2920.2009.02085.x
Degnan, P. H., and Moran, N. A. (2008). Diverse phage-encoded toxins in a protective insect endosymbiont. Appl. Environ. Microbiol. 74, 6782–6791. doi: 10.1128/AEM.01285-08
Degnan, P. H., Yu, Y., Sisneros, N., Wing, R. A., and Moran, N. A. (2009). Hamiltonella defensa, genome evolution of protective bacterial endosymbiont from pathogenic ancestors. Proc. Natl. Acad. Sci. U.S.A. 106, 9063–9068. doi: 10.1073/pnas.0900194106
Dion, E., Polin, S. E., Simon, J. C., and Outreman, Y. (2011). Symbiont infection affects aphid defensive behaviours. Biol. Lett. 7, 743–746. doi: 10.1098/rsbl.2011.0249
Douglas, A. E. (1989). Mycetocyte symbiosis in insects. Biol. Rev. Camb. Philos. Soc. 64, 409–434. doi: 10.1111/j.1469-185X.1989.tb00682.x
Douglas, A. E. (1998). Nutritional interactions in insect-microbial symbioses: aphids and their symbiotic bacteria Buchnera. Annu. Rev. Entomol. 43, 17–37. doi: 10.1146/annurev.ento.43.1.17
Dykstra, H. R., Weldon, S. R., Martinez, A. J., White, J. A., Hopper, K. R., Heimpel, G. E., et al. (2014). Factors limiting the spread of the protective symbiont Hamiltonella defensa in Aphis craccivora aphids. Appl. Environ. Microbiol. 80, 5818–5827. doi: 10.1128/AEM.01775-14
Fukatsu, T., and Nikoh, N. (1998). Two intracellular symbiotic bacteria from the mulberry psyllid anomoneura mori (insecta, homoptera). Appl. Environ. Microbiol. 64, 3599–3606.
Fukatsu, T., Nikoh, N., Kawai, R., and Koga, R. (2000). The secondary endosymbiotic bacterium of the pea aphid Acyrthosiphon pisum (Insecta, Homoptera). Appl. Environ. Microbiol. 66, 2748–2758. doi: 10.1128/AEM.66.7.2748-2758.2000
Guay, J. F., Boudreault, S., Michaud, D., and Cloutier, C. (2009). Impact of environmental stress on aphid clonal resistance to parasitoids: role of Hamiltonella defensa bacterial symbiosis in association with a new facultative symbiont of the pea aphid. J. Insect Physiol. 10, 919–926. doi: 10.1016/j.jinsphys.2009.06.006
Guidolin, A. S., and Cônsoli, F. L. (2017). Symbiont diversity of aphis (Toxoptera) citricidus, (Hemiptera: Aphididae) as influenced by host plants. Micro. Ecol. 73, 201–210. doi: 10.1007/s00248-016-0892-8
Koga, R., Tsuchida, T., and Fukatsu, T. (2003). Changing partners in an obligate symbiosis: a facultative endosymbiont can compensate for loss of the essential endosymbiont buchnera in an aphid. Proc. Biol. Sci. 270, 2543–2550. doi: 10.1098/rspb.2003.2537
Leonardo, T. E., and Muiru, G. T. (2003). Facultative symbionts are associated with host plant specialization in pea aphid populations. Proc. R. Soc. London. Sci. Ser. B 270, S209–S212. doi: 10.1098/rsbl.2003.0064
Li, T., Wu, X. J., Jiang, Y. L., Zhang, L., Duan, Y., Miao, J., et al. (2016). The genetic diversity of SMLS (Sitobion miscanthi Ltype symbiont), and its effect on the fitness, mitochondrial DNA diversity and Buchnera aphidicola dynamic of wheat aphid, Sitobion miscanthi (Hemiptera: Aphididae). Mol. Ecol. 25, 3142–3151. doi: 10.1111/mec.13669
Łukasik, P., Dawid, M. A., Ferrari, J., and Godfray, H.C. (2013). The diversity and fitness effects of infection with facultative endosymbionts in the grain aphid, Sitobion avenae. Oecologia 173, 985–996. doi: 10.1007/s00442-013-2660-5
Montllor, C. B., Maxmen, A., and Purcell, A. H. (2002). Facultative bacterial endosymbionts benefit pea aphids Acyrthosiphon pisum under heat stress. Ecol. Entomol. 27, 189–195. doi: 10.1046/j.1365-2311.2002.00393.x
Manzanomarín, A., and Latorre, A. (2014). Settling down: the genome of Serratia symbiotica from the aphid Cinara tujafilina zooms in on the process of accommodation to a cooperative intracellular life. Genome Biol. Evol. 6, 1683–1698. doi: 10.1093/gbe/evu133
Mclean, A. H., and Godfray, H. C. (2015). Evidence for specificity in symbiont-conferred protection against parasitoids. Proc. R. Soc. London. Sci. Ser. B 282, 1811–1819. doi: 10.1098/rspb.2015.0977
Moran, N. A., Degnan, P. H., Santos, S. R., Dunbar, H. E., and Ochman, H. (2005a). The players in a mutualistic symbiosis: insects, bacteria, viruses, and virulence genes. Proc. Natl. Acad. Sci. U.S.A.102, 16919–16926. doi: 10.1073/pnas.0507029102
Moran, N. A., Russell, J. A., Koga, R., and Fukatsu, T. (2005b). Evolutionary relationships of three new species of entero bacteriaceae living as symbionts of aphids and other insects. Appl. Environ. Microbiol. 71, 3302–3310. doi: 10.1128/AEM.71.6.3302-3310.2005
Nyabuga, F. N., Outreman, Y., Simon, J. C., Heckel, D. G., and Weisser, W. W. (2010). Effects of pea aphid secondary endosymbionts on aphid resistance and development of the aphid parasitoid aphidius ervi: a correlative study. Entomol. Experi. Et. Appl. 136, 243–253. doi: 10.1111/j.1570-7458.2010.01021.x
Ohtaka, C., and Ishikawa, H. (1991). Effects of heat treatment on the symbiotic system of an aphid mycetocyte. Symbiosis 11, 19–30.
Oliver, K. M., Campos, J., Moran, N. A., and Hunter, M. S. (2008). Population dynamics of defensive symbionts in aphids. Proc. R. Soc. Lond. B 275, 293–299. doi: 10.1098/rspb.2007.1192
Oliver, K. M., Degnan, P. H., Burke, G. R., and Moran, N. A. (2010). Facultative symbionts in aphids and the horizontal transfer of ecologically important traits. Annu. Rev. Entomol. 55, 247–266. doi: 10.1146/annurev-ento-112408-085305
Oliver, K. M., Moran, N. A., and Hunter, M. S. (2005). Variation in resistance to parasitism in aphids is due to symbionts not host genotype. Proc. Natl. Acad. Sci. U.S.A. 102, 12795–12800. doi: 10.1073/pnas.0506131102
Oliver, K., Moran, N., and Hunter, M. (2006). Costs and benefits of a superinfection of facultative symbionts in aphids. Pro. R. Soc. B Biol. Sci. 1591, 1273–1280. doi: 10.1098/rspb.2005.3436
Oliver, K. M., Russell, J. A., Moran, N. A., and Hunter, M. S. (2003). Facultative bacterial symbionts in aphids confer resistance to parasitic wasp. Proc. Natl. Acad. Sci. U.S.A. 4, 1803–1807. doi: 10.1073/pnas.0335320100
Oliver, K. M., Smith, A. H., and Russell, J. A. (2014). Defensive symbiosis in the real world—advancing ecological studies of heritable, protective bacteria in aphids and beyond. Func. Ecol. 28, 341–355. doi: 10.1111/1365-2435.12133
Piel, J. (2002). A polyketide synthase-peptide synthetase gene cluster from an uncultured bacterial symbiont of Paederus beetles. Proc. Natl. Acad. Sci. U.S.A. 99, 14002–14007. doi: 10.1073/pnas.222481399
Polin, S., Simon, J. C., and Outreman, Y. (2014). An ecological cost associated with protective symbionts of aphids. Ecol. Evol. 4, 836–840. doi: 10.1002/ece3.991
Rothacher, L., Ferrer Suay, M., and Vorburger, C. (2016). Bacterial endosymbionts protect aphids in the field and alter parasitoid community composition. Ecology 97, 1712–1723. doi: 10.1890/15-2022.1
Russell, J. A., and Moran, N. A. (2006). Costs and benefits of symbiont infection in aphids: variation among symbionts and across temperatures. Proc. R. Soc. Lond. B 273, 603–610. doi: 10.1098/rspb.2005.3348
Russell, J. A., Weldon, S., Smith, A. H., Kim, K. L., Hu, Y., Lukasik, P., et al. (2013). Uncovering symbiont-driven genetic diversity across North American pea aphids. Mol. Ecol. 22, 2045–2059. doi: 10.1111/mec.12211
Sandström, J. P., Russell, J. A., White, J. P., and Moran, N. A. (2001). Independent origins and horizontal transfer of bacterial symbionts of aphids. Mol. Ecol. 10, 217–228. doi: 10.1046/j.1365-294X.2001.01189.x
Sepúlveda, D. A., Zepedapaulo, F., Ramírez, C. C., Lavandero, B., and Figreroa, C. C. (2016). Diversity, frequency and geographic distribution of facultative bacterial endosymbionts in introduced aphid pests. Insect Sci. 3, 511–521. doi: 10.1111/1744-7917.12313
Su, Q., Oliver, K. M., Pan, H., Jiao, X. G., Liu, B. M., Xie, W., et al. (2013). Facultative symbiont hamiltonella confers benefits to Bemisia tabaci (Hemiptera: Aleyrodidae), an invasive agricultural pest worldwide. Environ. Entomol. 42, 1265–1271. doi: 10.1603/EN13182
Tsuchida, T., Koga, R., and Fukatsu, T. (2004). Host plant specialization governed by facultative symbiont. Science 303, 1989–1989. doi: 10.1126/science.1094611
Tsuchida, T., Koga, R., Shibao, H., Matsumto, T., and Fukatsu, T. (2002). Diversity and geographic distribution of secondary endosymbiotic bacteria in natural populations of the pea aphid, Acyrthosiphon pisum. Mol. Ecol. 11, 2123–2135. doi: 10.1046/j.1365-294X.2002.01606.x
Vandesompele, J., Katleen, D. P., Bruce, P., Nadine, V. R., Anne, D. P., and Rank, S. (2002). Accurate normalization of real-time quantitative RT-PCR data by geometric averaging of multiple internal control genes. Genome. Biol. 7:RESEARCH0034. doi: 10.1186/gb-2002-3-7-research0034
Vorburger, C., and Gouskov, A. (2011). Only helpful when required: a longevity cost of harbouring defensive symbionts. J. Evol. Biol. 24, 1611–1617. doi: 10.1111/j.1420-9101.2011.02292.x
Vorburger, C., and Rouchet, R. (2016). Are aphid parasitoids locally adapted to the prevalence of defensive symbionts in their hosts? BMC Evol. Biol. 16:271. doi: 10.1186/s12862-016-0811-0
Zhao, Y., Zhang, S., Luo, J. Y., Wang, C. Y., Lv, L. M., and Cui, J. J. (2016). Bacterial communities of the cotton aphid Aphis gossypii associated with Bt cotton in northern China. Sci. Rep. 6, 22958–22966. doi: 10.1038/srep22958
Keywords: Sitobion miscanthi, symbionts, Hamiltonella defensa, insect fitness, Buchnera aphidicola, relative abundance
Citation: Qian L, Jia F, Jingxuan S, Manqun W and Julian C (2018) Effect of the Secondary Symbiont Hamiltonella defensa on Fitness and Relative Abundance of Buchnera aphidicola of Wheat Aphid, Sitobion miscanthi. Front. Microbiol. 9:582. doi: 10.3389/fmicb.2018.00582
Received: 17 October 2017; Accepted: 14 March 2018;
Published: 29 March 2018.
Edited by:
Suhelen Egan, University of New South Wales, AustraliaReviewed by:
Gordon M. Bennett, University of California, Merced, United StatesRosario Gil, Universitat de València, Spain
Copyright © 2018 Qian, Jia, Jingxuan, Manqun and Julian. This is an open-access article distributed under the terms of the Creative Commons Attribution License (CC BY). The use, distribution or reproduction in other forums is permitted, provided the original author(s) and the copyright owner are credited and that the original publication in this journal is cited, in accordance with accepted academic practice. No use, distribution or reproduction is permitted which does not comply with these terms.
*Correspondence: Chen Julian, chenjulian@caas.cn