- 1Key Laboratory of Environment Correlative Dietology (Ministry of Education), College of Food Science and Technology, Huazhong Agricultural University, Wuhan, China
- 2State Key Laboratory of Agricultural Microbiology, Huazhong Agricultural University, Wuhan, China
- 3Department of Food Science and Human Nutrition, Iowa State University, Ames, IA, United States
- 4Hubei Collaborative Innovation Center for Green Transformation of Bio-Resources, College of Life Sciences, Hubei University, Wuhan, China
Control of biogenic amines (BAs) is critical to guarantee the safety of fermented meat products. The aim of this study is to evaluate the BAs formation and degradation abilities of lactic acid bacteria from Chinese bacon to obtain the beneficial candidate for BAs control. Seven lactic acid bacteria were selected from the typical Chinese bacon products, identified as Lactobacillus curvatus by 16S rDNA analysis. Then, genes analysis and high-performance liquid chromatography (HPLC) analysis were performed to evaluate the BAs formation and degradation abilities of as-selected strains. All L. curvatus strains were confirmed to harbor the genes encoding the tyrosine decarboxylase and ornithine decarboxylase, and they could produce tyramine, β-phenethylamine, putrescine, and cadaverine. In comparison, the lowest concentration of total BAs was obtained in L. curvatus G-1. Meanwhile, all L. curvatus strains were positive in amines oxidase gene analysis, and they could also degrade six common BAs, especially the L. curvatus G-1 with the highest degradation percentage (above 40%) for each BA. Furthermore, fermented meat model analysis verified that the L. curvatus G-1 could significantly reduce BAs. In conclusion, L. curvatus G-1 shows a low BAs-producing ability, as well as a high BAs-degrading ability, and this study provides a promising candidate for potential BAs control in fermented meat products.
Introduction
Biogenic amines (BAs), a group of low-molecular-weight nitrogenous compounds, are mainly produced through amino acid decarboxylation, which exists in most fermented foods such as cheese, yoghurt, sausage, wine, and beer (Landete et al., 2005; Lázaro et al., 2015; Guarcello et al., 2016). The BAs reported in fermented foods include histamine, tyramine, tryptamine, putrescine, cadaverine, and phenylethylamine, which are generated by decarboxylation of histidine, tyrosine, tryptophan, ornithine, lysine, and phenylalanine, respectively (Landete et al., 2007b; Romano et al., 2013; Diaz et al., 2015; Gardini et al., 2016). Moreover, putrescine can also be formed through deimination of agmatine (Coton et al., 2010). Low concentrations of BAs are essential for normal metabolic functions in animals, plants, and microorganisms. However, the excessive oral intake of BAs can lead to nausea, headache, rashes, and blood pressure changes (Ladero et al., 2010). Therefore, control of BAs within the safety level is critical for the safety of fermented foods.
Biogenic amines have been broadly reported as potentially toxic substances in fermented meat products (Lu et al., 2010; Zhang et al., 2013; Nie et al., 2014; Wang et al., 2015a,b). Lactic acid bacteria are dominant in fermented meat products and involved in the formation of color, texture, and flavor (De Martinis and Freitas, 2003; Cornu et al., 2011). However, there is also a safe risk from some lactic acid bacteria due to their excessive BAs accumulation abilities (Suzzi and Gardini, 2003). Microbial decarboxylases are responsible for forming BAs (Nie et al., 2014). As the starter cultures, lactic acid bacteria can hydrolyze meat proteins to release free amino acids (Silvina et al., 2010), and their decarboxylases can further catalyze amino acids to BAs (Suzzi and Gardini, 2003). On the other hand, some lactic acid bacteria can synthesize amine oxidases to degrade BAs (Dapkevicius et al., 2000; García-Ruiz et al., 2011). Different strains usually have different BAs formation and degradation abilities. Previous reports focused on screening of the BAs-degrading strains to reduce the BAs contents (Latorre-Moratalla et al., 2010; García-Ruiz et al., 2011; Cueva et al., 2012; Zaman et al., 2014), while only few reports aimed to select the strain with high BAs-degrading ability as well as low BAs-producing activity (Herrerofresno et al., 2012; Zhang et al., 2013), which will be more beneficial to control the BAs.
Chinese bacon products, such as Guangdong, Sichuan, and Hunan bacon, are the traditional and popular fermented meat products due to their unique flavor and texture (Huang et al., 2014). Chinese bacon products contain rich lactic acid bacteria (Liu et al., 2011), while no report evaluates their BAs formation and degradation abilities to screen the BAs-controlling bacteria. Therefore, screening the low BAs-productive lactic acid bacteria with high BAs-degrading ability from Chinese bacon products may provide novel candidates for BAs control.
Qualitative and quantitative tests of BAs have been broadly reported. High-performance liquid chromatography (HPLC) analysis can acquire reliable qualitative and quantitative data of individual amines (Ordóñez et al., 2016). In addition, the polymerase chain reaction (PCR) possesses the advantages of rapidity, sensitivity, and specificity to detect decarboxylase genes to indicate the potential BAs risk, and several research groups have utilized PCR technique to detect the BAs-producing lactic acid bacteria (Elsanhoty and Ramadan, 2016; Shiling et al., 2016). Therefore, the aim of this study is to evaluate the BAs formation and degradation abilities of lactic acid bacteria from Chinese bacon through PCR and HPLC analysis and then to select the beneficial candidate with low BAs formation and high BAs degradation ability for BAs control in fermented meat products.
Materials and Methods
Samples and Chemicals
Bacon samples were obtained from the supermarkets of Chengdu (Sichuan), Hunan (Changsha), Hubei (Wuhan), Guangdong (Guangzhou), Shanxi (Xian), and Gansu (Lanzhou) of China at the March of 2017. All samples were collected in three replicates, and stored at -20°C. The standard substances (histamine, tyramine, tryptamine, β-phenethylamine, putrescine, cadaverine, and 1, 7-dimethylheptane) were purchased from Sigma–Aldrich Co., LLC (St. Louis, MO, United States). Other chemicals were bought from Sinopharm Chemical Reagent Co., Ltd. (Shanghai, China).
Isolation of Lactic Acid Bacteria
Bacon samples (5 g) were crushed and added into 45 mL sterile water and rotated at 37°C and 140 rpm for 40 min. The liquid mixtures were diluted, spread onto the solid MRS medium (peptone 10 g/L, beef extract 8 g/L, yeast extract 4 g/L, glucose 20 g/L, diammonium hydrogen citrate 2 g/L, sodium acetate 5 g/L, K2HPO4 2 g/L, MgSO4 0.2 g/L, MnSO4 0.04 g/L, Tween 80 1 g/L, agar 14 g/L, pH = 5.7) added with 20 g/L CaCO3, and cultured at 37°C for 24 h in a vacuum bag. The single colony with the big dissolved calcium zone was selected as the potential lactic acid bacterium.
Identification of Strains
Genomic DNA of lactic acid bacteria was extracted using Gen-EluteTM Kit (Tiangen Biotech Co., Ltd, Beijing, China) according to the manufacturer’s instructions, and the DNA concentration and quality were estimated on a Nano Drop2000 spectrophotometer (Thermo, United States). The 16S rDNA sequence analysis was carried out according to our previous report (Wei et al., 2011). The 16S rDNA sequence was amplified using the universal primers of 27f (AGAGTTTGATCMTGGCTCAG) and 1492r (CTACGGCTACCTTGTTACGA), following the PCR procedure: 95°C for 5 min; 95°C for 45 s, 55°C for 1 min, 72°C for 1 min, 32 cycles; 72°C for 10 min; and 4°C for 10 min. PCR is performed in 25 μL reaction mixture containing 2 μL template DNA, 2.5 μL Easy-Taq Buffer, 2.5 μL dNTPs, 1 μL of each primer, 0.3 μL Easy-Taq enzyme, and 15.7 μL nuclease-free water. The PCR products were purified, recovered, and sequenced by the Tsingke Biological Technology Co., Ltd. (Wuhan, China). The sequence similarity analysis was carried out by the Blastn program1, and the phylogenetic tree was generated using the MEGA 5.0.
Detection of the Genes Related to BAs
Polymerase chain reaction amplification and sequence analysis were performed to confirm the presence of BAs-related genes of histidine decarboxylase (hdcA), tyrosine decarboxylase (tyrdc), ornithine decarboxylase (odc), agmatine deiminase (aguA and aguD), lysine decarboxylase (ldc), and multi-copper oxidase (sufI). The primers used in this study were listed in Table 1. PCR amplification is performed in 25 μL reaction mixture containing 2 μL of template DNA, 2.5 μL Easy-Taq Buffer, 2.5 μL dNTPs, 1 μL of each primer, and 0.3 μL Easy-Taq enzyme wherein nuclease-free water was added to reach 25 μL. The reactions were performed according to the parameters shown in Table 2. The PCR products were analyzed by electrophoresis on a 0.8% agarose gel and revealed under UV after staining with ethidium bromide. And the PCR amplicons were purified, recovered, and gene sequencing was performed by the Tsingke Biological Technology Co., Ltd. (Wuhan, China). The sequence similarity analysis was conducted through the Blastn program2.
Evaluation of BAs-Producing and Degradation Ability
To evaluate the BAs-producing ability, as-selected strains were inoculated into the MRS broth, respectively, incubated statically at 37°C for 24 h as the seed cultures, which were further transferred into 5 mL MRS broth supplemented with 1 g/L of histidine, tyrosine, tryptophan, phenylalanine, ornithine monohydrochloride, lysine, or agmatine sulfate salt. After static culturing at 37°C for 48 h, the concentrations of BAs were determined. For the BAs-degrading ability analysis, the 24 h cultures of each strain were harvested by centrifugation at 6000 ×g for 10 min, and the cell pellets were washed with 0.05 M phosphate buffer (pH = 7) and re-suspended (OD650 = 0.4) in 0.05 mol/L phosphate buffer consisting of 100 mg/L of histamine, tyramine, tryptamine, β-phenethylamine, putrescine, and cadaverine. The phosphate buffer without inoculation of Lactobacillus curvatus was used as the control. After static incubation at 37°C for 24 h, the concentrations of BAs were determined. The value of BA-degradation rate was determined based on an equation, i.e., M = [(A-B)/A] × 100%, where M is the percentage of BAs degradation, and A and B represent the initial BAs content and residual BAs content, respectively.
Fermented Meat Product Model Analysis
The fermented meat model was constructed to evaluate the BAs-controlling abilities of selected strains. The cells were inoculated into the MRS broth, statically cultured at 37°C for 24 h as the liquid seed cultures (OD650 = 0.8), which were then transferred (3%, v/w) into the fresh pork slices (10 g) added with 2% salt, 1% glucose, and 4% sucrose in sterilized flasks (50 mL). The cells were inoculated into the MRS broth, statically cultured at 37°C for 24 h as the seed cultures, which were then transferred into 10 g fresh pork slices containing 2% salt, 1% glucose, and 4% sucrose in sterilized flasks (50 mL). Thereafter, the mixtures were incubated at 37°C for 7 days, and the total BAs concentration of each sample was analyzed by HPLC. The fermented experiments were performed by inoculation with L. curvatus G-1 and L. curvatus B-1, respectively, as well as mixed L. curvatus G-1 and L. curvatus B-1, and the sample inoculated with equal volume of sterilized water was used as the control.
Determination of BAs
Analysis of BAs was performed according to a previous study (Kim et al., 2012). Briefly, to extract the BAs, 0.5 g solid sample or 0.5 mL liquid sample was mixed with 1.5 mL of 0.4 M HClO4 for 1 h. The extracts were centrifugated at 12,000 ×g for 10 min to collect the supernatant (250 μL), which was mixed with 25 μL of NaOH (2 M) and 75 μL of saturated NaHCO3 and then reacted at 50°C for 45 min after supplement with 500 μL of dansyl chloride (5 mg/mL). Then, 25 μL of NH4OH (25%) was added into the reactant to remove the residual dansyl chloride, and the final volume was increased to 1.5 mL with acetonitrile. The final reaction system was centrifuged at 2500 ×g for 5 min to obtain the supernatant, which was filtered by a 0.22 μm membrane for further analysis. The HPLC analysis was operated on an Agilent 1260 system under the parameters of Zorbax Eclipse XDB-C18 (4.6 mm × 250 mm, 5 μm), column temperature (30°C), flow rate (1 mL/min), and detection wavelength (254 nm). Using acetonitrile and H2O as mobile phase A and B, the solvent linear gradient procedure was set as: 50% A (0–3 min), 50–90% A (3–20 min), 90% A (20–29 min), 90–50% (29–32 min), and 50% A (32–35 min).
Statistical Analysis
The data were analyzed by one-way analysis of variance (ANOVA) using the statistical software SPSS 17.0, and means were compared by Duncan’s multiple range test at 5%.
Results
Isolation and Identification of Lactic Acid Bacteria From Bacon Samples
The typical Chinese bacon samples, including Sichuan bacon, Hunan bacon, Hubei bacon, Guangdong bacon, Shanxi, and Gansu bacon, were collected to isolate lactic acid bacteria. The BAs profiles of these samples were analyzed, and common six kinds of BAs were detected from different samples (Supplementary Table S1). All the samples showed the total BAs contents within the safe range, suggesting that the lactic acid bacteria in Chinese bacon may be conducive to control the BAs. CaCO3 can be dissolved by lactic acid bacteria to generate transparent zones, which is usually used to screen the candidates. Many clones showed the ability of dissolving calcium, and seven yellowish clones with big transparent zones were selected, named N-1, N-2, B-1, B-2, G-1, G-2, and GAN. The 16S rDNA fragments were amplified and sequenced to further identify the strains. Sequencing results revealed that the lengths of fragments were about 1468 bp, and all the sequences exhibited about 99% identity with that of the L. curvatus strains from the GenBank database (Table 3). The phylogenetic tree was shown in Figure 1, and as-selected seven strains were closely clustered with L. curvatus. The above results confirmed that these seven strains all belonged to L. curvatus, and corresponding 16S rDNA sequences were deposited in Genbank (accession numbers: MH194552, MH194553, MH194554, MH194555, MH194556, MH194557, and MH194558).
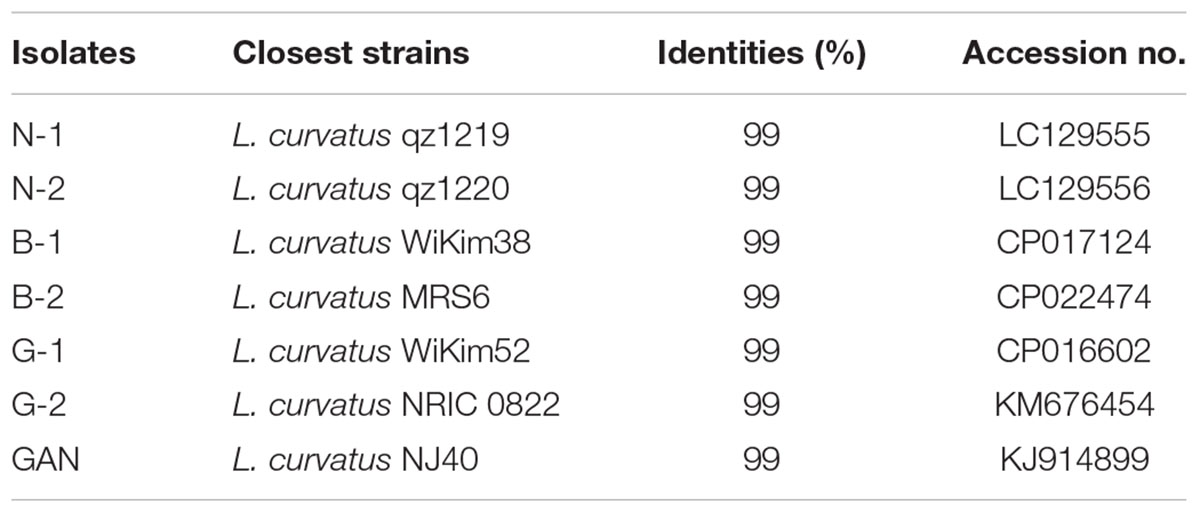
TABLE 3. 16S rDNA sequences similarities of isolated strains with representative lactic acid bacteria.
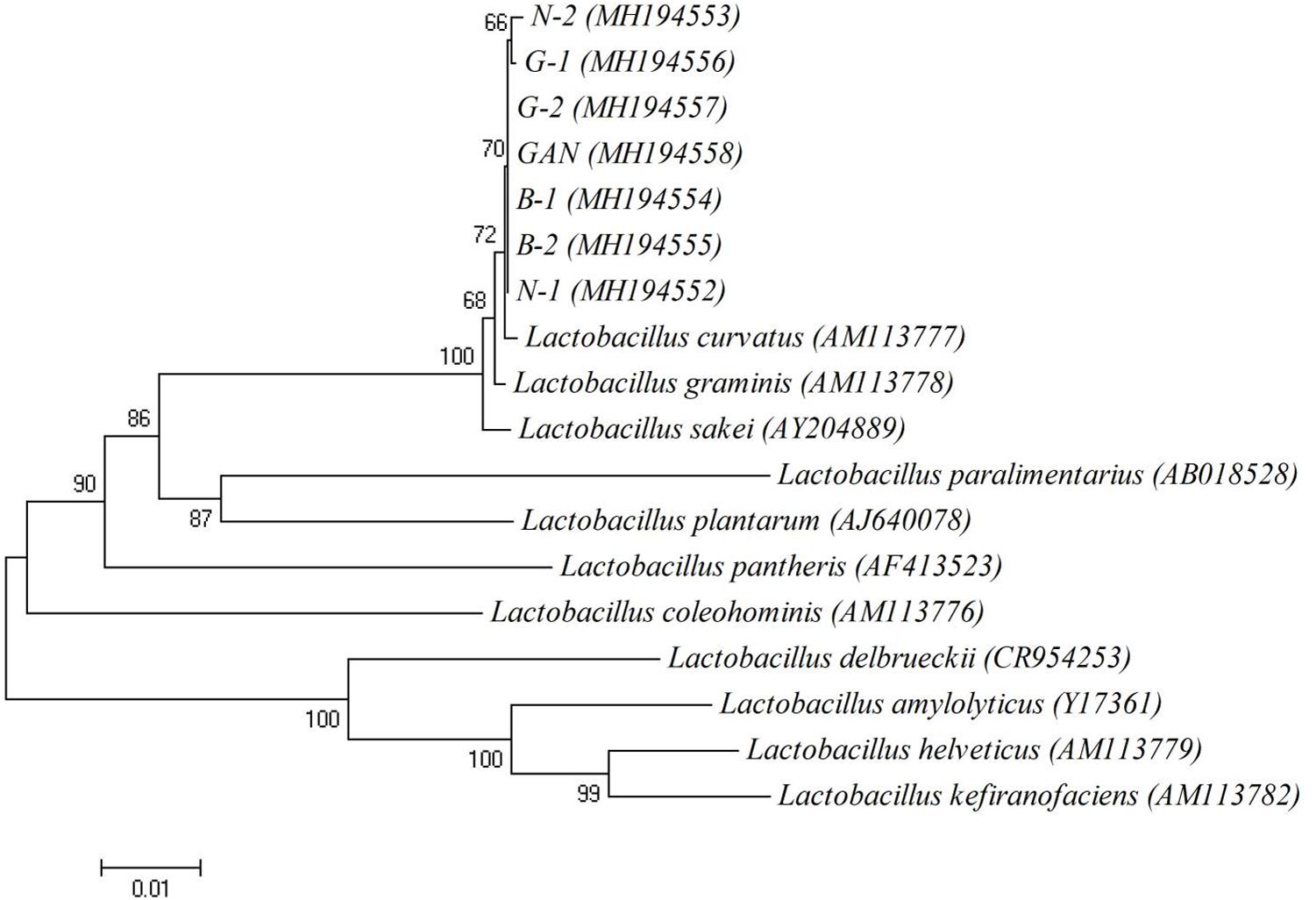
FIGURE 1. The phylogenetic tree based on 16S rDNA sequences. Numbers in parentheses represent accession numbers of 16S rDNA sequences of representative strains. The scale bar indicates 0.01 nucleotide substitution per position.
Detection of BA-Producing Genes of the Strains
To evaluate the BAs-producing abilities of seven L. curvatus strains from the gene level, PCR assays were performed to verify the presence of amino acid decarboxylase genes (hdcA, tyrdc, odc, and ldc) and agmatine deiminase (aguA and aguD). None of the L. curvatus strains was positive in histidine decarboxylase gene (hdcA), agmatine deiminase gene (aguA and aguD), and lysine decarboxylase gene (ldc) screenings with the primers HdC1/HdC2, AgmSq1/AgmSq2, AgD1/AgD2, and Cad2F/Cad2R. These results indicated that the L. curvatus strains isolated might not have cadaverine and histamine formation ability, and they might not produce putrescine via agmatine. Figure 2A shows that all the L. curvatus strains were positive in tyrosine decarboxylase gene detection, and the resulting PCR amplicons were about 1100 bp (MH225426, MH225427, MH225428, MH225429, MH225430, MH225431, and MH225432), highly homologous (about 99%) with the tyrdc gene from L. curvatus HSCC1737 (AB086652). All the strains also indicated the positive results in detection of the ornithine decarboxylase gene (Figure 2B), and the fragments were about 500 bp (MH225433, MH225434, MH225435, MH225436, MH225437, MH225438, and MH225439), showing high similarity (about 80%) with the odc gene from Oenococcus oeni (FR751077). The above results revealed that all the L. curvatus strains might have the abilities of generating tyramine and putrescine.
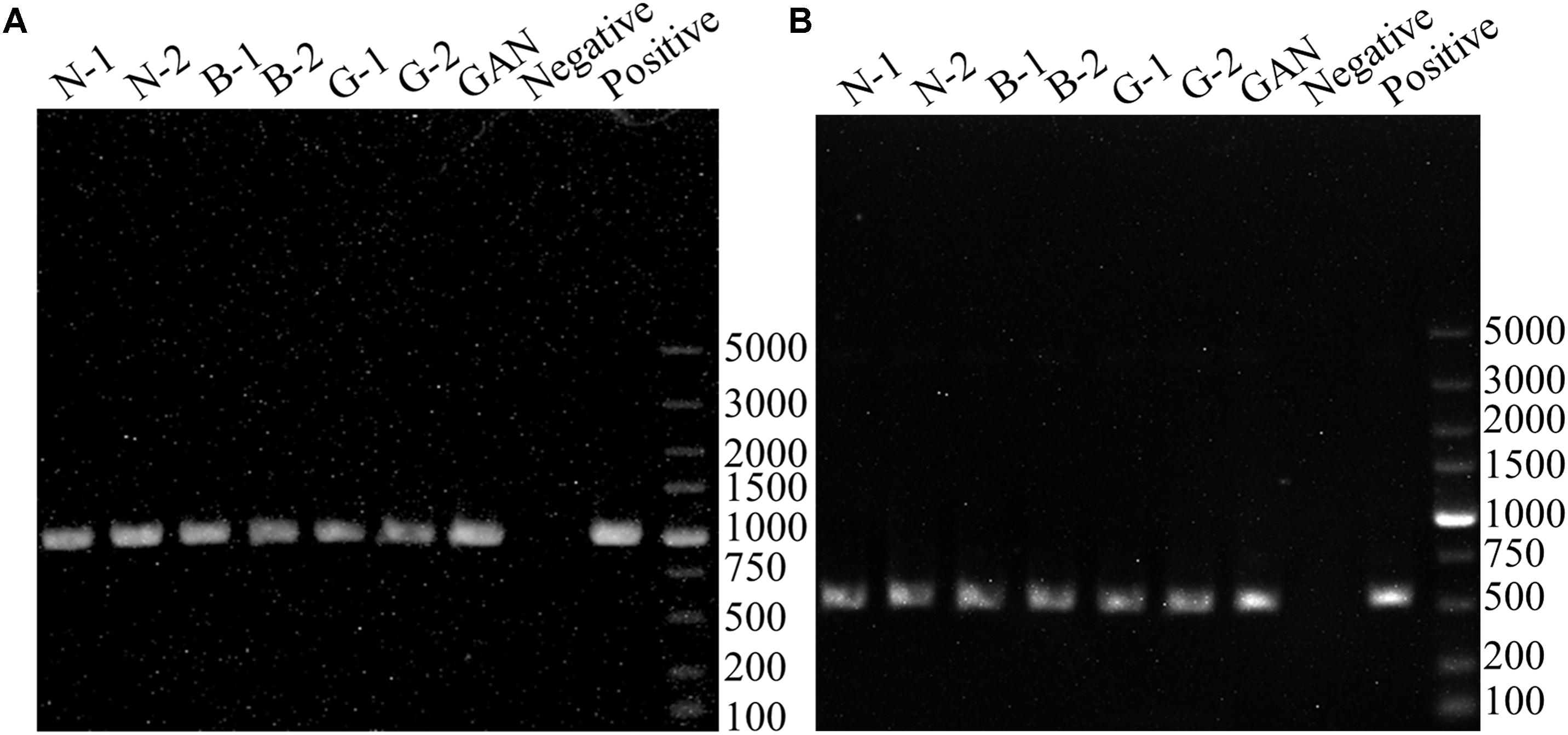
FIGURE 2. PCR results for the genes involved in BAs production. (A) PCR products using the primer pair TD2/TD5 for identification of tyrosine decarboxylase gene. (B) PCR products using the primer pair ODF/ODR for identification of ornithine decarboxylase gene. PCR positive controls (Enterococcus faecium DU-1 for tyrosine decarboxylase gene and Oenococcus oeni BJ for ornithine decarboxylase gene).
Evaluation of BAs-Producing Abilities of the Strains
The BAs-producing abilities of all strains were detected in MRS broth supplemented with corresponding precursors. After eliminating the BAs generated from MRS medium without precursors, four BAs were confirmed to be produced by all the L. curvatus strains, including tyramine, β-phenethylamine, putrescine, and cadaverine, and histamine and tryptamine were not detected. The dominant BA produced by L. curvatus strains was tyramine, followed by putrescine. Both ornithine and agmatine served as the precursors of putrescine, and the abilities of producing putrescine via ornithine were much higher than that of agmatine in our selected strains (Table 4). Additionally, all L. curvatus strains showed low cadaverine formation abilities. In comparison, the L. curvatus G-1 produced the lowest concentration of total BAs, especially the relative low level of tyramine and putrescine.
Detection of Gene Encoding Amines Degrading Enzyme
In lactic acid bacteria, the multi-copper oxidase showed a high ability of degrading BAs (Guarcello et al., 2016). Therefore, the primers of BCf/BCr were used to detect the multi-copper oxidase gene. All strains exhibited positive results with BCf/BCr primer pair (Figure 3), and the lengths of corresponding PCR products were about 350 bp (MH225440, MH225441, MH225442, MH225443, MH225444, MH225445, and MH225446). Moreover, all PCR amplicons showed a high similarity above 95% with the multi-copper oxidase gene from Lactobacillus paracasei CB9CT (KU962939). Above results indicated all L. curvatus strains probably possessed the multi-copper oxidase for degrading BAs. Therefore, the BAs degradation abilities of all L. curvatus strains were further analyzed.
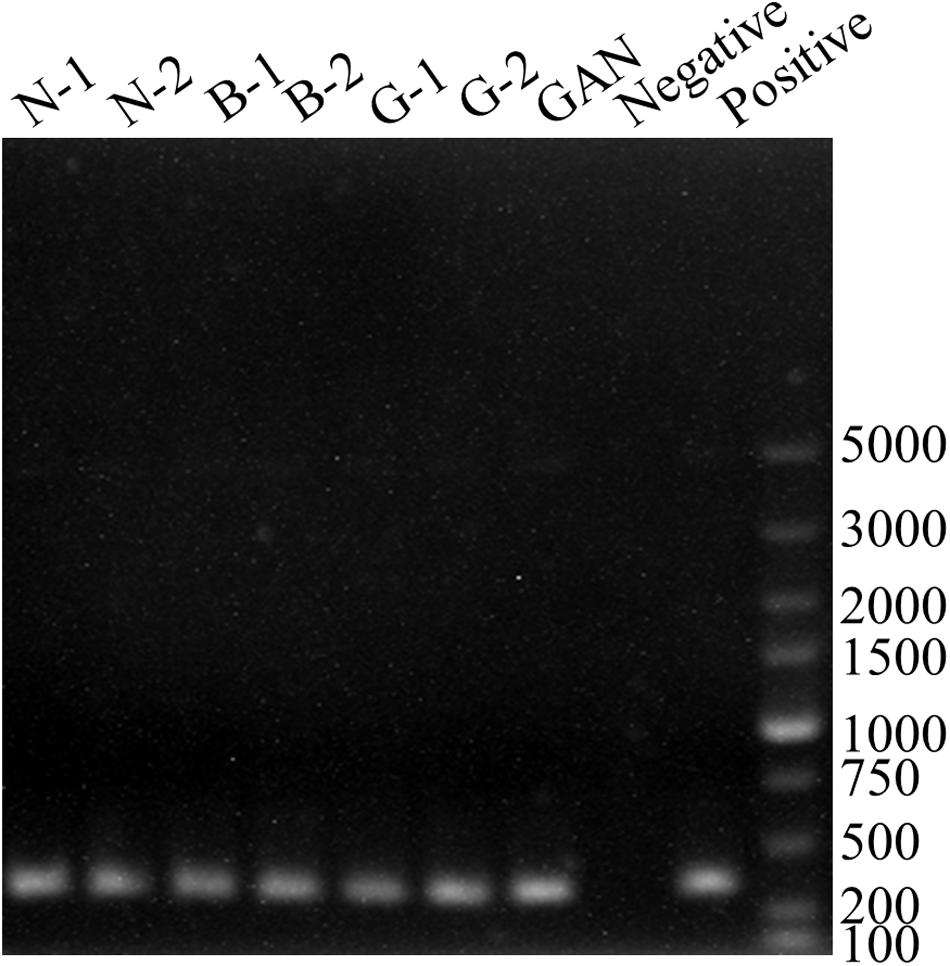
FIGURE 3. PCR results for genes involved in BAs degradation. PCR products using the primer pair BCf/BCr for identification of multi-copper oxidase gene. PCR positive control (Lactobacillus fermenti FJ for multi-copper oxidase gene).
Evaluation of the BAs Degradation Abilities of L. curvatus
The capacities of all L. curvatus strains to degrade BAs were assayed through the degradation test using the substrates of histamine, tyramine, tryptamine, β-phenethylamine, putrescine, and cadaverine. The BAs contents in broth and their corresponding controls were analyzed by HPLC. All the strains had the degradation abilities for each BA, especially for the tyramine, and different strains showed different degradation abilities (Table 5). In comparison, the L. curvatus G-1 demonstrated the highest degradation percentage, with the degradation efficiencies above 40% for all the BAs. In a whole, L. curvatus G-1 showed the highest BAs-degrading ability, as well as the lowest BAs-producing ability, and thus it exhibited the promising potential to control BAs.
The BAs-Controlling Capacity of L. curvatus G-1 in Fermented Meat Model
The L. curvatus G-1 might be the potential candidate for controlling the BAs due to its low BAs-producing ability and high BAs-degrading ability. Thus, we evaluated its BAs-controlling capacity in a fermented meat model. After culture at 37°C for 7 days, the total BAs contents of samples were analyzed and are shown in Figure 4. The control sample without inoculation of L. curvatus cells showed the highest total BAs content (1044.04 mg/kg), which was due to fermentation of native microbes in fresh meat. Inoculation with L. curvatus cells reduced the total BAs concentrations in each treatment. The lowest BAs concentration (318.19 mg/kg) was found in the sample inoculated with L. curvatus G-1, and it was much lower than that of L. curvatus B-1 (784.68 mg/kg), which agreed with their difference in BAs-producing and BAs-degrading abilities. The above results indicated that the L. curvatus G-1 had an excellent capacity for controlling BAs in fermented meat model.
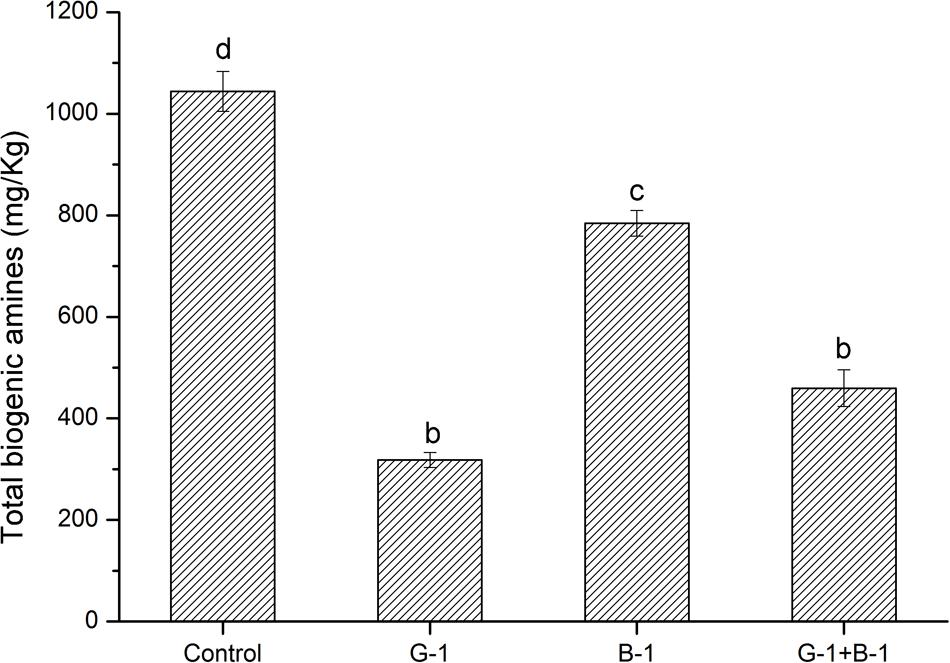
FIGURE 4. BAs contents in fermented meat product model inoculated with different strains. Different letters (a, b, c, etc.) indicate significantly different means at P < 0.05.
Discussion
The fermented meat products, usually processed from raw meat under open environment, undoubtedly consist of complex microorganisms, which are responsible for formation of good flavor and organoleptic characteristics. During the ripening of fermented meat products, proteins undergo degradation to generate large peptides, which are further hydrolyzed into oligopeptides and free amino acids (Landete et al., 2007a; Buňková et al., 2010). Some amino acids can be decarboxylated to BAs by microbes, which is undesirable for food safety. On the contrary, BAs can also be degraded by amino-oxidases of some microbes (Ladero et al., 2010). Lactic acid bacteria are usually used as starter cultures in meat products fermentation (Oki et al., 2011; Woraprayote et al., 2016), and they can produce lactic acid rapidly to decrease the pH of fermented meat product (Ladero et al., 2015), which can inhibit the growth of contaminant bacteria (Bover-Cid et al., 2001). Meanwhile, different lactic acid bacteria have different BAs formation and degradation abilities. Therefore, isolation of a lactic acid bacterium with low BAs formation and high BAs degradation ability is valuable to control the BAs in fermented meat products.
In order to screen a strain with low BAs formation and high BAs degradation ability, seven lactic acid bacteria were isolated from Chinese bacon. Based on 16S rDNA analysis, these seven strains were all identified as L. curvatus, which was reported as the predominant starter in some fermented meat products (Hugas et al., 1993; Freiding et al., 2011). Freiding et al. (2011) found that L. curvatus had no potential to produce histamine. Consistently, none of as-selected L. curvatus strains harbored histidine decarboxylase gene and produced histamine. Similar to previous reports (Freiding et al., 2011; Takebe et al., 2016), the tyrosine decarboxylase and ornithine decarboxylase genes were detected in as-selected L. curvatus strains, which agreed with the fact that all the strains could synthesize tyramine and putrescine. The enterococcal tyrosine decarboxylase was reported to be able to synthesize phenylethylamine from phenylalanine, which is structurally similar to tyrosine (Marcobal et al., 2006), and Landete et al. (2007b) also found that phenylethylamine was associated with tyramine production. Moreover, the L. curvatus strains were reported to be highly related to tyramine and β-phenylethylamine (Bover-Cid et al., 2001, 2008; De Las Rivas et al., 2008; Latorre-Moratalla et al., 2012, 2014; Li et al., 2012). Therefore, the phenylethylamine produced by L. curvatus strains isolated in this study may also result from the tyrosine decarboxylase. In addition, all isolated L. curvatus strains produced low level cadaverine, which is consistent with a previous study (Bover-Cid et al., 2008), while the lysine decarboxylase genes were not detected in all L. curvatus strains. Similarly, low level of putrescine was produced via agmatine, while agmatine deiminase genes were also negative in all isolated strains. These results indicate that unknown lysine decarboxylase or agmatine deiminase with low activities may exist in L. curvatus, which will be further investigated in the future. Suzzi and Gardini (2003) reported that the activities of amino acid decarboxylases were usually strain-specific. Among the seven strains, L. curvatus G-1 shows the lowest BAs-producing ability, which may be due to its low amino acid decarboxylase activity.
Bacteria with amine oxidase have the potential to reduce BAs in fermented meat products (Zaman et al., 2011). The amine oxidases also exist in lactic acid bacteria (Callejón et al., 2014; Guarcello et al., 2016). In a previous study, the multi-copper oxidase in lactic acid bacteria showed the potent ability to degrade BAs (Ladero et al., 2014), and L. curvatus was also reported to reduce the BAs in fermented vegetable and fermented sausage (Rabie et al., 2011; Mangia et al., 2013; Kim et al., 2017). Herein, all the L. curvatus strains isolated were confirmed to harbor multi-copper oxidase genes, and the sequences obtained were highly similar to that of L. paracasei CB9CT (Guarcello et al., 2016). The BAs degradation test indicated that all the L. curvatus strains isolated could degrade six common BAs. In comparison, the BAs-degrading capacity of L. curvatus G-1 was much higher than other strains. Therefore, L. curvatus G-1 was further assessed in a fermented meat product model, and our results show that L. curvatus G-1 can be used as starter cultures for reducing BAs in fermented meat product.
Conclusion
This study obtains seven L. curvatus strains from Chinese bacon, and they possess different BAs-producing and degrading abilities. Importantly, the L. curvatus G-1 not only indicates a relative low BAs-producing ability, but also exhibits a high BAs-degrading activity. Moreover, L. curvatus G-1 can efficiently control the BAs in the fermented meat model. This study provides a promising BAs-controlling strain of L. curvatus G-1, which will be further investigated as the starter cultures in the practical production of fermented meat products.
Author Contributions
XuW, LW, and LL designed the study. XiW and LL executed the experimental work and analyzed the data. XuW and LW contributed reagents and materials. LL, XuW, ZW, SC, and LW wrote and revised the manuscript. All authors read and approved the final manuscript.
Funding
This study was supported by the Fundamental Research Funds for the Central Universities (No. 2662016PY121) and the National Natural Science Foundation of China (No. 31501468).
Conflict of Interest Statement
The authors declare that the research was conducted in the absence of any commercial or financial relationships that could be construed as a potential conflict of interest.
Supplementary Material
The Supplementary Material for this article can be found online at: https://www.frontiersin.org/articles/10.3389/fmicb.2018.01015/full#supplementary-material
Footnotes
References
Bover-Cid, S., Hugas, M., Izquierdo-Pulido, M., and Vidal-Carou, M. C. (2001). Amino acid-decarboxylase activity of bacteria isolated from fermented pork sausages. Int. J. Food Microbiol. 66, 185–189. doi: 10.1016/S0168-1605(00)00526-2
Bover-Cid, S., Miguélez-Arrizado, M. J., Becker, B., Holzapfel, W. H., and Vidal-Carou, M. C. (2008). Amino acid decarboxylation by Lactobacillus curvatus CTC273 affected by the pH and glucose availability. Food Microbiol. 25, 269–277. doi: 10.1016/j.fm.2007.10.013
Buňková, L., Buňka, F., Klčovská, P., Mrkvička, V., Doležalová, M., and Kráčmar, S. (2010). Formation of biogenic amines by Gram-negative bacteria isolated from poultry skin. Food Chem. 121, 203–206. doi: 10.1016/j.foodchem.2009.12.012
Callejón, S., Sendra, R., Ferrer, S., and Pardo, I. (2014). Identification of a novel enzymatic activity from lactic acid bacteria able to degrade biogenic amines in wine. Appl. Microbiol. Biotechnol. 98, 185–198. doi: 10.1007/s00253-013-4829-6
Cornu, M., Billoir, E., Bergis, H., Beaufort, A., and Zuliani, V. (2011). Modeling microbial competition in food: application to the behavior of Listeria monocytogenes and lactic acid flora in pork meat products. Food Microbiol. 28, 639–647. doi: 10.1016/j.fm.2010.08.007
Coton, M., Coton, E., Lucas, P., and Lonvaud, A. (2004). Identification of the gene encoding a putative tyrosine decarboxylase of Carnobacterium divergens 508. Development of molecular tools for the detection of tyramine-producing bacteria. Food Microbiol. 21, 125–130. doi: 10.1016/j.fm.2003.10.004
Coton, M., Romano, A., Spano, G., Ziegler, K., Vetrana, C., Desmarais, C., et al. (2010). Occurrence of biogenic amine-forming lactic acid bacteria in wine and cider. Food Microbiol. 27, 1078–1085. doi: 10.1016/j.fm.2010.07.012
Cueva, C., García-Ruiz, A., González-Rompinelli, E., Bartolome, B., Martín- lvarez, P. J., Salazar, O., et al. (2012). Degradation of biogenic amines by vineyard ecosystem fungi. Potential use in winemaking. J. Appl. Microbiol. 112, 672–682. doi: 10.1111/j.1365-2672.2012.05243.x
Dapkevicius, M. L. N. E., Nout, M. J. R., Rombouts, F. M., Houben, J. H., and Wymenga, W. (2000). Biogenic amine formation and degradation by potential fish silage starter microorganisms. Int. J. Food Microbiol. 57, 107–114. doi: 10.1016/S0168-1605(00)00238-5
De Las Rivas, B., Ruiz-Capillas, C., Carrascosa, A. V., Curiel, J. A., Jiménez-Colmenero, F., and Muñoz, R. (2008). Biogenic amine production by Gram-positive bacteria isolated from Spanish dry-cured “chorizo” sausage treated with high pressure and kept in chilled storage. Meat Sci. 80, 272–277. doi: 10.1016/j.meatsci.2007.12.001
De Martinis, E. C. P., and Freitas, F. Z. (2003). Screening of lactic acid bacteria from Brazilian meats for bacteriocin formation. Food Control 14, 197–200. doi: 10.1016/S0956-7135(02)00092-0
Diaz, M., Del Rio, B., Ladero, V., Redruello, B., Fernández, M., Martin, M. C., et al. (2015). Isolation and typification of histamine-producing Lactobacillus vaginalis strains from cheese. Int. J. Food Microbiol. 215, 117–123. doi: 10.1016/j.ijfoodmicro.2015.08.026
Elsanhoty, R. M., and Ramadan, M. F. (2016). Genetic screening of biogenic amines production capacity from some lactic acid bacteria strains. Food Control 68, 220–228. doi: 10.1016/j.foodcont.2016.04.002
Fernández, M., Del Río, B., Linares, D. M., Martín, M. C., and Alvarez, M. A. (2006). Real-time polymerase chain reaction for quantitative detection of histamine-producing bacteria: use in cheese production. J. Dairy Sci. 89, 3763–3769. doi: 10.3168/jds.S0022-0302(06)72417-1
Freiding, S., Gutsche, K. A., Ehrmann, M. A., and Vogel, R. F. (2011). Genetic screening of Lactobacillus sakei and Lactobacillus curvatus strains for their peptidolytic system and amino acid metabolism, and comparison of their volatilomes in a model system. Syst. Appl. Microbiol. 34, 311–320. doi: 10.1016/j.syapm.2010.12.006
García-Ruiz, A., González-Rompinelli, E. M., Bartolomé, B., and Moreno-Arribas, M. V. (2011). Potential of wine-associated lactic acid bacteria to degrade biogenic amines. Int. J. Food Microbiol. 148, 115–120. doi: 10.1016/j.ijfoodmicro.2011.05.009
Gardini, F., Zogul, Y., Suzzi, G., Tabanelli, G., and Zogul, F. (2016). Technological factors affecting biogenic amine content in foods: a review. Front. Microbiol. 7:1218. doi: 10.3389/fmicb.2016.01218
Guarcello, R., De Angelis, M., Settanni, L., Formiglio, S., Gaglio, R., Minervini, F., et al. (2016). Selection of amine-oxidizing dairy lactic acid bacteria and identification of the enzyme and gene involved in the decrease of biogenic amines. Appl. Environ. Microbiol. 82, 6870–6880. doi: 10.1128/aem.01051-16
Herrerofresno, A., Martínez, N., Sánchezllana, E., Díaz, M., Fernández, M., Martin, M. C., et al. (2012). Lactobacillus casei strains isolated from cheese reduce biogenic amine accumulation in an experimental model. Int. J. Food Microbiol. 157, 297–304. doi: 10.1016/j.ijfoodmicro.2012.06.002
Huang, Y., Li, H., Huang, T., Li, F., and Sun, J. (2014). Lipolysis and lipid oxidation during processing of Chinese traditional smoke-cured bacon. Food Chem. 149, 31–39. doi: 10.1016/j.foodchem.2013.10.081
Hugas, M., Garriga, M., Aymerich, T., and Monfort, J. M. (1993). Biochemical characterization of lactobacilli from dry fermented sausages. Int. J. Food Microbiol. 18, 107–113. doi: 10.1016/0168-1605(93)90215-3
Kim, B., Byun, B. Y., and Mah, J. H. (2012). Biogenic amine formation and bacterial contribution in Natto products. Food Chem. 135, 2005–2011. doi: 10.1016/j.foodchem.2012.06.091
Kim, S.-H., Kang, K. H., Kim, S. H., Lee, S., Lee, S.-H., Ha, E.-S., et al. (2017). Lactic acid bacteria directly degrade N-nitrosodimethylamine and increase the nitrite-scavenging ability in kimchi. Food Control 71, 101–109. doi: 10.1016/j.foodcont.2016.06.039
Ladero, V., Calles-Enriquez, M., Fernandez, M. A., and Alvarez, M. (2010). Toxicological effects of dietary biogenic amines. Curr. Nutr. Food Sci. 6, 145–156. doi: 10.2174/157340110791233256
Ladero, V., Herrerofresno, A., Martinez, N., Río, B. D., Linares, D. M., Fernández, M., et al. (2014). Genome sequence analysis of the biogenic amine-degrading strain Lactobacillus casei 5b. Genome Announc. 2, e1199-13. doi: 10.1128/genomeA.01199-13
Ladero, V., Martín, M. C., Redruello, B., Mayo, B., Flórez, A. B., Fernández, M., et al. (2015). Genetic and functional analysis of biogenic amine production capacity among starter and non-starter lactic acid bacteria isolated from artisanal cheeses. Eur. Food Res. Technol. 241, 377–383. doi: 10.1007/s00217-015-2469-z
Ladero, V., Rattray, F. P., Mayo, B., Martín, M. C., Fernández, M., and Alvarez, M. A. (2011). Sequencing and transcriptional analysis of the biosynthesis gene cluster of putrescine-producing Lactococcus lactis. Appl. Environ. Microbiol. 77, 6409–6418. doi: 10.1128/aem.05507-11
Landete, J. M., De Las Rivas, B., Marcobal, A., and Muñoz, R. (2007a). Molecular methods for the detection of biogenic amine-producing bacteria on foods. Int. J. Food Microbiol. 117, 258–269. doi: 10.1016/j.ijfoodmicro.2007.05.001
Landete, J. M., Ferrer, S., Polo, L., and Pardo, I. (2005). Biogenic amines in wines from three spanish regions. J. Agric. Food Chem. 53, 1119–1124. doi: 10.1021/jf049340k
Landete, J. M., Pardo, I., and Ferrer, S. (2007b). Tyramine and phenylethylamine production among lactic acid bacteria isolated from wine. Int. J. Food Microbiol. 115, 364–368. doi: 10.1016/j.ijfoodmicro.2006.10.051
Latorre-Moratalla, M. L., Bover-Cid, S., Bosch-Fusté, J., Veciana-Nogués, M. T., and Vidal-Carou, M. C. (2014). Amino acid availability as an influential factor on the biogenic amine formation in dry fermented sausages. Food Control 36, 76–81. doi: 10.1016/j.foodcont.2013.07.038
Latorre-Moratalla, M. L., Bover-Cid, S., Bosch-Fusté, J., and Vidal-Carou, M. C. (2012). Influence of technological conditions of sausage fermentation on the aminogenic activity of L. curvatus CTC273. Food Microbiol. 29, 43–48. doi: 10.1016/j.fm.2011.08.004
Latorre-Moratalla, M. L., Bover-Cid, S., Talon, R., Garriga, M., Zanardi, E., Ianieri, A., et al. (2010). Strategies to reduce biogenic amine accumulation in traditional sausage manufacturing. LWT Food Sci. Technol. 43, 20–25. doi: 10.1016/j.lwt.2009.06.018
Lázaro, C. A., Conte-Júnior, C. A., Canto, A. C., Monteiro, M. L. G., Costa-Lima, B., Cruz, A. G. D., et al. (2015). Biogenic amines as bacterial quality indicators in different poultry meat species. LWT Food Sci. Technol. 60, 15–21. doi: 10.1016/j.lwt.2014.09.025
Li, L., Wang, P., Xu, X., and Zhou, G. (2012). Influence of various cooking methods on the concentrations of volatile N-nitrosamines and biogenic amines in dry-cured sausages. J. Food Sci. 77, C560. doi: 10.1111/j.1750-3841.2012.02667.x
Liu, S.-N., Han, Y., and Zhou, Z.-J. (2011). Lactic acid bacteria in traditional fermented Chinese foods. Food Res. Int. 44, 643–651. doi: 10.1016/j.foodres.2010.12.034
Lu, S., Xu, X., Shu, R., Zhou, G., Meng, Y., Sun, Y., et al. (2010). Characterization of biogenic amines and factors influencing their formation in traditional Chinese sausages. J. Food Sci. 75, M366–M372. doi: 10.1111/j.1750-3841.2010.01677.x
Mangia, N. P., Trani, A., Di Luccia, A., Faccia, M., Gambacorta, G., Fancello, F., et al. (2013). Effect of the use of autochthonous Lactobacillus curvatus, Lactobacillus plantarum and Staphylococcus xylosus strains on microbiological and biochemical properties of the Sardinian fermented sausage. Eur. Food Res. Technol. 236, 557–566. doi: 10.1007/s00217-013-1915-z
Marcobal, A., de las Rivas, B., and Muñoz, R. (2006). First genetic characterization of a bacterial beta-phenylethylamine biosynthetic enzyme in Enterococcus faecium RM58. FEMS Microbiol. Lett. 258, 144–149. doi: 10.1111/j.1574-6968.2006.00206.x
Nie, X., Zhang, Q., and Lin, S. (2014). Biogenic amine accumulation in silver carp sausage inoculated with Lactobacillus plantarum plus Saccharomyces cerevisiae. Food Chem. 153, 432–436. doi: 10.1016/j.foodchem.2013.12.093
Oki, K., Rai, A. K., Sato, S., Watanabe, K., and Tamang, J. P. (2011). Lactic acid bacteria isolated from ethnic preserved meat products of the Western Himalayas. Food Microbiol. 28, 1308–1315. doi: 10.1016/j.fm.2011.06.001
Ordóñez, J. L., Troncoso, A. M., García-Parrilla, M. D. C., and Callejón, R. M. (2016). Recent trends in the determination of biogenic amines in fermented beverages – A review. Anal. Chim. Acta 939, 10–25. doi: 10.1016/j.aca.2016.07.045
Rabie, M. A., Siliha, H., El-Saidy, S., El-Badawy, A. A., and Malcata, F. X. (2011). Reduced biogenic amine contents in sauerkraut via addition of selected lactic acid bacteria. Food Chem. 129, 1778–1782. doi: 10.1016/j.foodchem.2011.05.106
Romano, A., Trip, H., Lolkema, J. S., and Lucas, P. M. (2013). Three-component lysine/ornithine decarboxylation system in Lactobacillus saerimneri 30a. J. Bacteriol. 195, 1249–1254. doi: 10.1128/jb.02070-12
Shiling, L., Caihong, J., Xinglian, X., Chengjian, X., Kaixiong, L., and Ruihua, S. (2016). Improved screening procedure for biogenic amine production by lactic acid bacteria and Enterobacteria. Czech. J. Food. Sci. 33, 19–26. doi: 10.17221/197/2014-cjfs
Silvina, F., Constanza, L., and Graciela, V. (2010). Role of lactic acid bacteria during meat conditioning and fermentation: peptides generated as sensorial and hygienic biomarkers. Meat Sci. 86, 66–79. doi: 10.1016/j.meatsci.2010.04.023
Suzzi, G., and Gardini, F. (2003). Biogenic amines in dry fermented sausages: a review. Int. J. Food Microbiol. 88, 41–54. doi: 10.1016/S0168-1605(03)00080-1
Takebe, Y., Takizaki, M., Tanaka, H., Ohta, H., Niidome, T., and Morimura, S. (2016). Evaluation of the biogenic amine-production ability of lactic acid bacteria isolated from Tofu-misozuke. Food Sci. Technol. Res. 22, 673–678. doi: 10.3136/fstr.22.673
Wang, Y., Li, F., Zhuang, H., Chen, X., Li, L., Qiao, W., et al. (2015a). Effects of plant polyphenols and α-tocopherol on lipid oxidation, residual nitrites, biogenic amines, and N-nitrosamines formation during ripening and storage of dry-cured bacon. LWT Food Sci. Technol. 60, 199–206. doi: 10.1111/1750-3841.12796
Wang, Y., Li, F., Zhuang, H., Li, L., Chen, X., and Zhang, J. (2015b). Effects of plant polyphenols and α-tocopherol on lipid oxidation, microbiological characteristics, and biogenic amines formation in dry-cured bacons. J. Food Sci. 80, C547–C555. doi: 10.1111/1750-3841.12796
Wei, X., Luo, M., Xu, L., Zhang, Y., Lin, X., Kong, P., et al. (2011). Production of fibrinolytic enzyme from Bacillus amyloliquefaciens by fermentation of chickpeas, with the evaluation of the anticoagulant and antioxidant properties of chickpeas. J. Agric. Food. Chem. 59, 3957–3963. doi: 10.1021/jf1049535
Woraprayote, W., Malila, Y., Sorapukdee, S., Swetwiwathana, A., Benjakul, S., and Visessanguan, W. (2016). Bacteriocins from lactic acid bacteria and their applications in meat and meat products. Meat Sci. 120, 118–132. doi: 10.1016/j.meatsci.2016.04.004
Zaman, M. Z., Abu Bakar, F., Jinap, S., and Bakar, J. (2011). Novel starter cultures to inhibit biogenic amines accumulation during fish sauce fermentation. Int. J. Food Microbiol. 145, 84–91. doi: 10.1016/j.ijfoodmicro.2010.11.031
Zaman, M. Z., Bakar, F. A., Selamat, J., Bakar, J., Ang, S. S., and Chong, C. Y. (2014). Degradation of histamine by the halotolerant Staphylococcus carnosus FS19 isolate obtained from fish sauce. Food Control 40, 58–63. doi: 10.1016/j.foodcont.2013.11.031
Keywords: biogenic amines, formation, degradation, Lactobacillus cxurvatus, Chinese bacon
Citation: Li L, Wen X, Wen Z, Chen S, Wang L and Wei X (2018) Evaluation of the Biogenic Amines Formation and Degradation Abilities of Lactobacillus curvatus From Chinese Bacon. Front. Microbiol. 9:1015. doi: 10.3389/fmicb.2018.01015
Received: 07 February 2018; Accepted: 30 April 2018;
Published: 15 May 2018.
Edited by:
Chunbao Li, Nanjing Agricultural University, ChinaReviewed by:
Victor Ladero, Consejo Superior de Investigaciones Científicas (CSIC), SpainQingli Dong, University of Shanghai for Science and Technology, China
Miaoyun Li, Henan Agricultural University, China
Copyright © 2018 Li, Wen, Wen, Chen, Wang and Wei. This is an open-access article distributed under the terms of the Creative Commons Attribution License (CC BY). The use, distribution or reproduction in other forums is permitted, provided the original author(s) and the copyright owner are credited and that the original publication in this journal is cited, in accordance with accepted academic practice. No use, distribution or reproduction is permitted which does not comply with these terms.
*Correspondence: Ling Wang, wangling@mail.hzau.edu.cn Xuetuan Wei, weixuetuan@mail.hzau.edu.cn