- 1College of Food Science and Technology, Shanghai Ocean University, Shanghai, China
- 2Laboratory of Quality and Safety Risk Assessment for Aquatic Products on Storage and Preservation, Ministry of Agriculture and Rural Affairs of the People’s Republic of China, Shanghai, China
- 3Shanghai Engineering Research Center of Aquatic-Product Processing and Preservation, Shanghai, China
Vibrio parahaemolyticus causes the most seafood-attributed gastroenteritis outbreaks worldwide and studies on its pathogenesis during passage through the human digestive fluids are limited. An in vitro continuous model system mimicking passage through saliva, gastric and intestinal fluid was used to study the survival, morphology and virulence-related gene expression of a total of sixty pathogenic, and non-pathogenic V. parahaemolyticus strains. The changes to these three characteristics for the sixty V. parahaemolyticus strains were minimal on passage through the saliva fluid. No V. parahaemolyticus strains survived passage through gastric fluid with low pH values (2.0 and 3.0) and the cells, examined microscopically, were severely damaged. However, when the pH of gastric fluid increased to 4.0, the bacterial survival rate was 54.70 ± 1.11%, and the survival rate of pathogenic strains was higher when compared to non-pathogenic strains. Even though the bactericidal effect of intestinal fluid was lower than gastric fluid, virulence-related gene expression was enhanced in the intestinal fluid. Seafood matrices can significantly raise the pH level of gastric fluid and thus aid the survival of V. parahaemolyticus through passage from human gastric acid and progression of pathogenesis in the intestinal fluid. We confirmed these phenomena in the in vitro continuous digestion model.
Introduction
Vibrio parahemolyticus is the most common foodborne pathogen found in the marine and estuarine environment (Robert et al., 1969). Consumption of raw or undercooked seafood contaminated with high numbers of this bacterium causes acute gastroenteritis and serious septicemia (McLaughlin et al., 2005). The two major toxins of V. parahaemolyticus are heat resistant direct hemolysin (TDH) and heat resistant hemolysin related hemolysin (TRH), which are encoded by tdh and trh genes, respectively (Raimondi et al., 2000; Ceccarelli et al., 2013). TRH and TDH have high sequence homology (68%) where these toxins show beta-hemolytic activity on Wagatsuma agar and both show cytotoxicity ad enterotoxicity (Takeshi et al., 1990; Ohnishi et al., 2011). A type III secretion system (T3SS), which can utilize a needle-like apparatus to translocate effectors into the host cells, also contributes to the virulence of V. parahaemolyticus (Ham and Orth, 2012; Zhang and Orth, 2013). T3SS2 is associated with cytotoxicity in intestinal cells and enterotoxicity in infected animal models (Ham and Orth, 2012). However, there is limited information of the pathogenesis of V. parahaemolyticus through passage in the human digestive tract.
Takaaki used Caco-2 cells to reveal the infection pathway of V. parahaemolyticus (Shimohata et al., 2011) and a rabbit model was used to reveal how V. parahaemolyticus causes intestinal epithelial surface damage and progression of pathogenesis (Ritchie et al., 2012). However pathogenesis is dependent on V. parahaemolyticus, which in spite of being acid sensitive, survives the low pH in the gastric fluid. Survival and escape from the gastric fluid can be studied using an in vitro simulation model of digestive processes. This model system simulates pH changes, enzymes and gastric emptying, gastric peristalsis, secretion of digestive fluids, and various other dynamic processes (Molly et al., 1993; Havenaar and Minekus, 1996; Barroso et al., 2015).
This study aims to determine the survival rates, morphological changes, survival variability and related virulence gene expressions of V. parahaemolyticus in simulated digestive fluids (Saliva, Gastric fluid, and Intestinal fluid). This information will improve the understanding of the pathogenesis of V. parahaemolyticus in human digestive tract and provide information for design of additional control measures to reduce the human disease burden.
Materials and Methods
Vibrio parahaemolyticus Strains and Inoculum Preparation
The strain information is shown in Supplementary Table 1. The sixty strains of V. parahaemolyticus used in this study were collected from the American type culture collection (ATCC, 2 strains), from patients admitted to Shanghai hospital (28 strains), and from aquatic products sold from retail outlets in Shanghai (30 strains). Strains were stored at -80°C in glycerol test tubes with Tryptic Soy Broth (TSB, Land Bridge Technology, Beijing, China) and 50% (v/v) glycerol. Strains were steaked onto thiosulfate citrate bile salts sucrose (TCBS; Beijing Land Bridge Technology Company Ltd., Beijing, China) agar culture medium and incubated at 37°C for 18 h. A single colony from TCBS plate was transferred into 9 mL TSB containing 3% NaCl, and then cultured overnight at 37°C and 220 rpm. The overnight culture was then diluted with 0.1% peptone water (PW; Beijing Land Bridge Technology Company Ltd., Beijing, China) to an optical density value for 1.2 ± 0.02 at 600 nm (OD600). A further 100 fold dilution with 0.1% PW diluted was needed to obtain the appropriate number of bacteria for the digestion experiments. All the experiments were carried out in a Class 2 biological safety cabinet, where appropriate.
Preparation of Simulated Human Digestion Fluids and Detailed Digestion Protocol
The simulated human digestion fluids included saliva (SSF), gastric fluid (SGF), and intestinal fluid (SIF). Formulation of simulated digestion fluids as described by Minekus (Minekus et al., 2014) is shown in Table 1. 1 M HCl and 1 M NaOH were used to adjust the pH (SSF at 7.0, SGF at 2.0/3.0/4.0, SIF at 7.0) and all of the fluids were pre-warmed at 37°C before use. Figure 1A shows the specific steps of digestive fluids treatment for V. parahaemolyticus and Figure 1B shows a detailed protocol of a simulated continuous human digestion model. Plate counting method was used to enumerate bacteria surviving the digestive fluids treatment. Each sample was plated in duplicate on TCBS after appropriate 10-fold serial dilution by 0.1% PW and incubated at 37°C for 24 h for colony counting.
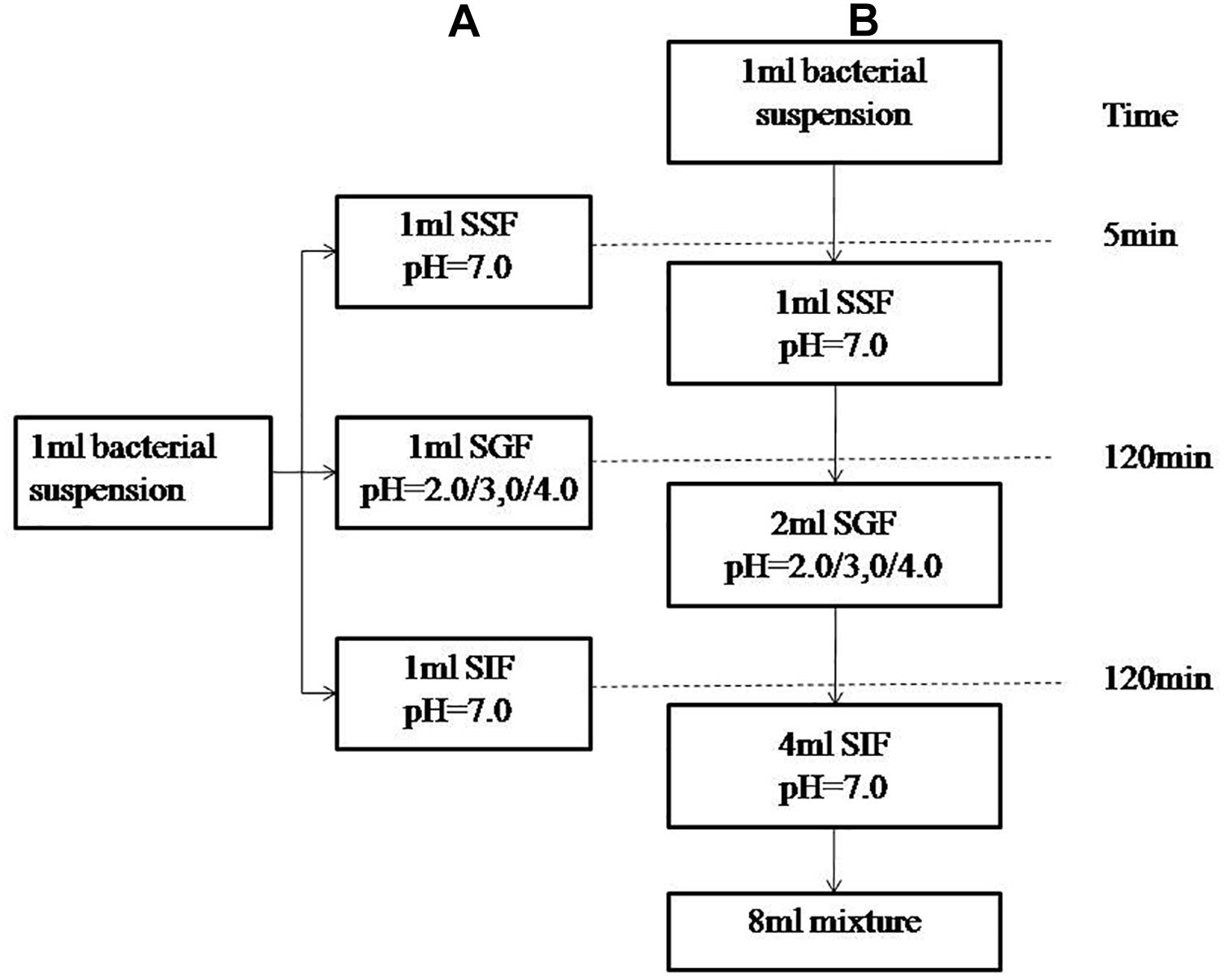
Figure 1. Schematic representation of the experimental designs. (A) describes experiment in which the bacterial suspensions were treated with simulated salvia fluid (SSF), simulated gastric fluid (SGF), and simulated intestinal fluid (SIF). (B) describes a three-step in vitro continuous digestion model. All the experiments were conducted at 37°C and at a shaking at the speed of 110 rpm.
Preparation and Inoculation of Aquatic Product Samples
Samples of fresh salmon, oysters and shrimps were purchased from a local supermarket in Shanghai, China, and stored at -20°C before treatment. After thawing, seafood samples were put into boiling water for 20 min to inactivate the native bacteria and then cooled to room temperature (25 ± 2°C) (Xie et al., 2012). A food processor (CombiMax K600, Braun, Germany) was used to reduce the particle size of the seafood samples as an adjunct to chewing. The speed of the chopping blade was set at 1500 rpm for 5 min to prepare the “chewed” salmon/shrimp/oysters (150 g sample and 20 ml sterile water) (Agrawal et al., 1997; Hermans et al., 2012; Lewis et al., 2012; Qing et al., 2013). Each chewed sample was inoculated with 1 ml of culture suspension (109 CFU/ml) for 30 min to allow for bacterial attachment at room temperature. The final concentration of V. parahaemolyticus inoculated on the seafood samples was 7 log10 CFU/g on average and each sample was prepared in duplicate. The inoculated aquatic products were mixed with SGF (pH = 1.6) in a ratio of 1:1 and cultured in a shaker which was set to 110 rpm and 37°C for 2 h.
Scanning Electron Microscopy (SEM) and Transmission Electron Microscopy (TEM)
In preparing a sample for SEM, 1 ml of bacteria suspension was fixed with 2.5% glutaraldehyde for 4 h at 4°C, and this sample was then dehydrated in a solution containing ascending concentrations of ethanol (30, 50, 70, 90, and 100% twice for 10 min each). A FEI Nova 450 scanning electron microscope was then used to observe the bacteria after the samples were gold plated. In prepping a sample for TEM, a 1% osmium acid solution was used to fix the sample for 2 h and dehydrated as described above. The dehydrated sample was then treated with a mixture of entrapped agents and acetone (V/V = 1/1) for 1 h and a Leica UC6 ultramicrotome was used to obtain ultrathin slices. These ultrathin slices were treated further with uranyl acetate solution saturated with 50% alcohol and lead citrate solution, respectively for 5 min before observation under a JEM2100 transmission electron microscope.
RNA Extraction and RT-PCR
RNA was extracted using a RNA Extraction Kit (Shanghai general Biotechnology Co., Ltd.) in accordance with the manufacturer’s instructions. The concentration of RNA was determined using absorbance at 260 nm (ND-1000 spectrophotometer, NanoDrop Technologies, Wilmington, DE, United States) and 200 ng total RNA was used for reverse transcription by RT kit and gDNA Eraser (Takar, Dalian, China) also in accordance with the manufacturer’s instructions.
Table 2 lists the gene function and the primers used for qPCR. The final volume of each qPCR reaction mixture was 20 μl and was made up with 0.4 μl ROX Π, 10 μl TaqMan Gene Expression Master Mix, 6 μl ddH2O, 2 μl of template cDNA, and 0.8 μM of forward and reverse PCR primers. Deionized water was used as a negative control in each operation. Duplicate reactions for the gene of interest and the 16S rRNA gene were run in the Applied Biosystems 7500 Fast Real-Time PCR System (Applied Biosystems, Carlsbad, CA, United States). Relative quantification was analyzed by the comparative CT method (ΔΔCT method) (Livak and Schmittgen, 2001).
Statistical Analysis
All data are presented as the mean ± standard deviation (SD). Statistical analysis was performed using SPSS statistical package 19.0 (SPSS Inc., Chicago, United States). The level of statistical significance (p < 0.05) was tested by analysis of variance (ANOVA).
Results
Survival and Morphological Changes of V. parahaemolyticus in Simulated Digestion Fluids
The survival of V. parahaemolyticus in SSF, is shown in Supplementary Table 2. The viable cell counts between control and treatment groups were not significantly different (P > 0.05). The survival rate of the 60 strains of V. parahaemolyticus ranged from 105.41 ± 0.43% to 89.46 ± 0.44%. The frequency distribution of bacterial viability after simulated saliva treatment is shown in Figure 2A, of which 10 isolates were over 100% and only 1 was below 90%. Both SEM and TEM images (Figure 2B) showed that SSF treatment had no significant effect on the viability of V. parahaemolyticus.
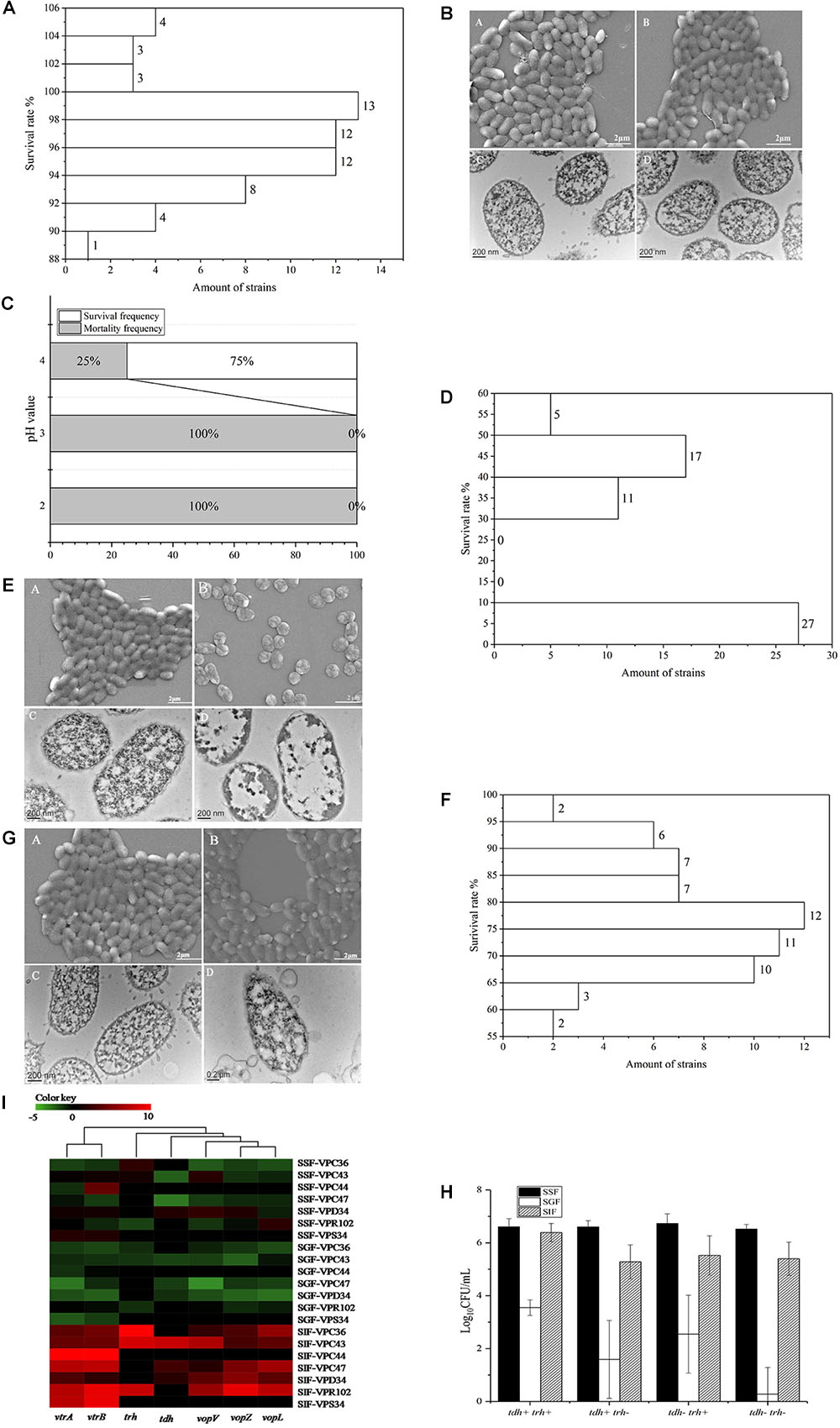
Figure 2. Survival of V. parahaemolyticus in simulated digestive fluids. (A) Distribution of V. parahaemolyticus viability [treatment (Log10CFU/mL)/control (Log10CFU/mL)] treated by SSF. (B) Representative photomicrographs of untreated V. parahaemolyticus by SEM (A) and TEM (C), V. parahaemolyticus treated by SSF by SEM (B) and TEM (D). (C) Mortality and survival frequency distribution of V. parahaemolyticus treated with SGF (pH = 2.0, 3.0, and 4.0). (D) Distribution of V. parahaemolyticus viability [treatment (Log10CFU/mL)/control (Log10CFU/mL)] treated by SGF (pH = 4.0). (E) Representative photomicrographs of untreated V. parahaemolyticus by SEM (A) and TEM (C), V. parahaemolyticus treated by SGF by SEM (B) and TEM (D). (F) Distribution of V. parahaemolyticus viability [treatment (Log10CFU/mL)/control(Log10CFU/mL)] treated by SIF. (G) Representative photomicrographs of untreated V. parahaemolyticus by SEM (A) and TEM (C), V. parahaemolyticus treated by SIF by SEM (B) and TEM (D). (H) Survival of V. parahaemolyticus from different genotypes in simulated digestive fluids, respectively. (I) Heatmap shows the relative expression of virulence-related genes in V. parahaemolyticus in simulated digestive fluids for 2 h, respectively. The color codes represent relative expressions, ranging from green (induced expression) to red (repressed expression).
Three different gastric fluids, SGF, with pH values of 2.0, 3.0, and 4.0 were chosen to study the survival of V. parahaemolyticus. The results of viable cell counts are shown in Supplementary Table 3 and in this fluid, the viable cell counts between control and treatment groups were significantly different (P < 0.01). In SGF at pH 4.0, the maximum survival rate was 54.70 ± 1.11%. None of the sixty V. parahaemolyticus isolates could survive in the simulated gastric fluid (SGF) of pH 2.0 and 3.0, while 75% of isolates were still viable at pH 4.0 (Figure 2C). The frequency distribution of bacterial viability after SGF (pH = 4.0) treatment is shown in Figure 2D. SGF also induced a drastic change in the cell morphology of V. parahaemolyticus (Figure 2E). The cell morphology, observed using SEM, changed from typical smooth rod-shaped V. parahaemolyticus to shrunken spherical-shaped bacteria. These spherical-shaped bacteria, observed under TEM, showed a cytoplasm that was aggregated and which no longer had obvious functional zones.
The survival of V. parahaemolyticus in SIF is shown in Supplementary Table 4. Analysis of this data indicated that viable cell counts between control and treatment groups were significantly different (P < 0.05). In the treatment group the survival rate among the 60 strains of V. parahaemolyticus ranged from 96.16 ± 0.19% to 56.78 ± 0.81% and the frequency distribution of this bacterial viability is shown in Figure 2F. Since none of the isolates were above 100% and 52 isolates were below 90%, the bactericidal effect of SIF on V. parahaemolyticus was greater than that of simulated saliva fluid. Although no significant difference was observed between the SIF treated V. parahaemolyticus and the control group under SEM, examination of TEM images showed changes in vesicular structure, thinner cell walls, and increased cytoplasmic division (Figure 2G).
The 60 V. parahaemolyticus isolates can be classified into four different genotypes according to the type of virulence gene in the DNA, tdh-/trh-, tdh-/trh+, tdh+/trh-, and tdh+/trh+. Survival of V. parahaemolyticus of different genotypes in the 3 simulated human digestion fluids is shown in Figure 2H. The survival of tdh+/trh+ strains was significantly higher than that of the other three genotypes and the tdh-/trh- was the most intolerant in SGF.
Vibrio parahaemolyticus Virulence-Related Gene Expression in Simulated Digestion Fluids
Seven strains were selected for virulence gene expression experiment These were the VPC36 (tdh-/trh+), VPC43 (tdh+/trh+), VPC44 (tdh-/trh-), VPC47 (tdh+/trh-), VPD34 (tdh+/trh-), VPR102 (tdh-/trh+), and VPS34 (tdh-/trh-) strains. Transcription of genes evaluated in this study is strongly influenced by the digestive fluids (Figure 2I). In simulated digestive fluids, virulence-related genes were significantly up-regulated only after SIF treatment, and most of them were downregulated in SSF and SGF. Gene clusters using the UNIFRAC framework is shown in the Figure 2I. The 7 genes can be divided into two main branches. The first branch consists of vtrA and vtrB genes coding for the transcriptional regulatory proteins, VtrA, and VtrB. The second branch consists of the genes encoding T3SS2 effectors proteins, vopV, vopZ, and vopL and the toxin genes trh, tdh. This second branch genes encode proteins which are related to cytotoxin and enterotoxin production.
Effects of Aquatic Products on Vibrio parahaemolyticus in Simulated Gastric Fluids
The gastric fluid is maintained at pH 1.6 during fasting and the effect of aquatic products on gastric pH is shown in Figure 3. When the seafood was added to the gastric fluid with a pH of 1.6, the pH of the salmon (A) decreased from the initial value of 7.30 to 5.31, the pH of the oyster (B) decreased from 7.20 to 5.41, and the pH of the shrimp (C) decreased from 7.00 to 4.76. VPD58 inoculated in aquatic products showed a significant decrease in the number of viable cells in the initial 10 min, and this change in viable numbers correlates well with the change of pH value. After 2 h of treatment, viable cell of VPD58 inoculated in salmons, oysters, and shrimps were 4.30 Log10CFU/mL, 5.04 Log10CFU/mL, and 4.08 Log10CFU/mL, respectively. However, pure VPD58 bacterial suspension treated with SGF for 2 h could not be cultured (Supplementary Table 3). This result confirms that seafood matrices can significantly raise the pH level of gastric fluid and assist in V. parahaemolyticus surviving passage from the gastric fluid.
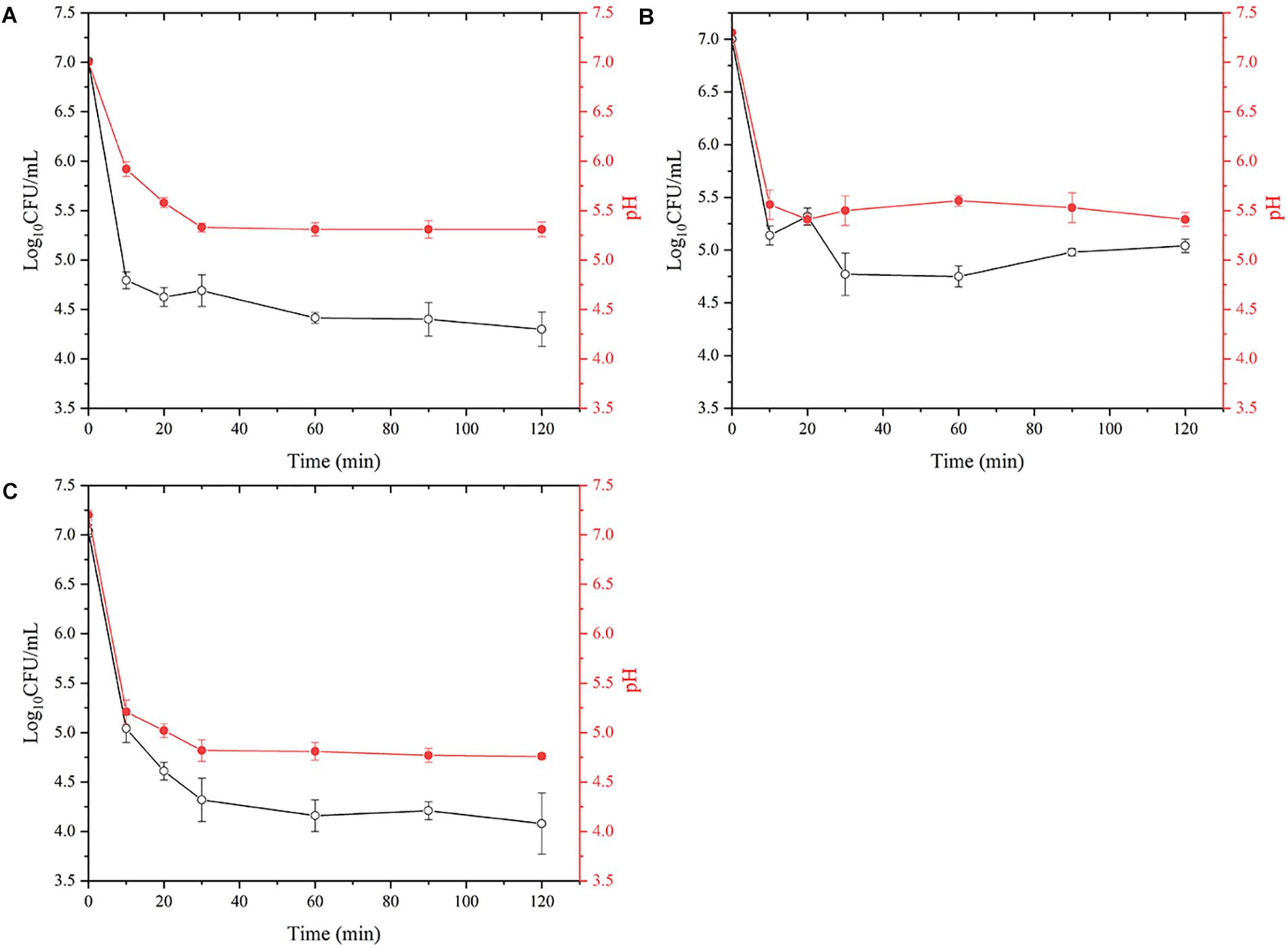
Figure 3. The effect of simulated gastric fluids treatment on the decrease of viable V. parahaemolyticus (VPD58) cells in salmon (A), oyster (B), and shrimp (C) and the change of pH.
Fate of Vibrio parahaemolyticus in an in vitro Continuous Digestion Model
The above batch experiments in SSF, SGF and SIF showed that SGF had the highest bactericidal effect on V. parahaemolyticus. However, human digestion is a continuous process and we processed the seven strains through an in vitro continuous digestion model to validate the changes to survival, morphology and virulence gene expressions (Figure 1A).
In the continuous model, the viability of V. parahaemolyticus did not change significantly after 5 min of passage, where the average cell concentration of all seven strains changed from 7.00 Log10CFU/mL to 6.75 Log10CFU/mL (Figure 4A). Observations under SEM and TEM also showed no significant difference (Figure 4B). After 125 min of treatment, the survival rate decreased significantly (p < 0.01). Average bacterial concentration of seven strains changed from 6.75 Log10CFU/mL to 3.13 Log10CFU/mL (Figure 4A). The cells were severely shrunk and the cellular contents were released (Figure 4B).
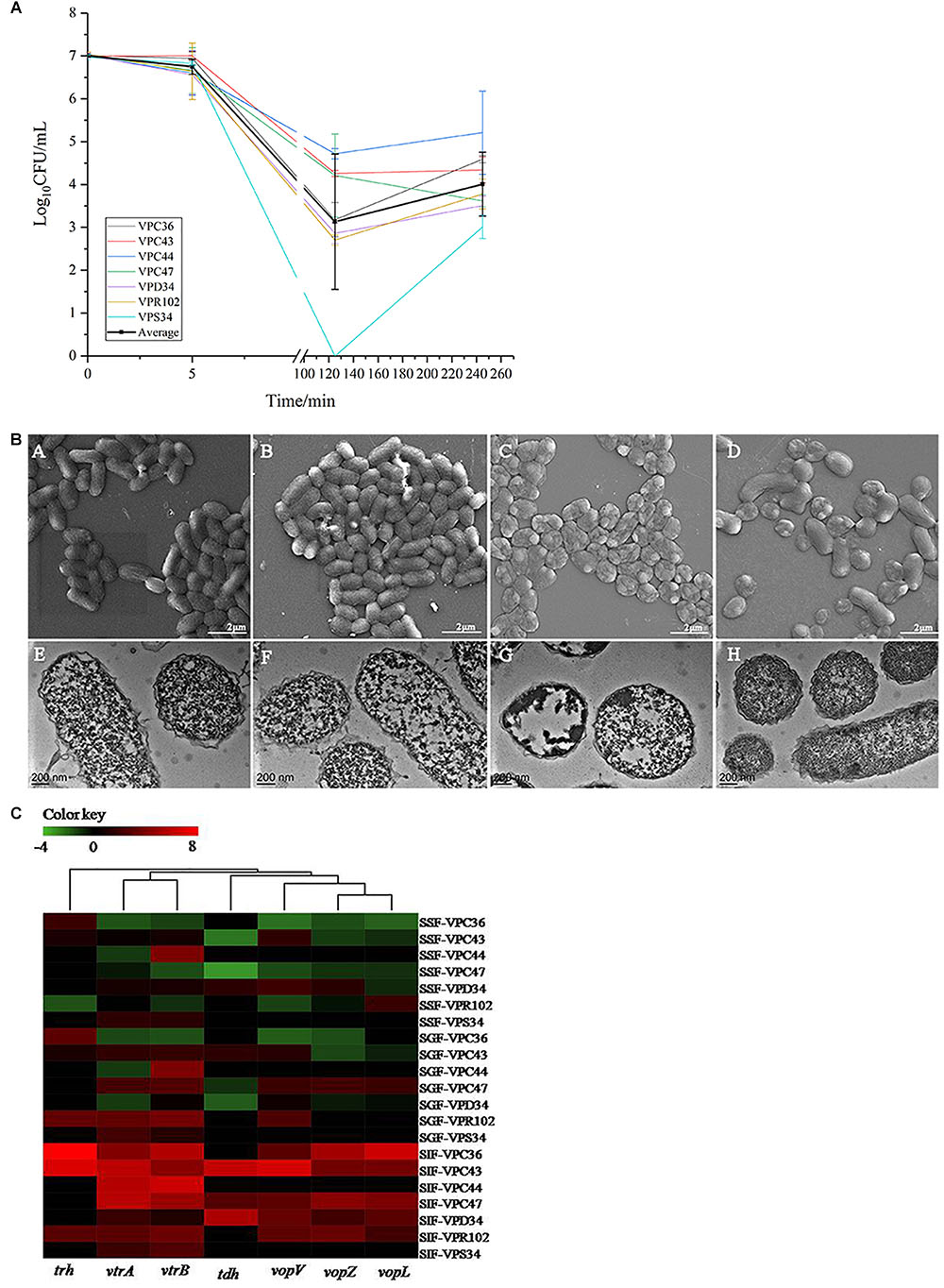
Figure 4. (A) The viable cell counts (average ± standard deviation) of V. parahaemolyticus during the in vitro static digestion model over times, black line represented for average amount. (B) Representative photomicrographs of V. parahaemolyticus in vitro static digestion model. Untreated group: SEM (A) and TEM (E), Saliva phase group: SEM (B) and TEM (F), Gastric phase group: SEM (C) and TEM (G), Intestinal phase group: SEM (D) and TEM (H), respectively. (C) Heatmap shows the relative expression of virulence-related genes in V. parahaemolyticus. The color codes represent relative expressions, ranging from green (induced expression) to red (repressed expression).
Interestingly, when V. parahaemolyticus were treated for 245 min, bacterial concentration of most isolates increased significantly (P > 0.05). Average bacterial concentration changed from 3.13 Log10CFU/mL to 4.01 Log10CFU/mL. The SEM images showed that some of the shrunk cells recovered to a smooth rod shape (Figure 4B). From the TEM pictures (Figure 4B), V. parahaemolyticus cells were distributed evenly and the cytoplasm were densely stained, which was different from than those treated with SIF in a batch system.
At the saliva phase, the overall gene expression was down-regulated where only gene vtrB in VPC47 was up-regulated 3.58 times. Interestingly, at the gastric phase, gene expression had both positive and negative fold changes, which was significantly different from the all negative fold changes shown in Figure 2I. Additionally at the gastric phase, genes were significantly up-regulated. Information of clustering using the UNIFRAC platform is shown in Figure 4C. The clustered genes are divided into two main branches, where the first branch consists of trh, vtrA and vtrB, and the second branch consists of tdh, vopV, vopZ, and vopL.
Discussion
Aquatic products are the main vectors for V. parahaemolyticus (Su and Liu, 2007) and the possible mechanism of this pathogenesis was validated in a simulated continuous digestive fluids model system which charts the dynamic changes to V. parahaemolyticus in a seafood matrix.
The most efficient barrier to survival and pathogenesis of V. parahaemolyticus was the human stomach which plays an important role in killing or inactivating foodborne pathogens. However, food entering the stomach can cause a temporary increase in the stomach pH, ranging from 1.3 to 6.0 (Jennifer et al., 1990). Additionally, Waterman and Small (1998) showed that high protein food can protect bacteria from gastric acid inactivation better than starchy foods. We confirmed this observation for acid sensitive V. parahaemolyticus in a seafood matrix.
The pH value of the intestine is around 7.0 (Michael, 2013) after gastric emptying and coupled to the body temperature of 37°C, provides an optimal growth environment for V. parahaemolyticus (Tang et al., 2015). In this study V. parahaemolyticus escaping the stomach environment was resuscitated and expression of the virulence genes was upregulated in the intestinal fluids. Intestinal fluids containing bile salts are known to bind to the VtrA/VtrC complex of V. parahaemolyticus and induce VtrB to activate T3SS2 (Li et al., 2016) and vtrA and vtrB deletion mutants cannot produce TDH and T3SS2-related proteins (Gotoh et al., 2010). VopV, VopZ, and VopL are the three main secretion effectors protein of T3SS2, which play important roles for V. parahaemolyticus pathogenicity and colonization in the intestine. Combining all the above results with findings of relevant scholars, we summarize our observation in Figure 5 (Raimondi et al., 2000; Gotoh et al., 2010; Ohnishi et al., 2011; Ham and Orth, 2012; Ritchie et al., 2012; Zhang and Orth, 2013; Li et al., 2016).
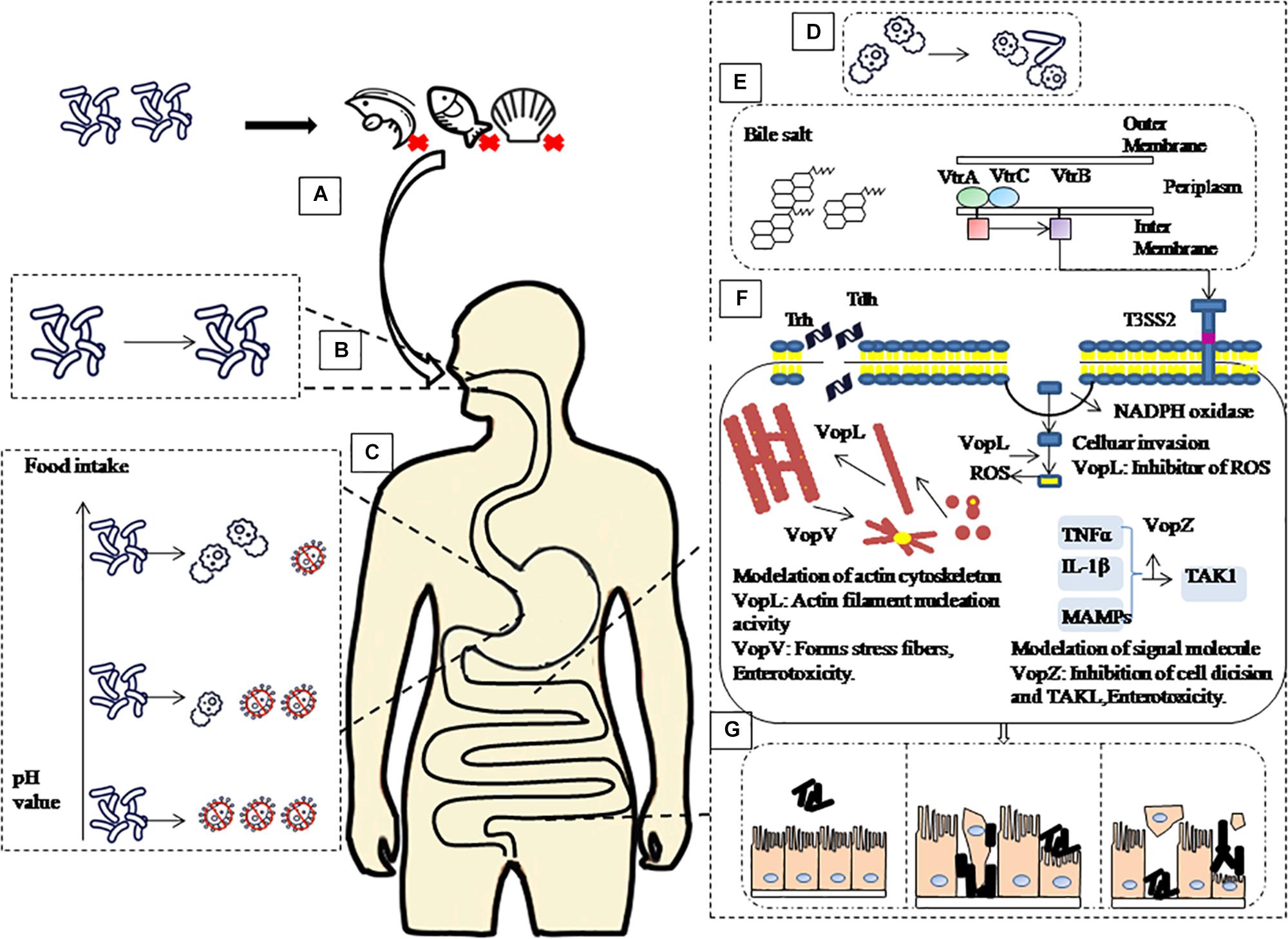
Figure 5. Schematic of pathogenic mechanism of V. parahaemolyticus through human digestive tract. (A) Contaminated aquatic products are the main source of V. parahaemolyticus infection. (B) After ingestion, V. parahaemolyticus passes through the mouth, but saliva has no significant inactivation effect on it. (C) then faces acidic conditions of the stomach before colonizing the small intestine. However, with the increase of gastric fluid pH caused by food intake, the survival rate of V. parahaemolyticus in the stomach will increase. (D) When V. parahaemolyticus reached the intestinal tract, the survival rate increased, and the expression of virulence-related genes increased significantly. (E) Under the stimulation of bile salt, VtrA/VtrC complex induced the expression of VtrB, in turn, VtrB activated T3SS2. (F) Tdh, Trh and effectors VopL, VopV, VopZ secreted by T3SS2 system infect host cells. (G) Ultimately, cause damage to intestinal epithelial cells leads to diseases such as gastroenteritis.
In this study, a significant difference was observed in survival rates of pathogenic (trh+trh+,trh+tdh-,tdh-trh+) and non-pathogenic (trh-tdh-) isolates after SGF treatment. The high survival rate of pathogenic strains in SGF indicates a positive correlation between acid resistance and pathogenicity and highlights heterogeneity in extreme environments. This heterogenous behavior of bacteria in stressful environment was studied by Brian (1998) and includes differences in virulence patterns, growth behavior, inactivation kinetics and biofilm formation capabilities. Delignette-Muller indicated that the heterogeneity of microbial strains can influence conclusions of microbial risk assessments (Delignette-muller and Rosso, 2000).
Conclusion
In conclusion, this study mainly focused on the survival, morphological changes and virulence gene expression of V. parahaemolyticus in simulated digestive fluids. An in vitro continuous digestion model was used to validate that V. parahaemolyticus contaminating aquatic products is capable of surviving passage through the oral and stomach environment to reach the intestinal tract. With the increase of gastric pH caused by food intake, V. parahaemolyticus could escape the bactericidal effect of gastric fluid, resuscitate in the intestine, and express virulence-related genes. This study indicated that seafood matrices can significantly raise the pH level of gastric fluid and thus aids the survival of V. parahaemolyticus through passage from human gastric acid and progression of pathogenesis in the intestinal fluid.
Data Availability
The raw data supporting the conclusions of this manuscript will be made available by the authors, without undue reservation, to any qualified researcher.
Author Contributions
YZ, YP, and PM conceived and supervised the study. SW, ZZ, and YP conducted the experiments. SW and ZZ analyzed the data and wrote the manuscript. ZZ, PM, YP, and YZ revised the manuscript.
Funding
This research was supported by the National Natural Science Foundation of China (Nos. 31571917 and 31671779), National Key R&D Program of China (No. 2018YFC1602205), Shanghai Agriculture Applied Technology Development Program (T20170404), Innovation Program of Shanghai Municipal Education Commission (2017-01-07-00-10-E00056), and the “Dawn” Program of Shanghai Education Commission (15SG48).
Conflict of Interest Statement
The authors declare that the research was conducted in the absence of any commercial or financial relationships that could be construed as a potential conflict of interest.
Supplementary Material
The Supplementary Material for this article can be found online at: https://www.frontiersin.org/articles/10.3389/fmicb.2019.01614/full#supplementary-material
References
Agrawal, K. R., Lucas, P. W., Prinz, J. F., and Bruce, I. C. (1997). Mechanical properties of foods responsible for resisting food breakdown in the human mouth. Arch. Oral Biol. 42, 1–9. doi: 10.1016/s0003-9969(96)00102-1
Barroso, E., Cueva, C., Peláez, C., Martínez-Cuesta, M. C., and Requena, T. (2015). Development of human colonic microbiota in the computer-controlled dynamic simulator of the gastrointestinal tract simgi. LWT Food Sci. Technol. 61, 283–289. doi: 10.1016/j.lwt.2014.12.014
Brian, L. M. (1998). Dealing with uncertainty in risk assessment. Hum. Ecol. Risk Assess. 4, 685–699. doi: 10.1080/10807039891284569
Ceccarelli, D., Hasan, N. A., Huq, A., and Colwell, R. R. (2013). Distribution and dynamics of epidemic and pandemic Vibrio parahaemolyticus virulence factors. Front. Cell. Infect. Microbiol. 3:97. doi: 10.3389/fcimb.2013.00097
Delignette-muller, M. L., and Rosso, L. (2000). Biological variability and exposure assessment. Int. J. Food Microbiol. 58, 203–212. doi: 10.1016/s0168-1605(00)00274-9
Feng, B., Guo, Z. R., Zhang, W. J., Pan, Y. J., and Zhao, Y. (2016). Metabolome response to temperature-induced virulence gene expression in two genotypes of pathogenic Vibrio parahaemolyticus. BMC Microbiol. 16:75. doi: 10.1186/s12866-016-0688-5
Gotoh, K., Kodama, T., Hiyoshi, H., Izutsu, K., Kwon-Sam, P., Dryselius, R., et al. (2010). Bile acid-induced virulence gene expression of Vibrio parahaemolyticus reveals a novel therapeutic potential for bile acid sequestrants. PLoS One 5:e13365. doi: 10.1371/journal.pone.0013365
Ham, H., and Orth, K. (2012). The role of type? secretion system 2 in Vibrio parahaemolyticus pathogenicity. J. Microbiol. 50, 719–725. doi: 10.1007/s12275-012-2550-2
Hermans, R. C. J., Larsen, J. K., Peter, H. C., and Engels, R. C. M. E. (2012). How much should I eat? situational norms affect young women’s food intake during meal time. Br. J. Nutr. 107, 588–594. doi: 10.1017/S0007114511003278
Jennifer, B. D., Rosemary, R. B., Lambros, C. D., Tanya, L. R., Stephen, P. S., Jeffrey, L. B., et al. (1990). Upper gastrointestinal (GI) pH in young, healthy men and women. Pharm. Res. 7, 756–761.
Lewis, H. B., Ahern, A. L., and Jebb, S. A. (2012). How much should I eat? a comparison of suggested portion sizes in the UK. Public Health Nutr. 15, 2110–2117. doi: 10.1017/S1368980012001097
Li, P., Rivera-Cancel, G., Kinch, L. N., Salomon, D., Tomchick, D. R., Grishin, N. V., et al. (2016). Bile salt receptor complex activates a pathogenic type? secretion system. eLife 5:e15718. doi: 10.7554/elife.15718
Livak, K. J., and Schmittgen, T. D. (2001). Analysis of relative gene expression data using real-time quantitative PCR and the 2(T)(-Delta Delta C) method. Methods 25, 402–408. doi: 10.1006/meth.2001.1262
McLaughlin, J. B., Depaola, A., Bopp, C. A., Martinek, K. A., Napolilli, N. P., Allison, C. G., et al. (2005). Outbreak of Vibrio parahaemolyticus gastroenteritis associated with alaskan oysters. N. Engl. J. Med. 353, 1463–1470.
Michael, B. (2013). Ecology and physiology of the intestinal tract. Curr. Top. Microbiol. Immunol. 358, 247–272. doi: 10.1007/82_2011_192
Minekus, M., Alminger, M., Alvito, P., Balance, S., Bohn, T., Bourlieu, C., et al. (2014). A standardised static in vitro digestion method suitable for food – an international consensus. Food Funct. 5, 1113–1124. doi: 10.1039/c3fo60702j
Molly, K., Woestyne, M., and Verstraete, W. (1993). Development of a 5-step multi-chamber reactor as a simulation of the human intestinal microbial ecosystem. Appl. Microbiol. Biotechnol. 39, 254–258. doi: 10.1007/bf00228615
Ohnishi, K., Nakahira, K., Unzai, S., Mayanagi, K., Hashimoto, H., Shiraki, K., et al. (2011). Relationship between heat-induced fibrillogenicity and hemolytic activity of thermostable direct hemolysin and a related hemolysin of Vibrio parahaemolyticus. FEMS Microbiol. Lett. 318, 10–17. doi: 10.1111/j.1574-6968.2011.02233.x
Qing, G., Ye, A., Lad, M., Dalgleish, D., and Singh, H. (2013). The breakdown properties of heat-set whey protein emulsion gels in the human mouth. Food Hydrocoll. 33, 215–224. doi: 10.1016/j.foodhyd.2013.03.008
Raimondi, F., Kao, J. P. Y., Fiorentini, C., Fabbri, A., Donelli, G., Gasparini, N., et al. (2000). Enterotoxicity and cytotoxicity of Vibrio parahaemolyticus thermostable direct hemolysin in in vitro systems. Infect. Immun. 68, 3180–3185. doi: 10.1128/iai.68.6.3180-3185.2000
Ritchie, J. M., Rui, H., Zhou, X., Iida, T., Kodoma, T., Ito, S., et al. (2012). Inflammation and disintegration of intestinal villi in an experimental model for Vibrio parahaemolyticus-induced diarrhea. PLoS Pathog. 8:e1002593. doi: 10.1371/journal.ppat.1002593
Robert, M. T., Procter, L. S., and Herbert, E. H. (1969). Morphological, cultural, biochemical, and serological comparison of Japanese strains of Vibrio parahemolyticus with related cultures isolated in the united states. J. Bacteriol. 98, 511–518.
Shimohata, T., Nakano, M., Lian, X., Shigeyama, T., Iba, H., Hamamoto, A., et al. (2011). Vibrio parahaemolyticus infection induces modulation of il-8 secretion through dual pathway via vp1680 in caco-2 cells. J. Infect. Dis. 203, 537–544. doi: 10.1093/infdis/jiq070
Su, Y. C., and Liu, C. (2007). Vibrio parahaemolyticus: a concern of seafood safety. Food Microbiol. 24, 549–558. doi: 10.1016/j.fm.2007.01.005
Takeshi, H., Yuxin, N., Atsuko, H., Myonsun, Y., Toshio, M., Tadashi, O., et al. (1990). Properties of a hemolysin related to the thermostable direct hemolysin produced by a Kanagawa phenomenon negative, clinical isolate of Vibrio parahaemolyticus. Can. J. Microbiol. 36, 395–399. doi: 10.1139/m90-069
Tang, X. Y., Zhao, Y., Sun, X. H., Xie, J., Pan, Y. J., and Malakar, P. (2015). Predictive model of Vibrio parahaemolyticus O3:K6 growth on cooked Litopenaeus vannamei. Ann. Microbiol. 65, 487–493. doi: 10.1007/s13213-014-0884-1
Wang, L., Ling, Y., Jiang, H. W., Qiu, Y. F., Qiu, J. F., Chen, H. P., et al. (2013). Apha is required for biofilm formation, motility, and virulence in pandemic Vibrio parahaemolyticus. Int. J. Food Microbiol. 160, 245–251. doi: 10.1016/j.ijfoodmicro.2012.11.004
Waterman, S. R., and Small, P. L. C. (1998). Acid-sensitive enteric pathogens are protected from killing under extremely acidic conditions of pH 2.5 when they are inoculated onto certain solid food sources. Appl. Environ. Microbiol. 64, 3882–3886.
Xie, J., Sun, X. H., Pan, Y. J., and Zhao, Y. (2012). Combining basic electrolyzed water pretreatment and mild heat greatly enhanced the efficacy of acidic electrolyzed water against vibrio parahaemolyticus on shrimp. Food Control 23, 320–324. doi: 10.1016/j.foodcont.2011.07.019
Keywords: Vibrio parahaemolyticus, digestion fluids, survival, pathogenesis, escape mechanism
Citation: Wang S, Zhang Z, Malakar PK, Pan Y and Zhao Y (2019) The Fate of Bacteria in Human Digestive Fluids: A New Perspective Into the Pathogenesis of Vibrio parahaemolyticus. Front. Microbiol. 10:1614. doi: 10.3389/fmicb.2019.01614
Received: 15 March 2019; Accepted: 28 June 2019;
Published: 16 July 2019.
Edited by:
Swapan K. Banerjee, Health Canada, CanadaReviewed by:
Ashima Kushwaha Bhardwaj, Independent Researcher, IndiaVeronica Lazar, University of Bucharest, Romania
Copyright © 2019 Wang, Zhang, Malakar, Pan and Zhao. This is an open-access article distributed under the terms of the Creative Commons Attribution License (CC BY). The use, distribution or reproduction in other forums is permitted, provided the original author(s) and the copyright owner(s) are credited and that the original publication in this journal is cited, in accordance with accepted academic practice. No use, distribution or reproduction is permitted which does not comply with these terms.
*Correspondence: Pradeep K. Malakar, pkmalakar@gmail.com; Yong Zhao, yzhao@shou.edu.cn