- 1College of Pharmacy, Fujian University of Traditional Chinese Medicine, Fuzhou, China
- 2College of Biological Science and Engineering, Fuzhou University, Fuzhou, China
- 3Centre for Drug Discovery, Satyabhama University, Chennai, India
At present, foodborne diseases (FBDs) caused by bacteria are gradually increasing every year, and the development of new antibiotics is an urgent necessity for human beings. To find novel antibacterial compounds, three sponge-derived fungal strains (SCSIOS02F40, F46, and F49) were investigated. As a result, Alternaria sp. SCSIOS02F49 was selected for investigation on its secondary metabolites because its ethyl acetate (EtOAc) extract of potato dextrose broth (PDB) culture showed rich metabolites and strong antibacterial activity. Two new dibenzopyrones with rare sulfate group (1–2), together with 10 known compounds (3–12), were isolated from the Alternaria sp. SCSIOS02F49. Their structures were confirmed by nuclear magnetic resonance (NMR), mass spectrometry (MS) data, and comparison with data from the relevant literature. Almost all compounds showed moderate inhibitory activity against eight foodborne bacteria (FBB) with minimum inhibitory concentration (MIC) values in the range of 15.6–250 μg/ml, and minimum bactericidal concentration (MBC) values in the range of 31.3–250 μg/ml. The antibacterial mechanism of compound 1 was preliminarily investigated using growth curves, scanning electron microscopy (SEM), and flow cytometry (FCM), which revealed that compound 1 altered the external structure of Staphylococcus aureus and caused the rupture or deformation of the cell membranes. This research provides lead compounds for the development of new antibiotics or microbial preservatives.
Introduction
As a growing public health problem throughout the world, foodborne diseases (FBDs) have resulted in almost 1 in 10 people being ill, leading to 420,000 deaths every year (Chandankere et al., 2020; Li et al., 2020). Among them, FBDs caused by Campylobacter pylori, Staphylococcus aureus, Listeria monocytogenes, Escherichia coli, Vibrio parahaemolyticus, Salmonella cholerae, Proteus mirabilis, Shigella flexneri, and other foodborne bacteria (FBB) have been widely reported and are of great concern (Tack et al., 2019, 2020). The most common and effective measure for the prevention of FBB was chemical control. However, the frequent use of chemical preservatives or antibiotics had an increased risk of drug resistance (Medjeldi et al., 2018; Qais et al., 2019). Therefore, the development of new agents against bacteria without risk and harmful side effects is an urgent necessity for human beings.
In recent years, marine fungi have received widespread attention because of their tremendous capacity to produce structurally unique compounds with a broad range of pharmacological properties (Radic and Strukelj, 2012; Pang et al., 2016; Fang et al., 2021; Guo et al., 2021). Over the last 5 years, discoveries of new compounds from marine fungi have outpaced discoveries from all other marine phyla. In previous studies, the most explored marine fungi were Penicillium and Aspergillus. Recently, novel compounds isolated from marine fungi, such as Acremonium, Alternaria, Pseudotrichosporon, Chaetomium, and Cladosporiosis, have been reported. Their biological activities mainly involve antibacterial, anti-inflammatory, anti-virus, anti-tumor, anti-fouling, enzyme inhibition, etc (Carroll et al., 2019, 2020, 2021).
As a continuing effort in the search for new bioactive metabolites from the endophytic fungi (Tian et al., 2015a,b, 2018a,b,c; Cong et al., 2020), three fungal strains isolated from a marine sponge were subjected to chemical [Thin layer chromatography (TLC) and high pressure liquid chromatography (HPLC)] and antibacterial activity screening. Alternaria sp. SCSIOS02F49 was then selected as the target strain for large-scale fermentation and further research because of its abundant metabolites and strong antibacterial activity. Two rare sulfate-modified dibenzopyones, Alterlactone 5′-O-sulfate (1) and 3′-hydroxyalternariol 5-O-methyl ether-3′-O-sulfate (2), together with 10 known compounds were isolated from the Alternaria sp. SCSIOS02F49 (Figure 1). The antibacterial activities of all compounds against eight FBB were evaluated, and the antibacterial mechanism of 1 was preliminarily investigated. Details of the isolation, structure elucidation, and bioactivity screening of these metabolites are reported herein.
Materials and Methods
General Experimental Procedures
The nuclear magnetic resonance (NMR) spectra were recorded on a Bruker AC 500 (600) M NMR (Bruker, Fällanden, Switzerland) spectrometer with tetramethylsilane (TMS) as an internal standard. HRESIMS data were measured on an Agilent 6550iFunnelQ-TOFLC/MS (Bruker, Fällanden, Switzerland). UV spectra were recorded on a Shimadzu UV-2600 UV-Vis spectrophotometer (Shimadzu, Kyoto, Japan). Semi-preparative reversed-phase HPLC [(SP-RP) HPLC] was performed on a YMC-Pack Pro C18 RS column (5 μm, 250 × 10 mm id; YMC, Kyoto, Japan) with a High-Performance Liquid Chromatograph Primaide equipped with a photodiode array (PDA) detector. Silica gel GF254 used for TLC was supplied by the Qingdao Marine Chemical Factory, Qingdao, China. Sephadex LH-20 gel (GE Healthcare, Uppsala, Sweden) was used. Flow cytometer (FCM) (Accuri C6 Plus, Becton, USA) and scanning electron microscope (SEM) (JSM-6700F, JEOL, Japan) were used in the research of antibacterial mechanism. Spots were detected on TLC under UV light or by heating by spraying with 12% H2SO4 in H2O.
Microbial Strains
Fungal strains were isolated from the sponge Callyspongia sp., which was collected from the sea area near Xuwen County (20.3265° N, 110.1750° E), Guangdong Province, China. The fungi SCSIOS02F40, F46, and F49 were identified as Aspergillus sp., Didymellaceae sp., and Alternaria sp., respectively, using a molecular biological protocol by DNA amplification and sequencing of the internally transcribed spacer (ITS) region (GenBank accession number KT164776, KU361223, and KU361224) (Supplementary Table 3). These three strains (MMPC NO. 5035-5037) were deposited at the Marine Microorganism Preservation Center, CAS Key Laboratory of Tropical Marine Bio-resources and Ecology, China. Bacteria strains of Staphylococcus aureus (ATCC25923), Listeria monocytogenes (CICC21633), Escherichia coli (ATCC25922), Proteus mirabilis (CMCC49005), Vibrio parahaemolyticus (ATCC17802), Pseudomonas fluorescens (ATCC13525), Salmonella choleraesuis (CICC13312), and Shigella flexneri (CMCC51571) were preserved in the Institute of Food and Marine Biological Resources, Fuzhou University.
Fermentation, Extraction, and Isolation
Strain F49 was cultured on Malt Broth-agar (MB-agar) plates at 25°C for 7 days. The seed medium (consisted of malt extract: 15 g, sea salt: 10 g, distilled water: 1,000 ml, pH: 7.4–7.8) was inoculated with strain F49 and incubated at 25°C for 72 h on a rotating shaker (170 rpm). The seed solution (10 ml) was then incubated on a rotary shaker (170 rpm) at 25°C for 15 d in 1,000 ml × 30 conical flasks containing the liquid medium (300 ml/flask) composed of 40 g of potato, 4 g of glucose, 2.5 g of sea salt, and 200 ml pure water. The fermented whole broth (9 L) was filtered through cheesecloth to separate it into filtrate and mycelia. The filtrate was concentrated under vacuum to about a quarter of the original volume and then extracted three times with EtOAc to give an EtOAc solution, while the mycelia were extracted three times with acetone. The acetone solution was evaporated under reduced pressure to afford an aqueous solution. The aqueous solution was extracted three times with EtOAc to give a residual extract. Both the EtOAc solutions were combined and concentrated under vacuum to give a final EtOAc extract (16.43 g). The EtOAc extract was subjected to silica gel column chromatography (CC) eluted with petroleum ether/EtOAc in a gradient eluent (v/v, 50:1, 20:1, 10:1, 5:1, 2:1, 1:1, 0:1) to obtain 12 fractions (fractions 1–12) on the basis of TLC. Fraction 10 (fr. 10) was subjected to Octadecylsilyl (ODS) chromatography eluted with MeOH/H2O in a gradient eluent (1:9, 2:3, 3:2, 4;1, 9:1), to give 4 subfractions (fr. 10.1–10.4). Fr. 10.2 was further purified by (SP-RP) HPLC eluting with CH3CN-H2O (15:85) to afford 1 (22.7 mg). In the same way, compounds 2 (8.4 mg) and 3 (6.4 mg) were purified from fr. 10.3 by (SP-RP) HPLC [25% methyl cyanide (MeCN), 75% H2O + 1‰ trifluoroacetic acid (TFA)]. Fr. 6 was purified by Sephadex LH-20 (CH3Cl/MeOH, 1:1) to give 3 subfractions (fr. 6.1–6.3). Fr. 6.1 was further purified on (SP-RP) HPLC by 35% MeCN to give 4 (8.4 mg), 5 (10.1 mg), and 6 (10.8 mg). Compounds 7 (9.3 mg), 8 (28.9 mg), 9 (2.3 mg), and 10 (3.7 mg) were purified from Fr. 6.2 by (SP-RP) HPLC (35% MeCN). Fr. 6.3 was further purified on (SP-RP) HPLC by 25% MeCN to give 11 (3.7 mg) and 12 (4.5 mg).
Alterlactone 5′-O-sulfate (1): Brown needle crystal; UV (MeOH) λmax (log ε) 203 (4.34), 253 (4.08), 286 (3.67) nm (Supplementary Figure 8); HRESIMS m/z 367.0126 [M - H]− (calcd for C15H12O9S, 368.01985) (Supplementary Figure 7); 1H and 13C NMR data, Table 1.
3'-hydroxyalternariol 5-O-methyl ether-3′-O-sulfate (2): Yellow amorphous solid; UV (MeOH) λmax 203 (4.48), 217 (4.42), 258 (4.55), 287 (3.94), 298 (3.90), 340 (3.89) nm (Supplementary Figure 16), HRESIMS m/z 367.0120 [M - H]− (calcd for C21H25O6, calcd for C15H12O9S, 368.01985) (Supplementary Figure 15); 1H and 13C NMR data, Table 1.
Antibacterial Bioassay
The diameter of the inhibition zone (DIZ) of compounds was evaluated according to the previously published literature (Yao et al., 2011). In short, 50 μl of compounds solutions at 500 μg/mL were injected into the small round hole (diameter of 6 mm) of LB agar medium containing bacterial suspension. After incubation at 37°C for 12 h, the DIZ was measured with a digital caliper. The minimum inhibitory concentration (MIC) of compounds was carried out with a 2-fold dilution method in a 96-well plate, and the minimum bactericidal concentration (MBC) of the compounds was determined with a suitably modified plate coating method (Wang et al., 2021). The brief process is as follows: The bacterial suspension (50 μl) and 50 μl of compounds solutions at different concentrations (the final concentration series is 0.12~250 μg/ml) were added to the 96-well plate. After 12 h of incubation at 37°C, the turbidity of the culture medium in each well was visually observed, and the final concentration of the dilution mass corresponding to the previous well, where the culture medium became turbid, was determined as the MIC value of compounds. According to the results of the MIC, 15 μl of the culture solution was aspirated and spread over the solid growth medium, which was incubated at a constant temperature in an incubator at 37°C for 24 h. The final concentration of the diluted mass is regarded as the MBC value of the test compounds. The positive control was tetracycline, and the blank group was a solvent that dissolves the samples.
Antibacterial Mechanism
Growth Curves Analysis
The growth curves of 1 against S. aureus were measured using the reported method (Lin et al., 2021). The bacterial suspension (1 × 106 CFU/ml) and LB medium containing the tested compounds were mixed at ultimate concentrations of 0.5 × MIC, 1.0 × MIC, and 2.0 × MIC into each well on a 96-well plate. After cultivating at 37°C, bacterial growth was monitored by the absorbance at 600 nm with the microplate reader, and OD600 nm values were acquired at selected time points (0, 1, 2, 3, 4, 5, 6, 7, 8, 9, 10, 11, 12, and 24 h).
SEM Analysis
Scanning electron microscopy was performed according to the method of a previously published manuscript (Lin et al., 2021). The logarithmic S. aureus was washed two times with polybutylene succinate (PBS) (pH 7.4, 0.1 M), then resuspended to a concentration of about 1 × 107 CFU/ml. The bacterial suspension was added into the medium containing 1.0 × MIC and 2.0 × MIC tested compounds and incubated at 37°C and 180 rpm for 6 h. The culture was centrifugated (3,000 rpm, 10 min), washed with 0.1 M PBS three times, and stabilized with 2.5% glutaraldehyde overnight at 4°C in the dark. Samples were dehydrated with gradient concentration (30, 40, 50%) ethanol, covered on a silicon wafer (5 mm × 5 mm), and dried naturally at room temperature. After spraying gold, the samples were observed and analyzed by SEM.
FCM Analysis
Flow cytometry analysis was conducted following the methodology of the literature (Du et al., 2018) with slight modifications. The logarithmic S. aureus (1 × 107 CFU/ml) was added into the medium containing 0.5 × MIC, 1.0 × MIC, 2.0 × MIC, and 4.0 × MIC tested compounds. All cultures (sample group and control group) were incubated at 37°C and 180 rpm for 2 h. The culture of 1 ml was washed with 0.1 M PBS (pH 7.4) and added with 2 μl of propidium iodide (PI) at room temperature in the dark for more than 40 min. Finally, the FCM was used for detection and analysis.
Results
Selection of Target Strain
Chemical screening (TLC and HPLC) and antibacterial activity screening were used to screen the three marine fungal strains, F40, F46, and F49 (Figure 2A). TLC analysis and HPLC fingerprint analysis (Figure 2B) showed that the EtOAc extract of potato dextrose broth (PDB) culture of F49 contained abundant and moderately polar secondary metabolites. Meanwhile, the results of antibacterial activity screening (Figure 2C) showed that the EtOAc extract of PDB culture of F49 (500 μg/ml) showed strong activity against three FBB (E. coli, S. aureus, and V. parahaemolyticus). Therefore, F49 was selected as the target strain for large-scale fermentation and further research, because of its abundant metabolites and strong antibacterial activity.
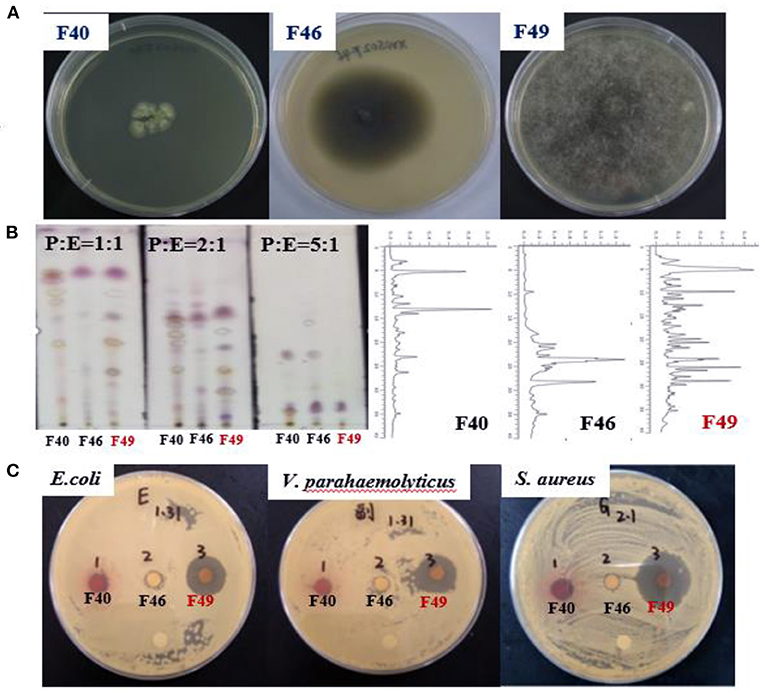
Figure 2. Selection of the target strain. (A) Three strains; (B) Chemical screening [Thin layer chromatography (TLC) and high pressure liquid chromatography (HPLC)]; (C) Antibacterial activity screening.
Structure Identification
Compound 1 was obtained as a brown needle crystal. The molecular formula C15H12O9S was determined by its HRESIMS at m/z 367.0126 [M–H]−, indicating 10 degrees of unsaturation (Supplementary Figure 7). The 1H NMR spectrum showed presence of one methoxy [δH 3.83 (s, H3-8)], one oxygenated methylene [δH 4.92 (d, J = 10.9 Hz, H-7'a), 4.90 (d, J = 10.9 Hz, H-7′b)], four olefinic protons [δH 6.54 (d, J = 2.5 Hz, H-4), 6.46 (d, J = 2.5 Hz, H-6), 7.03 (s, H-3'), 7.56 (s, H-6′)], and three exchangeable protons (δH 10.11, 11.96, 4.14) (Supplementary Figure 1). Analysis of the 13C NMR, DEPT, and HSQC spectroscopic data of 1 revealed 15 carbon signals, involving one methoxy (δC 55.9, CH3-8) and one methylene (δC 68.1, CH2-7′), four olefinic methines (δC 101.1, CH-4; δC 105.8, CH-6; δC 117.3, CH-3′; δC 123.0, CH-6'), four oxygenated quaternary carbons (δC 160.8, C-3; δC 162.9, C-5; δC 150.5, C-4'; δC 142.5, C-5′), one carboxyl carbon (δC 169.1, C-7), and four olefinic quaternary carbons (δC 140.1, C-1; δC 109.7, C-2; δC 130.1, C-1', δC 132.1, C-2′) (Table 1 and Supplementary Figures 1–4). The HMBC correlations of H2-7' (4.92, d, J = 10.9 Hz; 4.90, d, J = 10.9 Hz)/C-7 (δC 169.1), C-1′ (δC 130.1), C-2' (δC 132.1), and C-3′ (δC 117.3) indicated that the lactone ring (B ring) in 1 was not a common six-membered ring, but instead was a rare seven-membered ring, which formed through a hydroxylated methyl group (Table 1, Figure 3, and Supplementary Figure 5). These signals were closely related to those of alterlactone (Aly et al., 2008), except that the chemical shift of C-5′ in 1 appeared at a lower field (δC 142.5) than in alterlactone (δC 146.6). Combining MS, HMBC, and NOESY spectrum, it was easy to speculate a sulfate substituent at C-5′ in 1 (Figure 3 and Supplementary Figures 5–7). Thus, 1 was elucidated and named alterlactone 5′-O-sulfate.
Compound 2 possessed the same molecular formula as 1 (m/z 367.0126 [M–H]−, C15H12O9S). Comprehensive analysis of the 1H, 13C NMR, DEPT, and HSQC spectra revealed the presence of one methyl [δH/δC 2.73 (s)/25.2], one methoxy [δH/δC 3.92 (s)/56.3], three olefinic methines [δH/δC 6.66 (d, J = 2.0 Hz)/100.1; 7.27 (d, J = 2.0 Hz)/104.1; 6.85 (s)/118.8], ten quaternary carbons (δC 98.9, 110.5, 127.5, 133.7, 138.2, 145.9, 151.4, 164.6, 164.8, 166.7), and two exchangeable protons (δH 11.83, 9.70) (Table 1 and Supplementary Figures 9–12). These signals were closely related to those of 3′-hydroxyalternariol 5-O-methyl ether (3) (Supplementary Table 1 and Supplementary Figures 17, 18), except for the chemical shift change of the C ring, and the absence of an OH, which suggested that sulfate was located at C-3′ or C-4′ in 2. The strong HMBC correlations of H3-7′/C-1′, C-5′, and C-6′ (δC 133.7), and H-5′/C-1′, C-3′, and C-4′ (Figure 3 and Supplementary Figure 13), and NOESY correlations between H3-7′ and H-6, H-5′ indicated that CH3-7′ was located at C-6′ (Figure 3 and Supplementary Figure 14). The substitution of hydroxyl at C-4′ and sulfate at C-3′ was deduced by the HMBC correlations of OH-4′ (δC 9.70) to C-3′(δC 127.5), C-4′, and C-5′ (Figure 3 and Supplementary Figure 13). Further detailed analysis of MS, HMBC, and NOESY spectrum (Figure 3 and Supplementary Figures 13–15) proved that 2 was a new compound and named it 3′-hydroxyalternariol 5-O-methyl ether-3′-O-sulfate.
The remaining 10 known compounds were identified as 3′-hydroxyalternariol 5-O-methyl ether (3), alternariol (4), alternariol-5-O-methyl ether (5), altenusiol (6), isoaltenuene (7), altenuene (8), altenusin (9), 5′-methoxy-6-methyl-biphenyl-3,4,3′-triol (10), dihydroalterperylenol (11), and alterperylenol (12), by comparison of their NMR, MS, and specific rotation data with those described in the previously published manuscript (Shigemori et al., 1998; Aly et al., 2008; Zhang et al., 2012; Kim et al., 2014; Hildebrand et al., 2015).
Antibacterial Activity
The isolated compounds (1–12) were assessed in vitro for antibacterial activity against eight FBB, including six Gram-negative bacteria (E. coli, P. mirabilis, V. parahaemolyticus, P. fluorescens, S. choleraesuis, and S. flexneri) and two Gram-positive bacteria (S. aureus and L. monocytogenes). As shown in Table 2, Supplementary Figure 2, and Supplementary Figure 19, all compounds showed moderate antibacterial activity, with MIC values ranging from 15.63 to 125 μg/ml and MBC values ranging from 31.25 to 125 μg/ml. In terms of inhibiting Gram-positive bacteria, these compounds were far weaker than the tetracycline (positive control). However, they were close in terms of inhibiting and eliminating Gram-negative bacteria. Compounds 1 and 2 containing rare sulfate groups showed similar activity to analogues, indicating that sulfate groups or hydroxyl groups may not be necessary functional groups for antibacterial agents. This conclusion also provides a new idea for the subsequent research and development of this framework with low toxicity and high-efficiency antibiotics or preservatives, because it has been reported in the previous literature that quinolone can increase the toxicity of the compound (Shi et al., 2019).
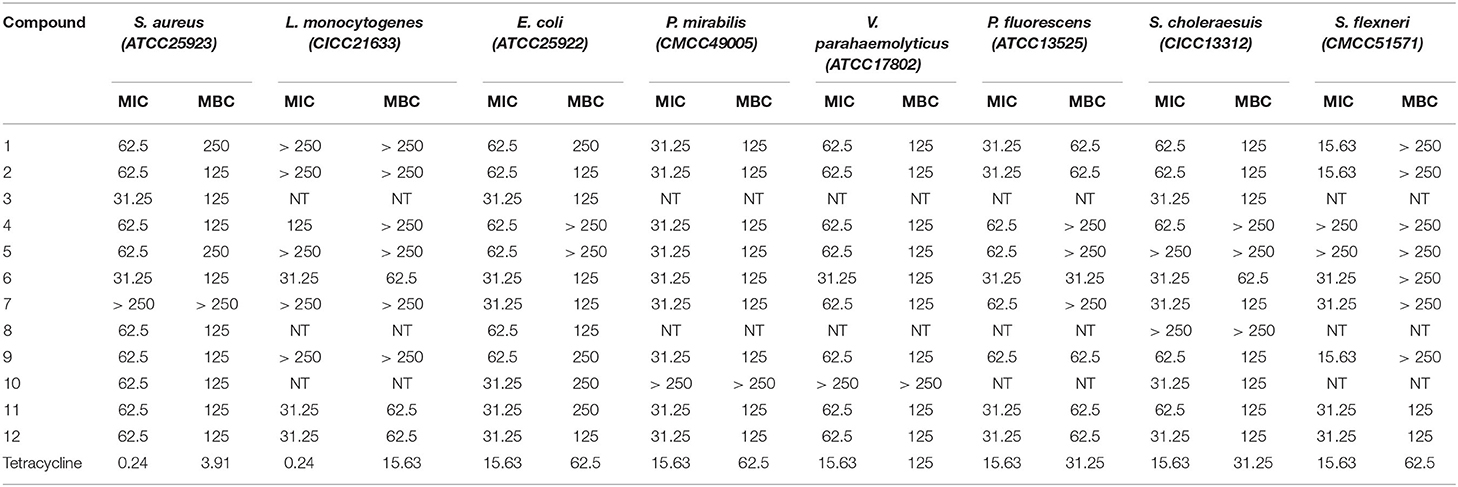
Table 2. The antibacterial activity of compounds 1–12 (MIC and MBC in μg/ml, tetracycline used as a positive control, “NT” means No Test).
Antibacterial Mechanism
Growth Curve Analysis
The growth curves of bacteria can reflect the effect of compounds on the growth of tested bacteria indirectly. According to the results of antibacterial assays, S. aureus was chosen to further investigate the growth curves of 1 at different concentrations (0.5 × MIC, 1.0 × MIC, and 2.0 × MIC). It can be seen that under the negative control and 0.5 × MIC treatment groups, the growth of S. aureus entered the logarithmic growth period after 1 h, and the number of bacterial colonies kept increasing within 24 h. When treated with 1.0 × MIC and 2.0 × MIC, the growth of S. aureus was almost completely stagnant, and there was no logarithmic period, which indicated that the bacteria were completely inhibited or even killed at the beginning of the drug treatment (Figure 4). The results showed that 1 exhibited very effective antibacterial activity against S. aureus, and its antibacterial effect was dose-dependent.
SEM Images of Bacteria Morphologies
Scanning electron microscopy was used to observe the morphological change of S. aureus after treatment by 1. In Figure 5A, the control bacteria cells preserved a typical staphylococcal arrangement structure, complete, smooth, and round cell surface, without obvious damage. In contrast, the cells of the 1.0 × MIC and 2.0 × MIC treatment groups were rough, wrinkled, irregular, and even with holes on the cell surface (Figures 5B,C). These observations indicated that the possible bacteriostatic mechanisms of 1 were to damage the cell membrane, thereby increasing its permeability.
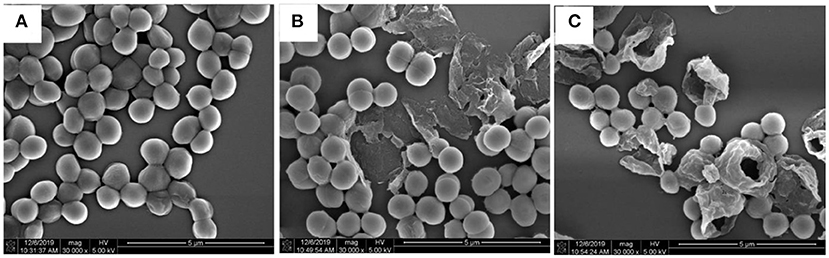
Figure 5. Scanning electron microscopy (SEM) images of S. aureus cells (× 30,000-fold). (A) Untreated S. aureus; (B) Treated S. aureus by compound 1 at 1.0 × MIC; (C) Treated S. aureus by compound 1 at 2.0 × MIC.
FCM Analysis
Propidium iodide is a kind of nucleic acid fluorescent dye, which cannot penetrate the intact cell membrane. However, if the cell membrane structure is destroyed and the membrane permeability increases, PI can bind to DNA nonspecifically. Therefore, the uptake of PI by cells can reflect the changes in cell membrane permeability indirectly. As shown in Figure 6A, the ratio of stained cells was only 21.4% without treatment. After incubation of S. aureus with 1 at different concentrations (0.5 × MIC, 1 × MIC, 2 × MIC, and 4 × MIC), the uptake amount of PI increased markedly and stained cells reached 34.5, 57.5, 98.8, and 96.4%, respectively (Figures 6B–E). These results showed that the cell membrane permeability of S. aureus was significantly changed by 1 in a dose-dependent manner.
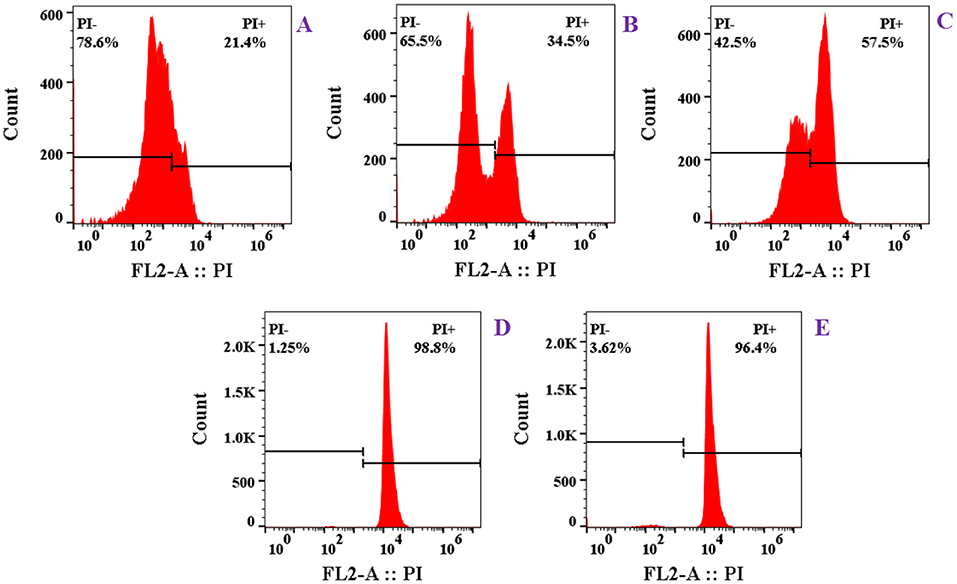
Figure 6. Effect of compound 1 on cell membrane permeability of S. aureus by flow cytometry (FCM) analysis: (A) control; (B) 0.5 × MIC; (C) 1.0 × MIC; (D) 2.0 × MIC; (E) 4.0 × MIC.
Conclusion
Infectious diseases caused by FBB are increasing every year, and it is very necessary to develop new antibacterial drugs. The secondary metabolites of marine fungi are a treasure trove of antibacterial drugs due to their novel structures, rich biological activity, and large output. In this study, three marine fungi, F40, F46, and F49, as the research object, were screened chemically and antibacterially. As a result, the Alternaria F49 was selected for further separation because its PDB fermentation product exhibited chemical richness and strong antibacterial activity at a concentration of 500 μg/ml.
The genus Alternaria is widely distributed in plants, soil, and marine environments. It can produce abundant secondary metabolites, such as biphenyls (altenusin), dibenzopyones (alternariol, alternariol-9-methyl ether, isoaltenuene, altenuene), perylene quinones (dihydroalterperylenol, alterperylenol), and other conjugated compounds (alternariol-3-glucoside, alternariol-3-sulfate) (Puntscher et al., 2019). In addition to the well-known toxicity, the pharmacological activities of these compounds have also attracted the attention of pharmacists and chemists. Altenusin displayed remarkable neuroprotective effects and could prevent tau fibrillization in vitro (Chua et al., 2017; Hou et al., 2021). Alterperylenol exhibited strong antibacterial activity (MIC of 1.95 μg/ml) (Zhao et al., 2018). In summary, two new sulfate-modified dibenzopyrones (1, 2) and 10 known compounds (3–12) were isolated from the screened marine fungus Alternaria sp. SCSIOS02F49. It is worth mentioning that almost all the compounds showed a broad spectrum against Gram negative and positive bacteria. At the same time, it was speculated that there is no obvious correlation between the substitution of sulfate and the antibacterial activity of compounds. Further, antibacterial mechanisms indicated that 1 could enhance the permeability of bacterial cell membranes. Our findings provided lead compounds for the development of low toxicity and high-efficiency antibiotics or microbial preservatives.
Data Availability Statement
The original contributions presented in the study are included in the article/Supplementary Material, further inquiries can be directed to the corresponding authors.
Author Contributions
YC conceived and designed the research, determined the structures, and wrote the manuscript. CL isolated the compound and tested the antibacterial activity. KK and LN provided the modification of this manuscript. YT conceived of and proposed the idea. All authors read and approved the manuscript and agreed to publish the manuscript.
Funding
This work was supported by National Natural Science Foundation of China (42006094), the Education and Research Projects for Young and Middle-aged Teachers of Fujian Educational Department (JAT190237), and the School Fund of Fujian University of Traditional Chinese Medicine (No. X 2019007).
Conflict of Interest
The authors declare that the research was conducted in the absence of any commercial or financial relationships that could be construed as a potential conflict of interest.
Publisher's Note
All claims expressed in this article are solely those of the authors and do not necessarily represent those of their affiliated organizations, or those of the publisher, the editors and the reviewers. Any product that may be evaluated in this article, or claim that may be made by its manufacturer, is not guaranteed or endorsed by the publisher.
Supplementary Material
The Supplementary Material for this article can be found online at: https://www.frontiersin.org/articles/10.3389/fmicb.2022.879674/full#supplementary-material
References
Aly, A. H., Edrada-Ebel, R., Indriani, I. D., Wray, V., Muller, W. E. G., Totzke, F., et al. (2008). Cytotoxic metabolites from the fungal endophyte Alternaria sp. and their subsequent detection in its host plant Polygonum senegalense. J. Nat. Prod. 71, 972–980. doi: 10.1021/np070447m
Carroll, A. R., Copp, B. R., Davis, R. A., Keyzers, R. A., and Prinsep, M. R. (2019). Marine natural products. Nat. Prod. Rep. 36, 122–173. doi: 10.1039/C8NP00092A
Carroll, A. R., Copp, B. R., Davis, R. A., Keyzers, R. A., and Prinsep, M. R. (2020). Marine natural products. Nat. Prod. Rep. 37, 175–223. doi: 10.1039/C9NP00069K
Carroll, A. R., Copp, B. R., Davis, R. A., Keyzers, R. A., and Prinsep, M. R. (2021). Marine natural products. Nat. Prod. Rep. 38, 362–413. doi: 10.1039/D0NP00089B
Chandankere, R., Ravikumar, Y., Zabed, H. M., Sabapathy, P. C., Yun, J. H., Zhang, G. Y., et al. (2020). Conversion of agroindustrial wastes to rhamnolipid by enterobacter sp. UJS-RC and its role against biofilm-forming foodborne pathogens. J. Agric. Food Chem. 68, 15478–15489. doi: 10.1021/acs.jafc.0c05028
Chua, S. W., Cornejo, A., van Eersel, J., Stevens, C. H., Vaca, I., Cueto, M., et al. (2017). The polyphenol altenusin inhibits in vitro fibrillization of tau and reduces induced tau pathology in primary neurons. Acs Chem. Neurosci. 8, 743–751. doi: 10.1021/acschemneuro.6b00433
Cong, B. L., Yin, X. F., Deng, A. F., Shen, J. H., Tian, Y. Q., Wang, S. Y., et al. (2020). Diversity of cultivable microbes from soil of the fildes peninsula, antarctica, and their potential application. Front. Microbiol. 11:570836. doi: 10.3389/fmicb.2020.570836
Du, H. C., Yang, J., Lu, X. H., Lu, Z. X., Bie, X. M., Zhao, H. Z., et al. (2018). Purification, characterization, and mode of action of plantaricin GZ1-27, a novel bacteriocin against Bacillus cereus. J. Agric. Food Chem. 66, 4716–4724. doi: 10.1021/acs.jafc.8b01124
Fang, S. T., Liu, X. H., Yan, B. F., Miao, F. P., Yin, X. L., Li, W. Z., et al. (2021). Terpenoids from the Marine-Derived Fungus Aspergillus sp. RR-YLW-12, Associated with the Red Alga Rhodomela confervoides. J. Nat. Prod. 84, 1763–1771. doi: 10.1021/acs.jnatprod.1c00021
Guo, X., Meng, Q., Niu, S., Liu, J., Guo, X., Sun, Z., et al. (2021). Epigenetic manipulation to trigger production of guaiane-type sesquiterpenes from a marine-derived spiromastix sp. fungus with antineuroinflammatory effects. J. Nat. Prod. 84, 1993–2003. doi: 10.1021/acs.jnatprod.1c00293
Hildebrand, A. A., Kohn, B. N., Pfeiffer, E., Wefers, D., Metzler, M., and Bunzel, M. (2015). Conjugation of the mycotoxins alternariol and alternariol monomethyl ether in tobacco suspension cells. J. Agricul. Food Chem. 63, 4728–4736. doi: 10.1021/acs.jafc.5b00806
Hou, Y. A., Li, J., Wu, J. C., Wu, Q. X., and Fang, J. G. (2021). Activation of cellular antioxidant defense system by naturally occurring dibenzopyrone derivatives confers neuroprotection against oxidative insults. ACS Chem. Neurosci. 12, 2798–2809. doi: 10.1021/acschemneuro.1c00023
Kim, N., Sohn, M. J., Koshino, H., Kim, E. H., and Kim, W. G. (2014). Verrulactone C with an unprecedented dispiro skeleton, a new inhibitor of Staphylococcus aureus enoyl-ACP reductase, from Penicillium verruculosum F375. Bioorganic Med. Chem. Lett. 24, 83–86. doi: 10.1016/j.bmcl.2013.11.071
Li, X., Tan, C. P., Liu, Y. F., and Xu, Y. J. (2020). Interactions between Food Hazards and Intestinal Barrier: impact on foodborne diseases. J. Agricul. Food Chem. 68, 14728–14738. doi: 10.1021/acs.jafc.0c07378
Lin, L. B., Gao, Y. Q., Han, R., Xiao, J., Wang, Y. M., Zhang, Q., et al. (2021). Alkylated salicylaldehydes and prenylated indole alkaloids from the endolichenic fungus aspergillus chevalieri and their bioactivities. J. Agric. Food Chem. 69, 6524–6534. doi: 10.1021/acs.jafc.1c01148
Medjeldi, S., Bouslama, L., Benabdallah, A., Essid, R., Haou, S., and Elkahoui, S. (2018). Biological activities, and phytocompounds of northwest Algeria Ajuga iva (L) extracts: partial identification of the antibacterial fraction. Microb. Pathog. 121, 173–178. doi: 10.1016/j.micpath.2018.05.022
Pang, K. L., Overy, D. P., Jones, E. B. G., Calado, M. D., Burgaud, G., Walker, A. K., et al. (2016). 'Marine fungi' and 'marine-derived fungi' in natural product chemistry research: toward a new consensual definition. Fungal Biol. Rev. 30, 163–175. doi: 10.1016/j.fbr.2016.08.001
Puntscher, H., Hankele, S., Tillmann, K., Attakpah, E., Braun, D., Kutt, M. L., et al. (2019). First insights into Alternaria multi-toxin in vivo metabolism. Toxicol. Lett. 301, 168–178. doi: 10.1016/j.toxlet.2018.10.006
Qais, F. A., Khan, M. S., and Ahmad, I. (2019). Broad-spectrum quorum sensing and biofilm inhibition by green tea against gram-negative pathogenic bacteria: Deciphering the role of phytocompounds through molecular modelling. Microb. Pathog. 126, 379–392. doi: 10.1016/j.micpath.2018.11.030
Radic, N., and Strukelj, B. (2012). Endophytic fungi-The treasure chest of antibacterial substances. Phytomedicine 19, 1270–1284. doi: 10.1016/j.phymed.2012.09.007
Shi, Y. N., Pusch, S., Shi, Y. M., Richter, C., Macia-Vicente, J. G., Schwalbe, H., et al. (2019). (+/-)-Alternarlactones A and B, two antiparasitic alternariol-like dimers from the fungus Alternaria alternata P1210 isolated from the halophyte salicornia sp. J. Organic Chem. 84, 11203–11209. doi: 10.1021/acs.joc.9b01229
Shigemori, H., Tenma, M., Shimazaki, K., and Kobayashi, J. (1998). Three new metabolites from the marine yeast Aureobasidium pullulans. J. Nat. Products 61, 696–698. doi: 10.1021/np980011u
Tack, D. M., Marder, E. P., Griffin, P. M., Cieslak, P. R., Dunn, J., Hurd, S., et al. (2019). Preliminary incidence and trends of infections with pathogens transmitted commonly through food - foodborne diseases active surveillance network, 10 U.S. Sites, 2015–2018. MMWR Morb Mortal Wkly Rep. 68, 369–373. doi: 10.15585/mmwr.mm6816a2
Tack, D. M., Ray, L., Griffin, P. M., Cieslak, P. R., Dunn, J., Rissman, T., et al. (2020). Preliminary incidence and trends of infections with pathogens transmitted commonly through food - foodborne diseases active surveillance network, 10 U.S. Sites, 2016–2019. MMWR Morb. Mortal Wkly Rep. 69, 509–514. doi: 10.15585/mmwr.mm6917a1
Tian, Y. Q., Lin, S. N., Zhou, H., Lin, S. T., Wang, S. Y., and Liu, Y. H. (2018a). Protuboxepin C and protuboxepin D from the sponge-derived fungus Aspergillus sp SCSIO XWS02F40. Nat. Prod. Res. 32, 2510–2515. doi: 10.1080/14786419.2017.1423303
Tian, Y. Q., Lin, S. T., Kumaravel, K., Zhou, H., Wang, S. Y., and Liu, Y. H. (2018b). Polyketide-derived metabolites from the sponge-derived fungus Aspergillus sp F40. Phytochem. Lett. 27, 74–77. doi: 10.1016/j.phytol.2018.06.009
Tian, Y. Q., Lin, X. P., Liu, J., Kaliyaperumal, K., Ai, W., Ju, Z. R., et al. (2015a). Ascomycotin A, a new citromycetin analogue produced by Ascomycota sp. Ind19F07 isolated from deep sea sediment. Nat. Prod. Res. 29, 820–826. doi: 10.1080/14786419.2014.988620
Tian, Y. Q., Lin, X. P., Wang, Z., Zhou, X. F., Qin, X. C., Kaliyaperumal, K., et al. (2015b). Asteltoxins with antiviral activities from the marine sponge-derived fungus aspergillus sp. SCSIO XWS02F40. Molecules 21:E34. doi: 10.3390/molecules21010034
Tian, Y. Q., Lin, X. P., Zhou, X. F., and Liu, Y. H. (2018c). Phenol derivatives from the sponge-derived fungus didymellaceae sp SCSIO F46. Front. Chem. 6:536. doi: 10.3389/fchem.2018.00536
Wang, Z. J., Jin, D. N., Zhou, Y., Sang, X. Y., Zhu, Y. Y., He, Y. J., et al. (2021). Bioactivity ingredients of chaenomeles speciosa against microbes: characterization by LC-MS and activity evaluation. J. Agricult. Food Chem. 69, 4686–4696. doi: 10.1021/acs.jafc.1c00298
Yao, W. R., Wang, H. Y., Wang, S. T., Sun, S. L., Zhou, J., and Luan, Y. Y. (2011). Assessment of the antibacterial activity and the antidiarrheal function of flavonoids from bayberry fruit. J. Agric Food Chem. 59, 5312–5317. doi: 10.1021/jf200211m
Zhang, S. Y., Li, Z. L., Bai, J., Wang, Y., Zhang, L. M., Wu, X., et al. (2012). A new perylenequinone from a halotolerant fungus, Alternaria sp M6. Chinese J. Natural Med. 10, 68–71. doi: 10.1016/S1875-5364(12)60015-6
Keywords: sponge-derived fungi, Alternaria sp., sulfate-modified dibenzopyrones, anti-foodborne bacteria activity, antibacterial mechanism
Citation: Chen Y, Liu C, Kumaravel K, Nan L and Tian Y (2022) Two New Sulfate-Modified Dibenzopyrones With Anti-foodborne Bacteria Activity From Sponge-Derived Fungus Alternaria sp. SCSIOS02F49. Front. Microbiol. 13:879674. doi: 10.3389/fmicb.2022.879674
Received: 20 February 2022; Accepted: 30 March 2022;
Published: 04 May 2022.
Edited by:
Jinwei Zhang, University of Exeter, United KingdomReviewed by:
Weaam Ebrahim, Mansoura University, EgyptAshraf Kariminik, Islamic Azad University Kerman, Iran
Copyright © 2022 Chen, Liu, Kumaravel, Nan and Tian. This is an open-access article distributed under the terms of the Creative Commons Attribution License (CC BY). The use, distribution or reproduction in other forums is permitted, provided the original author(s) and the copyright owner(s) are credited and that the original publication in this journal is cited, in accordance with accepted academic practice. No use, distribution or reproduction is permitted which does not comply with these terms.
*Correspondence: Lihong Nan, nlhong1152@163.com; Yongqi Tian, tianyongqi@fzu.edu.cn