- 1Center for Veterinary Medicine, U.S. Food and Drug Administration, Laurel, MD, United States
- 2School of Veterinary Medicine, University of California, Davis, Davis, CA, United States
- 3Acute Disease Epidemiology Section, Georgia Department of Public Health, Atlanta, GA, United States
- 4Department of Diagnostic Medicine/Pathobiology, College of Veterinary Medicine, Kansas State University, Manhattan, KS, United States
- 5Missouri State Public Health Laboratory, Jefferson City, MO, United States
- 6Wadsworth Center Division of Infectious Diseases, New York State Department of Health, Albany, NY, United States
- 7Division of Infectious Disease Epidemiology, Pennsylvania Department of Health, Harrisburg, PA, United States
- 8Animal Disease Research and Diagnostic Laboratory, Veterinary and Biomedical Sciences Department, South Dakota State University, Brookings, SD, United States
In 2019, the United States National Antimicrobial Resistance Monitoring System (NARMS) surveyed raw salmon, shrimp, and tilapia from retail grocery outlets in eight states to assess the prevalence of bacterial contamination and antimicrobial resistance (AMR) in the isolates. Prevalence of the targeted bacterial genera ranged among the commodities: Salmonella (0%–0.4%), Aeromonas (19%–26%), Vibrio (7%–43%), Pseudomonas aeruginosa (0.8%–2.3%), Staphylococcus (23%–30%), and Enterococcus (39%–66%). Shrimp had the highest odds (OR: 2.8, CI: 2.0–3.9) of being contaminated with at least one species of these bacteria, as were seafood sourced from Asia vs. North America (OR: 2.7; CI: 1.8–4.7) and Latin America and the Caribbean vs. North America (OR: 1.6; CI: 1.1–2.3) and seafood sold at the counter vs. sold frozen (OR: 2.1; CI: 1.6–2.9). Isolates exhibited pan-susceptibility (Salmonella and P. aeruginosa) or low prevalence of resistance (<10%) to most antimicrobials tested, with few exceptions. Seafood marketed as farm-raised had lower odds of contamination with antimicrobial resistant bacteria compared to wild-caught seafood (OR: 0.4, CI: 0.2–0.7). Antimicrobial resistance genes (ARGs) were detected for various classes of medically important antimicrobials. Clinically relevant ARGs included carbapenemases (blaIMI-2, blaNDM-1) and extended spectrum β-lactamases (ESBLs; blaCTX-M-55). This population-scale study of AMR in seafood sold in the United States provided the basis for NARMS seafood monitoring, which began in 2020.
Highlights
– The prevalence of seafood contamination with resistant bacteria was low.
– Carbapenemases (blaIMI-2, blaNDM-1) and ESBLs (blaCTX-M-55) were found in imported seafood.
– This work provides the basis for national tracking of seafood-borne AMR in the United States.
Introduction
An increasing number of Americans are consuming seafood. In 2019, the United States per capita annual seafood consumption reached 19.2 pounds (National Marine Fisheries Service, 2021), an increase of 1.5 pounds compared to per capita consumption in 2010. While lower than beef (57.9 pounds per capita in the United States in 2019) and chicken (95.1 pounds per capita in the United States in 2019; U.S. Department of Agriculture Economic Research Service, 2021), consumption of seafood is expected to increase. This trend is similar to that worldwide, reflecting rising incomes, transitions in dietary preferences and nutrition guidelines, and associated increases in production (Schar et al., 2020; U.S. Food and Drug Administration, 2020a). As of 2019, shrimp is the most consumed seafood product in the United States (4.7 pounds per capita), followed by salmon (3.1 pounds per capita), canned tuna (2.2 pounds per capita), Alaska pollock (0.996 pounds per capita), and tilapia (0.98 pounds per capita; National Fisheries Institute, 2022).
Aquaculture has grown over the decades to meet the increased consumer demand and improve sustainability of the food supply. Aquaculture now accounts for almost 50% of total seafood production (Food and Agriculture Organization of the United Nations, 2020). Seafood grown in aquaculture farms (as opposed to wild-caught seafood) are more likely to be exposed to antimicrobials, which are fed to fish and shellfish to combat disease resulting from intensive husbandry practices. There are only three antimicrobial drug classes (tetracyclines, phenicols, and potentiated sulfonamides) approved for the treatment and control of bacterial disease in aquaculture raised in the United States (U.S. Food and Drug Administration, 2020b). However, 65%–90% of seafood marketed in the United States is imported (Gephart et al., 2019; National Marine Fisheries Service, 2021) and may be exposed to additional drug classes (Schar et al., 2020), and many of these drugs are also used in human medicine (Smith, 2008). The use of antimicrobial drugs in aquaculture can precipitate the emergence of AMR in zoonotic pathogens that can then be directly transmitted to humans through consumption of or contact with contaminated product. Seafood can be a source of bacterial illness for humans in the United States. In 2010, the Centers for Disease Control and Prevention reported 143 seafood-associated outbreaks caused by a bacterial agent occurred in the United States during 1973 to 2006, with Vibrio parahaemolyticus being the most commonly reported agent (Iwamoto et al., 2010). AMR among seafood-borne bacteria could potentially decrease the effectiveness of antimicrobial treatments in humans. Also concerning, is the possibility of indirect transmission, or the enrichment and horizontal transfer of ARGs from bacteria in aquatic environments to related human pathogens.
A number of studies have shown that seafood are carriers of antimicrobial resistant bacteria (Zhao et al., 2003; Han et al., 2007; Khan et al., 2009; Tran et al., 2011; Wang et al., 2011; Nawaz et al., 2012; Ryu et al., 2012; Shaw et al., 2014; Boss et al., 2016; Elbashir et al., 2018; Jans et al., 2018). Recognizing that seafood could be a potential point of origin for emergence and enrichment of antimicrobial resistant bacteria in the United States, in 2020 the NARMS began national monitoring of resistant bacteria in retail seafood. To establish the optimal criteria for routine systematic seafood surveillance and monitoring and to estimate the resistant bacteria status of retail seafood sold in the United States, in 2019 NARMS conducted a year-long pilot study collecting retail raw shrimp, tilapia, and salmon from grocery outlets in eight states across the country. For the pilot study, we evaluated, in retail seafood, bacteria of public health importance (Salmonella, Pseudomonas aeruginosa, Vibrio spp.) and both spoilage-related and naturally occurring aquatic bacteria (Staphylococcus spp., Enterococcus spp., Aeromonas spp.) that were known to have sufficiently high prevalence in fish and shellfish (Tuševljak et al., 2012; Boss et al., 2016; Elbashir et al., 2018). We also looked for carbapenem-resistant organisms (CRO), using selective enrichment methods. The prevalence of the targeted bacterial genera, their AMR patterns, and ARG findings are presented herein. We also tested the statistical significance of associations between epidemiologic variables and both bacterial species of major and minor public health importance and the occurrence of antimicrobial resistant bacteria.
Materials and Methods
Sampling
Between January and December of 2019, samples of salmon (n = 710) and shrimp (n = 710) were purchased from supermarkets in participating NARMS sites: California, Georgia, Kansas, Missouri, New York, Pennsylvania, South Carolina, and South Dakota. Seafood was collected as fresh, frozen, or previously frozen raw samples. Tilapia (n = 214) also was collected from supermarkets in the above states (except for Pennsylvania) between September and December of 2019. A sample was defined as the unit that was purchased, and may have varied by purchase. For example sometimes a one pound bag of peeled frozen shrimp may have been purchased and other times a quarter pound of individual head-on-tail-on shrimp were purchased. Country of origin, raising claims (antibiotic use vs. organic and farm-raised vs. wild-caught), meat cut, and salmon variety were recorded for all samples, where applicable. Participating laboratories performed sample collection and preparation, and bacterial isolation.
Sample Preparation
A 25 gram portion of each seafood sample was aliquoted with 225 ml of Alkaline Peptone Water (APW, Vibrio; Thermo-Scientific, Waltham, MA), 225 ml lactose broth (Salmonella), or 225 ml Buffered Peptone Water (BPW; BD Difco™, Detroit, MI; Enterococcus, Aeromonas, P. aeruginosa, Staphylococcus, CRO) in a sterile stomacher bag and stomached or blended for 2 min, followed by incubation at 35°C for 24 h. Unless otherwise noted, the enriched mixture (1 μl) was streaked onto agar plates described below.
Bacterial Isolation and Identification
All samples were tested for Vibrio, P. aeruginosa, Enterococcus, Staphylococcus, and Aeromonas. However, only 506 salmon, 498 shrimp and 205 tilapia samples were tested for Salmonella as testing was stopped midway through the pilot due to low recovery. All samples collected were evaluated for the presence of CROs except for tilapia because testing was stopped midway due to the detection of intrinsic resistance mechanisms in the majority of sequenced isolates. For Vibrio, APW enrichments were streaked onto Thiosulfate-Citrate-Bile-Sucrose agar (BD Difco™) and incubated at 35°C for 18–24 h. Presumptive Vibrio characteristically appeared as green or yellow colonies. If more than one color appeared, one of each colored colony was selected. For Salmonella, 0.1 ml of lactose broth enrichment was transferred to 10 ml Rappaport-Vassiliadis (RVR10) medium (BD Difco™) and incubated at 42°C for 24 h. Each RVR10 culture was streaked to one XLT-4 (Thermo-Scientific, Remel™, Lenexa, KS) and one HE agar (Thermo-Scientific, Remel™) and incubated at 35°C for 24 h. One typical colony was selected per agar for further testing. For P. aeruginosa, BPW enrichments were streaked to either Pseudomonas Isolation Agar (PIA; BD Difco™) or MacConkey agar (MAC; Thermo-Scientific) and plates were incubated at 35°C for 18–48 h. Pseudomonas spp. appeared as colorless colonies on MAC and presumptive P. aeruginosa characteristically appeared as green/blue–green colonies on PIA. One colony was selected per sample. For Enterococcus, BPW enrichments were streaked to Enterococcosel agar (BD BBL™, Franklin Lakes, NJ) or Mannitol Salt Agar (MSA; BD BBL™) and incubated for 48 h at 35°C. One colony was selected from each sample. Staphylococcus were also isolated from MSA. After streaking with BPW enrichments, plates were incubated for 24 h at 35°C. One colony was selected per sample. For isolation of Aeromonas Cefsulodin-Irgasan-Novobiocin (CIN) agar (Thermo-Scientific, Remel™) was incubated for 24–48 h at 25°C. Presumptive Aeromonas colonies had a pale colonies with rose red centers. One colony was selected per sample. Isolation of CRO bacteria was performed by streaking BPW enrichments onto mSuperCarba CHROMagar™ (CHROMagar™, France) and incubating at 35°C–37°C for 18–24 h. One colony of each color (up to five from each sample) was picked. According to the package insert, the typical appearance of carbapenemase producing microorganisms is dark pink to red (E.coli), metallic blue (Coliforms), translucent, +/− natural pigmentation cream to green (Pseudomonas), Cream (Acinetobacter), colorless, natural pigmentation (other Gram-negative organisms). For all targeted bacteria, presumptive isolates were picked from their respective selective media, streaked to blood agar plates (BAP; Thermo-Scientific™) and incubated at 35°C for 24 h. If growth was pure, colonies were swabbed to Brucella broth (BD Difco™) with 15% glycerol mixture, frozen at −60°C to −80°C, and shipped to the United States Food and Drug Administration (FDA) Center for Veterinary Medicine (CVM) for additional analyses.
At FDA, all bacterial isolates received were confirmed using the VITEK® 2 Compact (n = 6,491; bioMérieux, France), except for isolates grown on mSuperCarba CHROMagar™. Only a subset of presumptive mSuperCarba CHROMagar™ isolates (N = 952) were confirmed on the VITEK® 2 Compact.
Antimicrobial Susceptibility Testing
Salmonella spp., P. aeruginosa, Vibrio spp., Staphylococcus spp., Enterococcus, and Aeromonas spp. isolates were tested for susceptibility to antimicrobials approved for use in aquaculture in the United States as well as other antimicrobial classes that may be used in other countries. Testing was performed at FDA CVM via the broth microdilution assay (Sensititre™ System, Thermo Fisher Scientific™) using methods recommended by the Clinical and Laboratory Standards Institute (CLSI; Clinical and Laboratory Standards Institute, 2016, 2019). Gram-negative bacteria were tested on either Gram-negative antimicrobial panel (Sensititre™ panel CMV4AGNF or CMV5AGNF, Thermo Fisher Scientific™). All 3 Salmonella and 20 P. aeruginosa underwent antimicrobial susceptibility testing (AST). Due to the large number of isolates recovered, a random subset of 179 Aeromonas isolates underwent AST. To examine associations between sample-based variables (e.g., geographic region of origin) and isolate-based variables (e.g., AMR) as described below, only one Vibrio isolate per positive sample was selected for AST. Of the selected isolates, all isolates from the following species were subjected to AST: V. parahaemolyticus (n = 91), V. cholerae (n = 30), and V. vulnificus (n = 1); and we tested a random subset of isolates of each species: V. metschnikovii (n = 53), V. fluvialis (n = 27), V. alginolyticus (n = 5) and mixed populations (n = 3). The antimicrobial classes tested on both panels were: aminoglycosides (gentamicin), β-lactam combination agents (amoxicillin-clavulanic acid), carbapenems (meropenem), cephems (cefoxitin, ceftriaxone), folate pathway inhibitors (sulfisoxazole and trimethoprim-sulfamethoxazole), macrolides (azithromycin), penicillins (ampicillin), phenicols (chloramphenicol), quinolones (ciprofloxacin, nalidixic acid), and tetracyclines (tetracycline). Isolates tested on the Sensititre™ CMV5AGNF panel were also tested against lipopeptides (colistin).
We tested 385 Enterococcus and 210 Staphylococcus isolates on the NARMS Gram-positive antimicrobial panel (Sensititre™ panel CMV4AGP, Thermo Fisher Scientific) that included the following antimicrobial classes and drugs: aminoglycosides (gentamicin, streptomycin), glycopeptides (vancomycin), glycylcyclines (tigecycline), lipopeptides (daptomycin), macrolides (erythromycin), nitrofurans (nitrofurantoin), orthosomycins (avilamycin), oxazolidones (linezolid), penicillins (ampicillin), phenicols (chloramphenicol), streptogramins (quinupristin-dalfopristin), quinolones (ciprofloxacin), and tetracyclines (tetracycline).
Interpretation of minimum inhibitory concentration (MIC) values was based on the CLSI clinical breakpoints for human infection treatment, when available (Clinical and Laboratory Standards Institute, 2016, 2019). Otherwise NARMS provisional cutoffs were used for azithromycin against Salmonella (interpreted as resistant if MIC ≥ 32 μg/ml) and tigecycline against Enterococcus (interpreted as resistant if MIC > 0.25 μg/ml; U.S. Food and Drug Administration, 2019). Because the isolates recovered from mSuperCarba CHROMagar™ did not undergo confirmatory phenotypic susceptibility testing for carbapenem antibiotics, those isolates are referred to as “presumptive CRO” from this point forward.
Identification of ARGs
A subset of presumptive CRO was randomly selected for whole genome sequencing (WGS) and all Salmonella were sequenced. Of the other targeted genera (Enterococcus, P. aeruginosa, Staphylococcus spp., Vibrio spp., and Aeromonas spp.), we performed WGS only on a subset of the isolates with resistance to at least one antimicrobial. Altogether, 370 isolates were sequenced including 3 Salmonella, 16 Aeromonas, 2 Enterococcus, 6 P. aeruginosa, 11 Staphylococcus, 44 Vibrio and 288 presumptive CRO. Isolates were sequenced on Illumina MiSeq™ using v3 reagent kits (Illumina Inc., San Diego, CA, United States) with 2 × 300 bp paired-end reads. The libraries were prepared with Nextera XT kit by Illumina, and raw sequences were assembled de novo using CLC Genomic Workbench (version 10.0). ARGs were identified in the assembled genomes with AMRFinder Plus 3.8 software.1 All isolate identifiers are listed in Supplementary Table S1, and can be found under NCBI Bioproject PRJNA800017. Speciation was done using the MLST software2 which incorporates components of the PubMLST database3 (Jolley and Maiden, 2010). WGS speciation was crosschecked with the VITEK® identification and when they differed, the WGS speciation was chosen for all analysis. If bacterial genera could not be resolved through in silico methods, VITEK® identification was used.
Statistical Analysis
The percent of samples that tested positive was calculated by dividing the number of samples that yielded the bacterial genera or species by the total number of samples of the seafood commodity collected.
The percent phenotypically resistant was calculated by dividing the number of isolates with the antimicrobial MIC at or above the CLSI clinical breakpoint for human infection treatment or the NARMS cutoff interpretive criterion by the total number of isolates of that bacterial species tested against that antimicrobial. Percent resistance was only calculated for antimicrobials with CLSI breakpoints or NARMS interpretive criteria. Isolates resistant to at least three classes of antimicrobials were considered multidrug resistant.
Two sets of multivariable logistic regression models were used (PROC LOGISTIC, SAS version 9.4 (SAS, Cary, NC)) to evaluate the association for a sample between epidemiologic risk factors (Table 1) and the following outcomes: (1) being culture positive for at least one of the targeted genera (Aeromonas, Salmonella, Staphylococcus, Enterococcus, P. aeruginosa, or Vibrio), or (2) contamination of a sample with an antimicrobial resistant isolate of the target bacterial genera (i.e., excluding presumptive CRO). In the first set of models, commodity was included as a predictor, but commodity specific variables (meat cut and salmon variety) were excluded. In the second set of models, multivariable regression was performed for each commodity. For both sets of models the following procedures were followed: Variables were first screened with a univariate analysis using p ≤ 0.05, then we improved model performance by grouping United Nation-derived subregions (Table 1) into respective U.N-defined geographic regions (United Nations Statistic Division, 2022; e.g., North America, Latin America and the Caribbean, Asia, Europe, Oceania). We grouped “fresh” and “previously frozen” packaging as “sold at the counter.” Variables with low variability (>90% of the samples in one category) were excluded from the analysis; these included raised with/without antibiotics and tilapia meat cut. Samples with missing demographic data (e.g., “Unknown”) were also excluded. The final multivariable models were constructed using the stepwise variable selection procedure (p < 0.25 for a variable to enter the model and keeping only variables with a significance level p ≤ 0.05). All two-way interactions were evaluated. The direction and magnitude of the statistical associations were interpreted using the odds ratios (OR) with 95% CI.
Results
Prevalence of Bacterial Contamination Among Sampled Seafood
Of the bacteria of interest, the predominant genus in all seafood samples was Enterococcus (Figure 1). Approximately 66% of shrimp samples were positive for this genus, as well as 52% of tilapia samples and 39% of salmon samples collected. Salmonella had the lowest prevalence, with one of 506 salmon samples yielding a Salmonella Reading and two of 498 shrimp samples yielding a Salmonella Teko and Salmonella Newport, respectively (Supplementary Table S1). No Salmonella were recovered from tilapia. Similarly, prevalence of P. aeruginosa was low in salmon (1.1%, 8/710), shrimp (0.9%, 6/710), and tilapia (2.3%, 5/214) samples (Figure 1). Aeromonas and Staphylococcus were present in ~20%–30% of all seafood samples. Vibrio had the most variable prevalence across the commodities, with 41% (290/710) of shrimp samples yielding isolates of this genus versus 9.0% (64/710) of salmon and 7.0% (14/214) of tilapia samples. Approximately 75% of salmon, 79% of shrimp, and 93% of tilapia samples yielded at least one presumptive CRO isolate. We identified 28 different genera among the 948 presumptive CRO isolates tested, with the most predominate being Pseudomonas (40% of all the isolates), followed by Stenotrophomonas and Acinetobacter (9.54% each), Serratia (8.5%), and Aeromonas (8.0%; Table 2).
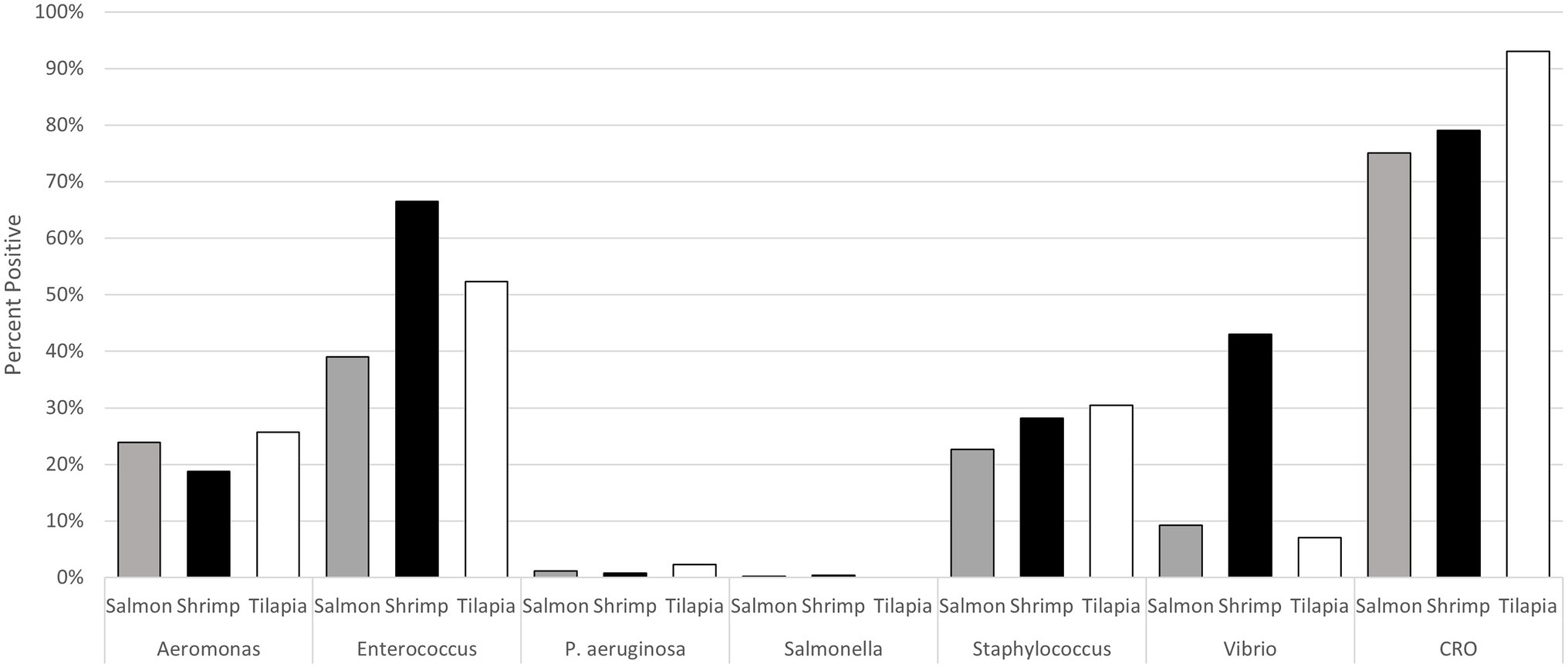
Figure 1. Prevalence of the target bacterial genera and presumptive carbapenem resistant microorganisms in the seafood samples collected from retail food stores in eight states in 2019.
Antimicrobial Resistance Prevalence
The prevalence of phenotypic AMR and distribution of antimicrobial drug MICs for the isolates are listed for the target bacteria in Tables 3, 4; Supplementary Figures 1–6. There was a consistently low prevalence of Gram-negative organisms that were classified as resistant. All three Salmonella were susceptible to all antimicrobials tested. For P. aeruginosa, four drugs (colistin, ciprofloxacin, gentamicin, and meropenem) have CLSI clinical breakpoints for human infection treatment (Clinical and Laboratory Standards Institute, 2019), and all isolates were susceptible to these four drugs. However, all of these isolates demonstrated MICs ≥ 32 μg/ml for amoxicillin-clavulanic acid, ampicillin, azithromycin, cefoxitin, chloramphenicol, and nalidixic acid, and ≥256 μg/ml for sulfisoxazole. Depending on the commodity, 27%–44% of Vibrio isolates, which are known to have some intrinsic β-lactam resistance mechanisms (Chiou et al., 2015), were resistant to ampicillin. Less than 7% of the Vibrio isolates exhibited resistance to other antimicrobials. Prevalence of resistance was low in Aeromonas isolates (8%; Table 5).
Among the Gram-positive organisms, Enterococcus spp. exhibited the highest prevalence of resistance, with the most common resistance to tetracycline (12%–24%), followed by erythromycin (3%–14%). Enterococcus faecium were also commonly resistant to quinupristin/dalfopristin, with the resistance prevalence ranging from 40% to 75%, depending on source. Prevalence of resistance in enterococci from tilapia was two to seven times higher than that in the isolates from shrimp or salmon for each chloramphenicol, erythromycin, nitrofurantoin, quinupristin-dalfopristin (E. faecium only), and streptomycin. Prevalence of tetracycline resistance in enterococci from tilapia and salmon was two times higher than that in isolates from shrimp. However, all enterococci from tilapia were susceptible to ampicillin, avilamycin, ciprofloxacin, daptomycin, gentamicin, tigecycline, and vancomycin. We found two enterococci from salmon that were resistant to avilamycin, an orthosomycin antimicrobial intended for use only in broiler chickens and weaner pigs. One of the isolates came from a product imported from Chile and the other from a product imported from Canada (Supplementary Table S1). Prevalence of daptomycin resistance in staphylococci from tilapia was 2 to 3 times higher than that found in isolates from shrimp or salmon. Less than 8% of all the Staphylococcus isolates from all sources were resistant to the other antimicrobials tested. Table 6 shows patterns of multidrug resistance (MDR, resistant to ≥3 classes), which was overall very low. Approximately 2% (21/1006) of isolates tested were MDR.
Risk Factor Analysis
Epidemiological risk factors (geographic region of origin, claims about antibiotic use, farm raising claims, and “sold as”) were analyzed for their association with isolation of at least one of the targeted bacterial genera (Tables 7, 8) and for association with the isolate resistance to at least one of the tested antimicrobials (Table 9). In the final multivariable logistic regression model, four epidemiologic risk factors were significantly associated with the sample contamination with one of the targeted bacterial genera: the sample being shrimp vs. salmon, originating from Asia or Latin America and the Caribbean vs. North America, and sold at the counter vs. purchased frozen. Looking at each seafood commodity independently, we found that for salmon, an Asian country of origin and counter purchasing increased the odds of bacterial contamination by 3.4- and 2.6-fold, respectively. A farm raising claim was the only variable significantly associated with bacterial contamination of a shrimp sample. The odds of recovery from farm-raised shrimp were 1.9 times higher than wild-caught shrimp.
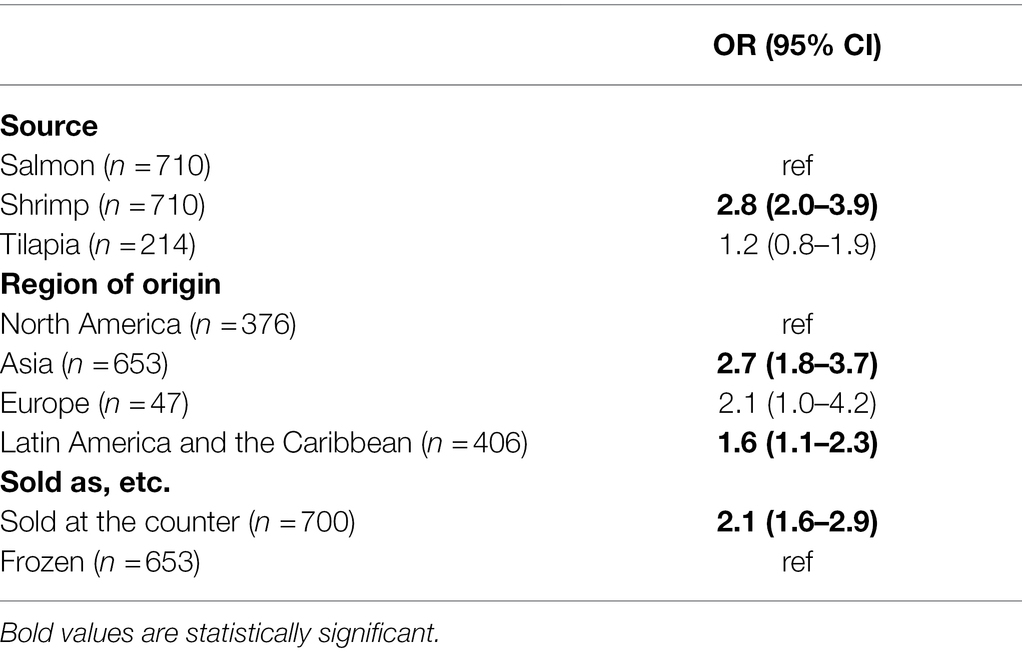
Table 7. Adjusted ORs for growth of at least one bacterium in all seafood samples. Risk factors that did not fit the model (i.e., farm raising claim) are not shown. (n=) is the number of samples analyzed.
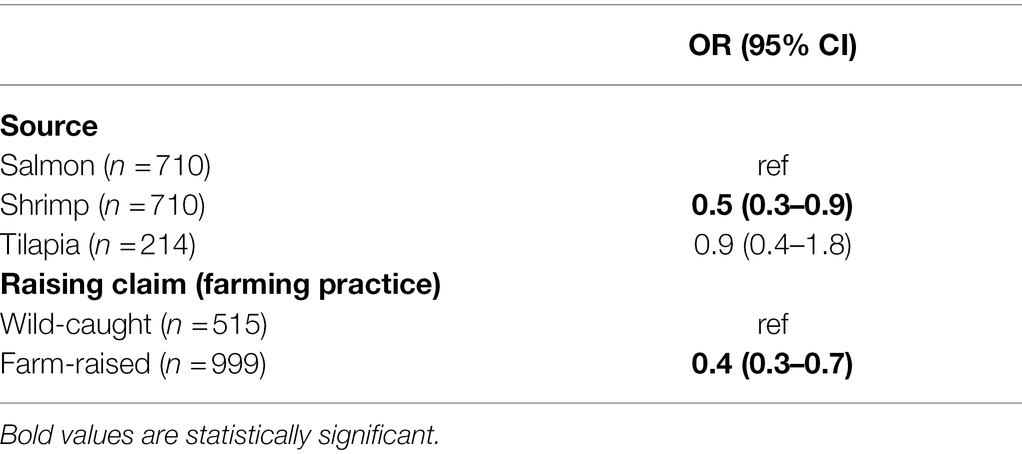
Table 9. Adjusted OR for resistance to at least antimicrobial. Risk factors that did not fit the model (i.e., region of origin and “sold-as”) are not shown. (n=) is the number of samples analyzed.
Due to low a prevalence of resistance overall in seafood, we examined the association of epidemiologic factors with resistance to at least one of the tested antimicrobials among the target genera (i.e., not including presumptive CRO). Using the full multivariable model with contamination of a sample with an antimicrobial resistant isolate of the target bacterial genera as the dependent variable, we found that shrimp were less resistant than salmon (OR 0.5, CI 0.3–0.9), and that farm-raised shrimp and salmon were 60% less likely to yield a resistant isolate. This odds ratio was most likely driven by a larger proportion of tetracycline and daptomycin-resistant isolates from wild-caught commodities (Supplementary Figure 7). However, there was greater diversity in resistance phenotypes among the isolates from farm-raised commodities.
ARGs
Thirty-three percent (26/79) of the non-CRO, non-Salmonella isolates that were sequenced carried no known resistance genes despite having phenotypic resistance to at least one of the antimicrobials tested (Supplementary Table S1). Similarly, 34% (98/288) of presumptive CRO isolates did not harbor any known ARGs even though they all exhibited decreased phenotypic susceptibility to carbapenems (as defined by the growth on carbapenem containing agar; Supplementary Table S1). Intrinsic or other as-yet-to-be-annotated genes were likely responsible for the phenotypic resistance in isolates without known ARGs. Table 10 lists the 156 unique ARGs identified from the 370 strains. A number of presumptive CRO had carbapenemase genes that are normal constituents of those bacteria, including blaL1 in Stenotrophomonas, cphA/cphA1 in Aeromonas, and members of the blaOXA-51-like and blaOXA-213-like families in Acinetobacter. We identified three isolates with carbapenemases that are known to transfer horizontally. An isolate each of Aeromonas spp. and an Acinetobacter baumanii each harbored the blaNDM-1 gene, and an isolate of Enterobacter cloacae carried the blaIMI-2 gene. All three isolates were recovered from shrimp imported from Southeast Asia. At least 76% of the 37 cephalosporinases identified were also common in the bacterial genera in which detected, including chromosomally encoded inducible AmpC β-lactamases in Pseudomonas (blaPDC alleles; Rodríguez-Martínez et al., 2009) and Acinetobacter (blaADC alleles; Hujer et al., 2005; Gordon and Wareham, 2010). We found mcr-3.3 in an Aeromonas isolated from CRO media, however the MIC was only 0.5 μg/ml. Other studies have shown that colistin susceptibility is determined by whether mcr-3.3 is located on the chromosome or a plasmid (Ling et al., 2017; Shen et al., 2018). We identified one extended spectrum β-lactamase (ESBL) gene, blaCTX-M-55, in an Aeromonas isolated from CIN media. The gene conferred resistance to ampicillin and ceftriaxone (Supplementary Table S1). The isolate also contained qnrS1, a combination seen before on an IncF plasmid in isolates from NARMS retail meat surveillance (Tyson et al., 2019). Although qnrS1 confers resistance to quinolones and reduced susceptibility to fluroquinolones in E. coli and Salmonella, this Aeromonas isolate was susceptible to ciprofloxacin (MIC = 0.015 μg/ml). Other genotype–phenotype comparisons were difficult to make given the lack of accepted interpretive criteria to categorize the phenotypic antimicrobial susceptibility of the isolates and the limited number of isolates sequenced, however some valuable information might be gleaned from the data. We found qnrVC genes in Vibrio susceptible to ciprofloxacin as well as tetracycline resistance genes, tet(34) and tet(35), in isolates susceptible to the drug (Supplementary Table S1).
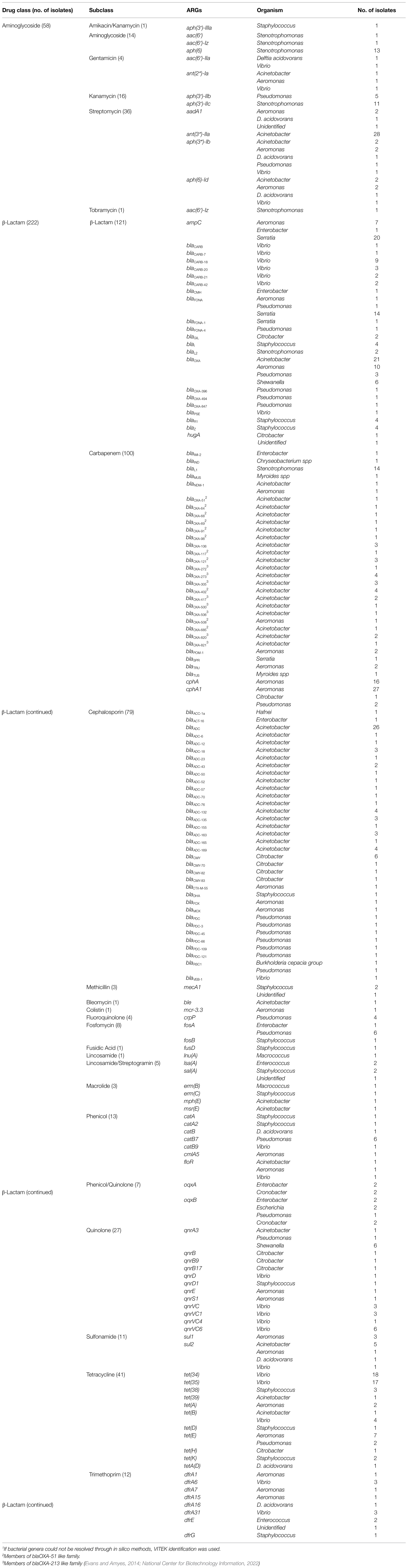
Table 10. AMR genes detected based on whole genome sequencing and annotation of the bacterial isolates1 from seafood samples.
Discussion
We examined the prevalence of several target bacterial genera in retail seafood as well as their resistance attributes. We found remarkably lower levels of Salmonella contamination (<1%) than was found in another large scale FDA survey of local and imported seafood collected from processors and distributors throughout the United States (Heinitz et al., 2000). In that 2000 study, 11.8% of raw fin fish/skin fish and 8.9% of raw crustaceans were positive for Salmonella. Although both the Heinitz study and our study used FDA-BAM methods to isolate Salmonella, Heinitz et al. used composite samples from 15 cases of product, likely resulting in greater prevalence of bacteria. Additionally, the majority of Salmonella were isolated from imports, which represented a higher proportion of samples than in our study, and may have also impacted prevalence. Finally, federal regulations or guidance published in the intervening period may have had some impact on Salmonella contamination. Levels of Salmonella, Aeromonas, Vibrio, and Enterococcus we found were more consistent with other studies (Tuševljak et al., 2012; Jans et al., 2018). Vibrio and Aeromonas were the most frequently isolated Gram-negative bacteria and Enterococcus were the most frequently isolated Gram-positive bacterium, making them potentially better candidates for tracking AMR. However, there is a need to establish internationally accepted MIC breakpoints (i.e., epidemiological cut-off values) for many drugs with activity against Vibrio and Aeromonas in order to track trends over time. There has been some progress in this area (Baron et al., 2017).
Shrimp had the highest odds of recovery of our target bacteria. Given its high consumption rate in the United States, this would certainly be a top priority seafood commodity to include in a long-term monitoring or surveillance program. If resources allow, salmon and tilapia should also be included in a United States-focused monitoring or surveillance program because they are also highly consumed. These data suggest a need for tracking domestically produced and imported seafood to assess the potential for consumer exposure to antimicrobial resistant bacteria. Sample collection could include a higher proportion of samples from Asia and Latin America and the Caribbean, as these were more likely to produce the target bacteria. When available, fresh or previously frozen seafood—especially salmon, would be preferred over frozen samples as thawed and fresh commodities were more likely to yield our target bacteria. Based on the bacterial prevalence results from this pilot study, NARMS began monitoring seafood in 2020 to test Vibrio, Enterococcus and Aeromonas species and lactose fermenters in shrimp, salmon, and tilapia.
Targeted bacteria exhibited pan-susceptibility (Salmonella and P. aeruginosa) or low levels of resistance (<10%) to antimicrobials with interpretive criteria for these organisms, with the exception of ampicillin (Vibrio), daptomycin (Staphylococcus), quinupristin-dalfopristin (E. faecium) and tetracycline (Enterococcus spp.). While many P. aeruginosa isolates did have MIC values at or above 32 μg/ml for other antimicrobials including amoxicillin-clavulanic acid, ampicillin, and chloramphenicol, it is known that this organism harbors a high number of intrinsic resistance mechanisms (Pang et al., 2019). Similarly, the ampicillin and daptomycin resistance phenotypes in Vibrio and Staphylococcus, respectively, are ostensibly mediated by mechanisms intrinsic to those bacteria. Some species of Vibrio have been shown to harbor chromosomally encoded class A carbenicillin-hydrolyzing β lactamases (blaCARB; Chiou et al., 2015), conferring intrinsic resistance to ampicillin. The blaCARB genes were present in all of Vibrio isolates sequenced for this study. Additionally, Staphylococcus sciuri, which comprised the majority of Staphylococcus spp. recovered, are intrinsically less susceptible to daptomycin (Sader and Jones, 2012; Schoenfelder et al., 2017) than other Staphylococcus species.
Resistance may also be mediated by acquisition of exogenous determinants as a result of antimicrobial selection pressure. Selection pressure from tetracyclines, which are approved for use in fish in the United States (U.S. Food and Drug Administration, 2020b) and are among the most frequently used and most consumed (in mg/kg) antimicrobials in aquatic food globally (Schar et al., 2020), and may have promoted the high levels of tetracycline resistance we observed in Enterococcus. Tetracyclines are not approved for use in shrimp produced in the United States (U.S. Food and Drug Administration, 2021), which may partially explain why tetracycline resistance levels were almost two-fold lower among enterococci from retail shrimp than retail salmon and tilapia. Streptogramins are not authorized for use in aquaculture in the United States, and there is little evidence of their use in other countries, therefore the presence of quinupristin-dalfopristin resistant E. faecium and Staphylococcus isolates remains to be investigated. There was no correlation between the presence of antimicrobial resistant bacteria and country of origin.
Although levels of resistance in Enterococcus were low overall, we did observe an appreciable difference between tilapia, salmon, and shrimp. There were no tilapia isolates resistant to tigecycline, vancomycin, gentamicin, daptomycin, or ampicillin, which may simply be due to a combination of the smaller number of isolates tested (n = 58), compared to salmon (n = 134) and shrimp (n = 193), and the low prevalence of resistance to these drugs overall. However, Enterococcus from tilapia had almost two-fold higher levels of resistance to chloramphenicol, erythromycin, nitrofurantoin, quinupristin-dalfopristin (E. faecium only), and streptomycin compared to isolates from shrimp or salmon, which could be a possible consequence of commodity-specific differences in the species composition of isolates with higher MICs. We observed a larger proportion of non-faecalis Enterococcus species among the resistant tilapia isolates (Supplementary Table S1) than among the resistant shrimp and salmon isolates. In contrast, species distribution of daptomycin resistant Staphylococcus isolates was similar across all commodities (Supplementary Table S1), despite tilapia isolates having two-fold higher levels of resistance to this drug compared to isolates from salmon and shrimp. A recent study suggests that tilapia receive more antimicrobials than shrimp and salmon (Schar et al., 2020). Indeed, 93% of tilapia collected in our study were farm-raised compared to just 67% of shrimp and 45% of salmon. However, the dosage and type of antimicrobials these animals receive are largely obscure due to inadequate statistics on antimicrobial usage in aquaculture. While one would assume that farm-raised shrimp and salmon would also be exposed to more antimicrobials and thus have a higher likelihood of harboring resistant bacteria than wild-caught seafood, that did not appear to be the case in our samples. We found that farm-raised shrimp and salmon had a 60% lower likelihood of containing resistant bacteria. This was in part explained by higher levels of resistance to tetracycline and daptomycin in bacteria from wild-caught shrimp and salmon, and also differential distribution of bacterial species among the raising claims (for example, S. sciuri, comprised 54% of Staphylococcus tested from wild-caught salmon, but only 17% of Staphylococcus from farm-raised salmon; Supplementary Figure 7). Notably, bacteria from farm-raised seafood were resistant to more types of antimicrobials than bacteria from wild caught seafood. Our results oppose those of others who have shown that farm raised shrimp are more abundant in ARGs compared to wild caught shrimp (Sharma et al., 2021). It is unclear why wild-caught shrimp and salmon in this study would harbor bacteria that are more resistant to these drugs, although a few possible scenarios could be at play including: exposure to sewage or agricultural pollution from farms, as-yet-unknown impacts from climate change and plastic pollution on coastal waters, and potential mislabeling of product.
Some bacteria considered to be hospital-acquired could have foodborne origins. Using selective media, we found carbapenem-resistant Acinetobacter baumannii in the aquatic environment from seafood that were both farm-raised and wild-caught. Acinetobacter are ubiquitous in the environment, and some Acinetobacter species, including A. baumannii are recognized as opportunistic fish pathogens (Dekić et al., 2018). Our findings suggest that A. baumannii could potentially be transmitted to humans through the seafood chain. Among the 55 Acinetobacter isolates that were sequenced, we identified 47 predicted genes, 36 of which were naturally occurring, including undescribed blaADC cephalosporinase and blaOXA oxacillinase alleles. Other than ant(3″)-IIa spectinomycin resistance genes, few clinically relevant acquired ARGs were found, supporting previous evidence that environmental strains appear different from highly resistant clinical isolates associated with nosocomial environments (Klotz et al., 2019).
One limitation of the study was that we were unable to sequence all isolates presenting a non-wild-type phenotype. This put us at a disadvantage to compare genotypes with respective phenotypes. Additionally, we could not confirm whether the genetic mechanisms were intrinsic to the organism or horizontally acquired. We suppose that intrinsic mechanisms common to a bacterial species would be less affected by the variables we tested in our model, and our odds ratio may potentially overestimate the association of source and farm raising claim with AMR. Lastly, no detailed information on differences in aquaculture practices and facilities was available on the sample packaging to help explain the significant differences in the bacterial contamination prevalence and antimicrobial resistant bacteria prevalence between seafood commodities or seafood from different regions. Despite these limitations, we were able to complete a multi-lab study using standardized methods to assess a population-scale prevalence of resistant bacteria in seafood. Our results correlated with others showing that bacteria with clinically relevant carbapenamase genes can be found in imported seafood (Janecko et al., 2016). We also identified a number of genes that might have clinical relevance when expressed in zoonotic pathogens such as Salmonella but are intrinsic to the environmental aquatic bacteria in which they were discovered (Table 10). Additional work is needed to determine if these genes are located on plasmids or transposable elements within chromosomes, as these aquatic bacteria may act as reservoirs for clinically relevant ARGs, and contribute to their potential dissemination.
Conclusion
Even though seafood has become an increasingly important source of animal protein for the United States general population over the past decade, it has been understudied in the area of foodborne AMR. Here, we have shown that while imported and domestically produced seafood can be contaminated with antimicrobial resistant bacteria, the prevalence of contamination with resistant bacteria is low. However, because antimicrobials are used in aquaculture it is worthwhile to continue monitoring these food commodities for resistant bacteria. Plans for continued monitoring must consider differences in AMR prevalence by seafood type, country of origin, and raising practices, and the presence of not-yet-identified ARGs in bacteria contaminating seafood. This seafood pilot study provides an important basis for the NARMS seafood surveillance that began in January 2020.
Data Availability Statement
The datasets presented in this study can be found in online repositories. The names of the repository/repositories and accession number(s) can be found in the article/Supplementary Material.
Author Contributions
HT, SA, EN, CW, GT, and PM contributed to the conception and design of the study. XL, MT-D’A, VV, RH, LM, NM’i, and LR conducted sampling. SA, SB, SY, CR-T, SS, XL, MT-D’A, VV, RH, LM, NM’i, LR, and SB-J all conducted microbial testing. CL conducted whole genome sequencing. CL and ES performed bioinformatic analysis. EN and HT performed statistical analysis. HT wrote the first draft of the manuscript. All authors contributed to the manuscript revision, read, and approved the submitted version.
Funding
This work is supported by the United States Food and Drug Administration.
Author Disclaimer
The views expressed in this article are those of the authors and do not necessarily reflect the official policy of the Department of Health and Human Services, the United States Food and Drug Administration, or the United States Government. The opinions expressed in this article are the author’s own and do not reflect the view of the National Institutes of Health. Reference to any commercial materials, equipment, or process does not in any way constitute approval, endorsement, or recommendation by the Food and Drug Administration.
Conflict of Interest
The authors declare that the research was conducted in the absence of any commercial or financial relationships that could be construed as a potential conflict of interest.
Publisher’s Note
All claims expressed in this article are solely those of the authors and do not necessarily represent those of their affiliated organizations, or those of the publisher, the editors and the reviewers. Any product that may be evaluated in this article, or claim that may be made by its manufacturer, is not guaranteed or endorsed by the publisher.
Acknowledgments
We acknowledge the work of the NARMS retail meat surveillance sites in the isolation and characterization of seafood isolates. We would especially like to thank the following individuals for their technical contributions and support of the project: Daniel Tadesse (FDA Center for Veterinary Medicine), Zachary Lau, and Kara Hendrickson-Guttum (South Dakota State University), Robert Mugabi and Tara N. Gaire (Kansas State University), Katie Yen Lee, Kurtis Lavelle, Anny Huang, and Macie Mieko Tanaka (University of California at Davis), Lisa Dettinger, Melinda Johnston, Bridgette Husband (Pennsylvania Department of Health), and Charles MacGowan (New York State Department of Health). We would also like to recognize the efforts of microbiology and epidemiology staff at the SC Department of Public Health and Environmental Control who participated in the study but were unable to coauthor this manuscript. This work was prepared while Victoriya Volkova was employed at Kansas State University.
Supplementary Material
The Supplementary Material for this article can be found online at: https://www.frontiersin.org/articles/10.3389/fmicb.2022.928509/full#supplementary-material
Footnotes
1. ^https://www.ncbi.nlm.nih.gov/pathogens/antimicrobial-resistance/AMRFinder/
References
Baron, S., Granier, S. A., Larvor, E., Jouy, E., Cineux, M., Wilhelm, A., et al. (2017). Aeromonas diversity and antimicrobial susceptibility in freshwater—an attempt to set generic epidemiological cut-off values. Front. Microbiol. 8:00503. doi: 10.3389/fmicb.2017.00503
Boss, R., Overesch, G., and Baumgartner, A. (2016). Antimicrobial resistance of Escherichia coli, enterococci, Pseudomonas aeruginosa, and Staphylococcus aureus from raw fish and seafood imported into Switzerland. J. Food Prot. 79, 1240–1246. doi: 10.4315/0362-028x.Jfp-15-463
Chiou, J., Li, R., and Chen, S. (2015). CARB-17 family of β-lactamases mediates intrinsic resistance to penicillins in Vibrio parahaemolyticus. Antimicrob. Agents Chemother. 59, 3593–3595. doi: 10.1128/aac.00047-15
Clinical and Laboratory Standards Institute (2016). Methods for Antimicrobial Dilution and Disk Susceptibility Testing of Infrequently Isolated or Fastidious Bacteria: Third edition. CLSI Document M45-Ed3. Wayne, PA. Clinical and Laboratory Standards Institute.
Clinical and Laboratory Standards Institute (2019). Performance Standards for Antimicrobial Susceptibility Testing: Thirtieth Edition. CLSI Document M100-Ed30. (Wayne, PA). Clinical and Laboratory Standards Institute.
Dekić, S., Klobučar, G., Ivanković, T., Zanella, D., Vucić, M., Bourdineaud, J. P., et al. (2018). Emerging human pathogen Acinetobacter baumannii in the natural aquatic environment: a public health risk? Int. J. Environ. Health Res. 28, 315–322. doi: 10.1080/09603123.2018.1472746
Elbashir, S., Parveen, S., Schwarz, J., Rippen, T., Jahncke, M., and DePaola, A. (2018). Seafood pathogens and information on antimicrobial resistance: a review. Food Microbiol. 70, 85–93. doi: 10.1016/j.fm.2017.09.011
Evans, B. A., and Amyes, S. G. B. (2014). OXA β-Lactamases. Clin. Microbiol. Rev. 27, 241–263. doi: 10.1128/CMR.00117-13
Food and Agriculture Organization of the United Nations (2020). The State of the World Fisheries and Aquaculture. Available at: https://doi.org/10.4060/ca9229en (Accessed 15 October 2021).
Gephart, J. A., Froehlich, H. E., and Branch, T. A. (2019). Opinion: to create sustainable seafood industries, the United States needs a better accounting of imports and exports. Proc. Natl. Acad. Sci. 116, 9142–9146. doi: 10.1073/pnas.1905650116
Gordon, N. C., and Wareham, D. W. (2010). Multidrug-resistant Acinetobacter baumannii: mechanisms of virulence and resistance. Int. J. Antimicrob. Agents 35, 219–226. doi: 10.1016/j.ijantimicag.2009.10.024
Han, F., Walker, R. D., Janes, M. E., Prinyawiwatkul, W., and Ge, B. (2007). Antimicrobial susceptibilities of Vibrio parahaemolyticus and Vibrio vulnificus isolates from Louisiana gulf and retail raw oysters. Appl. Environ. Microbiol. 73, 7096–7098. doi: 10.1128/AEM.01116-07
Heinitz, M. L., Ruble, R. D., Wagner, D. E., and Tatini, S. R. (2000). Incidence of Salmonella in fish and seafood. J. Food Prot. 63, 579–592. doi: 10.4315/0362-028X-63.5.579
Hujer, K. M., Hamza, N. S., Hujer, A. M., Perez, F., Helfand, M. S., Bethel, C. R., et al. (2005). Identification of a new allelic variant of the Acinetobacter baumannii cephalosporinase, ADC-7 β-lactamase: defining a unique family of class C enzymes. Antimicrob. Agents Chemother. 49, 2941–2948. doi: 10.1128/AAC.49.7.2941-2948.2005
Iwamoto, M., Ayers, T., Mahon, B. E., and Swerdlow, D. L. (2010). Epidemiology of seafood-associated infections in the United States. Clin. Microbiol. Rev. 23, 399–411. doi: 10.1128/cmr.00059-09
Janecko, N., Martz, S. L., Avery, B. P., Daignault, D., Desruisseau, A., Boyd, D., et al. (2016). Carbapenem-resistant Enterobacter spp. in retail seafood imported from Southeast Asia to Canada. Emerg. Infect. Dis. 22, 1675–1677. doi: 10.3201/eid2209.160305
Jans, C., Sarno, E., Collineau, L., Meile, L., Stärk, K. D. C., and Stephan, R. (2018). Consumer exposure to antimicrobial resistant bacteria from food at swiss retail level. Front. Microbiol. 9:00362. doi: 10.3389/fmicb.2018.00362
Jolley, K. A., and Maiden, M. C. J. (2010). BIGSdb: scalable analysis of bacterial genome variation at the population level. BMC Bioinform. 11:595. doi: 10.1186/1471-2105-11-595
Khan, A. A., Ponce, E., Nawaz, M. S., Cheng, C. M., Khan, J. A., and West, C. S. (2009). Identification and characterization of class 1 integron resistance gene cassettes among Salmonella strains isolated from imported seafood. Appl. Environ. Microbiol. 75, 1192–1196. doi: 10.1128/aem.02054-08
Klotz, P., Higgins, P. G., Schaubmar, A. R., Failing, K., Leidner, U., Seifert, H., et al. (2019). Seasonal occurrence and Carbapenem susceptibility of bovine Acinetobacter baumannii in Germany. Front. Microbiol. 10:00272. doi: 10.3389/fmicb.2019.00272
Ling, Z., Yin, W., Li, H., Zhang, Q., Wang, X., Wang, Z., et al. (2017). Chromosome-mediated mcr-3 variants in Aeromonas veronii from chicken meat. Antimicrob. Agents Chemother. 61, e01272–e01217. doi: 10.1128/AAC.01272-17
National Center for Biotechnology Information (2022). Bacterial Antimicrobial Resistance Reference Gene Database, version 2021-09-30.1, electronic dataset. Available at: https://www.ncbi.nlm.nih.gov/pathogens/refgene/ (Accessed 1 October 2021)
National Fisheries Institute (2022). Available at: https://aboutseafood.com/about/top-ten-list-for-seafood-consumption/ (Accessed 10 May 10 2022).
National Marine Fisheries Service (2021). Fisheries of the United States, 2019 [Online]. U.S. Department of Commerce, NOAA Current Fishery Statistics No. 2019. Available at: https://www.fisheries.noaa.gov/national/sustainable-fisheries/fisheries-united-states (Accessed 1 October 2021).
Nawaz, M., Khan, S. A., Tran, Q., Sung, K., Khan, A. A., Adamu, I., et al. (2012). Isolation and characterization of multidrug-resistant Klebsiella spp. isolated from shrimp imported from Thailand. Int. J. Food Microbiol. 155, 179–184. doi: 10.1016/j.ijfoodmicro.2012.02.002
Pang, Z., Raudonis, R., Glick, B. R., Lin, T. J., and Cheng, Z. (2019). Antibiotic resistance in Pseudomonas aeruginosa: mechanisms and alternative therapeutic strategies. Biotechnol. Adv. 37, 177–192. doi: 10.1016/j.biotechadv.2018.11.013
Rodríguez-Martínez, J. M., Poirel, L., and Nordmann, P. (2009). Extended-spectrum cephalosporinases in Pseudomonas aeruginosa. Antimicrob. Agents Chemother. 53, 1766–1771. doi: 10.1128/aac.01410-08
Ryu, S. H., Park, S. G., Choi, S. M., Hwang, Y. O., Ham, H. J., Kim, S. U., et al. (2012). Antimicrobial resistance and resistance genes in Escherichia coli strains isolated from commercial fish and seafood. Int. J. Food Microbiol. 152, 14–18. doi: 10.1016/j.ijfoodmicro.2011.10.003
Sader, H. S., and Jones, R. N. (2012). Antimicrobial activity of daptomycin in comparison to glycopeptides and other antimicrobials when tested against numerous species of coagulase-negative Staphylococcus. Diagn. Microbiol. Infect. Dis. 73, 212–214. doi: 10.1016/j.diagmicrobio.2012.02.005
Schar, D., Klein, E. Y., Laxminarayan, R., Gilbert, M., and Van Boeckel, T. P. (2020). Global trends in antimicrobial use in aquaculture. Sci. Rep. 10:21878. doi: 10.1038/s41598-020-78849-3
Schoenfelder, S. M., Dong, Y., Feßler, A. T., Schwarz, S., Schoen, C., Köck, R., et al. (2017). Antibiotic resistance profiles of coagulase-negative staphylococci in livestock environments. Vet. Microbiol. 200, 79–87. doi: 10.1016/j.vetmic.2016.04.019
Sharma, L., Nagpal, R., Jackson, C. R., Patel, D., and Singh, P. (2021). Antibiotic-resistant bacteria and gut microbiome communities associated with wild-caught shrimp from the United States versus imported farm-raised retail shrimp. Sci. Rep. 11:3356. doi: 10.1038/s41598-021-82823-y
Shaw, K. S., Rosenberg Goldstein, R. E., He, X., Jacobs, J. M., Crump, B. C., and Sapkota, A. R. (2014). Antimicrobial susceptibility of Vibrio vulnificus and Vibrio parahaemolyticus recovered from recreational and commercial areas of Chesapeake Bay and Maryland coastal bays. PLoS One 9:e89616. doi: 10.1371/journal.pone.0089616
Shen, Y., Xu, C., Sun, Q., Schwarz, S., Ou, Y., Yang, L., et al. (2018). Prevalence and genetic analysis of mcr-3-positive Aeromonas species from humans, retail meat, and environmental water samples. Antimicrob. Agents Chemother. 62, e00404–e00418. doi: 10.1128/AAC.00404-18
Smith, P. (2008). Antimicrobial resistance in aquaculture. Rev. Sci. Tech. 27, 243–264. doi: 10.20506/rst.27.1.1799
Tran, Q. T., Nawaz, M. S., Deck, J., Nguyen, K. T., and Cerniglia, C. E. (2011). Plasmid-mediated quinolone resistance in pseudomonas putida isolates from imported shrimp. Appl. Environ. Microbiol. 77, 1885–1887. doi: 10.1128/aem.01176-10
Tuševljak, N., Rajić, A., Waddell, L., Dutil, L., Cernicchiaro, N., Greig, J., et al. (2012). Prevalence of zoonotic Bacteria in wild and farmed aquatic species and seafood: a scoping study, systematic review, and Meta-analysis of published research. Foodborne Pathog. Dis. 9, 487–497. doi: 10.1089/fpd.2011.1063
Tyson, G. H., Li, C., Hsu, C.-H., Bodeis-Jones, S., and McDermott, P. F. (2019). Diverse Fluoroquinolone resistance plasmids From retail Meat E. coli in the United States. Front. Microbiol. 10:02826. doi: 10.3389/fmicb.2019.02826
U.S. Department of Agriculture Economic Research Service (2021). Food Availability Data. Available at: https://www.ers.usda.gov/data-products/food-availability-per-capita-data-system/ (Accessed October 1, 2021).
U.S. Food and Drug Administration (2019). The National Antimicrobial Reistance Monitoring System: NARMS Integrated Report, 2016–2017. (Rockville, MD: U.S. Department of Health and Human Services, Food and Drug Administration).
U.S. Food and Drug Administration (2020a). Advice about Eating Fish. Available at: https://www.fda.gov/Food/ResourcesForYou/Consumers/ucm393070.htm (Accessed October 1, 2021).
U.S. Food and Drug Administration (2020b). Animal Drugs @FDA. Available at: https://animaldrugsatfda.fda.gov/adafda/views/#/search (Accessed 18 August 2020).
U.S. Food and Drug Administration (2021). Approved Aquaculture Drugs. Available at: https://www.fda.gov/animal-veterinary/aquaculture/approved-aquaculture-drugs (Accessed October 8, 2021).
United Nations Statistic Division (2022). Standard country or area codes for statistical use. Available at: https://unstats.un.org/unsd/methodology/m49/ (Accessed 15 October 2021).
Wang, F., Jiang, L., Yang, Q., Han, F., Chen, S., Pu, S., et al. (2011). Prevalence and antimicrobial susceptibility of major foodborne pathogens in imported seafood. J. Food Prot. 74, 1451–1461. doi: 10.4315/0362-028x.Jfp-11-146
Keywords: antimicrobial resistance, seafood, retail food, United States, National Antimicrobial Resistance Monitoring System
Citation: Tate H, Ayers S, Nyirabahizi E, Li C, Borenstein S, Young S, Rice-Trujillo C, Saint Fleurant S, Bodeis-Jones S, Li X, Tobin-D’Angelo M, Volkova V, Hardy R, Mingle L, M’ikanatha NM, Ruesch L, Whitehouse CA, Tyson GH, Strain E and McDermott PF (2022) Prevalence of Antimicrobial Resistance in Select Bacteria From Retail Seafood—United States, 2019. Front. Microbiol. 13:928509. doi: 10.3389/fmicb.2022.928509
Edited by:
Xian-Zhi Li, Health Canada, CanadaReviewed by:
Marisa Haenni, Agence Nationale de Sécurité Sanitaire de l’Alimentation, de l’Environnement et du Travail (ANSES), FranceF. Carl Uhland, Public Health Agency of Canada (PHAC), Canada
Copyright © 2022 Tate, Ayers, Nyirabahizi, Li, Borenstein, Young, Rice-Trujillo, Saint Fleurant, Bodeis-Jones, Li, Tobin-D’Angelo, Volkova, Hardy, Mingle, M’ikanatha, Ruesch, Whitehouse, Tyson, Strain and McDermott. This is an open-access article distributed under the terms of the Creative Commons Attribution License (CC BY). The use, distribution or reproduction in other forums is permitted, provided the original author(s) and the copyright owner(s) are credited and that the original publication in this journal is cited, in accordance with accepted academic practice. No use, distribution or reproduction is permitted which does not comply with these terms.
*Correspondence: Heather Tate, heather.tate@fda.hhs.gov