- 1College of Food Science and Engineering, Ocean University of China, Qingdao, China
- 2State Key Laboratory of Food Science and Technology, China-Canada Joint Laboratory of Food Science and Technology (Nanchang), Key Laboratory of Bioactive Polysaccharides of Jiangxi, Nanchang University, Nanchang, China
- 3Istituto di Scienze e Tecnologie Chimiche “Giulio Natta”, CNR, Milan, Italy
Vibrio species are disseminated broadly in the marine environment. Some of them can cause severe gastroenteritis by contaminating seafood and drinking water, such as Vibrio parahaemolyticus, Vibrio cholerae, and Vibrio vulnificus. However, their pathogenic mechanism still needs to be revealed to prevent and reduce morbidity. This review comprehensively introduces and discusses the common pathogenic process of Vibrio including adhesion, cell colonization and proliferation, and resistance to host immunity. Vibrio usually produces pathogenic factors including hemolysin, type-III secretion system, and adhesion proteins. Quorum sensing, a cell molecular communication system between the bacterial cells, plays an important role in Vibrio intestinal invasion and colonization. The human immune system can limit the virulence of Vibrio or even kill the bacteria through different responses. The intestinal microbiota is a key component of the immune system, but information on its effects on physiological metabolism and pathogenicity of Vibrio is seldom available. In this review, the effects of intestinal microorganisms and their metabolites on the invasion and colonization of common pathogenic Vibrio and VBNC status cells are discussed, which is conducive to finding the next-generation prebiotics. The strategy of dietary intervention is discussed for food safety control. Finally, future perspectives are proposed to prevent Vibrio infection in aquaculture.
Introduction
Vibrio species are disseminated broadly in aquatic environments, including estuaries, marine coastal waters and sediments, and aquaculture settings (Bonnin-Jusserand et al., 2019; Valente and Wan, 2021). Vibrio cholerae, Vibrio parahaemolyticus, and Vibrio vulnificus are the most common pathogenic species that contaminate seafood and drinking water causing heavy food poisoning incidents and serious illness in humans (Thorstenson and Ullrich, 2021). Cholera, a severe disease that is still occasionally prevalent in developing countries, is caused by V. cholerae and transmitted through polluted food and water because of inadequate sanitation of the food and water chain supply (Islam et al., 2004). The infection of V. cholerae remains a global health concern because of the over 100,000 deaths per year by O1 and O139 serogroups (Cho et al., 2021). In human hosts, V. cholerae preferentially colonize the duodenum, producing cholera toxin (CT) and the toxin-coregulated pilus (TCP) for colonization and cellular damage.
Vibrio infection process contains the initial stage in the stomach, tropism to epithelial cell, through mucus layer, and adhesion and proliferation (Figure 1). After Vibrio invades the human intestinal tract, it produces complex and diverse pathogenic factors to ensure the infection of the host. These include thermostable direct hemolysin (TDH), TDH-related hemolysin (TRH), type III secretion system (T3SS), and adhesion proteins (Ritchie et al., 2012; Zhang and Kim, 2013), for example, the multivalent adhesion molecule (MAM) 7 by which the pathogen adheres to the epithelial lining of the small intestine during the early infection of V. parahaemolyticus (Krachler and Orth, 2011). In addition, the infected cells will face complex microecological relationships in the intestinal environment, which seriously affects the growth and colonization of Vibrio. Among them, quorum sensing (QS), the cell-to-cell communication system implemented by signaling molecules (autoinducer) and based on population density, may play one of the most important roles in intestinal colonization and invasion (Gode-Potratz and McCarter, 2011). It is found that QS can regulate adhesion factors of Vibrio, such as T3SS, MAM, and flagella. A previous study revealed the attachment of V. parahaemolyticus to mammalian cells was reduced from 80 to 35% in the absence of MAM-7 (Krachler and Orth, 2011). Therefore, it is significant to find concrete evidence which shows an association between QS and bacterial virulence for Vibrio (Zhang et al., 2012b; Bonnin-Jusserand et al., 2019).
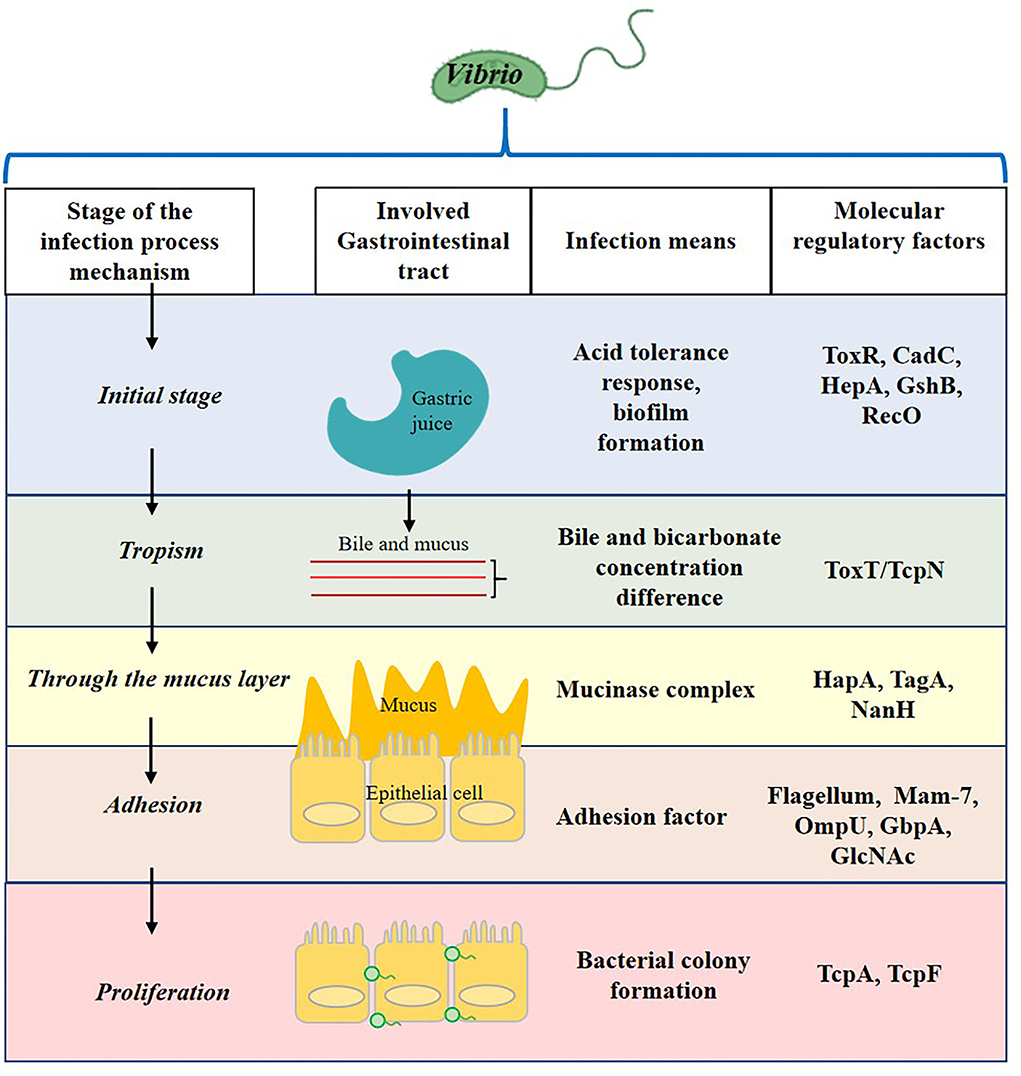
Figure 1. The involved gastrointestinal tract, infection means, and molecular regulatory factors of the Vibrio infection process.
To maintain the balance of intestinal microecology, native intestinal microorganisms will also inhibit and reject the invading microbial cells. Intestinal contents, including intestinal microbial metabolites and tissue secretions, may affect a series of physiological and growth behaviors of Vibrio, which may be completely different from that of pure culture in vitro, especially concerning the expression level of factors related to cell invasion and pathogenicity. The previous study injected V. vulnificus K44 or R41 into mice and found although the two strains induce macrophage apoptosis in vitro, only K44 could escape from the host defense in vivo (Kashimoto et al., 2003). In addition, V. harveyi autoinducer 1 (HAI-1) is the channel of QS. The in vivo experiment revealed it was inactive during infection of brine shrimp, which might suggest this signal had low stability or was not produced in vivo (Defoirdt et al., 2008).
When bacteria are exposed to some environmental stresses, such as low temperature and nutrition starvation, they enter an unusual physiological state in which they cannot grow on common culture media but still perform their cellular activities called viable but non-culturable (VBNC). Some Vibrio species, including V. cholerae, became a special survival form of bacteria (Ayrapetyan and Oliver, 2016). The pathogens, when in the VBNC state, are usually unable to cause disease, but in the human gastrointestinal tract they can restart growing, regain their pathogenic properties, and cause severe illness (Nicolò and Guglielmino, 2012). Thus, the interaction of Vibrio with intestinal microorganisms can trigger the restarting of Vibrio growth.
By now, little is known about the effects of intestinal microorganisms on the physiological metabolism and pathogenicity of Vibrio. In the current review, we focus on the effects of intestinal microbiota and their metabolites on the invasion and colonization ability of common pathogenic Vibrio species. The key gene expression of Vibrio including virulence and adhesion factors and the effects of microbiota on the restarting of VBNC state Vibrio are also discussed. Besides, this review aims to provide some suggestions for dietary intervention for food safety control involving Vibrio contamination and other pathogens.
Pathogenic mechanism of common pathogenic Vibrio species
The Vibrio pathogenic process usually includes adhesion of the pathogen to the intestinal mucus layer, invasion of host tissue, colonization and cell proliferation, and resistance to the host immunity, but V. cholerae is not invasive. During the process, Vibrio cells produce different types of toxins important for the virulence of the pathogen.
During adhesion to the host cell, the TCP, a type IV bundle-forming pilus, is the main colonization factor for the pathogenicity of V. cholerae. It is a polymer of subunits of TcpA, whose C-terminal region is exposed on the surface of pilus fiber to regulate the colonization functions of TCP. The TcpA was expressed in tomatoes to be an antigen for anti-colonization immunity (Sharma et al., 2008). The GbpA is the N-acetylglucosamine-binding protein in V. cholerae, participating in V. cholerae attachment. It has a four-domain structure, and three of them are involved in the colonization process (Wong et al., 2012). Other adhesion factors such as OmpU and MAM7 are also identified in Vibrio species (including non-cholerae ones) and their role depends on the context of the pathogenic process (Liu et al., 2018; Crisan and Hammer, 2020; Ganie et al., 2022). The MAM7, T3SS, and T6SS are the main factors associated with V. cholerae, V. parahaemolyticus, and Vibrio alginolyticus, and T3SS has a little correlation with V. vulnificus (Table 1). After the adhesion, certain Vibrio, including V. cholerae, V. parahaemolyticus, V. vulnificus, Vibrio harveyi, and V. alginolyticus, starts the biofilm formation that is the main step toward the disease development (Ashrafudoulla et al., 2020). In these conditions, gene expression at the stationary phase is regulated by bacterial cell-to-cell communication. The LuxR or its homolog is detected in all studied Vibrio to date and is considered to regulate cps gene expression positively for biofilm formation. Thus, the mature biofilm exhibits a gene expression pattern, which is beneficial for the resistance to environmental stressors (Wang et al., 2011). However, colonization of V. cholerae in the small intestine is negatively affected by the inhibition of motility, which also decreases biofilm formation in vitro (Purdy and Watnick, 2011). Other studies also indicate that biofilm formation of V. cholerae is critical for intestinal colonization (Silva and Benitez, 2016), but information on its in vivo formation is seldom available. The biofilm gene of vps was expressed and essential for intestinal colonization of V. cholerae O139 in Drosophila melanogaster (Blow et al., 2005). However, the mechanism by which flagellar movement affects surface adhesion in vivo is not fully understood. Silva and Benitez (2016) suggest that adhesion can be regulated by flagellar movement and sodium flux of membrane potential. The synergistic effect of flagella and mannose-sensitive hemagglutinin (MSHA) type IV pili of V. cholerae allows a surface interaction to enhance the adhesion (Utada et al., 2014). However, it is difficult to confirm such a mechanism in the detail because of the complex flagellar state in vivo.
The Gram-negative bacteria contain secretory systems that are essential for the invasion. Different secretory systems have been reported in Vibrio including T2SS, T3SS, and T6SS (Zhao et al., 2018). Among them, T2SS of V. vulnificus causes lysis and necrosis of epithelial cells (Jang et al., 2017). By examining 110 Vibrio strains, T3SS1 was detected in V. parahaemolyticus, V. alginolyticus, V. harveyi, and V. campbellii, but T3SS2 was only found in V. parahaemolyticus RIMD2210633 and ATCC33847 (Wu et al., 2020). T3SS2 was reported to be functional in a few V. parahaemolyticus and V. cholerae strains (Miller et al., 2019). T3SS1 mainly affects the biofilm formation, motility, and cytotoxicity and contributes to the survival of Vibrio in the environment, whereas T3SS2 of V. parahaemolyticus is involved in the negative regulation of the cellular inflammatory response, which is conducive to the process of evasion of host immune system by the pathogenic bacteria (Park et al., 2004; Calder et al., 2014). T3SS was reported to be mainly responsible for diarrhea caused by non-O1/O139 V. cholerae but played no role at all in O1/O139 ones (Shin et al., 2011). The V. parahaemolyticus that lack T3SS2 are unable to colonize the intestinal environment of infant rabbits and do not develop the disease symptoms in ligated ileal loops (Ritchie et al., 2012). Further study suggested that T3SS2-lacking V. parahaemolyticus could colonize the intestinal lumen of germ-free mice but it did not cause an obvious inflammatory response (Yang et al., 2019). T6SS, mainly studied in V. cholerae, participates in the adhesion to cultured cell monolayers and injects virulence factors (Ye et al., 2008). T6SS1 is most active under warm marine-like conditions (Salomon et al., 2013), and T6SS2 is active under low salt conditions (Yu et al., 2012).
During the pathogenic process, toxins produced by Vibrio depend on the context, that is, on the species and environments (Table 1). They cause severe symptoms like fever, watery and bloody diarrhea, and vomiting. The CT is the main virulence factor of V. cholerae infections encoded by ctxA and ctxB (Bonnin-Jusserand et al., 2019). Vibrio parahaemolyticus causes enterotoxicity and cytotoxicity due to TDH, TRH, and thermolabile hemolysin (TLH). Vibrio alginolyticus also contains the TDH and TRH like those found in V. parahaemolyticus (Cai et al., 2007). In Vibrio vulnificus, the vvhA gene encodes the toxin protein having hemolytic activity able to not only destroy the host red blood cells and release iron for the use of bacteria but also cause a strong cytotoxic effect that evolves into serious tissue necrosis (Chung et al., 2016). Many Vibrio species including V. cholerae, V. parahaemolyticus, V. vulnificus, and V. alginolyticus contain the toxR gene, which is an ancestral locus in Vibrio (Chen et al., 2012). Other virulence factors, such as urease and lipase, also affect the transcription of virulence genes in various Vibrio species (Baffone et al., 2001).
The colonization of Vibrio occurs by the effect of physical adhesion factors and after the antagonistic interaction between the pathogen with intestinal bacteria. It was proved that TDH facilitates the colonization of V. parahaemolyticus and V. alginolyticus by damaging the intestinal epithelial cells leading to the colonization of bacteria in a large number. Metalloprotease VvpE in V. vulnificus disrupts the intestinal wall by interacting with the intestinal proteins responsible for bacterial pathogenesis and promotes intestinal colonization of the pathogen (Lee et al., 2016).
The QS is cell-to-cell communication by which bacteria coordinate with each other based on cell density (Hawver et al., 2016). During resistance to the host immunity, V. cholerae has the efficient QS mechanism by which the cells communicate to produce pathogenic factors participating in hemolysis, biofilm formation, secretion systems, metabolic fitness, and swarming (Suckow et al., 2011; Shao and Bassler, 2014; Jemielita et al., 2018). QS regulates virulence factors of Vibrio through different bacterial densities. For example, at OD600 values of 0.05 to 0.2, AphA activated the expression of VPA0606 in V. parahaemolyticus to promote biofilm formation and increase bacterial mobility and cytotoxicity. At an OD600 value of 0.2 to 0.4, the transcriptional expression of ToxR is indirectly inhibited by AphA, resulting in reduced cytotoxicity (Zhang et al., 2017). It is known that QS regulates (i) ToxR expression that activates the expression of T3SS and T6SS (Li et al., 2019), (ii) the lateral flagellum genes that influence the movement and colonization of V. parahaemolyticus (Lu et al., 2019), (iii) LuxS that upregulates biofilm formation by stimulating flagella formation and exopolysaccharides production in V. alginolyticus (Ye et al., 2008). SmcR is the QS master regulator in V. vulnificus, which regulates the expression of hemolysin and elastase (Lee et al., 2007). The direct binding of the agent of antivirulence to SmcR was proved to effectively alleviate the virulence of V. vulnificus (Kim et al., 2020). QS and ToxS, as the centers of regulation, can regulate the genes involved in biofilm formation and those related to pathogenic factors to influence the virulence of V. cholerae, V. parahaemolyticus, and V. alginolyticus. In addition, cyclic diguanylate (c-di-GMP) is an allosteric activator that regulates the transition between sessility and motility in V. cholerae and V. vulnificus (Srivastava et al., 2014; Park et al., 2015). In turn, the intracellular concentration of c-di-GMP is regulated by σ54-dependent activator FlrA, biofilm activators VpsR and VpsT (Silva and Benitez, 2016). The elevated c-di-GMP level was reported to promote biofilm formation by V. vulnificus (Park et al., 2015). In V. cholerae, the VieA was reported to control c-di-GMP concentration regulating exopolysaccharide synthesis involved in biofilm formation (Tischler and Camilli, 2004).
Effects of intestinal microbiota on colonization and invasion of pathogenic Vibrio
There are many factors produced by intestinal normal microbiota (e.g., Escherichia coli), opportunistic pathogens (e.g., Pseudomonas aeruginosa and Bacteroides fragile), and probiotics in the intestinal tract that interfere with Vibrio pathogenesis (Gopalakrishnan et al., 2015; He et al., 2017) (Figure 2). Intestinal microbiota plays an important role in the regulation of virulence gene expression including MAM7, TDH, TRH, biofilm, and T6SS in Vibrio (Zhang et al., 2012a). The colonization of V. parahaemolyticus is influenced by the sigma factor of E. coli in the human intestinal tract (Yu et al., 2012). Bacteroides fragile protects the macrophages in the intestine and its incorporation into the intestinal epithelial cells also inhibits V. parahaemolyticus colonization (Li et al., 2017b). The metabolites, including short-chain fatty acids and amino sugars, of intestinal microbiota, were also reported to protect the epithelial cell from colonization and invasion of V. cholerae (Qin et al., 2020).
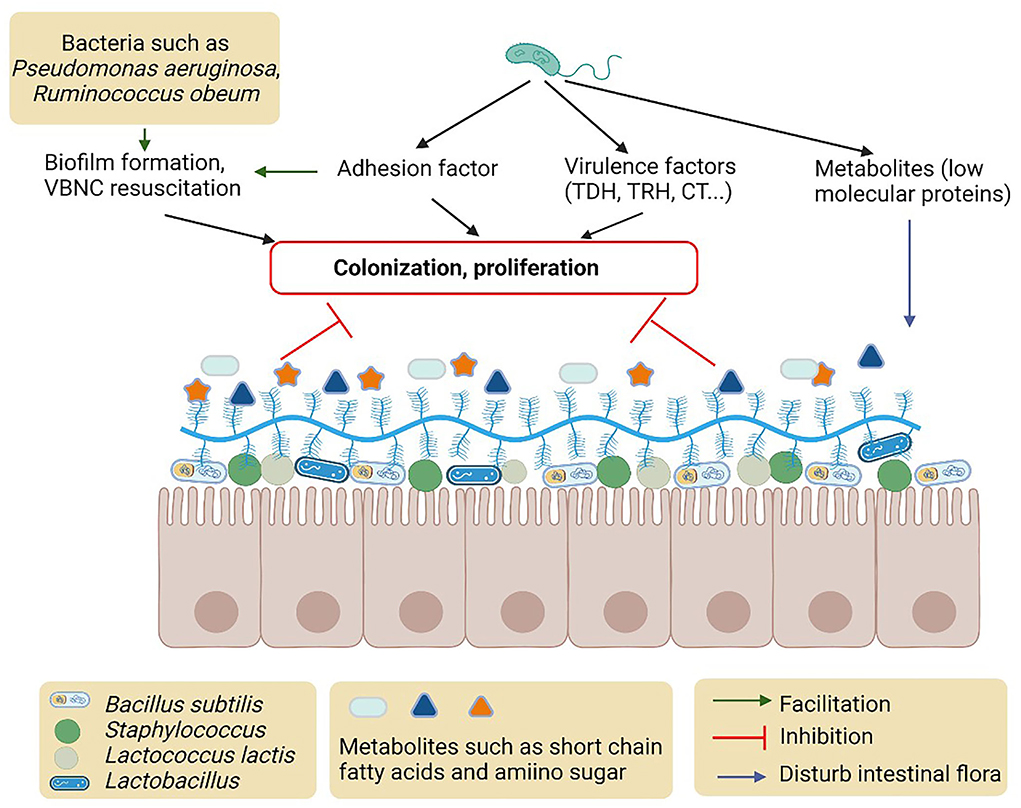
Figure 2. Possible mechanisms and metabolites of intestinal microbiota to inhibit colonization of proliferation of Vibrio.
It is observed that the heterogeneity of the intestinal microbial flora can significantly inhibit the invasion of the Vibrio. The colonization and invasion of Vibrio in the human intestine develop some clinical manifestations including diarrhea, vomiting, and high fever. After Vibrio infection, the intestinal wall will be damaged by the synthesis and secretion of toxic metabolites, and the structure of intestinal flora will be harmful to human health. V. cholerae infected the intestinal flora, while gut bacteria influenced the invasion of the pathogen. In mice, after eliminating much of the mouse gut microbiota by antibiotic treatment for 2 days, the immune response of host cells was reduced due to the change of heterogeneity of the intestinal microbial flora (Zhao et al., 2018). It was found that the microbiota possibly prevents infection of the invading pathogen by activating the host cell defense, competition for nutrients, or sites of adherence (Fukuda et al., 2011; Kamada et al., 2012). Competition for different nutrients by the intestinal normal flora acts as a barrier for the enteric pathogen. Metabolic competition for carbon sources, trace metals, and vitamins by the pathogenic bacteria and microbiota cause colonization resistance. Gut microbial can regulate microbial ecology by absorbing scarce vitamin B12 and inhibiting the growth of pathogens (Degnan et al., 2014).
Intestinal microbiota may also provoke V. cholerae by affecting the expression of T6SS (Zhao et al., 2018). This antagonism brings significant changes in the V. cholerae pathogenicity by enhancing MAM7, T6SS expression, and intestinal colonization. The antagonistic effect of V. cholerae on the intestinal microbiota can improve the pathogen's fitness which can make it easy for the pathogen to infect a new host (Kumar et al., 2020). The antagonism of V. cholerae may be related to the struggle of bacteria for nutrients, citrate utilization, and gluconeogenic action (Gopalakrishnan et al., 2015; Wang et al., 2018). After Vibrio enters the intestine, it inevitably competes with the intestinal microorganisms and even inhibits their growth through the synthesis of metabolites, thus interfering with the original intestinal microecological balance. The metabolites produced by Vibrio have a significant impact on intestinal microbiota. For example, V. alginolyticus primary metabolites may restrict Staphylococcus aureus to grow (Nursyam, 2017).
Effects of QS mediated by intestinal microbiota on Vibrio
Intestinal microbiota also mediates the QS to regulate adhesion, colonization, and invasion of Vibrio (Hsiao et al., 2014) (Figure 2). N-hexanoyl-L-homoserine lactone (AHL) of Pseudomonas aeruginosa affects the biofilm formation of V. parahaemolyticus in zebrafish. Pseudomonas aeruginosa increases the enzymatic activity of superoxide dismutase and lysozyme, the biofilm polysaccharides organization, and helps raise the phagocytic cell of the host immune system (Gopalakrishnan et al., 2015). Vibrio cholerae signaling factor interferes with the QS system of P. aeruginosa influencing the physiological conditions of intestinal microbiota. Upon V. cholerae infection, Ruminococcus obeum exhibits a consistent increase in its relative abundance. Ruminococcus obeum luxS (AI-2) expression increases significantly with V. cholerae invasion and causes QS-mediated repression of V. cholerae colonization factors. Ruminococcus obeum AI-2 reduces V. cholerae colonization and pathogenicity through a novel pathway, LuxP (Hsiao et al., 2014). Furthermore, it has been found that some strains of V. alginolyticus produce QS inhibitors that cause the stoppage of gut microbiota phenotypes of P. aeruginosa (Reina et al., 2019).
Effects of intestinal microorganisms and their metabolites on VBNC state Vibrio
Viable but nonculturable (VBNC) is the state in which the bacteria are unable to grow on the culture media but are still alive. It is considered an adaptive strategy for survival in Vibrio in which the pathogen escapes the unfavorable environment. There are different stress conditions including drying, oxidative stress, radiation disinfection, and antiseptic that cause the VBNC state of the pathogen. At the oxidative stress condition, V. vulnificus ATCC27562 becomes non-culturable (Abe et al., 2006). Vibrio cholerae O1 El Tor strain adopts the VBNC state at 4°C in artificial seawater within 35 days. Vibrio alginolyticus also adopts the VBNC state in the same conditions (Albertini et al., 2010; Xu et al., 2018).
The restarting from the VBNC state into the culturable state is possible when the environmental conditions are favorable, and the bacteria can perform all the normal metabolic activities. Currently, many strategies are tested for the restarting of VBNC bacteria. High temperature (Du et al., 2007), the addition of chemical substances into the environment (Mizunoe et al., 2000), and co-culture with host cells (Senoh et al., 2010) allowed the restarting of V. cholerae O1/O139 and V. parahaemolyticus 23552 from the VBNC state. The bacteria in the VBNC state co-cultured with Chinese hamster ovary cells stimulate the pathogen to recover its normal biological form.
Although there are still many problems to be understood about the recovery mechanism of VBNC bacteria, it has been found that many microbial metabolites play an important role. For example, some pathogens, such as E. coli O157:H7, can recover their active form using their autoinducers produced during biofilm formation (Liu et al., 2009). It is suggested that the metabolic molecule of pyruvate in the medium during the bacterial growth may promote the conversion of culturable to VBNC state (Morishige et al., 2013). However, the metabolic products that act as resuscitative agents remain unknown. Sodium pyruvate may be the key molecule in the resuscitation process of Salmonella enteritidis, which restores the biosynthesis of macromolecules such as DNA and proteins, thereby converting the VBNC cells to a culturable state (Mukamolova et al., 2002). Recently, a recovery-promoting factor (Rpf), as a secretory protein of Micrococcus luteus (Arana et al., 2004), was proved to participate in the restarting of VBNC cells, which may have either autocrine (by the same cells that produce them) or paracrine (may act on the nearby cells) signaling functions.
So far, good progress has been made in the research on the reactivation of VBNC bacteria entering the human intestine, but it remains unclear the role of intestinal microbiota and their metabolites, and the host-bacterial interactions (Li et al., 2014). Therefore, studies that shed light on the effects of intestinal microorganisms and their metabolites on the reactivation of the VBNC state Vibrio are very desirable.
Dietary intervention on Vibrio pathogenicity by regulating the intestinal microbiota
Probiotics and phages
The use of antibiotics and chemotherapeutic agents helps to control infections in the breeding industry, but they also cause the development of drug-resistant bacteria and food-safety related problems (Giri et al., 2015; Zhang et al., 2020b). Probiotics are considered a sustainable and promising solution because they are environment-friendly and help host growth, immunity, and disease control (Lazado and Caipang, 2014). Therefore, the use of probiotics in treating diseases of livestock is increasing. The consumption of probiotics and their functionally active substances improve the reasonable gut microbiota structure, which may help in the inhibition of the invasion of Vibrio (Table 2). The harmful effects of V. parahaemolyticus in mice can be minimized by taking probiotics such as Streptomyces and Lactobacillus in Litopenaeus vannamei, which enhance the intestinal microbiota (Reina et al., 2019). Also, the adhesion of V. parahaemolyticus can be lowered by the intake of probiotics (Satish Kumar et al., 2011). A significant reduction of Vibrio spp. was observed in the Pacific white shrimp after feeding it with microencapsulated probiotics, Bacillus subtilis, or Staphylococcus lactis (Boonanuntanasarn et al., 2016). The shrimp diet supplemented with Lactococcus lactis can increase resistance to V. alginolyticus and increase Lactobacillus and Bacillus count in the shrimp intestine and reduces the number of Vibrio species (Adel et al., 2017). The number of intestinal bacteria can be increased by Halomonas B12 which causes a significant reduction in Vibrio spp. bacterial count in Chinese shrimp (Zhang et al., 2009).
The types of microecological agents used to control intestinal microbiota are expanding. The addition of yeast and fermented vegetable product by Lactobacillus to the diet of Kuruma shrimp can boost the immunity resistance to V. parahaemolyticus (Elshopakey et al., 2018). In sea cucumber, V. alginolyticus, V. splendidus, and V. cyclitrophicus can be reduced by mixing different phage mixtures in a certain proportion, but in the experimental group treated with antibiotics, the number of Vibrio has not changed significantly (Li et al., 2020).
Carbohydrates
Diet plays an important role in the regulation of the intestinal microbiota (Krachler and Orth, 2011). Diarrheal disease is mainly treated through oral rehydration therapy comprising glucose. However, the virulence gene expression and toxin production were enhanced in a glucose-concentration manner. Instead, the rice-based oral rehydration therapy decreased the virulence determinants (Kühn et al., 2014). In addition, it is widely accepted that intestinal microbiota can be improved by dietary prebiotics (Boonanuntanasarn et al., 2016), and some oligosaccharides are effective in the anti-adhesion of pathogens in vitro and in vivo studies (Ofek et al., 2003). For example, the intestinal microbiota is improved by mannan oligosaccharide (MOS) in trout and causes the reduction of Vibrio (Dimitroglou et al., 2009). The L. vannamei fed with xylose oligosaccharide shows a significantly increase survival rate against the challenge of V. alginolyticus (Sun et al., 2019). Similarly, mice fed with chitosan show a higher survival rate than the control after infection with V. vulnificus (Lee et al., 2009).
It was reported that in the shrimp intestine, the bacterial count of normal gut microbiota significantly increased and the bacterial count of V. alginolyticus decreased with symbiotic supplemented dietary. Bacillus licheniformis and B. subtilis in the presence of isomalto-oligosaccharides in the feed increase resistance to V. alginolyticus, showing lower mortality in shrimps infected with V. alginolyticus compared to the control group (Zhang et al., 2011).
Vibrio cholerae adhesion to human intestinal epithelial cell line HT-29 can be significantly inhibited by pectin oligosaccharides (Wang et al., 2015). Human milk containing fucosylated oligosaccharides and sialic acid oligosaccharides can inhibit V. cholerae ATCC14034 adhesion to Caco-2 (Coppa et al., 2006). Beyond oligosaccharides, bacterial adhesion can also be inhibited by some monosaccharides (Wang et al., 2015).
Proteins
Vibrio is a very common pathogenic bacteria in aquaculture animals, and the use of vegetable protein raw materials, such as soybean meal, to replace fish meal has become one of the most important measures to reduce the cost of the industry. Therefore, the impact of different types of protein raw materials on intestinal microorganisms of aquacultural animals and the pathogenicity of Vibrio has become common concerns of the aquaculture industry. It is most common to regulate the intestinal microbiota of animals by diet in the breeding industry. Moreover, some studies also suggested that enrichment of diet with fermentation, probiotics, and trace elements can improve the resistance to pathogens by strengthening the immune system of aquatic animals (Siddik et al., 2019a). Resistance of European seabass to V. anguillarum was increased with a fish feeding prepared with fish oil and fish meal mixed in different proportions by enhancing the intestinal microbiota (Torrecillas et al., 2017). Previous studies found that the Aeromonas count was significantly reduced and Lactobacillus and Streptococcus were enhanced by adding fermented poultry by-product meal to fish meal, while the survival rate of the fish was increased after V. mimicus infection (Siddik et al., 2019a). The addition of tuna hydrolysate to the diet of juvenile Barramundi can increase resistance against V. harveyi infection in fish (Siddik et al., 2019b) and adding peptides also restricts Vibrio growth and increase host resistance to V. parahaemolyticus (Liao et al., 2019). Studies show that glycinin does not affect the normal microbial community of the fish intestine after feeding, but it significantly reduces the Vibrio count (Li et al., 2017a). Rainbow trout fed with soybean meal for 16 weeks have a low number of Vibrio spp. in the gut compared to those fed with the fish meal (Dimitroglou et al., 2009).
Lipids and organic acids
Lipid is the main component of food and an important energy source providing essential fatty acids for the body. In the current situation which bans antibiotics, plant essential oil attracts the interest of the aquaculture industry because of its significant bactericidal effect in vitro. Previous studies show that oregano essential oil can be used as a substitute for antibiotics because of its antibacterial activity, helping the body to prevent infections (Zhang et al., 2020a). It improves the intestinal bacteria of the animals and enhances the resistance of animals to Vibrio, the reduction of Vibrio, and increase of genera Propionibacterium, Brevinema, and Cotynebacterium were observed in the intestine of fish (Zhang et al., 2020a). The growth of V. vulnificus, V. parahaemolyticus, and V. cholerae can be significantly reduced in shrimp fed with oregano essential oil (Gracia-Valenzuela et al., 2014).
The antibacterial and intestinal repairing properties of some short-chain esters have also been observed. A study shows that the growth of V. cholerae ATCC25870 in the gut of Streptomycin-treated mice fed with monoglyceride was 1,000 times reduced in comparison with those fed with a normal diet (Petschow et al., 1998). The shrimp Penaeus vannamei fed with gelatinized polyhydroxy butyrate for 60 days acquired a 100% survival rate when infected with V. parahaemolyticus (Kiran et al., 2020).
Besides, high content of n-6 polyunsaturated fatty acids in the diet can help to strengthen body immunity. A notable reduction is a mortality of Atlantic salmon against the infection of V. anguillarum when sunflower oil was given in the diet (Bransden et al., 2003). Fat-soluble small molecules have also been shown to improve intestinal microbiota. Thymol and carvacrol have antibacterial properties when mixed in a certain ratio and can change the intestinal microbiota of tilapia (Ran et al., 2016).
To minimize the use of antibiotics and to improve the health in aquaculture, the aquafeed is supplemented with different organic acids (Wassef et al., 2020). The addition of organic acid to the diet can inhibit the growth of V. cholerae, V. harveyi, V. parahaemolyticus, V. vulnificus, V. alginolyticus, and V. campbellii and enhance the intestinal microbiota (da Silva et al., 2016). For example, the Vibrio spp. count significantly decreased when the diet was supplemented with apple cider vinegar and propionic acid (Pourmozaffar et al., 2019). After consuming organic acids and essential oils, the intestinal microbiota can diversify and be enriched in P. vannamei. Also, it was observed that the Lactobacillus growth was promoted and the resistance of shrimps to V. parahaemolyticus increased (He et al., 2017). The intestinal flora of the European seabass was enhanced when fed with sodium propionate, accompanied by a significant reduction of Vibrio spp. (Wassef et al., 2020). The consumption of citric acid also results in the reduction of Vibrio spp. in the Turbot gut (Chen et al., 2018).
Dietary intervention is a promising strategy to reduce the Vibrio pathogenicity in aquaculture. The antibiotic resistance of Vibrio impels the development of probiotics, phages, and other active compounds for reducing high morbidity. Such dietary intervention is usually involved in Vibrio colonization, nutrient competition, and virulence gene expression by regulating the intestinal microbiota. However, the Vibrio species in aquatic environments are different, and the intervention mechanism is discriminatory according to the aquatic livestock. Therefore, the rational design of dietary intervention is required based on the clear pathogenic mechanism of Vibrio species on specific livestock. The reagent dosage and safety are other factors to consider for the economic feasibility of aquaculture.
Challenges and future perspectives
Nowadays, the mechanism of Vibrio pathogenicity has been explored by controlling the environmental variables, including temperature, salinity, and host organisms. However, several underlying mechanisms, such as biofilm formation and propagation mode worldwide, remain unclear, which is possibly due to the specificity of different Vibrio species during the pathogenic process. They differ in colonization and toxicity to the same host and environment. Therefore, network analysis and database establishment are beneficial to extend the technology application for preventing Vibrio in aquaculture. In addition, Vibrio pathogenicity to host organisms is a dynamic process involving energy and substance metabolism networks. To accurately elucidate the pathogenic mechanism, the combination of metabolic tools (such as multi-omics analysis) and experimental animal models is a promising systematic strategy. The multi-omics analysis including transcriptomics, metabolomics, and proteomics can provide the information involved in molecular mechanisms during the pathogenic process.
Besides, too many scientific problems including the exact mechanism by which the intestinal commensal bacteria interfere with the virulence gene expression are not well understood. To better control intestinal diseases caused by Vibrio and ensure intestinal health and food safety, studies that shed light on the complex mechanism of Vibrio after entering the intestine must be pursued. The new models of human gut microbiomes with Vibrio are needed to find the candidate probiotics. The oral vaccines or probiotics exhibit different efficacies in human populations, which are mainly ascribed to the gut microbiome. The probiotics can be applied in aquaculture and the food industry to improve food safety by enhancing the host defense system. Finally, once the infection occurs in aquaculture, the efficient detection of Vibrio species is significant for alleviating the infection spread to reduce economic losses. Therefore, the development of quick and easy-to-operate detection methods is essential in future studies.
Conclusion
Until now, numerous studies on the pathogenicity of Vibrio were conducted. According to the current understanding, the pathogenic factors of Vibrio include TDH and TRH, type III secretion systems, and adhesion factors. Moreover, when the pathogen achieves an appropriate number of bacterial cell density, the QS mechanism is activated by the expression of these virulence factors. However, the depth and breadth of the current research on the differences between the specific mechanisms of growth, colonization, infection, and pathogenicity of Vibrio invading the intestinal environment and the in vitro environment are still insufficient. With increasing attention paid to the intestinal microecological environment, especially the role of intestinal microbiota for the immune system, the interaction between Vibrio and intestinal microorganisms is undoubtedly an important direction to deepen our understanding of the pathogenicity of Vibrio. After Vibrio invades the intestinal tract, it may disturb the normal ecological balance of the intestinal tract and may cause intestinal wall damage or dysfunction through pathogenic factors. At the same time, intestinal microbiota tries to prevent the growth of the pathogen in different ways including colonization resistance, competition for nutrients, antibiotic production, and resistance to adhesion of the pathogen to the mucus membrane and enhancing the immunity of the host to the pathogen.
Author contributions
HS: conceptualization, supervision, and writing—review and editing. CZ: investigation, supervision, and writing—review and editing. XF: writing—review and editing. SK, JW, and ZL: investigation and writing—review. QK: editing. HM: conceptualization, supervision, funding acquisition, and writing—review and editing. FS: conceptualization and writing—review and editing. All authors contributed to the article and approved the submitted version.
Funding
This work was supported by the National Key Research and Development (R&D) Program of China (2017YFC1600703).
Conflict of interest
The authors declare that the research was conducted in the absence of any commercial or financial relationships that could be construed as a potential conflict of interest.
Publisher's note
All claims expressed in this article are solely those of the authors and do not necessarily represent those of their affiliated organizations, or those of the publisher, the editors and the reviewers. Any product that may be evaluated in this article, or claim that may be made by its manufacturer, is not guaranteed or endorsed by the publisher.
References
Abe, A., Ohashi, E., Ren, H., Hayashi, T., and Endo, H. (2006). Isolation of a viable but non-culturable suppression mutant of Vibrio vulnificus: role of antioxidant enzymes in surviving stationary phase and low temperatures. Fish. Sci. 72, 656–664. doi: 10.1111/j.1444-2906.2006.01196.x
Adel, M., El-Sayed, A., Yeganeh, S., Dadar, M., and Giri, S. S. (2017). Effect of potential probiotic Lactococcus lactis subsp. lactis on growth performance, intestinal microbiota, digestive enzyme activities, and disease resistance of Litopenaeus vannamei. Probiotics Antimicrob. Proteins 9, 150–156. doi: 10.1007/s12602-016-9235-9
Albertini, M. C., Accorsi, A., Teodori, L., Pierfelici, L., and Citterio, B. (2010). Use of multiparameter analysis for Vibrio alginolyticus viable but nonculturable state determination. Cytom. Part A. 69A, 260–265. doi: 10.1002/cyto.a.20263
Arana, I., Seco, C., Epelde, K., Muela, A., Fernández-Astorga, A., Barcina, I., et al. (2004). Relationships between Escherichia coli cells and the surrounding medium during survival processes. Anton. Leeuw. 86, 189–199. doi: 10.1023/B:ANTO.0000036146.28808.93
Ashrafudoulla, M., Mizan, M. F. R., Park, S. H., and Ha, S. D. (2020). Current and future perspectives for controlling vibrio biofilms in the seafood industry: a comprehensive review. Crit. Rev. Food Sci. 61, 1827–1851. doi: 10.1080/10408398.2020.1767031
Ayrapetyan, M., and Oliver, J. D. (2016). The viable but non-culturable state and its relevance in food safety. Curr. Opin. Food Sci. 8, 127–133. doi: 10.1016/j.cofs.2016.04.010
Baffone, W., Citterio, B., Vittoria, E., Casaroli, A., Pianetti, A., Campana, R., et al. (2001). Determination of several potential virulence factors in Vibrio spp. isolated from sea water. Food Microbiol. 18, 479–488. doi: 10.1006/fmic.2001.0441
Blow, N. S., Salomon, R. N., Garrity, K., Reveillaud, I., Kopin, A., Jackson, F. R., et al. (2005). Vibrio cholerae infection of Drosophila melanogaster mimics the human disease cholera. PLoS Pathogens 1, e8. doi: 10.1371/journal.ppat.0010008
Bonnin-Jusserand, M., Copin, S., Le Bris, C., Brauge, T., Gay, M., Brisabois, A., et al. (2019). Vibrio species involved in seafood-borne outbreaks (Vibrio cholerae, V. parahaemolyticus and V. vulnificus): review of microbiological versus recent molecular detection methods in seafood products. Crit. Rev. Food Sci. 59, 597–610. doi: 10.1080/10408398.2017.1384715
Boonanuntanasarn, S., Wongsasak, U., Pitaksong, T., and Chaijamrus, S. (2016). Effects of dietary supplementation with β-glucan and synbiotics on growth, haemolymph chemistry, and intestinal microbiota and morphology in the Pacific white shrimp. Aquacult. Nutr. 22, 837–845. doi: 10.1111/anu.12302
Bransden, M. P., Carter, C. G., and Nichols, P. D. (2003). Replacement of fish oil with sunflower oil in feeds for Atlantic salmon (Salmo salar L.): effect on growth performance, tissue fatty acid composition and disease resistance. Comp. Biochem. Phys, Part B. 135, 611–625. doi: 10.1016/S1096-4959(03)00143-X
Cai, S. H., Wu, Z. H., Jian, J. C., and Lu, Y. S. (2007). Cloning and expression of gene encoding the thermostable direct hemolysin from Vibrio alginolyticus strain HY9901, the causative agent of vibriosis of crimson snapper (Lutjanus erythopterus). J. Appl. Microbiol. 103, 289–296. doi: 10.1111/j.1365-2672.2006.03250.x
Calder, T., Santos, M., Attah, V., Klimko, J., and Orth, K. (2014). Structural and regulatory mutations in Vibrio parahaemolyticus type III secretion systems display variable effects on virulence. FEMS. Microbiol. Lett. 361, 107–114. doi: 10.1111/1574-6968.12619
Chen, C., Wang, Q. B., Liu, Z. H., Zhao, J. J., Jiang, X., Sun, H. Y., et al. (2012). Characterization of role of the toxR gene in the physiology and pathogenicity of Vibrio alginolyticus. Anton. Leeuw. 101, 281–288. doi: 10.1007/s10482-011-9632-8
Chen, Z., Zhao, S., Liu, Y., Yang, P., Ai, Q., Zhang, W., et al. (2018). Dietary citric acid supplementation alleviates soybean meal-induced intestinal oxidative damage and micro-ecological imbalance in juvenile turbot, Scophthalmus maximus L. Aquac. Res. 49, 3804–3816. doi: 10.1111/are.13847
Cho, J. Y., Liu, R., Macbeth, J. C., and Hsiao, A. (2021). The interface of Vibrio cholerae and the gut microbiome. Gut Microbes 13, 1937015. doi: 10.1080/19490976.2021.1937015
Chung, H. Y., Kim, Y. T., Kim, S., Na, E. J., Ku, H. J., Lee, K. H., et al. (2016). Complete genome sequence of Vibrio vulnificus FORC_017 isolated from a patient with a hemorrhagic rash after consuming raw dotted gizzard shad. Gut Pthog. 8, 22. doi: 10.1186/s13099-016-0104-6
Church, S. R., Lux, T., Baker-Austin, C., Buddington, S. P., and Michell, S. L. (2016). Vibrio vulnificus type 6 secretion system 1 contains anti-bacterial properties. PLoS ONE 11, e0165500. doi: 10.1371/journal.pone.0165500
Coppa, G. V., Zampini, L., Galeazzi, T., Facinelli, B., Ferrante, L., Capretti, R., et al. (2006). Human milk oligosaccharides inhibit the adhesion to caco-2 cells of diarrheal pathogens: Escherichia coli, Vibrio cholerae, and Salmonella fyris. Pediatr. Res. 59, 377–382. doi: 10.1203/01.pdr.0000200805.45593.17
Crisan, C. V., and Hammer, B. K. (2020). The Vibrio cholerae type VI secretion system: toxins, regulators and consequences. Environ. Microbiol. 22, 4112–4122. doi: 10.1111/1462-2920.14976
da Silva, A., Vieira, B. C., Mouriño, F. D., Bolivar, J. L. P. N., and Seiffert, W. Q. (2016). Butyrate and propionate improve the growth performance of Litopenaeus vannamei. Aquac. Res. 47, 612–623. doi: 10.1111/are.12520
Defoirdt, T., Boon, N., Sorgeloos, P., Verstraete, W., and Bossier, P. (2008). Quorum sensing and quorum quenching in Viabrio harveyi: lessons learned from in vivo work. ISME J. 2, 19–26. doi: 10.1038/ismej.2007.92
Degnan, P., Taga, E., and Goodman, A. (2014). Vitamin B12 as a modulator of gut microbial ecology. Cell Metab. 20, 769–778. doi: 10.1016/j.cmet.2014.10.002
Dimitroglou, A., Merrifield, D. L., Moate, R., Davies, S. J., Spring, P., Sweetman, J., et al. (2009). Dietary mannan oligosaccharide supplementation modulates intestinal microbial ecology and improves gut morphology of rainbow trout, Oncorhynchus mykiss (Walbaum). J. Anim. Sci. 87, 3226–3234. doi: 10.2527/jas.2008-1428
Du, M., Chen, J., Zhang, X., Li, A., Li, Y., Wang, Y., et al. (2007). Retention of virulence in a viable but nonculturable Edwardsiella tarda isolate. Appl. Environ. Microb. 73, 1349–1354. doi: 10.1128/AEM.02243-06
Elshopakey, G. E., Risha, E. F., Abdalla, O. A., Okamura, Y., Harada, S., Kishida, S., et al. (2018). Efficacy of dietary fermented vegetable product on immune response, up-regulation of immune-related genes and protection of kuruma shrimp (Marsupenaeus japonicus) against Vibrio parahaemolyticus. Aquaculture 497, 431–439. doi: 10.1016/j.aquaculture.2018.08.013
Fukuda, S., Toh, H., Hase, K., Oshima, K., Nakanishi, Y., Yoshimura, K., et al. (2011). Bifidobacteria can protect from enteropathogenic infection through production of acetate. Nature 469, 543–547. doi: 10.1038/nature09646
Ganie, H. A., Choudhary, A., and Baranwal, S. (2022). Structure, regulation, and host interaction of outer membrane protein U (OmpU) of Vibrio species. Microb. Pathogenesis 162, 105267. doi: 10.1016/j.micpath.2021.105267
Giri, S. S., Sen, S. S., Chi, C., Kim, H. J., Yun, S., Park, S. C., et al. (2015). Effect of guava leaves on the growth performance and cytokine gene expression of Labeo rohita and its susceptibility to Aeromonas hydrophila infection. Fish Shellfish Immunol. 46, 217–224. doi: 10.1016/j.fsi.2015.05.051
Gode-Potratz, C. J., and McCarter, L. L. (2011). Quorum sensing and silencing in Vibrio parahaemolyticus. J. Bacteriol. 193, 4224–4237. doi: 10.1128/JB.00432-11
Gopalakrishnan, V., Rengarajan, J., and Chen, J. C. (2015). N-hexanoyl-L-homoserine lactone-degrading Pseudomonas aeruginosa PsDAHP1 protects zebrafish against Vibrio parahaemolyticus infection. Fish Shellfish Immunol. 42, 204–212. doi: 10.1016/j.fsi.2014.10.033
Gracia-Valenzuela, M. H., Vergara-Jiménez, M. J., Baez-Flores, M. E., and Cabrera-Chavez, F. (2014). Antimicrobial effect of dietary oregano essential oil against Vibrio bacteria in shrimps. Arch. Biol. Sci. 66, 1367–1370. doi: 10.2298/ABS1404367G
Hawver, L. A., Jung, S. A., and Ng, W. L. (2016). Specificity and complexity in bacterial quorum-sensing systems. FEMS Microbiol. Rev. 40, 738–752. doi: 10.1093/femsre/fuw014
He, W., Rahimnejad, S., Wang, L., Song, K., Lu, K., Zhang, C., et al. (2017). Effects of organic acids and essential oils blend on growth, gut microbiota, immune response and disease resistance of Pacific white shrimp (Litopenaeus vannamei) against Vibrio parahaemolyticus. Fish Shellfish Immun. 70, 164–173. doi: 10.1016/j.fsi.2017.09.007
Hsiao, A., Ahmed, A. M., Subramanian, S., Griffin, N. W., Drewry, L. L., Petri, W. A., et al. (2014). Members of the human gut microbiota involved in recovery from Vibrio cholerae infection. Nature 515, 423–426. doi: 10.1038/nature13738
Islam, M. S., Mahmuda, S., Morshed, M. G., Bakht, H. B., Khan, M. N., Sack, R. B., et al. (2004). Role of cyanobacteria in the persistence of Vibrio choterae O139 in saline microcosms. Can. J. Microbiol. 50, 127–131. doi: 10.1139/w03-114
Jang, K. K., Lee, Z. W., Kim, B., Jung, Y. H., Han, H. J., Kim, M. H., et al. (2017). Identification and characterization of Vibrio vulnificus plp Aencoding a phospholipase A2 essential for pathogenesis. J. Biol. Chem. 292, 17129–17143. doi: 10.1074/jbc.M117.791657
Jemielita, M., Wingreen, N. S., and Bassler, B. L. (2018). Quorum sensing controls Vibrio cholerae multicellular aggregate formation. Elife 7, e42057. doi: 10.7554/eLife.42057.044
Kamada, N., Kim, Y. G., Sham, H. P., Vallance, B. A., Puente, J. L., Martens, E. C., et al. (2012). Regulated virulence controls the ability of a pathogen to compete with the gut microbiota. Science 336, 1325–1329. doi: 10.1126/science.1222195
Kashimoto, T., Ueno, S., Hanajima, M., Hayashi, H., Akeda, Y., Miyoshi, S., et al. (2003). Vibrio vulnificus induces macrophage apoptosis in vitro and in vivo. Infect. Immun. 71, 533–535. doi: 10.1128/IAI.71.1.533-535.2003
Kaur, S., Sharma, P., Kalia, N., Singh, J., and Kaur, S. (2018). Anti-biofilm properties of the fecal probiotic lactobacilli against vibrio spp. Front. Cell. Infect. Microbiol. 8, 120. doi: 10.3389/fcimb.2018.00120
Kim, S. M., Park, J., Kim, M. S., Song, H., Jo, A., Park, H., et al. (2020). Phenotypic discovery of an antivirulence agent against Vibrio vulnificus via modulation of quorum-sensing regulator SmcR. ACS Infect. Dis. 6, 3076–3082. doi: 10.1021/acsinfecdis.0c00587
Kiran, G. S., Priyadharshini, S., Sajayan, A., Ravindran, A., Priyadharshini, G. B., Ramesh, U., et al. (2020). Dietary administration of gelatinised polyhydroxybutyrate to Penaeus vannamei improved growth performance and enhanced immune response against Vibrio parahaemolyticus. Aquaculture 517, 734773. doi: 10.1016/j.aquaculture.2019.734773
Krachler, A. M., and Orth, K. (2011). Functional characterization of the interaction between bacterial adhesin multivalent adhesion molecule 7 (MAM7) protein and its host cell ligands. J. Biol. Chem. 286, 38939–38947. doi: 10.1074/jbc.M111.291377
Kühn, J., Finger, F., Bertuzzo, E., Borgeaud, S., Gatto, M., Rinaldo, A., et al. (2014). Glucose- but not rice-based oral rehydration therapy enhances the production of virulence determinants in the human pathogen Vibrio cholerae. PLoS Negl. Trop. Dis. 8, e3347. doi: 10.1371/journal.pntd.0003347
Kumar, A., Das, B., and Kumar, N. (2020). Vibrio Pathogenicity island-1: the master determinant of cholera pathogenesis. Front. Cell. Infect. Microbiol. 10, 561296. doi: 10.3389/fcimb.2020.561296
Lazado, C. C., and Caipang, C. M. A. (2014). Atlantic cod in the dynamic probiotics research in aquaculture. Aquaculture 424, 53–62. doi: 10.1016/j.aquaculture.2013.12.040
Lee, B. C., Kim, M. S., Choi, S. H., Kim, K. Y., and Kim, T. S. (2009). In vitro and in vivo antimicrobial activity of water-soluble chitosan oligosaccharides against Vibrio vulnificus. Int. J. Mol. Med. 24, 327–333. doi: 10.3892/ijmm_00000236
Lee, J. H., Rhee, J. E., Park, U., Ju, H. M., Lee, B. C., Kim, T. S., et al. (2007). Identification and functional analysis of Vibrio vulnificus SmcR, a novel global regulator. J Microbiol. Biotechnol. 17, 325–334. Available online at: https://www.ncbi.nlm.nih.gov/pubmed/18051765
Lee, S. J., Jung, Y. H., Ryu, J. M., Jang, K. K., Choi, S. H., Han, H. J., et al. (2016). VvpE mediates the intestinal colonization of Vibrio vulnificus by the disruption of tight junctions. Int. J. Med. Microbiol. 306, 10–19. doi: 10.1016/j.ijmm.2015.10.006
Li, L., Mendis, N., Trigui, H., Oliver, J. D., and Faucher, S. P. (2014). The importance of the viable but non-culturable state in human bacterial pathogens. Front. Microbiol. 5, 258. doi: 10.3389/fmicb.2014.00258
Li, L., Meng, H., Gu, D., Li, Y., and Jia, M. (2019). Molecular mechanisms of Vibrio parahaemolyticus pathogenesis. Microbiol. Res. 222, 43–51. doi: 10.1016/j.micres.2019.03.003
Li, Y., Yang, P., Zhang, Y., Ai, Q., Xu, W., Zhang, W., et al. (2017a). Effects of dietary glycinin on the growth performance, digestion, intestinal morphology and bacterial community of juvenile turbot, Scophthalmus maximus L. Aquaculture 479, 125–133. doi: 10.1016/j.aquaculture.2017.05.008
Li, Z., Deng, H., Zhou, Y., Tan, Y., Wang, X., Han, Y., et al. (2017b). Bioluminescence imaging to track bacteroides fragilis inhibition of Vibrio parahaemolyticus infection in mice. Front. Cell. Infect. Microbiol. 7, 170. doi: 10.3389/fcimb.2017.00170
Li, Z., Ren, H., Li, Q., Murtaza, B., Li, X., Zhang, J., et al. (2020). Exploring the effects of phage cocktails in preventing Vibrio infections in juvenile sea cucumber (Apostichopus japonicus) farming. Aquaculture 515, 734599. doi: 10.1016/j.aquaculture.2019.734599
Liao, X. Z., Hu, S. K., Wang, B., Qin, H. P., Zhao, J. C., He, Z. H., et al. (2019). Dietary supplementation with polypeptides improved growth performance, antibacterial immune and intestinal microbiota structure of Litopenaeus vannamei. Fish Shellfish Immun. 92, 480–488. doi: 10.1016/j.fsi.2019.06.033
Liu, M., Yang, S. S., Zheng, C. K., Luo, X. S., Bei, W. C., Cai, P., et al. (2018). Binding to type I collagen is essential for the infectivity of Vibrio parahaemolyticus to host cells. Cell. Microbiol. 20, e12856. doi: 10.1111/cmi.12856
Liu, Y., Wang, C., Tyrrell, G., Hrudey, S. E., and Li, X. F. (2009). Induction of Escherichia coli O157:H7 into the viable but non-culturable state by chloraminated water and river water, and subsequent resuscitation. Environ. Microbiol. Rep. 1, 155–161. doi: 10.1111/j.1758-2229.2009.00024.x
Lu, R., Tang, H., Qiu, Y., Yang, W., Yang, H., Zhou, D., et al. (2019). Quorum sensing regulates the transcription of lateral flagellar genes in Vibrio parahaemolyticus. Future Microbiol. 14, 1043–1053. doi: 10.2217/fmb-2019-0048
Miller, K. A., Tomberlin, K. F., and Dziejman, M. (2019). Vibrio variations on a type three theme. Curr. Opin. Microbiol. 7, 66–73. doi: 10.1016/j.mib.2018.12.001
Mizunoe, Y., Wai, S. N., Ishikawa, T., Takade, A., and Yoshida, S. I. (2000). Resuscitation of viable but nonculturable cells of Vibrio parahaemolyticus induced at low temperature under starvation. FEMS Microbiol. Lett. 186, 115–120. doi: 10.1111/j.1574-6968.2000.tb09091.x
Morishige, Y., Fujimori, K., and Amano, F. (2013). Differential resuscitative effect of pyruvate and its analogues on VBNC (viable but non-culturable) Salmonella. Microbes Environ. 28, 180–186. doi: 10.1264/jsme2.ME12174
Mukamolova, G. V., Turapov, O. A., Kazarian, K., Telkov, M., Kaprelyants, A. S., Kell, D. B., et al. (2002). The rpf gene of Micrococcus luteus encodes an essential secreted growth factor. Mol. Microbiol. 46, 611–621. doi: 10.1046/j.1365-2958.2002.03183.x
Nicolò, M. S., and Guglielmino, S. P. P. (2012). “Viable but nonculturable bacteria in food,” in: Public Health–Methodology, Environmental and Systems Issues, ed. J. Maddock (Rjeka: InTech), 189–216.
Nursyam, H. (2017). Antibacterial activity of metabolites products of Vibrio alginolyticus isolated from Sponge haliclona sp. against Staphylococcus aureus. Ital. J. Food Saf. 6, 6237. doi: 10.4081/ijfs.2017.6237
Ofek, I., Hasty, D. L., and Sharon, N. (2003). Anti-adhesion therapy of bacterial diseases: prospects and problems. FEMS Immunol. Med. Microbiol. 38, 181–191. doi: 10.1016/S0928-8244(03)00228-1
Park, J. H., Lim, J. G., and Choi, S. H. (2015). Effects of elevated intracellular cyclic di-GMP levels on biofilm formation and transcription profiles of Vibrio vulnificus. 24, 771–776. doi: 10.1007/s10068-015-0100-5
Park, K. S., Ono, T., Rokuda, M., Jang, M. H., Okada, K., Iida, T., et al. (2004). Functional characterization of two type III secretion systems of Vibrio parahaemolyticus. Infect. Immun. 72, 6659–6665. doi: 10.1128/IAI.72.11.6659-6665.2004
Petschow, B. W., Batema, R. P., Talbott, R. D., and Ford, L. L. (1998). Impact of medium-chain monoglycerides on intestinal colonisation by Vibrio cholerae or enterotoxigenic Escherichia coli. J. Med. Microbiol. 47, 383–389. doi: 10.1099/00222615-47-5-383
Pourmozaffar, S., Hajimoradloo, A., Paknejad, H., and Rameshi, H. (2019). Effect of dietary supplementation with apple cider vinegar and propionic acid on hemolymph chemistry, intestinal microbiota and histological structure of hepatopancreas in white shrimp, Litopenaeus vannamei. Fish Shellfish Immun. 86, 900–905. doi: 10.1016/j.fsi.2018.12.019
Purdy, A. E., and Watnick, P. I. (2011). Spatially selective colonization of the arthropod intestine through activation of Vibrio cholerae biofilm formation. Proc. Natl. Acad. Sci. USA 108, 19737–19742. doi: 10.1073/pnas.1111530108
Qin, Z., Yang, X., Chen, G., Park, C., and Liu, Z. (2020). Crosstalks between gut microbiota and Vibrio cholerae. Front. Cell. Infect. Mi. 10, 582554. doi: 10.3389/fcimb.2020.582554
Ran, C., Hu, J., Liu, W., Liu, Z., He, S., Dan, B. C. T., et al. (2016). Thymol and carvacrol affect hybrid tilapia through the combination of direct stimulation and an intestinal microbiota-mediated effect: insights from a germ-Free Zebrafish model. J Nutr 146, 1132–1140. doi: 10.3945/jn.115.229377
Reina, J. C., Pérez-Victoria, I., Martín, J., and Llamas, I. (2019). A quorum-sensing inhibitor strain of Vibrio alginolyticus blocks Qs-controlled phenotypes in Chromobacterium violaceum and Pseudomonas aeruginosa. Mar. Drugs 9, 494. doi: 10.3390/md17090494
Ritchie, J. M., Rui, H., Zhou, X., Iida, T., Kodoma, T., Ito, S., et al. (2012). Inflammation and disintegration of intestinal villi in an experimental model for Vibrio parahaemolyticus induced diarrhea. PLoS pathog. 8, e1002593. doi: 10.1371/journal.ppat.1002593
Salomon, D., Gonzalez, H., Updegraff, B. L., and Orth, K. (2013). Vibrio parahaemolyticus type VI secretion system 1 is activated in marine conditions to target bacteria, and is differentially regulated from system 2. PLoS One 8, 61086. doi: 10.1371/journal.pone.0061086
Satish Kumar, R., Kanmani, P., Yuvaraj, N., Paari, K. A., Pattukumar, V., Arul, V., et al. (2011). Lactobacillus plantarum AS1 binds to cultured human intestinal cell line HT-29 and inhibits cell attachment by enterovirulent bacterium Vibrio parahaemolyticus. Lett. Appl. Microbiol. 53, 481–487. doi: 10.1111/j.1472-765X.2011.03136.x
Sengupta, C., Ekka, M., Arora, S., Dhaware, P. D., Chowdhury, R., Raychaudhuri, S., et al. (2017). Cross feeding of glucose metabolism byproducts of Escherichia coli human gut isolates and probiotic strains affect survival of Vibrio cholerae. Gut Pathog. 9, 3. doi: 10.1186/s13099-016-0153-x
Senoh, M., Ghosh-Banerjee, J., Ramamurthy, T., Hamabata, T., Kurakawa, T., Takeda, M., et al. (2010). Conversion of viable but nonculturable Vibrio cholerae to the culturable state by co-culture with eukaryotic cells. Microbiol. Immun. 54, 502–507. doi: 10.1111/j.1348-0421.2010.00245.x
Shao, Y., and Bassler, B. L. (2014). Quorum regulatory small RNAs repress type VI secretion in Vibrio cholerae. Mol. Microbiol. 92, 921–930. doi: 10.1111/mmi.12599
Sharma, M. K., Singh, N. K., Jani, D., Sisodia, R., Thungapathra, M., Gautam, J. K., et al. (2008). Expression of toxin co-regulated pilus subunit A (TCPA) of Vibrio cholerae and its immunogenic epitopes fused to cholera toxin B subunit in transgenic tomato (Solanum lycopersicum). Plant Cell Rep. 27, 307–318. doi: 10.1007/s00299-007-0464-y
Shin, O. S., Tam, V. C., Suzuki, M., Ritchie, J. M., Bronson, R. T., Waldor, M. K., et al. (2011). Type III secretion is essential for the rapidly fatal diarrheal disease caused by non-O1, non-O139 Vibrio cholerae. MBio. 2, e00106–e00111. doi: 10.1128/mBio.00106-11
Siddik, M., Howieson, J., and Fotedar, R. (2019b). Beneficial effects of tuna hydrolysate in poultry by-product meal diets on growth, immune response, intestinal health and disease resistance to Vibrio harveyi in juvenile barramundi, Lates calcarifer. Fish Shellfish Immunol. 89, 61–70. doi: 10.1016/j.fsi.2019.03.042
Siddik, M. A., Fotedar, R., Chaklader, M. R., Foysal, M. J., Nahar, A., Howieson, J., et al. (2019a). Fermented animal source protein as substitution of fishmeal on intestinal microbiota, immune-related cytokines and resistance to Vibrio mimicus in freshwater crayfish (Cherax cainii). Front. Physiol. 10, 1635. doi: 10.3389/fphys.2019.01635
Silva, A. J., and Benitez, J. A. (2016). Vibrio cholerae biofilms and cholera pathogenesis. PLoS Negl. Trop. Dis. 10, e0004330. doi: 10.1371/journal.pntd.0004330
Srivastava, D., Hsieh, M. L., Khataokar, A., Neiditch, M. B., and Waters, C. M. (2014). Cyclic di-GMP inhibits Vibrio cholerae motility by repressing induction of transcription and inducing extracellular polysaccharide production. Mol. Microbiol. 90, 1262–1276. doi: 10.1111/mmi.12432
Suckow, G., Seitz, P., and Blokesch, M. (2011). Quorum sensing contributes to natural transformation of Vibrio cholerae in a species-specific manner. J. Bacteriol. 193, 4914–4924. doi: 10.1128/JB.05396-11
Sun, Y., Wang, G., Peng, K., Huang, Y., Cao, J., Huang, W., et al. (2019). Effects of dietary xylo-oligosaccharides on growth performance, immunity and Vibrio alginolyticus resistance of juvenile Litopenaeus vannamei. Aquac. Res. 50, 358–365. doi: 10.1111/are.13911
Thorstenson, C. A., and Ullrich, M. S. (2021). Ecological fitness of Vibrio cholerae, Vibrio parahaemolyticus, and Vibrio vulnificus in a small-scale population dynamics study. Front. Mar. Sci. 8, 623988. doi: 10.3389/fmars.2021.623988
Tischler, A. D., and Camilli, A. (2004). Cyclic diguanylate (c-di-GMP) regulates Vibrio cholerae biofilm formation. Mol. Microbiol. 53, 857–869. doi: 10.1111/j.1365-2958.2004.04155.x
Torrecillas, S., Caballero, M. J., Mompel, D., Montero, D., Zamorano, M. J., Robaina, L., et al. (2017). Disease resistance and response against Vibrio anguillarum intestinal infection in European seabass (Dicentrarchus labrax) fed low fish meal and fish oil diets. Fish Shellfish Immunol. 67, 302–311. doi: 10.1016/j.fsi.2017.06.022
Utada, A. S., Bennett, R. R., Fong, J. C. N., Gibiansky, M. L., Yildiz, F. H., Golestanian, R., et al. (2014). Vibrio cholerae use pili and flagella synergistically to effect motility switching and conditional surface attachment. Nat. Commun. 5, 4913. doi: 10.1038/ncomms5913
Valente, C. D., and Wan, A. H. L. (2021). Vibrio and major commercially important vibriosis diseases in decapod crustaceans. J. Invertebr. Pathol. 181, 107527. doi: 10.1016/j.jip.2020.107527
Wang, H., Wu, J. H., Ayala, J. C., Benitez, J. A., and Silva, A. J. (2011). Interplay among cyclic diguanylate, HapR, and the general stress response regulator (RpoS) in the regulation of Vibrio cholerae hemagglutinin/protease. J. Bacteriol. 193, 6529–6538. doi: 10.1128/JB.05166-11
Wang, J., Xing, X., Yang, X., Jung, I. J., Hao, G., Chen, Y., et al. (2018). Gluconeogenic growth of Vibrio cholerae is important for competing with host gut microbiota. J. Med. Microbiol. 67, 1628–1637. doi: 10.1099/jmm.0.000828
Wang, S., Wang, J., Mou, H., Luo, B., and Jiang, X. (2015). Inhibition of adhesion of intestinal pathogens (Escherichia coli, Vibrio cholerae, Campylobacter jejuni, and Salmonella Typhimurium) by common oligosaccharides. Foodborne Pathog. Dis. 12, 360–365. doi: 10.1089/fpd.2014.1835
Wassef, E. A., Saleh, N. E., Abdel-Meguid, N. E., Barakat, K. M., Abdel-Mohsen, H. H., and El-bermawy, N. M. (2020). Sodium propionate as a dietary acidifier for European seabass (Dicentrarchus labrax) fry: immune competence, gut microbiome, and intestinal histology benefits. Aquac. Int. 28, 95–111. doi: 10.1007/s10499-019-00446-7
Wong, E., Vaaje-Kolstad, G., Ghosh, A., Hurtado-Guerrero, R., Konarev, P. V., Ibrahim, A. F. M., et al. (2012). The Vibrio cholerae Colonization Factor GbpA Possesses a Modular Structure that Governs Binding to Different Host Surfaces. PloS Pathog. 8, e1002373. doi: 10.1371/journal.ppat.1002373
Wu, C., Zhao, Z., Liu, Y., Zhu, X., and Shi, Y. (2020). Type iii secretion 1 effector gene diversity among Vibrio isolates from coastal areas in China. Front. Cell Infect. Mi. 10, 301. doi: 10.3389/fcimb.2020.00301
Xu, T., Cao, H., Zhu, W., Wang, M., Du, Y., Yin, Z., et al. (2018). RNA-seq-based monitoring of gene expression changes of viable but non-culturable state of Vibrio cholerae induced by cold seawater. Environ. Microbiol. Rep. 10, 594–604. doi: 10.1111/1758-2229.12685
Yang, H., Souza, S., antos, d. e., Lee, M., Law, J., Chimalapati, H. T., Verdu, S. E. F., et al. (2019). A novel mouse model of enteric Vibrio parahaemolyticus infection reveals that the type III secretion system 2 effector VopC plays a key role in tissue invasion and gastroenteritis. Mbio. 10, e02608–e02619. doi: 10.1128/mBio.02608-19
Ye, J., Ma, Y., Liu, Q., Zhao, D. L., Wang, Q. Y., Zhang, Y. X., et al. (2008). Regulation of Vibrio alginolyticus virulence by the LuxS quorum-sensing system. J. Fish. Dis. 31, 161–169. doi: 10.1111/j.1365-2761.2007.00882.x
Yu, Y., Yang, H., Li, J., Zhang, P., Wu, B., Zhu, B., et al. (2012). Putative type VI secretion systems of Vibrio parahaemolyticus contribute to adhesion to cultured cell monolayers. Arch. Microbiol. 194, 827–835. doi: 10.1007/s00203-012-0816-z
Zhang, L., Krachler, A. M., Broberg, C. A., Li, Y., Mirzaei, H., Gilpin, C. J., et al. (2012a). Type III effector VopC mediates invasion for Vibrio species. Cell Rep. 1, 453–460. doi: 10.1016/j.celrep.2012.04.004
Zhang, L., Mai, K., Tan, B., Ai, Q., Qi, C., Xu, W., et al. (2009). Effects of dietary administration of probiotic Halomonas sp. B12 on the intestinal microflora, immunological parameters, and midgut histological structure of Shrimp, Fenneropenaeus chinensis. J. World Aquacult. Soc. 40, 58–66. doi: 10.1111/j.1749-7345.2008.00235.x
Zhang, L. L., and Kim, O. (2013). Virulence determinants for Vibrio parahaemolyticus infection. Curr. Opin. Microbiol. 16, 70–77. doi: 10.1016/j.mib.2013.02.002
Zhang, Q., Tan, B., Mai, K., Zhang, W., Ma, H., Ai, Q., et al. (2011). Dietary administration of Bacillus (B. licheniformis and B. subtilis) and isomaltooligosaccharide influences the intestinal microflora, immunological parameters and resistance against Vibrio alginolyticus in shrimp, Penaeus japonicus (Decapoda: Penaeidae). Aquac. Res. 42, 943–952. doi: 10.1111/j.1365-2109.2010.02677.x
Zhang, R., Wang, X. W., Liu, L. L., Cao, Y. C., and Zhu, H. (2020a). Dietary oregano essential oil improved the immune response, activity of digestive enzymes, and intestinal microbiota of the koi carp, Cyprinus carpio. Aquaculture 518, 734781. doi: 10.1016/j.aquaculture.2019.734781
Zhang, X. H., He, X. X., and Austin, B. (2020b). Vibrio harveyi: a serious pathogen of fish and invertebrates in mariculture. Mar. Life Sci. Technol. 2, 231–245. doi: 10.1007/s42995-020-00037-z
Zhang, Y., Gao, H., Osei-Adjei, G., Zhang, Y., Yang, W., Yang, H., et al. (2017). Transcriptional regulation of the type VI secretion system 1 genes by quorum sensing and ToxR in Vibrio parahaemolyticus. Front. Microbiol. 8, 2005. doi: 10.3389/fmicb.2017.02005
Zhang, Y., Qiu, Y., Tan, Y., Guo, Z., Yang, R., Zhou, D., et al. (2012b). Transcriptional regulation of opaR, qrr2-4 and aphA by the master quorum-sensing regulator OpaR in Vibrio parahaemolyticus. PLoS ONE 7, e34622. doi: 10.1371/journal.pone.0034622
Keywords: Vibrio, intestinal microflora, physiological metabolism, pathogenicity, colonization
Citation: Sun H, Zhu C, Fu X, Khattak S, Wang J, Liu Z, Kong Q, Mou H and Secundo F (2022) Effects of intestinal microbiota on physiological metabolism and pathogenicity of Vibrio. Front. Microbiol. 13:947767. doi: 10.3389/fmicb.2022.947767
Received: 19 May 2022; Accepted: 29 July 2022;
Published: 23 August 2022.
Edited by:
Jeffrey H. Withey, Wayne State University, United StatesCopyright © 2022 Sun, Zhu, Fu, Khattak, Wang, Liu, Kong, Mou and Secundo. This is an open-access article distributed under the terms of the Creative Commons Attribution License (CC BY). The use, distribution or reproduction in other forums is permitted, provided the original author(s) and the copyright owner(s) are credited and that the original publication in this journal is cited, in accordance with accepted academic practice. No use, distribution or reproduction is permitted which does not comply with these terms.
*Correspondence: Haijin Mou, mousun@ouc.edu.cn; Francesco Secundo, francesco.secundo@scitec.cnr.it
†These authors have contributed equally to this work