- 1Memory Studies Laboratory, Physiology Department, Universidade Federal do Rio Grande do Norte, Natal, Brazil
- 2Brain Institute, Universidade Federal do Rio Grande do Norte, Natal, Brazil
- 3Behavioral Neuroscience Laboratory, Pharmacology Department, Universidade Federal de São Paulo, São Paulo, Brazil
- 4Laboratory of Neuroscience and Bioprospecting of Natural Products, Department of Biosciences, Universidade Federal de São Paulo, São Paulo, Brazil
The cause of Alzheimer’s disease (AD) remains uncertain. The accumulation of amyloid peptides (Aβ) is the main pathophysiological hallmark of the disease. Spatial deficit is an important initial sign of AD, while other types of memory impairments that appear in later stages. The Barnes maze allows the detection of subtle alterations in spatial search by the analysis of use of different strategies. Previous findings showed a general performance deficit in this task following long-term (35 days) infusion of Aβ, which corresponds to the moderate or severe impairments of the disease. In the present study, we evaluated the effects of a low-dose 15-day long treatment with Aβ peptides on spatial and non-spatial strategies of rats tested in the Barnes maze. Aβ peptides (0.5 μL/site/day; 30 pmoL solution of Aβ1–40:Aβ1–42 10:1) or saline were bilaterally infused into the CA1 (on the first treatment day) and intraventricularly (on the following 15 days) in 6-month-old Wistar male rats. Aβ infusion induced a deficit in the performance (increased latency and distance traveled to reach the target compared to saline group). In addition, a significant association between treatment and search strategy in the retrieval trial was found: Aβ group preferred the non-spatial search strategy, while saline group preferred the spatial search. In conclusion, the protocol of Aβ infusion used here induced a subtle cognitive deficit that was specific to spatial aspects. Indeed, animals under Aβ treatment still showed retrieval, but using non-spatial strategies. We suggest that this approach is potentially useful to the study of the initial memory deficits in early AD.
Introduction
Alzheimer disease (AD) is a neurodegenerative disorder characterized by atypical neural activity, dysfunction and loss of synapses and neurons, accompanied by progressive cognitive decline (Braak et al., 1992; West et al., 1994; Eustache et al., 2004; Sadek et al., 2004). The main pathophysiological hallmarks of AD are amyloid plaques and neurofibrillary tangles in the brain tissue (Serrano-Pozo et al., 2011). The exact cause of AD remains unknown, although the accumulation of amyloid peptides has been suggested as the cause of cytotoxicity. This accumulation is the basis of the amyloid cascade hypothesis for AD and precedes the first manifestations of cognitive decline (Braak and Braak, 1991; Hardy and Selkoe, 2002; Desikan et al., 2013; Sperling et al., 2011, 2014).
Recent studies have investigated human spatial learning and memory in virtual reality environments (Iaria et al., 2009; Head and Isom, 2010; Plancher et al., 2010). This approach has been useful to show age-related cognitive hippocampal dysfunctions, similarly to studies in rodent models (McNaughton et al., 1989; Poucet et al., 1991; McDonald and White, 1993; Pouzet et al., 2002). The results highlight the importance of protocols for early detection of such impairment (Foster et al., 2012). Further, spatial deficits detected in navigation tasks have been proposed as an important initial sign of AD, and this feature distinguishes this condition from other types of age-related cognitive impairments (Lithfous et al., 2013). In addition, deficits in other types of memory usually arise in later stages of the disease progression (Sá et al., 2012; Tarawneh and Holtzman, 2012).
Spatial tasks have been employed to investigate cognitive decline in rodents, and some of the tasks allow the detection of subtle changes in spatial behavior. This feature is relevant to the evaluation of mild hippocampal impairment, such as the initial degenerative process in animal models of AD. The Barnes maze is as spatial task in which the animals are trained to reach a hidden shelter guided by visual cues (Barnes, 1979; Barnes et al., 1980, 1989, 1990; Harrison et al., 2006; O’Leary and Brown, 2012). In this task, memory is evaluated by latency and distance to reach the target, time in target quadrant and number of errors (Sunyer et al., 2007; Attar et al., 2013; Rojanathammanee et al., 2013). In addition, subtle alterations in spatial search can be detected by the analysis of different search strategies to solve the task (Harrison et al., 2006). This behavioral task has been used to investigate spatial memory impairment in models of neurodegenerative conditions, including AD (Pompl et al., 1999; Reiserer et al., 2007; O’Leary and Brown, 2009).
Brain infusion of Aβ peptides is a well recognized rodent model of AD (Kowall et al., 1991; Nitta et al., 1994; Frautschy et al., 1996; Yamada and Nabeshima, 2000; Kang et al., 2015). However, most of the Aβ infusion studies focus the intermediate or late stages of the disease (Dickey et al., 2003, 2004; Palop et al., 2003; Blanchard et al., 2008, 2009), an approach that would diminish the contribution to the efficiency of potential neuroprotective treatments. In general, the protocols are conducted with large concentrations or long treatment durations (Han et al., 2011; Tran et al., 2011; Cioanca et al., 2013). To our knowledge, only one study investigated the effects of Aβ treatment in mice tested in the Barnes maze. This work showed a general performance deficit following long-term (35 days) infusion, without evaluation of different strategies (Morzelle et al., 2016). Here, we used a protocol of Aβ infusions that induces subtle memory deficits, which would be similar to initial stages in humans. The effects of this treatment were investigated in Barnes maze general performance and use of spatial or non-spatial search strategies. We hypothesize that the protocol of Aβ infusion used here will induce subtle deficits in the maze performance, specifically in the use of spatial strategies.
Materials and Methods
Animals
Seventeen six-month-old male Wistar rats (350–500 g) were kept in four or five animals per cage (30 × 37 × 16 cm) under controlled conditions of temperature (25 ± 1°C) and luminosity (12/12 h light/dark cycle, with lights turned on 7:00 a.m. and turned off 7:00 p.m.). Food and water were provided ad libitum. All rats were handled during 3 days, at least 5 min per day, previously to the beginning of the experiments. All procedures were in accordance to the Brazilian Law for the use of animals in scientific research (law 11.794) and approved by the local ethics committee (CEUA/UFRN, protocol no. 50/2014). All efforts were made to minimize pain, suffering or animal discomfort. Before the procedures, animals were allocated in the experimental room for habituation. The behavioral experiments were conducted in the mornings, beginning at 8 a.m.
Stereotaxic Surgery
After anesthesia with ketamine (100 mg/kg) and xylazin (50 mg/kg), the animals were submitted to stereotaxic surgery and cannulas were implanted bilaterally into dorsal hippocampus subregion CA1 (AP: −4.2 mm; LL: ±3.0 mm and DV: −2.5 mm) and one in the lateral ventricle (i.c.v.; AP: −1.0 mm; LL: ±1.8 mm and DV: −3.3 mm). Right or left hemispheric location was randomized among rats. The coordinates were chosen according to a rat brain atlas (Paxinos and Watson, 2007) and adjusted considering age. The microinjections were performed with an infusion pump and a 10 μL microsyringe (Hamilton Company, Reno, NV, USA) connected to a 26-gauge steel needle into the brain sites.
Beta-Amyloid (Aβ) Infusion
Animals received infusions (0.5 μL at the rate of 1 μL/min) of Aβ or vehicle i.c.v. and CA1 (bilateral) on the first day, followed by 14 daily i.c.v. infusions. Animals in the Aβ group (n = 9) received a 1:10 solution of 1–42 and 1–40 Aβ peptides (Iwatsubo et al., 1994; Suzuki et al., 1994; Gravina et al., 1995), equivalent to 30 pmoL per day, diluted in 0.9% saline. Before the beginning of infusions, each Aβ peptide solution was separetaly heated at 37°C for 3 days (Pike et al., 1991). Afterwards, 1–42 and 1–40 Aβ peptides (Sigma Chemical Co. St. Louis, MO, USA) were put together and left at 37°C for 24 h. Control animals receive the same volume of saline (n = 5). Two saline and one Aβ animals were excluded from the analyses because they did not reach the target in the probe session.
Barnes Maze
The Barnes maze is a spatial learning apparatus in which the animals search for an escape box (Barnes, 1979). The maze is a dark circular platform (120 cm diameter), elevated 90 cm from the floor, containing 20 holes (10 cm in diameter) disposed circularly at the edge of the platform. One of the holes (target hole) is connected to an escape box (10 × 10 × 15 cm). There were visual cues on the walls, located 50 cm distant from the apparatus. During the behavioral sessions, the lights were turned on (420 lux) to increase escape motivation. All experimental sessions were recorded by a video camera placed above the apparatus and analyzed with a video-tracking software (ANY-maze, Stoelting, USA).
In the habituation session, the animals were allowed to freely explore the apparatus during 150 s or until the entrance in escape box. Afterwards, the animals were submitted to a set of four daily training sessions, each one with two trials. The inter-trials interval was the duration of cleaning the apparatus (with a 5% alcohol solution). The first training session was performed immediately after the habituation. All trials lasted 300 s or until the animals reached the escape box. However, if the rats did not reach the target hole, the experimenter gently guided the animal towards it at the end of the trial. After reaching the escape box, animals remained inside for 60 s. The escape box is always located in the same place during the training set, but not during the previous habituation session. Retrieval of spatial learning was evaluated in the probe session, which was conducted 3 days after the last training day. The procedure was similar to the training trials, but the escape box was removed. At the beginning of each session, the animals were placed in an opaque container at the center of the maze. The container was then pulled up, and the animal was released to explore the maze. Between animals, the maze was cleaned and rotated to avoid odor cues (Figure 1).
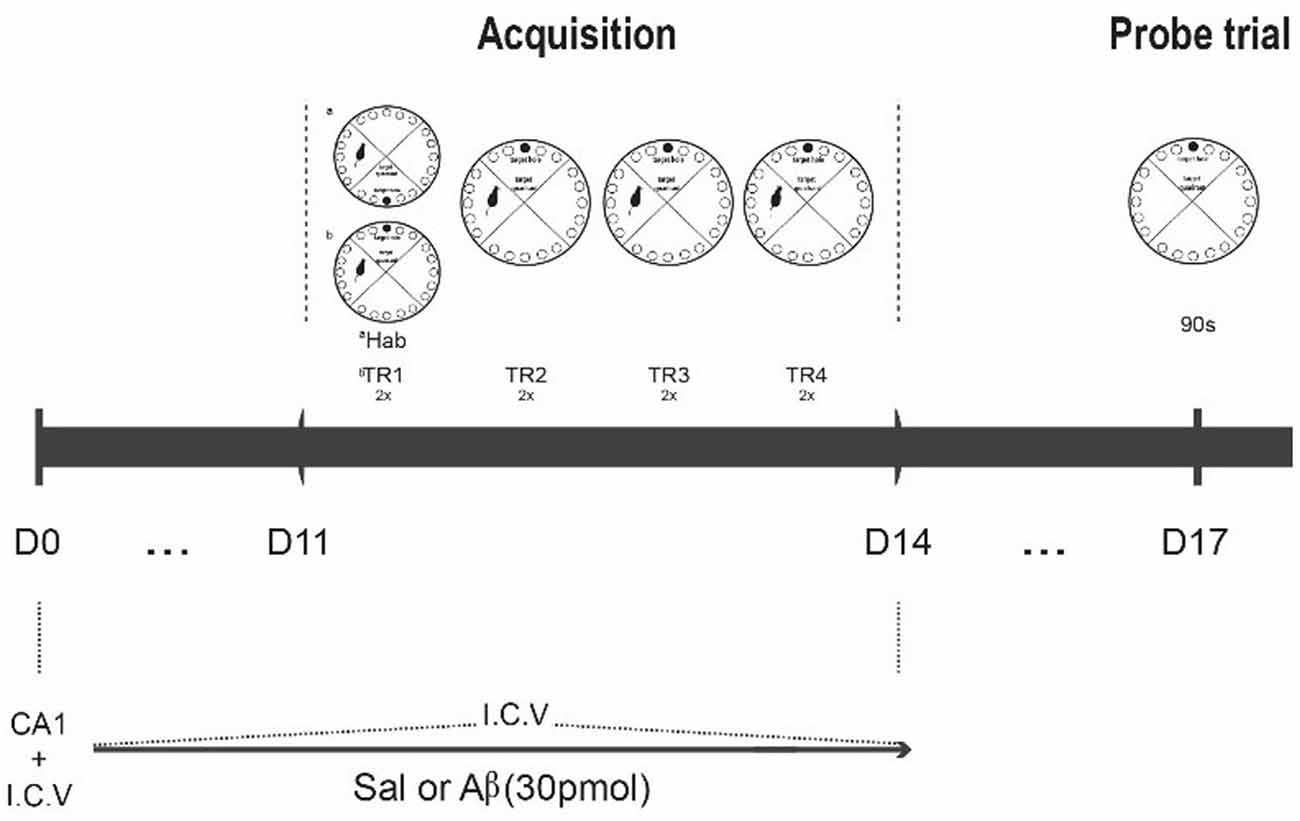
Figure 1. Experimental design. Rats were infused with saline or Aβ solution for 15 days (D0 to D14); at D0, CA1 and I.C.V. infusion; from D1 to D11, only I.C.V. infusion. After the 11th infusion, the rats were submitted to the habituation phase (Hab), followed by acquisition phase (two series of four two-trial daily trainings, Tr1 to Tr4, D11 to D14). On the 3rd day after the last training day, they were tested for task retrieval (90 s probe trial, D17). The perfusion for immunohistochemistry was conducted 1 h after the probe trial.
The parameters analyzed were the percentage of time in the quadrants of the maze, the latency to reach the target hole, the distance traveled until reaching the target hole and the number of errors. We also analyzed search strategies (the kind of search performed to reach target), and analysis was conducted on the basis of previous studies (Bach et al., 1995; Harrison et al., 2006), with minor modifications. The search strategies were categorized into three types: spatial (or direct), serial or random (the two latter were considered non-spatial strategies). One search was computed each time the animal visited the target hole in the probe trial. The direct (spatial) strategy was computed when the animal moved directly to the target hole or to an adjacent hole before visiting the target hole. The serial strategy was computed when there were visits to at least three sequential holes in clockwise or counter-clockwise direction previously to the visit to the target hole. The random strategy was computed when at least three visits before the target hole happened in an unsystematic manner, i.e., the animal visited non-adjacent holes before visiting the target. The type of strategy was analyzed by the following approaches: (1) initial and last search (the strategy used to reach the target for the first and the last time in the probe trial); (2) total preference in the probe trial (the percent of use of each strategy across the whole probe trial (the number of times the animal used a certain strategy/total number of searches); and (3) the mean percentage of preferred strategy in each group.
Data Analyses
We used Shapiro-Wilk test to check data distribution. We applied one-way ANOVA, one- or two-sample t-tests according to the parameter analyzed. For analyses across sessions, we performed repeated-measures ANOVA. Bonferroni post hoc test was applied when necessary. Fisher’s exact test (x2) was used for the analysis of the association between treatment (Aβ/saline) and preferred strategy, as well as the magnitude of this association in 95% confidence interval (odds ratio, OR, 95% CI). Data are shown as mean ± SEM. We determined the level of significance at p < 0.05.
Results
The latency to reach the escape hole diminished over training sessions in both groups, indicating similar learning curves (F(1.7,21.2) = 16.688, p < 0.001, Bonferroni post hoc; Figure 2A). Indeed, we did not find differences between groups (F(1,12) = 1.592, p = 0.231) or factors interaction (F(1.7,21.2) = 2.103, p = 0.151). However, in the probe trial, Aβ group displayed longer latency to reach the target hole for the first time (t(12) = −2.679, p = 0.02; Figure 2B).
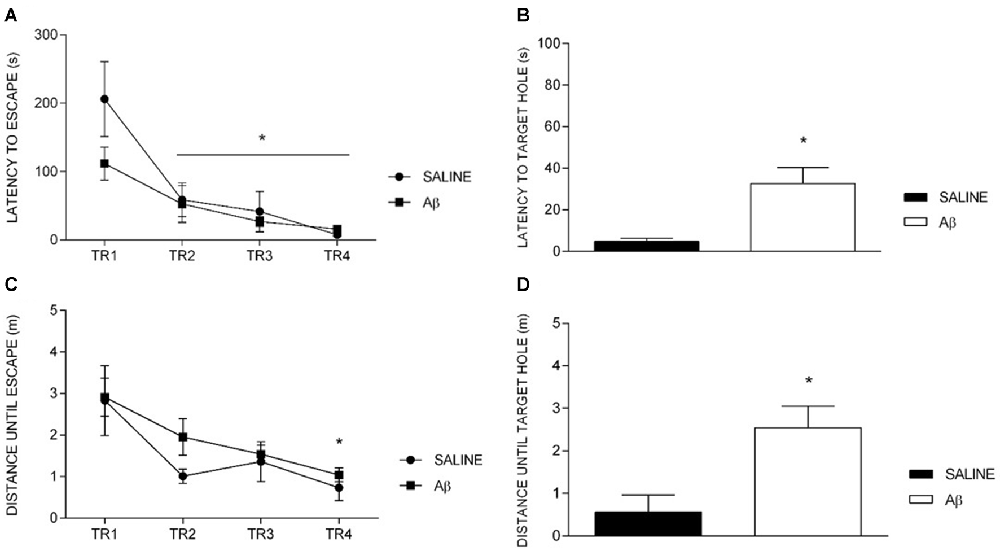
Figure 2. Latency and distance until escape in training (TR) sessions and until target hole in probe trial. (A,C) Effect of session for both groups (repeated measures two-way ANOVA, Bonferroni post hoc); *p < 0.05 compared to TR1. (B,D) Unpaired t-test; *p < 0.05 compared to SALINE. Mean ± SEM.
We observed the same pattern of results for the distance traveled until the escape hole across the training sessions (session effect [F(3,36) = 9.638, p < 0.001], without group effect [F(1,12) = 1.005, p = 0.336] or interaction [F(3,36) = 0.509, p = 0.678], Bonferroni post hoc; Figure 2C). On the probe trial, we found that Aβ groups showed longer distance traveled until the target than saline (t(12) = −2.636, p = 0.022; Figure 2D).
The number of errors reduced across the training sessions for both groups (F(3,36) = 5.419, p = 0.004, Bonferroni post hoc; Figure 3A). In addition, we did not find differences between groups (F(1,12) = 0.573, p = 0.464) or factors interaction (F(3,36) = 0.218, p = 0.883). Likewise, on the probe trial, there was no difference between the groups (t(12) = −0.985, p = 0.344; Figure 3B).
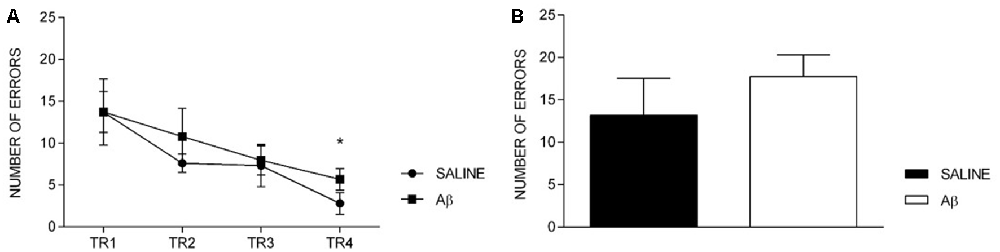
Figure 3. Number of errors in training sessions (TR, A) and probe trial (B). (A) Effect of session for both groups (repeated measures two-way ANOVA, Bonferroni post hoc); *p < 0.05 compared to TR1. (B) Unpaired t-test; p > 0.05. Mean ± SEM.
Additionally, both groups showed increased time in the target quadrant across trainings (F(3,36) = 14.954, p < 0.001, Bonferroni post hoc; Figure 4A), without treatment effect (F(1,12) = 0.416, p = 0.531) or factors interaction (F(3,36) = 0.946, p = 0.429). On the probe trial, there was no difference between groups (t(12) = 0.736, p = 0.476; Figure 4B).
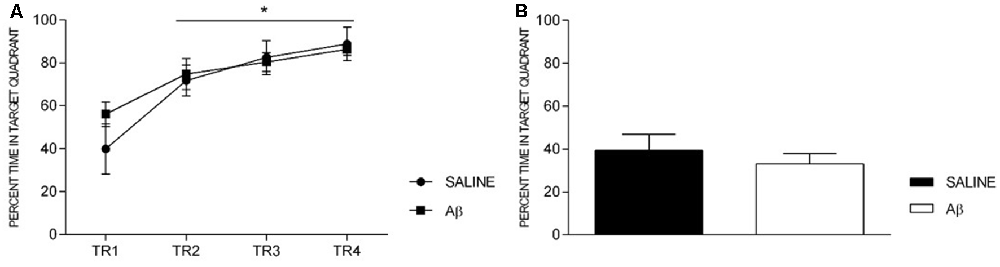
Figure 4. Percent time in target quadrant in training sessions (TR, A) and probe trial (B). (A) Effect of session for both groups (repeated measures two-way ANOVA, Bonferroni post hoc); *p < 0.05 compared to TR1. (B) Unpaired t-test; p > 0.05. Mean ± SEM.
We found a significant effect of treatment on the search strategy in the probe trial. Saline group preferred the spatial strategy as initial choice compared to Aβ group (Fisher’s exact test, = 95.998, p < 0.001; Figure 5A). The probability that saline group had used the spatial strategy was 32 times higher than Aβ group (OR 32.36, 95% CI: 14.61–71.69).
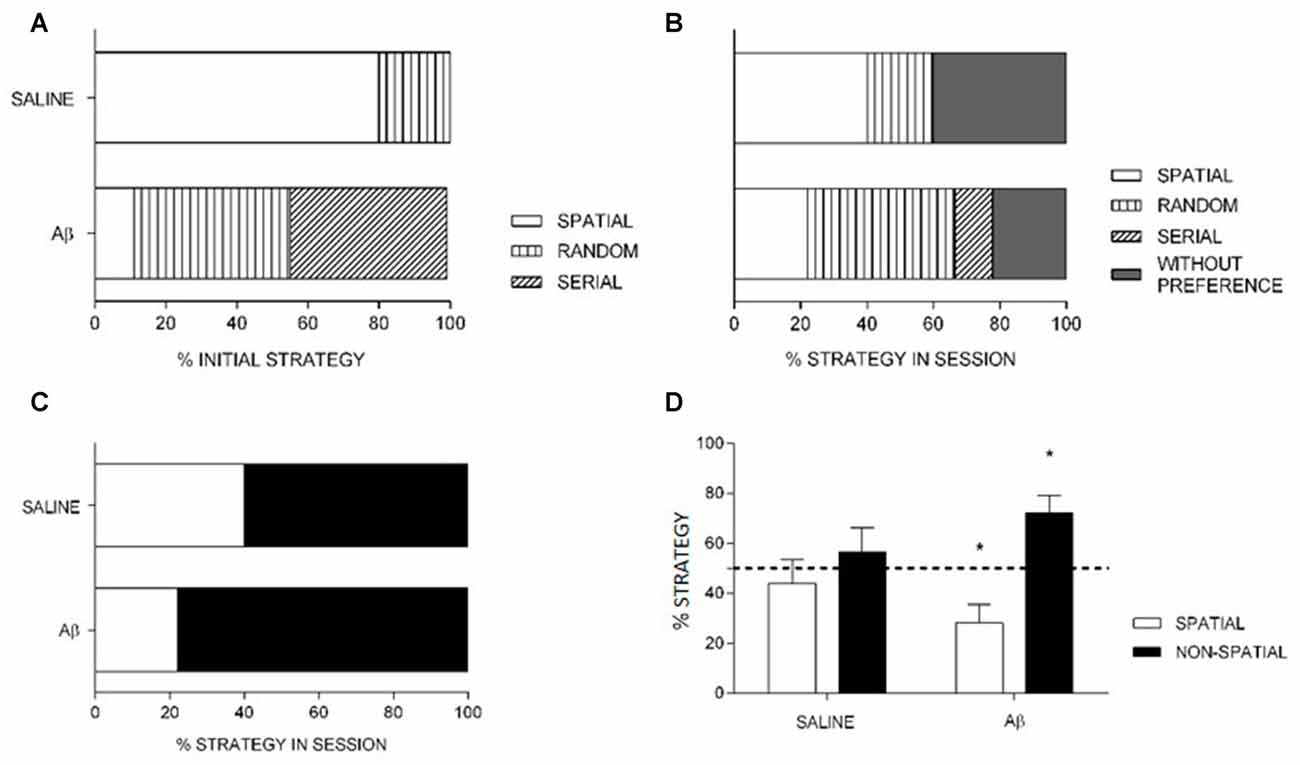
Figure 5. Percentage of strategy use at initial choice (A) and in the total (B,C) probe trial. (A,B) Saline had increased initial (A) and total (B) spatial strategy choice compared to Aβ group. (C) The preferential use of spatial strategy by saline is sustained when spatial, non-spatial and without preference are grouped into non-spatial strategy. (A–C) Data are percentage of strategy compared between the groups. Fisher’s exact test, x2, p < 0.05. (D) Percentage of spatial and non-spatial strategies (mean + SEM) differed from chance for Aβ, but not for saline group (one-sample t-test). *p < 0.05 compared to chance (dashed line).
Considering the search strategies in the whole probe session, the Aβ group used less spatial search strategy compared to saline (Fisher’s exact test, = 20.276, p < 0.001; OR 5.091, 95% IC 2.456–10.553, Figure 5B). In addition, the analysis of groups by strategy in the whole probe session (including animals that preferred random or serial strategies and had no preference into the non-spatial category), Aβ group sustains the preferential use of non-spatial search strategies compared to saline animals (Fisher’s exact test, = 7.574, p = 0.009; OR 2.364, 95% IC 1.272–4.392; Figure 5C).
We also analyzed the last choice, i.e., the strategy used to reach the target for the last time in the probe session. We found that the Aβ group persevered on non-spatial strategies, while saline-treated animals changed preference towards the end of the probe session. Indeed, within subjects analyses (first vs. last choices) confirmed the change of preference in saline group ( = 72.000, p < 0.001; OR, 16.000, 95% CI: 8.002–31.994), and the persistence of preference for non-spatial strategies in the Aβ group ( = 4.391, p = 0.056; OR, 0.438, 95% CI: 0.200–0.961). In addition, the use of strategies as last choice did not differ between groups ( = 3.196, p = 0.082; OR 2.045, % CI: 0.924–4.529). In Table 1, we show the percent of animals that show spatial and non-spatial preferences for the first three and the last choice in the probe session. We show four choices because it was the minimum number of strategies used by a subject in the probe session.
The comparison of means of percentage of strategies in the groups in the whole probe session showed that saline group used possible search strategies similarly (spatial, t(4) = −0.636, p = 0.559; non-spatial, t(4) = 0.685, p = 0.531), while the Aβ group preferred non-spatial search strategies (spatial, t(8) = −3.020, p = 0.017; non-spatial, t(8) = 3.121, p = 0.014; Figure 5D).
Discussion
We observed that both groups showed reduced distance and latency to reach target and number of errors, and increased percent time in the target quadrant across the trainings, indicating that all the animals learned the task (Barnes, 1979; Pompl et al., 1999). Moreover, we found that animals that received Aβ peptides showed impairments when the latency and distance to reach the target were analyzed in the probe trial. However, no differences were found to the other performance parameters (number of errors and percent time in the target quadrant) in the probe session, suggesting that Aβ animals showed some extent of retrieval. In addition, we found that Aβ-infused animals preferentially used non-spatial search strategies, corroborating an impairment in spatial retrieval, despite the performance was not completely impaired. Finally, there were no differences in the distance traveled in the maze between saline and Aβ groups, indicating that Aβ peptides infusion did not modify motor activity (data not shown).
Our data are in line with previous studies that suggest that the amyloid aggregation impairs hippocampal connectivity, which is necessary for spatial navigation and for strategy choice (Pouzet et al., 2002; Savonenko et al., 2005; O’Leary and Brown, 2009). In the present study, when the search strategies to reach target were analyzed, saline group preferred the spatial strategy to the non-spatial strategy, while Aβ animals had the opposite preference. This outcome suggests that the deficit promoted by beta-amyloid was revealed by the kind of search navigation, which has modified the spatial performance. Of note, when the analysis of search strategies was performed for mean percentage of search strategy within the groups, Aβ animals showed increased preference for non-spatial search strategies. However, saline animals did not show differences from chance for both strategies. This apparently contradictory outcome might be due to a tendency to randomization of safe place search towards the end of the session, because the escape box is no longer present (Harrison et al., 2006). Indeed, in line with previous findings, saline animals changed preference across the probe session, and preferred spatial strategies as first choice as opposed to non-spatial strategies as last choice. Conversely, Aβ animals persevered on the non-spatial strategies, showing preference for this type of navigation towards the end of the probe session as well (see Table 1).
The Barnes maze has been used in studies of spatial learning and memory in transgenic models of AD (Pompl et al., 1999; Reiserer et al., 2007; O’Leary and Brown, 2009). Pompl et al. (1999) suggested that transgenic animals have difficulty to use spatial reference memory to reach a specific place (Chapman et al., 1999; Chen et al., 2000; Janus, 2004). This outcome resembles the memory deficit found in AD patients (Kaskie and Storandt, 1995) due to hippocampal function impairment (McNaughton et al., 1989; Poucet et al., 1991; McDonald and White, 1993). Rodriguez et al. (2013) demonstrated that APOE4 (an AD risk allele) mice showed delayed learning and impaired retrieval in the Barnes task. These animals also showed reduction in length and density of dendritic spines in the medial entorhinal cortex, and these alterations were related to early-impaired spatial cognition. Prut et al. (2007) showed that the AD transgenic mice APP23 had increased latency to reach the target and number of errors throughout the trainings in the Barnes maze, along with plaque deposition in the hippocampus and neocortex. Moreover, these animals had delays to switch from non-spatial to spatial strategies in the course of training. In the present study, we did not observe alterations during the training sessions of the Barnes maze, probably due to the mild nature of the cognitive deficit induced by our protocol. Indeed, we only observed spatial retrieval impairment in the probe trial.
In line with the deficit in the retrieval of spatial information, Janus (2004) found that AD transgenic mice (TgCRND8) did not use spatial strategies in the Morris water maze; they learned to reach the goal using a non-spatial strategy. Hamm et al. (2017) have also studied the transgenic TgCRND8 model, and showed an impaired association between object and place in a hippocampal dependent-task. In general, these previous studies suggest that the impairment in learning and retrieval of memory in AD models are due to deficits in the use of spatial strategies promoted by Aβ peptides increase and/or accumulation. In line with these findings, the results reported here show subtle alterations in spatial performance and the preferential use of non-spatial strategies after Aβ peptides infusion, suggesting a similarity with the early stages of AD.
Human versions of rodent spatial mazes in virtual environments have been used for detection of spatial memory deficits induced by hippocampal damage (Skelton et al., 2000; Moffat et al., 2001; Astur et al., 2002; Bohbot et al., 2004; Kumaran et al., 2007; Bartsch et al., 2010; Goodrich-Hunsaker et al., 2010). These investigations of spatial navigation in humans could allow early detection of dementia, which is relevant for early therapeutic intervention.
Nevertheless, similar to rodent tasks, the continuous exposition to such tasks diminishes the possibility of impairment detection (Jansen et al., 2010). On the other hand, the delayed retrieval assessment allows the detection of the episodic memory deficit (Albert, 1997; Foster, 1999). In this respect, contrary to the results described in the present study, Hamm et al. (2017) did not verify an impairment in the Barnes maze performance. This discrepancy is probably due to the reduced number of training trials and increased retrieval delay in our study. Thus, these protocol modifications allowed the detection of a spatial deficit similarly to the delayed retrieval protocol used in humans for the study of early impairments in AD.
The detailed etiology of Alzheimer remains unknown, although a great amount of evidence points to Aβ peptides as contributing factors to the neuronal dysfunctions and cognitive deficits of the disease (Braak and Braak, 1991; Dickey et al., 2003, 2004; Palop et al., 2003; Eustache et al., 2004; Sadek et al., 2004). The protocol of Aβ infusion used here induced a subtle cognitive deficit that was specific to spatial aspects, possibly compatible with prodromic characteristics of AD. Animals under Aβ treatment showed some extent of retrieval, but using non-spatial strategies. Further, these animals persevered on non-spatial choices until the end of the probe session. The results indicate that a task that can be solved by both spatial and non-spatial strategies is sensitive to detect deficits that could go unnoticed otherwise. The detection of subtle changes on cognition in an animal model can be useful to investigations of mechanisms as well as tests of potential neuroprotective approaches. We suggest that this approach is potentially useful to the study of the initial memory deficits in early AD.
Author Contributions
PTM, RHL and RHS designed the research and wrote the article. PTM, ACQA, YSRM, LEMB and CLCC collected and analyzed the data. MRC and AMR contributed with theoretical and technical support.
Funding
The research was supported by fellowships from Conselho Nacional de Desenvolvimento Científico e Tecnológico (CNPq) (grant # 402054/2010-5), Coordenação de Aperfeiçoamento de Pessoal de Nível Superior (CAPES), Fundação de Apoio à Pesquisa do Estado do Rio Grande do Norte (FAPERN/PRONEX) and Fundação de Amparo à Pesquisa do Estado de São Paulo (FAPESP) (grant #2015/12308-5).
Conflict of Interest Statement
The authors declare that the research was conducted in the absence of any commercial or financial relationships that could be construed as a potential conflict of interest.
Acknowledgments
The authors would like to thank Mateus Oliveira Silva, Sara Sophia Guedes and Marília Felipe for technical support.
References
Albert, M. S. (1997). The ageing brain: normal and abnormal memory. Philos. Trans. R. Soc. Lond. B Biol. Sci. 352, 1703–1709. doi: 10.1098/rstb.1997.0152
Astur, R. S., Taylor, L. B., Mamelak, A. N., Philpott, L., and Sutherland, R. J. (2002). Humans with hippocampus damage display severe spatial memory impairments in a virtual Morris water task. Behav. Brain Res. 132, 77–84. doi: 10.1016/S0166-4328(01)00399-0
Attar, A., Liu, T., Chan, W. T., Hayes, J., Nejad, M., Lei, K., et al. (2013). A shortened Barnes maze protocol reveals memory deficits at 4-months of age in the triple-transgenic mouse model of Alzheimer’s disease. PLoS One 8:e80355. doi: 10.1371/journal.pone.0080355
Bach, M. E., Hawkins, R. D., Osman, M., Kandel, E. R., and Mayford, M. (1995). Impairment of spatial but not contextual memory in CaMKII mutant mice with a selective loss of hippocampal LTP in the range of the theta frequency. Cell 81, 905–915. doi: 10.1016/0092-8674(95)90010-1
Barnes, C. A. (1979). Memory deficits associated with senescence: a neurophysiological and behavioral study in the rat. J. Comp. Physiol. Psychol. 93, 74–104. doi: 10.1037/h0077579
Barnes, C. A., Eppich, C., and Rao, G. (1989). Selective improvement of aged rat short-term spatial memory by 3,4-diaminopyridine. Neurobiol. Aging 10, 337–341. doi: 10.1016/0197-4580(89)90045-6
Barnes, C. A., Markowska, A. L., Ingram, D. K., Kametani, H., Spangler, E. L., Lemken, V. J., et al. (1990). Acetyl-1-carnitine. 2: effects on learning and memory performance of aged rats in simple and complex mazes. Neurobiol. Aging 11, 499–506. doi: 10.1016/0197-4580(90)90110-l
Barnes, C. A., Nadel, L., and Honig, W. K. (1980). Spatial memory deficit in senescent rats. Can. J. Psychol. 34, 29–39. doi: 10.1037/h0081022
Bartsch, T., Schönfeld, R., Müller, F. J., Alfke, K., Leplow, B., Aldenhoff, J., et al. (2010). Focal lesions of human hippocampal CA1 neurons in transient global amnesia impair place memory. Science 328, 1412–1415. doi: 10.1126/science.1188160
Blanchard, J., Decorte, L., Noguès, X., and Micheau, J. (2009). Characterization of cognition alteration across the course of the disease in APP751SL mice with parallel estimation of cerebral Aβ deposition. Behav. Brain Res. 201, 147–157. doi: 10.1016/j.bbr.2009.02.005
Blanchard, J., Martel, G., Guillou, J.-L., Noguès, X., and Micheau, J. (2008). Impairment of spatial memory consolidation in APP751SL mice results in cue-guided response. Neurobiol. Aging 29, 1011–1021. doi: 10.1016/j.neurobiolaging.2007.02.010
Bohbot, V. D., Iaria, G., and Petrides, M. (2004). Hippocampal function and spatial memory: evidence from functional neuroimaging in healthy participants and performance of patients with medial temporal lobe resections. Neuropsychology 18, 418–425. doi: 10.1037/0894-4105.18.3.418
Braak, H., and Braak, E. (1991). Demonstration of amyloid deposits and neurofibrillary changes in whole brain sections. Brain Pathol 1, 213–216. doi: 10.1111/j.1750-3639.1991.tb00661.x
Braak, H., Jellinger, K., Braak, E., and Bohl, J. (1992). Allocortical neurofibrillary changes in progressive supranuclear palsy. Acta Neuropathol. 84, 478–483. doi: 10.1007/bf00304466
Chapman, P. F., White, G. L., Jones, M. W., Cooper-Blacketer, D., Marshall, V. J., Irizarry, M., et al. (1999). Impaired synaptic plasticity and learning in aged amyloid precursor protein transgenic mice. Nat. Neurosci. 2, 271–276. doi: 10.1038/6374
Chen, G., Chen, K. S., Knox, J., Inglis, J., Bernard, A., Martin, S. J., et al. (2000). A learning deficit related to age and β-amyloid plaques in a mouse model of Alzheimer’s disease. Nature 408, 975–979. doi: 10.1038/35050103
Cioanca, O., Hritcu, L., Mihasan, M., and Hancianu, M. (2013). Cognitive-enhancing and antioxidant activities of inhaled coriander volatile oil in amyloid β(1–42) rat model of Alzheimer’s disease. Physiol. Behav. 120, 193–202. doi: 10.1016/j.physbeh.2013.08.006
Desikan, R. S., McEvoy, L. K., Holland, D., Thompson, W. K., Brewer, J. B., Aisen, P. S., et al. (2013). Apolipoprotein E epsilon4 does not modulate amyloid-β-associated neurodegeneration in preclinical Alzheimer disease. AJNR Am. J. Neuroradiol. 34, 505–510. doi: 10.3174/ajnr.a3267
Dickey, C. A., Gordon, M. N., Mason, J. E., Wilson, N. J., Diamond, D. M., Guzowski, J. F., et al. (2004). Amyloid suppresses induction of genes critical for memory consolidation in APP + PS1 transgenic mice. J. Neurochem. 88, 434–442. doi: 10.1111/j.1471-4159.2004.02185.x
Dickey, C. A., Loring, J. F., Montgomery, J., Gordon, M. N., Eastman, P. S., and Morgan, D. (2003). Selectively reduced expression of synaptic plasticity-related genes in amyloid precursor protein + presenilin-1 transgenic mice. J. Neurosci. 23, 5219–5226.
Eustache, F., Piolino, P., Giffard, B., Viader, F., De La Sayette, V., Baron, J. C., et al. (2004). ‘In the course of time’: a PET study of the cerebral substrates of autobiographical amnesia in Alzheimer’s disease. Brain 127, 1549–1560. doi: 10.1093/brain/awh166
Foster, T. C. (1999). Involvement of hippocampal synaptic plasticity in age-related memory decline. Brain Res. Rev. 30, 236–249. doi: 10.1016/s0165-0173(99)00017-x
Foster, T. C., Defazio, R. A., and Bizon, J. L. (2012). Characterizing cognitive aging of spatial and contextual memory in animal models. Front. Aging Neurosci. 4:12. doi: 10.3389/fnagi.2012.00012
Frautschy, S. A., Yang, F., Calderón, L., and Cole, G. M. (1996). Rodent models of Alzheimer’s disease: rat Aβ infusion approaches to amyloid deposits. Neurobiol. Aging 17, 311–321. doi: 10.1016/0197-4580(95)02073-x
Goodrich-Hunsaker, N. J., Livingstone, S. A., Skelton, R. W., and Hopkins, R. O. (2010). Spatial deficits in a virtual water maze in amnesic participants with hippocampal damage. Hippocampus 20, 481–491. doi: 10.1002/hipo.20651
Gravina, S. A., Ho, L., Eckman, C. B., Long, K. E., Otvos, L. Jr., Younkin, L. H., et al. (1995). Amyloid β protein (Aβ) in Alzheimer’s disease brain. Biochemical and immunocytochemical analysis with antibodies specific for forms ending at Aβ40 or Aβ42(43). J. Biol. Chem. 270, 7013–7016. doi: 10.1074/jbc.270.13.7013
Hamm, V., Héraud, C., Bott, J. B., Herbeaux, K., Strittmatter, C., Mathis, C., et al. (2017). Differential contribution of APP metabolites to early cognitive deficits in a TgCRND8 mouse model of Alzheimer’s disease. Sci. Adv. 3:e1601068. doi: 10.1126/sciadv.1601068
Han, M., Liu, Y., Tan, Q., Zhang, B., Wang, W., Liu, J., et al. (2011). Therapeutic efficacy of stemazole in a β-amyloid injection rat model of Alzheimer’s disease. Eur. J. Pharmacol. 657, 104–110. doi: 10.1016/j.ejphar.2011.01.065
Hardy, J., and Selkoe, D. J. (2002). The amyloid hypothesis of Alzheimer’s disease: progress and problems on the road to therapeutics. Science 297, 353–356. doi: 10.1126/science.1072994
Harrison, F. E., Reiserer, R. S., Tomarken, A. J., and McDonald, M. P. (2006). Spatial and nonspatial escape strategies in the Barnes maze. Learn. Mem. 13, 809–819. doi: 10.1101/lm.334306
Head, D., and Isom, M. (2010). Age effects on wayfinding and route learning skills. Behav. Brain Res. 209, 49–58. doi: 10.1016/j.bbr.2010.01.012
Iaria, G., Palermo, L., Committeri, G., and Barton, J. J. (2009). Age differences in the formation and use of cognitive maps. Behav. Brain Res. 196, 187–191. doi: 10.1016/j.bbr.2008.08.040
Iwatsubo, T., Odaka, A., Suzuki, N., Mizusawa, H., Nukina, N., and Ihara, Y. (1994). Visualization of Aβ 42(43) and Aβ 40 in senile plaques with end-specific Aβ monoclonals: evidence that an initially deposited species is Aβ42(43). Neuron 13, 45–53. doi: 10.1016/0896-6273(94)90458-8
Jansen, P., Schmelter, A., and Heil, M. (2010). Spatial knowledge acquisition in younger and elderly adults: a study in a virtual environment. Exp. Psychol. 57, 54–60. doi: 10.1027/1618-3169/a000007
Janus, C. (2004). Search strategies used by APP transgenic mice during navigation in the Morris water maze. Learn. Mem. 11, 337–346. doi: 10.1101/lm.70104
Kang, S., Moon, N. R., Kim, D. S., Kim, S. H., and Park, S. (2015). Central acylated ghrelin improves memory function and hippocampal AMPK activation and partly reverses the impairment of energy and glucose metabolism in rats infused with β-amyloid. Peptides 71, 84–93. doi: 10.1016/j.peptides.2015.07.005
Kaskie, B., and Storandt, M. (1995). Visuospatial deficit in dementia of the Alzheimer type. Arch. Neurol. 52, 422–425. doi: 10.1001/archneur.1995.00540280120025
Kowall, N. W., Beal, M. F., Busciglio, J., Duffy, L. K., and Yankner, B. A. (1991). An in vivo model for the neurodegenerative effects of β amyloid and protection by substance P. Proc. Natl. Acad. Sci. U S A 88, 7247–7251. doi: 10.1073/pnas.88.16.7247
Kumaran, D., Hassabis, D., Spiers, H. J., Vann, S. D., Vargha-Khadem, F., and Maguire, E. A. (2007). Impaired spatial and non-spatial configural learning in patients with hippocampal pathology. Neuropsychologia 45, 2699–2711. doi: 10.1016/j.neuropsychologia.2007.04.007
Lithfous, S., Dufour, A., and Despres, O. (2013). Spatial navigation in normal aging and the prodromal stage of Alzheimer’s disease: insights from imaging and behavioral studies. Ageing Res. Rev. 12, 201–213. doi: 10.1016/j.arr.2012.04.007
McDonald, R. J., and White, N. M. (1993). A triple dissociation of memory systems: hippocampus, amygdala, and dorsal striatum. Behav. Neurosci. 107, 3–22. doi: 10.1037/0735-7044.107.1.3
McNaughton, B. L., Barnes, C. A., Meltzer, J., and Sutherland, R. J. (1989). Hippocampal granule cells are necessary for normal spatial learning but not for spatially-selective pyramidal cell discharge. Exp. Brain Res. 76, 485–496. doi: 10.1007/bf00248904
Moffat, S. D., Zonderman, A. B., and Resnick, S. M. (2001). Age differences in spatial memory in a virtual environment navigation task. Neurobiol. Aging 22, 787–796. doi: 10.1016/s0197-4580(01)00251-2
Morzelle, M. C., Salgado, J. M., Telles, M., Mourelle, D., Bachiega, P., Buck, H. S., et al. (2016). Neuroprotective effects of pomegranate peel extract after chronic infusion with amyloid-β peptide in mice. PLoS One 11:e0166123. doi: 10.1371/journal.pone.0166123
Nitta, A., Itoh, A., Hasegawa, T., and Nabeshima, T. (1994). β-Amyloid protein-induced Alzheimer’s disease animal model. Neurosci. Lett. 170, 63–66. doi: 10.1016/0304-3940(94)90239-9
O’Leary, T. P., and Brown, R. E. (2009). Visuo-spatial learning and memory deficits on the Barnes maze in the 16-month-old APPswe/PS1dE9 mouse model of Alzheimer’s disease. Behav. Brain Res. 201, 120–127. doi: 10.1016/j.bbr.2009.01.039
O’Leary, T. P., and Brown, R. E. (2012). The effects of apparatus design and test procedure on learning and memory performance of C57BL/6J mice on the Barnes maze. J. Neurosci. Methods 203, 315–324. doi: 10.1016/j.jneumeth.2011.09.027
Palop, J. J., Jones, B., Kekonius, L., Chin, J., Yu, G. Q., Raber, J., et al. (2003). Neuronal depletion of calcium-dependent proteins in the dentate gyrus is tightly linked to Alzheimer’s disease-related cognitive deficits. Proc. Natl. Acad. Sci. U S A 100, 9572–9577. doi: 10.1073/pnas.1133381100
Paxinos, G., and Watson, C. (2007). The Rat Brain in Stereotaxic Coordinates. 6th Edn. Oxford: Elsevier.
Pike, C. J., Walencewicz, A. J., Glabe, C. G., and Cotman, C. W. (1991). In vitro aging of β-amyloid protein causes peptide aggregation and neurotoxicity. Brain Res. 563, 311–314. doi: 10.1016/0006-8993(91)91553-d
Plancher, G., Gyselinck, V., Nicolas, S., and Piolino, P. (2010). Age effect on components of episodic memory and feature binding: a virtual reality study. Neuropsychology 24, 379–390. doi: 10.1037/a0018680
Pompl, P. N., Mullan, M. J., Bjugstad, K., and Arendash, G. W. (1999). Adaptation of the circular platform spatial memory task for mice: use in detecting cognitive impairment in the APP(SW) transgenic mouse model for Alzheimer’s disease. J. Neurosci. Methods 87, 87–95. doi: 10.1016/s0165-0270(98)00169-1
Poucet, B., Herrmann, T., and Buhot, M. C. (1991). Effects of short-lasting inactivations of the ventral hippocampus and medial septum on long-term and short-term acquisition of spatial information in rats. Behav. Brain Res. 44, 53–65. doi: 10.1016/s0166-4328(05)80239-6
Pouzet, B., Zhang, W. N., Feldon, J., and Rawlins, J. N. (2002). Hippocampal lesioned rats are able to learn a spatial position using non-spatial strategies. Behav. Brain Res. 133, 279–291. doi: 10.1016/s0166-4328(02)00007-4
Prut, L., Abramowski, D., Krucker, T., Levy, C. L., Roberts, A. J., Staufenbiel, M., et al. (2007). Aged APP23 mice show a delay in switching to the use of a strategy in the Barnes maze. Behav. Brain Res. 179, 107–110. doi: 10.1016/j.bbr.2007.01.017
Reiserer, R. S., Harrison, F. E., Syverud, D. C., and McDonald, M. P. (2007). Impaired spatial learning in the APPSwe + PSEN1∆E9 bigenic mouse model of Alzheimer’s disease. Genes Brain Behav. 6, 54–65. doi: 10.1111/j.1601-183x.2006.00221.x
Rodriguez, G. A., Burns, M. P., Weeber, E. J., and Rebeck, G. W. (2013). Young APOE4 targeted replacement mice exhibit poor spatial learning and memory, with reduced dendritic spine density in the medial entorhinal cortex. Learn. Mem. 20, 256–266. doi: 10.1101/lm.030031.112
Rojanathammanee, L., Puig, K. L., and Combs, C. K. (2013). Pomegranate polyphenols and extract inhibit nuclear factor of activated T-cell activity and microglial activation in vitro and in a transgenic mouse model of Alzheimer disease. J. Nutr. 143, 597–605. doi: 10.3945/jn.112.169516
Sá, F., Pinto, P., Cunha, C., Lemos, R., Letra, L., Simões, M., et al. (2012). Differences between early and late-onset Alzheimer’s disease in neuropsychological tests. Front. Neurol. 3:81. doi: 10.3389/fneur.2012.00081
Sadek, J. R., Johnson, S. A., White, D. A., Salmon, D. P., Taylor, K. I., Delapena, J. H., et al. (2004). Retrograde amnesia in dementia: comparison of HIV-associated dementia, Alzheimer’s disease, and Huntington’s disease. Neuropsychology 18, 692–699. doi: 10.1037/0894-4105.18.4.692
Savonenko, A., Xu, G. M., Melnikova, T., Morton, J. L., Gonzales, V., Wong, M. P., et al. (2005). Episodic-like memory deficits in the APPswe/PS1dE9 mouse model of Alzheimer’s disease: relationships to β-amyloid deposition and neurotransmitter abnormalities. Neurobiol. Dis. 18, 602–617. doi: 10.1016/j.nbd.2004.10.022
Serrano-Pozo, A., Frosch, M. P., Masliah, E., and Hyman, B. T. (2011). Neuropathological alterations in Alzheimer disease. Cold Spring Harb. Perspect. Med. 1:a006189. doi: 10.1101/cshperspect.a006189
Skelton, R. W., Bukach, C. M., Laurance, H. E., Thomas, K. G., and Jacobs, J. W. (2000). Humans with traumatic brain injuries show place-learning deficits in computer-generated virtual space. J. Clin. Exp. Neuropsychol. 22, 157–175. doi: 10.1076/1380-3395(200004)22:2;1-1;ft157
Sperling, R. A., Jack, C. R. Jr., Black, S. E., Frosch, M. P., Greenberg, S. M., Hyman, B. T., et al. (2011). Amyloid-related imaging abnormalities in amyloid-modifying therapeutic trials: recommendations from the Alzheimer’s Association Research Roundtable Workgroup. Alzheimers Dement. 7, 367–385. doi: 10.1016/j.jalz.2011.05.2351
Sperling, R. A., Rentz, D. M., Johnson, K. A., Karlawish, J., Donohue, M., Salmon, D. P., et al. (2014). The A4 study: stopping AD before symptoms begin? Sci. Transl. Med. 6:228fs13. doi: 10.1126/scitranslmed.3007941
Sunyer, B., Patil, S., Hoger, H., and Lubec, G. (2007). Barnes maze, a useful task to assess spatial reference memory in the mice. Nat. Protoc. doi: 10.1038/nprot.2007.390 [Epub ahead of print].
Suzuki, N., Cheung, T. T., Cai, X. D., Odaka, A., Otvos, L. Jr., Eckman, C., et al. (1994). An increased percentage of long amyloid β protein secreted by familial amyloid β protein precursor (β APP717) mutants. Science 264, 1336–1340. doi: 10.1126/science.8191290
Tarawneh, R., and Holtzman, D. M. (2012). The clinical problem of symptomatic Alzheimer disease and mild cognitive impairment. Cold Spring Harb. Perspect. Med. 2:a006148. doi: 10.1101/cshperspect.a006148
Tran, T. T., Srivareerat, M., Alhaider, I. A., and Alkadhi, K. A. (2011). Chronic psychosocial stress enhances long-term depression in a subthreshold amyloid-β rat model of Alzheimer’s disease. J. Neurochem. 119, 408–416. doi: 10.1111/j.1471-4159.2011.07437.x
West, M. J., Coleman, P. D., Flood, D. G., and Troncoso, J. C. (1994). Differences in the pattern of hippocampal neuronal loss in normal ageing and Alzheimer’s disease. Lancet 344, 769–772. doi: 10.1016/s0140-6736(94)92338-8
Keywords: neurodegenerative disease, β-amyloid peptide, barnes maze, navigation, strategy
Citation: Macêdo PT, Aquino ACQ, Meurer YSR, Brandão LEM, Campêlo CLC, Lima RH, Costa MR, Ribeiro AM and Silva RH (2018) Subtle Alterations in Spatial Memory Induced by Amyloid Peptides Infusion in Rats. Front. Aging Neurosci. 10:18. doi: 10.3389/fnagi.2018.00018
Received: 04 September 2017; Accepted: 15 January 2018;
Published: 30 January 2018.
Edited by:
Fernanda Laezza, University of Texas Medical Branch, United StatesReviewed by:
Eddy A. Van Der Zee, University of Groningen, NetherlandsAlmira Vazdarjanova, Augusta University, United States
Copyright © 2018 Macêdo, Aquino, Meurer, Brandão, Campêlo, Lima, Costa, Ribeiro and Silva. This is an open-access article distributed under the terms of the Creative Commons Attribution License (CC BY). The use, distribution or reproduction in other forums is permitted, provided the original author(s) and the copyright owner are credited and that the original publication in this journal is cited, in accordance with accepted academic practice. No use, distribution or reproduction is permitted which does not comply with these terms.
*Correspondence: Regina H. Silva, reginahsilva@gmail.com