- 1Life and Health Sciences Research Institute (ICVS), School of Medicine, University of Minho, Braga, Portugal
- 2ICVS/3B’s—PT Government Associate Laboratory, Braga, Portugal
- 3Clinical Academic Center — Braga, Braga, Portugal
Functional hemispheric asymmetries have been described in different cognitive processes, such as decision-making and motivation. Variations in the pattern of left/right activity have been associated with normal brain functioning, and with neuropsychiatric diseases. Such asymmetries in brain activity evolve throughout life and are thought to decrease with aging, but clear associations with cognitive function have never been established. Herein, we assessed functional laterality during a working memory task (N-Back) in a healthy aging cohort (over 50 years old) and associated these asymmetries with performance in the test. Activity of lobule VI of the cerebellar hemisphere and angular gyrus was found to be lateralized to the right hemisphere, while the precentral gyrus presented left > right activation during this task. Interestingly, 1-Back accuracy was positively correlated with left > right superior parietal lobule activation, which was mostly due to the influence of the left hemisphere. In conclusion, although regions were mostly symmetrically activated during the N-Back task, performance in working memory in aged individuals seems to benefit from lateralized involvement of the superior parietal lobule.
Introduction
The human brain presents several marked structural asymmetries such as the Yakovlevian torque (Toga and Thompson, 2003; Hugdahl, 2011) or the asymmetry of the planum temporale (Takao et al., 2011), as well as chemical left/right unbalances in dopaminergic (Glick et al., 1982), noradrenergic (Oke et al., 1978) and opioidergic (Watanabe et al., 2015) systems.
It is known since the early observations by Mark Dax and Paul Broca (Broca, 1861; see also Roe and Finger, 1996; Manning and Thomas-Antérion, 2011) that the brain also distributes its functional load in an asymmetrical fashion. Numerous imaging studies have confirmed these observations reporting an asymmetric recruitment of brain regions not only for language processing but also for emotion (Costanzo et al., 2015), motivation (Poole and Gable, 2014; Hughes et al., 2015), memory (Otsuka et al., 2008; Brambilla et al., 2015) and even general intelligence (Santarnecchi et al., 2015). A relevant body of literature also describes abnormal lateralization associated with neuropsychiatric diseases such as autism (Conti et al., 2016), schizophrenia (Royer et al., 2015) and dyslexia (Leonard and Eckert, 2008; Altarelli et al., 2014) suggesting that fine-tuned asymmetry is fundamental for, or at least reflects, proper functioning. In aged individuals, marked structural asymmetries have been identified (Esteves et al., 2017) and functional studies have systematically reported decreases in lateralization; such has been observed in word encoding/retrieval (Cabeza et al., 1997; Madden et al., 1999; Stebbins et al., 2002), working memory (Reuter-Lorenz et al., 2000), face recognition (Grady et al., 2002), inhibitory control (Nielson et al., 2002), risk-taking (Lee et al., 2008) and error processing (Zhu et al., 2010). Such bilateral activity pattern has been hypothesized to result from a compensatory recruitment (Cabeza, 2002) and, in fact, as cognitively efficient older performers recruit additional contra-lateral networks (Cabeza et al., 2002; Erickson et al., 2007) symmetrical activation could be a correlate of good cognitive aging.
Nonetheless, the differential left/right hemisphere involvement in working memory, both in the general population and in distinct age groups, is still not clear. We hypothesize that left and right areas are involved in working memory in different degrees in an older population. Herein, we evaluated functional laterality in an aged cohort using a cognitively stringent working memory task—N-Back (Vermeij et al., 2012)—in two different memory loads (1- and 2-back tasks), aiming to assess the validity of our hypothesis.
Materials and Methods
Ethics Statement
This study was performed in accordance with the Declaration of Helsinki (59th amendment) and approved by national and local ethics review boards (Hospital de Braga, Centro Hospitalar do Alto Ave and Unidade Local de Saúde do Alto Minho) and by the national data protection entity (Comissão Nacional de Protecção de Dados). All volunteers signed informed consent and all medical and research professionals who had access to participants’ identity signed a Statement of Responsibility and Confidentiality.
Subjects
The sample used in the present study was recruited from the Switchbox project. Briefly, a large sample, representative of the older Portuguese population in terms of sex and education, was cognitively characterized (n = 1051, after inclusion/exclusion criteria; subjects were randomly selected from the Guimarães and Vizela local area health authority registries (Costa et al., 2013; Santos et al., 2013, 2014)). For this study, we started with a subcohort of 60 participants; 31 participants were selected because they showed above-minimum accuracy in the N-back task (see below); these subjects displayed high overall cognitive performance (for further detail regarding the participants’ selection process, tests used for the cognitive assessment and the group formation, please consult (Marques et al., 2016)). All participants were right-handed.
Task Design and Minimum Performance Definition
During the fMRI session, participants performed a modified version of the verbal N-Back task. The task was composed by four different conditions: rest condition (Figure 1, top), 0-Back (Figure 1, second row from the top—control condition), 1-Back (Figure 1, third row from the top—low working memory load) and 2-Back (Figure 1, bottom—high working memory load). Four blocks of every condition (16 blocks in total) were pseudo-randomly distributed throughout the experiment. Each block was initiated by the presentation of an instruction card indicating the block condition (6000 ms) followed by 16 trial cards and ended with a pause card (10,000 ms). Each trial card contained a letter which was presented for 500 ms, followed by a fixation cross card for 2000 ms. Response was allowed up to the first 1900 ms of the fixation cross card, totaling 2400 ms of response time.
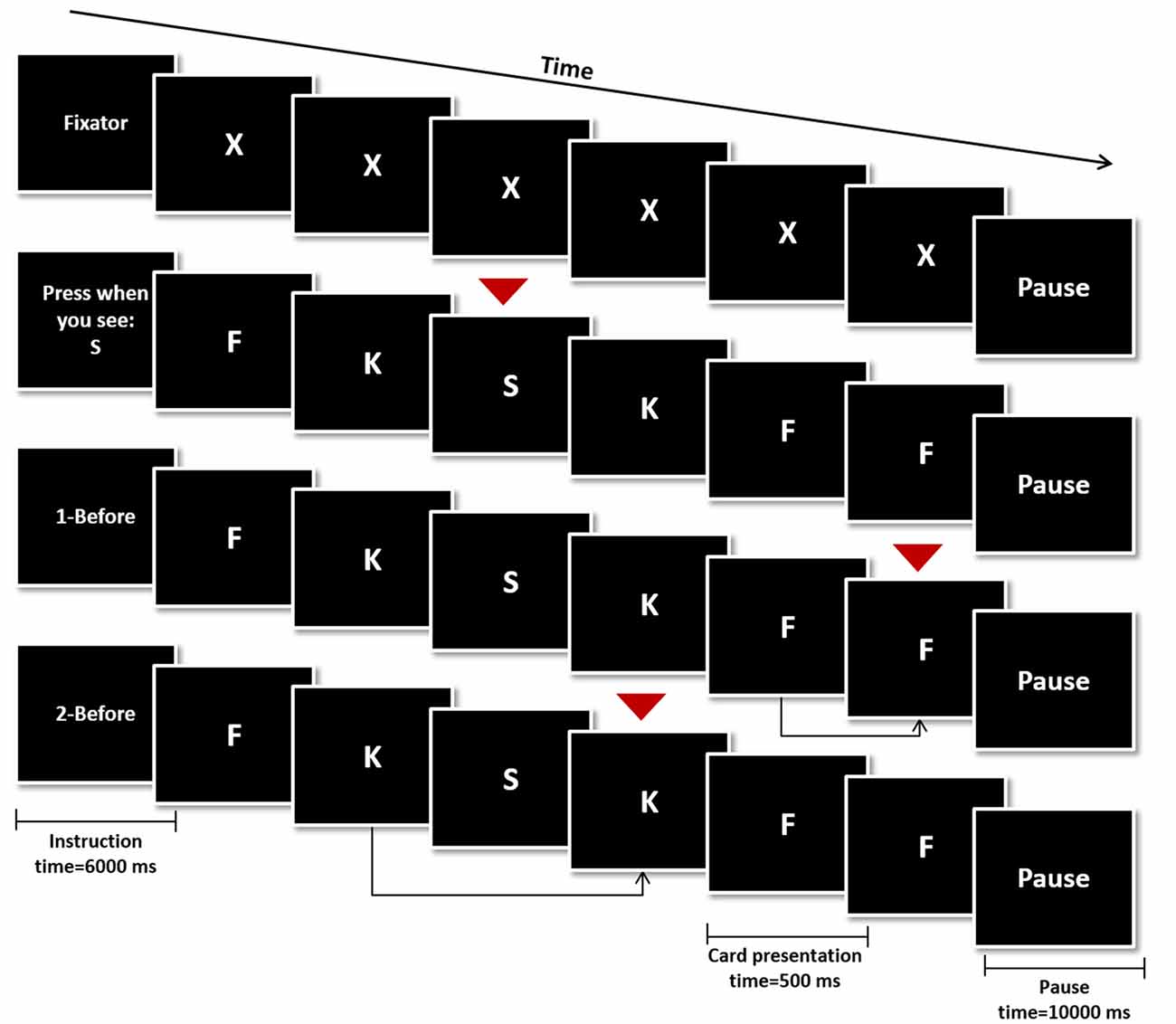
Figure 1. Schematic representation of the N-Back Task. The four conditions were initiated by an instruction card (6000 ms), followed by the presentation of 16 trial cards (500 ms) and ended with a pause card (10,000 ms). In the rest condition the letter “X” was presented in every trial and none was a target card (top). In the remaining conditions, target cards (red arrows) were a specific letter (0-Back—second row from the top), any card similar to the one shown in the previous trial (1-Back—third row from the top) or any card similar to the one shown two trials before (2-Back—bottom row).
Participants were instructed to press a button if the trial card presented was a target card during the control and experimental conditions (0−, 1− and 2-Back). On 0-Back blocks, the target letter was presented in the instruction card and participants were instructed to press the button in trial cards showing the same letter. On 1-Back, the target was any letter equal to the one presented one trial before and on 2-Back, subjects should press the button if the letter corresponded to the one presented two trials before. Finally on the rest condition blocks participants were instructed to remain still, looking at the screen, without pressing any button while the letter “X” was presented in trial cards.
Inclusion of subjects in the fMRI analysis was dependent on N-Back performance. Accuracy (Acc) per block was calculated as Acc = 1−(inc/4), where inc = number of incorrect answers in each block (omissions and non-target button presses) and 4 is the maximum number of possible correct responses per block. The worst trial of each condition was excluded and subjects were included in the analyses if average Acc ≥ 0.5 (i.e., number of correct responses ≥ number of incorrect responses), in order to guarantee subject’s engagement in the task. Following these criteria 31 and 22 participants were included for1-Back and 2-Back analyses, respectively.
Image Acquisition
Acquisitions were performed on a clinically approved Siemens Magnetom Avanto 1.5 T (Siemens Medical Solutions, Elangen, Germany) scanner in Hospital de Braga, using a 12-channel receive-only Siemens head coil. Two acquisitions were used in the current work: as a structural acquisition a T1-weighted magnetization-prepared rapid gradient echo (MPRAGE) sequence with repetition time (TR) = 2730 ms, echo time (TE) = 3.5 ms, flip angle (FA) = 7°, field of view (FoV) = 256 × 256 mm, with isotropic resolution of 1 mm and no slice-gap; the functional acquisition consisted of a T2-weighted Echo Planar Imaging (EPI) sequence, sensible to blood oxygen level dependent (BOLD) contrast, with TR = 2000 ms, TE = 30 ms, FA = 90°, FoV = 224 × 224 mm, in plane resolution = 3.5 × 3.5 mm, slice thickness = 4 mm, slice-gap = 0.48 mm slice gap, 30 interleaved ascending slices and with 456 volumes for a total of 15 min and 12 s acquisition time. The task was presented using the fully integrated fMRI system IFIS-SA (Invivo Corporation, Orlando, FL, USA) and the same system was used to record the subject key-press responses.
fMRI and Statistical Analysis
Pre-processing of functional data was performed using SPM81 and consisted of the following steps: slice timing correction, within subject registration of each volume to the first volume of the acquisition in order to correct for head movement, non-linear spatial normalization to MNI standard space, resampling to 2 mm isotropic resolution, spatial smoothing using an 8 mm FWHM Gaussian kernel and high-pass temporal filtering at 128 s.
The first-level analysis was performed using the General Linear Model (GLM) framework implemented in SPM. For the included participants, a total of 14 regressors were introduced in the model: one regressor modeling the instruction and pause cards; one regressor for the rest condition blocks; three regressors, one per experimental condition (0−, 1− and 2-Back) modeling the three included blocks; three regressors, one per experimental condition modeling the block with worst accuracy, excluding it from the analysis; six movement regressors. Contrasts of interest were set to create maps of increased or decreased activity in low working memory load (1- > 0-Back and 1- < 0-Back), high working memory load (2- > 0-Back and 2- < 0-Back) and high working memory load compared to low working memory load (2- > 1-Back and 2- < 1-Back). In the second-level analysis (group level) one-sample t-tests were performed for the above-mentioned contrasts and areas were considered active for each condition if the corresponding family wise error (FWE) corrected p-values were <0.05 at the voxel level.
Laterality assessment was performed based on the regions of the Automated Anatomical Labeling (AAL) atlas (Tzourio-Mazoyer et al., 2002) in which active voxels were found and were based on the magnitude signal change as previously described (Jansen et al., 2006). Briefly, mean maximum activation was calculated for each region of interest (ROI) as the mean of the 5% of voxels evidencing the highest T-score. Voxels with signal intensity change higher than 50% of the mean maximum activation were included.
Statistical analyses were performed on Matlab R2009b software (The MathWorks, Inc., Natick, MA, United States) and images were computed in Prism 6 (GraphPad Software Inc.) and MRIcro (Rorden and Brett, 2000). Independent and paired analyses were performed respectively for population comparisons and assessment of left/right differences in activation. Non-parametric tests were used whenever normality could not be confirmed. For linear regressions, laterality degree (LD) was calculated as L = AL − AR, where AL = left activation of the specified region on the specified contrast and AR = right activation of the specified region on the specified contrast. P-value < 0.05 was considered the threshold for statistical significance and Bonferroni correction was applied whenever multiple comparisons were performed. Data is shown as mean ± standard deviation.
Results
Population Characterization
Thirty-one subjects showed above-minimum accuracy for inclusion in 1- vs. 0-Back analysis respectively and 22 out of these 31 were also included for 2- vs. 0-Back and 2- vs. 1-Back analyses. Included individuals significantly differed from the remaining population of 29 subjects in terms of gender (32.258 vs. 57.143% female), age (60.290 ± 7.708 vs. 69.035 ± 7.356 years old; Z = 3.861; p < 0.001; Cohen’s d = 1.161) and education (8.484 ± 4.545 vs. 5.000 ± 3.162 years of formal education; Z = 3.515; p < 0.001; Cohen’s d = 0.890).
N-Back-Associated BOLD Response and Patterns of Asymmetry
One-sample t-tests for contrasts 1-Back > 0-Back (Figure 2, top), 1-Back < 0-Back (Figure 2, bottom), 2-Back > 0-Back (Figure 3, top) and 2-Back < 0-Back (Figure 3, bottom) showed that BOLD response during N-Back performance followed the expected patterns (Owen et al., 2005). No activated/deactivated areas were found in the 2- vs. 1-Back contrasts.
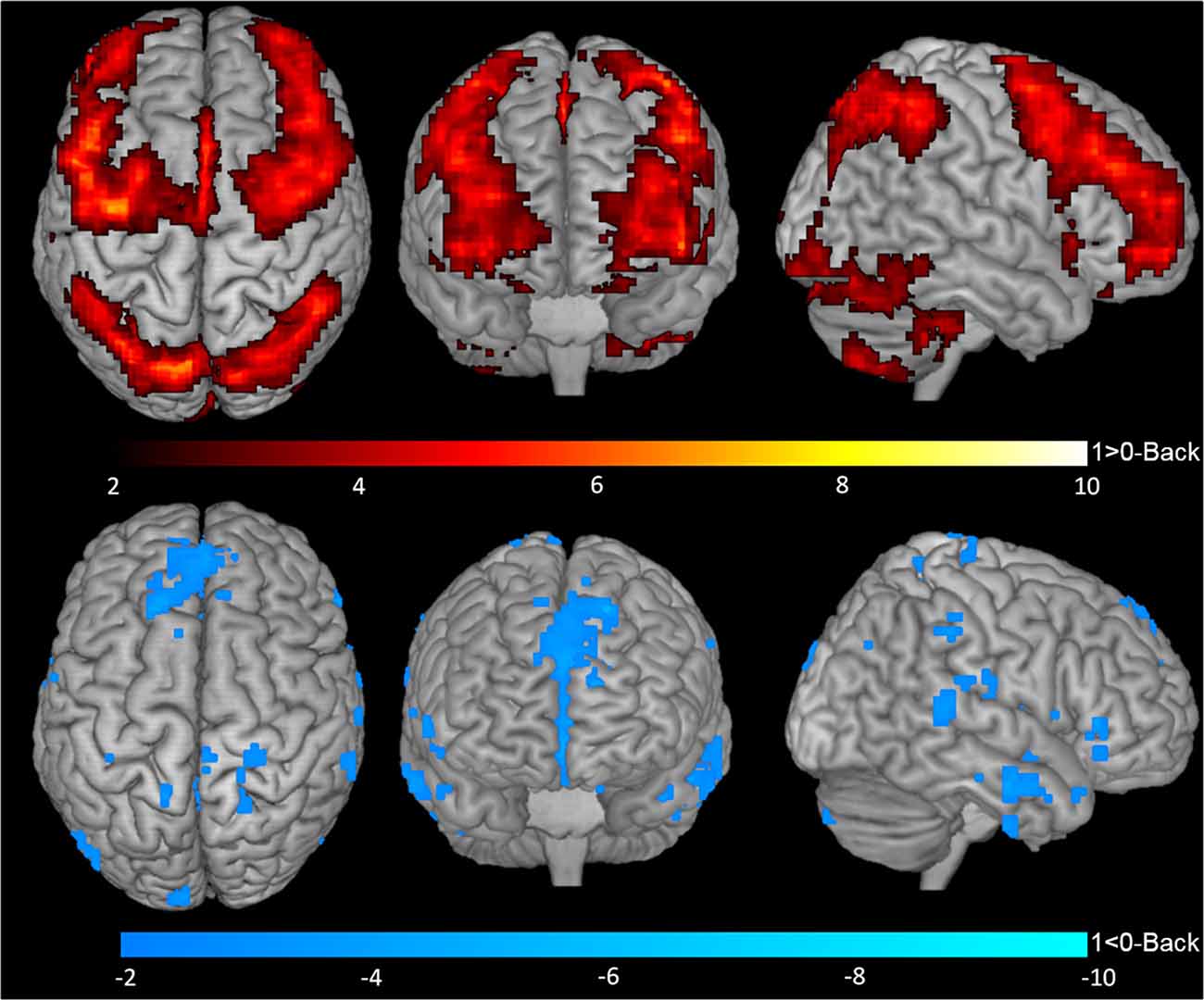
Figure 2. Blood oxygen level dependent (BOLD) response during 1-Back performance. Representation of 1-Back performance functional activation (1-Back > 0-Back—top) and deactivation (1-Back < 0-Back—bottom) in axial (left), coronal (middle) and sagittal (right) views. Hotter and colder colors represent respectively increased activation and deactivation.
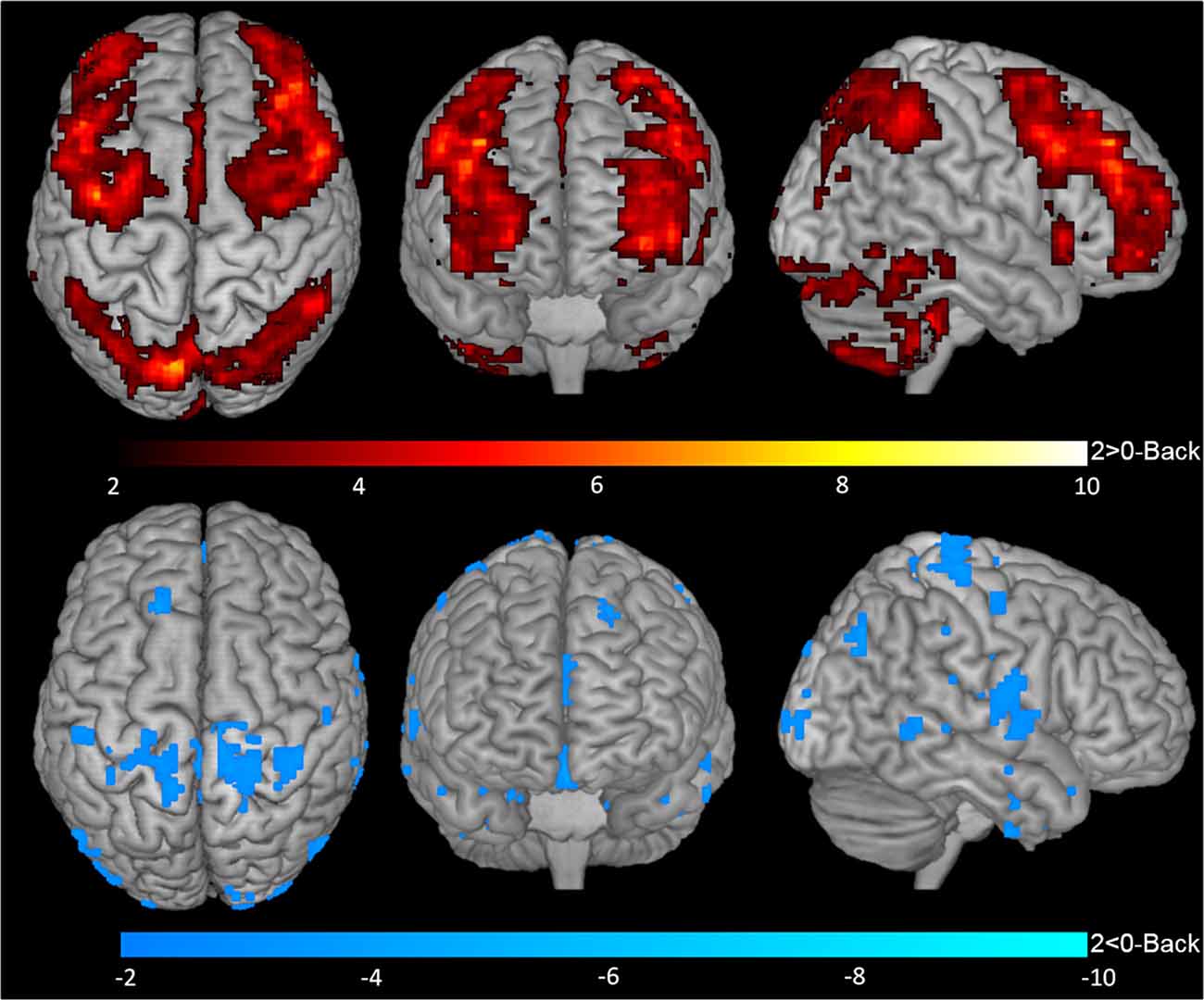
Figure 3. BOLD response during 2-Back performance. Representation of 2-Back performance functional activation (2-Back > 0-Back—top) and deactivation (2-Back < 0-Back—bottom) in axial (left), coronal (middle) and sagittal (right) views. Hotter and colder colors represent respectively increased activation and deactivation.
A total of 20 ROIs were found to be either activated (1-Back > 0-Back) or deactivated (1-Back < 0-Back) in the 1-Back condition (Table 1, Figure 2). These comprised the superior and inferior parietal lobules, Crus I of cerebellar hemisphere, orbital part of the middle frontal gyrus, lobules VI and VII of cerebellar hemisphere, middle frontal and precentral gyri, Crus II of cerebellar hemisphere, supplementary motor area, pars triangularis of the inferior frontal gyrus, lobule VIII of cerebellar hemisphere, superior frontal and angular gyri, precuneus, insula, rolandic operculum, medial frontal and superior temporal gyri and medial orbitofrontal cortex.
During the 2-Back part of the task, 14 regions were involved (Table 1, Figure 3), namely superior and inferior parietal lobules, Crus I of cerebellar hemisphere, orbital part of the middle frontal gyrus, lobule VI of cerebellar hemisphere, precentral gyrus, pars opercularis of the inferior frontal gyrus, middle frontal gyrus, pars triangularis of the inferior frontal gyrus, superior occipital, pars orbitalis of the inferior frontal gyrus, precuneus, lobules IV and V of cerebellar hemisphere and insula.
Asymmetry of the activated areas was analyzed by comparing left and right BOLD responses. During 1-Back performance (Figure 4, Supplementary Table S1), R > L activation was found in the lobule VI of the cerebellum (α = 0.016; Z = 3.331; Cohen’s d = −0.197) and in the angular gyrus (α = 0.010; Z = 3.469; Cohen’s d = −0.497). L > R activation occurred in the precentral gyrus (α < 0.001; Z = 4.527; Cohen’s d = 0.655).
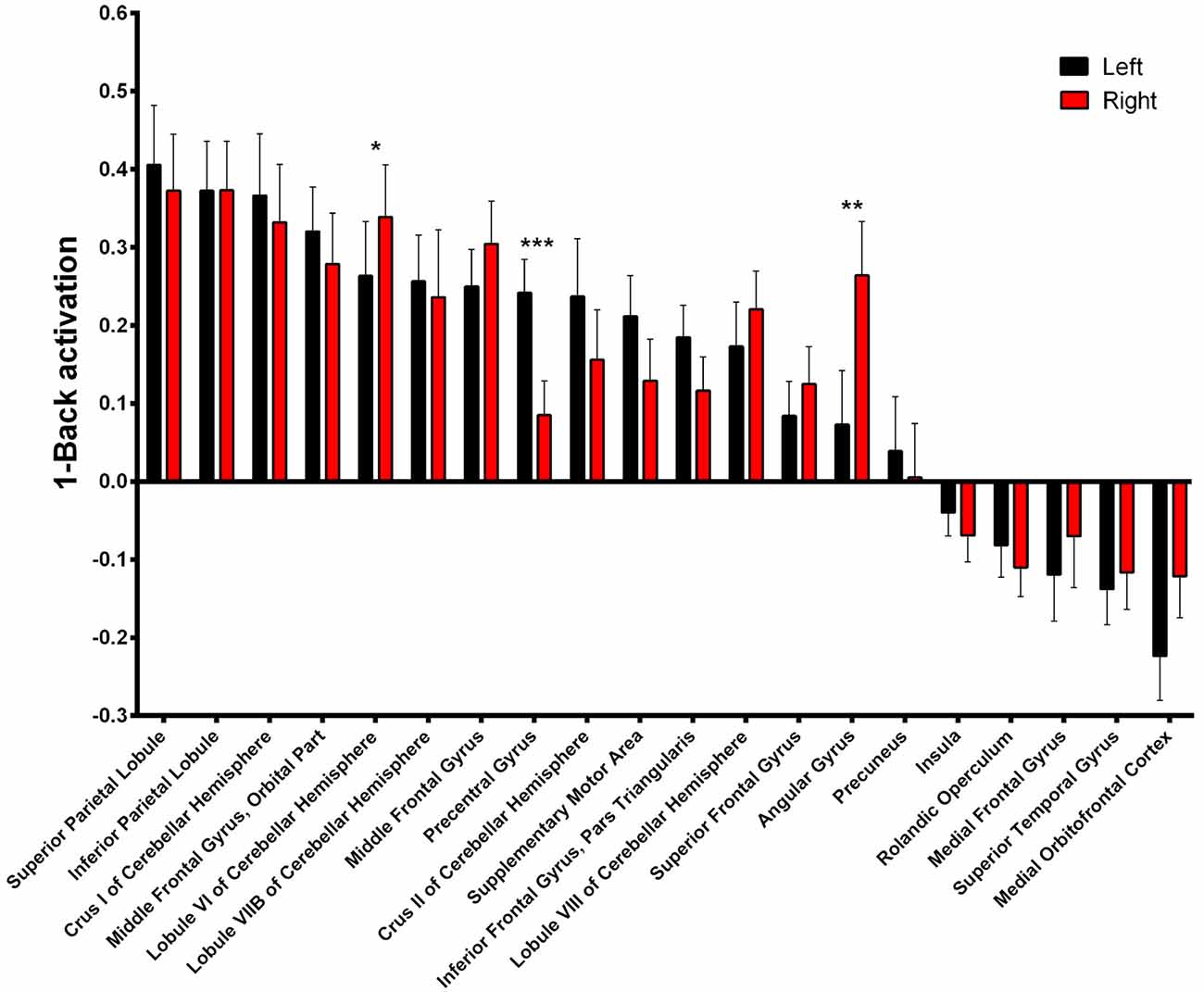
Figure 4. 1-Back-related BOLD asymmetries. Comparison between left and right activations/deactivations during 1-Back performance. *α < 0.05, **α < 0.01, ***α < 0.001 in Bonferroni-corrected paired comparisons.
During 2-Back trials (Figure 5, Supplementary Table S2) R > L activation could be identified in the lobule VI of the cerebellum α = 0.028; Z = 3.068; Cohen’s d = −0.215) while L > R activation was found in the precentral gyrus (α = 0.001; Z = 3.945; Cohen’s d = 0.685).
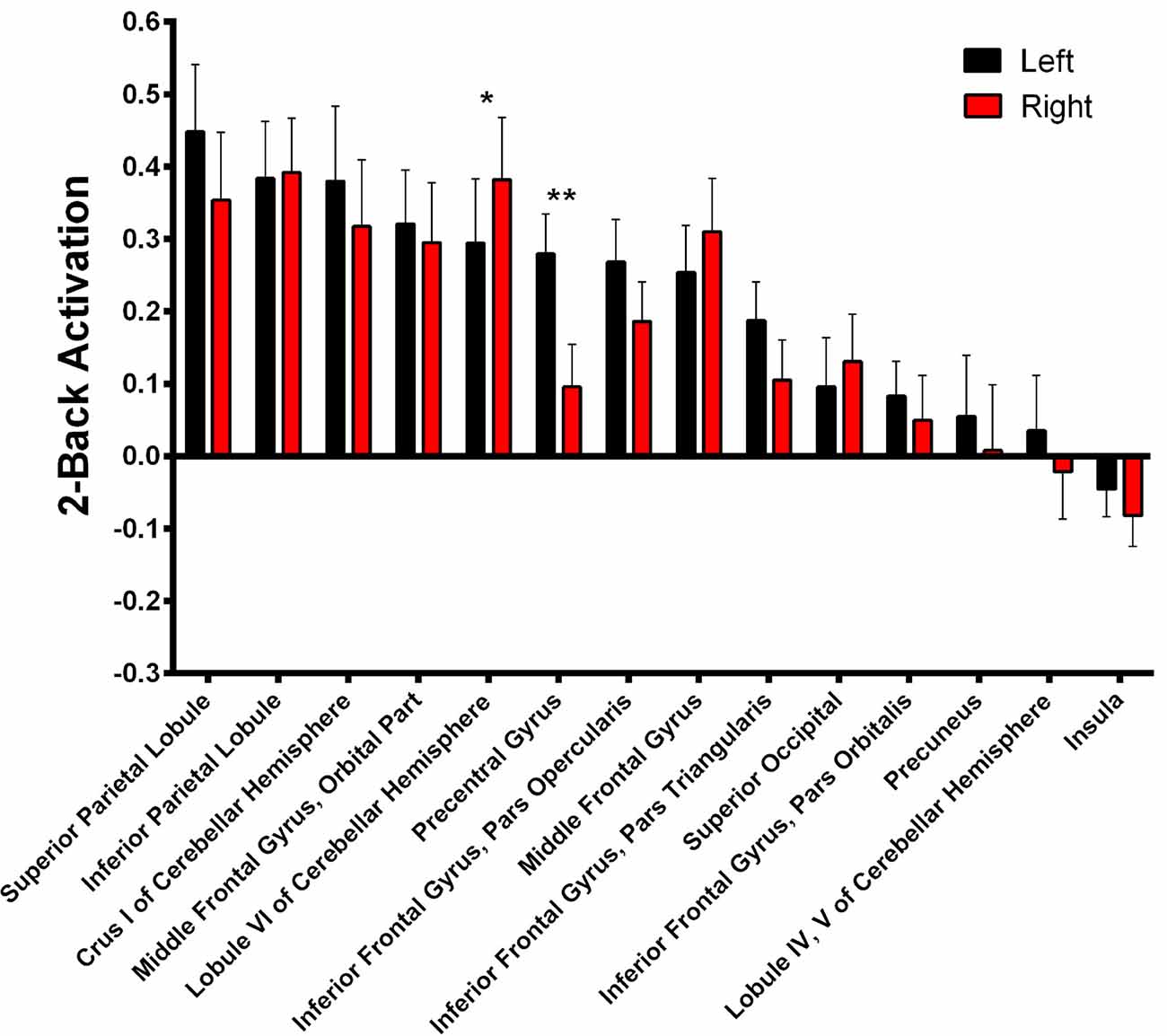
Figure 5. 2-Back-related BOLD asymmetries. Comparison between left and right activations/deactivations during 2-Back performance. *α < 0.05, **α < 0.01 in Bonferroni-corrected paired comparisons.
Lateralized Activation in the Superior Parietal Lobule Is Associated With N-Back Performance
The impact of functional laterality on working memory was determined by establishing models with N-Back Acc as the dependent variable and LD as the independent variable. Superior parietal lobule 1-Back LD showed a positive correlation with Acc during this task. More specifically, we found that a leftward asymmetry in the activation of the superior parietal lobe was associated with better performance (α = 0.033; β = 0.247; R2 = 0.293—Figure 6A, Supplementary Table S3). Importantly, this association was maintained when age and education were added as co-variables in the regression model (p = 0.001; β = 0.270; R2 = 0.416). Simple 1-Back Acc vs. left or right BOLD response correlations showed that this association is mainly due to the left hemisphere contribution (Figure 6B).
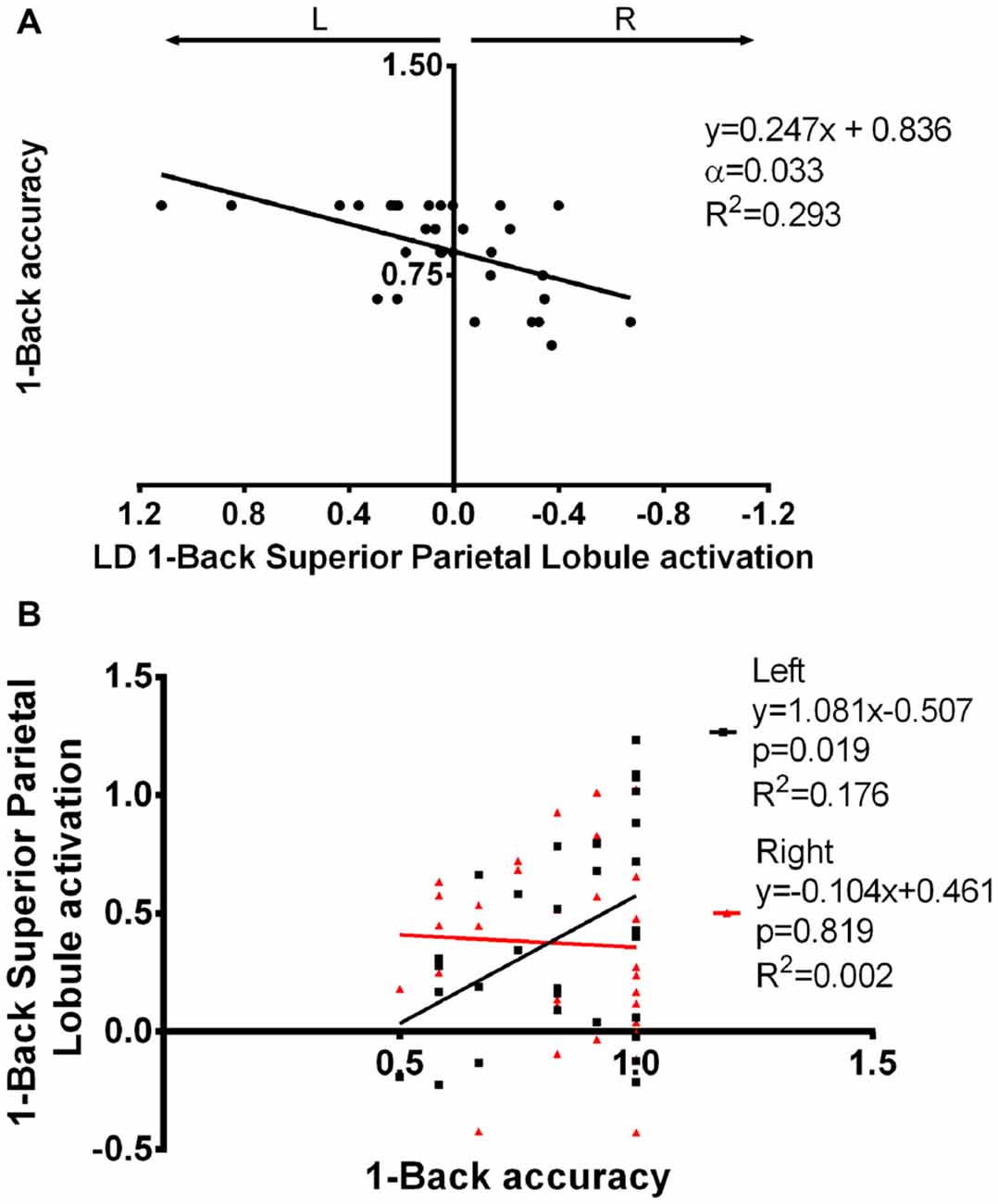
Figure 6. Superior parietal lobule’s LD in association with 1-Back accuracy. (A) Superior parietal lobule’s LD was positively correlated with 1-Back accuracy, i.e., increased left to right difference was associated with better performance. (B) The association seen in (A) seems to be mainly due to a positive correlation between left superior parietal lobule activation and task performance. LD, laterality degree; α, Bonferroni corrected p-value.
No associations between performance and asymmetric activation of brain hemispheres were found in the 2-Back task.
Discussion
In the present study we tested the hypothesis that task related BOLD response occurred in a lateralized fashion which could be associated with working memory performance in an older population. We demonstrated that the majority of the activated areas are similarly activated on the left and right hemispheres, although some asymmetries could be observed. Importantly, superior parietal lobule laterality was positively correlated with 1-Back performance.
The classic example of functional laterality is the well-known left lateralization for language (Toga and Thompson, 2003; Hugdahl, 2011), but degree of asymmetry has also been shown to predict performance in cognitive domains such as verbal and visuospatial ability (Gotts et al., 2013). Herein we evaluated functional lateralization during a verbal N-Back task (1- and 2-Back). The N-Back is a common tool for the study of working memory with a well-established pattern of cortical activation, which utilizes 0-Back as a reference task (Cohen et al., 1994; Owen et al., 2005; Yüksel et al., 2018). Our observations were in accordance with the expected pattern and recruited areas included the inferior parietal lobule, lateral cerebellum and prefrontal cortex (Owen et al., 2005). Asymmetrical activity however, was restricted to the lobule VI of the cerebellar hemisphere (R > L, 1- and 2-Back), precentral (L > R, 1- and 2-Back) and angular gyri (R > L, 1-Back).
The Hemispheric Asymmetry Reduction in Older Adults (HAROLD) model (Cabeza, 2002) postulates that high-performing older adults present compensatory bilateral activity. Cabeza et al. (2002) have shown that prefrontal cortex PET activation was more symmetrical in aged good performers when compared with young individuals or aged poor performers during a working memory task, suggesting a positive correlation between symmetry and performance. Even though these findings have been widely corroborated (Cabeza et al., 2004; Bergerbest et al., 2009; Piefke et al., 2012), some studies are still finding conflicting evidence (Vermeij et al., 2014; Brambilla et al., 2015) and more recently, the Compensation-Related Utilization of Neural Circuits Hypothesis (CRUNCH) was suggested (Reuter-Lorenz and Cappell, 2008; Schneider-Garces et al., 2010). This model postulates that this age-related symmetry is not necessarily due to homotopic activation, but results from an overall compensatory overactivation that happens not only in older adults, but also in younger subjects performing higher load cognitive tasks. In fact, from the 60 individuals initially included in our assay, only about 50% were able to perform the 2-Back task at an acceptable accuracy, indicating a high difficulty level for this specific population and therefore explaining the low number of lateralized areas.
Nonetheless, regarding the lateralized areas, both the right lateralization of the lobule VI of the cerebellar hemisphere and the precentral gyrus’s leftward bias may be associated with motor responses during the task. The latter is a classic motor area whose direction of activation follows the same trend found in the supplementary motor area in the 1-> 0-Back contrast, strongly suggesting an involvement of motor planning and/or execution. Additionally, left and right activation of the precentral gyrus seem similar in both presented contrasts, which is in accordance with previous reports of a load independent activation of the left sensorimotor cortex during the N-Back task (Jansma et al., 2000). Similarly, right but not left lobule VI of the cerebellar hemisphere is activated during right finger tapping (Stoodley and Schmahmann, 2009; Stoodley et al., 2010), although an involvement in working memory cannot be discarded as it was also previously reported as independent of motor performance both in an individual study (Stoodley et al., 2010) and in a meta-analysis (Stoodley and Schmahmann, 2009). Regarding the right > left asymmetry of the angular gyrus, this is the first report of functional lateralization in a working memory task. In fact, its activation in this function has been mostly regarded as bilateral (Carlson et al., 1998; Blokland et al., 2008). Nevertheless, this area has consistently shown lateralized activity in multiple other functions such as semantic and number processing, attention or spatial cognition (Seghier, 2013).
Interestingly, leftward asymmetry of the superior parietal lobule was correlated with better performance in the 1-Back task, which was mainly due to an association between left activation and accuracy. This area is known to be involved in working memory (Rottschy et al., 2013) and Koenigs et al. (2009) have previously found a lateralized effect, in which right lesions decreased working memory performance involving visuospatial manipulation. The authors were not able to find asymmetry effects in other types of working memory (including N-Back), which could be due to the low number of subjects (9 right vs. 4 left lesions). On the other hand, Otsuka et al. (2008) have determined that superior parietal lobule left activation was positively correlated with executive function while right activation was associated with short-term storage, further confirming the importance of laterality in this region.
In conclusion, herein we generated data confirming the areas activated during the N-Back task (Owen et al., 2005) and the reduced number of functionally lateralized areas, potentially due to age (Cabeza, 2002) or to high cognitive load (Reuter-Lorenz and Cappell, 2008). Importantly, lateralized areas were consistent in the two loads of working memory assessed and we were able to show for the first time a direct correlation between functional lateralization of an area and working memory performance. In fact, superior parietal lobule leftward lateralization was associated with improved accuracy in the 1-Back, highlighting the importance of left/right balance for ideal performance.
Author Contributions
NCS and NS designed the cohort and coordinated the evaluations. ME and HL-A designed the study and wrote the first draft of the manuscript. CP-N recruited the subjects. TCC performed the neuropsychological tests. PM and JMS performed the fMRI acquisitions. ME, RM, PM, AA, NCS, NS and HL-A analyzed and interpreted the data.
Funding
This work was supported by NORTE-01-0145-FEDER-000013, supported by the Northern Portugal Regional Operational Programme (NORTE 2020), under the Portugal 2020 Partnership Agreement, through the European Regional Development Fund (FEDER), and was funded by the European Commission (FP7) “SwitchBox—Maintaining health in old age through homeostasis” (Seventh Framework Programme; Contract HEALTH-F2-2010-259772), by FEDER through the Competitiveness Factors Operational Programme (COMPETE) and by National Funds, through the Foundation for Science and Technology (FCT) under the scope of the project POCI-01-0145-FEDER-007038, by the Fundação Calouste Gulbenkian (Portugal; Contract Grant No: P-139977; project “TEMPO—Better mental health during ageing based on temporal prediction of individual brain ageing trajectories”) and by “PANINI—Physical Activity and Nutrition Influences In Ageing” (European Commission (Horizon 2020), Contract GA 675003); Fundação para a Ciência e a Tecnologia (FCT) (Grant Nos. SFRH/BD/52291/2013 to ME and PD/BD/106050/2015 to CP-N via Inter-University Doctoral Programme in Ageing and Chronic Disease (PhDOC), SFRH/BPD/80118/2011 to HL-A and SFRH/BD/90078/2012 to TCC); and FCT/MEC and ON.2–ONOVONORTE—North Portugal Regional Operational Programme 2007/2013, of the National Strategic Reference Framework (NSRF) 2007/2013, through FEDER (project FCTANR/NEU-OSD/0258/2012 to RM).
Conflict of Interest Statement
The authors declare that the research was conducted in the absence of any commercial or financial relationships that could be construed as a potential conflict of interest.
Footnotes
Supplementary Material
The Supplementary Material for this article can be found online at: https://www.frontiersin.org/articles/10.3389/fnagi.2018.00058/full#supplementary-material
References
Altarelli, I., Leroy, F., Monzalvo, K., Fluss, J., Billard, C., Dehaene-Lambertz, G., et al. (2014). Planum temporale asymmetry in developmental dyslexia: revisiting an old question. Hum. Brain Mapp. 35, 5717–5735. doi: 10.1002/hbm.22579
Bergerbest, D., Gabrieli, J. D., Whitfield-Gabrieli, S., Kim, H., Stebbins, G. T., Bennett, D. A., et al. (2009). Age-associated reduction of asymmetry in prefrontal function and preservation of conceptual repetition priming. Neuroimage 45, 237–246. doi: 10.1016/j.neuroimage.2008.10.019
Blokland, G. A. M., McMahon, K. L., Hoffman, J., Zhu, G., Meredith, M., Martin, N. G., et al. (2008). Quantifying the heritability of task-related brain activation and performance during the N-back working memory task: a twin fMRI study. Biol. Psychol. 79, 70–79. doi: 10.1016/j.biopsycho.2008.03.006
Brambilla, M., Manenti, R., Ferrari, C., and Cotelli, M. (2015). Better together: left and right hemisphere engagement to reduce age-related memory loss. Behav. Brain Res. 293, 125–133. doi: 10.1016/j.bbr.2015.07.037
Broca, P. (1861). Remarques sur le sie’ge de la faculte ‘du language articule’, suivies d’une observation d’aphe’mie (perte de la parole). Bull. Soc. Anat. 36, 330–357.
Cabeza, R. (2002). Hemispheric asymmetry reduction in older adults: the HAROLD model. Psychol. Aging 17, 85–100. doi: 10.1037/0882-7974.17.1.85
Cabeza, R., Anderson, N. D., Locantore, J. K., and McIntosh, A. R. (2002). Aging gracefully: compensatory brain activity in high-performing older adults. Neuroimage 17, 1394–1402. doi: 10.1006/nimg.2002.1280
Cabeza, R., Daselaar, S. M., Dolcos, F., Prince, S. E., Budde, M., and Nyberg, L. (2004). Task-independent and task-specific age effects on brain activity during working memory, visual attention and episodic retrieval. Cereb. Cortex 14, 364–375. doi: 10.1093/cercor/bhg133
Cabeza, R., Grady, C. L., Nyberg, L., McIntosh, A. R., Tulving, E., Kapur, S., et al. (1997). Age-related differences in neural activity during memory encoding and retrieval: a positron emission tomography study. J. Neurosci. 17, 391–400.
Carlson, S., Martinkauppi, S., Rämä, P., Salli, E., Korvenoja, A., and Aronen, H. J. (1998). Distribution of cortical activation during visuospatial n-back tasks as revealed by functional magnetic resonance imaging. Cereb. Cortex 8, 743–752. doi: 10.1093/cercor/8.8.743
Cohen, J. D., Forman, S. D., Braver, T. S., Casey, B. J., Servan-Schreiber, D., and Noll, D. C. (1994). Activation of the prefrontal cortex in a nonspatial working memory task with functional MRI. Hum. Brain Mapp. 1, 293–304. doi: 10.1002/hbm.460010407
Conti, E., Calderoni, S., Gaglianese, A., Pannek, K., Mazzotti, S., Rose, S., et al. (2016). Lateralization of brain networks and clinical severity in toddlers with autism spectrum disorder: a HARDI diffusion MRI study. Autism Res. 9, 382–392. doi: 10.1002/aur.1533
Costa, P. S., Santos, N. C., Cunha, P., Palha, J. A., and Sousa, N. (2013). The use of bayesian latent class cluster models to classify patterns of cognitive performance in healthy ageing. PLoS One 8:e71940. doi: 10.1371/journal.pone.0071940
Costanzo, E. Y., Villarreal, M., Drucaroff, L. J., Ortiz-Villafañe, M., Castro, M. N., Goldschmidt, M., et al. (2015). Hemispheric specialization in affective responses, cerebral dominance for language and handedness: Lateralization of emotion, language, and dexterity. Behav. Brain Res. 288, 11–19. doi: 10.1016/j.bbr.2015.04.006
Erickson, K. I., Colcombe, S. J., Wadhwa, R., Bherer, L., Peterson, M. S., Scalf, P. E., et al. (2007). Training-induced plasticity in older adults: effects of training on hemispheric asymmetry. Neurobiol. Aging 28, 272–283. doi: 10.1016/j.neurobiolaging.2005.12.012
Esteves, M., Marques, P., Magalhães, R., Castanho, T. C., Soares, J. M., Almeida, A., et al. (2017). Structural laterality is associated with cognitive and mood outcomes: an assessment of 105 healthy aged volunteers. Neuroimage 153, 86–96. doi: 10.1016/j.neuroimage.2017.03.040
Glick, S. D., Ross, D. A., and Hough, L. B. (1982). Lateral asymmetry of neurotransmitters in human brain. Brain Res. 234, 53–63. doi: 10.1016/0006-8993(82)90472-3
Gotts, S. J., Jo, H. J., Wallace, G. L., Saad, Z. S., Cox, R. W., and Martin, A. (2013). Two distinct forms of functional lateralization in the human brain. Proc. Natl. Acad. Sci. U S A 110, E3435–E3444. doi: 10.1073/pnas.1302581110
Grady, C. L., Bernstein, L. J., Beig, S., and Siegenthaler, A. L. (2002). The effects of encoding task on age-related differences in the functional neuroanatomy of face memory. Psychol. Aging 17, 7–23. doi: 10.1037/0882-7974.17.1.7
Hugdahl, K. (2011). Hemispheric asymmetry: contributions from brain imaging. Wiley Interdiscip. Rev. Cogn. Sci. 2, 461–478. doi: 10.1002/wcs.122
Hughes, D. M., Yates, M. J., Morton, E. E., and Smillie, L. D. (2015). Asymmetric frontal cortical activity predicts effort expenditure for reward. Soc. Cogn. Affect. Neurosci. 10, 1015–1019. doi: 10.1093/scan/nsu149
Jansen, A., Menke, R., Sommer, J., Förster, A. F., Bruchmann, S., Hempleman, J., et al. (2006). The assessment of hemispheric lateralization in functional MRI—robustness and reproducibility. Neuroimage 33, 204–217. doi: 10.1016/j.neuroimage.2006.06.019
Jansma, J. M., Ramsey, N. F., Coppola, R., and Kahn, R. S. (2000). Specific versus nonspecific brain activity in a parametric N-back task. Neuroimage 12, 688–697. doi: 10.1006/nimg.2000.0645
Koenigs, M., Barbey, A. K., Postle, B. R., and Grafman, J. (2009). Superior parietal cortex is critical for the manipulation of information in working memory. J. Neurosci. 29, 14980–14986. doi: 10.1523/JNEUROSCI.3706-09.2009
Lee, T. M., Leung, A. W., Fox, P. T., Gao, J. H., and Chan, C. C. (2008). Age-related differences in neural activities during risk taking as revealed by functional MRI. Soc. Cogn. Affect. Neurosci. 3, 7–15. doi: 10.1093/scan/nsm033
Leonard, C. M., and Eckert, M. A. (2008). Asymmetry and dyslexia. Dev. Neuropsychol. 33, 663–681. doi: 10.1080/87565640802418597
Madden, D. J., Turkington, T. G., Provenzale, J. M., Denny, L. L., Hawk, T. C., Gottlob, L. R., et al. (1999). Adult age differences in the functional neuroanatomy of verbal recognition memory. Hum. Brain Mapp. 7, 115–135. doi: 10.1002/(sici)1097-0193(1999)7:2<115::aid-hbm5>3.0.co;2-n
Manning, L., and Thomas-Antérion, C. (2011). Marc Dax and the discovery of the lateralisation of language in the left cerebral hemisphere. Rev. Neurol. 167, 868–872. doi: 10.1016/j.neurol.2010.10.017
Marques, P. C., Soares, J. M., Magalhães, R. J., Santos, N. C., and Sousa, N. J. (2016). Macro- and micro-structural white matter differences correlate with cognitive performance in healthy aging. Brain Imaging Behav. 10, 168–181. doi: 10.1007/s11682-015-9378-4
Nielson, K. A., Langenecker, S. A., and Garavan, H. (2002). Differences in the functional neuroanatomy of inhibitory control across the adult life span. Psychol. Aging 17, 56–71. doi: 10.1037/0882-7974.17.1.56
Oke, A., Keller, R., Mefford, I., and Adams, R. N. (1978). Lateralization of norepinephrine in human thalamus. Science 200, 1411–1413. doi: 10.1126/science.663623
Otsuka, Y., Osaka, N., and Osaka, M. (2008). Functional asymmetry of superior parietal lobule for working memory in the elderly. Neuroreport 19, 1355–1359. doi: 10.1097/WNR.0b013e32830e000f
Owen, A. M., McMillan, K. M., Laird, A. R., and Bullmore, E. (2005). N-back working memory paradigm: a meta-analysis of normative functional neuroimaging studies. Hum. Brain Mapp. 25, 46–59. doi: 10.1002/hbm.20131
Piefke, M., Onur, Ö. A., and Fink, G. R. (2012). Aging-related changes of neural mechanisms underlying visual-spatial working memory. Neurobiol. Aging 33, 1284–1297. doi: 10.1016/j.neurobiolaging.2010.10.014
Poole, B. D., and Gable, P. A. (2014). Affective motivational direction drives asymmetric frontal hemisphere activation. Exp. Brain Res. 232, 2121–2130. doi: 10.1007/s00221-014-3902-4
Reuter-Lorenz, P. A., and Cappell, K. A. (2008). Neurocognitive aging and the compensation hypothesis. Curr. Dir. Psychol. Sci. 17, 177–182. doi: 10.1111/j.1467-8721.2008.00570.x
Reuter-Lorenz, P. A., Jonides, J., Smith, E. E., Hartley, A., Miller, A., Marshuetz, C., et al. (2000). Age differences in the frontal lateralization of verbal and spatial working memory revealed by PET. J. Cogn. Neurosci. 12, 174–187. doi: 10.1162/089892900561814
Roe, D., and Finger, S. (1996). Gustave Dax and his fight for recognition: an overlooked chapter in the early history of cerebral dominance. J. Hist. Neurosci. 5, 228–240. doi: 10.1080/09647049609525672
Rorden, C., and Brett, M. (2000). Stereotaxic display of brain lesions. Behav. Neurol. 12, 191–200. doi: 10.1155/2000/421719
Rottschy, C., Caspers, S., Roski, C., Reetz, K., Dogan, I., Schulz, J. B., et al. (2013). Differentiated parietal connectivity of frontal regions for “what” and “where” memory. Brain Struct. Funct. 218, 1551–1567. doi: 10.1007/s00429-012-0476-4
Royer, C., Delcroix, N., Leroux, E., Alary, M., Razafimandimby, A., Brazo, P., et al. (2015). Functional and structural brain asymmetries in patients with schizophrenia and bipolar disorders. Schizophr. Res. 161, 210–214. doi: 10.1016/j.schres.2014.11.014
Santarnecchi, E., Tatti, E., Rossi, S., Serino, V., and Rossi, A. (2015). Intelligence-related differences in the asymmetry of spontaneous cerebral activity. Hum. Brain Mapp. 36, 3586–3602. doi: 10.1002/hbm.22864
Santos, N. C., Costa, P. S., Cunha, P., Cotter, J., Sampaio, A., Zihl, J., et al. (2013). Mood is a key determinant of cognitive performance in community-dwelling older adults: a cross-sectional analysis. Age 35, 1983–1993. doi: 10.1007/s11357-012-9482-y
Santos, N. C., Costa, P. S., Cunha, P., Portugal-Nunes, C., Amorim, L., Cotter, J., et al. (2014). Clinical, physical and lifestyle variables and relationship with cognition and mood in aging: a cross-sectional analysis of distinct educational groups. Front. Aging Neurosci. 6:21. doi: 10.3389/fnagi.2014.00021
Schneider-Garces, N. J., Gordon, B. A., Brumback-Peltz, C. R., Shin, E., Lee, Y., Sutton, B. P., et al. (2010). Span, CRUNCH, and beyond: working memory capacity and the aging brain. J. Cogn. Neurosci. 22, 655–669. doi: 10.1162/jocn.2009.21230
Seghier, M. L. (2013). The angular gyrus: multiple functions and multiple subdivisions. Neuroscientist 19, 43–61. doi: 10.1177/1073858412440596
Stebbins, G. T., Carrillo, M. C., Dorfman, J., Dirksen, C., Desmond, J. E., Turner, D. A., et al. (2002). Aging effects on memory encoding in the frontal lobes. Psychol. Aging 17, 44–55. doi: 10.1037/0882-7974.17.1.44
Stoodley, C. J., and Schmahmann, J. D. (2009). Functional topography in the human cerebellum: a meta-analysis of neuroimaging studies. Neuroimage 44, 489–501. doi: 10.1016/j.neuroimage.2008.08.039
Stoodley, C. J., Valera, E. M., and Schmahmann, J. D. (2010). An fMRI study of intra-individual functional topography in the human cerebellum. Behav. Neurol. 23, 65–79. doi: 10.3233/BEN-2010-0268
Takao, H., Abe, O., Yamasue, H., Aoki, S., Sasaki, H., Kasai, K., et al. (2011). Gray and white matter asymmetries in healthy individuals aged 21–29 years: a voxel-based morphometry and diffusion tensor imaging study. Hum. Brain Mapp. 32, 1762–1773. doi: 10.1002/hbm.21145
Toga, A. W., and Thompson, P. M. (2003). Mapping brain asymmetry. Nat. Rev. Neurosci. 4, 37–48. doi: 10.1038/nrn1009
Tzourio-Mazoyer, N., Landeau, B., Papathanassiou, D., Crivello, F., Etard, O., Delcroix, N., et al. (2002). Automated anatomical labeling of activations in SPM using a macroscopic anatomical parcellation of the MNI MRI single-subject brain. Neuroimage 15, 273–289. doi: 10.1006/nimg.2001.0978
Vermeij, A., Van Beek, A. H., Olde Rikkert, M. G., Claassen, J. A., and Kessels, R. P. (2012). Effects of aging on cerebral oxygenation during working-memory performance: a functional near-infrared spectroscopy study. PLoS One 7:e46210. doi: 10.1371/journal.pone.0046210
Vermeij, A., Van Beek, A. H., Reijs, B. L., Claassen, J. A., and Kessels, R. P. (2014). An exploratory study of the effects of spatial working-memory load on prefrontal activation in low- and high-performing elderly. Front. Aging Neurosci. 6:303. doi: 10.3389/fnagi.2014.00303
Watanabe, H., Fitting, S., Hussain, M. Z., Kononenko, O., Iatsyshyna, A., Yoshitake, T., et al. (2015). Asymmetry of the endogenous opioid system in the human anterior cingulate: a putative molecular basis for lateralization of emotions and pain. Cereb. Cortex 25, 97–108. doi: 10.1093/cercor/bht204
Yüksel, D., Dietsche, B., Konrad, C., Dannlowski, U., Kircher, T., and Krug, A. (2018). Neural correlates of working memory in first episode and recurrent depression: an fMRI study. Prog. Neuropsychopharmacol. Biol. Psychiatry 84, 39–49. doi: 10.1016/j.pnpbp.2018.02.003
Keywords: laterality, N-back, aging, fMRI, superior parietal
Citation: Esteves M, Magalhães R, Marques P, Castanho TC, Portugal-Nunes C, Soares JM, Almeida A, Santos NC, Sousa N and Leite-Almeida H (2018) Functional Hemispheric (A)symmetries in the Aged Brain—Relevance for Working Memory. Front. Aging Neurosci. 10:58. doi: 10.3389/fnagi.2018.00058
Received: 09 January 2018; Accepted: 20 February 2018;
Published: 12 March 2018.
Edited by:
Jesus Avila, Universidad Autonoma de Madrid, SpainReviewed by:
Miguel Medina, Centro de Investigación Biomédica en Red sobre Enfermedades Neurodegenerativas, SpainIsidre Ferrer, Universitat de Barcelona, Spain
Copyright © 2018 Esteves, Magalhães, Marques, Castanho, Portugal-Nunes, Soares, Almeida, Santos, Sousa and Leite-Almeida. This is an open-access article distributed under the terms of the Creative Commons Attribution License (CC BY). The use, distribution or reproduction in other forums is permitted, provided the original author(s) and the copyright owner are credited and that the original publication in this journal is cited, in accordance with accepted academic practice. No use, distribution or reproduction is permitted which does not comply with these terms.
*Correspondence: Hugo Leite-Almeida, aHVnb2FsbWVpZGFAbWVkLnVtaW5oby5wdA==