- 1Department of Biotechnology, Faculty of Biological Sciences, Quaid-i-Azam University, Islamabad, Pakistan
- 2National Institute of Lasers and Optronics, Pakistan Atomic Energy Commission, Islamabad, Pakistan
- 3CAS Key Laboratory for Biomedical Effects of Nanomaterials and Nanosafety, CAS Center for Excellence in Nanoscience, National Center for Nanoscience and Technology, Beijing, China
- 4Department of Pharmacy, University of Peshawar, Peshawar, Pakistan
- 5Department of Life Sciences, King Fahd University of Petroleum and Minerals, Dhahran, Saudi Arabia
- 6Department of Eastern Medicine and Surgery, Qarshi University, Lahore, Pakistan
- 7Department of Pharmacy, University of Malakand, Chakdara, Pakistan
- 8Department of Life Sciences and Chemistry, Faculty of Health, Jacobs University Bremen, Bremen, Germany
- 9Pakistan Academy of Sciences, Islamabad, Pakistan
Alzheimer’s disease (AD) is characterized by cognitive inability manifested due to the accumulation of β-amyloid, formation of hyper phosphorylated neurofibrillary tangles, and a malfunctioned cholinergic system. The degeneration integrity of the neuronal network can appear long after the onset of the disease. Nanotechnology-based interventions have opened an exciting area via theranostics of AD in terms of tailored nanomedicine, which are able to target and deliver drugs across the blood–brain barrier (BBB). The exciting interface existing between medicinal plants and nanotechnology is an emerging marvel in medicine, which has delivered promising results in the treatment of AD. In order to assess the potential applications of the medicinal plants, their derived components, and various nanomedicinal approaches, a review of literature was deemed as necessary. In the present review, numerous phytochemicals and various feats in nanomedicine for the treatment of AD have been discussed mechanistically for the first time. Furthermore, recent trends in nanotechnology such as green synthesis of metal nanoparticles with reference to the treatment of AD have been elaborated. Foreseeing the recent progress, we hope that the interface of medicinal plants and nanotechnology will lead to highly effective theranostic strategies for the treatment of AD in the near future.
Introduction
Alzheimer’s disease (AD) is a chronic neurodegenerative disorder characterized by memory loss, impaired cognitive function, and behavioral instability, predominantly among the elderly population (Minati et al., 2009). In the brain of AD patients, the excessive production of proteins [β-amyloid (Aβ) and hyperphosphorylated tau] leads to the loss of synaptic connections and neuronal impairment in the hippocampus and cerebral cortex. The aforementioned events thus, lead to the degenerative diseases like AD and dementia (Selkoe, 2001). The complex pathophysiological aspects of AD brain have been extensively studied; however, an effective medicine is still unavailable for the complete treatment of AD (Ayaz et al., 2016). In clinical trials, several claimed potential anti-Alzheimer drugs failed to prove their effectiveness (Cummings et al., 2016). In order to combat the situation, alternative and cutting edge approaches like nanomedicine have been proposed as a potential treatment modality. In this review paper, emerging phyto-therapeutic and nanomedicinal approaches for treating AD are discussed. In addition, recent trends in emerging theranostics for AD using NPs delivery across the blood–brain barrier (BBB) and their mechanism of action are elaborated.
Prevalence and Etiology of AD
The AD is one of the major causes of dementia in an estimated 60–80% of cases. According to the Alzheimer’s Association Report 2017, about 5.5 million individuals have AD (Alzheimer’s and Association, 2017). Early clinical symptoms of AD patients include impairment in memory, e.g., current conversations, names or events, while later symptoms comprises poor communication, misperception, confusion, misjudgment, changed attitude, and finally, difficulty in speaking, swallowing, and walking (Khachaturian, 1985). In the United States, AD was reported in 4.7 million individuals aged 65 years or older during 2010, which is estimated to reach 13.8 million cases by 2050. Similarly, a considerable number of deaths were observed among older AD patients. In 2010, ∼600,000 deaths occurred among people with AD at the age of 65 years or older, which is expected to reach 1.6 million by 2050 (Hebert et al., 2013).
The Aβ and tau proteins are the key factors to be considered in AD treatment. Currently, researchers are trying to develop drugs that can minimize amyloid synthesis/accumulation and tau aggregation/phosphorylation. The AD is associated with multiple factors of unknown etiology, and various cases can be observed with mutations in different genes, such as in presenilins (PS1 and PS2) and Aβ precursor protein (APP) (Bird, 2008). The apolipoprotein E (APOE) genes are also considered to be a risk factor for AD. Similarly, different proteins like APOE, APP, β amyloid cleaving enzyme (BACE), presenilin 1 and 2 (PS1 and PS2), and secretases (BACE1) are the key factors in the pathogenesis of AD (Kim et al., 2009). Therefore, researchers have focused on the synthesis of novel inhibitors for PS1, BACE, and BACE1 for the possible treatment of AD. Investigators are also trying to determine the efficacy of cholinesterase (ChE) inhibitors in the brain (Ayaz et al., 2017b; Ovais et al., 2018b). The appliance of new-generation acetyl and butyrylcholinesterase (AChE/BChE) inhibitors has been tested in the clinical trials for AD (Giacobini, 2004). Additional approaches such as hormone therapy and use of antioxidants, cholesterol-lowering mediators, anti-inflammatory substances, and vaccinations have also been studied for the treatment of AD (Yiannopoulou and Papageorgiou, 2013).
Role of the BBB in AD
The BBB is a delicate membrane synthesized by the endothelial cells, which makes up the inside layer of cerebral microvessels. The BBB controls the entrance of plasma components, red blood cells, and leukocytes into the central nervous system (CNS), while exporting the neurotoxic molecules from the brain to the blood. Two additional sites were found in the CNS, which make up a barrier between the blood and cerebrospinal fluid (CSF), e.g., the arachnoid epithelium constituting the central layer of the meninges and choroid plexus epithelium. These distinctive BBB membranes encompass a group of physical, transport, and metabolic barriers that separate the neural environment from the blood (Abbott et al., 2010). The integrity of the BBB is controlled by the brain microvessel endothelial cells (BMECs) having distinctive luminal (apical) and abluminal (basolateral) membrane compartments (Zlokovic, 2011). They are an essential part of the neurovascular unit (NVU) in conjunction with pericytes, vascular smooth muscle cells from the vessel wall, neurons, and glial cells. Besides maintaining the chemical composition of the brain interstitial fluid, the NVU regulates BBB permeability and cerebral blood flow, which is necessary to maintain useful neuronal circuits (Daneman and Prat, 2015). Impairment in the BBB and NVU has severe pathological characteristics. These include outflow of circulating materials from the plasma into the CNS, changes in transporters that lead to poor nutrient supply and addition of neurotoxins in the CNS, and changes in the expression or secretion of proteins by NVU cells responsible for inflammation, oxidative stress, and neuronal damage. Recently, it has also been reported that the abnormal NVU cells result in different CNS pathologies such as dementia, neurodegeneration, and cognitive failure in AD (Erickson and Banks, 2013). Previously, the CNS was thought of as an immune-privileged organ, missing a lymphatic system and protected from the peripheral blood cells by the BBB. Conversely, the phenomenon is clear now that the BBB has the ability to respond to soluble factors and plasma proteins and interconnect with the peripheral immune system cells, thus forming neuroimmune system interactions, validating the concept that neuroinflammation leads to AD pathology (Heneka et al., 2015). Hence, the brain cannot be considered as an immune-privileged organ, and abnormal NVUs have to be withheld for peripheral immune cells and circulating soluble molecules that mediate immune responses (Zenaro et al., 2015).
Alternative Therapy Using Medicinal Plants
Due to severe side effects of current therapies, alternative therapies such as use of medicinal plants for the treatment of AD and other diseases are a vital focus of researchers (Ali M. et al., 2017; Ayaz et al., 2017a; Sadiq et al., 2018). The medicinal plants have been reported to enhance the memory and learning process that normally decline with AD (Ahmad et al., 2016; Ayaz et al., 2017b; Zohra et al., 2018). From the current literature, we have summarized potential anti-Alzheimer compounds isolated from various medicinal plants (Table 1). Currently, several studies report on the phytochemicals that have been clinically proven with significant anti-AD potentials. Some of these bioactive compounds and their mechanisms of action against AD have been discussed in the following sections.
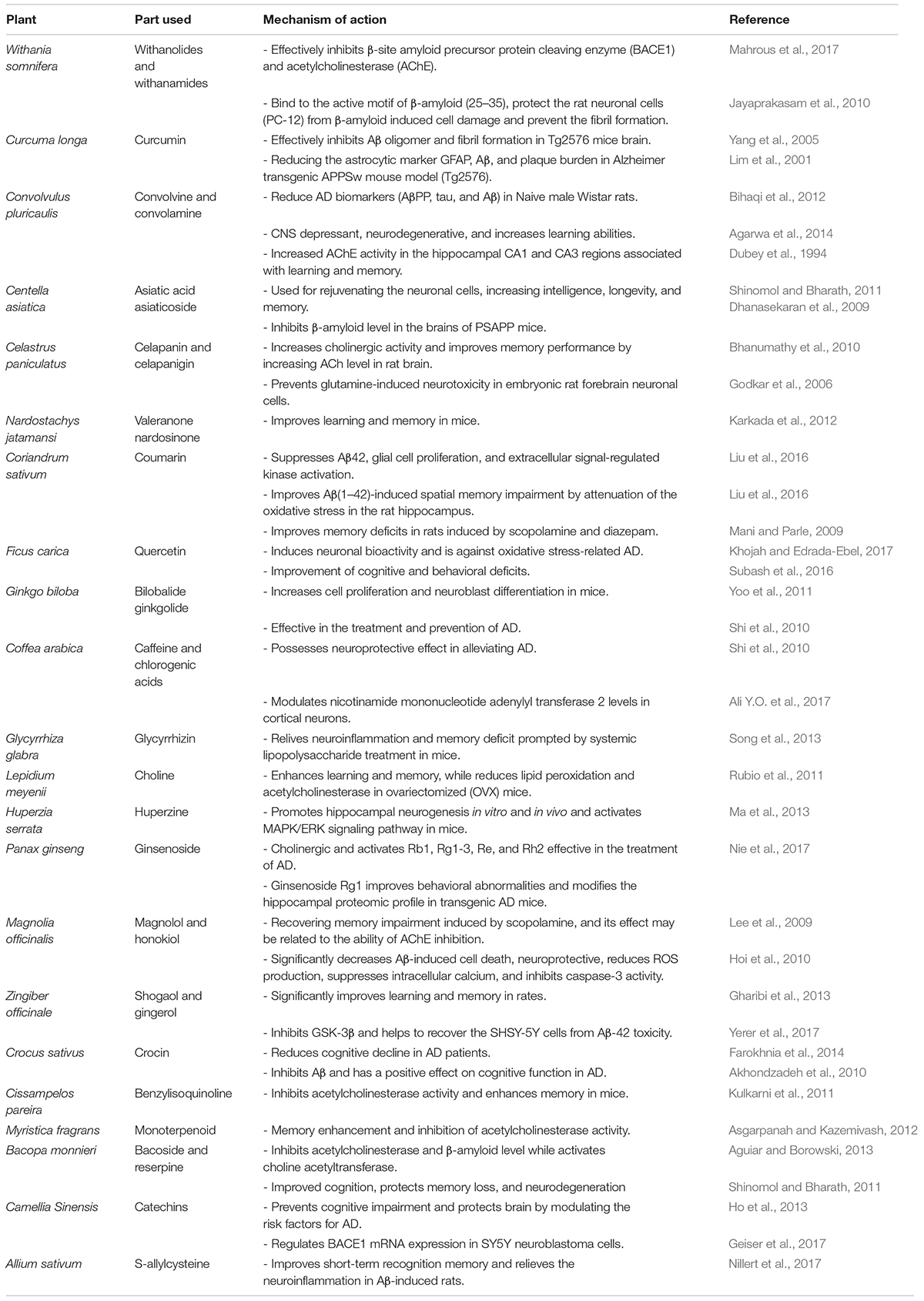
TABLE 1. The major medicinal plants with potential bioactive compounds and their mechanisms of action against AD.
Withanolides and Withanamides
Withania somnifera (WS), an essential medicinal plant, has been in use for the treatment of AD for the past 3000 years. The crude extract of this plant possesses withanamides A and C that can effectively bind to Aβ and inhibit fibril synthesis in the rat neuronal cells (PC-12) (Jayaprakasam et al., 2010; Ali et al., 2018). In a current study, withanolide S, isolated from different parts of WS, showed dual inhibitory activities against BACE1 and AChE (Mahrous et al., 2017; Puerta et al., 2017). In another study, computational tools were used to determine the bioactive compounds of WS against AD. The ligands (anaferine, anahygrine, cuscohygrine, and isaopelletierine) of WS were found to be neurologically active as agonists at the neuronal nicotinic acetylcholine receptors (nAChR) (Remya et al., 2016). Vareed et al. (2014) investigated the BBB permeability of withanamides that are present in W.somnifera fruit extract using animal models via high-performance liquid chromatography and quadrupole time of flight mass spectrometer system. Results of the study concluded that four withanamides significantly crossed BBB and their major peaks were identical both in brain tissue homogenates and plant crude extracts (Vareed et al., 2014).
Curcumin
Curcumin is the active ingredient isolated from Curcuma longa, carrying significant effects against AD. It has an antiamyloidogenic potential, which inhibits Aβ aggregation and downregulates BACE1 expression. It also possesses antitau function by inhibiting tau hyperphosphorylation and plays a key role in tau tangle clearance. In a randomized, placebo-controlled double-blinded study, Larry Baum et al. reported that curcumin exhibits neuroprotective effects in AD patients via several mechanisms, including the inhibition of Aβ aggregation, the inflammatory pathways, and free radical-induced neurodegeneration (Baum et al., 2008).
In another study, curcumin significantly reduced pro-inflammatory cytokines levels like interleukin (IL) 1β and oxidized proteins. Moreover, curcumin therapy considerably declined soluble and insoluble Aβ load in brain tissues and reduced astrocytic marker glial fibrillary acidic protein (GFAP) (Lim et al., 2001). The free radicals-scavenging, anti-amyloid, and anti-inflammatory effects of curcumin were also analyzed in transgenic and lipopolysaccharide (LPS) induced AD model. In both animal models, curcumin therapy reduced Aβ load and the chronic inflammation via inhibition of inflammatory cytokines (Begum et al., 2008). Furthermore, it can be used as a neuroprotective agent that promotes neuroplasticity and inhibits AChE activity (Serafini et al., 2017). Several other studies signify the potential use of curcumin in the management of neurodegenerative disorders like AD and its possible mechanism of action (Baum and Ng, 2004; Aggarwal and Sung, 2009).
In a study, Tsai et al. (2011) investigated the BBB penetration potentials of curcumin-loaded poly (lactic-co-glycolic acid) (PLGA) nanoparticles (NPs). Curcumin-brain concentrations and retention times were considerably increased in the cerebral cortex and hippocampus tissues of the animal brain (Tsai et al., 2011). Likewise, curcumin therapy is also reported to inhibit cerebral ischemia and reperfusion injury via prevention of BBB damage (Jiang et al., 2007). Yet another study on nano-curcumin preparations revealed significant improvements in cognitive function and reduction in amyloid load in transgenic animal model of AD (Cheng et al., 2013).
Asiatic Acid and Asiaticoside
Centella asiatica is an important medicinal plant containing asiatic acid (AA) and asiaticoside. These neuroprotective phytochemicals have the potential to cross the BBB. Asiatic acid increases the viability of differentiated human neuroblastoma SH-SY5Y cells (Ternchoocheep et al., 2017). The neuroprotective effects of asiaticoside have been reported in primary cultured mouse cortical neurons exposed to glutamate-induced excitotoxicity invoked by N-methyl-D-aspartate (NMDA). It downregulates NMDA receptor subtype 2B (NMDAR2B or NR2B), which shows that asiaticoside can protect neurons from excitotoxicity induced by NMDA exposure via obstructing cell apoptosis and calcium overload (Qi et al., 2014). Asiatic acid isolated from C. asiatica is reported to mediate its neuroprotective effects via reduction of the BBB permeability and mitochondrial injury (Krishnamurthy et al., 2009).
Celapanin and Celapanigin
Phytochemical studies of Celastrus paniculatus (CP) show the presence of alkaloids like celapanin and celapanigin, which are extensively used as neuroprotective agents, memory enhancers, and in different CNS disorders. A recent study has proposed the protective effects of CP against 3-nitropropionic acid (3-NP)-induced neurotoxicity. It also prevents glutamine-induced neurotoxicity in embryonic rat forebrain neuronal cells. Glutamate receptor and/or NMDA receptor antagonists have shown neuroprotective effects against such damage (Godkar et al., 2006; Malik et al., 2017).
Valeranone and Nardosinone
Nardostachys jatamansi, a flowering herb that contains valeranone and nardosinone enhances the levels of key neurotransmitters such as gamma-aminobutyric acid (GABA), norepinephrine, dopamine, serotonin, and 5-hydroxyindoleacetic acid in the brain. Valeranone and nardosinone also inhibit inflammatory cytokines IL-1β, IL-6, and tumor necrosis factor alpha (TNF-α). Furthermore, they deactivate p38 mitogen-activated protein kinases (MAPKs) (Akram and Nawaz, 2017) https://www.ncbi.nlm.nih.gov/pubmed/26371857. It is also reported that nardosinone acts as an enhancer of nerve growth factor (NGF) (Zhang C. et al., 2014), dibutyryl cyclic AMP, and staurosporine-induced neurite outgrowth from PC12D cells by amplifying both the MAP kinase-dependent and -independent signaling pathways of dibutyryl-cAMP (dbcAMP) and staurosporine (Li et al., 2003). Nordosinone has been recently reported to significantly cross BBB and exhibit strong antidepressant activity (Jalali et al., 2018).
Coumarin
Coriandrum sativum, known as coriander, is an important medicinal plant, which contains coumarin as an active ingredient that has memory-enhancing and neuroprotective effects (Mani and Parle, 2009). C. sativum extract intake by AD model flies increases the reactive oxygen species (ROS) levels and number of glial cells. It also inhibits the epidermal growth factor receptor and induced phosphorylation of extracellular signal-regulated kinase (ERK) (Laribi et al., 2015). Currently, a series of 7-substituted coumarin derivatives have been designed and synthesized to display ChE and monoamine oxidase (MAO-B) inhibitory activities. The molecular modeling determined that the tested compounds are able to inhibit AChE-induced Aβ aggregation and, therefore, can be considered as promising multifunctional lead compounds for the treatment of AD patients (Joubert et al., 2017).
Among the natural coumarins, osthole is reported to exhibit neuroprotective effects via inhibition of oxidative stress-induced cerebral ischemia and inhibition of BBB disruption (Mao et al., 2011; Chen Z. et al., 2015). Several coumarin derivatives are reported to selectively inhibit MAO-B enzyme implicated in AD and prevent Aβ1-42 aggregation. Some of the test compounds cross BBB, chelate metal ions, and exhibit low toxicity in cell lines, which signify their use as potential anti-AD agents (Huang et al., 2015; Jameel et al., 2016).
Quercetin
Quercetin, an important polyphenol found in Ficus carica, has the ability to improve learning and memory when used in the treatment of AD (Biswas et al., 2015). It is considered as a multifunctional neuroprotective agent (Ossola et al., 2009). Currently, the effect of oral administration of nanoencapsulated quercetin was determined in a mouse model of AD. It was observed that the oral administration of nanoencapsulated quercetin in zein NPQ reduced the cognition- and memory-impairment characteristics of senescence-accelerated prone mouse (SAMP8). These observations appeared to be related with a decreased expression of the hippocampal astrocyte marker GFAP (Puerta et al., 2017).
Quercetin is reported to significantly cross BBB (Faria et al., 2014), accumulate in the target brain tissues of animal models, and reduce the oxidative stress-mediated neurodegeneration (Ishisaka et al., 2011). Moreover, quercetin is also reported to exhibit neuroprotective effects via inhibition of NO excessive production and overexpression of iNOS and downregulation of pro-inflammatory gene expressions like TNF-α, COX-2, and IL-1βin zebrafish (Zhang et al., 2011). Nanoencapsulated quercetin is reported to inhibit ischemic reperfusion-mediated neuronal damage in animal models (Ghosh et al., 2013).
Bilobalide and Ginkgolide
Ginkgo biloba (GB) contains very important constituents such as bilobalide and ginkgolide. They have been extensively studied for their CNS effects (Maclennan et al., 2002) and can be used for the possible treatment of AD patients (Ahlemeyer and Krieglstein, 2003; Shi et al., 2010). The dietary treatment of mice with GB extract upregulates the expression of genes encoding neuronal tyrosine/threonine phosphatase 1 and microtubule-associated tau in the cerebral cortex. The GB extract can also upregulate two mitochondrial DNA-encoded genes, subunit III of cytochrome-c oxidase and NADH dehydrogenase, subunit 1 (ND1), indicating a fundamental mechanism that may underlie bilobalide-induced neuroprotection and its role in cognitive impairment (DeFeudis, 2002). Recently, it has also been reported that the ectopic expression of APE1 enables neuronal cells to overcome the oxidative damage caused by Aβ25-35. Moreover, ginkgolide has modified the mitochondrial OXPHOS against Aβ25-35-induced oxidative stress and regulated the reactive oxygen and/or nitrogen species (ROS/RNS) level in the existence of ectopic APE1. This study portrays an alternative approach in the therapeutic potential for AD by harnessing the synergistic neuroprotective roles of apurinic/apyrimidinic endonuclease 1 (APE1) and ginkgolide (Kaur et al., 2015).
Ginkgolides A, B, C, J, and bilobalide isolated from G. biloba significantly cross BBB using in vitro and in vivo permeability models and inhibit oxidative stress-induced cognitive dysfunctions (Sharma et al., 2000; Madgula et al., 2010). In another brain permeability study, ginkgolide B passed BBB and inhibited reperfusion-induced ischemic brain damage (Fang et al., 2010; Lang et al., 2010).
Caffeine and Chlorogenic Acids
Coffea arabica is well known for the coffee, which is a common drink linked to a number of health benefits, including its key role to intervene AD. Different coffee varieties contain caffeine and chlorogenic acids in different concentrations, which possess neuroprotective effects observed in various in vitro and in vivo studies (Rebai et al., 2017). Recently, nicotinamide mononucleotide adenylyl transferase 2 (NMNAT2) was reported as a key neuronal factor that delivers strong neuroprotection in numerous preclinical models of neurological disorders. Systemically administered caffeine was identified as a positive modulator that restores NMNAT2 expression in tauopathy mouse model (rTg4510) to normal levels (Ali Y.O. et al., 2017). Caffeine and 5-caffeoylquinic acid (chlorogenic acid)-enriched coffee extensively crosses BBB and exhibits numerous CNS effects including neurotropic, anti-AD, and neuroprotective properties (Ito et al., 2008; Chu et al., 2009; Kwon et al., 2010; Cropley et al., 2012; Esquivel and Jiménez, 2012; Oboh et al., 2013).
Glycyrrhizin
Glycyrrhizin (GRZ) is a triterpenoid saponin, majorly found in Glycyrrhiza glabra. The GRZ exhibited spatial memory-enhancing and ameliorating effects on cognitive impairment induced by Aβ injection into the hippocampus in vitro and in vivo (Grzybowska et al., 1998; Chen et al., 2017). Currently, it is being reported that GRZ effectively reduced neuroinflammation and ameliorated the memory deficits induced by systemic LPS treatment. The effects of GRZ were found to be mediated through inhibition of pro-inflammatory cytokines TNF-α, IL-1β, and microglial activation in the brain tissues. This study supports that GRZ may be a putative therapeutic drug for neurodegenerative diseases that are associated with cognitive deficits such as in AD (Song et al., 2013).
The BBB permeability of 18β-glycyrrhetinic acid, an active metabolite of GRZ, was reported by Tabuchi et al. (2012) using in vitro and in vivo models. Study results revealed that the test compound, after oral administration, significantly crossed the BBB in both the in vitro BBB permeability model as well as animal models (Tabuchi et al., 2012). Oral pretreatment with GRZ is reported to thwart surgery-mediated cognitive dysfunctions via inhibition of neuroinflammation and AD symptoms (Chen et al., 2017).
Choline
Choline is required for the production of acetylcholine, an important neurotransmitter for memory, brain, and nervous system functions. It also plays important roles in modulating gene expression, cell membrane signaling, lipid transport and metabolism, and early brain development. Nutritional intake of choline in AD patients can influence cognitive functions via an effect on phosphatidylcholine (PC)-containing eicosapentaenoic and docosahexaenoic acids and polyunsaturated species of PC, levels of which are reduced in brain that is linked with higher memory performance (Blusztajn et al., 2017). In a current study, perinatal choline supplementation was reported to reduce amyloidosis and increase choline acetyltransferase expression in the hippocampus of APPswePS1dE9 (APP/PS1) AD mice. The data propose that dietary intake of choline during fetal development and early postnatal life can establish a preemptive approach for AD (Mellott et al., 2017).
Huperzine
Huperzine A (HupA) is a lycopodium alkaloid found in Huperzia serrata. The HupA was reported as an effective cholinergic agent. It is also effective in acute seizures as tested in a rat model. Therefore, HupA is a safe drug for AD patients who show both mnemonic and epileptic symptoms (Rafii et al., 2011). Moreover, it has also been reported that HupA can penetrate the BBB due to its interaction with efflux transmembrane transporters (ABCB1 and ABCG2). In transgenic animal models, the brain-to-plasma concentration ratio of Huperzine A was significantly increased as compared with the wild type mice due to ABCB1 that played a predominant role in the efflux of Huperzine A across BBB (Li et al., 2017).
Ginsenoside
Ginsenosides Rg1 (GRg1) and Rb1 (GRb1) are found to be the active ingredients of ginseng, which exhibits potential efficacy in prevention and treatment of CNS disorders and neurodegenerative diseases like AD. Currently, Rb1 and baicalin have been reported to promote proliferation and differentiation of endogenous neural stem cells (NSCs) in AD rat models. It also shows a decreased nuclear pyknosis and pyramidal cell defects in hippocampus of rats by increasing the expression of nestin, GFAP, and neuron specific-enolase (NSE) proteins and thereby improves cognitive function in AD rats (Zhao et al., 2018). Recently, the neuroprotective effects and its transport across BBB was reported by Rui et al. (2009) using in vitro and in vivo rat models. Ginsenoside Rg1 exhibited significant neuroprotective potentials, whereas, its passage across BBB was extremely poor. Ginsenoside Rg1 ameliorates cerebral ischemia via downregulation of protease-activated receptor 1 (PAR-1) expression (Xie et al., 2015) and offers protection against BBB disruption in brain injury model (Zhou et al., 2014; Chen W. et al., 2015).
Magnolol and Honokiol
Magnolol and honokiol are the bioactive compounds found in Magnolia officinalis. They are neuroprotective and decrease ROS production, intracellular calcium and caspase-3 activities. Therefore, these phytochemicals or their analogs may be explored as therapeutic drugs for AD (Hoi et al., 2010). Honokiol was reported as to reduce AβO-induced hippocampal neuronal apoptosis, ROS production, and loss of mitochondrial membrane potential in a dose-dependent manner. Moreover, honokiol inhibited ABO-mediated NF-κB activation as well as inhibited the up regulation of amyloid precursor protein (APP) and beta secretase enzyme (BACE1). Therefore, honokiol may be a potential candidate in AD therapy (Wang et al., 2017). Honokiol is reported to cross BBB and effectively target CNS disorders like gliosarcoma (Wang et al., 2011) and cerebral ischemia via inhibition of NF-κB (Zhang et al., 2013) and offer protection against brain ischemic-reperfusion injury via disruption of PSD95–nNOS interactions (Hu et al., 2013).
Shogaol and Gingerol
Zingiber officinale contains two major therapeutic agents, shogaol and gingerol, that could recover the SHSY-5Y cells from Aβ1-42 oligomer and aggregate toxicity. Currently, 6-gingerol and 6-shogaol have been found as neuroprotective agents, which can inhibit the GSK-3β activity (Yerer et al., 2017). It was found that 6-shogaol influenced neuritogenic activity in PC-12 cells by activating the MAPK, extracellular signal-regulated kinase 1 and 2 (ERK1/2), phosphatidylinositol 3-kinase (PI3K), and protein kinase B (AKT) signaling pathways. Hence, 6-shogaol can act as a NGF mimicking agent, which can be used as a preventive therapeutic agent in neurodegenerative diseases (Seow et al., 2017).
Crocin
Crocin is a water-soluble carotenoid found in Crocus sativus and possesses the capability to improve learning and memory as well as protect brain cells. Recently, crocin has been reported for its considerable effects on Aβ and tau proteins. Crocin revealed multifunctional protective activities in the brain and could be a promising agent when applied as a supplement or drug for the treatment of AD (Finley and Gao, 2017). Crocin has been reported to improve locomotor activities in animal models (Karami et al., 2013), provide protection against oxidative stress and cerebral ischemia (Zheng et al., 2007), and maintain BBB integrity (Zhang et al., 2017).
Benzylisoquinoline
Cissampelos pareira contains benzylisoquinoline that exerts nootropic activity in AD. It is a memory-enhancing agent, inhibits AChE activity, and enhances memory in mice (Kulkarni et al., 2011). A novel series of benzylisoquinoline derivatives have also been evaluated. The results have shown that most of the compounds have significant inhibitory potentials against ChEs (James et al., 2016), human cholinesterases (h-ChEs), and self-induced Aβ aggregation (Xu et al., 2014).
Monoterpenoid
Monoterpenoids in Lavandula luisieri have been identified as potent inhibitors of β-secretase (BACE-1), an aspartic protease involved in the conversion of APP to Aβ in animal models. Currently, an active monoterpene, necrodane ketone, 2,3,4,4-tetramethyl-5-methylenecyclopent-2-enone was tested for its inhibitory mechanism against BACE-1. This monoterpene revealed a dose-dependent inhibition of BACE-1 in cellular and mouse models of AD (Videira et al., 2014). Recently, 80 types of aroma compounds including monoterpenes were screened for in vitro inhibitory activity at a concentration of 200 μM against recombinant human BACE1 (Marumoto et al., 2017).
Bacoside and Reserpine
Bacopa monnieri, known as a memory-enhancing herb, contains key therapeutic agents bacoside and reserpine. It promotes free radicals scavenger mechanisms and protects cells in the prefrontal cortex, hippocampus, and striatum against cytotoxicity and DNA damage implicated in AD. It protects the cholinergic neurons and reduces anticholinesterase activity comparable with donepezil, rivastigmine, and galantamine (Chaudhari et al., 2017). The mechanism of action of reserpine was determined in Caenorhabditis elegans where it alleviated the toxicity of Aβ. The afore-mentioned study was conducted to evaluate the ∼2.8 kb promoter region of FLP-11, driving the expression of green florescent protein, which can be a critical mediator of alleviation of Aβ toxicity through acetylcholine and reserpine (Saharia et al., 2016). The PLGA-loaded bacoside-A NPs are reported to effectively deliver the drug across BBB and mediate its neuropharmacological actions (Jose et al., 2014; Chaudhary and Bist, 2017).
Catechins
Catechins found in Camellia sinensis prevent cognitive impairment among the elderly. It provides protection to the brain and modulates the risk factors of AD (Preedy, 2012). Currently, the human-derived cell line (SH-SY5Y) was treated with native oligomers (epicatechin and theaflavin) that reduce Aβ-induced BACE1 expression. The data show that the antioxidant activity of catechins and theaflavins may be more essential in downregulating BACE1 mRNA expression than their capability to inhibit Aβ oligomerization (Geiser et al., 2017). Catechins can cross the BBB and mediate various neuroprotective effects (Faria et al., 2011; Wu et al., 2012).
S-allylcysteine
Garlic extract (GE) contains S-allylcysteine, which has been reported for multiple biological activities, including anti-inflammatory effect and its influence on Aβ1-42-induced cognitive dysfunction and neuroinflammation (Kodera et al., 2002; Pérez-Severiano et al., 2004). The GE was found to be useful by improving the short-term recognition memory and relieve the neuroinflammation in Aβ-induced adult male Wister rats (Nillert et al., 2017). Recently, a research group has reviewed different antioxidant mechanisms (scavenging of free radicals and prooxidant species, induction of antioxidant enzymes, activation of Nrf2 factor, inhibition of prooxidant enzymes, and chelating effects) involved in the protective actions of aged GE (AGE) and S-allyl-L-cysteine (SAC), thereby, emphasizing their potential use as therapeutic agents (Colín-González et al., 2012).
Treating Alzheimer’s: A Triple Challenge
Treating brain diseases, in general, and AD, in particular, is a special challenge. First of all, the intricate and specific system that the brain possesses in the form of BBB analyzes and monitors the transport of every molecule that crosses the brain. It consists of endothelial cells, astrocytes, pericytes, and microglial cells (Johansson, 1990). The highly tight junctions between the endothelial cells act as a border patrol system, which analyze the molecules on the basis of their lipophilicity, size, surface charge, and hydrogen-bonding potential. Priority is given to small lipophilic molecules with size less than 500 Da and the entry of these molecules is restricted, which do not fall under the specific criteria of structural and physicochemical properties (Abbott et al., 2010). In addition, even if the molecule of choice does get entry across the BBB, there is a high chance of it being thrown out due to the number of efflux transporters such as the ATP-binding cassette family (P-glycoprotein ABCB1), the multidrug resistance-related proteins (MRPs, ABCC1, 2, and 5) and the breast cancer resistance protein (BCRP, ABCG2) present in the endothelium of the brain _ENREF_3 (Begley, 2004; Ayaz et al., 2017d).
In addition to the transport-related issues, limited knowledge of the basic pathology of AD is another challenge in more effective drug therapy (Sperling et al., 2013). Many mechanisms leading to the pathology have been proposed, and the disease is attributed to be the result of a number of overlapping phenomena such as inflammation, iron dysregulation, oxidative damage, cholesterol metabolism (Galimberti and Scarpini, 2010), and “amyloid plaque” hypothesis. Despite this, there is no FDA-approved drug or carrier system with the disease-modifying potential for AD (Casey et al., 2010). Moreover, the third and major limitation in the successful therapy of AD is the absence of tools for early detection of the disease. Biochemical alterations at the cellular level start around 10–20 years before the onset of cognitive decline (Beason-Held et al., 2013). It has been proposed that the failure of various therapeutic approaches may be attributed to the fact that until the time of appearance of clinical symptoms, irreversible damage has already been done.
Currently Approved Drugs for Alzheimer’s and Their Shortcomings
Until now, the FDA has approved drugs only for the symptomatic treatment of AD. The five that have been approved target neurotransmitter imbalance at different stages of disease; they include AChE inhibitors; tacrine (1st generation), donepezil, rivastigmine, and galantamine (2nd generation) (Ayaz et al., 2015). One of the five approved drugs by the FDA for the symptomatic treatment of AD is an NMDA receptor blocker, memantine. The pharmacokinetic profile of drugs affecting acetylcholine and NMDA is given in Table 2.
Despite the beneficial effects of improvement in everyday life of patients, reduction in emotional impact on caregivers, and overall care cost, the large variations between the effectiveness and efficacy of these drugs make their cost-effectiveness controversial (Loveman et al., 2006). This large gap in the efficacy profile is majorly due to peripheral stimulation of cholinergic system that results in gastrointestinal tract (GIT)-related side effects. For instance, GIT-based adverse drug reactions (ADRs) were the major causes for trial discontinuation, with mean frequency of nausea being 11% for donepezil, 44% for rivastigmine, and 24% for galantamine across various trials of AChE inhibitors (AChEI) (Casey et al., 2010). Moreover, these drugs have shown poor long-term maintenance of the improvement of symptoms with studies showing that benefits of AChEI can be endured for up to 4 years with decline in the cognition below baseline levels after 1 year of therapy (Winblad and Jelic, 2004).
All these drawbacks can be attributed to the poor pharmacokinetic profile of these therapeutic moieties, including low bioavailability, volatility, oxidation, hydrolysis, limited transport across BBB, and tendency for drug–drug interactions (Mangialasche et al., 2010). As AD majorly affects the elderly, the comorbidities, polypharmacy, and non-compliance have a huge impact in decreasing the efficacy of the treatment regimen (Noetzli and Eap, 2013). Therefore, there is a major need for the development of multipurpose moieties with potent therapeutic potential against AD.
Mechanistic Basis of the Nanoparticle-Based Treatment
The unknown pathology and increase in the elderly population point toward a growing need for the development of therapeutic molecules specific to AD etiology. Many researchers around the world have developed NPs aiming at a different molecular mechanism of AD, in the hope of pinpointing the basic cause or limiting factor (Nazem and Mansoori, 2011; Table 3). There are four major and basic points in the pathophysiology of AD that can be targeted by use of NPs. (1) Neurotransmitter modulators; (2) anti-amyloid beta immunotherapy; (3) metal stress reducers, and (4) inflammatory inhibitors.
Neurotransmitter Modulators
It is known that presynaptic deficits of Ach contribute significantly toward cognitive dysfunctions and induction of memory impairment (Ullah et al., 2016). Interestingly, it is noted in animal models that degeneration of neurons in the basal forebrain and medial septum leads to substantial decline in Ach levels in the cerebral cortex and hippocampus, respectively. Moreover, the biochemical evaluation of brain from patients with AD also showed considerable deficits in the neocortical levels of AChE, an enzyme involved in the synthesis of Ach (Ayaz et al., 2014). The NPs capable of transporting the acetylcholine-modulating drugs across the BBB should restore the imbalance of the Ach in AD patients and should ameliorate the deterioration of memory and improve cognitive function. It was reported that rivastigmine-loaded lipid NPs (RL) have successfully normalized the cortical Ach level and subsequently improved the deterioration of spatial memory in animal models treated with AlCl3 (Ismail et al., 2013). In their study, rats that were coadministered with RL and AlCl3 showed normalization of BACE1 gene expression as compared with its 316% overexpression in rats treated with AlCl3 only. Furthermore, the histological evaluation showed that coadministration of RL prevented the formation of Aβ plaque and, hence, strengthens the argument that normalization of Ach is associated with an anti-Alzheimer effect.
Amyloid Beta-Targeted Immunotherapy
The Aβs were considered as therapeutic agents for active immunity after it was noted that transgenic AD mouse developed anti-Aβ antibodies when immunized with Aβ42. The antibody development resulted in improved cognition and decreased amyloid plaque number. The mechanism involves binding of anti-amyloid antibody to the amyloid plaque followed by the disaggregation of plaques and, hence, removal from the brain (Modi et al., 2010). Previously, external brain molecules like heparin and gelsolin were thought to trap or “sink-in” the Aβ and, hence, decrease its concentration in the brain (Fonseca-Santos et al., 2015). Both active and passive immunotherapy targeting Aβ forms the leading approach in the treatment of AD.
For active immunotherapy, Aβ subfragments were used in combination with NPs as their delivery across BBB is low. This approach was used by Songjiang and Lixiang (2009) to develop intramembranous Aβ fragment-loaded chitosan NPs for enhanced brain delivery. The ELISA data from the study showed that the formulation has significant potential to induce immunogenicity (Songjiang and Lixiang, 2009). Similarly, Agyare et al. (2008) developed chitosan polymeric NPs with tripolyphosphate (TPP) and loaded them with the polyamine-modified F(ab’) portion of anti-amyloid antibody (IgG4.1). The biodistribution studies in mice and transport study across bovine BMECs (BBMECs) showed that NPs were able to trancytose across BBMEC, and, hence, are proposed to target brain amyloid (Agyare et al., 2008).
In addition to active immunity with Aβ fragments, passive immunization is widely exploited for potential therapeutic regimen in AD, as indicated by several Aβ-specific antibodies being present in various phases of clinical trials (Table 4). However, very few studies have exploited antibodies in combination with NPs for the treatment of AD. Currently, the first synthetic study of Aβ1-42 monoclonal antibody-tagged polymeric NPs (125 nm) has been reported for the treatment of AD in transgenic (Tg) 2576 mice. The study showed that animals treated with mAb-tagged NPs exhibit significant reduction in the soluble Aβ peptide and its oligomer level in the brain and considerable increase of the Aβ levels in plasma. Moreover, the results demonstrated complete recovery of memory deficits in Tg AD mice, which further strengthens the fact that antibody-mediated treatment may provide the ultimate long-term and personalized therapeutic strategy for AD treatment (Carradori et al., 2018).
Metal Stress Modulators
It is evident that metabolism of zinc, cupric, and iron metal ions regulates Aβ toxicity, senile plaques (SPs), and neurofibrillary tangles (NFTs) formation. It has also been reported that SP and AB plaques are enriched with copper, iron, and zinc (James et al., 2016). In addition to promoting the conversion of Aβ to oligomers and fibrils and hyperphosphorylation of tau, these metal ions gets reduced when they undergo binding with Aβ, leading to generation of H2O2 – the main source of oxidative stress in Aβ toxicity (França et al., 2017).
Metal chelators loaded onto NPs can easily gain access to brain, bind to the metal particles, and leave the brain along with their conjugated metal ions and, hence, provide an easy means to reduce metal stress. Cui et al. (2005) reported the synthesis of Cu (I) chelator D-penicillamine conjugated ethanolamine-based NPs. The study showed successful uptake of NPs in the brain, and the NPs along with released penicillamine were able to resolubalize more than 40 % of Cu–Ab conjugates when treated with 0.1 N NaOH (Cui et al., 2005). Similarly, Liu et al. (2009) reported the syntheses of polystyrene-based – iron chelator conjugate (Nano-N2PY) and its ability to inhibit Aβ aggregation-mediated cytotoxicity in human cortical neuronal cells. These preliminary in vitro studies can provide a strong background for further in vivo evaluation of metal-conjugated NPs as AD therapeutics.
Anti-inflammatory Agents
It is established that oxidative stress related to Aβ toxicity results in mitochondrial damage, which leads to cell death and neuronal damage. Anti-inflammatory and antioxidant agents, such as CoQ10, have established a role in mitochondrial bioenergetics and reduction of oxidative stress secondary to Aβ toxicity (Garrido-Maraver et al., 2014). The CoQ10-loaded trimethylated chitosan (TMC)–PLGA-based NPs (TMC-coated D,L-lactide-co-glycolide) were synthesized, and it was investigated that the drug-loaded TMC–PLGA NP formulation was biocompatible, showed higher accumulation in the brain, and with great reduction in memory impairment, restoring it to almost normal level in comparison with CoQ10-loaded PLGA NPs without TMC. The formulation also helped decrease Aβ concentration and Aβ fibril formation; this can be attributed to the effect of Co Q10 on dissolution of SP (Garrido-Maraver et al., 2014).
The NPs as Anti-Alzheimer Moieties; Overcoming the Existing Barriers
Traditional drugs used for AD have low bioavailability, limited transport, and controversial long-term effects; therefore, a tremendous number of studies have reported on the use of nano-scale particles to overcome these limitations. There are different strategies that are employed to use NPs of various origins to improve pharmacokinetic profiles; (i) increase targeted delivery of drugs across BBB (Rocha, 2013), (ii) increase efficacy of already available approved agents, and (iii) provide a multifunctional platform that can help in theranostic applications.
The NPs by virtue of their small size, modifiable surface properties, and tunable morphology act as ideal candidates to overcome all the hurdles that a brain drug delivery (DDR) system needs to evade (Lockman et al., 2002). With the large loading capacity, NPs are good candidates for carrying increased amounts of drug across the BBB and increasing the brain-to-blood ratio of the already available drugs (Fazil et al., 2012).
Various strategies have been adopted to enhance the effect of these tiny cargos for better therapeutics.
(1) Lipophilic NPs like liposomes for enhanced permeation of hydrophilic drugs across BBB (Smith et al., 2010).
(2) Hydrophilic polymer-based NPs are employed to impart stealth character and act as a parenteral sustained drug-release system by prolonging the blood retention time (Wilson et al., 2010). Thus, by providing a higher concentration gradient between blood and brain, they facilitate the passive diffusion of drug across BBB (Masserini, 2013).
(3) Mucoadhesive polymeric NPs are used for enhanced nasal delivery of drugs through direct endocytosis-mediated uptake across BBB (Mistry et al., 2009).
(4) Targeted NPs with surface ligands for receptor-mediated transcytosis (Ulbrich et al., 2009).
Conventional NPs
Nanoparticles that consist of polymers, metal, or lipids without any delivery enhancing or targeting moiety to guide their transport are sometimes referred to as conventional NPs. These were the first ones to be used in AD nano-therapeutics.
Polymer-Based Delivery System
Polymeric NPs have been used extensively for AD drug delivery to enhance the efficacy of already available drugs.
Chitosan NPs
Chitosan is a biocompatible, biodegradable, and non-toxic (De Campos et al., 2001) linear polyamine polymer with reactive amine and hydroxyl surface groups (Dutta et al., 2004). Owing to the presence of reactive sites, this harmless polymer has chameleon-like characteristics. By virtue of these surface groups, it can have various modifiable properties like (i) adjustable drug-loading capacity_ENREF_3, (ii) hydrophilic–lipophilic balance (HLB) value, (iii) pH sensitivity, (iv) controlled drug release, (v) targeting ability, and (vi) surface adsorption (Shu and Zhu, 2000; Liang et al., 2008; Wang et al., 2008).
The chitosan backbone can be chemically altered to adjust the HLB value of the resultant polymer (Agnihotri et al., 2004). It is suggested that the hydrophilic polymer-based DDR system that prolong the blood retention time may act as a parenteral sustained drug-release system. This characteristic of chitosan has been utilized in increasing the brain delivery of anti-Alzheimer drugs that can freely cross the BBB (Wilson et al., 2010). Chitosan has also been used to increase the brain-to-blood ratio of drugs with poor ability to cross BBB. This was achieved by using the muco-adhesive properties of the polymer by Fazil et al. (2012) in developing rivastigmine-loaded chitosan NP for intranasal delivery of drug to brain.
PLGA NPs
Poly (lactic-co-glycolic acid) forms one of the most attractive nanomaterials to synthesize NPs for Alzheimer’s treatment. Its properties of biocompatibility, biodegradability, modifiable surface properties, stealth character, and ability to develop modified and sustained drug releasing nano-depots make it a perfect nanodevice (Danhier et al., 2012).
Md et al. (2014) prepared nano-particulate DDR system for donepezil using PLGA. The formulation showed sustained release and efficient brain targeting in SD rats (Md et al., 2014). Similarly, in another study, Wang et al. (2010) showed that coenzyme Q(10)-loaded TMC surface-modified NPs (TMC/PLGA-NP) greatly improved the memory impairment in mice. Senile plaques and biochemical parameters also showed brain targeting potential of the TMC/PLGA-NPs (Wang et al., 2010).
Cyanoacrylate NPs
Cyanoacrylate polymer provides a biocompatible and bioerosive medium for the synthesis of targeted NPs for treatment of AD. Its modifiable surface properties provide a very good medium for development of the sustained release drug delivery vehicle with long blood circulating times (Yordanov, 2012). Wilson et al. (2008) used poly (n-butyl cyanoacrylate) NPs for enhanced delivery of tacrine and rivastigmine. The group developed rivastigmine- and tacrine-loaded polysorbate 80-coated poly (n-butyl cyanoacrylate) NPs in two different studies. It was noted that there was a 3.82- and 4-fold increase in the concentration of rivastigmine and tacrine in brain, respectively, with the nano-formulation as compared with the free drug (Wilson et al., 2008).
Liposomal NPs for AD Drug Delivery
Liposomes are colloidal particles prepared by exposing phospholipids to the aqueous phase (Anwar et al., 2017). They facilitate loading of hydrophilic drugs into the aqueous interior of liposomes, thus preventing them from acid degradation, first pass effect, and delayed renal clearance (Smith et al., 2010).
Mutlu et al. (2011) have developed multilamillar rivastigmine-loaded liposomal NPs associated with permeability enhancer-like sodium-taurocholate or dimethyl-beta-cyclodextrin. Rivastigmine-sodium-taurocholate liposomes showed the highest apparent permeability coefficient in Madin-Darby Canine Kidney cells monolayer and the highest AChE inhibition in Balb-C type mice when administered through oral/intraperitoneal route (Mutlu et al., 2011). Similarly, Smith et al. (2010) have reported increased oral bioavailability by twofold and improved neuronal (SweAPP N2a cells) α-secretase inducing ability by 91% in vitro of epigallocatechin-3-gallate (EGCG) with use of nano-lipidic particles.
Stealth NPs
As BBB favors delivery of lipophilic, neutral, and small-sized particles, most of the early NPs employed for brain delivery of therapeutic agents are hydrophobic in nature (Sahni et al., 2011). Conventional NPs overcome a number of hindrance factors associated with conventional oral drug delivery such as acid degradation, first pass effect, and low brain-to-blood ratio (Di Stefano et al., 2011). The phenomenon of surface opsonization and subsequent removal from the bloodstream through reticuloendothelial system and Kupffer cells in liver and spleen leads to substantial decrease in the systemic and local therapeutic drug concentration. In the blood, hydrophobic and charged NPs undergo rapid surface opsonization within seconds to minutes as compared to hydrophilic or neutral NPs. This surface property is used to prepare the so-called “stealth” NPs with long blood-circulating times by coating them with specific polymer. Typical characteristics of polymer used to induce stealth character to NPs include flexibility and hydrophilicity. Several polymers that are used for such purposes in the brain DDR system include natural; chitosan, semisynthetic; polyethylene glycol (PEG), poly vinyl alcohol (PVA), polyvinylpyrrolidone (PVP), PEG-based copolymer; like poloxamer, poloxamines, and polysorbates (Salmaso and Caliceti, 2013). Tanifum et al. (2012) synthesized stealth liposomal NPs incorporated with Aβ-targeting fluorescent lipid conjugate, 1,2-distearoyl-sn-glycero-3-phosphoethanolamine-N-[methoxy-XO4-(polyethylene glycol-3400)] sodium salt (DSPE-PEG3400-XO4), where methoxy-XO4 serves as targeting and fluorescent moiety. When administered intravenously, nano-conjugate systems showed long circulating time with high efficiency for Aβ plaques in brain sections of APP/PSEN1 transgenic mice (Tanifum et al., 2012). Figure 1 shows the injected-targeted liposomes crossing the BBB of APP/PSEN1 mice and labeled parenchymal Ab deposits.
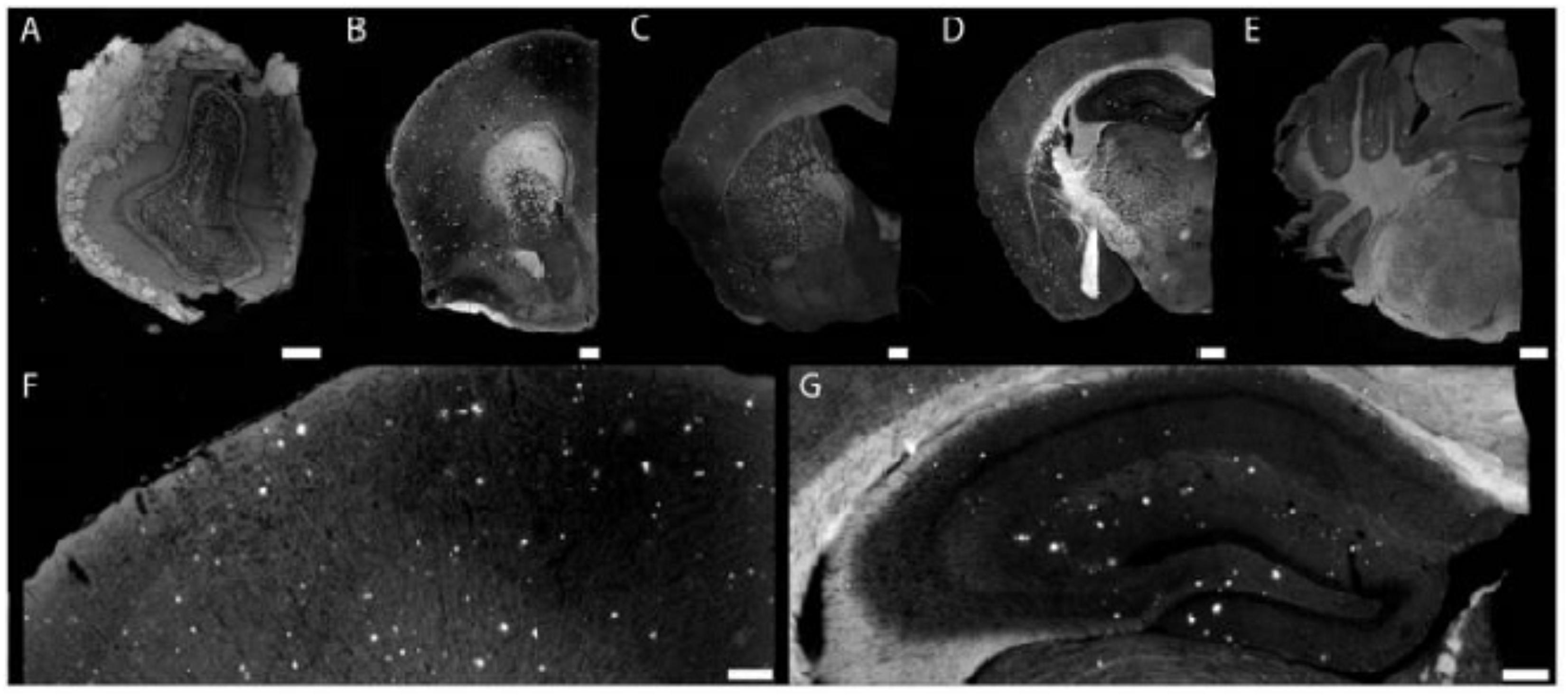
FIGURE 1. Injected targeted liposomes cross the blood–brain barrier of APP/PSEN1 mice and labeled parenchymal Ab deposits. Composite images (A–E) of olfactory bulb (A) showing plaque pathology within the granule cell layer. Nanoparticles bound to plaques at the level of the septo-striatum (B). Cortical pathology at the septo-diencephalic (C). Hippocampal and cortical pathology (D) within the caudal diencephalon. Binding to cerebellar plaques (found within APP mice) within the rostral mesencephalon (E). An example of cortical plaque pathology (F) visualized at 10× magnification. Hippocampal plaque pathology (G) is similar to previously reported studies. Scale bar = 100 mm (adopted with permission from Tanifum et al., 2012).
Nasal Route for Enhanced Delivery Across BBB
Many studies have used the nasal route for enhanced delivery of drugs across BBB because increased permeability of the nasal epithelia to drugs due to tight junction opening between apical cells provides a better route for increased drug delivery (Pardeshi and Belgamwar, 2013). It was noted that the brain delivery of drug through the nasal route is affected by the size, surface charge, and hydrophilic–lipophilic balance value of the NPs. The NPs with a size of less than 20 nm are proposed to follow the paracellular transport as compared with NPs of size 100–200 nm, which have been proposed to follow the transcellular route of drug transport. Similarly, particles with a dominant hydrophilic character follow the aqueous paracellular route of transport. Moreover, increase in cationic charge over the chitosan NPs showed increase in muco-adhesion to the nasal mucous and, hence, increased delivery across BBB (Mistry et al., 2009).
This attribute was used by Zhang C. et al. (2014) where they reported the synthesis of Solanum tuberosum lectin-modified polyethylene glycol-polylactide-polyglycolide NPs for enhanced intranasal delivery of basic fibroblast growth factor. The study showed 1.7-fold higher concentration of drug when administered intranasally as compared with intravenously as determined by the area under concentration–time graph of the brain based on the radioiodine isotopic method. Moreover, the neuroprotective effect of the intranasal preparation was higher as compared with the intravenous preparation as determined by the mirror water maze task in AD rats, indicating that the system is a good brain DDR system for peptide- and protein-based drugs. Similarly, in another study, Arumugam et al. (2008) showed that intranasal delivery of rivastigmine-loaded multilamellar liposomes results in higher concentration and longer half-life in the brain of Wister rats in comparison to intranasal (non- liposomal) and oral preparation.
Targeted NPs for Delivery Across BBB
The advent of new strategies such as targeting agents, specific cell receptor ligands, and monoclonal antibodies have shifted the paradigm from non-specific neurotransmitter-based symptomatic treatment toward more personalized and targeted therapeutics that aim to disrupt unique biochemical processes necessary for plaque formation and its development (Bhaskar et al., 2010). Many researchers have explored the possibility of limiting the off-site binding and enhancing drug delivery of NPs across BBB by employing a targeting agent for specific BBB and Aβ receptors (Ulbrich et al., 2009). These agents include, but are not limited to apolipoprotein-E receptor-binding peptide and mAbs directed to transferrin and insulin receptors for BBB targeting and anti-amyloid beta antibody against Aβ42 for Aβ plaques targeting (Rocha, 2013). Similarly, targeting agents directed toward tau protein and GSH are also under various clinical trials.
In an in vitro study, Canovi et al. (2011) showed that nano-liposomes decorated with an anti-Aβ monoclonal antibody (Aβ-MAb) have a strong potential as vector for targeting Aβ1-42 peptides in vitro and plaque deposits in postmortem AD tissue. In addition to antibody-based targeting agents, several types of amyloid-specific cell receptor ligands are employed for targeted delivery of drug carriers across BBB. Gobbi et al. (2010) demonstrated in an in vitro immune-staining study that phosphatidic acid (PA)- and cardiolipin (CL)-decorated liposomes successfully interacted with Aβ1-42 aggregates, indicating that these liposomes are a potential vector for targeted delivery of anti-amyloid agents. In another similar study, Markoutsa et al. (2012) reported synthesis of dual-targeting liposomal NPs of two types: anti-Aβ–MAb (Aβ–MAb)-decorated immune-liposomes (LIP) and dually decorated LIP (dd-LIP) with OX-26 (directed toward transferrin receptor) and Aβ–MAb using biotin–STREP–biotin method. Both types of NPs showed an increase in binding (and transport) across human brain endothelial hCMEC/D3 cells model of BBB, indicating the potential of the targeting agent in increasing the binding and transport of NPs across BBB. The dd-LIP showed lysosomal uptake and it was proposed that the transport was receptor mediated, but validation of permeability across BBB needs further in vivo-based studies (Markoutsa et al., 2011, 2012). The group also reported, in another study, the synthesis of mono and dually decorated LIP tagged with anti-transferrin mAb alone and in combination with peptide derivative of apolipoprotein E3 (APOE) to target the low-density lipoprotein receptor-related protein (LPR), respectively. The PEG–LIP were taken as control (Markoutsa et al., 2014). In vitro studies in hCMEC/D3 cell monolayer showed additive effect of dual targeting in increasing the delivery across the monolayer, but in vivo studies in FVB mice indicated increased delivery of dual-LIP across BBB in comparison to control, but not to mono-LIP. This variable response in in vitro and in vivo studies was attributed to the presence of serum proteins. It was suggested that use of serum proteins in in vitro tests will be a predictive tool for targeting ability.
Moreover, benefits of the double-targeting approach were also developed for bifunctional liposomes tagged with apolipoprotein-E receptor-binding peptide for BBB targeting and with PA for Aβ binding (Balducci et al., 2014). The study in APP/presenilin 1 transgenic mice showed that the formulation successfully reduced brain Aβ burden and ameliorated memory impairment.
Diagnostic NPs for AD
As stated earlier, the inability to pinpoint the basic pathological event or maker of AD usually results in delayed diagnosis and treatment (Ayaz et al., 2017c). Many NPs have been checked for the delivery of diagnostic machinery across the BBB. This should ideally consist of unique fluorescent active or magnetic nanomaterial that can be detected non-invasively, packaged into biocompatible nano-vesicles decorated with a detecting ligand specific to early stage biomarkers of AD (Giljohann and Mirkin, 2009; Azria et al., 2017).
The fact is that radiolabeled Aβ40 peptide binds reversibly and specifically to diffuse and neuritic plaques and cerebrovascular amyloid in brain tissue obtained from AD patient. Radiolabeled Aβ40 peptide can be imaged in vivo non-invasively from the basis for its use in diagnostic and theranostic modalities related to AD. In order to improve its delivery across the BBB, targeting agents such as mAb, fusion proteins, and natural diamines are linked to it (Rocha, 2013). Some of these targeting strategies have been used in combination of NPs.
Yin et al. (2015) synthesized dimercaptosuccinic acid-coated magnetic NPs (MNPs) tagged with anti-amyloid antibodies. They showed that the synthesized NPs were biocompatible and biologically active with the potential of being used for early detection of AD (Yin et al., 2015). Similarly, in another study, Tanifum et al. (2016) reported the synthesis of amyloid fibril-binding ligand ET6-21. The ligand was used to prepare amyloid-targeted liposomes, tagged with Gd chelates and indocyanine green for visualization by magnetic resonance imaging (MRI) and infrared microscopy, respectively. The study showed successful binding of the particles to the plaques after intravenous administration in transgenic 2576 and APP (mutant APP) mouse models of AD. The study indicated the potential for development of MRI imaging agents for detection of amyloid plaques. Similarly, another group synthesized curcumin-loaded super paramagnetic iron oxide NPs of <100 nm for targeting of amyloid plaques (Cheng et al., 2015). The synthesized NPs were successful in differentiating the transgenic 2576 mouse brains having Aβ plaques from non-transgenic mouse models, indicating their potential as a diagnostic agent in AD.
Moreover, recent studies showed that Aβ oligomer instigate the early stage of memory loss and thus, is a better candidate as a biomarker of early-stage identification of AD. Viola et al. (2015) synthesized Aβ oligomer-targeted molecular MRI probes by attaching AβOs-specific monoclonal antibody (NU4) to the nitro-dopamine and polyethylene glycol stabilized 12–16 nm magnetite NPs (Viola et al., 2015). The study showed significant clinical potential of the AβO–MRI probe for identification of synapto-toxic oligomers in 5xFAD mouse model following intranasal delivery.
Theranostic NPs for Delivery Across BBB
Many preclinical studies have established the role of NPs as carriers and use of specific surface ligands for targeting the NP for AD separately. This leads to the development of multimodal preparations having characteristics of drug carriers tagged with targeting moieties and diagnostic agents. These magical NPs with all-in-one characteristics are the next-generation particles termed as “theranostic agent.”
Papadia et al. (2017) synthesized multifunctional liposomal NPs decorated with curcumin–lipid ligand (TREG) with affinity toward amyloid species along with ligands to target the transferrin and the LDL receptors of the BBB. The study showed successful targeting of brain and inhibition of amyloid peptide aggregation (Papadia et al., 2017). Similarly, Rotman et al. (2015) have also developed two liposomal formulations based on 1,2-dimyristoyl-sn-glycero-3-phosphocholine and egg-yolk PC encapsulating indium-111-labeled Aβ-binding llama single-domain antibody fragments (VHH-pa2H) and were coated with glutathione–PEG. The animal study in APPswe/PS1dE9 double transgenic mice showed the highest standard uptake in brain with GSH–PEG EYPC, indicating the theranostic potential of the prepared formulation (Rotman et al., 2015).
Green-Synthesized NPs as Future AD Theranostic Agents
Recent developments at the interface of green chemistry and nanotechnology indicate significant potential in the biomedical sciences from theranostic perspectives (Ovais et al., 2016, 2017b, 2018a,d; Barabadi et al., 2017b; Khalil et al., 2017a). However, their potential is largely untapped in case of the neurodegenerative diseases like Alzheimer’s. The use of other synthesis methods (physical and chemical) is often discouraged as they possess certain inherent disadvantages such as toxicity and cost (Khalil et al., 2017b; Ovais et al., 2017a). Moreover, in a recent work, it was reported that some chemicals used during the synthesis of NPs through a chemical route can remain attached on the surface of the NPs and, therefore, could not be used for biomedical application. Therefore, tremendous interest has been growing over the last decade to fabricate materials of interest using a green process (Emmanuel et al., 2017). Usually, the green chemistry-based approach includes the use of medicinal plants or pure phytochemicals that have potential medicinal value. These phytochemicals are involved in the chelating as well as stabilizing of NPs (Barabadi et al., 2017a; Ovais et al., 2017b, 2018c; Khalil et al., 2018).
Recently, Suganthy et al. (2017) reported the neuroprotective nature of biogenic gold NPs synthesized through Terminalia arjuna. Their results indicated the significant neuroprotective nature and biocompatibility of biogenic gold NPs. The biogenic gold NPs successfully inhibited the ChE enzymes, reduced the Aβ fibrillation process, and also destabilized the mature fibrils at very low concentrations. The functionalization of chemically synthesized gold NPs with trehalose has significantly improved the inhibition of protein aggregation as well as the mature fibril disintegration and possesses the potential to be used in photothermal therapies (Mandal et al., 2017). Functionalization of bioinspired gold NPs with such antiamyloidogenic molecules can be a fruitful strategy for further enhancing their neuroprotective nature. Another research contribution directed toward the neuroprotective behavior included the biogenic platinum NPs biosynthesized through Bacopa monnieri. The authors concluded that the neuroprotective potential of biogenic platinum nanoparticles is mediated via inhibition of free radicals generation and antioxidant effects (Nellore et al., 2013). Plant phytochemical-based functionalization of NPs has yielded amazing results. Recently, polyphenol-based functionalization of selenium NPs was achieved. In the research, nanoscaled selenium was coated with EGCG; a polyphenol found in the tea. The EGCG is known for its neuroprotective potential and possesses the ability to inhibit various amyloid-forming proteins such as amyloid beta, transthyretin, α-synuclein, and huntingtin, which are involved in the progression of AD. The EGCG-stabilized Se NPs were further coated with Tet-1 proteins, having strong neuronal affinity. The Tet1-Se@EGCH nanosystem induced effective inhibition of the fibrillation process of Aβ and also disintegrated the already-matured fibrils as shown in Figure 2. In addition, the indicated nanosystem was found to inhibit the DNA fragmentation and ROS generation while found to be effective at very low concentrations (Zhang J. et al., 2014). There is potential to replace the EGCG with other plant-based chemical entities. Curcumin or curcumin-based derivatives have yielded impressive properties to halt the disease progression of Alzheimer’s. Curcumin in conjunction with the benzothiazolinone possesses strong affinity for binding to amyloid and, hence, can be used to deliver curcumin or other natural products with anti-Alzheimer potential as summarized in Figure 3 (Megill et al., 2013; Fang et al., 2014).
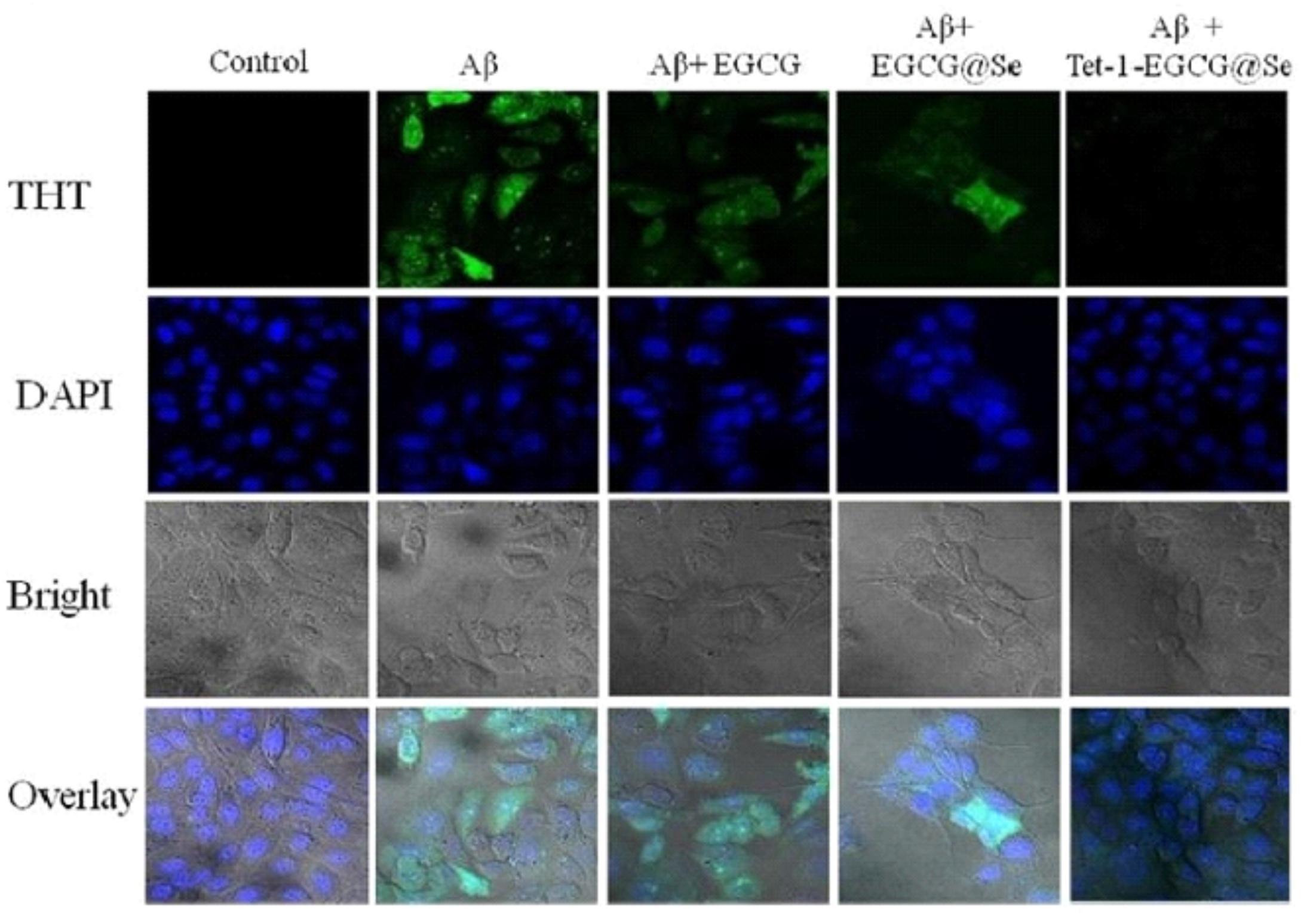
FIGURE 2. Tet-1-EGCG@Se preventing Aβ aggregation in PC12 cells. The presence of intracellular Aβ fibrils was evaluated by ThT staining in PC12 cells in the absence and presence of EGCG, EGCG@Se, and Tet-1-EGCG@Se. The cells were pretreated with an Aβ monomer for 6 h to allow access of Aβ to the cytoplasm, sequentially incubated with EGCG, EGCG@Se, or Tet-1-EGCG@Se for an additional 48 h, and visualized under a fluorescence microscope (adopted with permission from Zhang J. et al., 2014).
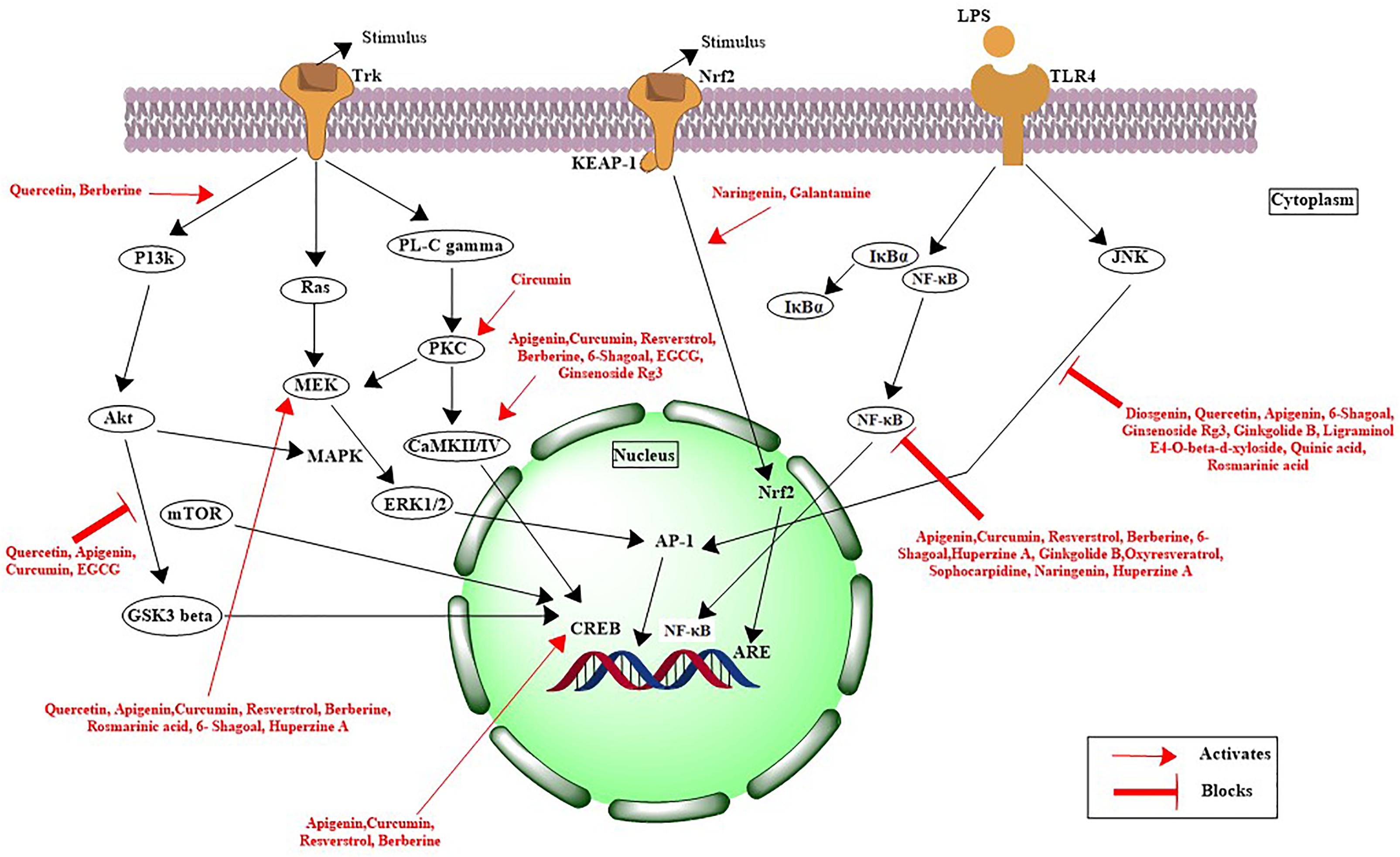
FIGURE 3. Mechanistic pathways for natural products involved in neuroprotection against Alzheimer’s disease.
Conclusion and Future Prospects
Recent research on phyto and nanomedicines represents a potential area of interest in the treatment of AD. Numerous phytochemicals have proven promising results in AD therapy, while with the emerging feat of tailored nanomedicines, the effectiveness can be increased several folds by efficient delivery of the active ingredients to the target sites. Moreover, a relatively untapped area of research at the interface of medicinal plants and nanotechnology is the biogenic synthesis of metal NPs for AD treatment.
The occurrence of metal and metal-based NPs in the brain can be toxic as well as beneficial. The NPs like TiO2, ZnO, Ag, and Au NPs etc. can induce oxidative damage in the brain. However, selenium NPs are reported to have an antioxidant role in the brain. Largely, these mentioned NPs were synthesized through chemistry-based methods. The green chemistry-based methods can alter the properties of these NPs. The science of biogenic NPs is still in the nascent phase, and theranostic NPs of variable and interesting features can be produced to alleviate the menace of AD.
Author Contributions
MO, NZ, and IA conceived the idea and drafted the manuscript. AK, AR, MA, AS, FU, and ZS contributed in manuscript drafting, language correction, and technical corrections. All the authors read and approved the manuscript.
Conflict of Interest Statement
The authors declare that the research was conducted in the absence of any commercial or financial relationships that could be construed as a potential conflict of interest.
The reviewer EL and handling Editor declared their shared affiliation at the time of the review.
References
Aalinkeel, R., Kutscher, H. L., Singh, A., Cwiklinski, K., Khechen, N., Schwartz, S. A., et al. (2018). Neuroprotective effects of a biodegradable poly (lactic-co-glycolic acid)-ginsenoside Rg3 nanoformulation: a potential nanotherapy for Alzheimer’s disease? J. Drug Target. 26, 182–193. doi: 10.1080/1061186X.2017.1354002
Abbott, N. J., Patabendige, A. A., Dolman, D. E., Yusof, S. R., and Begley, D. J. (2010). Structure and function of the blood–brain barrier. Neurobiol. Dis. 37, 13–25. doi: 10.1016/j.nbd.2009.07.030
Agarwa, P., Sharma, B., Fatima, A., and Jain, S. K. (2014). An update on Ayurvedic herb Convolvulus pluricaulis Choisy. Asian Pac. J. Trop. Biomed. 4, 245–252. doi: 10.1016/S2221-1691(14)60240-9
Aggarwal, B. B., and Sung, B. (2009). Pharmacological basis for the role of curcumin in chronic diseases: an age-old spice with modern targets. Trends Pharmacol. Sci. 30, 85–94. doi: 10.1016/j.tips.2008.11.002
Agnihotri, S. A., Mallikarjuna, N. N., and Aminabhavi, T. M. (2004). Recent advances on chitosan-based micro-and nanoparticles in drug delivery. J. Control. Release 100, 5–28. doi: 10.1016/j.jconrel.2004.08.010
Aguiar, S., and Borowski, T. (2013). Neuropharmacological review of the nootropic herb Bacopa monnieri. Rejuvenation Res. 16, 313–326. doi: 10.1089/rej.2013.1431
Agyare, E. K., Curran, G. L., Ramakrishnan, M., Caroline, C. Y., Poduslo, J. F., and Kandimalla, K. K. (2008). Development of a smart nano-vehicle to target cerebrovascular amyloid deposits and brain parenchymal plaques observed in Alzheimer’s disease and cerebral amyloid angiopathy. Pharm. Res. 25, 2674–2684. doi: 10.1007/s11095-008-9688-y
Ahlemeyer, B., and Krieglstein, J. (2003). Pharmacological studies supporting the therapeutic use of Ginkgo biloba extract for Alzheimer’s disease. Pharmacopsychiatry 36, 8–14. doi: 10.1055/s-2003-40454
Ahmad, S., Ullah, F., Sadiq, A., Ayaz, M., Imran, M., Ali, I., et al. (2016). Chemical composition, antioxidant and anticholinesterase potentials of essential oil of Rumex hastatus D. Don collected from the North West of Pakistan. BMC Complement. Alternat. Med. 16:29. doi: 10.1186/s12906-016-0998-z
Akhondzadeh, S., Sabet, M. S., Harirchian, M. H., Togha, M., Cheraghmakani, H., Razeghi, S., et al. (2010). A 22-week, multicenter, randomized, double-blind controlled trial of Crocus sativus in the treatment of mild-to-moderate Alzheimer’s disease. Psychopharmacology 207, 637–643. doi: 10.1007/s00213-009-1706-1
Akram, M., and Nawaz, A. (2017). Effects of medicinal plants on Alzheimer’s disease and memory deficits. Neural Regen. Res. 12, 660–670. doi: 10.4103/1673-5374.205108
Ali, M., Khan, T., Fatima, K., Ali, Q. U. A., Ovais, M., Khalil, A. T., et al. (2018). Selected hepatoprotective herbal medicines: evidence from ethnomedicinal applications, animal models, and possible mechanism of actions. Phytother. Res. 32, 199–215. doi: 10.1002/ptr.5957
Ali, M., Muhammad, S., Shah, M. R., Khan, A., Rashid, U., Farooq, U., et al. (2017). Neurologically potent molecules from Crataegus oxyacantha; isolation, anticholinesterase inhibition, and molecular docking. Front. Pharmacol. 8:327. doi: 10.3389/fphar.2017.00327
Ali, Y. O., Bradley, G., and Lu, H.-C. (2017). Screening with an NMNAT2-MSD platform identifies small molecules that modulate NMNAT2 levels in cortical neurons. Sci. Rep. 7:43846. doi: 10.1038/srep43846
Alzheimer’s and Association (2017). 2017 Alzheimer’s disease facts and figures. Alzheimers Dement. 13, 325–373. doi: 10.1016/j.jalz.2017.02.001
Anwar, A., Ovais, M., Khan, A., and Raza, A. (2017). Docetaxel-loaded solid lipid nanoparticles: a novel drug delivery system. IET Nanobiotechnol. 11, 621–629. doi: 10.1049/iet-nbt.2017.0001
Arumugam, K., Subramanian, G., Mallayasamy, S., Averineni, R., Reddy, M., and Udupa, N. (2008). A study of rivastigmine liposomes for delivery into the brain through intranasal route. Acta Pharm. 58, 287–297. doi: 10.2478/v10007-008-0014-3
Asgarpanah, J., and Kazemivash, N. (2012). Phytochemistry and pharmacologic properties of Myristica fragrans hoyutt: a review. Afr. J. Biotechnol. 11, 12787–12793. doi: 10.5897/AJB12.1043
Ayaz, M., Junaid, M., Ahmed, J., Ullah, F., Sadiq, A., Ahmad, S., et al. (2014). Phenolic contents, antioxidant and anticholinesterase potentials of crude extract, subsequent fractions and crude saponins from Polygonum hydropiper L. BMC Complement. Altern. Med. 14:145. doi: 10.1186/1472-6882-14-145
Ayaz, M., Junaid, M., Ullah, F., Sadiq, A., Khan, M. A., Ahmad, W., et al. (2015). Comparative chemical profiling, cholinesterase inhibitions and anti-radicals properties of essential oils from Polygonum hydropiper L: a preliminary anti-Alzheimer’s study. Lipids Health Dis. 14:141. doi: 10.1186/s12944-015-0145-8
Ayaz, M., Junaid, M., Ullah, F., Sadiq, A., Ovais, M., Ahmad, W., et al. (2016). Chemical profiling, antimicrobial and insecticidal evaluations of Polygonum hydropiper L. BMC Complement. Altern. Med. 16:502. doi: 10.1186/s12906-016-1491-4
Ayaz, M., Junaid, M., Ullah, F., Sadiq, A., Shahid, M., Ahmad, W., et al. (2017a). GC-MS analysis and gastroprotective evaluations of crude extracts, isolated saponins, and essential oil from Polygonum hydropiper L. Front. Chem. 5:58. doi: 10.3389/fchem.2017.00058
Ayaz, M., Junaid, M., Ullah, F., Subhan, F., Sadiq, A., Ali, G., et al. (2017b). Anti-Alzheimer’s studies on β-sitosterol isolated from Polygonum hydropiper L. Front. Pharmacol. 8:697. doi: 10.3389/fphar.2017.00697
Ayaz, M., Sadiq, A., Junaid, M., Ullah, F., Subhan, F., and Ahmed, J. (2017c). Neuroprotective and anti-aging potentials of essential oils from aromatic and medicinal plants. Front. Aging Neurosci. 9:168. doi: 10.3389/fnagi.2017.00168
Ayaz, M., Subhan, F., Sadiq, A., Ullah, F., Ahmed, J., and Sewell, R. (2017d). Cellular efflux transporters and the potential role of natural products in combating efflux mediated drug resistance. Front. Biosci. 22, 732–756. doi: 10.2741/4513
Azria, D., Blanquer, S., Verdier, J.-M., and Belamie, E. (2017). Nanoparticles as contrast agents for brain nuclear magnetic resonance imaging in Alzheimer’s disease diagnosis. J. Mater. Chem. B 5, 7216–7237. doi: 10.1039/C7TB01599B
Balducci, C., Mancini, S., Minniti, S., La Vitola, P., Zotti, M., Sancini, G., et al. (2014). Multifunctional liposomes reduce brain β-amyloid burden and ameliorate memory impairment in Alzheimer’s disease mouse models. J. Neurosci. 34, 14022–14031. doi: 10.1523/JNEUROSCI.0284-14.2014
Barabadi, H., Alizadeh, A., Ovais, M., Ahmadi, A., Shinwari, Z. K., and Saravanan, M. (2017a). Efficacy of green nanoparticles against cancerous and normal cell lines: a systematic review and meta-analysis. IET Nanobiotechnol. 12, 377–391. doi: 10.1049/iet-nbt.2017.0120
Barabadi, H., Ovais, M., Shinwari, Z. K., and Saravanan, M. (2017b). Anti-cancer green bionanomaterials: present status and future prospects. Green Chem. Lett. Rev. 10, 285–314. doi: 10.1080/17518253.2017.1385856
Baum, L., Lam, C. W. K., Cheung, S. K.-K., Kwok, T., Lui, V., Tsoh, J., et al. (2008). Six-month randomized, placebo-controlled, double-blind, pilot clinical trial of curcumin in patients with Alzheimer disease. J. Clin. Psychopharmacol. 28, 110–113. doi: 10.1097/jcp.0b013e318160862c
Baum, L., and Ng, A. (2004). Curcumin interaction with copper and iron suggests one possible mechanism of action in Alzheimer’s disease animal models. J. Alzheimers Dis. 6, 367–377. doi: 10.3233/JAD-2004-6403
Beason-Held, L. L., Goh, J. O., An, Y., Kraut, M. A., O’Brien, R. J., Ferrucci, L., et al. (2013). Changes in brain function occur years before the onset of cognitive impairment. J. Neurosci. 33, 18008–18014. doi: 10.1523/JNEUROSCI.1402-13.2013
Begley, D. J. (2004). ABC transporters and the blood-brain barrier. Curr. Pharm. Des. 10, 1295–1312. doi: 10.2174/1381612043384844
Begum, A. N., Jones, M. R., Lim, G. P., Morihara, T., Kim, P., Heath, D. D., et al. (2008). Curcumin structure-function, bioavailability, and efficacy in models of neuroinflammation and Alzheimer’s disease. J. Pharmacol. Exp. Ther. 326, 196–208. doi: 10.1124/jpet.108.137455
Bhanumathy, M., Harish, M., Shivaprasad, H., and Sushma, G. (2010). Nootropic activity of Celastrus paniculatus seed. Pharm. Biol. 48, 324–327. doi: 10.3109/13880200903127391
Bhaskar, S., Tian, F., Stoeger, T., Kreyling, W., de la Fuente, J. M., Grazú, V., et al. (2010). Multifunctional nanocarriers for diagnostics, drug delivery and targeted treatment across blood-brain barrier: perspectives on tracking and neuroimaging. Part. Fibre Toxicol. 7:3. doi: 10.1186/1743-8977-7-3
Bihaqi, S. W., Singh, A. P., and Tiwari, M. (2012). Supplementation of Convolvulus pluricaulis attenuates scopolamine-induced increased tau and Amyloid precursor protein (AβPP) expression in rat brain. Indian J. Pharmacol. 44, 593–598. doi: 10.4103/0253-7613.100383
Bird, T. D. (2008). Genetic aspects of Alzheimer disease. Genet. Med. 10, 231–239. doi: 10.1097/GIM.0b013e31816b64dc
Biswas, K., Islam, A., Sharmin, T., and Biswas, P. K. (2015). In-vitro cholinesterase inhibitory activity of dry fruit extract of Phyllanthus emblica relevant to the treatment of Alzheimer’s disease. J. Phytopharmacol. 4, 5–8.
Blusztajn, J. K., Slack, B. E., and Mellott, T. J. (2017). Neuroprotective actions of dietary choline. Nutrients 9:E815. doi: 10.3390/nu9080815
Bohrmann, B., Baumann, K., Benz, J., Gerber, F., Huber, W., Knoflach, F., et al. (2012). Gantenerumab: a novel human anti-Aβ antibody demonstrates sustained cerebral amyloid-β binding and elicits cell-mediated removal of human amyloid-β. J. Alzheimers Dis. 28, 49–69. doi: 10.3233/JAD-2011-110977
Canovi, M., Markoutsa, E., Lazar, A. N., Pampalakis, G., Clemente, C., Re, F., et al. (2011). The binding affinity of anti-Aβ1-42 MAb-decorated nanoliposomes to Aβ1-42 peptides in vitro and to amyloid deposits in post-mortem tissue. Biomaterials 32, 5489–5497. doi: 10.1016/j.biomaterials.2011.04.020
Carradori, D., Balducci, C., Re, F., Brambilla, D., Le Droumaguet, B., Flores, O., et al. (2018). Antibody-functionalized polymer nanoparticle leading to memory recovery in Alzheimer’s disease-like transgenic mouse model. Nanomedicine 14, 609–618. doi: 10.1016/j.nano.2017.12.006
Casey, D. A., Antimisiaris, D., and O’Brien, J. (2010). Drugs for Alzheimer’s disease: are they effective? Pharm. Ther. 35, 208–211.
Chaudhari, K. S., Tiwari, N. R., Tiwari, R. R., and Sharma, R. S. (2017). Neurocognitive effect of nootropic drug brahmi (Bacopa monnieri) in Alzheimer’s disease. Ann. Neurosci. 24, 111–122. doi: 10.1159/000475900
Chaudhary, B., and Bist, R. (2017). Protective manifestation of bacoside A and bromelain in terms of cholinesterases, gamma-amino butyric acid, serotonin level and stress proteins in the brain of dichlorvos-intoxicated mice. Cell Stress Chaperones 22, 371–376. doi: 10.1007/s12192-017-0773-1
Chen, W., Guo, Y., Yang, W., Zheng, P., Zeng, J., and Tong, W. (2015). Protective effect of ginsenoside Rb1 on integrity of blood–brain barrier following cerebral ischemia. Exp. Brain Res. 233, 2823–2831. doi: 10.1007/s00221-015-4352-3
Chen, Z., Mao, X., Liu, A., Gao, X., Chen, X., Ye, M., et al. (2015). Osthole, a natural coumarin improves cognitive impairments and BBB dysfunction after transient global brain ischemia in C57 BL/6J mice: involvement of Nrf2 pathway. Neurochem. Res. 40, 186–194. doi: 10.1007/s11064-014-1483-z
Chen, X., Hua, H.-P., Liang, L., and Liu, L.-J. (2017). The oral pretreatment of glycyrrhizin prevents surgery-induced cognitive impairment in aged mice by reducing neuroinflammation and Alzheimer’s-related pathology via HMGB1 inhibition. J. Mol. Neurosci. 63, 385–395. doi: 10.1007/s12031-017-0989-7
Cheng, K. K., Chan, P. S., Fan, S., Kwan, S. M., Yeung, K. L., Wang, Y.-X. J., et al. (2015). Curcumin-conjugated magnetic nanoparticles for detecting amyloid plaques in Alzheimer’s disease mice using magnetic resonance imaging (MRI). Biomaterials 44, 155–172. doi: 10.1016/j.biomaterials.2014.12.005
Cheng, K. K., Yeung, C. F., Ho, S. W., Chow, S. F., Chow, A. H., and Baum, L. (2013). Highly stabilized curcumin nanoparticles tested in an in vitro blood–brain barrier model and in Alzheimer’s disease Tg2576 mice. AAPS J. 15, 324–336. doi: 10.1208/s12248-012-9444-4
Chu, Y.-F., Brown, P. H., Lyle, B. J., Chen, Y., Black, R. M., Williams, C. E., et al. (2009). Roasted coffees high in lipophilic antioxidants and chlorogenic acid lactones are more neuroprotective than green coffees. J. Agric. Food Chem. 57, 9801–9808. doi: 10.1021/jf902095z
Colín-González, A. L., Santana, R. A., Silva-Islas, C. A., Chánez-Cárdenas, M. E., Santamaría, A., and Maldonado, P. D. (2012). The antioxidant mechanisms underlying the aged garlic extract-and S-allylcysteine-induced protection. Oxid. Med. Cell. Longev. 2012:907162. doi: 10.1155/2012/907162
Cropley, V., Croft, R., Silber, B., Neale, C., Scholey, A., Stough, C., et al. (2012). Does coffee enriched with chlorogenic acids improve mood and cognition after acute administration in healthy elderly? A pilot study. Psychopharmacology 219, 737–749. doi: 10.1007/s00213-011-2395-0
Cui, Z., Lockman, P. R., Atwood, C. S., Hsu, C.-H., Gupte, A., Allen, D. D., et al. (2005). Novel D-penicillamine carrying nanoparticles for metal chelation therapy in Alzheimer’s and other CNS diseases. Eur. J. Pharm. Biopharm. 59, 263–272. doi: 10.1016/j.ejpb.2004.07.009
Cummings, J., Morstorf, T., and Lee, G. (2016). Alzheimer’s drug-development pipeline: 2016. Alzheimers Dement. (N.Y.) 2, 222-232. doi: 10.1016/j.trci.2016.07.001
Daneman, R., and Prat, A. (2015). The blood–brain barrier. Cold Spring Harb. Perspect. Biol. 7:a020412. doi: 10.1101/cshperspect.a020412
Danhier, F., Ansorena, E., Silva, J. M., Coco, R., Le Breton, A., and Préat, V. (2012). PLGA-based nanoparticles: an overview of biomedical applications. J. Control. Release 161, 505–522. doi: 10.1016/j.jconrel.2012.01.043
De Campos, A. M., Sánchez, A., and Alonso, M. J. (2001). Chitosan nanoparticles: a new vehicle for the improvement of the delivery of drugs to the ocular surface. Application to cyclosporin A. Int. J. Pharm. 224, 159–168. doi: 10.1016/S0378-5173(01)00760-8
DeFeudis, F. V. (2002). Effects of Ginkgo biloba extract (EGb 761) on gene expression: possible relevance to neurological disorders and age-associated cognitive impairment. Drug Dev. Res. 57, 214–235. doi: 10.1002/ddr.10151
Dhanasekaran, M., Holcomb, L. A., Hitt, A. R., Tharakan, B., Porter, J. W., Young, K. A., et al. (2009). Centella asiatica extract selectively decreases amyloid β levels in hippocampus of Alzheimer’s disease animal model. Phytother. Res. 23, 14–19. doi: 10.1002/ptr.2405
Di Stefano, A., Iannitelli, A., Laserra, S., and Sozio, P. (2011). Drug delivery strategies for Alzheimer’s disease treatment. Expert Opin. Drug Deliv. 8, 581–603. doi: 10.1517/17425247.2011.561311
Dubey, G., Pathak, S., and Gupta, B. (1994). Combined effect of Brahmi (Bacopa monniera) and Shankhpushpi (Convolvulus pluricaulis) on cognitive functions. Pharmacopsychoecol 7, 249–251.
Dutta, P. K., Dutta, J., and Tripathi, V. (2004). Chitin and chitosan: chemistry, properties and applications. J. Sci. Ind. Res. 63, 20–31.
Emmanuel, R., Saravanan, M., Ovais, M., Padmavathy, S., Shinwari, Z. K., and Prakash, P. (2017). Antimicrobial efficacy of drug blended biosynthesized colloidal gold nanoparticles from Justicia glauca against oral pathogens: a nanoantibiotic approach. Microb. Pathog. 113, 295–302. doi: 10.1016/j.micpath.2017.10.055
Erickson, M. A., and Banks, W. A. (2013). Blood–brain barrier dysfunction as a cause and consequence of Alzheimer’s disease. J. Cereb. Blood Flow Metab. 33, 1500–1513. doi: 10.1038/jcbfm.2013.135
Esquivel, P., and Jiménez, V. M. (2012). Functional properties of coffee and coffee by-products. Food Res. Int. 46, 488–495. doi: 10.1016/j.foodres.2011.05.028
Fang, L., Gou, S., Liu, X., Cao, F., and Cheng, L. (2014). Design, synthesis and anti-Alzheimer properties of dimethylaminomethyl-substituted curcumin derivatives. Bioorg. Med. Chem. Lett. 24, 40–43. doi: 10.1016/j.bmcl.2013.12.011
Fang, W., Deng, Y., Li, Y., Shang, E., Fang, F., Lv, P., et al. (2010). Blood brain barrier permeability and therapeutic time window of Ginkgolide B in ischemia–reperfusion injury. Eur. J. Pharm. Sci. 39, 8–14. doi: 10.1016/j.ejps.2009.10.002
Faria, A., Meireles, M., Fernandes, I., Santos-Buelga, C., Gonzalez-Manzano, S., Dueñas, M., et al. (2014). Flavonoid metabolites transport across a human BBB model. Food Chem. 149, 190–196. doi: 10.1016/j.foodchem.2013.10.095
Faria, A., Pestana, D., Teixeira, D., Couraud, P.-O., Romero, I., Weksler, B., et al. (2011). Insights into the putative catechin and epicatechin transport across blood-brain barrier. Food Funct. 2, 39–44. doi: 10.1039/C0FO00100G
Farlow, M. R., Andreasen, N., Riviere, M.-E., Vostiar, I., Vitaliti, A., Sovago, J., et al. (2015). Long-term treatment with active Aβ immunotherapy with CAD106 in mild Alzheimer’s disease. Alzheimers Res. Ther. 7:23. doi: 10.1186/s13195-015-0108-3
Farokhnia, M., Shafiee Sabet, M., Iranpour, N., Gougol, A., Yekehtaz, H., Alimardani, R., et al. (2014). Comparing the efficacy and safety of Crocus sativus L. with memantine in patients with moderate to severe Alzheimer’s disease: a double-blind randomized clinical trial. Hum. Psychopharmacol. 29, 351–359. doi: 10.1002/hup.2412
Fazil, M., Md, S., Haque, S., Kumar, M., Baboota, S., Sahni, J. K., et al. (2012). Development and evaluation of rivastigmine loaded chitosan nanoparticles for brain targeting. Eur. J. Pharm. Sci. 47, 6–15. doi: 10.1016/j.ejps.2012.04.013
Finley, J. W., and Gao, S. (2017). A perspective on Crocus sativus L.(Saffron) constituent crocin: a potent water-soluble antioxidant and potential therapy for Alzheimer’s disease. J. Agric. Food Chem. 65, 1005–1020. doi: 10.1021/acs.jafc.6b04398
Fonseca-Santos, B., Gremião, M. P. D., and Chorilli, M. (2015). Nanotechnology-based drug delivery systems for the treatment of Alzheimer’s disease. Int. J. Nanomedicine 10, 4981–5003. doi: 10.2147/IJN.S87148
França, M. B., Lima, K. C., and Eleutherio, E. C. (2017). Oxidative stress and amyloid toxicity: insights from yeast. J. Cell. Biochem. 118, 1442–1452. doi: 10.1002/jcb.25803
Galimberti, D., and Scarpini, E. (2010). Treatment of Alzheimer’s disease: symptomatic and disease-modifying approaches. Curr. Aging Sci. 3, 46–56. doi: 10.2174/1874609811003010046
Garrido-Maraver, J., Cordero, M. D., Oropesa-Ávila, M., Vega, A. F., De La Mata, M., Pavón, A. D., et al. (2014). Coenzyme q10 therapy. Mol. Syndromol. 5, 187–197. doi: 10.1159/000360101
Geiser, R. J., Chastain, S. E., and Moss, M. A. (2017). Regulation of Bace1 Mrna expression in Alzheimer’S disease by green tea catechins and black tea theaflavins. Biophys. J. 112:362a. doi: 10.1016/j.bpj.2016.11.1965
Gharibi, A., Khalili, M., Kiasalari, Z., and Hoseinirad, M. (2013). The effect of Zingiber officinalis L. on learning and memory in rats. J. Bas. Clin. Pathophysiol. 2, 2013–2014.
Ghosh, A., Sarkar, S., Mandal, A. K., and Das, N. (2013). Neuroprotective role of nanoencapsulated quercetin in combating ischemia-reperfusion induced neuronal damage in young and aged rats. PLoS One 8:e57735. doi: 10.1371/journal.pone.0057735
Giacobini, E. (2004). Cholinesterase inhibitors: new roles and therapeutic alternatives. Pharmacol. Res. 50, 433–440. doi: 10.1016/j.phrs.2003.11.017
Giljohann, D. A., and Mirkin, C. A. (2009). Drivers of biodiagnostic development. Nature 462, 461–464. doi: 10.1038/nature08605
Gobbi, M., Re, F., Canovi, M., Beeg, M., Gregori, M., Sesana, S., et al. (2010). Lipid-based nanoparticles with high binding affinity for amyloid-β1–42 peptide. Biomaterials 31, 6519–6529. doi: 10.1016/j.biomaterials.2010.04.044
Godkar, P., Gordon, R., Ravindran, A., and Doctor, B. (2006). Celastrus paniculatus seed oil and organic extracts attenuate hydrogen peroxide-and glutamate-induced injury in embryonic rat forebrain neuronal cells. Phytomedicine 13, 29–36. doi: 10.1016/j.phymed.2003.11.011
Grzybowska, B., Słoczyñski, J., Grabowski, R., Wcisło, K., Kozłowska, A., Stoch, J., et al. (1998). Chromium oxide/alumina catalysts in oxidative dehydrogenation of isobutane. J. Catal. 178, 687–700. doi: 10.1006/jcat.1998.2203
Hebert, L. E., Weuve, J., Scherr, P. A., and Evans, D. A. (2013). Alzheimer disease in the United States (2010–2050) estimated using the 2010 census. Neurology 80, 1778–1783. doi: 10.1212/WNL.0b013e31828726f5
Heneka, M. T., Carson, M. J., El Khoury, J., Landreth, G. E., Brosseron, F., Feinstein, D. L., et al. (2015). Neuroinflammation in Alzheimer’s disease. Lancet Neurol. 14, 388–405. doi: 10.1016/S1474-4422(15)70016-5
Ho, Y.-S., Hung, C. H.-L., and Chang, R. C.-C. (2013). Green tea and neurodegeneration in Alzheimer’s disease, in Tea in Health and Disease Prevention, ed. V. R. Preedy (New York, NY: Elsevier), 691–704.
Hoi, C. P., Ho, Y. P., Baum, L., and Chow, A. H. (2010). Neuroprotective effect of honokiol and magnolol, compounds from Magnolia officinalis, on beta-amyloid-induced toxicity in PC12 cells. Phytother. Res. 24, 1538–1542. doi: 10.1002/ptr.3178
Hu, Z., Bian, X., Liu, X., Zhu, Y., Zhang, X., Chen, S., et al. (2013). Honokiol protects brain against ischemia–reperfusion injury in rats through disrupting PSD95–nNOS interaction. Brain Res. 1491, 204–212. doi: 10.1016/j.brainres.2012.11.004
Huang, M., Xie, S.-S., Jiang, N., Lan, J.-S., Kong, L.-Y., and Wang, X.-B. (2015). Multifunctional coumarin derivatives: monoamine oxidase B (MAO-B) inhibition, anti-β-amyloid (Aβ) aggregation and metal chelation properties against Alzheimer’s disease. Bioorg. Med. Chem. Lett. 25, 508–513. doi: 10.1016/j.bmcl.2014.12.034
Ishisaka, A., Ichikawa, S., Sakakibara, H., Piskula, M. K., Nakamura, T., Kato, Y., et al. (2011). Accumulation of orally administered quercetin in brain tissue and its antioxidative effects in rats. Free Radic. Biol. Med. 51, 1329–1336. doi: 10.1016/j.freeradbiomed.2011.06.017
Ismail, M. F., ElMeshad, A. N., and Salem, N. A.-H. (2013). Potential therapeutic effect of nanobased formulation of rivastigmine on rat model of Alzheimer’s disease. Int. J. Nanomedicine 8, 393–406. doi: 10.2147/IJN.S39232
Ito, H., Sun, X.-L., Watanabe, M., Okamoto, M., and Hatano, T. (2008). Chlorogenic acid and its metabolite m-coumaric acid evoke neurite outgrowth in hippocampal neuronal cells. Biosci. Biotechnol. Biochem. 72, 885–888. doi: 10.1271/bbb.70670
Jalali, S., Zarrinhaghighi, A., Sadraei, S., Ghasemi, Y., Sakhteman, A., and Faridi, P. (2018). A system pharmacology study for deciphering anti depression activity of Nardostachys jatamansi. Curr. Drug Metab. 19, 469–476. doi: 10.2174/1389200219666180305151011
Jameel, E., Umar, T., Kumar, J., and Hoda, N. (2016). Coumarin: a privileged scaffold for the design and development of antineurodegenerative agents. Chem. Biol. Drug Des. 87, 21–38. doi: 10.1111/cbdd.12629
James, S. A., Churches, Q. I., de Jonge, M. D., Birchall, I. E., Streltsov, V., McColl, G., et al. (2016). Iron, copper, and zinc concentration in Aβ plaques in the APP/PS1 mouse model of Alzheimer’s disease correlates with metal levels in the surrounding neuropil. ACS Chem. Neurosci. 8, 629–637. doi: 10.1021/acschemneuro.6b00362
Jayaprakasam, B., Padmanabhan, K., and Nair, M. G. (2010). Withanamides in Withania somnifera fruit protect PC-12 cells from β-amyloid responsible for Alzheimer’s disease. Phytother. Res. 24, 859–863.
Jiang, J., Wang, W., Sun, Y. J., Hu, M., Li, F., and Zhu, D. Y. (2007). Neuroprotective effect of curcumin on focal cerebral ischemic rats by preventing blood–brain barrier damage. Eur. J. Pharmacol. 561, 54–62. doi: 10.1016/j.ejphar.2006.12.028
Johansson, B. B. (1990). The physiology of the blood-brain barrier, in Circulating Regulatory Factors and Neuroendocrine Function, eds J. C. Porter and D. Ježová (Boston, MA: Springer), 25–39. doi: 10.1007/978-1-4684-5799-5_2
Jose, S., Sowmya, S., Cinu, T., Aleykutty, N., Thomas, S., and Souto, E. (2014). Surface modified PLGA nanoparticles for brain targeting of Bacoside-A. Eur. J. Pharm. Sci. 63, 29–35. doi: 10.1016/j.ejps.2014.06.024
Joubert, J., Foka, G. B., Repsold, B. P., Oliver, D. W., Kapp, E., and Malan, S. F. (2017). Synthesis and evaluation of 7-substituted coumarin derivatives as multimodal monoamine oxidase-B and cholinesterase inhibitors for the treatment of Alzheimer’s disease. Eur. J. Med. Chem. 125, 853–864. doi: 10.1016/j.ejmech.2016.09.041
Karami, M., Bathaie, S. Z., Tiraihi, T., Habibi-Rezaei, M., Arabkheradmand, J., and Faghihzadeh, S. (2013). Crocin improved locomotor function and mechanical behavior in the rat model of contused spinal cord injury through decreasing calcitonin gene related peptide (CGRP). Phytomedicine 21, 62–67. doi: 10.1016/j.phymed.2013.07.013
Karkada, G., Shenoy, K., Halahalli, H., and Karanth, K. (2012). Nardostachys jatamansi extract prevents chronic restraint stress-induced learning and memory deficits in a radial arm maze task. J. Nat. Sci. Biol. Med. 3, 125–132. doi: 10.4103/0976-9668.101879
Kaur, N., Dhiman, M., Perez-Polo, J. R., and Mantha, A. K. (2015). Ginkgolide B revamps neuroprotective role of apurinic/apyrimidinic endonuclease 1 and mitochondrial oxidative phosphorylation against Aβ25–35-induced neurotoxicity in human neuroblastoma cells. J. Neurosci. Res. 93, 938–947. doi: 10.1002/jnr.23565
Kennedy, M. E., Stamford, A. W., Chen, X., Cox, K., Cumming, J. N., Dockendorf, M. F., et al. (2016). The BACE1 inhibitor verubecestat (MK-8931) reduces CNS β-amyloid in animal models and in Alzheimer’s disease patients. Sci. Transl. Med. 8:363ra150. doi: 10.1126/scitranslmed.aad9704
Khachaturian, Z. S. (1985). Diagnosis of Alzheimer’s disease. Arch. Neurol. 42, 1097–1105. doi: 10.1001/archneur.1985.04060100083029
Khalil, A. T., Ovais, M., Ullah, I., Ali, M., Jan, S. A., Shinwari, Z. K., et al. (2017a). Bioinspired synthesis of pure massicot phase lead oxide nanoparticles and assessment of their biocompatibility, cytotoxicity and in-vitro biological properties. Arab. J. Chem. (in press). doi: 10.1016/j.arabjc.2017.08.009
Khalil, A. T., Ovais, M., Ullah, I., Ali, M., Shinwari, Z. K., Khamlich, S., et al. (2017b). Sageretia thea (Osbeck.) mediated synthesis of zinc oxide nanoparticles and its biological applications. Nanomedicine 12, 1767–1789. doi: 10.2217/nnm-2017-0124
Khalil, A. T., Ovais, M., Ullah, I., Ali, M., Shinwari, Z. K., Hassan, D., et al. (2018). Sageretia thea (Osbeck.) modulated biosynthesis of NiO nanoparticles and their in vitro pharmacognostic, antioxidant and cytotoxic potential. Artif. Cells Nanomed. Biotechnol. 46, 838–852. doi: 10.1080/21691401.2017.1345928
Khojah, H., and Edrada-Ebel, R. (2017). Identification of bioactive metabolites from Ficus carica and their neuroprotective effects of Alzheimer’s disease. Int. J. Med. Health Sci. 11, 2277–4505.
Kim, J., Basak, J. M., and Holtzman, D. M. (2009). The role of apolipoprotein E in Alzheimer’s disease. Neuron 63, 287–303. doi: 10.1016/j.neuron.2009.06.026
Kodera, Y., Suzuki, A., Imada, O., Kasuga, S., Sumioka, I., Kanezawa, A., et al. (2002). Physical, chemical, and biological properties of S-allylcysteine, an amino acid derived from garlic. J. Agric. Food Chem. 50, 622–632. doi: 10.1021/jf0106648
Krishnamurthy, R. G., Senut, M. C., Zemke, D., Min, J., Frenkel, M. B., Greenberg, E. J., et al. (2009). Asiatic acid, a pentacyclic triterpene from Centella asiatica, is neuroprotective in a mouse model of focal cerebral ischemia. J. Neurosci. Res. 87, 2541–2550. doi: 10.1002/jnr.22071
Kulkarni, P. D., Ghaisas, M. M., Chivate, N. D., and Sankpal, P. S. (2011). Memory enhancing activity of Cissampelos pareira in mice. Int. J. Pharm. Pharma. Sci. 3, 206–211.
Kwon, S.-H., Lee, H.-K., Kim, J.-A., Hong, S.-I., Kim, H.-C., Jo, T.-H., et al. (2010). Neuroprotective effects of chlorogenic acid on scopolamine-induced amnesia via anti-acetylcholinesterase and anti-oxidative activities in mice. Eur. J. Pharmacol. 649, 210–217. doi: 10.1016/j.ejphar.2010.09.001
Lang, D., Ude, C., Wurglics, M., Schubert-Zsilavecz, M., and Klein, J. (2010). Brain permeability of bilobalide as probed by microdialysis before and after middle cerebral artery occlusion in mice. J. Pharm. Pharm. Sci. 13, 607–614. doi: 10.18433/J31C7Q
Laribi, B., Kouki, K., M’Hamdi, M., and Bettaieb, T. (2015). Coriander (Coriandrum sativum L.) and its bioactive constituents. Fitoterapia 103, 9–26. doi: 10.1016/j.fitote.2015.03.012
Lee, Y. K., Yuk, D. Y., Kim, T. I., Kim, Y. H., Kim, K. T., Kim, K. H., et al. (2009). Protective effect of the ethanol extract of Magnolia officinalis and 4-O-methylhonokiol on scopolamine-induced memory impairment and the inhibition of acetylcholinesterase activity. J. Nat. Med. 63, 274–282. doi: 10.1007/s11418-009-0330-z
Li, J., Yue, M., Zhou, D., Wang, M., and Zhang, H. (2017). Abcb1a but not Abcg2 played a predominant role in limiting the brain distribution of Huperzine A in mice. Food Chem. Toxicol. 107, 68–73. doi: 10.1016/j.fct.2017.06.005
Li, J., Zhou, L., Ye, D., Huang, S., Shao, K., Huang, R., et al. (2011). Choline-derivate-modified nanoparticles for brain-targeting gene delivery. Adv. Mater. 23, 4516–4520. doi: 10.1002/adma.201101899
Li, P., Matsunaga, K., Yamakuni, T., and Ohizumi, Y. (2003). Nardosinone, the first enhancer of neurite outgrowth-promoting activity of staurosporine and dibutyryl cyclic AMP in PC12D cells. Dev. Brain Res. 145, 177–183. doi: 10.1016/S0165-3806(03)00239-6
Liang, X.-F., Wang, H.-J., Tian, H., Luo, H., and Chang, J. (2008). Syntheis, structure and properties of novel quaternized carboxymethyl chitosan with drug loading capacity. Acta Phys. Chim. Sin. 24, 223–229. doi: 10.1016/S1872-1508(08)60011-X
Lim, G. P., Chu, T., Yang, F., Beech, W., Frautschy, S. A., and Cole, G. M. (2001). The curry spice curcumin reduces oxidative damage and amyloid pathology in an Alzheimer transgenic mouse. J. Neurosci. 21, 8370–8377. doi: 10.1523/JNEUROSCI.21-21-08370.2001
Liu, G., Men, P., Kudo, W., Perry, G., and Smith, M. A. (2009). Nanoparticle–chelator conjugates as inhibitors of amyloid-β aggregation and neurotoxicity: a novel therapeutic approach for Alzheimer disease. Neurosci. Lett. 455, 187–190. doi: 10.1016/j.neulet.2009.03.064
Liu, Q. F., Jeong, H., Lee, J. H., Hong, Y. K., Oh, Y., Kim, Y.-M., et al. (2016). Coriandrum sativum suppresses Aβ42-induced ROS increases, glial cell proliferation, and ERK activation. Am. J. Chin. Med. 44, 1325–1347. doi: 10.1142/S0192415X16500749
Lockman, P., Mumper, R., Khan, M., and Allen, D. (2002). Nanoparticle technology for drug delivery across the blood-brain barrier. Drug Dev. Ind. Pharm. 28, 1–13. doi: 10.1081/DDC-120001481
Loveman, E., Green, C., Kirby, J., Takeda, A., Picot, J., Payne, E., et al. (2006). The clinical and cost-effectiveness of donepezil, rivastigmine, galantamine and memantine for Alzheimer’s disease. Health Technol. Assess. 10, iii–iv, ix–xi, 1–160.
Ma, T., Gong, K., Yan, Y., Zhang, L., Tang, P., Zhang, X., et al. (2013). Huperzine A promotes hippocampal neurogenesis in vitro and in vivo. Brain Res. 1506, 35–43. doi: 10.1016/j.brainres.2013.02.026
Maclennan, K. M., Darlington, C. L., and Smith, P. F. (2002). The CNS effects of Ginkgo biloba extracts and ginkgolide B. Prog. Neurobiol. 67, 235–257. doi: 10.1016/S0301-0082(02)00015-1
Madgula, V. L., Avula, B., Yu, Y.-B., Wang, Y.-H., Tchantchou, F., Fisher, S., et al. (2010). Intestinal and blood-brain barrier permeability of ginkgolides and bilobalide: in vitro and in vivo approaches. Planta Med. 76, 599–606. doi: 10.1055/s-0029-1240601
Mahrous, R., Ghareeb, D. A., Fathy, H. M., EL-Khair, R. M. A., and Omar, A. A. (2017). The protective effect of Egyptian Withania somnifera against Alzheimer’s. Med. Aromat Plants 6:285. doi: 10.4172/2167-0412.1000285
Malik, J., Karan, M., and Dogra, R. (2017). Ameliorating effect of Celastrus paniculatus standardized extract and its fractions on 3-nitropropionic acid induced neuronal damage in rats: possible antioxidant mechanism. Pharm. Biol. 55, 980–990. doi: 10.1080/13880209.2017.1285945
Mandal, S., Debnath, K., Jana, N. R., and Jana, N. R. (2017). Trehalose-functionalized gold nanoparticle for inhibiting intracellular protein aggregation. Langmuir 33, 13996–14003. doi: 10.1021/acs.langmuir.7b02202
Mangialasche, F., Solomon, A., Winblad, B., Mecocci, P., and Kivipelto, M. (2010). Alzheimer’s disease: clinical trials and drug development. Lancet Neurol. 9, 702–716. doi: 10.1016/S1474-4422(10)70119-8
Mani, V., and Parle, M. (2009). Memory-enhancing activity of Coriandrum sativum in rats. Pharmacologyonline 2, 827–839.
Mao, X., Yin, W., Liu, M., Ye, M., Liu, P., Liu, J., et al. (2011). Osthole, a natural coumarin, improves neurobehavioral functions and reduces infarct volume and matrix metalloproteinase-9 activity after transient focal cerebral ischemia in rats. Brain Res. 1385, 275–280. doi: 10.1016/j.brainres.2011.02.015
Markoutsa, E., Pampalakis, G., Niarakis, A., Romero, I. A., Weksler, B., Couraud, P. -O., et al. (2011). Uptake and permeability studies of BBB-targeting immunoliposomes using the hCMEC/D3 cell line. Eur. J. Pharm. Biopharm. 77, 265–274. doi: 10.1016/j.ejpb.2010.11.015
Markoutsa, E., Papadia, K., Clemente, C., Flores, O., and Antimisiaris, S. G. (2012). Anti-Aβ-MAb and dually decorated nanoliposomes: effect of Aβ1-42 peptides on interaction with hCMEC/D3 cells. Eur. J. Pharm. Biopharm. 81, 49–56. doi: 10.1016/j.ejpb.2012.02.006
Markoutsa, E., Papadia, K., Giannou, A., Spella, M., Cagnotto, A., Salmona, M., et al. (2014). Mono and dually decorated nanoliposomes for brain targeting, in vitro and in vivo studies. Pharm. Res. 31, 1275–1289. doi: 10.1007/s11095-013-1249-3
Marumoto, S., Okuno, Y., and Miyazawa, M. (2017). Inhibition of β-secretase activity by monoterpenes, sesquiterpenes, and C13 norisoprenoids. J. Oleo Sci. 66, 851–855. doi: 10.5650/jos.ess16188
Masserini, M. (2013). Nanoparticles for brain drug delivery. ISRN Biochem. 2013:238428. doi: 10.1155/2013/238428
Md, S., Ali, M., Baboota, S., Sahni, J. K., Bhatnagar, A., et al. (2014). Preparation, characterization, in vivo biodistribution and pharmacokinetic studies of donepezil-loaded PLGA nanoparticles for brain targeting. Drug Dev. Ind. Pharm. 40, 278–287. doi: 10.3109/03639045.2012.758130
Megill, A., Lee, T., DiBattista, A. M., Song, J. M., Spitzer, M. H., Rubinshtein, M., et al. (2013). A tetra (ethylene glycol) derivative of benzothiazole aniline enhances Ras-mediated spinogenesis. J. Neurosci. 33, 9306–9318. doi: 10.1523/JNEUROSCI.1615-12.2013
Mellott, T. J., Huleatt, O. M., Shade, B. N., Pender, S. M., Liu, Y. B., Slack, B. E., et al. (2017). Perinatal choline supplementation reduces amyloidosis and increases choline acetyltransferase expression in the hippocampus of the APPswePS1dE9 Alzheimer’s disease model mice. PLoS One 12:e0170450. doi: 10.1371/journal.pone.0170450
Meng, Q., Wang, A., Hua, H., Jiang, Y., Wang, Y., Mu, H., et al. (2018). Intranasal delivery of Huperzine A to the brain using lactoferrin-conjugated N-trimethylated chitosan surface-modified PLGA nanoparticles for treatment of Alzheimer’s disease. Int. J. Nanomedicine 13:705–718. doi: 10.2147/IJN.S151474
Minati, L., Edginton, T., Grazia Bruzzone, M., and Giaccone, G. (2009). Reviews: current concepts in Alzheimer’s disease: a multidisciplinary review. Am. J. Alzheimers Dis. Other Demen. 24, 95–121. doi: 10.1177/1533317508328602
Mistry, A., Stolnik, S., and Illum, L. (2009). Nanoparticles for direct nose-to-brain delivery of drugs. Int. J. Pharm. 379, 146–157. doi: 10.1016/j.ijpharm.2009.06.019
Modi, G., Pillay, V., and Choonara, Y. E. (2010). Advances in the treatment of neurodegenerative disorders employing nanotechnology. Ann. N. Y. Acad. Sci. 1184, 154–172. doi: 10.1111/j.1749-6632.2009.05108.x
Mutlu, N. B., Debbbim, Z., Yılmaz, Ş., Eşsiz, D., and Nacar, A. (2011). New perspective for the treatment of Alzheimer diseases: liposomal rivastigmine formulations. Drug Dev. Ind. Pharm. 37, 775–789. doi: 10.3109/03639045.2010.541262
Nazem, A., and Mansoori, G. A. (2011). Nanotechnology for Alzheimer’s disease detection and treatment. Insciences J. 1, 169–193. doi: 10.5640/insc.0104169
Nellore, J., Pauline, C., and Amarnath, K. (2013). Bacopa monnieri phytochemicals mediated synthesis of platinum nanoparticles and its neurorescue effect on 1-methyl 4-phenyl 1,2,3,6 tetrahydropyridine-induced experimental parkinsonism in zebrafish. J. Neurodegener. Dis. 2013:972391. doi: 10.1155/2013/972391
Nie, L., Xia, J., Li, H., Zhang, Z., Yang, Y., Huang, X., et al. (2017). Ginsenoside Rg1 ameliorates behavioral abnormalities and modulates the hippocampal proteomic change in triple transgenic mice of Alzheimer’s disease. Oxid. Med. Cell. Longev. 2017:6473506. doi: 10.1155/2017/6473506
Nillert, N., Pannangrong, W., Welbat, J. U., Chaijaroonkhanarak, W., Sripanidkulchai, K., and Sripanidkulchai, B. (2017). Neuroprotective effects of aged garlic extract on cognitive dysfunction and neuroinflammation induced by β-amyloid in rats. Nutrients 9:24. doi: 10.3390/nu9010024
Noetzli, M., and Eap, C. B. (2013). Pharmacodynamic, pharmacokinetic and pharmacogenetic aspects of drugs used in the treatment of Alzheimer’s disease. Clin. Pharmacokinet. 52, 225–241. doi: 10.1007/s40262-013-0038-9
Oboh, G., Agunloye, O. M., Akinyemi, A. J., Ademiluyi, A. O., and Adefegha, S. A. (2013). Comparative study on the inhibitory effect of caffeic and chlorogenic acids on key enzymes linked to Alzheimer’s disease and some pro-oxidant induced oxidative stress in rats’ brain-in vitro. Neurochem. Res. 38, 413–419. doi: 10.1007/s11064-012-0935-6
Ossola, B., Kääriäinen, T. M., and Männistö, P. T. (2009). The multiple faces of quercetin in neuroprotection. Expert Opin. Drug Saf. 8, 397–409. doi: 10.1517/14740330903026944
Ovais, M., Ahmad, I., Khalil, A. T., Mukherjee, S., Javed, R., Ayaz, M., et al. (2018a). Wound healing applications of biogenic colloidal silver and gold nanoparticles: recent trends and future prospects. Appl. Microbiol. Biotechnol. 102, 4305–4318. doi: 10.1007/s00253-018-8939-z
Ovais, M., Ayaz, M., Khalil, A. T., Shah, S. A., Jan, M. S., Raza, A., et al. (2018b). HPLC-DAD finger printing, antioxidant, cholinesterase, and α-glucosidase inhibitory potentials of a novel plant Olax nana. BMC Complement. Altern. Med. 18:1. doi: 10.1186/s12906-017-2057-9
Ovais, M., Khalil, A. T., Islam, N. U., Ahmad, I., Ayaz, M., Saravanan, M., et al. (2018c). Role of plant phytochemicals and microbial enzymes in biosynthesis of metallic nanoparticles. Appl. Microbiol. Biotechnol. doi: 10.1007/s00253-018-9146-7 [Epub ahead of print].
Ovais, M., Khalil, A. T., Raza, A., Islam, N. U., Ayaz, M., Saravanan, M., et al. (2018d). Multifunctional theranostic applications of biocompatible green-synthesized colloidal nanoparticles. Appl. Microbiol. Biotechnol. 102, 4393–4408. doi: 10.1007/s00253-018-8928-2
Ovais, M., Khalil, A. T., Raza, A., Khan, M. A., Ahmad, I., Islam, N. U., et al. (2016). Green synthesis of silver nanoparticles via plant extracts: beginning a new era in cancer theranostics. Nanomedicine 12, 3157–3177. doi: 10.2217/nnm-2016-0279
Ovais, M., Nadhman, A., Khalil, A. T., Raza, A., Khuda, F., Sohail, M. F., et al. (2017a). Biosynthesized colloidal silver and gold nanoparticles as emerging leishmanicidal agents: an insight. Nanomedicine 12, 2807–2819. doi: 10.2217/nnm-2017-0233
Ovais, M., Raza, A., Naz, S., Islam, N. U., Khalil, A. T., Ali, S., et al. (2017b). Current state and prospects of the phytosynthesized colloidal gold nanoparticles and their applications in cancer theranostics. Appl. Microbiol. Biotechnol. 101, 3551–3565. doi: 10.1007/s00253-017-8250-4
Palle, S., and Neerati, P. (2017). Quercetin nanoparticles attenuates scopolamine induced spatial memory deficits and pathological damages in rats. Bull. Fac. Pharm. Cairo Univ. 55, 101–106. doi: 10.1016/j.bfopcu.2016.10.004
Papadia, K., Giannou, A. D., Markoutsa, E., Bigot, C., Vanhoute, G., Mourtas, S., et al. (2017). Multifunctional LUV liposomes decorated for BBB and amyloid targeting-B. In vivo brain targeting potential in wild-type and APP/PS1 mice. Eur. J. Pharm. Sci. 102, 180–187. doi: 10.1016/j.ejps.2017.03.010
Pardeshi, C. V., and Belgamwar, V. S. (2013). Direct nose to brain drug delivery via integrated nerve pathways bypassing the blood–brain barrier: an excellent platform for brain targeting. Expert Opin. Drug Deliv. 10, 957–972. doi: 10.1517/17425247.2013.790887
Pérez-Severiano, F., Salvatierra-Sánchez, R., Rodrıguez-Pérez, M., Cuevas-Martınez, E. Y., Guevara, J., Limón, D., et al. (2004). S-Allylcysteine prevents amyloid-β peptide-induced oxidative stress in rat hippocampus and ameliorates learning deficits. Eur. J. Pharmacol. 489, 197–202. doi: 10.1016/j.ejphar.2004.03.001
Puerta, E., Suárez-Santiago, J. E., Santos-Magalhães, N. S., Ramirez, M. J., and Irache, J. M. (2017). Effect of the oral administration of nanoencapsulated quercetin on a mouse model of Alzheimer’s disease. Int. J. Pharm. 517, 50–57. doi: 10.1016/j.ijpharm.2016.11.061
Qi, F.-Y., Yang, L., Tian, Z., Zhao, M.-G., Liu, S.-B., and An, J.-Z. (2014). Neuroprotective effects of Asiaticoside. Neural Regen. Res. 9, 1275–1282. doi: 10.4103/1673-5374.137574
Rafii, M., Walsh, S., Little, J., Behan, K., Reynolds, B., Ward, C., et al. (2011). A phase II trial of huperzine A in mild to moderate Alzheimer disease. Neurology 76, 1389–1394. doi: 10.1212/WNL.0b013e318216eb7b
Rebai, O., Belkhir, M., Sanchez-Gomez, M. V., Matute, C., Fattouch, S., and Amri, M. (2017). Differential molecular targets for neuroprotective effect of chlorogenic acid and its related compounds against glutamate induced excitotoxicity and oxidative stress in rat cortical neurons. Neurochem. Res. 42, 3559–3572. doi: 10.1007/s11064-017-2403-9
Remya, C., Dileep, K., Variayr, E., and Sadasivan, C. (2016). An in silico guided identification of nAChR agonists from Withania somnifera. Front. Life Sci. 9, 201–213. 10.1080/21553769.2016.1207569 doi: 10.1080/21553769.2016.1207569
Rocha, S. (2013). Targeted drug delivery across the blood brain barrier in Alzheimer’s disease. Curr. Pharm. Des. 19, 6635–6646. doi: 10.2174/13816128113199990613
Rotman, M., Welling, M. M., Bunschoten, A., de Backer, M. E., Rip, J., Nabuurs, R. J., et al. (2015). Enhanced glutathione PEGylated liposomal brain delivery of an anti-amyloid single domain antibody fragment in a mouse model for Alzheimer’s disease. J. Control. Release 203, 40–50. doi: 10.1016/j.jconrel.2015.02.012
Rubio, J., Qiong, W., Liu, X., Jiang, Z., Dang, H., Chen, S.-L., et al. (2011). Aqueous extract of black maca (Lepidium meyenii) on memory impairment induced by ovariectomy in mice. Evid. Based Complement. Alternat. Med. 2011:253958. doi: 10.1093/ecam/nen063
Rui, W., Yan-Nan, L., Guang-Ji, W., Hai-Ping, H., Xiao-Lan, W., and Fang, Z. (2009). Neuroprotective effects and brain transport of ginsenoside Rg1. Chin. J. Nat. Med. 7, 315–320. doi: 10.3724/SP.J.1009.2008.00315
Sadiq, A., Zeb, A., Ullah, F., Ahmad, S., Ayaz, M., Rashid, U., et al. (2018). Chemical characterization, analgesic, antioxidant, and anticholinesterase potentials of essential oils from Isodon rugosus Wall. ex. Benth. Front. Pharmacol. 9:623. doi: 10.3389/fphar.2018.00623
Saharia, K., Kumar, R., Gupta, K., Mishra, S., and Subramaniam, J. R. (2016). A novel way of amelioration of amyloid beta induced toxicity in Caenorhabditis elegans. Ann. Neurosci. 23, 149–154. doi: 10.1159/000449180
Sahni, J. K., Doggui, S., Ali, J., Baboota, S., Dao, L., and Ramassamy, C. (2011). Neurotherapeutic applications of nanoparticles in Alzheimer’s disease. J. Control. Release 152, 208–231. doi: 10.1016/j.jconrel.2010.11.033
Salloway, S., Sperling, R., Fox, N. C., Blennow, K., Klunk, W., Raskind, M., et al. (2014). Two phase 3 trials of bapineuzumab in mild-to-moderate Alzheimer’s disease. N. Engl. J. Med. 370, 322–333. doi: 10.1056/NEJMoa1304839
Salmaso, S., and Caliceti, P. (2013). Stealth properties to improve therapeutic efficacy of drug nanocarriers. J. Drug Deliv. 2013:374252. doi: 10.1155/2013/374252
Serafini, M. M., Catanzaro, M., Rosini, M., Racchi, M., and Lanni, C. (2017). Curcumin in Alzheimer’s disease: Can we think to new strategies and perspectives for this molecule? Pharmacol. Res. 124, 146–155. doi: 10.1016/j.phrs.2017.08.004
Selkoe, D. J. (2001). Alzheimer’s disease: genes, proteins, and therapy. Physiol. Rev. 81, 741–766. doi: 10.1152/physrev.2001.81.2.741
Seow, S. L. S., Hong, S. L., Lee, G. S., Malek, S. N. A., and Sabaratnam, V. (2017). 6-shogaol, a neuroactive compound of ginger (jahe gajah) induced neuritogenic activity via NGF responsive pathways in PC-12 cells. BMC Complement. Altern. Med. 17:334. doi: 10.1186/s12906-017-1837-6
Sevigny, J., Chiao, P., Bussière, T., Weinreb, P. H., Williams, L., Maier, M., et al. (2016). The antibody aducanumab reduces Aβ plaques in Alzheimer’s disease. Nature 537, 50–56. doi: 10.1038/nature19323
Sharma, H. S., Drieu, K., Alm, P., and Westman, J. (2000). Role of nitric oxide in blood-brain barrier permeability, brain edema and cell damage following hyperthermic brain injury. An experimental study using EGB-761 and Gingkolide B pretreatment in the rat, in Brain Edema XI. Acta Neurochirurgica Supplements, ed. A. D. Mendelow (Vienna: Springer),81–86.
Shen, J., Zhao, Z., Shang, W., Liu, C., Zhang, B., Zhao, L., et al. (2017). Ginsenoside Rg1 nanoparticle penetrating the blood–brain barrier to improve the cerebral function of diabetic rats complicated with cerebral infarction. Int. J. Nanomedicine 12, 6477–6486. doi: 10.2147/IJN.S139602
Shi, C., Liu, J., Wu, F., and Yew, D. T. (2010). Ginkgo biloba extract in Alzheimer’s disease: from action mechanisms to medical practice. Int. J. Mol. Sci. 11, 107–123. doi: 10.3390/ijms11010107
Shinomol, G. K., and Bharath, M. M. (2011). Exploring the role of “Brahmi”(Bacopa monnieri and Centella asiatica) in brain function and therapy. Recent Pat. Endocr. Metab. Immune Drug Discov. 5, 33–49. doi: 10.2174/187221411794351833
Shu, X., and Zhu, K. (2000). A novel approach to prepare tripolyphosphate/chitosan complex beads for controlled release drug delivery. Int. J. Pharm. 201, 51–58. doi: 10.1016/S0378-5173(00)00403-8
Siemers, E. R., Sundell, K. L., Carlson, C., Case, M., Sethuraman, G., Liu-Seifert, H., et al. (2016). Phase 3 solanezumab trials: secondary outcomes in mild Alzheimer’s disease patients. Alzheimers Dement. 12, 110–120. doi: 10.1016/j.jalz.2015.06.1893
Smith, A., Giunta, B., Bickford, P. C., Fountain, M., Tan, J., and Shytle, R. D. (2010). Nanolipidic particles improve the bioavailability and α-secretase inducing ability of epigallocatechin-3-gallate (EGCG) for the treatment of Alzheimer’s disease. Int. J. Pharm. 389, 207–212. doi: 10.1016/j.ijpharm.2010.01.012
Song, J.-H., Lee, J.-W., Shim, B., Lee, C.-Y., Choi, S., Kang, C., et al. (2013). Glycyrrhizin alleviates neuroinflammation and memory deficit induced by systemic lipopolysaccharide treatment in mice. Molecules 18, 15788–15803. doi: 10.3390/molecules181215788
Songjiang, Z., and Lixiang, W. (2009). Amyloid-beta associated with chitosan nano-carrier has favorable immunogenicity and permeates the BBB. AAPS PharmSciTech 10, 900–905. doi: 10.1208/s12249-009-9279-1
Sperling, R. A., Karlawish, J., and Johnson, K. A. (2013). Preclinical Alzheimer disease—the challenges ahead. Nat. Rev. Neurol. 9, 54–58. doi: 10.1038/nrneurol.2012.241
Subash, S., Essa, M. M., Braidy, N., Al-Jabri, A., Vaishnav, R., Al-Adawi, S., et al. (2016). Consumption of fig fruits grown in Oman can improve memory, anxiety, and learning skills in a transgenic mice model of Alzheimer’s disease. Nutr. Neurosci. 19, 475–483. doi: 10.1179/1476830514Y.0000000131
Suganthy, N., Ramkumar, V. S., Pugazhendhi, A., Benelli, G., and Archunan, G. (2017). Biogenic synthesis of gold nanoparticles from Terminalia arjuna bark extract: assessment of safety aspects and neuroprotective potential via antioxidant, anticholinesterase, and antiamyloidogenic effects. Environ. Sci. Pollut. Res. 25, 10418–10433. doi: 10.1007/s11356-017-9789-4
Sun, D., Li, N., Zhang, W., Zhao, Z., Mou, Z., Huang, D., et al. (2016). Design of PLGA-functionalized quercetin nanoparticles for potential use in Alzheimer’s disease. Colloids Surf. B Biointerfaces 148, 116–129. doi: 10.1016/j.colsurfb.2016.08.052
Tabuchi, M., Imamura, S., Kawakami, Z., Ikarashi, Y., and Kase, Y. (2012). The blood–brain barrier permeability of 18β-glycyrrhetinic acid, a major metabolite of glycyrrhizin in Glycyrrhiza root, a constituent of the traditional Japanese medicine yokukansan. Cell. Mol. Neurobiol. 32, 1139–1146. doi: 10.1007/s10571-012-9839-x
Tanifum, E. A., Dasgupta, I., Srivastava, M., Bhavane, R. C., Sun, L., Berridge, J., et al. (2012). Intravenous delivery of targeted liposomes to amyloid-β pathology in APP/PSEN1 transgenic mice. PLoS One 7:e48515. doi: 10.1371/journal.pone.0048515
Tanifum, E. A., Ghaghada, K., Vollert, C., Head, E., Eriksen, J. L., and Annapragada, A. (2016). A novel liposomal nanoparticle for the imaging of amyloid plaque by magnetic resonance imaging. J. Alzheimers Dis. 52, 731–745. doi: 10.3233/JAD-151124
Ternchoocheep, K., Surangkul, D., and Ysothonsreekul, S. (2017). The recovery and protective effects of asiatic acid on differentiated human neuroblastoma SH-SY5Y cells cytotoxic-induced by cholesterol. Asian Pac. J. Trop. Biomed. 7, 416–420. doi: 10.1016/j.apjtb.2017.01.012
Tiwari, S. K., Agarwal, S., Seth, B., Yadav, A., Nair, S., Bhatnagar, P., et al. (2013). Curcumin-loaded nanoparticles potently induce adult neurogenesis and reverse cognitive deficits in Alzheimer’s disease model via canonical Wnt/β-catenin pathway. ACS Nano 8, 76–103. doi: 10.1021/nn405077y
Tsai, Y.-M., Chien, C.-F., Lin, L.-C., and Tsai, T.-H. (2011). Curcumin and its nano-formulation: the kinetics of tissue distribution and blood–brain barrier penetration. Int. J. Pharm. 416, 331–338. doi: 10.1016/j.ijpharm.2011.06.030
Ulbrich, K., Hekmatara, T., Herbert, E., and Kreuter, J. (2009). Transferrin-and transferrin-receptor-antibody-modified nanoparticles enable drug delivery across the blood–brain barrier (BBB). Eur. J. Pharm. Biopharm. 71, 251–256. doi: 10.1016/j.ejpb.2008.08.021
Ullah, F., Ayaz, M., Sadiq, A., Hussain, A., Ahmad, S., Imran, M., et al. (2016). Phenolic, flavonoid contents, anticholinesterase and antioxidant evaluation of Iris germanica var; florentina. Nat. Prod. Res. 30, 1440–1444. doi: 10.1080/14786419.2015.1057585
Vareed, S. K., Bauer, A. K., Nair, K. M., Liu, Y., Jayaprakasam, B., and Nair, M. G. (2014). Blood–brain barrier permeability of bioactive withanamides present in Withania somnifera fruit extract. Phytother. Res. 28, 1260–1264. doi: 10.1002/ptr.5118
Videira, R., Castanheira, P., Gradddos, M. R., Resende, R., Salgueiro, L., Faro, C., et al. (2014). Dose-dependent inhibition of BACE-1 by the monoterpenoid 2, 3, 4, 4-tetramethyl-5-methylenecyclopent-2-enone in cellular and mouse models of Alzheimer’s disease. J. Nat. Prod. 77, 1275–1279. doi: 10.1021/np400903w
Viola, K. L., Sbarboro, J., Sureka, R., De, M., Bicca, M. A., Wang, J., et al. (2015). Towards non-invasive diagnostic imaging of early-stage Alzheimer’s disease. Nat. Nanotechnol. 10, 91–98. doi: 10.1038/nnano.2014.254
Vitthal, K. U., Pillai, M., and Kininge, P. (2013). Study of solid lipid nanoparticles as a carrier for bacoside. Int. J. Pharma Biosci. 3, 414–426.
Wang, M., Li, Y., Ni, C., and Song, G. (2017). Honokiol attenuates oligomeric amyloid β1-42-induced Alzheimer’s disease in mice through attenuating mitochondrial apoptosis and inhibiting the nuclear factor kappa-B signaling pathway. Cell. Physiol. Biochem. 43, 69–81. doi: 10.1159/000480320
Wang, X., Chi, N., and Tang, X. (2008). Preparation of estradiol chitosan nanoparticles for improving nasal absorption and brain targeting. Eur. J. Pharm. Biopharm. 70, 735–740. doi: 10.1016/j.ejpb.2008.07.005
Wang, X., Duan, X., Yang, G., Zhang, X., Deng, L., Zheng, H., et al. (2011). Honokiol crosses BBB and BCSFB, and inhibits brain tumor growth in rat 9L intracerebral gliosarcoma model and human U251 xenograft glioma model. PLoS One 6:e18490. doi: 10.1371/journal.pone.0018490
Wang, Z. H., Wang, Z. Y., Sun, C. S., Wang, C. Y., Jiang, T. Y., and Wang, S. L. (2010). Trimethylated chitosan-conjugated PLGA nanoparticles for the delivery of drugs to the brain. Biomaterials 31, 908–915. doi: 10.1016/j.biomaterials.2009.09.104
Wilson, B., Samanta, M. K., Santhi, K., Kumar, K. P. S., Paramakrishnan, N., and Suresh, B. (2008). Poly (n-butylcyanoacrylate) nanoparticles coated with polysorbate 80 for the targeted delivery of rivastigmine into the brain to treat Alzheimer’s disease. Brain Res. 1200, 159–168. doi: 10.1016/j.brainres.2008.break01.039
Wilson, B., Samanta, M. K., Santhi, K., Kumar, K. S., Ramasamy, M., and Suresh, B. (2010). Chitosan nanoparticles as a new delivery system for the anti-Alzheimer drug tacrine. Nanomedicine 6, 144–152. doi: 10.1016/j.nano.2009.04.001
Winblad, B., and Jelic, V. (2004). Long-term treatment of Alzheimer disease: efficacy and safety of acetylcholinesterase inhibitors. Alzheimer Dis. Assoc. Disord. 18, S2–S8. doi: 10.1097/01.wad.0000127495.10774.a4
Wu, L., Zhang, Q.-L., Zhang, X.-Y., Lv, C., Li, J., Yuan, Y., et al. (2012). Pharmacokinetics and blood–brain barrier penetration of (+)-catechin and (-)-epicatechin in rats by microdialysis sampling coupled to high-performance liquid chromatography with chemiluminescence detection. J. Agric. Food Chem. 60, 9377–9383. doi: 10.1021/jf301787f
Xie, C.-L., Li, J.-H., Wang, W.-W., Zheng, G.-Q., and Wang, L.-X. (2015). Neuroprotective effect of ginsenoside-Rg1 on cerebral ischemia/reperfusion injury in rats by downregulating protease-activated receptor-1 expression. Life Sci. 121, 145–151. doi: 10.1016/j.lfs.2014.12.002
Xu, Z.-C., Wang, X.-B., Yu, W.-Y., Xie, S.-S., Li, S.-Y., and Kong, L.-Y. (2014). Design, synthesis and biological evaluation of benzylisoquinoline derivatives as multifunctional agents against Alzheimer’s disease. Bioorg. Med. Chem. Lett. 24, 2368–2373. doi: 10.1016/j.bmcl.2014.03.058
Yang, F., Lim, G. P., Begum, A. N., Ubeda, O. J., Simmons, M. R., Ambegaokar, S. S., et al. (2005). Curcumin inhibits formation of amyloid β oligomers and fibrils, binds plaques, and reduces amyloid in vivo. J. Biol. Chem. 280, 5892–5901. doi: 10.1074/jbc.M404751200
Yerer, M. B., Tiryaki, M. K., and Demirpolat, E. (2017). GSK-3beta inhibitory effects of 6-gingerol and 6-shogaol help to the recovery of SHSY-5Y cells after amyloid beta1–42 oligomer or aggregate toxicity. J. Cell. Biotechnol. 2, 145–157. doi: 10.3233/JCB-15035
Yiannopoulou, K. G., and Papageorgiou, S. G. (2013). Current and future treatments for Alzheimer’s disease. Ther. Adv. Neurol. Disord. 6, 19–33. doi: 10.1177/1756285612461679
Yin, Z., Yu, T., and Xu, Y. (2015). Preparation of amyloid immuno-nanoparticles as potential MRI contrast agents for Alzheimer’s disease diagnosis. J. Nanosci. Nanotechnol. 15, 6429–6434. doi: 10.1166/jnn.2015.11296
Yoo, D. Y., Nam, Y., Kim, W., Yoo, K.-Y., Park, J., Lee, C. H., et al. (2011). Effects of Ginkgo biloba extract on promotion of neurogenesis in the hippocampal dentate gyrus in C57BL/6 mice. J. Vet. Med. Sci. 73, 71–76. doi: 10.1292/jvms.10-0294
Yordanov, G. (2012). Poly (alkyl cyanoacrylate) nanoparticles as drug carriers: 33 years later. Bulg. J. Chem. 1, 61–72.
Zenaro, E., Pietronigro, E., Della Bianca, V., Piacentino, G., Marongiu, L., Budui, S., et al. (2015). Neutrophils promote Alzheimer’s disease–like pathology and cognitive decline via LFA-1 integrin. Nat. Med. 21, 880–886. doi: 10.1038/nm.3913
Zhang, C., Chen, J., Feng, C., Shao, X., Liu, Q., Zhang, Q., et al. (2014). Intranasal nanoparticles of basic fibroblast growth factor for brain delivery to treat Alzheimer’s disease. Int. J. Pharm. 461, 192–202. doi: 10.1016/j.ijpharm.2013.11.049
Zhang, J., Zhou, X., Yu, Q., Yang, L., Sun, D., Zhou, Y., et al. (2014). Epigallocatechin-3-gallate (EGCG)-stabilized selenium nanoparticles coated with Tet-1 peptide to reduce amyloid-β aggregation and cytotoxicity. ACS Appl. Mater. Interfaces 6, 8475–8487. doi: 10.1021/am501341u
Zhang, P., Liu, X., Zhu, Y., Chen, S., Zhou, D., and Wang, Y. (2013). Honokiol inhibits the inflammatory reaction during cerebral ischemia reperfusion by suppressing NF-κB activation and cytokine production of glial cells. Neurosci. Lett. 534, 123–127. doi: 10.1016/j.neulet.2012.11.052
Zhang, X., Fan, Z., and Jin, T. (2017). Crocin protects against cerebral-ischemia-induced damage in aged rats through maintaining the integrity of blood-brain barrier. Restor. Neurol. Neurosci. 35, 65–75. doi: 10.3233/RNN-160696
Zhang, Z. J., Cheang, L. C. V., Wang, M. W., and Lee, S. M.-Y. (2011). Quercetin exerts a neuroprotective effect through inhibition of the iNOS/NO system and pro-inflammation gene expression in PC12 cells and in zebrafish. Int. J. Mol. Med. 27, 195–203.
Zhao, J., Lu, S., Yu, H., Duan, S., and Zhao, J. (2018). Baicalin and ginsenoside Rb1 promote the proliferation and differentiation of neural stem cells in Alzheimer’s disease model rats. Brain Res. 1678, 187–194. doi: 10.1016/j.brainres.2017.10.003
Zheng, Y.-Q., Liu, J.-X., Wang, J.-N., and Xu, L. (2007). Effects of crocin on reperfusion-induced oxidative/nitrative injury to cerebral microvessels after global cerebral ischemia. Brain Res. 1138, 86–94. doi: 10.1016/j.brainres.2006.12.064
Zhou, Y., Li, H.-Q., Lu, L., Fu, D.-L., Liu, A.-J., Li, J.-H., et al. (2014). Ginsenoside Rg1 provides neuroprotection against blood brain barrier disruption and neurological injury in a rat model of cerebral ischemia/reperfusion through downregulation of aquaporin 4 expression. Phytomedicine 21, 998–1003. doi: 10.1016/j.phymed.2013.12.005
Zlokovic, B. V. (2011). Neurovascular pathways to neurodegeneration in Alzheimer’s disease and other disorders. Nat. Rev. Neurosci. 12, 723–738. doi: 10.1038/nrn3114
Zohra, T., Ovais, M., Khalil, A. T., Qasim, M., Ayaz, M., and Shinwari, Z. K. (2018). Extraction optimization, total phenolic, flavonoid contents, HPLC-DAD analysis and diverse pharmacological evaluations of Dysphania ambrosioides (L.) Mosyakin & Clemants. Nat. Prod. Res. doi: 10.1080/14786419.2018.1437428 [Epub ahead of print].
Keywords: Alzheimer’s disease, nanotechnology, medicinal plants, green synthesis, theranostic NPs
Citation: Ovais M, Zia N, Ahmad I, Khalil AT, Raza A, Ayaz M, Sadiq A, Ullah F and Shinwari ZK (2018) Phyto-Therapeutic and Nanomedicinal Approaches to Cure Alzheimer’s Disease: Present Status and Future Opportunities. Front. Aging Neurosci. 10:284. doi: 10.3389/fnagi.2018.00284
Received: 03 April 2018; Accepted: 30 August 2018;
Published: 23 October 2018.
Edited by:
Antonio Camins, University of Barcelona, SpainReviewed by:
Elena Sanchez López, University of Barcelona, SpainJordi Olloquequi, Universidad Autónoma de Chile, Chile
Copyright © 2018 Ovais, Zia, Ahmad, Khalil, Raza, Ayaz, Sadiq, Ullah and Shinwari. This is an open-access article distributed under the terms of the Creative Commons Attribution License (CC BY). The use, distribution or reproduction in other forums is permitted, provided the original author(s) and the copyright owner(s) are credited and that the original publication in this journal is cited, in accordance with accepted academic practice. No use, distribution or reproduction is permitted which does not comply with these terms.
*Correspondence: Muhammad Ovais, bW92YWlzQGJzLnFhdS5lZHUucGs= Muhammad Ayaz, YXlhenVvcEBnbWFpbC5jb20= orcid.org/0000-0002-4299-2445
†Present address: Muhammad Ovais, University of Chinese Academy of Sciences, Beijing, China