- 1Life and Health Sciences Research Institute, School of Medicine, University of Minho, Braga, Portugal
- 2ICVS/3B's, PT Government Associate Laboratory, Braga, Portugal
- 3Clinical Academic Center–Braga, Braga, Portugal
Sleep is a ubiquitous phenomenon, essential to the organism homeostasis. Notwithstanding, there has been an increasing concern with its disruption, not only within the context of pathological conditions, such as neurologic and psychiatric diseases, but also in health. In fact, sleep complaints are becoming particularly common, especially in middle-aged and older adults, which may suggest an underlying susceptibility to sleep quality loss and/or its consequences. Thus, a whole-brain modeling approach to study the shifts in the system can cast broader light on sleep quality mechanisms and its associated morbidities. Following this line, we sought to determine the association between the standard self-reported measure of sleep quality, the Pittsburgh Sleep Quality Index (PSQI) and brain correlates in a normative aging cohort. To this purpose, 86 participants (age range 52–87 years) provided information regarding sociodemographic parameters, subjective sleep quality and associated psychological variables. A multimodal magnetic resonance imaging (MRI) approach was used, with whole-brain functional and structural connectomes being derived from resting-state functional connectivity (FC) and probabilistic white matter tractography (structural connectivity, SC). Brain regional volumes and white matter properties associations were also explored. Results show that poor sleep quality was associated with a decrease in FC and SC of distinct networks, overlapping in right superior temporal pole, left middle temporal and left inferior occipital regions. Age displayed important associations with volumetric changes in the cerebellum cortex and white matter, thalamus, hippocampus, right putamen, left supramarginal and left lingual regions. Overall, results suggest that not only the PSQI global score may act as a proxy of changes in FC/SC in middle-aged and older individuals, but also that the age-related regional volumetric changes may be associated to an adjustment of brain connectivity. These findings may also represent a step further in the comprehension of the role of sleep disturbance in disease, since the networks found share regions that have been shown to be affected in pathologies, such as depression and Alzheimer's disease.
Introduction
Sleep is a recurring and reversible neurobehavioral state that involves reduced responsiveness to external stimuli and is frequently accompanied by postural recumbence and behavioral quiescence (Carskadon and Dement, 2011). Propensity to sleep is determined by the interaction of the circadian rhythm (“process C”), controlled by the suprachiasmatic nucleus, and a sleep homeostatic process (“process S”), that increasingly drives the need for sleep as a function of the time spent awake (Borbély, 1982; Borbély et al., 2016). For the individual to thrive and efficiently cope with the waking day demands, guidelines advise a human adult to sleep 7–9 h every day (Hirshkowitz et al., 2015) and to have sleep continuity parameters (i.e., sleep latency, awakenings >5 min, wake after sleep onset and sleep efficiency) within a specific range (see Ohayon et al., 2017 for details). In fact, having a “good sleep” ensures metabolic homeostasis, cerebral clearance, adequate immune function and overall good cognitive and mental status (Stickgold, 2006; Tononi and Cirelli, 2006; Cirelli and Tononi, 2008; Xie et al., 2013; Irwin, 2015; Freeman et al., 2017; Shokri-Kojori et al., 2018). However, factors, such as inappropriate exposure to light or food, lifestyle schedules, work, or psychological morbidity can interfere with the appropriate timing and duration of the sleep/wake cycle, leading to wide-range adverse effects on health (Schwartz et al., 1999; Stickgold, 2006; Wulff et al., 2010; Archer and Oster, 2015). More so, age also emerges as a critical modifier of sleep-wake patterns, being responsible for a shorter overall sleep duration and increase in sleep fragmentation and fragility, mostly after the fifth decade of age (Mander et al., 2017). In addition, in an increasingly older population (United Nations, Department of Economic and Social Affairs, Population Division, 2013), sleep dissatisfaction is one of the most common complaints in primary care (Aikens and Rouse, 2005) contributing not only for a growth vulnerability to disease, but also for a considerable economic burden, consequence of the costs of sleep aids and work absenteeism (Hillman et al., 2006). In addition, the dyad sleep-depression is of relevant weight since not only sleep problems may underlie an increased risk for the middle-aged or older individuals to be depressed (Almeida and Pfaff, 2005), but are also a robust predictor of incident depression (Livingston et al., 1993; Mallon et al., 2000).
In view of these associations and comorbidities, and because many neurological and psychiatric disorders share underlying brain network disturbances (Buckholtz and Meyer-Lindenberg, 2012; Uhlhaas and Singer, 2012; Deco and Kringelbach, 2014), it is vital to determine how one's sleep complaints and perceptions affect neural circuitries and overall biological systems. On this, the Pittsburgh Sleep Quality Index (PSQI) (Buysse et al., 1989; Landry et al., 2015), a standard subjective measure of overall sleep quality, may provide important and clinically relevant insights. In fact, magnetic resonance imaging (MRI) studies using this composite measure have revealed an association between poor subjective sleep quality and reduced volume within the right superior frontal cortex in cross-sectional analyses, as well as a widespread increased rate of atrophy in frontal, temporal and parietal regions in longitudinal analyses (Sexton et al., 2014). Poor sleep has also been associated with alterations in functional connectivity (FC) of resting state (e.g., default mode network) and attentional networks (Sämann et al., 2010; De Havas et al., 2012; Kaufmann et al., 2016; Scullin, 2017), amygdalar circuits (Shao et al., 2014), as well as in the dorsal nexus-dorsolateral prefrontal cortex connection (Bosch et al., 2013). However, different measures of sleep quality are considered in different studies and most of them tend to evaluate how specific networks behave under strict experimental protocols (e.g., sleep deprivation protocols) or in target populations (e.g., mostly young adults or individuals with sleep pathologies), thus providing a limited view of the occurring mechanisms and processes.
A whole-brain multimodal MRI approach can, therefore, provide new insights into general principles of brain function from health to disease (Deco and Kringelbach, 2014). Hence, in the present study, we used this approach to explore the association between subjective sleep quality (PSQI global score) and FC and structural connectivity (SC). PSQI global score will provide an overall value of the previous month sleep quality. Specifically, we hypothesized that this measure will provide an important overall view of sleep disruption parameters that may affect FC of a network with nodes in frontal, temporal, parietal and occipital regions during restful wake, given the described behavior of these regions during sleep and in sleep pathologies. Furthermore, because PSQI evaluates sleep quality over a 1-month period, we also hypothesized that it will be possible to observe the impact of poor sleep quality in SC.
Methods
Ethics Statement
The present study was conducted in accordance with the principles expressed in the Declaration of Helsinki (59th amendment) and was approved by the local and national ethics committees. All participants gave informed written consent after the study aims were explained.
Participants
The participants included in the present study are part of a cohort recruited for the SWITCHBOX Consortium project (www.switchbox-online.eu/). They were randomly selected from Guimarães and Vizela local area health authority registries as representative of the general middle-aged and older Portuguese population for age, gender and education (Costa et al., 2013; Santos et al., 2014). Primary exclusion criteria included inability to understand the informed consent, participant choice to withdraw from the study, incapacity and/or inability to attend the MRI session, dementia and/or diagnosed neuropsychiatric and/or neurodegenerative disorder (medical records). A sample of 120 individuals was invited to the follow-up assessment based on neuropsychological scores. Eighty-six individuals agreed to be re-evaluated (n = 17 declined to participate; n = 2 could not be reached; n = 6 could not be reassessed due to multiple schedules incompatibilities) and from these, 77 were able to perform an MRI scanning session. All MRI acquisitions were obtained during the afternoon to avoid morning and night circadian fluctuations.
Questionnaires
All participants were asked, in a structured interview format given participants' age and educational status, about the following information: sociodemographic; subjective sleep quality (Pittsburgh Sleep Quality Index, PSQI) (Buysse et al., 1989; Del Rio João et al., 2017); depressive symptoms (Geriatric Depression Scale, GDS) (Yesavage et al., 1982); sleepiness (Epworth Sleepiness Scale, ESS) (Johns, 1991; Santos, 2001). In the questionnaires used, the higher the score, the poorer the outcome. Furthermore, in the present study, PSQI global score was considered as a continuum.
Actigraphy
Actigraphy is a valuable tool in the study of sleep and circadian rhythms given its ability to measure 24 over 24-h activity (Ancoli-Israel et al., 2003; Landry et al., 2015). ActiSleep+ (Firmware 2.2.1; ActiGraph, LLC, Pensacola, Florida, USA) is a small (4.6 × 3.3 × 1.5 cm), electronic, light weight (19 grams), water proof, tri-axial wrist-worn device, that measures activity “counts” and was initialized at a sample rate of 30 Hz to record activities for free-living conditions. The obtained information was then downloaded using ActiLife 6 software (v 6.9.0; ActiGraph, LLC, Pensacola, FL, USA) and integrated into 60-s epochs for posterior analysis using Cole-Kripke algorithm (Cole et al., 1992). In the present study, 64 participants (of the 86 invited for the study) agreed to use the monitor in the non-dominant wrist for a 7-days period. For each participant, the mean of the 7 days for the following parameters were obtained: total time in bed, total sleep time, sleep latency, sleep efficiency, wake after sleep onset, number of awakenings and time of each awakening. Participants were instructed to never remove the monitor. However, in case of removal, they were instructed to register it in the sleep diary they had to fill for each of the 7 days. Similarities between the period of assessment using ActiSleep+ and the month assessed by PSQI was addressed by asking the participants for that information. For the analysis of the actigraphy information, data from 31 participants was admissible. Exclusion criteria included differences between the actigraphic week and the month assessed by PSQI (n = 8); < 6 days of actigraphic data (n = 3); sleep diaries not matching quality standards (e.g., not properly filled) (n = 22).
MRI Data Acquisition
The imaging session was performed at the Hospital of Braga (Braga, Portugal) on a clinical approved Siemens Magnetom Avanto 1.5 T MRI scanner (Siemens Medical Solutions, Erlangen, Germany), using a 12-channel receive-only head-coil. All acquisitions were performed between 2 and 6 p.m. to control for circadian fluctuations. The imaging protocol consisted of three types of acquisitions: structural, resting-state (rs) and diffusion weighted imaging (DWI). For the structural acquisition, a 3D T1-weighted magnetization prepared rapid gradient echo (MPRAGE) sequence was used. The used parameters were: 176 sagittal slices, TR/TE = 2,730/3.48 ms, flip angle = 7°, slice thickness = 1.0 mm, in-plane resolution = 1.0 × 1.0 mm2, FoV = 256 × 256 mm. For the rs-fMRI acquisition, a blood oxygen level dependent (BOLD) sensitive echo-planar imaging (EPI) sequence was used and the following parameters were used: 30 axial slices, TR = 2.0 s, TE = 30 ms, flip angle = 90°, slice thickness = 3.5 mm, slice gap = 0.48 mm, in-plane resolution = 3.5 × 3.5 mm2, FoV = 224 × 224 mm and 190 volumes. During this resting state scan, participants were instructed to be awake, with their eyes closed, as motionless as possible and they should try not to think of anything in particular and let the mind wander. We choose this approach because our population are middle-aged and older individuals that usually tend to feel very uncomfortable inside the MRI machine, thus moving a lot. Therefore, to reduce the discomfort and movement inside the MRI machine, we choose to ask the participants to stay awake with their eyes closed. In the end of this session, all participants confirmed that they had not fallen asleep. For the Diffusion Weighted Imaging (DWI) scan, a spin-echo echo-planar imaging (SE-EPI) sequence with the following parameters was used: TR = 8,800 ms, TE = 99 ms, FoV = 240 × 240 mm, acquisition matrix = 120 × 120, 61 slices, slice thickness = 2 mm, 30 non-collinear gradient directions with b = 1,000 s/mm2, one b = 0 s/mm2 acquisition and two as the total number of repetitions.
MRI Data Pre-processing
A certified neuro-radiologist visually inspected all acquisitions to confirm that they were not affected by critical head motion (certifying its quality) and that participants had no brain lesions/pathology.
The structural scans of each subject were segmented using FreeSurfer toolkit version 5.1 (http://surfer.nmr.mgh.harvard.edu), which implements a semi-automated segmentation workflow and allows the segmentation of Gray Matter (GM), White Matter (WM) and subcortical regions. For a complete description of the stages of processing implemented in this pipeline see Desikan, Destrieux, and Fischl works (Fischl et al., 2002, 2004; Desikan et al., 2006; Destrieux et al., 2010). Fischl and colleagues (Fischl et al., 2002) validated the procedures against manual segmentations, with robust results across sessions, scanner platforms, updates, and scanner field strengths (Jovicich et al., 2009). The quality of the segmentations was visually inspected and corrections conducted as indicated in Freesurfer guidelines. For the present study, values of intracranial volume (ICV), total gray matter volume (GMV), white matter hypointensities volume (WMSA) and regional brain volumes according to Desikan (Desikan et al., 2006) and Destrieux (Destrieux et al., 2010) segmentations were considered.
rs-fMRI data pre-processing was performed using FMRIB Software Library (FSL v5.07; http://fsl.fmrib.ox.ac.uk/fsl/; Smith et al., 2004) tools. The first five volumes of the rs-fMRI acquisition were removed to allow the habituation of the subjects to the EPI sequence noise and environment, as well as to avoid the spin history effects on the first volumes and the confounding effects over those (Friston et al., 1996). Furthermore, given that our population are middle-aged and older individuals, and likely extra sensitive to the MRI environment, we considered this step to be important. The remaining data was corrected for slice timing (for Siemens interleaved acquisition) followed by head motion correction using the mean image as reference. In order to reduce potential contamination of motion on functional connectivity, motion scrubbing (Power et al., 2012) was also performed, in order to identify and further exclude time-points where head motion could be critical. One subject was excluded for having more than 10 motion-contaminated time-points. Each subject functional dataset was then spatially normalized to the Montreal Neurological Institute (MNI) standard space through an indirect procedure that included: (i) skull stripping of the mean image of the functional acquisition and of the structural acquisition allowing the isolation of brain signal; (ii) rigid-body registration of the mean functional image to the skull stripped structural scan; (iii) affine registration of the structural scan to the MNI T1 template; (iv) non-linear registration of the structural scan to the MNI T1 template using the affine transformation previously estimated as the initial alignment; (v) non-linear transformation of the functional acquisition to MNI standard space trough the concatenation and application of the rigid-body transformation and the non-linear warp followed by resampling to 2 mm isotropic voxel size. Linear regression of motion parameters, mean WM and cerebrospinal fluid (CSF) (extracted using the FSL white matter and CSF tissue priors) signal and motion outliers was performed and the residuals of the regression were band-pass temporal filtered (0.01–0.08 Hz) and used for the subsequent analysis.
Pre-processing of diffusion data was performed using the FSL toolbox FDT (https://fsl.fmrib.ox.ac.uk/fsl/fslwiki/FDT; Behrens et al., 2003). The pre-processing consisted of: (i) eddy current and movement correction and matching rotation of the diffusion directions; (ii) isolation of brain signal by extraction of the skull; for the probabilistic tractography analysis this was followed by (iii) calculation of the non-linear normalization from MNI space to the subjects native space by concatenating an affine registration from structural to the diffusion space to the inverse of the previously described warp map from native structural to MNI space; (iv) the normalization was applied to the AAL atlas to bring all its ROIs to native diffusion space; (v) local modeling of the diffusion parameters was done using bedpostx which runs Markov Chain Monte Carlo sampling to build voxel wise distributions of the diffusion parameters; for the voxel wise diffusion parameters analysis (ii) was followed by (vi) the diffusion tensor was fitted to the data and the scalar maps were computed. This was achieved using DTIFIT that is part of the FDT Toolbox. DTIFIT fits a diffusion tensor model at each voxel and generates the scalar maps of fractional anisotropy (FA) and mean diffusivity (MD), and eigenvector and eigenvalue maps. Axial Diffusivity (AD) scalar map was defined as the principal diffusion eigenvalue (L1) and radial diffusivity (RD) as the mean of the second and third eigenvalues ((L2 + L3)/2).
MRI Data Analysis
A connectomics approach was used for resting state functional and diffusion structural connectivity analysis and the networks were built using the Anatomical Automatic Labeling (AAL) atlas. For the functional data analysis, the mean signal across time was extracted for each of the 116 ROIs and for each subject. Then, Pearson correlations between each possible pair of regions were computed and a symmetric adjacency matrix R, where each entry rij represents the Pearson correlation coefficient between the time series of region i and j, was obtained. These matrices were transformed into Z-score matrices by the application of Fisher's r-to-Z transformation to assure the normality of the correlation coefficients. To increase the statistical power of the analysis, the network-based statistic (NBS) procedure implemented in the NBS toolbox (https://sites.google.com/site/bctnet/comparison/nbs) was used (Zalesky et al., 2010). NBS evaluates the null hypothesis at the level of interconnected edges (i.e., subnetworks) surviving a predefined primary threshold (instead of considering the null hypothesis at the single edge level). The null hypothesis assumes that a sub-network with similar number of edges, surpassing the primary threshold, occurs by chance. It is recommended the use of different primary thresholds in order to capture different effects. In the present study, three different primary thresholds were used (P < 0.01, P < 0.005, P < 0.001) to capture less pronounced but more extent effects (less stringent primary threshold—P < 0.01) as well as localized and pronounced effects (most stringent threshold—P < 0.001). Five thousand permutations were performed and networks were considered significant at a network size corrected level of P < 0.05. To simplify visualization of the results, when similar networks were found across the threshold levels, we favor the presentation of the network surviving the higher threshold. BrainNet viewer (http://www.nitrc.org/projects/bnv/) was used for visualization purposes.
For the diffusion data probtrackx from the FDT toolbox (Behrens et al., 2007) was used to estimate the structural connectivity by calculating the number of streamlines connecting each pair of ROIs from the atlas, through sampling the principle directions previously calculated at each voxel. A total of 5,000 streamlines were attempted per-voxel. This resulted in a matrix representing the number of streamlines reaching from one ROI to the other. This matrix was normalized by first dividing each line by the number of voxels × number of streamlines and then the upper and lower triangles were averaged to give an undirected connectivity. To filter the connectivity matrix, keeping only connections significantly different from zero, a one-sample t-test was done at each connection. Only connections with a p lower than 0.01 were considered. To test the structural networks the same procedures for NBS described for functional connectivity were applied. Voxel-wise statistical analysis of scalar maps was performed using tract-based spatial statistics (TBSS) procedures (Smith et al., 2006), also implemented in FSL, following thresholding option TFCE (Threshold-Free Cluster Enhancement). For TBSS, first, the FA maps of each participant were slightly eroded and the end slices were zeroed, so that potential outliers from diffusion tensor fitting could be removed. Then, a non-linear registration was applied to align all FA images to a 1 × 1 × 1 mm standard space. In order to perform this, the FA image from each subject was non-linearly registered to each other in order to find the most representative one (i.e., the one that requires the least warping to align all images) that served as the study specific template. This template image was then affine transformed into Montreal Neurological Institute (MNI) 152 standard space and each FA map was transformed into standard space by combining the non-linear transformation to the FA target with the affine transformation into MNI space. Then, all FA images were averaged and the resulting image was skeletonized. The resultant skeleton image was thresholded at 0.3 so that skeleton regions including multiple tissue types could be removed. Finally, all scalar maps (FA, MD, AD, and RD) were projected onto the mean FA skeleton using the transformations applied to the FA images.
Statistical Analysis
Statistical analysis using SPSS version 22 (IBM, SPSS, Chicago, IL, USA) was used to determine the association between psychological, actigraphic, clinical and sociodemographic variables. The normality assumption for each variable was tested and non-parametric tests used when the assumption was not met. Bonferroni correction was used for multiple comparison testing and the significance level was set at p < 0.003.
For the statistical testing of FC and SC it were considered: a Network Based Statistics (NBS, https://sites.google.com/site/bctnet/comparison/nbs; Zalesky et al., 2010) approach, corrected for the size of the network, and a model that included GDS total score, PSQI global score, the interactions “PSQI × GDS,” “PSQI × Age,” and “PSQI × Sex” (as independent variables), age, sex, and years of education (as covariates). NBS tests the hypothesis in two stages: first, at each possible individual network connection by applying a user defined significance threshold; second, by identifying sub-networks composed of connections whose significance surpasses the threshold and determining its significance according to the network size. The sub-networks significance was calculated by comparing their sizes to the distribution of the size of sub-networks obtained through 5,000 random permutations of the original hypothesis. Because different thresholds can yield topologically different networks, three thresholds were tested at p < 0.01, p < 0.005, and p < 0.001. If the networks found at different thresholds are found to be equivalent, only the most significant one will be presented and discussed. The statistical analysis of the skeletonized maps of FA, MD, AD and RD was performed using permutation-based cross-subject statistics implemented in randomize, distributed with FSL. The model created was then used to test the main effect of PSQI variable and the PSQI by age, sex, years of formal education and GDS interactions. Ten thousand permutations were performed in the inference of each contrast of interest.
Volumetric data statistical analysis was performed ROI-wise using Matlab R2009b software (www.mathworks.com). A mix of Desikan (Desikan et al., 2006) and Destrieux (Destrieux et al., 2010) areas were considered for the subdivision of brain regions to be analyzed and a regression model considering sex, age, education, GDS total score, PSQI global score, ICV, the interactions “PSQI × Age” and “PSQI × sex” (independent variables) and the ROI volume of each area (dependent variable) was used. The choice of this regional brain areas was performed considering the most relevant areas regarding sleep that have been considered in different studies. White matter hypointensities and non-white matter hypointensities were also analyzed under this model. Bonferroni correction was used for multiple comparisons testing.
Results
Cohort Characterization
The study sample was composed by 46 males and 40 females, with a mean age of 67.40 (±8.155) years and a median of 4 (IQR = 3) years of education. More than half of the participants were married (77.9%), retired (75.6%) and presented a body mass index (BMI) above the considered normal range (93% with BMI above 25). 20% of the participants were on benzodiazepines use. See Table 1 for more details.
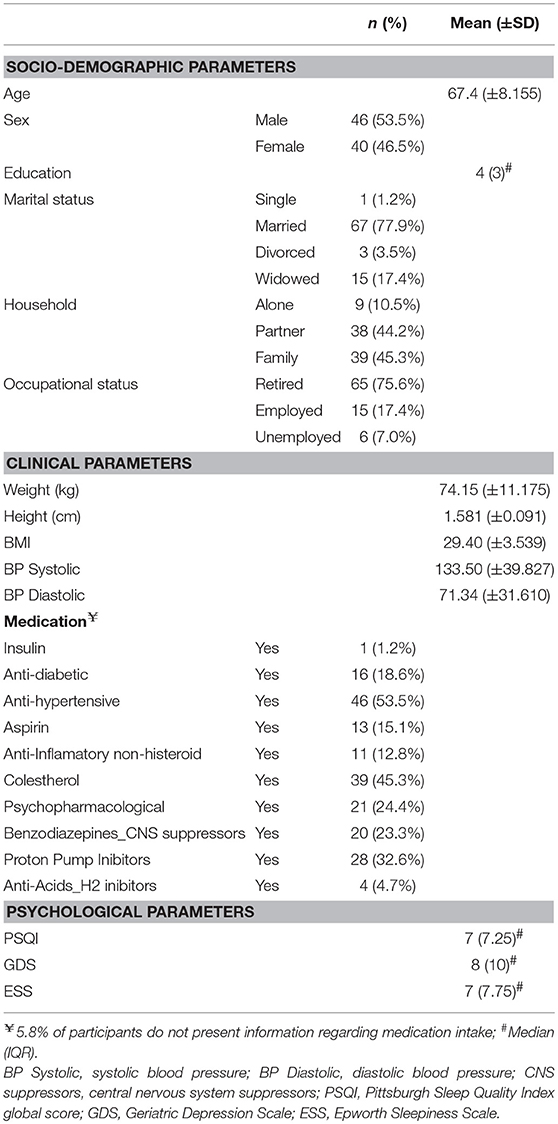
Table 1. Cohort characterization in terms of socio-demographic factors and clinical and psychological parameters.
Subjective Sleep Quality Associates With Actigraphic Parameters
Almost half (49%) of the participants had poor sleep quality (PSQI global score higher than 5). No statistically significant differences were found for PSQI global score and sex (U = 766.00, p = 0.180). No statistically significant association was found between PSQI global score and depressive symptomatology (GDS; rho = 0.279, p = 0.011) but depressive symptoms and PSQI subdomains “Day Dysfunction” (rho = 0.342, p = 0.002), “Sleep Disturbance” (rho = 0.377, p < 0.001), and “Medication” (rho = 0.421, p < 0.001) were found to be associated. Furthermore, education was negatively correlated with the frequency of sleep medication intake (rho = −0.337, p = 0.002) and with depressive symptoms (rho = −0.474, p < 0.001). Results are presented in detail in Table 2.
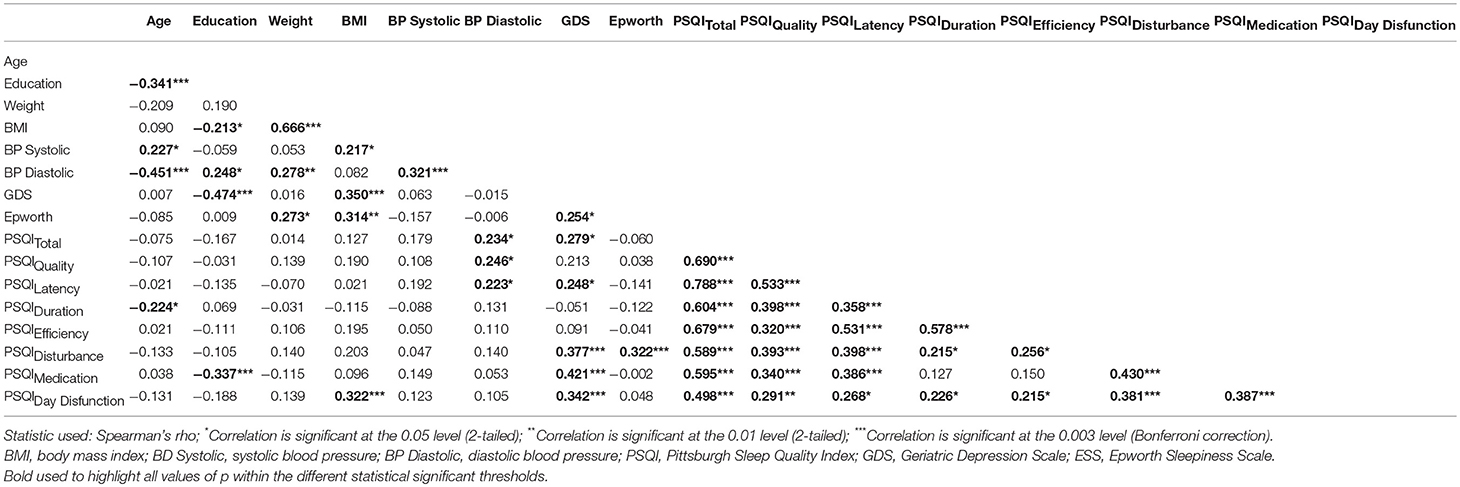
Table 2. Correlation between subjective sleep quality and demographic, clinical, and psychological parameters.
To complement subjective sleep quality information, we asked a random sub-sample of participants to wear an actigraphic device concomitantly to the fill of a sleep diary for a 7-days period. No differences were found regarding age, depressive symptomatology and subjective sleep quality between this sub-sample and the total cohort (Table 3). Actigraphy results revealed an association between the actigraphy-derived measures “Total Time in Bed” (TTB) and “Total Sleep Time” (TST) and the PSQI subdomain “Efficiency” (rhoTTB = 0.583, p = 0.001; rhoTST = 0.597, p < 0.001). Results also showed that sleepiness and the actigraphy-derived “Wake After Sleep Onset” were correlated (WASO, rho = 0.550, p = 0.002; Table 4).
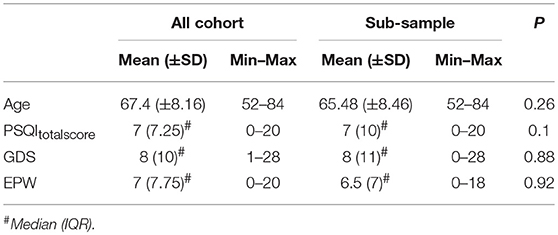
Table 3. Differences regarding age, PSQI global score, GDS, and ESS between the original cohort and the subsample that used actigraphy.
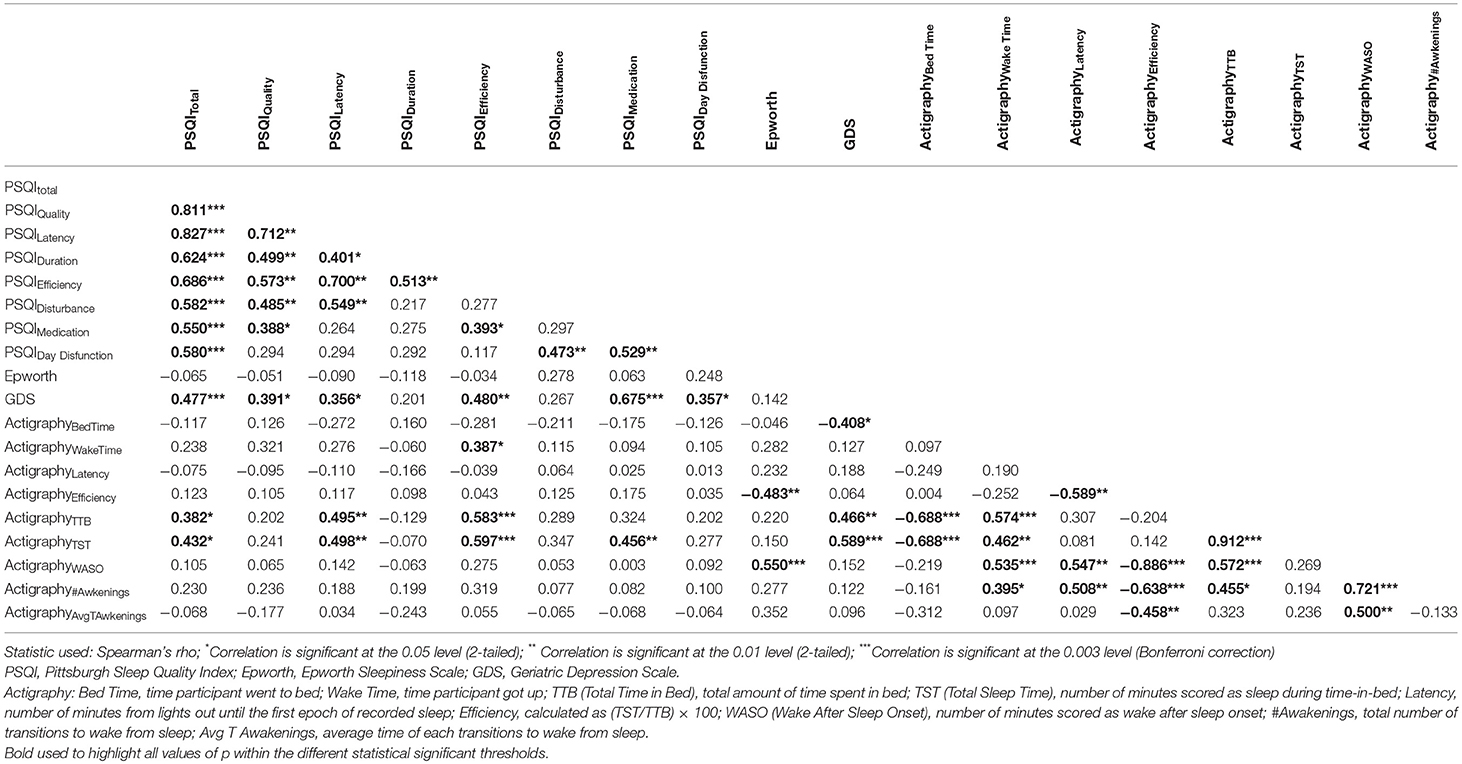
Table 4. Correlation between subjective sleep quality, sleepiness, depressive symptoms, and actigraphic parameters.
Brain Connectivity Is Associated With Subjective Sleep Quality
The association of subjective sleep quality with brain connectivity was then assessed using a whole-brain multimodal approach with a restrict connection threshold of p < 0.001 and correcting for the size of the network. Results revealed that individuals with poor sleep quality (i.e., higher PSQI global score) had decreased FC in a network with its principal nodes in bilateral inferior parietal regions (nodes 61 and 62) projecting to frontal (nodes 5, 9, and 15), temporal (nodes 81, 82, 84, and 85) and supramarginal (node 64) regions, as well as to the insula (node 30) and Rolandic Operculum (node 18) (network p = 0.034, 13 connections; Figure 1A; Table 5). A statistically significant negative association was also found between “Age × PSQI” interaction and FC of a network with its principal nodes in the left inferior occipital (node 53) and left inferior parietal regions (node 61), as well as with the precuneus (nodes 67 and 68) (network p = 0.0042, 13 connections; Figure 1B; Table 5). This network also comprised nodes in the right hippocampus (node 38), left middle temporal region (node 85), right inferior parietal region (node 62) and right rectus (node 28). Further analysis of “Age × PSQI” interaction revealed an inflection point at 67 years of age (Figure 1B), after which variations in PSQI global scores produced higher FC alterations when compared to the younger individuals of the cohort. In addition, the association between the amount of fibers connecting different brain areas and the PSQI global score revealed that the most altered connections involved left lingual (node 47), left caudate (node 71), right orbital medial frontal (node 26) and left rectus (node 27) nodes (network p = 0.0344; Figure 1C; Table 5). We next explored the structural connection patterns within the patterns of functional interactions. Data showed that there was no overlapping network of the SC and FC associated to subjective sleep quality, but only specific nodes, namely, the right superior temporal pole, left middle temporal and left inferior occipital regions (Figure 2). The left middle temporal region was an overlapping node for the three networks found.
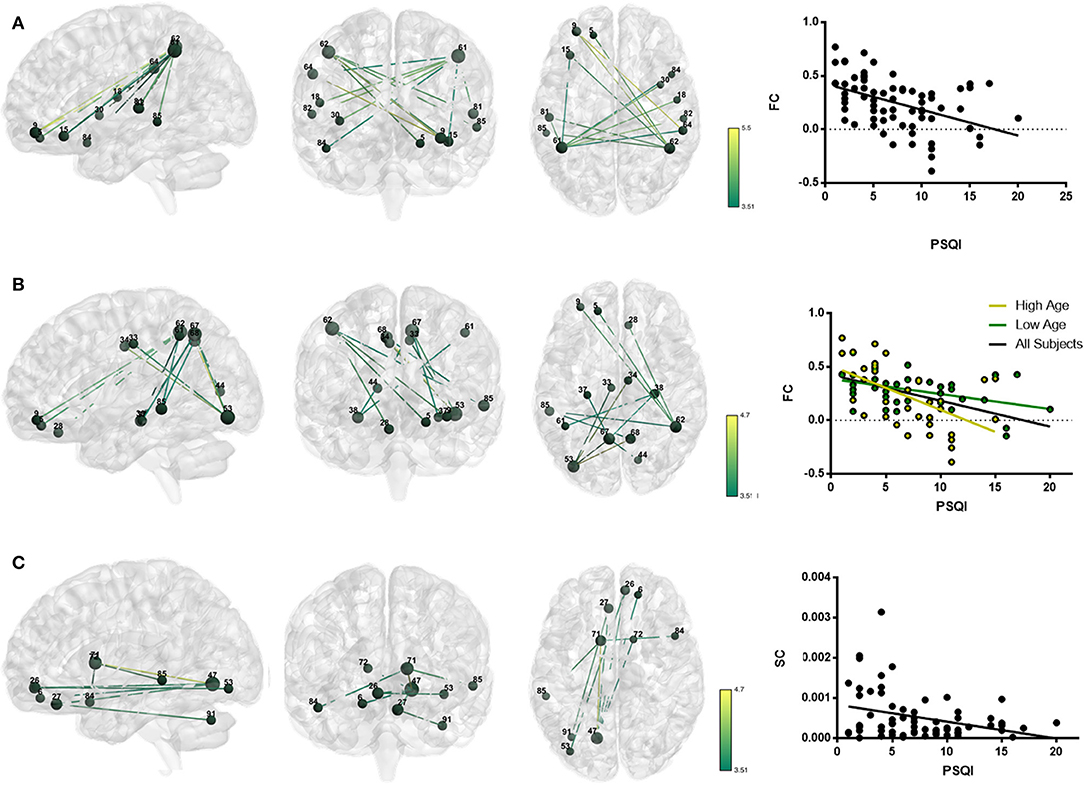
Figure 1. Whole-brain networks with altered functional and structural connectivity (FC and SC) in association with subjective sleep quality using p = 0.001 as primary threshold. Color scheme: higher connectivity from green to yellow; Nodes: Larger nodes, higher structural connectivity. (A) FC in association with PSQI (controlled for age, sex, and GDS); (B) FC in association with “Age × PSQI interaction” (controlled for sex and GDS); (C) SC in association with PSQI (controlled for age, sex and GDS). Numbers' legend: (5) Frontal_Sup_Orb_L; (6) Frontal_Sup_Orb_R; (9) Frontal_Mid_Orb_L; (15) Frontal_Inf_Orb_L; (18) Rolandic_Oper_R; (26) Frontal_Med_Orb_R; (27) Rectus_L; (28) Rectus_R; (30) Insula_R; (33) Cingulum_Mid_L; (34) Cingulum_Mid_R; (37) Hippocampus_L; (38) Hippocampus_R; (44) Calcarine_R; (47) Lingual_L; (53) Occipital_Inf_L; (61) Parietal_Inf_L; (62) Parietal_Inf_R; (64) SupraMarginal_R; (67) Precuneus_L; (68) Precuneus_R; (71) Caudate_L; (72) Caudate_R; (81) Temporal_Sup_L; (82) Temporal_Sup_R; (84) Temporal_Pole_Sup_R; (85) Temporal_Mid_L; (91) Cerebelum_Crus1_L.
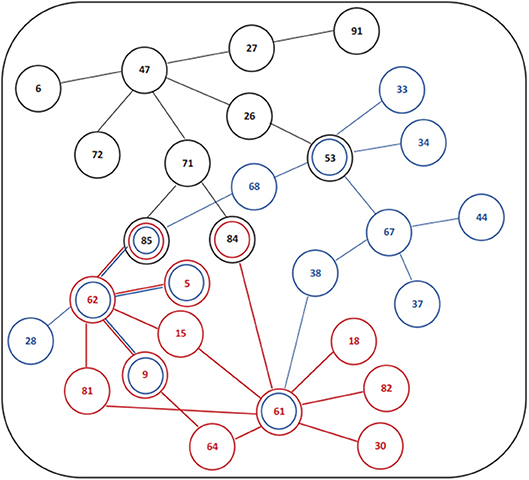
Figure 2. Schematic design or functional and structural connectivity in association with subjective sleep quality. (Red) Association PSQI and FC; (Blue) Association FC and “Age × PSQI” interaction; (Black) Association PSQI and SC. Number legends: (5) Frontal_Sup_Orb_L; (6) Frontal_Sup_Orb_R; (9) Frontal_Mid_Orb_L; (15) Frontal_Inf_Orb_L; (18) Rolandic_Oper_R; (26) Frontal_Med_Orb_R; (27) Rectus_L; (28) Rectus_R; (30) Insula_R; (33) Cingulum_Mid_L; (34) Cingulum_Mid_R; (37) Hippocampus_L; (38) Hippocampus_R; (44) Calcarine_R; (47) Lingual_L; (53) Occipital_Inf_L; (61) Parietal_Inf_L; (62) Parietal_Inf_R; (64) SupraMarginal_R; (67) Precuneus_L; (68) Precuneus_R; (71) Caudate_L; (72) Caudate_R; (81) Temporal_Sup_L; (82) Temporal_Sup_R; (84) Temporal_Pole_Sup_R; (85) Temporal_Mid_L; (91) Cerebelum_Crus1_L.
White Matter Properties and Subjective Sleep Quality
No statistically significant results were found regarding the association of white matter properties and sleep quality.
Volumetric Changes and Subjective Sleep Quality
Age and depressive symptomatology, but not PSQI global score, were significantly correlated with regional brain volumes (Table 6). Specifically, an increase in age was associated to a decrease in the volumes of cerebellum white matter (left: r2 = 0.742, p < 0.001; right: r2 = 0.674, p < 0.001), cerebellum cortex (left: r2 = 0.643; p = 0.01; right: r2 = 0.632, p = 0.013), thalamus (left: r2 = 0.531; p = 0.009; right: r2 = 0.543; p = 0.001), hippocampus (left: r2 = 0.463; p = 0.011; right: r2 = 0.421; p = 0.004), right putamen (r2 = 0.414; p = 0.033), left lingual (r2 = 0.341; p = 0.014) and left supramarginal (r2 = 0.472; p = 0.032). Furthermore, while increases in white matter hypointensities (r2 = 0.427; p = 0.003) were associated to increased age, a negative association is observed between the depressive symptomatology measured by GDS and lower left putamen volumes (r2 = 0.415; p = 0.046).
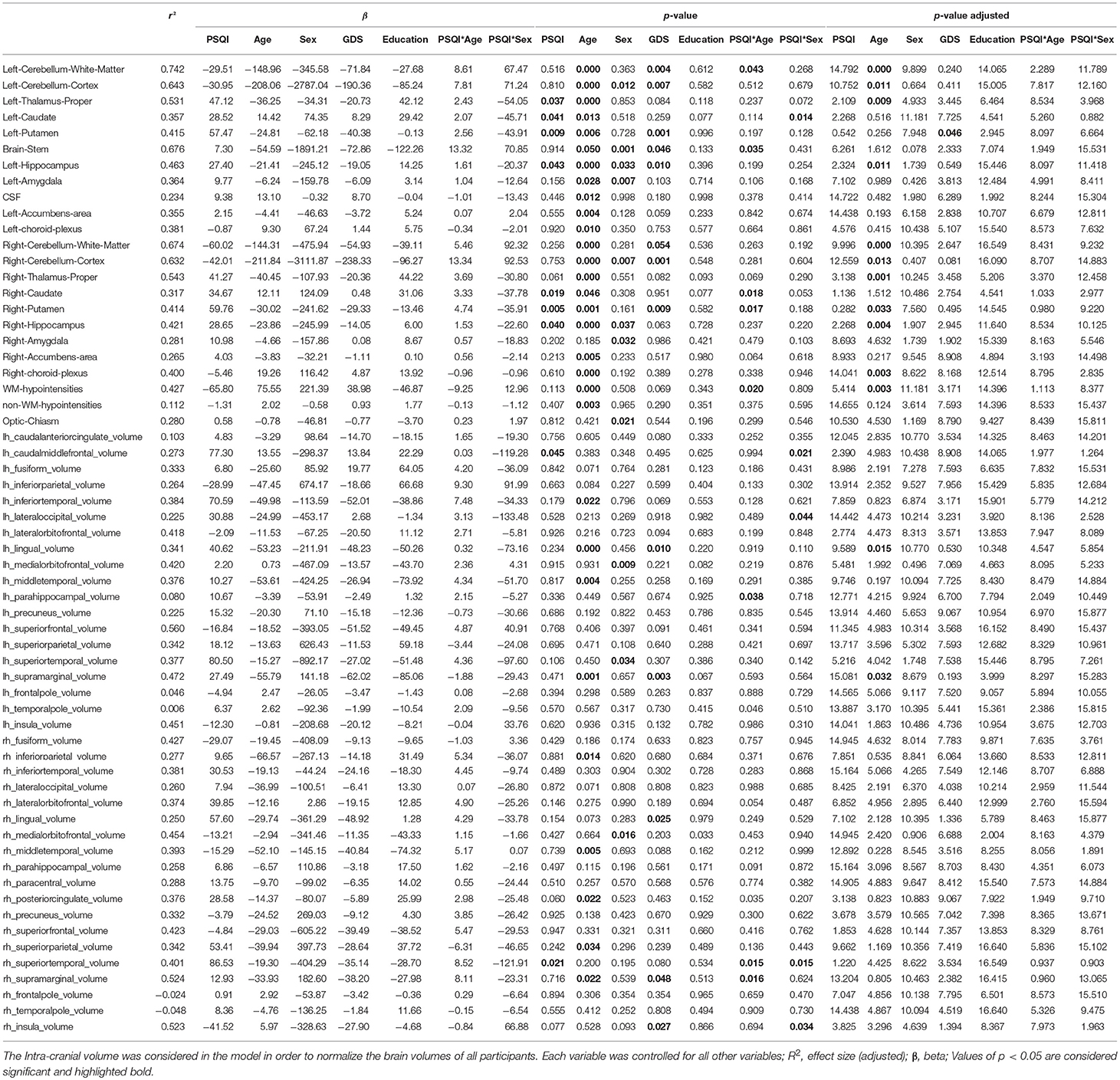
Table 6. Regional brain volumes association with age, sex, subjective sleep quality, and depressive symptoms.
Discussion
Herein, we applied a multimodal whole-brain MRI approach to determine, in a normative aging cohort, the association between subjective sleep quality measured by PSQI global score, and brain connectivity. Results showed that poor sleep quality was associated with decreased FC and SC of two distinct networks, with overlapping nodes in the right superior temporal pole and in the left middle temporal region. The obtained data also showed that for older individuals, smaller increases in PSQI global score are sufficient to decrease FC in a network that has some overlapping nodes with the above-mentioned SC and FC networks. The left middle temporal region was significantly affected in all the found networks. Overall, the results indicate that network connectivity is adjustable in face of subjective sleep quality alterations and that a 1-month measure of sleep quality allows the observation of SC changes. In addition, the impacted networks, which are relevant for language, environment perception and assessment and self-awareness, seem to also permit inferences about a subjects' quality of sleep.
Across aging a concomitant increase in sleep complaints and individuals' vulnerability to psychological distress and medical conditions occurs (Bliwise, 1993; Bliwise et al., 1993; Maggi et al., 1998; Foley et al., 2004; Stranges et al., 2012; Scullin and Bliwise, 2015; Mander et al., 2017). However, prevalent as they are, it is still debatable whether these sleep complaints are a product of age itself, a consequence of age-related medical and psychiatric conditions, or a mix of both (Mander et al., 2017). Here, 49% of the participants had poor sleep quality over the previous month and of those, 34% were on benzodiazepines use and 28% used other type of psychopharmacological medication. In line with this result is the found association between PSQI subdomains “Day Dysfunction,” “Sleep Disturbance,” and “Medication” and depressive symptoms. In addition, participants with lower education levels had higher scores not only in PSQI subdomain “Medication,” which was also found in previous studies (e.g., Sivertsen et al., 2015), but also in GDS (depressive symptoms). In fact, more attention should be given to the weight of socio-demographic factors, given its association to health vulnerabilities, such as access to care or comprehension of health information. This is also relevant because it supports the concern about participants' cognitive and mental status and its influence in their ability to properly recall information. Herein, to address this limitation, PSQI information was combined with actigraphic measurement. A possible bias was addressed by the confirmation that no differences existed between the initial cohort and the subsample that used the ActiSleep+ units. The association between PSQI global score, its subdomains and actigraphic parameters was also tested. Important correlations between PSQI subdomain “sleep efficiency” and actigraphic TTB and TST were observed, as well as between sleepiness and WASO (actigraphy derived). No association was found between PSQI global score and actigraphy, which we speculate to be due to the combination of the small sample size and the number of variables tested. This limitation should be addressed in future studies by increasing the number of participants wearing actigraphy units. Additionally, because changes in body composition (e.g., increase of body fat mass and decrease of muscle mass) are common in aging (St-Onge, 2005), we also determined the distribution of BMI in our cohort and its association with sleep quality. Results showed that 56% of participants were overweight and 37.2% obese. These numbers are in line with the EuroStat information (Database–Eurostat1) that shows a high prevalence of overweight individuals from middle to older age. However, despite common, high BMI promotes an increased vulnerability to chronic diseases, such as sleep apnea, diabetes or cardiovascular diseases, which can further affect sleep quality (St-Onge, 2005; Hoevenaar-Blom et al., 2011).
In our study, we were not able to perform polysomnography to exclude non-diagnosed sleep conditions; we just relied on clinical information from their clinical processes. It is, therefore, important to state this as a limitation of the study. However, given that the purpose was to characterize a normative aging cohort, and it is common to have underdiagnosed sleep disturbances in the general population, we did not consider that this limitation would invalidate our study or its results.
The aging process also carries alterations to the neuronal system. In the present cohort, age-related changes in regional brain volumes occurred in areas involving cognitive function, motor behavior and emotional processing, which goes in line with other studies (Bernard and Seidler, 2014; Ritchie et al., 2015). Of note, we found a volumetric decrease of the putamen, which can be related not only to the aging process but also to the presence of depressive symptoms. Interestingly, the decrease of the volume of the left putamen was significantly associated with global cognitive decline in older individuals with memory complaints and Alzheimer's disease, exceeding the strength of the left hippocampal correlation to cognitive performance (de Jong et al., 2008). This is particularly relevant because our results show the association between the volume of left putamen and depressive symptoms and, in a previous work from our team (Santos et al., 2013) these depressive symptoms were a determinant factor for poor cognitive performance. Furthermore, despite no associations between regional brain volumes and PSQI global score were observed, some of the age-affected areas are relevant nodes in the FC and SC networks that in our study were found to be associated with subjective sleep quality. Thus, it is plausible to consider that age-related changes in brain regional volumes may be modulating not only SC, but also the FC of the networks associated to sleep quality. While it is easier to correlate brain volumetric changes with SC, the same does not apply to FC, given its plasticity, flexibility, and reorganization capabilities (Bullmore and Sporns, 2009; van den Heuvel et al., 2009; Tewarie et al., 2014; Meier et al., 2016; Fjell et al., 2017). We speculate that the age-related changes in brain regional volumes can modulate the number of connections between regions, which, in turn, will enable changes in regions that are working synchronously. We also cogitate that these can be a bidirectional relation, which means that it is possible that, at this point, we are also seeing the cumulative effect of disrupted (poor) sleep throughout lifetime and how much resilience people still have to it. Previous studies exploring the association between subjective sleep quality and brain parameters have used a single functional imaging modality. Here, we address the question with a multimodal approach, thus providing for a more comprehensive view of the phenomenon and casting some light in the still highly debated relationship between structural and functional brain connectivity. By complementing FC and SC we provide not only a measure of synchrony between regions (FC), but also evidence regarding the fiber connections and their integrity state (SC). Our results showed that three different networks were impacted by subjective sleep quality—two affected only by PSQI and the other by the interaction “PSQI × Age”—and that the left middle temporal region was the only node overlapping the three. When reflecting about the meaning of this result, two recent studies have to be considered: one from Van Someren and colleagues, in which results showed that medial temporal lobe atrophy was strongly associated to sleep-wake rhythm fragmentation (Van Someren et al., 2018); and another from Lauriola and colleagues, showing that despite the association between sleep disruptions and subjective cognitive decline, there was no correlation between sleep changes and the medial temporal lobe (Lauriola et al., 2017). Beyond the obvious methodological differences that can justify what seems to be contradictory results between these studies, another argument arises: maybe sleep changes precede medial temporal lobe atrophy (Liguori et al., 2017). In our results, the middle temporal region also presents a decrease of volume with age, so, maybe we are not only observing the effect of general age-related adjustments, but also age-related adjustments concerning chronic or cumulative effects of intermittent sleep disruption throughout lifetime. Furthermore, this region has been suggested to contribute to our ability to understand action and non-dominant semantic association, allowing semantic retrieval to be shaped to suit a task or context (Davey et al., 2016). Having this in mind, and despite that the real value of the finding is still uncertain, we hypothesize that the left middle temporal region, when connected to specific nodes of each of the found networks, may be shaping our sense of self and our sense of the world, by selectively retrieving information relevant for our action and context. In fact, these changes are observed in individuals with depression, schizophrenia or Alzheimer's disease, possibly supporting the bidirectional link between sleep and these pathologies. Furthermore, this could partially explain why improving sleep may help managing or even improving depressive, schizophrenic or Alzheimer's disease symptoms (Greicius et al., 2004; Onitsuka et al., 2004; Veer et al., 2010; Li et al., 2016, 2018; Yun et al., 2017; Shokri-Kojori et al., 2018; Son et al., 2018). Thus, the potential of these results for clinical practice is of relevance, as it opens new perspectives to intervene timely in face of sleep disturbances.
On the association between subjective sleep quality and FC, our results, indicate that poor sleep quality is correlated with a decrease in the synchronized activity of a network with important nodes the inferior parietal regions and left orbital middle frontal region. The FC of these areas was also found to be altered in other studies using sleep deprivation protocols or addressing good sleepers against poor sleepers or insomniacs (Sämann et al., 2010; Chen et al., 2014; Dai et al., 2014; Nie et al., 2015; Yeo et al., 2015; Krause et al., 2017), suggesting that these regions may have the potential to be markers of sleep disturbances. For example, it has been described that insomniac patients present an overall cortical hyperarousal during sleep, which is thought to reflect persistent sensory processing and subsequent shallower sleep (Desseilles et al., 2008). As a consequence, during wakefulness, these individuals present a decreased metabolism in subcortical (thalamus, hypothalamus and brainstem reticular formation) as well as in cortical regions (bilateral prefrontal cortex, left superior temporal, parietal, and occipital cortices; Desseilles et al., 2008). Our normative aged community-dwellers, in a restful wake MRI condition, also had a decreased connectivity in some of these regions, supporting the idea of a possible continuum from health to disease in sleep. A connection involving the inferior parietal region and the insula was also observed. The relevance of this finding is in the fact that the insula has a role in temporal and bodily states (Chen et al., 2014) integration, which in arousal networks may underlie the misperception of sleep quality and subjective distress in insomnia (Chen et al., 2014). In addition, the consistently impaired inferior parietal region has led to the hypothesis that this region may also be used as an early marker for the effects of 24-h sleep deprivation, serving as an indicator of unexplored behavioral impairments (De Havas et al., 2012). Some of the nodes of the networks associated to the PSQI global score are also relevant nodes for known networks, such as the default mode network, the attentional network and networks involved in reward, stress and social interaction. The structural connection patterns within the patterns of dynamic (“functional”) interactions (Sporns, 2003) showed that subjective sleep quality was also correlated to alterations in the SC of a network with nodes in the left lingual region, left caudate, left rectus and right medial orbitofrontal regions. These regions have also been previously described to be implicated in sleep disturbances (Kay et al., 2016; Kay and Buysse, 2017). Remarkably, in all the results that we had none seem to suggest that compensatory mechanisms exist in what concerns to poor sleep quality.
There are, however, some limitations that should be addressed, namely the ones imposed by the study design and sample size. While we have established novel associations between subjective sleep and brain properties, the cause-effect relation is still uncertain at this point. A longitudinal approach should be considered in further work. A larger sample size is also of need in order to incorporate other relevant variables that influence the sleep process, such as presence of relevant pathologies and use of medication and to promote a trait stratification analysis. The blood oxygenation level-dependent (BOLD) signal in functional MRI has been an increasingly used tool, but because it depends on hemodynamic parameters, like blood pressure, it is sensitive to medical conditions affecting the cardiovascular system. In our results, a weak but statistically significant association between diastolic blood pressure and PSQI global score was found. Since poor sleep quality is associated with increased vulnerability to cardiovascular diseases (Lao et al., 2018), we explored in our statistical model, whether diastolic blood pressure could be acting as a confounding effect. The results remained the same: the same networks were found and they were all statistically significant. On the other hand, the methodology employed in this study provides a novel perspective on the biology of sleep quality under normative conditions. Not only we considered a standard measure of sleep quality already in use in the clinical practice, but we also imaged whole-brain changes in MRI, thus allowing pinpointing the most relevant changes in the complex and dynamic brain networks. Moreover, by using a normative aging cohort, we managed to capture the associations of sleep quality with biological processes under daily-life conditions, which could provide a much more realistic view and understanding of sleep with all of its environmental interactions and thus, be of more utility in the design of possible future clinical interventions.
To sum up, the present study shows that middle-aged and older individuals in their normal aging process, display alterations in brain FC and SC of complex brain networks in association with subjective sleep complaints. This may be of relevance in the future, not only to design interventions that are effective in improving sleep quality but also to delineate studies that allow a better understanding of the mechanisms involving subjective sleep quality and its associated comorbidities.
Author Contributions
NS and NCS conceived the study and LA contributed to the study design. LA, TC, and CP-N performed participants' recruitment. LA and TC performed the psychological assessments. RM, PSM, and PM performed the MRI acquisitions and did the MRI data pre-processing. LA, RM, and AC perform the data analysis. LA wrote the first draft of the manuscript and all authors contributed for the following and final versions of the manuscript.
Funding
Financial support was provided by FEDER funds through the Operational Programme Competitiveness Factors-COMPETE and National Funds through FCT-Foundation for Science and Technology under the project POCI-01-0145-FEDER-007038, by the project NORTE-01-0145-FEDER-000013 [supported by the Northern Portugal Regional Operational Programme (NORTE 2020), under the Portugal 2020 (P2020) Partnership Agreement, through the European Regional Development Fund (FEDER)], by POCI-01-0145-FEDER-016428 [supported by the Operational Programme Competitiveness and Internationalization (COMPETE 2020) and the Regional Operational Program of Lisbon and National Funding through Portuguese Foundation for Science and Technology (FCT, Portugal)], and by the Portuguese North Regional Operational Programme [ON.2 – O Novo Norte, under the National Strategic Reference Framework (QREN), through FEDER]. The work was also developed under the scope of the projects SwitchBox (European Commission, FP7; contract HEALTH-F2-2010-259772) and TEMPO-Better mental health during aging based on temporal prediction of individual brain aging trajectories (Fundação Calouste Gulbenkian; Contract grant number P-139977). LA, TC, RM, PSM, and CP-N were supported by FCT PhD scholarships [SFRH/BD/101398/2014 to LA; SFRH/BD/90078/2012 to TC; PDE/BDE/113604/2015 from the PhD-iHES Programme to RM; PDE/BDE/113601/2015 to PSM; PD/BD/106050/2015 from the Inter-University Doctoral Programme in Aging and Chronic Disease (PhDOC) to CP-N] and AC by a scholarship from the project NORTE-08-5639-FSE-000041 (NORTE 2020; UMINHO/BD/51/2017). NCS was a recipient of a Research Assistantship by the through the FCT Investigator Programme 200∞ Ciência.
Conflict of Interest Statement
The authors declare that the research was conducted in the absence of any commercial or financial relationships that could be construed as a potential conflict of interest.
Acknowledgments
We would also like to thank Miguel Pais-Vieira for the attentive reading and useful insight on the manuscript.
Footnotes
1. ^Database–Eurostat. Available online at: http://ec.europa.eu/eurostat/web/health/health-status-determinants/data/database?p_p_id=NavTreeportletprod_WAR_NavTreeportletprod_INSTANCE_8D6M1FwWY36d&p_p_lifecycle=0&p_p_state=normal&p_p_mode=view&p_p_col_id=column-2&p_p_col_count=2 (Accessed January 6, 2018).
References
Aikens, J. E., and Rouse, M. E. (2005). Help-seeking for insomnia among adult patients in primary care. J. Am. Board Fam. Pract. 18, 257–261. doi: 10.3122/jabfm.18.4.257
Almeida, O. P., and Pfaff, J. J. (2005). Sleep complaints among older general practice patients: association with depression. Br. J. Gen. Pract. 55, 864–866.
Ancoli-Israel, S., Cole, R., Alessi, C., Chambers, M., Moorcroft, W., and Pollak, C. P. (2003). The role of actigraphy in the study of sleep and circadian rhythms. Sleep 26, 342–392. doi: 10.1093/sleep/26.3.342
Archer, S. N., and Oster, H. (2015). How sleep and wakefulness influence circadian rhythmicity: effects of insufficient and mistimed sleep on the animal and human transcriptome. J. Sleep Res. 24, 476–493. doi: 10.1111/jsr.12307
Behrens, T. E. J., Berg, H. J., Jbabdi, S., Rushworth, M. F. S., and Woolrich, M. W. (2007). Probabilistic diffusion tractography with multiple fibre orientations: what can we gain? Neuroimage 34, 144–155. doi: 10.1016/j.neuroimage.2006.09.018
Behrens, T. E. J., Woolrich, M. W., Jenkinson, M., Johansen-Berg, H., Nunes, R. G., Clare, S., et al. (2003). Characterization and propagation of uncertainty in diffusion-weighted MR imaging. Magn. Reson. Med. 50, 1077–1088. doi: 10.1002/mrm.10609
Bernard, J. A., and Seidler, R. D. (2014). Moving forward: age effects on the cerebellum underlie cognitive and motor declines. Neurosci. Biobehav. Rev. 42, 193–207. doi: 10.1016/j.neubiorev.2014.02.011
Bliwise, D. L., Friedman, L., and Yesavage, J. A. (1993). Depression as a confounding variable in the estimation of habitual sleep time. J. Clin. Psychol. 49, 471–477. doi: 10.1002/1097-4679(199307)49:4<471::AID-JCLP2270490403>3.0.CO;2-7
Borbély, A. A., Daan, S., Wirz-Justice, A., and Deboer, T. (2016). The two-process model of sleep regulation: a reappraisal. J. Sleep Res. 25, 131–143. doi: 10.1111/jsr.12371
Bosch, O. G., Rihm, J. S., Scheidegger, M., Landolt, H.-P., Stämpfli, P., Brakowski, J., et al. (2013). Sleep deprivation increases dorsal nexus connectivity to the dorsolateral prefrontal cortex in humans. Proc. Natl. Acad. Sci. U.S.A. 110, 19597–19602. doi: 10.1073/pnas.1317010110
Buckholtz, J. W., and Meyer-Lindenberg, A. (2012). Psychopathology and the human connectome: toward a transdiagnostic model of risk for mental illness. Neuron 74, 990–1004. doi: 10.1016/j.neuron.2012.06.002
Bullmore, E., and Sporns, O. (2009). Complex brain networks: graph theoretical analysis of structural and functional systems. Nat. Rev. Neurosci. 10, 186–198. doi: 10.1038/nrn2575
Buysse, D. J., Reynolds, C. F., Monk, T. H., Berman, S. R., and Kupfer, D. J. (1989). The Pittsburgh sleep quality index: a new instrument for psychiatric practice and research. Psychiatry Res. 28, 193–213. doi: 10.1016/0165-1781(89)90047-4
Carskadon, M. A., and Dement, W. C. (2011). “Chapter 2–normal human sleep: an overview,” in Principles and Practice of Sleep Medicine, 5th Edn., eds. M. H. Kryger, T. Roth, and W. C. Dement (Philadelphia, PA: W.B. Saunders), 16–26.
Chen, M. C., Chang, C., Glover, G. H., and Gotlib, I. H. (2014). Increased insula coactivation with salience networks in insomnia. Biol. Psychol. 97, 1–8. doi: 10.1016/j.biopsycho.2013.12.016
Cirelli, C., and Tononi, G. (2008). Is sleep essential? PLoS Biol. 6:e216. doi: 10.1371/journal.pbio.0060216
Cole, R. J., Kripke, D. F., Gruen, W., Mullaney, D. J., and Gillin, J. C. (1992). Automatic sleep/wake identification from wrist activity. Sleep 15, 461–469. doi: 10.1093/sleep/15.5.461
Costa, P. S., Santos, N. C., Cunha, P., Palha, J. A., and Sousa, N. (2013). The use of bayesian latent class cluster models to classify patterns of cognitive performance in healthy ageing. PLoS ONE 8:e71940. doi: 10.1371/journal.pone.0071940
Dai, X.-J., Peng, D.-C., Gong, H.-H., Wan, A.-L., Nie, X., Li, H.-J., et al. (2014). Altered intrinsic regional brain spontaneous activity and subjective sleep quality in patients with chronic primary insomnia: a resting-state fMRI study. Neuropsychiatr. Dis. Treat. 10, 2163–2175. doi: 10.2147/NDT.S69681
Davey, J., Thompson, H. E., Hallam, G., Karapanagiotidis, T., Murphy, C., De Caso, I., et al. (2016). Exploring the role of the posterior middle temporal gyrus in semantic cognition: integration of anterior temporal lobe with executive processes. Neuroimage 137, 165–177. doi: 10.1016/j.neuroimage.2016.05.051
De Havas, J. A., Parimal, S., Soon, C. S., and Chee, M. W. L. (2012). Sleep deprivation reduces default mode network connectivity and anti-correlation during rest and task performance. Neuroimage 59, 1745–1751. doi: 10.1016/j.neuroimage.2011.08.026
de Jong, L. W., van der Hiele, K., Veer, I. M., Houwing, J. J., Westendorp, R. G. J., Bollen, E. L. E. M., et al. (2008). Strongly reduced volumes of putamen and thalamus in Alzheimer's disease: an MRI study. Brain 131, 3277–3285. doi: 10.1093/brain/awn278
Deco, G., and Kringelbach, M. L. (2014). Great expectations: using whole-brain computational connectomics for understanding neuropsychiatric disorders. Neuron 84, 892–905. doi: 10.1016/j.neuron.2014.08.034
Del Rio João, K. A., Becker, N. B., de Neves Jesus, S., and Isabel Santos Martins, R. (2017). Validation of the portuguese version of the pittsburgh sleep quality index (PSQI-PT). Psychiatry Res. 247, 225–229. doi: 10.1016/j.psychres.2016.11.042
Desikan, R. S., Ségonne, F., Fischl, B., Quinn, B. T., Dickerson, B. C., Blacker, D., et al. (2006). An automated labeling system for subdividing the human cerebral cortex on MRI scans into gyral based regions of interest. Neuroimage 31, 968–980. doi: 10.1016/j.neuroimage.2006.01.021
Desseilles, M., Dang-Vu, T., Schabus, M., Sterpenich, V., Maquet, P., and Schwartz, S. (2008). Neuroimaging insights into the pathophysiology of sleep disorders. Sleep 31, 777–794. doi: 10.1093/sleep/31.6.777
Destrieux, C., Fischl, B., Dale, A., and Halgren, E. (2010). Automatic parcellation of human cortical gyri and sulci using standard anatomical nomenclature. Neuroimage 53, 1–15. doi: 10.1016/j.neuroimage.2010.06.010
Fischl, B., Salat, D. H., Busa, E., Albert, M., Dieterich, M., Haselgrove, C., et al. (2002). Whole brain segmentation: automated labeling of neuroanatomical structures in the human brain. Neuron 33, 341–355. doi: 10.1016/S0896-6273(02)00569-X
Fischl, B., Salat, D. H., van der Kouwe, A. J. W., Makris, N., Ségonne, F., Quinn, B. T., et al. (2004). Sequence-independent segmentation of magnetic resonance images. Neuroimage 23(Suppl. 1), S69–S84. doi: 10.1016/j.neuroimage.2004.07.016
Fjell, A. M., Sneve, M. H., Grydeland, H., Storsve, A. B., Amlien, I. K., Yendiki, A., et al. (2017). Relationship between structural and functional connectivity change across the adult lifespan: a longitudinal investigation. Hum. Brain Mapp. 38, 561–573. doi: 10.1002/hbm.23403
Foley, D., Ancoli-Israel, S., Britz, P., and Walsh, J. (2004). Sleep disturbances and chronic disease in older adults: results of the 2003 National Sleep Foundation Sleep in America Survey. J. Psychosom. Res. 56, 497–502. doi: 10.1016/j.jpsychores.2004.02.010
Freeman, D., Sheaves, B., Goodwin, G. M., Yu, L.-M., Nickless, A., Harrison, P. J., et al. (2017). The effects of improving sleep on mental health (OASIS): a randomised controlled trial with mediation analysis. Lancet Psychiatry 4, 749–758. doi: 10.1016/S2215-0366(17)30328-0
Friston, K. J., Williams, S., Howard, R., Frackowiak, R. S., and Turner, R. (1996). Movement-related effects in fMRI time-series. Magn. Reson. Med. 35, 346–355. doi: 10.1002/mrm.1910350312
Greicius, M. D., Srivastava, G., Reiss, A. L., and Menon, V. (2004). Default-mode network activity distinguishes Alzheimer's disease from healthy aging: evidence from functional MRI. Proc. Natl. Acad. Sci. U.S.A. 101, 4637–4642. doi: 10.1073/pnas.0308627101
Hillman, D. R., Murphy, A. S., and Pezzullo, L. (2006). The economic cost of sleep disorders. Sleep 29, 299–305. doi: 10.1093/sleep/29.3.299
Hirshkowitz, M., Whiton, K., Albert, S. M., Alessi, C., Bruni, O., DonCarlos, L., et al. (2015). National Sleep Foundation's sleep time duration recommendations: methodology and results summary. Sleep Health J. Natl. Sleep Found. 1, 40–43. doi: 10.1016/j.sleh.2014.12.010
Hoevenaar-Blom, M. P., Spijkerman, A. M. W., Kromhout, D., van den Berg, J. F., and Verschuren, W. M. M. (2011). Sleep duration and sleep quality in relation to 12-year cardiovascular disease incidence: the MORGEN study. Sleep 34, 1487–1492. doi: 10.5665/sleep.1382
Irwin, M. R. (2015). Why sleep is important for health: a psychoneuroimmunology perspective. Annu. Rev. Psychol. 66, 143–172. doi: 10.1146/annurev-psych-010213-115205
Johns, M. W. (1991). A new method for measuring daytime sleepiness: the Epworth sleepiness scale. Sleep 14, 540–545. doi: 10.1093/sleep/14.6.540
Jovicich, J., Czanner, S., Han, X., Salat, D., van der Kouwe, A., Quinn, B., et al. (2009). MRI-derived measurements of human subcortical, ventricular and intracranial brain volumes: reliability effects of scan sessions, acquisition sequences, data analyses, scanner upgrade, scanner vendors and field strengths. Neuroimage 46, 177–192. doi: 10.1016/j.neuroimage.2009.02.010
Kaufmann, T., Elvsåshagen, T., Alnæs, D., Zak, N., Pedersen, P. Ø., Norbom, L. B., et al. (2016). The brain functional connectome is robustly altered by lack of sleep. Neuroimage 127, 324–332. doi: 10.1016/j.neuroimage.2015.12.028
Kay, D. B., and Buysse, D. J. (2017). Hyperarousal and beyond: new insights to the pathophysiology of insomnia disorder through functional neuroimaging studies. Brain Sci. 7:E23. doi: 10.3390/brainsci7030023
Kay, D. B., Karim, H. T., Soehner, A. M., Hasler, B. P., Wilckens, K. A., James, J. A., et al. (2016). Sleep-wake differences in relative regional cerebral metabolic rate for glucose among patients with insomnia compared with good sleepers. Sleep 39, 1779–1794. doi: 10.5665/sleep.6154
Krause, A. J., Simon, E. B., Mander, B. A., Greer, S. M., Saletin, J. M., Goldstein-Piekarski, A. N., et al. (2017). The sleep-deprived human brain. Nat. Rev. Neurosci. 18, 404–418. doi: 10.1038/nrn.2017.55
Landry, G. J., Best, J. R., and Liu-Ambrose, T. (2015). Measuring sleep quality in older adults: a comparison using subjective and objective methods. Front. Aging Neurosci. 7:116. doi: 10.3389/fnagi.2015.00166
Lao, X. Q., Liu, X., Deng, H.-B., Chan, T.-C., Ho, K. F., Wang, F., et al. (2018). Sleep quality, sleep duration, and the risk of coronary heart disease: a prospective cohort study with 60,586 adults. J. Clin. Sleep Med. 14, 109–117. doi: 10.5664/jcsm.6894
Lauriola, M., Esposito, R., Delli Pizzi, S., Zambotti, M., de Londrillo, F., Kramer, J. H., et al. (2017). Sleep changes without medial temporal lobe or brain cortical changes in community-dwelling individuals with subjective cognitive decline. Alzheimers Dement. J. Alzheimers Assoc. 13, 783–791. doi: 10.1016/j.jalz.2016.11.006
Li, C., Ma, X., Dong, M., Yin, Y., Hua, K., Li, M., et al. (2016). Abnormal spontaneous regional brain activity in primary insomnia: a resting-state functional magnetic resonance imaging study. Neuropsychiatr. Dis. Treat. 12, 1371–1378. doi: 10.2147/NDT.S109633
Li, Z., Chen, R., Guan, M., Wang, E., Qian, T., Zhao, C., et al. (2018). Disrupted brain network topology in chronic insomnia disorder: a resting-state fMRI study. Neuroimage Clin. 18, 178–185. doi: 10.1016/j.nicl.2018.01.012
Liguori, C., Izzi, F., Mercuri, N. B., and Placidi, F. (2017). Commentary: sleep changes without medial temporal lobe or brain cortical changes in community-dwelling individuals with subjective cognitive decline. Front. Neurol. 8:262. doi: 10.3389/fneur.2017.00262
Livingston, G., Blizard, B., and Mann, A. (1993). Does sleep disturbance predict depression in elderly people? a study in inner London. Br. J. Gen. Pract. 43, 445–448.
Maggi, S., Langlois, J. A., Minicuci, N., Grigoletto, F., Pavan, M., Foley, D. J., et al. (1998). Sleep complaints in community-dwelling older persons: prevalence, associated factors, and reported causes. J. Am. Geriatr. Soc. 46, 161–168. doi: 10.1111/j.1532-5415.1998.tb02533.x
Mallon, L., Broman, J. E., and Hetta, J. (2000). Relationship between insomnia, depression, and mortality: a 12-year follow-up of older adults in the community. Int. Psychogeriatr. 12, 295–306. doi: 10.1017/S1041610200006414
Mander, B. A., Winer, J. R., and Walker, M. P. (2017). Sleep and human aging. Neuron 94, 19–36. doi: 10.1016/j.neuron.2017.02.004
Meier, J., Tewarie, P., Hillebrand, A., Douw, L., van Dijk, B. W., Stufflebeam, S. M., et al. (2016). A mapping between structural and functional brain networks. Brain Connect. 6, 298–311. doi: 10.1089/brain.2015.0408
Nie, X., Shao, Y., Liu, S.-Y., Li, H.-J., Wan, A.-L., Nie, S., et al. (2015). Functional connectivity of paired default mode network subregions in primary insomnia. Neuropsychiatr. Dis. Treat. 11, 3085–3093. doi: 10.2147/NDT.S95224
Ohayon, M., Wickwire, E. M., Hirshkowitz, M., Albert, S. M., Avidan, A., Daly, F. J., et al. (2017). National Sleep Foundation's sleep quality recommendations: first report. Sleep Health 3, 6–19. doi: 10.1016/j.sleh.2016.11.006
Onitsuka, T., Shenton, M. E., Salisbury, D. F., Dickey, C. C., Kasai, K., Toner, S. K., et al. (2004). Middle and inferior temporal gyrus gray matter volume abnormalities in chronic schizophrenia: an MRI study. Am. J. Psychiatry 161, 1603–1611. doi: 10.1176/appi.ajp.161.9.1603
Power, J. D., Barnes, K. A., Snyder, A. Z., Schlaggar, B. L., and Petersen, S. E. (2012). Spurious but systematic correlations in functional connectivity MRI networks arise from subject motion. Neuroimage 59, 2142–2154. doi: 10.1016/j.neuroimage.2011.10.018
Ritchie, S. J., Dickie, D. A., Cox, S. R., Valdes Hernandez, M. del C., Corley, J., Royle, N. A., et al. (2015). Brain volumetric changes and cognitive ageing during the eighth decade of life. Hum. Brain Mapp. 36, 4910–4925. doi: 10.1002/hbm.22959
Sämann, P. G., Tully, C., Spoormaker, V. I., Wetter, T. C., Holsboer, F., Wehrle, R., et al. (2010). Increased sleep pressure reduces resting state functional connectivity. Magn. Reson. Mater. Phys. Biol. Med. 23, 375–389. doi: 10.1007/s10334-010-0213-z
Santos, C. (2001). Avaliação da Sonolência Diurna Excessiva: Adaptação Cultural e Linguística da Escala de Sonolência de Epworth Para a População Portuguesa. Dissertation, Superior School of Technology of Porto (Porto).
Santos, N. C., Costa, P. S., Cunha, P., Cotter, J., Sampaio, A., Zihl, J., et al. (2013). Mood is a key determinant of cognitive performance in community-dwelling older adults: a cross-sectional analysis. AGE 35, 1983–1993. doi: 10.1007/s11357-012-9482-y
Santos, N. C., Costa, P. S., Cunha, P., Portugal-Nunes, C., Amorim, L., Cotter, J., et al. (2014). Clinical, physical and lifestyle variables and relationship with cognition and mood in aging: a cross-sectional analysis of distinct educational groups. Front. Aging Neurosci. 6:21. doi: 10.3389/fnagi.2014.00021
Schwartz, S., McDowell Anderson, W., Cole, S. R., Cornoni-Huntley, J., Hays, J. C., and Blazer, D. (1999). Insomnia and heart disease: a review of epidemiologic studies. J. Psychosom. Res. 47, 313–333. doi: 10.1016/S0022-3999(99)00029-X
Scullin, M. K. (2017). Do older adults need sleep? A review of neuroimaging, sleep, and aging studies. Curr. Sleep Med. Rep. 3, 204–214. doi: 10.1007/s40675-017-0086-z
Scullin, M. K., and Bliwise, D. L. (2015). Sleep, cognition, and normal aging: integrating a half-century of multidisciplinary research. Perspect. Psychol. Sci. J. Assoc. Psychol. Sci. 10, 97–137. doi: 10.1177/1745691614556680
Sexton, C. E., Storsve, A. B., Walhovd, K. B., Johansen-Berg, H., and Fjell, A. M. (2014). Poor sleep quality is associated with increased cortical atrophy in community-dwelling adults. Neurology 83, 967–973. doi: 10.1212/WNL.0000000000000774
Shao, Y., Lei, Y., Wang, L., Zhai, T., Jin, X., Ni, W., et al. (2014). Altered resting-state amygdala functional connectivity after 36 hours of total sleep deprivation. PLoS ONE 9:e112222. doi: 10.1371/journal.pone.0112222
Shokri-Kojori, E., Wang, G.-J., Wiers, C. E., Demiral, S. B., Guo, M., Kim, S. W., et al. (2018). β-Amyloid accumulation in the human brain after one night of sleep deprivation. Proc. Natl. Acad. Sci. U.S.A. 115, 4483–4488. doi: 10.1073/pnas.1721694115
Sivertsen, B., Madsen, I. E. H., Salo, P., Tell, G. S., and Øverland, S. (2015). Use of sleep medications and mortality: The Hordaland Health Study. Drugs Real World Outcomes 2, 123–128. doi: 10.1007/s40801-015-0023-8
Smith, S. M., Jenkinson, M., Johansen-Berg, H., Rueckert, D., Nichols, T. E., Mackay, C. E., et al. (2006). Tract-based spatial statistics: voxelwise analysis of multi-subject diffusion data. Neuroimage 31, 1487–1505. doi: 10.1016/j.neuroimage.2006.02.024
Smith, S. M., Jenkinson, M., Woolrich, M. W., Beckmann, C. F., Behrens, T. E. J., Johansen-Berg, H., et al. (2004). Advances in functional and structural MR image analysis and implementation as FSL. Neuroimage 23(Suppl. 1), S208–S219. doi: 10.1016/j.neuroimage.2004.07.051
Son, Y.-D., Kang, J. M., Cho, S.-J., Lee, J.-S., Hwang, H. Y., and Kang, S.-G. (2018). fMRI brain activation in patients with insomnia disorder during a working memory task. Sleep Breath 22, 487–493. doi: 10.1007/s11325-017-1575-5
Sporns, O. (2003). Network analysis, complexity, and brain function. Complexity 8, 56–60. doi: 10.1002/cplx.10047
Stickgold, R. (2006). Neuroscience: a memory boost while you sleep. Nature 444, 559–560. doi: 10.1038/nature05309
St-Onge, M.-P. (2005). Relationship between body composition changes and changes in physical function and metabolic risk factors in aging. Curr. Opin. Clin. Nutr. Metab. Care 8, 523–528.
Stranges, S., Tigbe, W., Gómez-Olivé, F. X., Thorogood, M., and Kandala, N.-B. (2012). Sleep problems: an emerging global epidemic? findings from the INDEPTH WHO-SAGE study among more than 40,000 older adults from 8 countries across Africa and Asia. Sleep 35, 1173–1181. doi: 10.5665/sleep.2012
Tewarie, P., Hillebrand, A., van Dellen, E., Schoonheim, M. M., Barkhof, F., Polman, C. H., et al. (2014). Structural degree predicts functional network connectivity: a multimodal resting-state fMRI and MEG study. Neuroimage 97, 296–307. doi: 10.1016/j.neuroimage.2014.04.038
Tononi, G., and Cirelli, C. (2006). Sleep function and synaptic homeostasis. Sleep Med. Rev. 10, 49–62. doi: 10.1016/j.smrv.2005.05.002
Uhlhaas, P. J., and Singer, W. (2012). Neuronal dynamics and neuropsychiatric disorders: toward a translational paradigm for dysfunctional large-scale networks. Neuron 75, 963–980. doi: 10.1016/j.neuron.2012.09.004
United Nations Department of Economic and Social Affairs, Population Division (2013). World Population Ageing 2013. New York, NY: WHO.
van den Heuvel, M. P., Mandl, R. C. W., Kahn, R. S., and Hulshoff Pol, H. E. (2009). Functionally linked resting-state networks reflect the underlying structural connectivity architecture of the human brain. Hum. Brain Mapp. 30, 3127–3141. doi: 10.1002/hbm.20737
Van Someren, E. J. W., Oosterman, J. M., Van Harten, B., Vogels, R. L., Gouw, A. A., Weinstein, H. C., et al. (2018). Medial temporal lobe atrophy relates more strongly to sleep-wake rhythm fragmentation than to age or any other known risk. Neurobiol. Learn. Mem. doi: 10.1016/j.nlm.2018.05.017. [Epub ahead of print].
Veer, I. M., Beckmann, C., Van Tol, M.-J., Ferrarini, L., Milles, J., Veltman, D., et al. (2010). Whole brain resting-state analysis reveals decreased functional connectivity in major depression. Front. Syst. Neurosci. 4:41. doi: 10.3389/fnsys.2010.00041
Wulff, K., Gatti, S., Wettstein, J. G., and Foster, R. G. (2010). Sleep and circadian rhythm disruption in psychiatric and neurodegenerative disease. Nat. Rev. Neurosci. 11, 589–599. doi: 10.1038/nrn2868.
Xie, L., Kang, H., Xu, Q., Chen, M. J., Liao, Y., Thiyagarajan, M., et al. (2013). Sleep drives metabolite clearance from the adult brain. Science 342, 373–377. doi: 10.1126/science.1241224
Yeo, B. T. T., Tandi, J., and Chee, M. W. L. (2015). Functional connectivity during rested wakefulness predicts vulnerability to sleep deprivation. Neuroimage 111, 147–158. doi: 10.1016/j.neuroimage.2015.02.018
Yesavage, J. A., Brink, T. L., Rose, T. L., Lum, O., Huang, V., Adey, M., et al. (1982). Development and validation of a geriatric depression screening scale: a preliminary report. J. Psychiatr. Res. 17, 37–49. doi: 10.1016/0022-3956(82)90033-4
Yun, J.-Y., Kim, J.-C., Ku, J., Shin, J.-E., Kim, J.-J., and Choi, S.-H. (2017). The left middle temporal gyrus in the middle of an impaired social-affective communication network in social anxiety disorder. J. Affect. Disord. 214, 53–59. doi: 10.1016/j.jad.2017.01.043
Keywords: Pittsburgh Sleep Quality Index, PSQI, MRI, whole-brain modeling, brain connectivity, resting-state
Citation: Amorim L, Magalhães R, Coelho A, Moreira PS, Portugal-Nunes C, Castanho TC, Marques P, Sousa N and Santos NC (2018) Poor Sleep Quality Associates With Decreased Functional and Structural Brain Connectivity in Normative Aging: A MRI Multimodal Approach. Front. Aging Neurosci. 10:375. doi: 10.3389/fnagi.2018.00375
Received: 29 August 2018; Accepted: 29 October 2018;
Published: 20 November 2018.
Edited by:
Patrizia Mecocci, University of Perugia, ItalyReviewed by:
Robert Trampel, Max-Planck-Gesellschaft (MPG), GermanyStefano Delli Pizzi, Università degli Studi G. d'Annunzio Chieti e Pescara, Italy
Copyright © 2018 Amorim, Magalhães, Coelho, Moreira, Portugal-Nunes, Castanho, Marques, Sousa and Santos. This is an open-access article distributed under the terms of the Creative Commons Attribution License (CC BY). The use, distribution or reproduction in other forums is permitted, provided the original author(s) and the copyright owner(s) are credited and that the original publication in this journal is cited, in accordance with accepted academic practice. No use, distribution or reproduction is permitted which does not comply with these terms.
*Correspondence: Nadine Correia Santos, bnNhbnRvc0BtZWQudW1pbmhvLnB0