- 1College of Animal Sciences, Zhejiang University, Hangzhou, China
- 2Institute of Apicultural Research, Chinese Academy of Agricultural Sciences, Beijing, China
Alzheimer’s disease (AD) is characterized clinically by progressive cognitive decline and pathologically by the accumulation of amyloid-β (Aβ) in the brain. Royal jelly (RJ), a secretion of honeybee hypopharyngeal and mandibular glands, has previously been shown to have anti-aging and neuromodulatory activities. In this study, we discovered that 3 months of RJ treatment substantially ameliorated behavioral deficits of APP/PS1 mice in the Morris Water Maze (MWM) test and step-down passive avoidance test. Our data also showed that RJ significantly diminished amyloid plaque pathology in APP/PS1 mice. Furthermore, RJ alleviated c-Jun N-terminal kinase (JNK) phosphorylation-induced neuronal apoptosis by suppressing oxidative stress. Importantly, hippocampal cyclic adenosine monophosphate (cAMP), p-PKA, p-CREB and BDNF levels were significantly increased in the APP/PS1 mice after RJ treatment, indicating that the cAMP/PKA/CREB/BDNF pathway might be related to the ameliorative effect of RJ on cognitive decline. Collectively, these results provide a scientific basis for using RJ as a functional food for targeting AD pathology.
Introduction
Alzheimer’s disease (AD) is an age-related neurodegenerative disease characterized by progressive loss of cognitive and memory function (Citron, 2010). AD afflicts more than 45 million individuals worldwide and has an increasing incidence, which has serious adverse impact on the growing elderly population as well as their families (Prince et al., 2015). Currently, mounting evidence has shown that the extracellular deposition of amyloid-β (Aβ) in the brain, which will lead to insoluble senile plaques (SPs), is a critical hallmark of AD (Campion et al., 2016). It has been reported that Aβ induced the progression of other pathological abnormalities, including intraneuronal neurofibrillary tangles (NFTs), which are caused by hyperphosphorylated tau, neuroinflammation, and neuronal loss. Conversely, these pathological events also aggravated the deposition of Aβ and further promoted AD progression (Tanzi and Bertram, 2005; Bettens et al., 2013). Aβ is produced by amyloid precursor protein (APP) through the amyloidogenic pathway. In the first stage of Aβ generation, the proteolytic cleavage of APP by β-secretase (BACE1) produces part-soluble APP peptide-β (sAPPβ) and C-terminal fragment-β (CTFβ), which are further cleaved by γ-secretase to generate hydrophobic Aβ polypeptides (Aβ40 and Aβ42; Dong et al., 2006). In addition, Aβ-degrading enzymes, including insulin-degrading enzyme (IDE) and neprilysin (NEP), are responsible for the clearance of Aβ in the brain. They are capable of cleaving and converting Aβ polypeptide to benign forms (Mukherjee et al., 2000; Shirotani et al., 2001). Hence, it is widely accepted that excessive deposition of Aβ can be explained by the imbalance between formation and degradation (Hardy and Selkoe, 2002).
Currently, treatment strategies targeting the enhancement of brain Aβ clearing activities, such as Aβ immunotherapy, are being extensively studied (Mangialasche et al., 2010). However, the use of therapeutic antibodies may cause a series of side effects, including vasogenic edema (Salloway et al., 2009), microhemorrhage (Nicoll et al., 2003) and neuronal hyperactivity (Busche et al., 2015). In addition, recent documents showed that Aβ immunotherapy could not improve cognitive deficits in AD patients (Doody et al., 2014). Likewise, tau aggregation inhibitors failed to improve the memory and cognitive function during AD progression (Gauthier et al., 2016). In view of the above failures, there is a need for new drugs to inhibit the cognitive deficits of AD and alleviate the associated neuropathology. Several recent studies have shown the positive effects of natural functional foods in cell culture experiments as well as in animal models of AD (Reddy et al., 2018; Youn et al., 2018). Royal jelly (RJ), a bee product produced by the hypopharyngeal and mandibular glands of worker bees, is a traditional functional food (Sabatini et al., 2009). RJ comprises water, protein, carbohydrates, vitamin, lipids, acetylcholine and other bioactive substances that endow RJ with a variety of pharmacological activities, including anti-inflammatory activity (You et al., 2018), anti-oxidative activity (Guo et al., 2009) and anti-aging activity (Honda et al., 2015). Importantly, there are several reports suggesting that RJ has neuromodulatory activity in different animal models. Hattori et al. (2010b) showed that orally administered RJ improved the cognitive impairment of trimethyltin (TMT)-treated mice. Zamani et al. (2012) found a neuroprotective role for RJ in streptozotocin (STZ) induced sporadic AD rat models. Wang et al. (2015) have verified that supplementation with RJ significantly promoted lifespan and stress resistance in C. elegans. Their subsequent studies further showed that RJ alleviated Aβ toxicity in C. elegans, and revealed the potential function of RJ in AD treatment (Wang et al., 2016). However, it is not clear whether RJ treatment contributes to the alleviation of familial AD. Thus, in this study, we assessed the effects of RJ on AD pathology and cognitive function in APP/PS1 double transgenic mice and further investigated the underlying mechanisms.
Materials and Methods
Gas Chromatography (GC) Detection
The content of trans-10-hydroxy-2-decenoic acid (10-HDA), 10-hydroxydecanoic acid (10-HDAA) and sebacic acid (SEA) in RJ samples were measured using GC. 0.25 g lyophilized RJ powder was extracted with 20 mL ethanol for 15 min and 5 mL methyl 4-hydroxybenzoate was added as internal standard. Next the ethanol solvent was removed, 1 mL ether was added and filtered through 0.22 μm filter membrane. After evaporation of the ether, 400 μL pyridine and 80 μL N, O-Bis(trimethylsilyl) trifluoroacetamide (BSTFA; Sigma, Kawasaki, Japan) were added, sealed and heated at 60°C for 60 min to derivatize samples. All derivatized extracts were analyzed on Gas Chromatography-Flame Ionization Detector (GC-FID; GC-2010, Shimadzu, Kyoto, Japan) using InertCap-5 column (30 m × 0.25 mm I.D. × 0.25 μm film). One microliter sample was injected with the help of AOC-20i auto injector (Shimadzu, Kyoto, Japan). Nitrogen was used as a carrier gas and flow was kept constant at 1 mL/min. The injector was held at 280°C and worked on split mode (split ratio 10:1). The initial column temperature was 60°C and held for 5 min, then raised to 300°C at 5°C/min and held for 5 min. The detector was performed at 325°C.
Animals and Treatments
Ten-month-old APP/PS1 transgenic mice with a C57BL/6 background (B6C3-Tg (APPswe, PSEN1dE9) 85Dbo/J) and age-matched C57BL/6 mice were purchased from the Model Animal Research Center of Nanjing University (Nanjing, China). All experiments were approved by the Institutional Animal Care and Use Committee of Zhejiang Chinese Medical University (IACUC Approval No: ZSLL-2017-079) and were performed according to the guidelines from the Laboratory Animal Research Center of Zhejiang Chinese Medical University (Certificate No. SYXK, Zhejiang, 2013-0184, China). The experimental design of behavioral and biochemical analysis is shown in Supplementary Figure S1. We used equal numbers of female and male APP/PS1 mice at the age of 10 months to explore the effects of RJ on AD after 3 months administration. After 1 week of acclimatization, the mice were divided into the following groups: (1) Wt group, wild-type mice were given intragastric administration of saline; (2) Tg group, APP/PS1 transgenic mice were given intragastric administration of saline; (3) TgRJ group, APP/PS1 transgenic mice were given intragastric administration of RJ; and (4) WtRJ group, wild-type mice were given intragastric administration of RJ. Each group had 10 mice. All groups received oral administration of saline or RJ at a dose of 300 mg/kg/d for 3 months. Behavioral analysis was carried out at 54th and 82th day using Morris Water Maze (MWM) test, and at 89th day using step-down passive avoidance test.
MWM Test
The spatial learning-memory abilities of mice were assessed by MWM test as described previously (Seung et al., 2018). Briefly, a circular water tank (height 40 cm, diameter 100 cm) was filled with water maintained at 22–25°C. The escape platform (height 35 cm, diameter 6 cm) was placed submerged 1–1.5 cm below the surface of water. Soybean milk powder was added to make water opaque before the test. The navigation test was conducted once a day for six consecutive days with one constant hidden platform point in quadrant 2 and three rotational starting points. The limit time was 120 s/trail and the trail will end as soon as mice stayed on the hidden escape platform for 5 s. Escape latency and swim path tracking of each mouse were recorded by a camera mounted above the center of tank. The probe test was carried out 24 h after the last navigation test to evaluate the memory consolidation of mice. During the probe test, the escape platform was removed and mice could swim freely for 120 s. The time spent in the target quadrant and swim path tracking of each mouse were recorded. All data were analyzed using video-tracking software (SMART, version 2.5.15).
Step-Down Passive Avoidance Test
The step-down passive avoidance test was performed 1 day after the MWM test as described in previous reports with minor modifications (Ballesta et al., 2012). The equipment comprised five plastic black chambers with parallel, stainless-steel grids and a plastic platform was positioned in a corner of each chamber. Before the training session, mice were gently placed on the grid floor and each mouse was given 5 min for adaptation. After that, the electric currents (36 V) were delivered and maintained for 300 s. The mice would jump onto the platform to avoid the electric shock and the number of errors of each mouse (number of times that the mouse stepped down from platform) was recorded. After a 24 h interval, the retention test was carried out and the electric shock was removed, mice were placed on the platform and the step-down latency of each mouse was recorded. The cut-off time in the training session and the retention session was set to 300 s.
Brain Tissue Preparation
After the behavior tests, the mice were weighed and deeply anesthetized with pentobarbital sodium (45 mg/kg, intraperitoneally). The whole brain tissues were rapidly removed from the skull on ice. They were then cut sagittally into left and right hemispheres. The cortex and hippocampus in the right hemispheres were dissected on ice, snap frozen in liquid nitrogen and stored at −80°C for biochemical analysis. The left hemispheres were fixed in 4% paraformaldehyde for at least 24 h and then embedded with paraffin and cut into 6 μm sections for further staining analysis.
Histological Examinations
Immunohistochemical (IHC) Staining
The methods of IHC staining have been reported previously (Pan et al., 2018). Briefly, sections were blocked with 3% BSA for 30 min, then incubated with primary antibodies overnight at 4°C, including BACE1 (1:100, Santa Cruz Biotechnology, USA), IDE (1:100, Santa Cruz Biotechnology, USA), β-amyloid (B-4, 1:100, Santa Cruz Biotechnology, USA). PBS was used as a negative control instead of the primary antibody. Subsequently, the sections were washed with PBS three times and were incubated with secondary antibody for 1 h at room temperature. Sections were counterstained with hematoxylin and visualized with DAB (ZSJQ, Beijing, China). The captured images were analyzed using Image Pro Plus 6.0 software (Media Cybernetics, Rockville, MD, USA). For quantification, we calculated BACE1, IDE- and β-amyloid-positive areas under 40× magnification (hippocampus) or under 20× magnification (cortex) in three random fields in the brain of each mouse, and the staining was quantified as fraction of immune-positive staining to the total area measured.
Fluorescence Microscopy
The fixed brain sections were blocked with 3% BSA for 30 min, exposed to the anti-cleaved caspase-3 antibody (1:100, Abcam, ab13847) overnight at 4°C and then placed in a wet box containing a little water. After incubation, the sections were washed twice with ice-cold PBS and incubated with Alexa Fluor 594-conjugated goat anti-rabbit IgG (1:250) for 50 min at 37°C in dark. They were then incubated with DAPI solution (Sangon Biotechnology, Co. Ltd., Shanghai, China) at room temperature for 10 min. Immunofluorescence images were acquired using a confocal laser microscope (Leica, TCS SP5, Germany).
Thioflavin-T Staining
Thioflavin-T is a kind of fluorochrome that specifically binds to amyloid deposits, and they can be excited to produce green fluorescence, which is used to evaluate the amount of Aβ protein (Zhang et al., 2013). Briefly, slides were deparaffinized and rehydrated in descending grades of ethanol, placed in Mayer’s hematoxylin for 5 min, rinsed twice in double distilled water, incubated with 1% thioflavin-T (Dalian Meilun Biological Technology Co. Ltd., China) for 10 min and changed distilled water three times, cover-slipped in a neutral glycerol, and examined with a Hg-lamp for fluorescence excitation using a Zeiss inverted microscope (Axiovert 200, Carl Zeiss, USA).
Brain Aβ Enzyme-Linked Immunosorbent Assay (ELISA)
Brain tissues were homogenized in PBS containing 1% SDS and a protease inhibitor cocktail (Roche), and were centrifuged at 10,000 g for 1 h at 4°C. Then, the supernatant was collected as SDS-soluble fraction. In the meantime, the pellets were resuspended and homogenized in 70% formic acid. After centrifugation, the supernatant was collected as the formic-soluble (insoluble) fraction and neutralized with 1 M Tris buffer (pH = 11). Aβ1–40 and Aβ1–42 levels in the brain were determined using Aβ1–40 and Aβ1–42 ELISA kits purchased from the Jiancheng Bioengineering Institute (Nanjing, China) according to the manufacturer’s instructions. The protein concentrations of supernatant were tested using a commercial bicinchoninic acid (BCA) kit (Beyotime Biotechnology, Hangzhou, China) and the normalized amounts of Aβ were expressed as pg/mg of protein.
Malonaldehyde (MDA) Contents in the Plasma and Brain
The MDA content level in the plasma and brain were measured using a commercial kit (Jiancheng Bioengineering Institute, Nanjing, China) according to the manufacturer’s instructions. Brain tissues were homogenized in PBS and centrifuged at 3,000 rpm for 20 min at 4°C. The supernatant was collected and stored at −80°C until use.
The Cyclic Adenosine Monophosphate (cAMP) Assay
Cyclic adenosine monophosphate (cAMP) levels in the hippocampus were measured using commercial ELISA kits (Elabscience Biotechnology Co., Ltd, China). Mice hippocampus tissues were homogenized in PBS, and then the homogenates were centrifuged at 5,000 g for 15 min at 4°C to remove the particles. The protein concentrations of supernatant were tested using BCA Protein Assay Kit and the cAMP levels were expressed as pmol/mg protein.
Western Blot
Western blot analysis was conducted as described previously (Guo et al., 2015). Protein extraction kit was purchased from KeyGEN BioTECH, Co., Ltd. (Jiangsu, China). The protease inhibitor, phosphatase inhibitor and PMSF were added into RIPA buffer before homogenizing the brain tissues. Protein concentrations were measured using a BCA Protein Assay Kit, and equal amounts of protein (40 μg) were separated by sodium dodecyl sulfate-polyacrylamide gel electrophoresis (SDS-PAGE) and then transferred onto polyvinylidene difluoride (PVDF) membranes (Millipore, Billerica, MA). PVDF membranes were blocked with 5% skim milk at room temperature for 1 h to avoid nonspecific binding and immunoblots were incubated overnight at 4°C with primary antibodies. The primary antibodies we used in this study are listed in Table 1. After primary antibody binding, horseradish peroxidase-conjugated secondary antibodies were incubated for 1 h at room temperature. Blots were developed by the ECL method, and band intensities were quantified using ImageJ Software.
Statistical Analysis
All data are expressed as the mean ± SEM. Student’s t-test or analyses of variance (ANOVA) with a post hoc Tukey’s test were used to determine statistical differences, and P values < 0.05 were considered statistically significant. Statistical analyses were performed using GraphPad Prism 6.0 (GraphPad Software, Inc., La Jolla, CA, USA).
Results
Identification of Fatty Acids in RJ Samples
10-HDA, 10-HDAA and SEA are the main RJ acids, and they were quantified by GC analysis in this study (Figure 1). The percentage of 10-HDA in the RJ was 4.87%, which showed that the RJ used in the present study was of qualified quality.
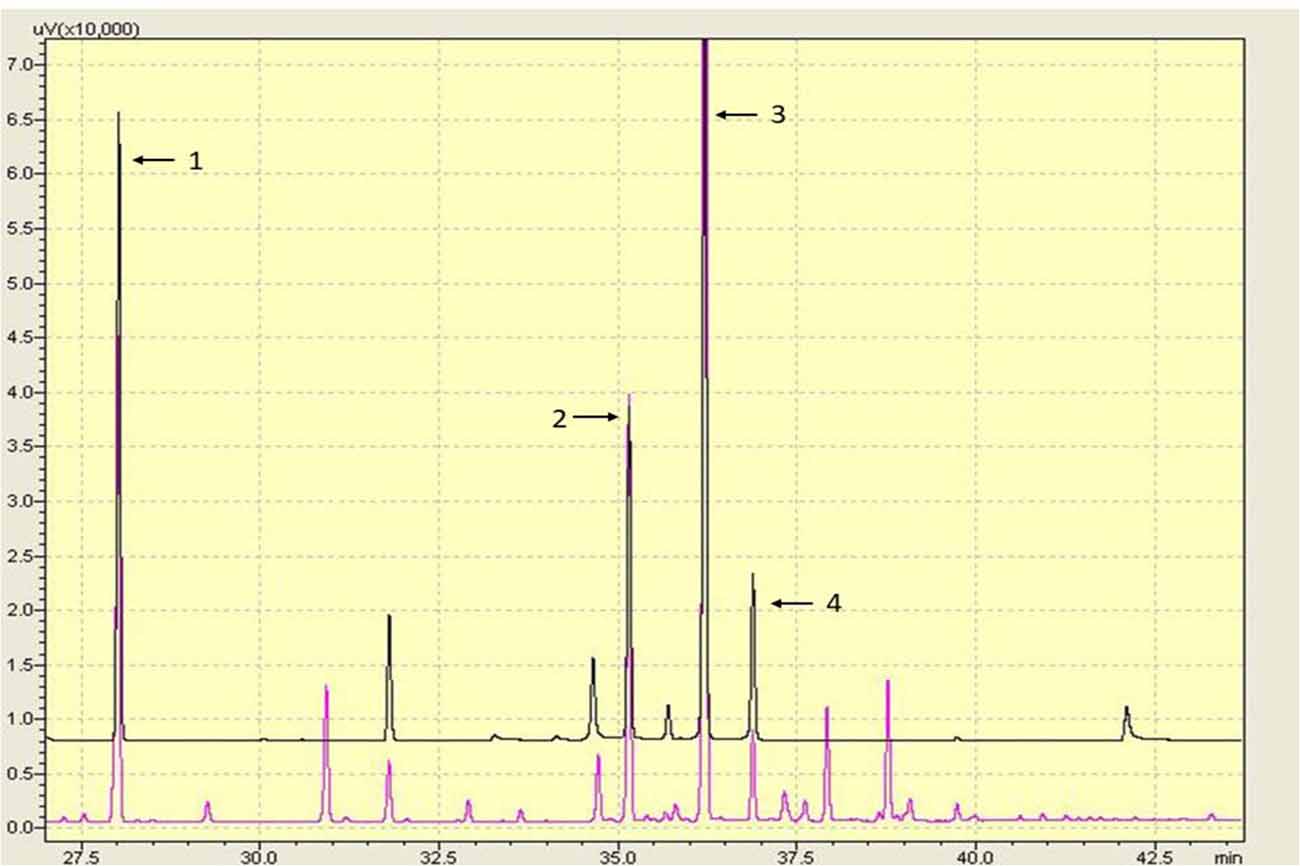
Figure 1. Gas chromatography (GC) chromatography of the lyophilized royal jelly (RJ) powder. Peaks: (1) methyl 4-hydroxybenzoate; (2) 10-hydroxydecanoic acid (10-HDAA); (3) trans-10-hydroxy-2-decenoic acid (10-HDA); and (4) Sebacic acid (SEA).
RJ Ameliorates Cognitive Deficits in APP/PS1 Mice
In the acquisition trial, mice in the Wt group and WtRJ group could find the submerged platform by a well-organized trajectory after 5 days of training. However, the path tracking of Tg mice was relatively random and disorganized compared with Wt mice. After oral administration of RJ for 3 months, the mice in the TgRJ group had shorter path lengths and selective search tracking, which demonstrated that the memory and learning functions of Tg mice were greatly improved (Figure 2A). The average search times to find the hidden platform (escape latency) of each group during six consecutive days were recorded. As shown in Figure 2B, RJ treatment could shorten the search time of Tg mice and there were significant differences on day 5 and day 6 between the Tg group and TgRJ group (P < 0.01). On day 6, the escape latency of the TgRJ group was significantly decreased by 50% compared with the Tg group. In addition, the area under each curve was calculated for quantification of the memory function. The AuC-latency in the Tg group showed a remarkable increase compared to the control values. Compared with the Tg group, a significant decrease in the AuC-latency was observed in the TgRJ group (P < 0.01), suggesting that RJ treatment for 3 months could improve memory and learning functions in APP/PS1 mice (Figure 2C). In the probe trial, the percent of time spent in the target quadrant represents the memory retention of the hidden platform location. RJ treatment for 3 months significantly improved memory retention in APP/PS1 mice (Figures 2D,E).
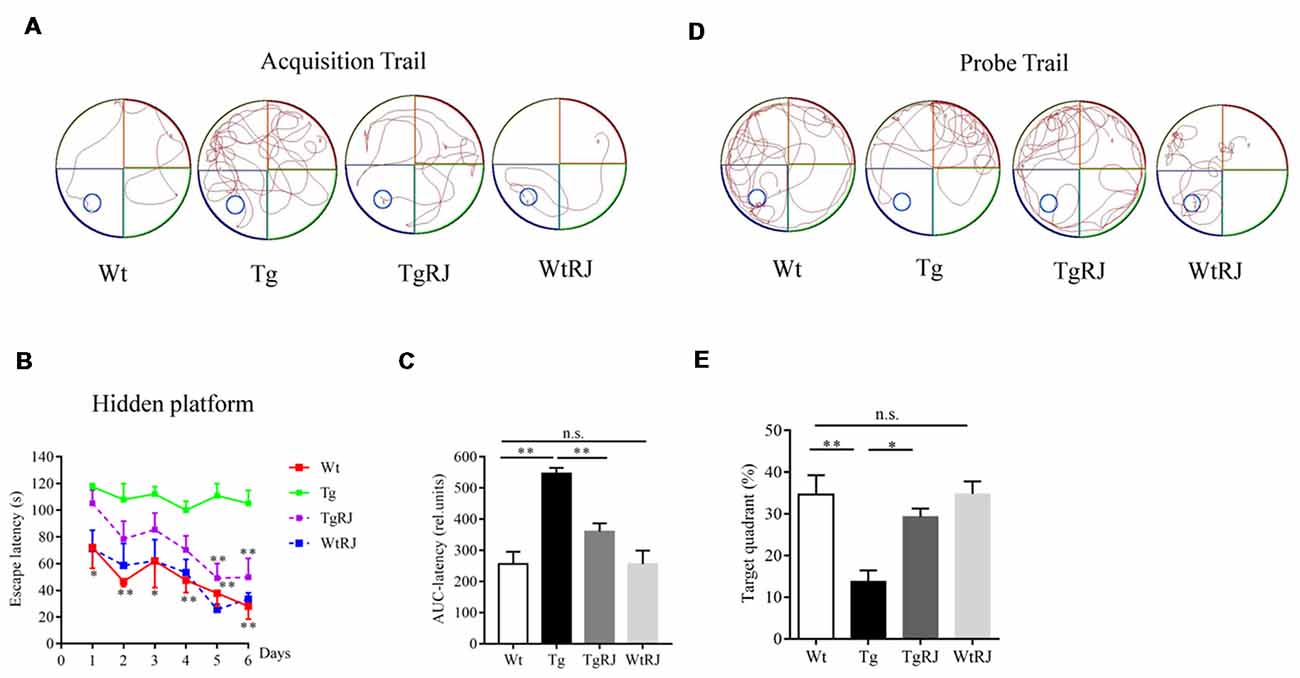
Figure 2. RJ treatment rescued impaired learning and memory function in APP/PS1 mice in the Morris Water Maze (MWM) test. (A) Representative path tracking in the acquisition trial with a hidden platform. (B) Escape latency in the MWM test plotted against the training days. Data are presented as the mean ± SEM. *P < 0.05, **P < 0.01 compared to the Tg group. (C) The area under the curve (AuC) of the escape latency was calculated for statistical comparison. (D) Representative path tracking in the probe trial without a hidden platform. (E) The percentage of searching time that mice of each group spent in the target quadrant where the platform was located on days 1–6. n = 6–10 mice per group. Data are presented as the mean ± SEM. *P < 0.05, **P < 0.01, n.s. non-significant.
The step-down passive avoidance test was carried out the day after the MWM test. The average number of errors in the Tg group was higher than in the Wt group (P < 0.05). Moreover, 3 months of oral administration of RJ in APP/PS1 mice significantly reduced the number of errors by more than 3 times (P < 0.05; Figure 3A). RJ treatment could reverse the memory function of APP/PS1 mice as reflected by an increased step-down latency (P < 0.01; Figure 3B). In addition, there was no difference in the number of errors and step-down latencies between the Wt group and WtRJ group (P > 0.05).
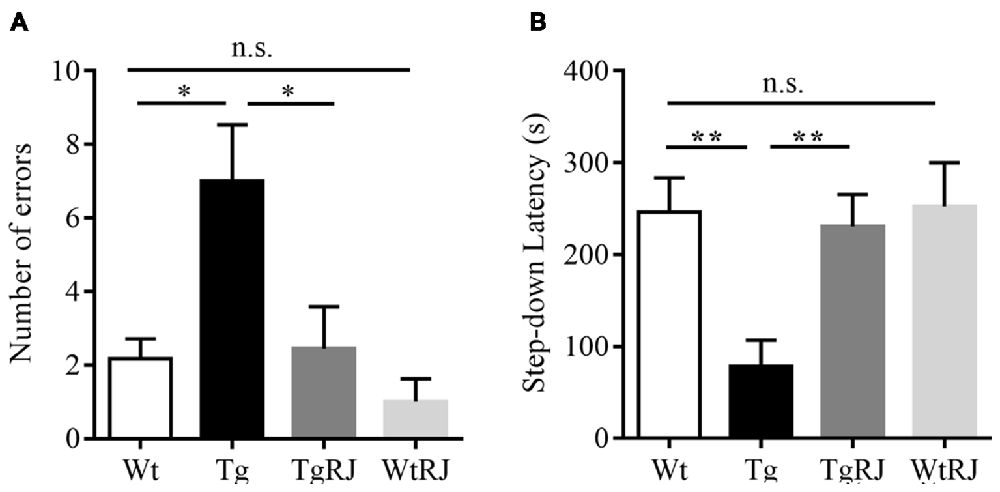
Figure 3. Effect of RJ on the step-down passive avoidance test in APP/PS1 mice. (A) The error frequency to step down from a platform after electric shock. (B) The latency of the step-down response onto the grid floor 24 h after the training. n = 6–10 mice per group. Data are presented as the mean ± SEM. *P < 0.05, **P < 0.01, n.s. non-significant.
RJ Reduces the Aβ Burden in the Hippocampus and Cortex of APP/PS1 Mice
In the SDS-soluble fraction, RJ-treated APP/PS1 mice had significantly less Aβ than the Tg group (P < 0.01). The level of Aβ in the brain homogenates of the TgRJ group was reduced by 25% for soluble Aβ40 and 40% for soluble Aβ42 compared with the Tg group levels (Figure 4A). The reduction of RJ on insoluble Aβ levels was more pronounced than the effect on soluble Aβ levels, and the insoluble Aβ40 and Aβ42 levels were reduced by approximately 60% in the TgRJ group (P < 0.01; Figure 4B). The amount of insoluble Aβ was much more than the amount of soluble Aβ in the brain of APP/PS1 mice, illustrating that insoluble Aβ is the main form of aggregated Aβ. IHC staining of Aβ also showed that RJ could inhibit the progression of AD by reducing the total area and number of SPs both in the cortex and hippocampus (Figures 4C–G). Western blot and thioflavin-T staining were carried out to further corroborate these findings (Supplementary Figure S2).
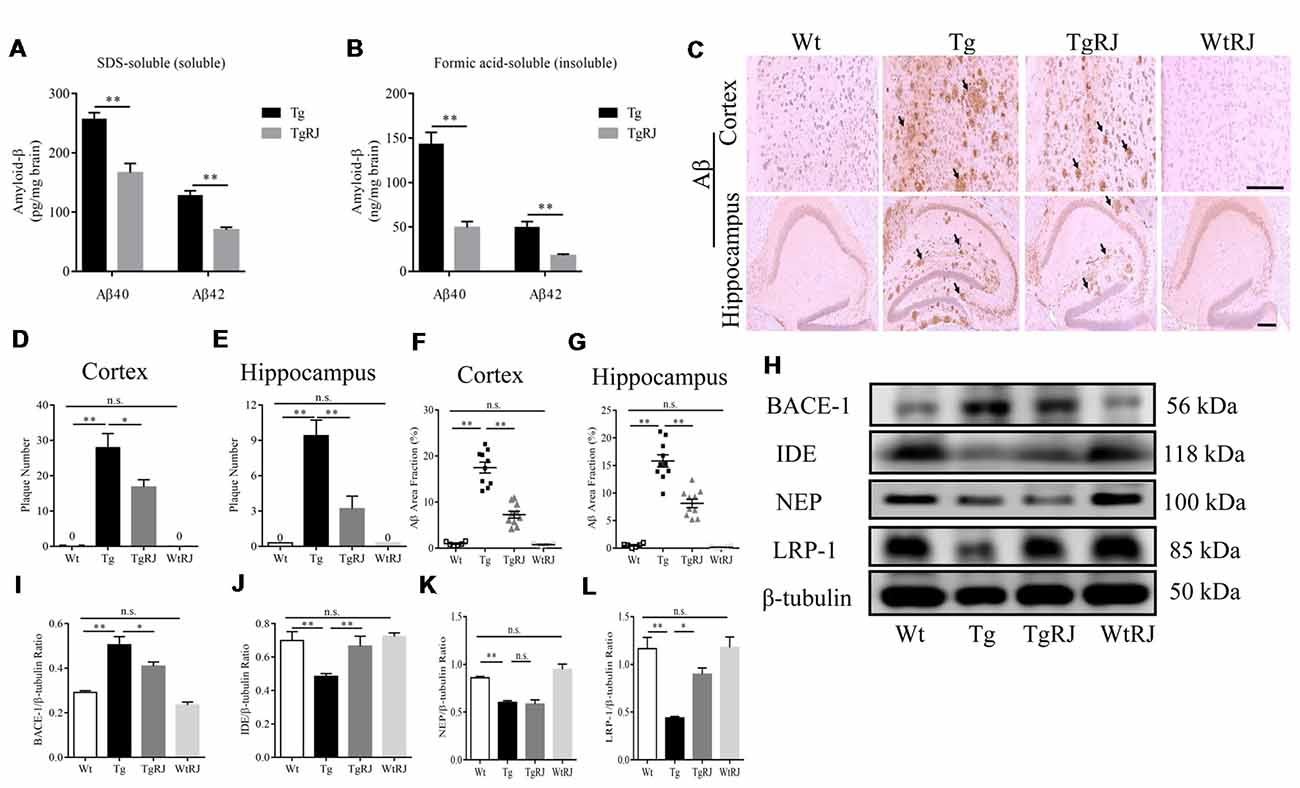
Figure 4. RJ treatment reduced the brain Aβ burden of APP/PS1 mice. (A,B) The levels of SDS-soluble and -insoluble (formic acid soluble) Aβ were measured using enzyme-linked immunosorbent assays (ELISAs). (C) The Aβ plaques in the cortex and hippocampus were estimated after immunohistochemical (IHC) staining with the Aβ antibody (B-4), the number of plaques per view (D,E; cortex, 20× magnification; hippocampus, 40× magnification) and proportions of the positive area (F,G) were calculated, three sections per animal. Scale bar = 200 μm. The arrows point out the representative senile plaques (SPs). Data are presented as the mean ± SEM. *P < 0.05, **P < 0.01, n.s. non-significant. (H–L) Immunoblot analysis of β-secretase (BACE1), insulin-degrading enzyme (IDE), neprilysin (NEP) and lipoprotein receptor-related protein-1 (LRP-1) in the brain homogenates. n = 6–10 mice per group. Data are presented as the mean ± SEM. *P < 0.05, **P < 0.01, n.s. non-significant.
To investigate the potential mechanisms responsible for the effect of RJ on Aβ, we examined the expression of BACE1, IDE, NEP and lipoprotein receptor-related protein-1 (LRP-1) using Western blot. The expression of BACE1 was remarkably elevated in the brains of the Tg group compared with the Wt group levels (P < 0.01). RJ decreased the expression of BACE1 in the brain homogenates of APP/PS1 mice (P < 0.05; Figures 4H,I). Additionally, IHC staining of BACE1 also showed that RJ reduced BACE1 expression by 44% in the cortex (P < 0.01) and by 24% in the hippocampus (P < 0.05; Supplementary Figures S3A–C). Regarding Aβ-degrading enzyme IDE, RJ treatment upregulated IDE expression at a significant level when compared with the Tg group (Figures 4H,J), and this result was confirmed by IHC staining of IDE (Supplementary Figures S3D–F). However, the expression of another Aβ-degrading enzyme, NEP, was not increased after RJ treatment (Figures 4H,K). The levels of Aβ transport receptors across the blood-brain barrier (BBB), low-density LRP-1 was also measured. LRP-1 expression in the Tg group was significantly decreased compared with the Wt group (P < 0.01), and RJ upregulated the protein expression of LRP-1 in the brain homogenates of APP/PS1 mice at a significant level (P < 0.05; Figures 4H,L). These results suggested that RJ may reduce the deposition of Aβ in APP/PS1 mice by regulating the production, degradation and clearance process. There was no difference in BACE1, IDE, NEP and LRP1 expression between the Wt group and the WtRJ group (P > 0.05).
RJ Ameliorates Oxidative Stress in APP/PS1 Mice
Oxidative stress makes a significant contribution to neurological deterioration, and oxidative damage to lipids, proteins and DNA in the central nervous system occurs in patients suffering from AD (Mancuso et al., 2010). Several lines of investigation have revealed that RJ is a potent anti-oxidative agent (Guo et al., 2009). MDA serves as a sensitive index for evaluating the oxidative stress response and was used as a marker for lipid peroxidation in the brain (Kowalczuk and Stryjeckazimmer, 2002). To determine the effect of RJ on oxidative stress, we tested the MDA levels both in both the plasma and brains of mice. The results showed that the MDA levels in the plasma and brains of the Tg group were significantly increased compared with the Wt group levels, and RJ substantially blunted this increase (Figure 5).
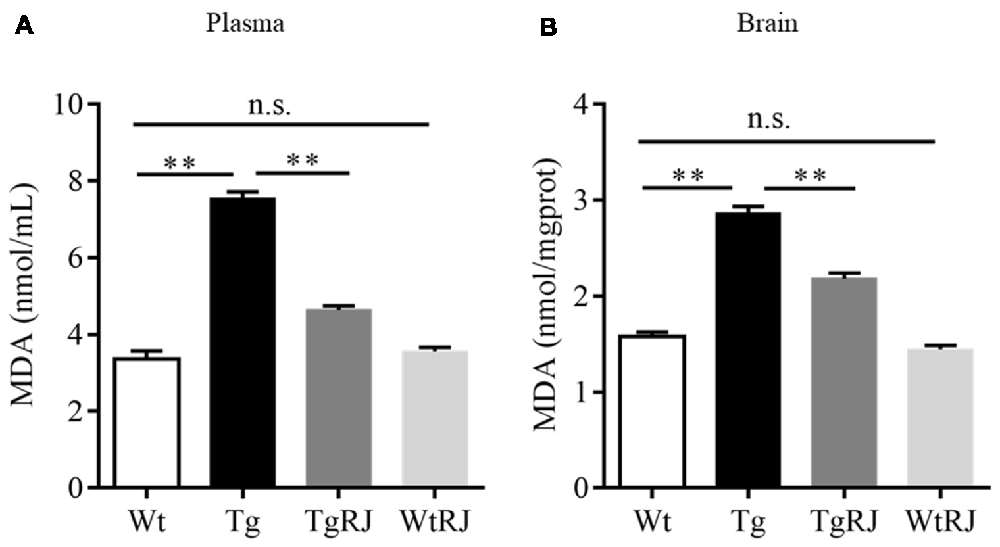
Figure 5. RJ treatment decreased malonaldehyde (MDA) levels in the plasma (A) and brains (B) of APP/PS1 mice. n = 6–10 mice per group. Data are presented as the mean ± SEM. **P < 0.01, n.s. non-significant.
RJ Inhibits Neuronal Apoptosis in the Hippocampus of APP/PS1 Mice
Previous studies reported that oxidative stress plays a critical role in neuronal apoptosis (Jiang et al., 2016). Immunofluorescence staining was used to explore whether RJ could alleviate neuronal apoptosis in the hippocampus of APP/PS1 mice. Our results showed that the protein expression of cleaved caspase-3 in the hippocampus of APP/PS1 mice was significantly increased compared with the Wt group but was decreased by RJ treatment (Figure 6A). Neuronal apoptosis was also determined by Western blots, we assessed the expression levels of pro-apoptotic markers including p-Jun N-terminal kinase (JNK), cleaved caspase-3, cleaved caspase-9, bax and the anti-apoptotic marker bcl-2 in the hippocampus of mice. According to our results, RJ treatment could suppress the activation of caspase-3 by inhibiting the phosphorylation of JNK and downregulating the bax/bcl-2 ratio (Figures 6B–F). However, RJ has no effect on the protein expression of cleaved caspase-9 (P > 0.05; Figures 6B,D).
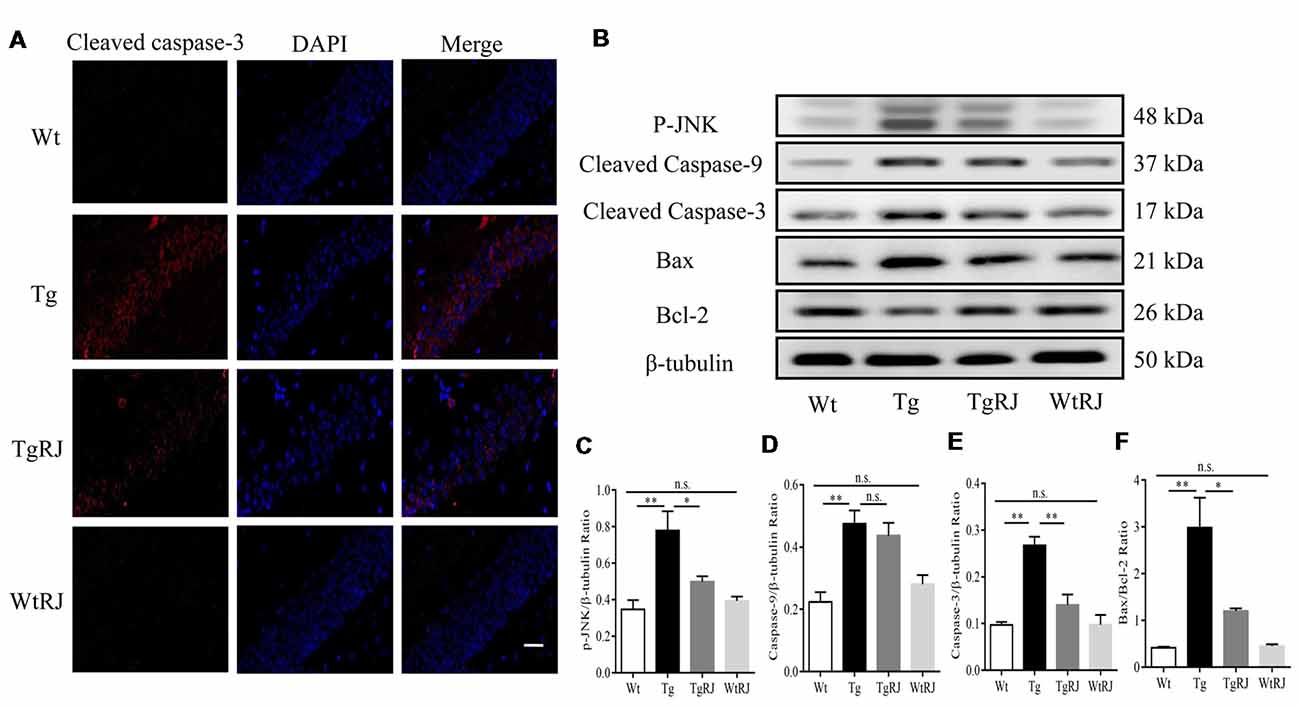
Figure 6. RJ treatment inhibited neuronal apoptosis in APP/PS1 mice. (A) Immunostaining of cleaved caspase-3 protein in the hippocampus was performed with a specific primary antibody, and fluorescence was developed using Alexa 594-conjugated anti-rabbit secondary antibody. Scale bar = 40 μm. (B–F) The expression of p-Jun N-terminal kinase (JNK), bax, bcl-2, cleaved caspase-9 and cleaved caspase-3 in the hippocampus of mice was detected by Western blot. n = 6–10 mice per group. Data are presented as the mean ± SEM. *P < 0.05, **P < 0.01, n.s. non-significant.
Effect of RJ on the cAMP/PKA/CREB/BDNF Pathway in the Hippocampus of APP/PS1 Mice
To investigate the effect of RJ on the cAMP/PKA/CREB/BDNF pathway in the hippocampus of APP/PS1 mice, we examined the changes in cAMP, p-PKA, t-PKA, p-CREB, t-CREB and BDNF protein expressions via ELISA and Western blot assays. Our results showed that, compared with the Wt group, cAMP, p-PKA, p-CREB and BDNF levels were significantly decreased in the Tg group. However, 3 months treatment with RJ remarkedly stimulated this pathway by increasing cAMP, p-PKA, p-CREB and BDNF levels without changing t-PKA and t-CREB levels (Figure 7).
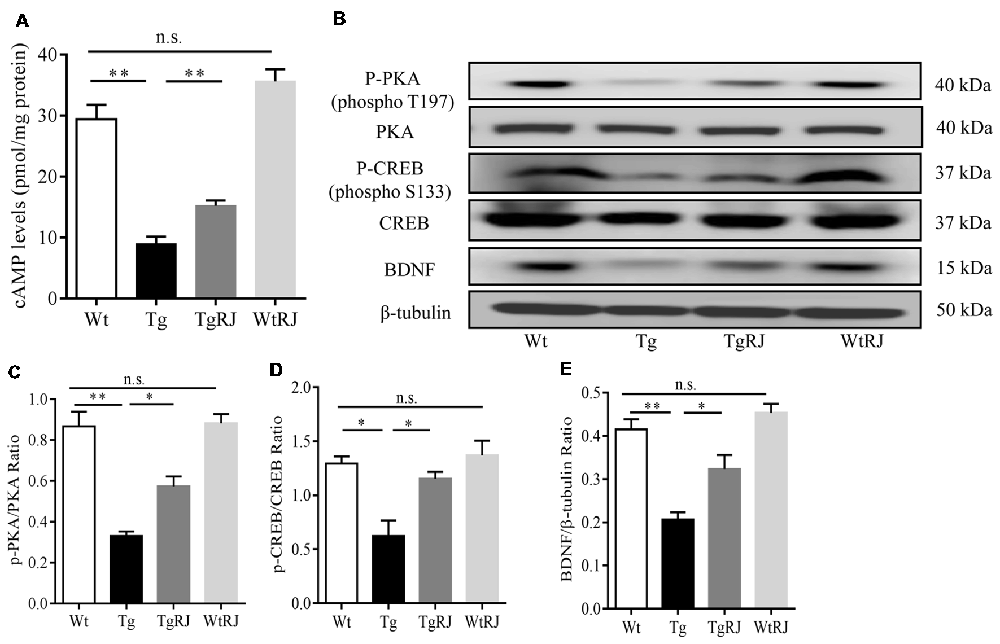
Figure 7. RJ treatment stimulated the cAMP/PKA/CREB/BDNF pathway in the hippocampus of APP/PS1 mice. The protein expression levels of cAMP, p-PKA, PKA, p-CREB, CREB and brain-derived nerve factor (BDNF) were examined using Western blot (A–E). n = 6–10 mice per group. Data are presented as the mean ± SEM. *P < 0.05, **P < 0.01, n.s. non-significant.
Discussion
APP/PS1 mice were structured based on Aβ pathology, learning-memory deficits accompanied by detectable cerebral Aβ at 4 months, significant amyloidosis at 6–7 months, and further aggravates pathology at 10 months (Blanchard et al., 2003; Jankowsky et al., 2004). Hence, in the present study, 10-month-old APP/PS1 mice were used as AD models. We conducted MWM test after 2 months of administration RJ and the results showed that spatial learning and memory functions were impaired in APP/PS1 mice, which was in accordance with previous studies (Liu et al., 2014). However, the learning and memory functions of RJ-treated APP/PS1 mice showed no significant improvement compared with saline-treated APP/PS1 mice (Supplementary Figure S4). Thus, we prolonged the RJ-treated time to 3 months. The hippocampus, entorhinal cortex and cingulate cortex are three important areas for spatial memory function and transformation of short-term memory to long-term memory, and damage in these regions is associated with memory loss in AD (Khan et al., 2014; López et al., 2014; Chang et al., 2016). Thus, it is possible that RJ exerts a protective effect on learning and memory functions by inhibiting or repairing hippocampal and cortical lesions in AD brains.
The hippocampus and cortex are vulnerable to damage in the brains of AD patients, and this damage can lead to pathologic changes (Fjell et al., 2014). In view of this, we conducted IHC staining to explore whether RJ could work against the deposition of Aβ in the hippocampus and cortex of AD brains. In line with the behavioral studies, we observed that RJ-treated APP/PS1 mice had significantly less Aβ deposition than vehicle-treated APP/PS1 mice both in the hippocampus and cortex. The imbalance of Aβ production and degradation is an important causative factor in AD progression (Zhu et al., 2013). In this light, we examined the APP proteolytic pathway, the Aβ catalytic pathway and the Aβ transportation pathway in this study. Interestingly, our results showed that the protective effect of RJ could be partly attributed to the increased levels of LRP-1. This result is particularly significant as the efflux transport of Aβ across the BBB is the most crucial prerequisite to Aβ clearance (Erickson and Banks, 2013). In future studies, we will use LRP-1 knockout APP/PS1 mice to completely clarify the role of LRP-1 in the RJ-induced clearance of Aβ. BACE1 and γ-secretase are needed for the amyloidogenic pathological processing of APP to generate Aβ fragments (Willem et al., 2015). Our results showed that the expression of BACE1 was greatly increased in APP/PS1 mice compared with Wt mice. However, it has been reported that there are no differences in the production rate of Aβ in the brains of AD patients and cognitively normal controls (Mawuenyega et al., 2010). Our results led to the hypothesis that γ-secretase may be the rate-limiting enzyme and its expression may remain unchanged in APP/PS1 mice when compared with wild-type mice. The underlying mechanisms of this divergence need further exploration.
Aβ strongly correlates with oxidative stress-related pathology, including cellular dysfunction and death, and subsequent cognitive impairment. Oxidative stress increases the amount of APP and then further aggravates AD pathology (Jiang et al., 2016). This study verified that oxidative stress is a therapeutic target of RJ in AD. AMP N1-oxide, a unique compound not found in natural products other than RJ, has important neurothropic activity in brain function. It could induce the generation of neurites and inhibit cell growth through adenosine A2A receptor-mediated protein kinase A (PKA) signaling and potentiate the development of astrocytes (Hattori et al., 2010a). Additionally, 10-HDA could easily cross the BBB and exert unique neuromodulatory activity due to its smaller size. Importantly, 10-HDA initiates neurogenesis by neural stem/progenitor cells (Hattori et al., 2007a). Thus, one hypothesis is that AMP N1-oxide and 10-HDA are active components in RJ that alleviate cognitive deficits and Aβ accumulation in the APP/PS1 mouse model. In AD brains, activation of c-JNK has been demonstrated in neurons and dystrophic neurites (Shoji et al., 2000). It has been described that JNK signaling induces activator protein (AP)-1-dependent Bax and caspase activation, which results in neuronal apoptosis (Putcha et al., 2003). Inhibition of the JNK pathway significantly reduced the toxicity attributable to Aβ. Our results showed that RJ inhibited JNK-induced neuronal apoptosis, which may be a mechanism underlying the ameliorative effect of RJ on cognitive deficits.
Previous studies reported that cAMP signal transduction is disrupted in AD brains, and cAMP signal transduction disruption is responsible for memory impairment and neuronal loss (Yamamoto et al., 2000). Decreased cAMP levels inhibit the activation of PKA, followed by reduced levels of phosphorylated cAMP response element binding protein (CREB). BDNF, a downstream gene mediated by CREB, is a neurotrophin with well-established properties of promoting neuronal survival (Chen et al., 2017). Aβ at a sublethal concentration downregulates the BDNF signaling in cultured cortical neurons (Tong et al., 2004). Additionally, preclinical reports have described that AD transgenic mouse models show decreased cortical BDNF expression (Peng et al., 2009). Changes in BDNF levels may lead to cognitive deficits (Nieto et al., 2013). Thus, it is possible that RJ alleviated cognitive dysfunction and neuronal apoptosis by elevating BDNF levels. cAMP plays an important part in the immune process, and the imbalance of cAMP in inflammatory cells leads to inflammatory disorders (Aronoff et al., 2004). Certain inflammatory mediators are potent drivers of AD (Wyss-Coray, 2006). Therefore, increased cAMP levels not only promote BDNF expression but also alleviate neuroinflammation, which may underlie RJ’s protective effect on cognitive function. G protein-coupled receptors (GPCRs) are a superfamily of cell surface receptors. The rhodopsin family of GPCRs represent approximately 85% of the GPCR superfamily. The adenosine receptor consists of four subtypes (A1, A2A, A2B, and A3) whose role is primarily to regulate cAMP accumulation in a wide variety of tissues (Prosser et al., 2017). AMP N1-oxide, a unique compound of RJ, elicited neuronal differentiation of PC12 cells through adenosine A2A receptor-mediated PKA signaling (Hattori et al., 2007b). Thus, we made a preliminary speculation that the unregulation of cAMP pathway may be supported by A2A signal-mediated activation of CREB.
In summary, our data demonstrate that RJ substantially improved cognitive deficits and reduced SPs of APP/PS1 mice via stimulation of the cAMP/PKA/CREB/BDNF pathway and inhibition of neuronal apoptosis. Our findings show that RJ may be a promising medicine for AD and lay the foundation for therapeutic development of AD focusing on natural products.
Author Contributions
MY and YP conceived and designed the experiments. MY, YC and YP performed the experiments. MY and YL analyzed the data. YP, MY, JS and YW contributed to the reagents, materials and analysis tools. MY, YL, KW and FH wrote the article.
Funding
The research was supported by National Natural Science Foundation of China (No. 31872431) and the earmarked fund for Modern Agro-industry Technology Research System from the Ministry of Agriculture of China (CARS-44).
Conflict of Interest Statement
The authors declare that the research was conducted in the absence of any commercial or financial relationships that could be construed as a potential conflict of interest.
Abbreviations
AD, Alzheimer’s disease; Aβ, Amyloid-β; SPs, Senile plaques; NFTs, Neurofibrillary tangles; APP, Amyloid precursor protein; BACE1, β-secretase; sAPPβ, Soluble APP peptide-β; CTFβ, C-terminal fragment-β; IDE, Insulin-degrading enzyme; NEP, Neprilysin; RJ, Royal jelly; 10-HDA, Trans-10-hydroxy-2-decenoic acid; MWM, Morris Water Maze; MDA, Malondialdehyde; LRP-1, Lipoprotein receptor-related protein 1; AP, Activator protein; cAMP, Cyclic adenosine monophosphate; PKA, Protein kinase A; CREB, cAMP-response element binding protein; BDNF, Brain-derived nerve factor; TMT, Trimethyltin; STZ, Streptozotocin; GPCRs, G protein-coupled receptors.
Supplementary Material
The Supplementary Material for this article can be found online at: https://www.frontiersin.org/articles/10.3389/fnagi.2018.00428/full#supplementary-material
References
Aronoff, D. M., Canetti, C., and Peters-Golden, M. (2004). Prostaglandin E2 inhibits alveolar macrophage phagocytosis through an E-prostanoid 2 receptor-mediated increase in intracellular cyclic AMP. J. Immunol. 173, 559–565. doi: 10.4049/jimmunol.173.1.559
Ballesta, J. J., del Pozo, C., Castelló-Banyuls, J., and Faura, C. C. (2012). Selective down-regulation of α4β2 neuronal nicotinic acetylcholine receptors in the brain of uremic rats with cognitive impairment. Exp. Neurol. 236, 28–33. doi: 10.1016/j.expneurol.2012.03.020
Bettens, K., Sleegers, K., and Van Broeckhoven, C. (2013). Genetic insights in Alzheimer’s disease. Lancet Neurol. 12, 92–104. doi: 10.1016/S1474-4422(12)70259-4
Blanchard, V., Moussaoui, S., Czech, C., Touchet, N., Bonici, B., Planche, M., et al. (2003). Time sequence of maturation of dystrophic neurites associated with Aβ deposits in APP/PS1 transgenic mice. Exp. Neurol. 184, 247–263. doi: 10.1016/s0014-4886(03)00252-8
Busche, M. A., Grienberger, C., Keskin, A. D., Song, B., Neumann, U., Staufenbiel, M., et al. (2015). Decreased amyloid-β and increased neuronal hyperactivity by immunotherapy in Alzheimer’s models. Nat. Neurosci. 18, 1725–1727. doi: 10.1038/nn.4163
Campion, D., Pottier, C., Nicolas, G., Le Guennec, K. K., and Rovelet-Lecrux, A. (2016). Alzheimer disease: modeling an Aβ-centered biological network. Mol. Psychiatry 21, 861–871. doi: 10.1038/mp.2016.38
Chang, Y. T., Huang, C. W., Chen, N. C., Lin, K. J., Huang, S. H., Chang, W. N., et al. (2016). Hippocampal amyloid burden with downstream fusiform gyrus atrophy correlate with face matching task scores in early stage Alzheimer’s disease. Front. Aging Neurosci. 8:145. doi: 10.3389/fnagi.2016.00145
Chen, S. D., Wu, C. L., Hwang, W. C., and Yang, D. I. (2017). More insight into BDNF against neurodegeneration: anti-apoptosis, anti-oxidation and suppression of autophagy. Int. J. Mol. Sci. 18:E545. doi: 10.3390/ijms18030545
Citron, M. (2010). Alzheimer’s disease: strategies for disease modification. Nat. Rev. Drug. Discov. 9, 387–398. doi: 10.1038/nrd2896
Dong, Y., Tan, J., Cui, M. Z., Zhao, G., Mao, G., Singh, N., et al. (2006). Calpain inhibitor MDL28170 modulates Aβ formation by inhibiting the formation of intermediate Aβ46 and protecting Aβ from degradation. FASEB J. 20, 331–333. doi: 10.1096/fj.05-4524fje
Doody, R. S., Thomas, R. G., Farlow, M., Iwatsubo, T., Vellas, B., Joffe, S., et al. (2014). Phase 3 trials of solanezumab for mild-to-moderate Alzheimer’s disease. N. Engl. J. Med. 370, 311–321. doi: 10.1056/NEJMoa1312889
Erickson, M. A., and Banks, W. A. (2013). Blood-brain barrier dysfunction as a cause and consequence of Alzheimer’s disease. J. Cereb. Blood Flow Metab. 33, 1500–1513. doi: 10.1038/jcbfm.2013.135
Fjell, A. M., McEvoy, L., Holland, D., Dale, A. M., and Walhovd, K. B. (2014). What is normal in normal aging? effects of aging, amyloid and Alzheimer’s disease on the cerebral cortex and the hippocampus. Prog. Neurobiol. 117, 20–40. doi: 10.1016/j.pneurobio.2014.02.004
Gauthier, S., Feldman, H. H., Schneider, L. S., Wilcock, G. K., Frisoni, G. B., Hardlund, J. H., et al. (2016). Efficacy and safety of tau-aggregation inhibitor therapy in patients with mild or moderate Alzheimer’s disease: a randomised, controlled, double-blind, parallel-arm, phase 3 trial. Lancet 388, 2873–2884. doi: 10.1016/S0140-6736(16)31275-2
Guo, H., Kouzuma, Y., and Yonekura, M. (2009). Structures and properties of antioxidative peptides derived from royal jelly protein. Food Chem. 113, 238–245. doi: 10.1016/j.foodchem.2008.06.081
Guo, C., Zhang, Y. X., Wang, T., Zhong, M. L., Yang, Z. H., Hao, L. J., et al. (2015). Intranasal deferoxamine attenuates synapse loss via up-regulating the P38/HIF-1α pathway on the brain of APP/PS1 transgenic mice. Front. Aging Neurosci. 7:104. doi: 10.3389/fnagi.2015.00104
Hardy, J., and Selkoe, D. J. (2002). The amyloid hypothesis of Alzheimer’s disease: progress and problems on the road to therapeutics. Science 297, 353–356. doi: 10.1126/science.1072994
Hattori, N., Nomoto, H., Fukumitsu, H., Mishima, S., and Furukawa, S. (2007a). Royal jelly and its unique fatty acid, 10-hydroxy-trans-2-decenoic acid, promote neurogenesis by neural stem/progenitor cells in vitro. Biomed. Res. 28, 261–266. doi: 10.2220/biomedres.28.261
Hattori, N., Nomoto, H., Fukumitsu, H., Mishima, S., and Furukawa, S. (2007b). Royal jelly-induced neurite outgrowth from rat pheochromocytoma PC12 cells requires integrin signal independent of activation of extracellular signal-regulated kinases. Biomed. Res. 28, 139–146. doi: 10.2220/biomedres.28.139
Hattori, N., Nomoto, H., Fukumitsu, H., Mishima, S., and Furukawa, S. (2010a). AMP N(1)-oxide, a unique compound of royal jelly, induces neurite outgrowth from PC12 cells via signaling by protein kinase a independent of that by mitogen-activated protein kinase. Evid. Based. Complement. Alternat. Med 7, 63–68. doi: 10.1093/ecam/nem146
Hattori, N., Ohta, S., Sakamoto, T., Mishima, S., and Furukawa, S. (2010b). Royal jelly facilitates restoration of the cognitive ability in trimethyltin-intoxicated mice. Evid. Based. Complement. Alternat. Med 2011:165968. doi: 10.1093/ecam/nep029
Honda, Y., Araki, Y., Hata, T., Ichihara, K., Ito, M., Tanaka, M., et al. (2015). 10-Hydroxy-2-decenoic acid, the major lipid component of royal jelly, extends the lifespan of Caenorhabditis elegans through dietary restriction and target of rapamycin signaling. J. Aging Res. 2015:425261. doi: 10.1155/2015/425261
Jankowsky, J. L., Slunt, H. H., Gonzales, V., Jenkins, N. A., Copeland, N. G., and Borchelt, D. R. (2004). APP processing and amyloid deposition in mice haplo-insufficient for presenilin 1. Neurobiol. Aging 25, 885–892. doi: 10.1016/j.neurobiolaging.2003.09.008
Jiang, T., Sun, Q., and Chen, S. (2016). Oxidative stress: a major pathogenesis and potential therapeutic target of antioxidative agents in Parkinson’s disease and Alzheimer’s disease. Prog. Neurobiol. 147, 1–19. doi: 10.1016/j.pneurobio.2016.07.005
Khan, U. A., Liu, L., Provenzano, F. A., Berman, D. E., Profaci, C. P., Sloan, R., et al. (2014). Molecular drivers and cortical spread of lateral entorhinal cortex dysfunction in preclinical Alzheimer’s disease. Nat. Neurosci. 17, 304–311. doi: 10.1038/nn.3606
Kowalczuk, K., and Stryjeckazimmer, M. (2002). The influence of oxidative stress on the level of malondialdehyde (MDA) in different areas of the rabbit brain. Ann. Univ. Mariae. Curie. Skłodowska. Med. 57, 160–164.
Liu, M., Chen, F., Sha, L., Wang, S., Tao, L., Yao, L., et al. (2014). (-)-Epigallocatechin-3-gallate ameliorates learning and memory deficits by adjusting the balance of TrkA/p75NTR signaling in APP/PS1 transgenic mice. Mol. Neurobiol. 49, 1350–1363. doi: 10.1007/s12035-013-8608-2
López, M. E., Bruña, R., Aurtenetxe, S., Pineda-Pardo, J. Á., Marcos, A., Arrazola, J., et al. (2014). Alpha-band hypersynchronization in progressive mild cognitive impairment: a magnetoencephalography study. J. Neurosci. 34, 14551–14559. doi: 10.1523/JNEUROSCI.0964-14.2014
Mancuso, M., Orsucci, D., LoGerfo, A., Calsolaro, V., and Siciliano, G. (2010). Clinical features and pathogenesis of Alzheimer’s disease: involvement of mitochondria and mitochondrial DNA. Adv. Exp. Med. Biol. 685, 34–44. doi: 10.1007/978-1-4419-6448-9_4
Mangialasche, F., Solomon, A., Winblad, B., Mecocci, P., and Kivipelto, M. (2010). Alzheimer’s disease: clinical trials and drug development. Lancet Neurol. 9, 702–716. doi: 10.1016/S1474-4422(10)70119-8
Mawuenyega, K. G., Sigurdson, W., Ovod, V., Munsell, L., Kasten, T., Morris, J. C., et al. (2010). Decreased clearance of CNS β-amyloid in Alzheimer’s disease. Science 330:1774. doi: 10.1126/science.1197623
Mukherjee, A., Song, E., Kihiko-Ehmann, M., Goodman, J. P. Jr., Pyrek, J. S., Estus, S., et al. (2000). Insulysin hydrolyzes amyloid β peptides to products that are neither neurotoxic nor deposit on amyloid plaques. J. Neurosci. 20, 8745–8749. doi: 10.1523/jneurosci.20-23-08745.2000
Nicoll, J. A. R., Wilkinson, D., Holmes, C., Steart, P., Markham, H., and Weller, R. O. (2003). Neuropathology of human Alzheimer disease after immunization with amyloid-β peptide: a case report. Nat. Med. 9, 448–452. doi: 10.1038/nm840
Nieto, R., Kukuljan, M., and Silva, H. (2013). BDNF and schizophrenia: from neurodevelopment to neuronal plasticity, learning and memory. Front. Psychiatry 4:45. doi: 10.3389/fpsyt.2013.00045
Pan, Y., Xu, J., Chen, C., Chen, F., Jin, P., Zhu, K., et al. (2018). Royal jelly reduces cholesterol levels, ameliorates Aβ pathology and enhances neuronal metabolic activities in a rabbit model of Alzheimer’s disease. Front. Aging Neurosci. 10:50. doi: 10.3389/fnagi.2018.00050
Peng, S., Garzon, D. J., Marchese, M., Klein, W., Ginsberg, S. D., Francis, B. M., et al. (2009). Decreased brain-derived neurotrophic factor depends on amyloid aggregation state in transgenic mouse models of Alzheimer’s disease. J. Neurosci. 29, 9321–9329. doi: 10.1523/JNEUROSCI.4736-08.2009
Prince, M., Wimo, A., Guerchet, M., Ali, G.-C., Wu, Y.-T., and Prina, M. (2015). “World Alzheimer Report 2015,” in The Global Impact of Dementia-an Analysis of Prevalence, Incidence, Cost and Trends, London: Alzheimer’s Disease International.
Prosser, R. S., Ye, L., Pandey, A., and Orazietti, A. (2017). Activation processes in ligand-activated G protein-coupled receptors: a case study of the adenosine A2A receptor. Bioessays 39:1700072. doi: 10.1002/bies.201700072
Putcha, G. V., Le, S., Frank, S., Besirli, C. G., Clark, K., Chu, B., et al. (2003). JNK-mediated BIM phosphorylation potentiates BAX-dependent apoptosis. Neuron 38, 899–914. doi: 10.1016/s0896-6273(03)00355-6
Reddy, P. H., Manczak, M., Yin, X., Grady, M. C., Mitchell, A., Tonk, S., et al. (2018). Protective effects of indian spice curcumin against amyloid β in alzheimer’s disease. J. Alzheimers Dis. 61, 843–866. doi: 10.3233/JAD-170512
Sabatini, A. G., Marcazzan, G. L., Caboni, M. F., Bogdanov, S., and Almeida-Muradian, L. B. D. (2009). Quality and standardisation of royal jelly. J. Apiproduct Apimedical Sci. 1, 16–21. doi: 10.3896/ibra.4.01.1.04
Salloway, S., Sperling, R., Gilman, S., Fox, N. C., Blennow, K., Raskind, M., et al. (2009). A phase 2 multiple ascending dose trial of bapineuzumab in mild to moderate Alzheimer disease. Neurology 73, 2061–2070. doi: 10.1212/WNL.0b013e3181c67808
Seung, T. W., Park, S. K., Kang, J. Y., Kim, J. M., Park, S. H., Kwon, B. S., et al. (2018). Ethyl acetate fraction from hibiscus sabdariffa L. attenuates diabetes-associated cognitive impairment in mice. Food Res. Int. 105, 589–598. doi: 10.1016/j.foodres.2017.11.063
Shirotani, K., Tsubuki, S., Iwata, N., Takaki, Y., Harigaya, W., Maruyama, K., et al. (2001). Neprilysin degrades both amyloid β peptides 1–40 and 1-42 most rapidly and efficiently among thiorphan- and phosphoramidon-sensitive endopeptidases. J. Biol. Chem. 276, 21895–21901. doi: 10.1074/jbc.M008511200
Shoji, M., Iwakami, N., Takeuchi, S., Waragai, M., Suzuki, M., Kanazawa, I., et al. (2000). JNK activation is associated with intracellular β-amyloid accumulation. Brain Res. Mol. Brain Res. 85, 221–233. doi: 10.1016/s0169-328x(00)00245-x
Tanzi, R. E., and Bertram, L. (2005). Twenty years of the Alzheimer’s disease amyloid hypothesis: a genetic perspective. Cell 120, 545–555. doi: 10.1016/j.cell.2005.02.008
Tong, L., Balazs, R., Thornton, P. L., and Cotman, C. W. (2004). β-amyloid peptide at sublethal concentrations downregulates brain-derived neurotrophic factor functions in cultured cortical neurons. J. Neurosci. 24, 6799–6809. doi: 10.1523/JNEUROSCI.5463-03.2004
Wang, X., Cao, M., and Dong, Y. (2016). Royal jelly promotes DAF-16-mediated proteostasis to tolerate β-amyloid toxicity in C. elegans model of Alzheimer’s disease. Oncotarget 7, 54183–54193. doi: 10.18632/oncotarget.10857
Wang, X., Cook, L. F., Grasso, L. M., Cao, M., and Dong, Y. (2015). Royal jelly-mediated prolongevity and stress resistance in caenorhabditis elegans is possibly modulated by the interplays of DAF-16, SIR-2.1, HCF-1 and 14–3-3 proteins. J. Gerontol. 70, 827–838. doi: 10.1093/gerona/glu120
Willem, M., Tahirovic, S., Busche, M. A., Ovsepian, S. V., Chafai, M., Kootar, S., et al. (2015). η-Secretase processing of APP inhibits neuronal activity in the hippocampus. Nature 526, 443–447. doi: 10.1038/nature14864
Wyss-Coray, T. (2006). Inflammation in Alzheimer disease: driving force, bystander or beneficial response? Nat. Med. 12, 1005–1015. doi: 10.1038/nm1484
Yamamoto, M., Götz, M. E., Ozawa, H., Luckhaus, C., Saito, T., Rosler, M., et al. (2000). Hippocampal level of neural specific adenylyl cyclase type I is decreased in Alzheimer’s disease. Biochim. Biophys. Acta 1535, 60–68. doi: 10.1016/s0925-4439(00)00083-1
You, M. M., Chen, Y. F., Pan, Y. M., Liu, Y. C., Tu, J., Wang, K., et al. (2018). Royal jelly attenuates LPS-induced inflammation in BV-2 microglial cells through modulating NF-κB and p38/JNK signaling pathways. Mediators Inflamm. 2018:7834381. doi: 10.1155/2018/7834381
Youn, K., Lee, S., and Jun, M. (2018). Gamma -linolenic acid ameliorates Aβ-induced neuroinflammation through NF-κB and MAPK signalling pathways. J. Funct. Foods 42, 30–37. doi: 10.1016/j.jff.2017.12.065
Zamani, Z., Reisi, P., Alaei, H., and Pilehvarian, A. A. (2012). Effect of royal jelly on spatial learning and memory in rat model of streptozotocin-induced sporadic Alzheimer’s disease. Adv. Biomed. Res. 1:26. doi: 10.4103/2277-9175.98150
Zhang, Q. Q., Shi, J. Q., Xu, J., Cheng, X. X., Zhu, H. Q., and Chen, J. (2013). Application of thioflavin T staining in detection of β-amyloid. Chin. J. Pathol. 42:764.
Keywords: royal jelly, Alzheimer’s disease, cognitive deficits, amyloid-β, cAMP-response element binding protein, apoptosis
Citation: You M, Pan Y, Liu Y, Chen Y, Wu Y, Si J, Wang K and Hu F (2019) Royal Jelly Alleviates Cognitive Deficits and β-Amyloid Accumulation in APP/PS1 Mouse Model Via Activation of the cAMP/PKA/CREB/BDNF Pathway and Inhibition of Neuronal Apoptosis. Front. Aging Neurosci. 10:428. doi: 10.3389/fnagi.2018.00428
Received: 01 October 2018; Accepted: 11 December 2018;
Published: 04 January 2019.
Edited by:
Antonio Camins, University of Barcelona, SpainReviewed by:
Heike Rebholz, City College of New York (CUNY), United StatesMurali Vijayan, Texas Tech University Health Sciences Center, United States
Copyright © 2019 You, Pan, Liu, Chen, Wu, Si, Wang and Hu. This is an open-access article distributed under the terms of the Creative Commons Attribution License (CC BY). The use, distribution or reproduction in other forums is permitted, provided the original author(s) and the copyright owner(s) are credited and that the original publication in this journal is cited, in accordance with accepted academic practice. No use, distribution or reproduction is permitted which does not comply with these terms.
*Correspondence: Fuliang Hu, ZmxodUB6anUuZWR1LmNu