- 1Department of Neurology, Nanjing Drum Tower Hospital, Nanjing University Medical School, Nanjing, China
- 2Jiangsu Key Laboratory for Molecular Medicine, Nanjing University Medical School, Nanjing, China
- 3Jiangsu Province Stroke Center for Diagnosis and Therapy, Nanjing, China
- 4Nanjing Neuropsychiatry Clinic Medical Center, Nanjing, China
Background and Objective: Subjective cognitive decline (SCD) is considered a preclinical state of Alzheimer’s disease (AD) and may represent a more advanced preclinical status than amnestic mild cognitive impairment (aMCI). Our aim was to explore changes in the white matter (WM) microstructure and their correlation with cognitive function in these AD-spectrum patients.
Methods: Diffusion tensor images from 43 individuals with normal cognition (NC), 38 SCD patients, and 36 aMCI patients were compared using an atlas-based segmentation strategy. The correlation between diffusion parameters and cognitive function was further analyzed.
Results: The anatomical pattern of WM impairment was generally similar between SCD and aMCI patients. However, aMCI patients showed significantly lower fractional anisotropy (i.e., corpus callosum forceps major and forceps minor) and increased mean diffusivity [i.e., bilateral anterior thalamic radiation (ATR), left corticospinal tract (CST), forceps minor, left cingulum (cingulate gyrus), left cingulum hippocampus, and left inferior fronto-occipital fasciculus (IFO)] in some tracts than did SCD subjects, indicating a disruption in WM microstructural integrity in the aMCI. Individuals with microstructural disruption in forceps minor, left cingulum (cingulate gyrus), and left cingulum hippocampus tracts performed worse in general cognition and memory function tests, as indicated by line regression analysis.
Conclusion: SCD individuals had extensive WM microstructural damage in a pattern similar to that seen in aMCI, although presenting a cognitive performance comparable with that of cognitively healthy individuals. Our results suggest that WM integrity might precede objectively measurable memory decline and may be a potential early biomarker for AD.
Introduction
Alzheimer’s disease (AD) is a neurodegenerative disorder characterized by progressive cognitive impairment and the loss of daily living abilities, and its pathological processes are thought to occur decades before clinical symptoms were detectable. Amnestic mild cognitive impairment (aMCI), a transitional zone between normal ageing and clinically probable AD, has a dementia conversion rate as high as 10–15% per year (Petersen, 2004). Threatened by the lack of curative treatments for AD and aMCI, advancing the time window for intervention would offer an alternative opportunity to slow down or reverse the deterioration.
Subjective cognitive decline (SCD) refers to the subjective experience of cognitive decline in memory and/or other cognitive functions, without any objective evidence of cognitive impairment (Jessen et al., 2014). Given that elderly people with AD usually experience SCD prior to the onset of dementia, SCD has been regarded as the earliest stage in the continuous progression to AD (Reisberg et al., 2008; Hafkemeijer et al., 2013). Subjective decline in memory vs. in other cognitive domains could result in a higher risk of conversion to subsequent aMCI or AD (Rönnlund et al., 2015; Buckley et al., 2016). A recent review has shown that compared with age-matched controls, individuals with SCD suffered a 4.5-fold higher risk of progressing to subsequent MCI, and the risk of eventual progression to AD increased by 6.5-fold (Lin et al., 2019). Another meta-analysis has suggested that approximately 25% of elderly people with SCD will develop MCI due to AD in the next 4 years (Mitchell et al., 2014). Because, SCD may be the first symptom in the pathological cascade of AD, it is of great value to identify AD-related pathological markers in the SCD population.
Previous studies using cerebrospinal fluid (CSF), structural or functional magnetic resonance imaging (MRI), fluorodeoxyglucose positron emission tomography (FDG-PET), and Pittsburgh compound B-positron emission tomography (PiB-PET) have provided substantial evidence that SCD is linked to underlying AD pathology (Sun et al., 2015; Cheng et al., 2017). For example, decreased amyloid beta protein 42 (Aβ-42) and elevated tau level in CSF were found in patients with SCD (Visser et al., 2009). SCD patients also tend to present AD/aMCI-like gray matter atrophy, decreased metabolism, and increased amyloid load, especially in the hippocampus (Vannini et al., 2017; Zhao et al., 2019).
Although less studied, white matter (WM) degeneration has also been demonstrated as an important feature in the spectrum of AD, as identified by histological examination and neuroimaging studies (Caso et al., 2016). A meta-analysis of diffusion tensor imaging (DTI) research revealed that WM microstructural alterations in aMCI and AD were widespread throughout the brain (Sexton et al., 2011), particularly in direct connections from the ventromedial frontal cortex to the medial temporal lobe (MTL) and from the precuneus to the MTL by the way of retrosplenial cortex (Salat et al., 2010). To a certain extent, these regional tissue changes resembled the anatomic connectivity of MTL structures, which have almost equal effects on memory processes, whereas the executive function alterations are related to the frontal-temporal (especially MTL) WM microstructural damage (Sui and Rajapakse, 2018). Cingulum bundle and fornix are the core WM tracts connecting directly to the MTL. The cingulum bundle is a prominent tract that interconnects the frontal, parietal, and medial temporal sites while also connecting the subcortical nuclei to the cingulate gyrus (Bubb et al., 2018). It is mainly responsible for communications between components of the limbic system, which is vital for normal cognition (Burgel et al., 2006). Impaired integrity of the cingulum microstructure was detected in aMCI and AD, which correlated with memory, attention/executive function, language, and visuo-spatial function impairment (Choo et al., 2010; Wang et al., 2012; Kantarci et al., 2017). Fornix projects from the hippocampus to other brain regions and is also responsible for episodic memory loss in older adults with AD and aMCI (Copenhaver et al., 2006). Actually, the pattern of WM alterations seen in DTI was also anatomically concordant with that of gray matter atrophy, initially starting from the limbic bundles (i.e., MTL) and gradually progressing to the temporal lobe, parietal lobe, and frontal lobe WM tracts (Nowrangi et al., 2013; Konukoglu et al., 2016) as the clinical AD disease progresses. Moreover, this pattern of diffusion abnormalities is associated with the neurofibrillary tangle pathology stage, as well as clinical disease severity (Kantarci et al., 2017). Regarding the association between SCD and preclinical AD dementia, we speculated that WM microstructural changes may occur as early as SCD, yet the characteristics remain to be further investigated.
DTI is an advanced MRI quantitative technique for studying the microstructure of WM by utilizing the diffusion motion characteristics of water molecule interactions in tissues. It can sensitively detect the destruction of the WM microstructure such as demyelination, axonal injury, edema, or necrosis (Shaikh et al., 2018). Fractional anisotropy (FA) and mean diffusivity (MD) are two commonly employed diffusion parameters in the analysis of DTI data. FA reflects the directional variation of water molecule diffusion measured in the different directions (Soares et al., 2013; Shaikh et al., 2018), whereas MD represents the average diffusivity (Soares et al., 2013; Shaikh et al., 2018). The FA decrease and MD increase are parallel to the loss of myelin and axonal membranes that restrict random motion of water molecules along the WM tracts. Together, alterations in FA and MD indicate disruption in WM microstructural integrity. AD subjects had lower FA and higher MD within the temporal, parietal, and prefrontal lobes than had cognitively healthy aging adults in a large body of studies (Sexton et al., 2011; Acosta-Cabronero and Nestor, 2014). Therefore, we expect a similar pattern to be found in aMCI and SCD. The purpose of this study was to explore the differences in WM integrity among cognitively normal elderly, aMCI, and SCD and to analyze the correlation between WM integrity and cognitive function.
Materials and Methods
Participants
A total of 117 right-handed Chinese Han subjects (43 normal controls and 38 SCD and 36 aMCI patients) were enrolled in this study at the Department of Neurology of the Nanjing Drum Tower Hospital of Nanjing University Medical School from June 2016 to May 2019. This study was approved by the ethics committees of the Nanjing Drum Tower Hospital of Nanjing University Medical School (clinical trial government identifier: NCT01364246), and written informed consent was obtained from each subject prior to participation. All subjects underwent a comprehensive neuropsychological test and 3.0-T whole brain MRI scanning, a routine blood test, and a general medical examination by an experienced neurologist. The cognitive functions of all the subjects were evaluated by an experienced neuropsychologist using the Chinese version of the Mini-Mental State Examination (MMSE) and the Beijing version of Montreal Cognitive Assessment (MoCA; Lu et al., 2011) as general cognitive function screening; the auditory verbal learning test Huashan version (AVLT) as an episodic memory function assessment (Zhao et al., 2012); and the Clinical Dementia Rating (CDR) scale, activities of daily living (ADL) assessment, Hamilton Depression Rating Scale (HAMD), and Hamilton Anxiety Rating Scale (HAMA). The inclusion criteria for normal control group were as follows: (1) no reported cognition complaints; (2) normal MMSE, MoCA, and AVLT scores adjusted for age and education; and (3) CDR score = 0 and ADL score = 8. Patients with SCD were diagnosed based on the research criteria for pre-MCI SCD (Jessen et al., 2014): (1) self-reported experience of continuous decline in memory (within the last 5 years); (2) MMSE, MoCA, and AVLT test scores all within the normal range after adjusting for age and years of education; and (3) CDR score = 0 and ADL score = 8. The Petersen criteria were applied in the diagnosis of aMCI (Petersen, 2004): (1) subjective memory complaint confirmed by an informant; (2) objective memory impairment detected by the MoCA or AVLT (at least 1.5 standard deviations below normative values for age and/or education); (3) preserved general cognitive function (MMSE ≥ 24); (4) CDR score = 0.5; (5) ADL score = 8; and (6) failure to meet the criteria for dementia according to National Institute on Aging and the Alzheimer’s Association (NIA-AA) criteria. Exclusion criteria for all the subjects were as follows: (1) age less than 50 years old; (2) a history of stroke; (3) central nervous system diseases that could cause cognitive decline, including vascular dementia, Parkinson’s disease, epilepsy, central nervous system infection, subarachnoid hemorrhage, or multiple sclerosis; (4) the presence of WM lesions equal to or higher than Fazekas grade II (Wahlund et al., 2001); (5) severe depression (HAMD ≥ 17), anxiety (HAMA ≥ 21), schizophrenia or other mental illness; (6) severe systemic diseases such as heart failure and kidney dysfunction; (7) history of drugs or alcohol abuse; (8) intolerance of MRI examination or inability to complete neuropsychological testing; and (9) other diseases that may affect cognition.
Image Acquisition
All MRI scans were acquired using 3.0-T scanner [Achieva 3.0 T TX (eight-channel head coil) or 3.0 T Ingenia (32-channel head coil), Philips, Eindhoven, Netherlands] at Nanjing Drum Tower Hospital. Subjects were placed in the supine position. Sagittal T1-weighted MR images were performed by a three-dimensional turbo fast echo acquisition with the following parameters: repetition time (TR) = 9.8 ms, echo time (TE) = 4.6 ms, inversion time (TI) = 900 ms, flip angle = 8°, field of view (FOV) = 256 × 256 mm, matrix = 256 × 256, number of slices = 192, and slice thickness = 1 mm. DTI data were obtained using an echo planar imaging (EPI) sequence with the following parameters: in 32 non-collinear directions diffusion encoding (b = 1,000 s/mm2 for each direction) and one image with no diffusion weighting (b = 0 s/mm2), TR = 9,154 ms, TE = 55 ms, flip angle = 90°, matrix size = 112 × 112, FOV = 224 × 224 mm, and slice thickness = 2.5 mm. The total scan lasted 13 min. Additionally, axial T2-weighted, diffusion-weighted imaging (DWI) sequence, and fluid-attenuated inversion recovery (FLAIR) sequence were collected to detect acute or subacute infarctions and visible WM damage.
Diffusion Tensor Imaging Processing
The atlas-based segmentation strategy was employed to investigate diffusion abnormalities in this study. The processing of DTI data was carried out with PANDA software, following the default pipeline setting1 (Cui et al., 2013). PANDA is a toolbox to perform analyses and calculations of brain diffusion images, which integrates FSL2, Diffusion Tool kit3, and MRIcron4. The major steps include the following: (1) converting original data (DICOM) to the NIFIT format; (2) removing non-brain tissue; (3) correcting eddy current and head motion; (4) adjusting the diffusion gradient direction; and (5) calculating the diffusion tensor metrics, including FA, MD, radial diffusivity, and axial diffusivity. Finally, the FA images in the native space were nonlinearly registered to the FA standard template in the Montreal Neurological Institute (MNI) space using FSL’s FNIRT command. To observe changes in the major WM tracts, all DTI metrics were registered to the JHU WM Tractography Atlas (Hua et al., 2008). Under the present research, only FA and MD diffusion tensor metrics were discussed. The WM fiber pathways of interest were as follows (Figure 1): anterior thalamic radiation (ATR); CST; cingulum (cingulate gyrus; CgC); cingulum (hippocampus; CgH); corpus callosum (forceps major); corpus callosum (forceps minor); inferior fronto-occipital fasciculus (IFO); inferior longitudinal fasciculus (ILF); superior longitudinal fasciculus (SLF); uncinate fasciculus (UF); and superior longitudinal fasciculus-temporal part (tSLF). All of the tracts were evaluated in both hemispheres, except for the corpus callosum (forceps major) and corpus callosum (forceps minor).
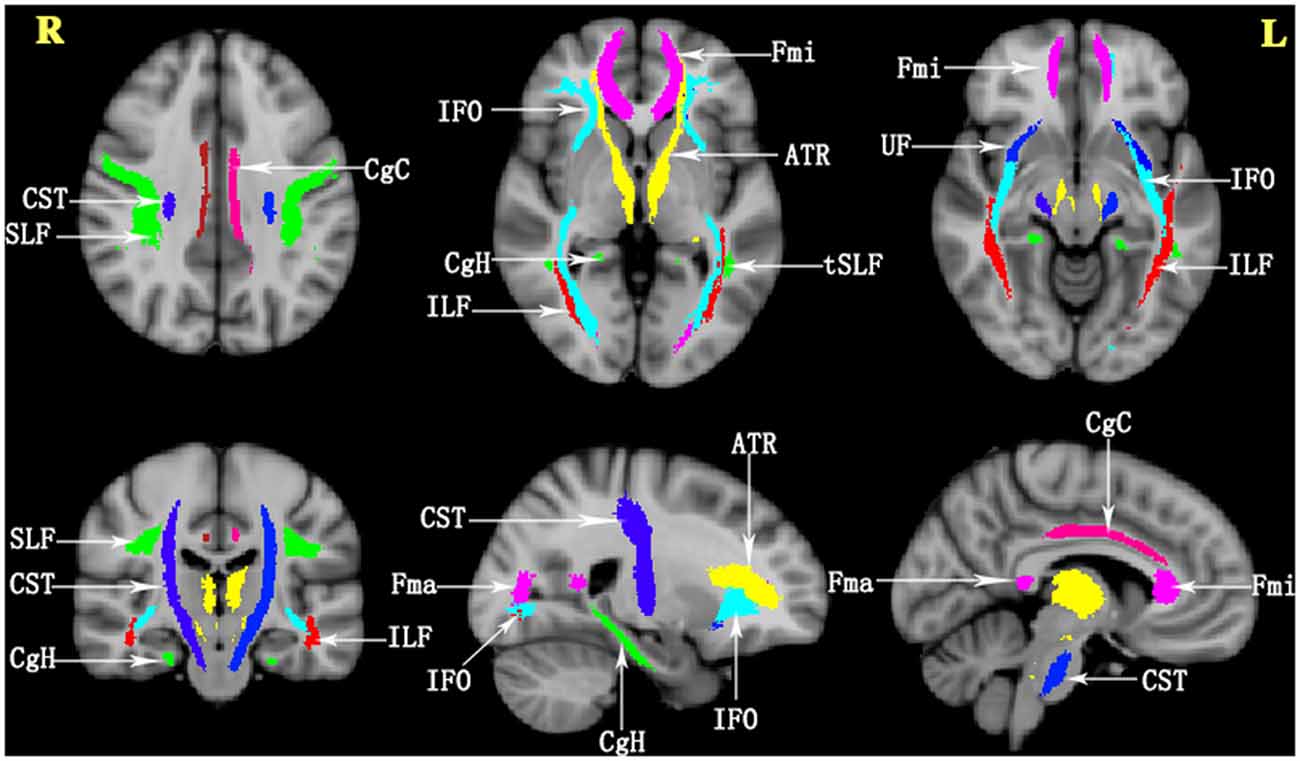
Figure 1. The details of the tracts in the JHU White Matter (WM) Tractography Atlas in the present study. ATR, anterior thalamic radiation; CST, corticospinal tract; CgC, cingulum (cingulate gyrus); CgH, cingulum (hippocampus); Fma, forceps major; Fmi, forceps minor; IFO, inferior fronto-occipital fasciculus; ILF, inferior longitudinal fasciculus; SLF, superior longitudinal fasciculus; UF, uncinate fasciculus; tSLF, superior longitudinal fasciculus-temporal part; L, left; R, right.
Statistical Analysis
All statistical analyses were performed using the Statistical Package for Social Science (SPSS, v20.0)5. The categorization of demographic variables was expressed in terms of frequency and percentage (%), and differences were assessed using the chi-square test. Continuous demographic variables are presented as mean ± standard deviation. One-way analysis of variance (ANOVA) was used to compare the differences in the studied variables (i.e., the results of ADL, HAMA, HAMD, and demographic information) across groups. If the ADL, HAMA, HAMD, and demographic information had significant difference across three groups, Post hoc analyses were performed to further explore the details of these group differences using least significant difference (LSD) pairwise comparisons. Furthermore, analysis of covariance (ANCOVA) was used to analyze the results of the cognitive evaluations (MMSE, MoCA, and AVLT) and the diffusion metrics of FA and MD values difference in 20 WM tracts of interest among three groups, with age, gender, and years of education as covariates. Bonferroni correction was conducted to adjust the false-positive rate (P < 0.05/20), and significant results further underwent pairwise comparison. To investigate the relationship between the cognitive test scores (MMSE, MoCA, AVLT-immediate recall, AVLT-delayed recall, and AVLT-recognition) and the diffusion parameters of FA and MD, the Pearson correlation analysis was initially performed. The tracts with significance were further included in the stepwise multiple linear regression model, with cognitive scores as dependent variables; FA and MD as independent variables; and age, gender, and years of education as covariates. P < 0.05 was considered statistically significant.
Results
Demographic and Neuropsychological Characteristics
The demographic information and neuropsychological performance of the three groups are shown in Table 1. There were no significant differences in years of education, gender, vascular risk factors, family history of dementia, and HAMD and HAMA scores among the three groups. However, the NC group was younger overall than the aMCI and SCD groups (it should be noted that the age effect was removed in all subsequent DTI analyses). The comparisons between SCD and NC groups revealed no significant differences in MMSE, MoCA, AVLT-immediate recall, AVLT-delayed recall, or AVLT-recognition scores. General cognitive function (i.e., MMSE and MoCA scores) and memory ability (i.e., AVLT-immediate recall, AVLT-delayed recall, and AVLT-recognition scores) were significantly lower in the aMCI group than in the SCD and NC groups.
Group Comparisons of Atlas-Based Tracts
In general, the diffusion metrics FA and MD in the SCD group were between those of the NC and aMCI groups, as shown in Tables 2, 3. ANOVA revealed that FA values in the forceps major and forceps minor, as well as MD values in the bilateral ATR, left CST, left CgC, left CgH, forceps minor, and left IFO, were significantly different among the three groups after Bonferroni correction.
Post hoc comparisons showed that the FA values in the forceps major and forceps minor were significantly decreased in both the SCD group and aMCI group relative to those in the NC group (Figure 2; Table 4), whereas MD values were significantly higher in more extensive regions, including in the bilateral ATR, left CST, left CgC, left CgH, forceps minor, and left IFO (Figure 3; Table 4). However, the SCD group, when compared with the aMCI group, presented significantly lower MD values in the left CgH and forceps minor (Figure 3; Table 4).
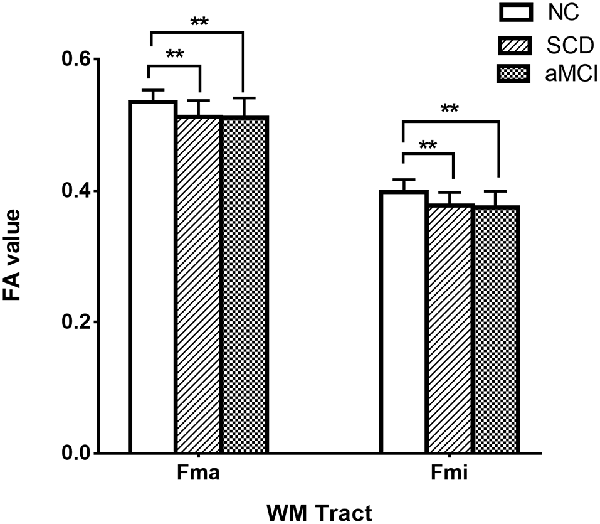
Figure 2. Post hoc analysis of FA values for tracts that remained significant after Bonferroni correction in the three groups. NC, normal control; SCD, subjective cognitive decline; aMCI, amnestic mild cognitive impairment; Fma, forceps major; Fmi, forceps minor; WM, white matter; FA, fractional anisotropy; **P < 0.01.
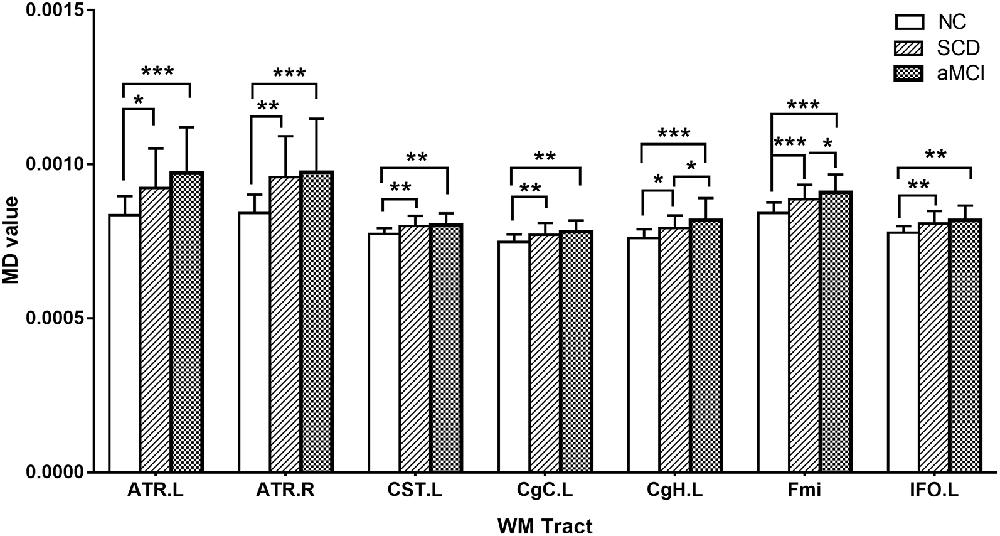
Figure 3. Post hoc analysis of MD values for tracts that remained significant after Bonferroni correction in the three groups. NC, normal control; SCD, subjective cognitive decline; aMCI, amnestic mild cognitive impairment; ATR, anterior thalamic radiation; CST, corticospinal tract; CgC, cingulum (cingulate gyrus); CgH, cingulum (hippocampus); Fmi, forceps minor; IFO, inferior fronto-occipital fasciculus; MD, mean diffusivity; L, left; R, right; WM, white matter; *P < 0.05; **P < 0.01; ***P < 0.001.
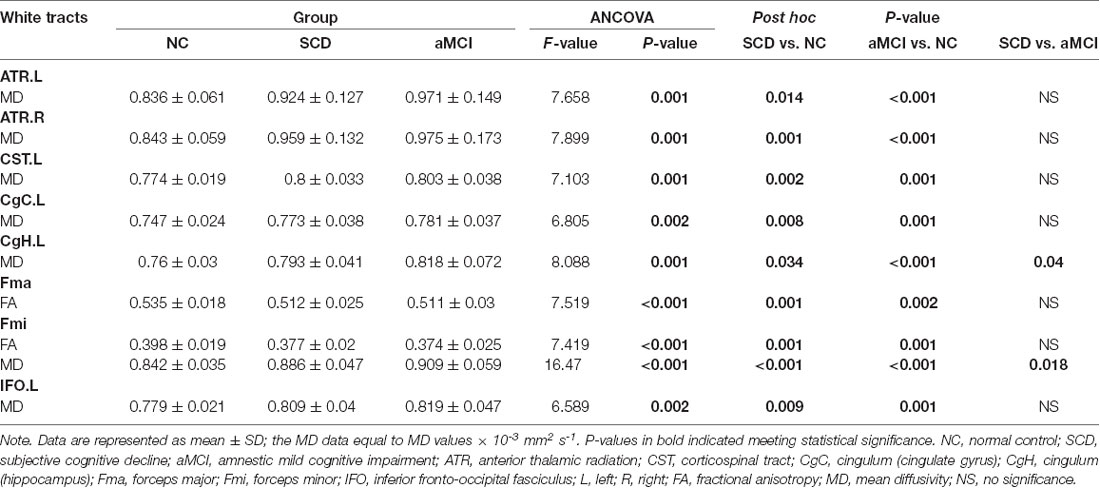
Table 4. Post hoc analysis of the significant tracts surviving Bonferroni correction in the three groups.
Relationship Between Diffusion Metrics and Neuropsychological Scores
The linear regression analysis results between cognitive function scores and FA values in the overall sample are presented in Table 5. In our study, the FA value in the left CgH was independently positively correlated with MMSE (β = 0.335, P < 0.001) and AVLT-immediate recall (β = 0.275, P = 0.002) scores; the FA values in the left CgC were independently correlated with performance on MoCA (β = 0.295, P < 0.001), AVLT-delayed recall (β = 0.339, P < 0.001), and AVLT-recognition (β = 0.296, P < 0.001) assessments.
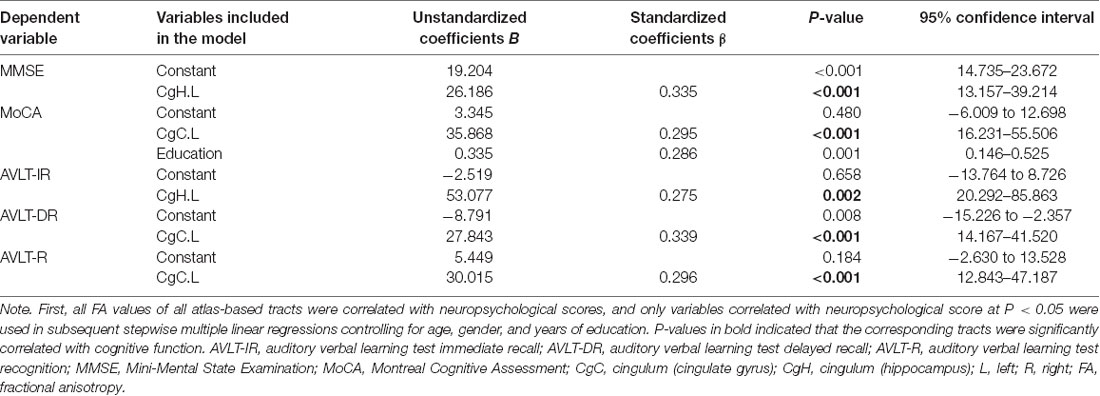
Table 5. Multiple linear regression models for different cognitive scores and correlated FA variables.
In addition, the results of the regression analysis of the cognitive function scores and the MD values within the entire sample are shown in Table 6. MD values in the left CgH were independently negatively correlated with MMSE (β = −1.89, P = 0.04) and MoCA (β = −0.227, P = 0.015) scores. MD values in the left CgC were negatively correlated with MMSE (β = −0.193, P = 0.037) and AVLT-recognition (β = −0.377, P < 0.001) scores. MD values in the forceps minor were independently correlated with MoCA (β = −0.245, P = 0.009), AVLT-immediate recall (β = −0.337, P < 0.001), and AVLT-delayed recall (β = −0.439, P < 0.001) scores.
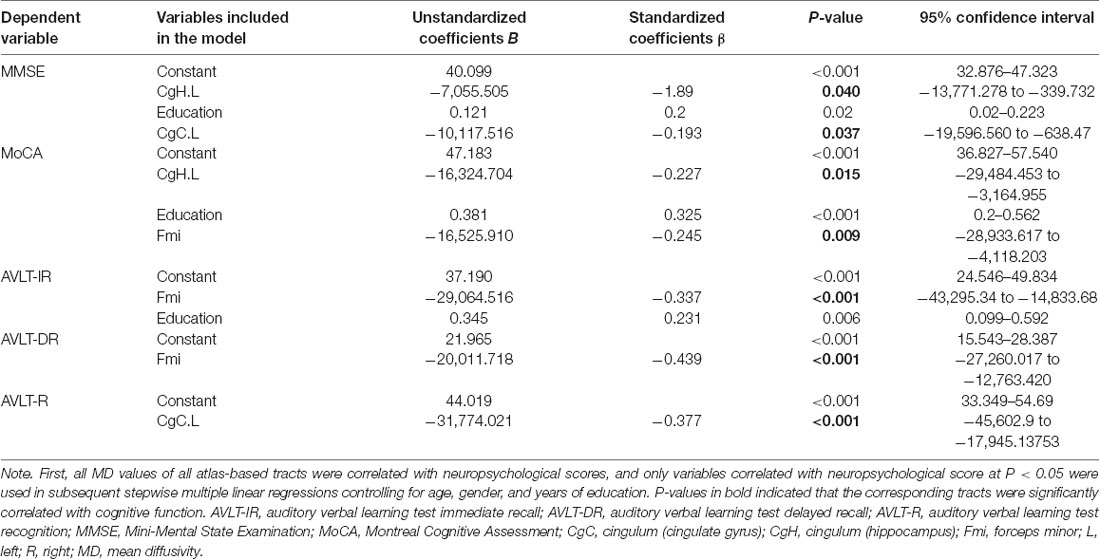
Table 6. Multiple linear regression models for different cognitive scores and correlated MD variables.
Discussion
In the present study, changes in the microstructural integrity of WM in AD-spectrum patients were revealed using DTI metrics. The anatomical pattern of WM impairment was similar in both SCD and aMCI patients, and the damage was less severe in SCD patients than in aMCI patients. In addition, significant correlations between diffusion metrics and cognition suggested that those individuals with WM microstructural disruption performed worse in general cognition and memory function tests. These results supported the previous theory that the DTI index can be a sensitive measure for detecting changes of WM microstructural in AD degenerative processes and can offer a new insight into AD pathophysiology (Caso et al., 2016).
Research interest is shifting to increasingly earlier stages, as the origin of AD and keys to treatment probably lie in the prevention of progression to a fully fledged disease (Slot et al., 2018). It should be noted that SCD has been shown to be the first clinical manifestation of AD, and there is increasing interest in the study of individuals meeting SCD criteria to assess the progression of this disease in the subjects who have a higher risk of cognitive decline (Sánchez-Benavides et al., 2018). Therefore, it is essential to evaluate SCD participant characteristics related to cognitive complaints. In the present study, SCD subjects exhibited significantly lower FA and significantly higher MD across extensive regions, as expected from previous AD-spectrum studies (Caso et al., 2016). Specifically, individuals with SCD showed an intermediate level of microstructure changes between those seen in NC and aMCI patients, whereas the damaging patterns of WM fibers were similar to those seen in aMCI. These brain regions showing compromised WM integrity were parallel to the results of a recent SCD study by Ohlhauser et al., who also discovered widespread significant impairment in superior and inferior longitudinal fasciculi, fronto-occipital fasciculi, and corpus callosum (Ohlhauser et al., 2019). Some previous studies have detected impaired WM integrity in the MTL in SCD patients than in normal controls using TBSS analysis, which also provided additional evidence for our research results (Wang et al., 2012; Selnes et al., 2013). Our findings demonstrate that distinctive WM integrity alterations can occur early in SCD, although neuropsychological test results of these patients are comparable to those of healthy controls. Thus, DTI indexes can be a sensitive and reliable imaging technique to detect changes in WM microstructure, even in this very early stage of AD. Moreover, the present approach may contribute to the development of strategies to stratify SCD subjects with different risk levels for AD.
The impaired WM pathways (including the ATR, forceps minor, forceps major, CgC, CgH, and IFO) were the tracts connecting earliest affected gray matter structures in AD (i.e., hippocampus, cingulate gyrus, medial prefrontal cortex, and posterior cingulate cortex). These findings are in line with previous studies reporting decreased FDG metabolism, increased Aβ deposition, and pathological tau accumulation in these regions in SCD, which closely coincided with the imaging features found in early AD patients (Snitz et al., 2015; Buckley et al., 2017; Vannini et al., 2017). In that sense, our findings showing altered WM tracts connecting these main cortical hubs of cognitive brain networks may provide novel insights into the pathological mechanisms of AD from the WM neurodegeneration perspective. Furthermore, most of the mentioned tracts link brain areas belonging to the default mode network, which has consistently been found to be affected across the development of AD (Zhang et al., 2010). Regions in the default mode network, such as the medial prefrontal cortex, are related to working memory, whereas MTL regions are involved in sustaining long-term memory. Many studies have demonstrated that individuals with SCD show abnormal activity in the default mode network, as assessed by functional MRI (Rodda et al., 2009, 2011). Thus, the damaged WM tracts linking the default mode network could be partly responsible for these functional changes.
In addition, the present aMCI group showed significantly higher MD values in the left CgH and forceps minor than in those of SCD patients. These results were in line with the findings by Parra et al., whose participants were young presymptomatic presenilin 1 mutation carriers. These young hereditary AD carriers showed a significant increase in MD values in bilateral CgH and forceps minor (Parra et al., 2015). Jung et al. (2015) also found that in the aMCI group, compared with the SCD group, only the MD value in the left CgH was significantly increased. Therefore, we speculate that the destruction of the WM integrity in the left CgH and forceps minor may be crucial for progressive cognitive impairment. A significant of numerical increases was also observed in MD of the left CgH and forceps minor in SCD and aMCI groups compared with the NC.
The multiple general linear regression model analysis showed that after age, gender, and years of education were adjusted, FA and/or MD values in the left CgC, left CgH, and forceps minor were independently correlated with overall cognitive function and memory function. The lower the FA value and the higher the MD value were, the worse the cognitive performance. The cingulum, as the core structure in the Papez circuit of the cholinergic system, is an important pathway to maintain communication among the limbic system, which plays a role in perception, executive control, episodic memory, and understanding (Bubb et al., 2018). The disruption of the cingulum can lead to the disruption of communication from the hippocampus and cingulate gyrus to the cerebral cortex, subsequently impairing memory and other cognitive domains. The forceps minor connects the bilateral prefrontal cortex, where the encoding and extraction of episodic memory occur (Jeong et al., 2015). Grambaite et al. (2010) noticed in SCD and aMCI subjects who had decreased FA values in the forceps minor were related to verbal memory. Parra’s study found that an increase in the MD values in the forceps minor and left CgH could predict poor short-term memory task performance (Parra et al., 2015), which agreed with our results. Hence, we can infer that WM microstructural impairment may be an important predictor of overall cognitive and memory function and an early marker of the development of AD.
There are still some limitations to this study. First, this study is cross-sectional and is insufficient for predicting the clinical outcomes of SCD and aMCI patients. Second, the present sample of SCD and aMCI participants was recruited based on clinical criteria, which may not have displayed the underlying AD pathology. Future studies should add biomarker information to better characterize the findings. Therefore, these data should be interpreted with caution. Third, WM microstructures may be different owing to the diverse stages of SCD, but currently, there are no clear criteria to classify the severity of SCD. In addition, our study focused only on the changes in the WM microstructure of SCD patients. It is unclear whether these changes are related to gray matter atrophy, functional changes, or CSF pathological indicators. Finally, we only focused on the most commonly used metrics (FA and MD), but other metrics in DTI, such as radial and axial diffusivity, will be investigated subsequently. The longitudinal change pattern of WM microstructure from preclinical to clinical AD needs to be confirmed by large sample longitudinal studies.
In summary, SCD individuals had extensive WM microstructural damage in a pattern similar to that seen in aMCI. DTI-based diffusion parameters could be useful imaging biomarkers for the early diagnosis and stage monitoring of AD. Considering that individuals with SCD are at high risk for aMCI and AD, future studies are needed to characterize individuals with SCD longitudinally to identify individuals who may eventually develop AD, enabling more timely interventions.
Data Availability Statement
The datasets generated for this study are available on request to the corresponding author.
Ethics Statement
The studies involving human participants were reviewed and approved by the ethics committees of the Affiliated Drum Tower Hospital of Nanjing University Medical School (clinical trials government identifier: NCT01364246). The patients/participants provided their written informed consent to participate in this study. Written informed consent was obtained from the individual(s) for the publication of any potentially identifiable images or data included in this article.
Author Contributions
HZ and FB designed the study, collected the data, and edited the manuscript. YX designed the study and edited the manuscript. CL collected the data, wrote and edited the manuscript, and performed the statistics. RQ wrote and edited the manuscript. ML, DY, LH, and RL collected the data. HC validated the statistics.
Funding
This research was partly supported by the National Natural Science Foundation of China (Nos. 81630028, 81822013, and 81771157), the Key Research and Development Program of Jiangsu Province of China (BE2016610), Jiangsu Province Key Medical Discipline (ZDXKA2016020), Jiangsu Provincial Key Medical Talents (No. ZDRCA2016085), and Jiangsu Province “333” project, Nanjing Medical Science and Technique Development Foundation (ZKX13020).
Conflict of Interest
The authors declare that the research was conducted in the absence of any commercial or financial relationships that could be construed as a potential conflict of interest.
Footnotes
- ^ http://www.nitrc.org/projects/panda/
- ^ https://fsl.fmrib.ox.ac.uk/fsl/fslwiki
- ^ http://www.trackvis.org/dtk/
- ^ https://www.nitrc.org/projects/mricron
- ^ http://www-01.ibm.com/software/analytics/spss
References
Acosta-Cabronero, J., and Nestor, P. J. (2014). Diffusion tensor imaging in Alzheimer’s disease: insights into the limbic-diencephalic network and methodological considerations. Front. Aging Neurosci. 6:266. doi: 10.3389/fnagi.2014.00266
Bubb, E. J., Metzler-Baddeley, C., and Aggleton, J. P. (2018). The cingulum bundle: anatomy, function, and dysfunction. Neurosci. Biobehav. Rev. 92, 104–127. doi: 10.1016/j.neubiorev.2018.05.008
Buckley, R. F., Hanseeuw, B., Schultz, A. P., Vannini, P., Aghjayan, S. L., Properzi, M. J., et al. (2017). Region-specific association of subjective cognitive decline with tauopathy independent of global β-amyloid burden. JAMA Neurol. 74, 1455–1463. doi: 10.1001/jamaneurol.2017.2216
Buckley, R. F., Maruff, P., Ames, D., Bourgeat, P., Martins, R. N., Masters, C. L., et al. (2016). Subjective memory decline predicts greater rates of clinical progression in preclinical Alzheimer’s disease. Alzheimers Dement. 12, 796–804. doi: 10.1016/j.jalz.2015.12.013
Bürgel, U., Amunts, K., Hoemke, L., Mohlberg, H., Gilsbach, J. M., and Zilles, K. (2006). White matter fiber tracts of the human brain: three-dimensional mapping at microscopic resolution, topography and intersubject variability. Neuroimage 29, 1092–1105. doi: 10.1016/j.neuroimage.2005.08.040
Caso, F., Agosta, F., and Filippi, M. (2016). Insights into white matter damage in Alzheimer’s disease: from postmortem to in vivo diffusion tensor MRI studies. Neurodegener. Dis. 16, 26–33. doi: 10.1159/000441422
Cheng, Y. W., Chen, T. F., and Chiu, M. J. (2017). From mild cognitive impairment to subjective cognitive decline: conceptual and methodological evolution. Neuropsychiatr. Dis. Treat. 13, 491–498. doi: 10.2147/ndt.s123428
Choo, I. H., Lee, D. Y., Oh, J. S., Lee, J. S., Lee, D. S., Song, I. C., et al. (2010). Posterior cingulate cortex atrophy and regional cingulum disruption in mild cognitive impairment and Alzheimer’s disease. Neurobiol. Aging 31, 772–779. doi: 10.1016/j.neurobiolaging.2008.06.015
Copenhaver, B. R., Rabin, L. A., Saykin, A. J., Roth, R. M., Wishart, H. A., Flashman, L. A., et al. (2006). The fornix and mammillary bodies in older adults with Alzheimer’s disease, mild cognitive impairment, and cognitive complaints: a volumetric MRI study. Psychiatry Res. 147, 93–103. doi: 10.1016/j.pscychresns.2006.01.015
Cui, Z., Zhong, S., Xu, P., He, Y., and Gong, G. (2013). PANDA: a pipeline toolbox for analyzing brain diffusion images. Front. Hum. Neurosci. 7:42. doi: 10.3389/fnhum.2013.00042
Grambaite, R., Stenset, V., Reinvang, I., Walhovd, K. B., Fjell, A. M., and Fladby, T. (2010). White matter diffusivity predicts memory in patients with subjective and mild cognitive impairment and normal CSF total tau levels. J. Int. Neuropsychol. Soc. 16, 58–69. doi: 10.1017/s1355617709990932
Hafkemeijer, A., Altmann-Schneider, I., Oleksik, A. M., van de Wiel, L., Middelkoop, H. A., van Buchem, M. A., et al. (2013). Increased functional connectivity and brain atrophy in elderly with subjective memory complaints. Brain Connect. 3, 353–362. doi: 10.1089/brain.2013.0144
Hua, K., Zhang, J., Wakana, S., Jiang, H., Li, X., Reich, D. S., et al. (2008). Tract probability maps in stereotaxic spaces: analyses of white matter anatomy and tract-specific quantification. NeuroImage 39, 336–347. doi: 10.1016/j.neuroimage.2007.07.053
Jeong, W., Chung, C. K., and Kim, J. S. (2015). Episodic memory in aspects of large-scale brain networks. Front. Hum. Neurosci. 9:454. doi: 10.3389/fnhum.2015.00454
Jessen, F., Amariglio, R. E., van Boxtel, M., Breteler, M., Ceccaldi, M., Chetelat, G., et al. (2014). A conceptual framework for research on subjective cognitive decline in preclinical Alzheimer’s disease. Alzheimers Dement. 10, 844–852. doi: 10.1016/j.jalz.2014.01.001
Jung, W. B., Lee, Y. M., Kim, Y. H., and Mun, C. W. (2015). Automated classification to predict the progression of Alzheimer’s disease using whole-brain volumetry and DTI. Psychiatry Investig. 12, 92–102. doi: 10.4306/pi.2015.12.1.92
Kantarci, K., Murray, M. E., Schwarz, C. G., Reid, R. I., Przybelski, S. A., Lesnick, T., et al. (2017). White-matter integrity on DTI and the pathologic staging of Alzheimer’s disease. Neurobiol. Aging 56, 172–179. doi: 10.1016/j.neurobiolaging.2017.04.024
Konukoglu, E., Coutu, J. P., Salat, D. H., and Fischl, B. (2016). Multivariate statistical analysis of diffusion imaging parameters using partial least squares: application to white matter variations in Alzheimer’s disease. NeuroImage 134, 573–586. doi: 10.1016/j.neuroimage.2016.04.038
Lin, Y., Shan, P. Y., Jiang, W. J., Sheng, C., and Ma, L. (2019). Subjective cognitive decline: preclinical manifestation of Alzheimer’s disease. Neurol. Sci. 40, 41–49. doi: 10.1007/s10072-018-3620-y
Lu, J., Li, D., Li, F., Zhou, A., Wang, F., Zuo, X., et al. (2011). Montreal cognitive assessment in detecting cognitive impairment in Chinese elderly individuals: a population-based study. J. Geriatr. Psychiatry Neurol. 24, 184–190. doi: 10.1177/0891988711422528
Mitchell, A. J., Beaumont, H., Ferguson, D., Yadegarfar, M., and Stubbs, B. (2014). Risk of dementia and mild cognitive impairment in older people with subjective memory complaints: meta-analysis. Acta Psychiatr. Scand. 130, 439–451. doi: 10.1111/acps.12336
Nowrangi, M. A., Lyketsos, C. G., Leoutsakos, J. M., Oishi, K., Albert, M., Mori, S., et al. (2013). Longitudinal, region-specific course of diffusion tensor imaging measures in mild cognitive impairment and Alzheimer’s disease. Alzheimers Dement. 9, 519–528. doi: 10.1016/j.jalz.2012.05.2186
Ohlhauser, L., Parker, A. F., Smart, C. M., and Gawryluk, J. R. (2019). White matter and its relationship with cognition in subjective cognitive decline. Alzheimers Dement. 11, 28–35. doi: 10.1016/j.dadm.2018.10.008
Parra, M. A., Saarimaki, H., Bastin, M. E., Londono, A. C., Pettit, L., Lopera, F., et al. (2015). Memory binding and white matter integrity in familial Alzheimer’s disease. Brain 138, 1355–1369. doi: 10.1093/brain/awv048
Petersen, R. C. (2004). Mild cognitive impairment as a diagnostic entity. J. Intern. Med. 256, 183–194. doi: 10.1111/j.1365-2796.2004.01388.x
Reisberg, B., Prichep, L., Mosconi, L., John, E. R., Glodzik-Sobanska, L., Boksay, I., et al. (2008). The pre-mild cognitive impairment, subjective cognitive impairment stage of Alzheimer’s disease. Alzheimers Dement. 4, S98–S108. doi: 10.1016/j.jalz.2007.11.017
Rodda, J. E., Dannhauser, T. M., Cutinha, D. J., Shergill, S. S., and Walker, Z. (2009). Subjective cognitive impairment: increased prefrontal cortex activation compared to controls during an encoding task. Int. J. Geriatr. Psychiatry 24, 865–874. doi: 10.1002/gps.2207
Rodda, J., Dannhauser, T., Cutinha, D. J., Shergill, S. S., and Walker, Z. (2011). Subjective cognitive impairment: functional MRI during a divided attention task. Eur. Psychiatry 26, 457–462. doi: 10.1016/j.eurpsy.2010.07.003
Rönnlund, M., Sundström, A., Adolfsson, R., and Nilsson, L. G. (2015). Self-reported memory failures: associations with future dementia in a population-based study with long-term follow-up. J. Am. Geriatr. Soc. 63, 1766–1773. doi: 10.1111/jgs.13611
Salat, D. H., Tuch, D. S., van der Kouwe, A. J., Greve, D. N., Pappu, V., Lee, S. Y., et al. (2010). White matter pathology isolates the hippocampal formation in Alzheimer’s disease. Neurobiol. Aging 31, 244–256. doi: 10.1016/j.neurobiolaging.2008.03.013
Sánchez-Benavides, G., Grau-Rivera, O., Suárez-Calvet, M., Minguillon, C., Cacciaglia, R., Gramunt, N., et al. (2018). Brain and cognitive correlates of subjective cognitive decline-plus features in a population-based cohort. Alzheimers Res. Ther. 10:123. doi: 10.1186/s13195-018-0449-9
Selnes, P., Aarsland, D., Bjornerud, A., Gjerstad, L., Wallin, A., Hessen, E., et al. (2013). Diffusion tensor imaging surpasses cerebrospinal fluid as predictor of cognitive decline and medial temporal lobe atrophy in subjective cognitive impairment and mild cognitive impairment. J. Alzheimers Dis. 33, 723–736. doi: 10.3233/jad-2012-121603
Sexton, C. E., Kalu, U. G., Filippini, N., Mackay, C. E., and Ebmeier, K. P. (2011). A meta-analysis of diffusion tensor imaging in mild cognitive impairment and Alzheimer’s disease. Neurobiol. Aging 32, 2322.e5–2322.e18. doi: 10.1016/j.neurobiolaging.2010.05.019
Shaikh, S., Kumar, A., and Bansal, A. (2018). Diffusion tensor imaging: An overview. Neurol. India 66, 1603–1611. doi: 10.4103/0028-3886.246233
Slot, R. E. R., Verfaillie, S. C. J., Overbeek, J. M., Timmers, T., Wesselman, L. M. P., Teunissen, C. E., et al. (2018). Subjective cognitive impairment cohort (SCIENCe): study design and first results. Alzheimers Res. Ther. 10:76. doi: 10.1186/s13195-018-0390-y
Snitz, B. E., Lopez, O. L., McDade, E., Becker, J. T., Cohen, A. D., Price, J. C., et al. (2015). Amyloid-β imaging in older adults presenting to a memory clinic with subjective cognitive decline: a pilot study. J. Alzheimers Dis. 48, S151–S159. doi: 10.3233/jad-150113
Soares, J. M., Marques, P., Alves, V., and Sousa, N. (2013). A hitchhiker’s guide to diffusion tensor imaging. Front. Neurosci. 7:31. doi: 10.3389/fnins.2013.00031
Sui, X. and Rajapakse, J. C. (2018). Profiling heterogeneity of Alzheimer’s disease using white-matter impairment factors. Neuroimage Clin. 20, 1222–1232. doi: 10.1016/j.nicl.2018.10.026
Sun, Y., Yang, F. C., Lin, C. P., and Han, Y. (2015). Biochemical and neuroimaging studies in subjective cognitive decline: progress and perspectives. CNS Neurosci. Ther. 21, 768–775. doi: 10.1111/cns.12395
Vannini, P., Hanseeuw, B., Munro, C. E., Amariglio, R. E., Marshall, G. A., Rentz, D. M., et al. (2017). Hippocampal hypometabolism in older adults with memory complaints and increased amyloid burden. Neurology 88, 1759–1767. doi: 10.1212/wnl.0000000000003889
Visser, P. J., Verhey, F., Knol, D. L., Scheltens, P., Wahlund, L. O., Freund-Levi, Y., et al. (2009). Prevalence and prognostic value of CSF markers of Alzheimer’s disease pathology in patients with subjective cognitive impairment or mild cognitive impairment in the DESCRIPA study: a prospective cohort study. Lancet Neurol. 8, 619–627. doi: 10.1016/S1474-4422(09)70139-5
Wahlund, L. O., Barkhof, F., Fazekas, F., Bronge, L., Augustin, M., Sjogren, M., et al. (2001). A new rating scale for age-related white matter changes applicable to MRI and CT. Stroke 32, 1318–1322. doi: 10.1161/01.str.32.6.1318
Wang, Y., West, J. D., Flashman, L. A., Wishart, H. A., Santulli, R. B., Rabin, L. A., et al. (2012). Selective changes in white matter integrity in MCI and older adults with cognitive complaints. Biochim. Biophys. Acta 1822, 423–430. doi: 10.1016/j.bbadis.2011.08.002
Zhang, H. Y., Wang, S. J., Liu, B., Ma, Z. L., Yang, M., Zhang, Z. J., et al. (2010). Resting brain connectivity: changes during the progress of Alzheimer disease. Radiology 256, 598–606. doi: 10.1148/radiol.10091701
Zhao, Q., Lv, Y., Zhou, Y., Hong, Z., and Guo, Q. (2012). Short-term delayed recall of auditory verbal learning test is equivalent to long-term delayed recall for identifying amnestic mild cognitive impairment. PLoS One 7:e51157. doi: 10.1371/journal.pone.0051157
Keywords: Alzheimer’s disease, subjective cognitive decline, amnestic mild cognitive impairment, diffusion tensor imaging, white matter pathway
Citation: Luo C, Li M, Qin R, Chen H, Yang D, Huang L, Liu R, Xu Y, Bai F and Zhao H (2020) White Matter Microstructural Damage as an Early Sign of Subjective Cognitive Decline. Front. Aging Neurosci. 11:378. doi: 10.3389/fnagi.2019.00378
Received: 05 August 2019; Accepted: 23 December 2019;
Published: 28 January 2020.
Edited by:
Ignacio Torres-Aleman, Spanish National Research Council (CSIC), SpainReviewed by:
Diana López-Barroso, University of Málaga, SpainMarina Weiler, National Institutes of Health (NIH), United States
Copyright © 2020 Luo, Li, Qin, Chen, Yang, Huang, Liu, Xu, Bai and Zhao. This is an open-access article distributed under the terms of the Creative Commons Attribution License (CC BY). The use, distribution or reproduction in other forums is permitted, provided the original author(s) and the copyright owner(s) are credited and that the original publication in this journal is cited, in accordance with accepted academic practice. No use, distribution or reproduction is permitted which does not comply with these terms.
*Correspondence: Hui Zhao, emhhb2h1aWd5YzIwMDJAMTYzLmNvbQ==; Feng Bai, YmFpZmVuZzUxNUAxMjYuY29t