- INSERM UMR1093-CAPS, Université Bourgogne Franche-Comté, UFR des Sciences du Sport, Dijon, France
Several sensorimotor modifications are known to occur with aging, possibly leading to adverse outcomes such as falls. Recently, some of those modifications have been proposed to emerge from motor planning deteriorations. Motor planning of vertical movements is thought to engage an internal model of gravity to anticipate its mechanical effects on the body-limbs and thus to genuinely produce movements that minimize muscle effort. This is supported, amongst other results, by direction-dependent kinematics where relative durations to peak accelerations and peak velocity are shorter for upward than for downward movements. The present study compares the motor planning of fast and slow vertical arm reaching movements between 18 young (24 ± 3 years old) and 17 older adults (70 ± 5 years old). We found that older participants still exhibit strong directional asymmetries (i.e., differences between upward and downward movements), indicating that optimization processes during motor planning persist with healthy aging. However, the size of these differences was increased in older participants, indicating that gravity-related motor planning changes with age. We discuss this increase as the possible result of an overestimation of gravity torque or increased weight of the effort cost in the optimization process. Overall, these results support the hypothesis that feedforward processes and, more precisely, optimal motor planning, remain active with healthy aging.
Introduction
Aging is associated with various sensorimotor modifications. Vision (Owsley, 2011), vestibular function (Alberts et al., 2019), and proprioception (Goble et al., 2009) decline progressively. Walking and balance control are impaired (Laughton et al., 2003), and upper-limb movements become slower and more variable (Yan et al., 1998). These sensorimotor alterations lead to adverse effects such as falls, the most frequent cause of injuries in older adults (Grisso et al., 1990; World Health Organization, Ageing and Life Course Unit, 2008; Robinovitch et al., 2013). Because the number of older adults has fiercely increased in the past few decades, understanding the mechanisms responsible for age-related sensorimotor alterations is paramount.
Motor planning is a brain process specifying how a movement will be performed. Given a motor goal—e.g., grasping a cup of coffee—and a set of constraints—e.g., the gravitational pull on the arm and cup—this process selects the movement trajectory to be accomplished amongst a myriad of possible trajectories that would all attain the desired goal (Franklin and Wolpert, 2011; Wong et al., 2015). For example, previous studies have shown that motor planning adapts reaching trajectories to changing inertial and gravitational constraints in young adults (Gaveau and Papaxanthis, 2011; Vu et al., 2016a). There are pieces of evidence suggesting that aging alters motor planning (Kanekar and Aruin, 2014; Kubicki et al., 2016; Casamento-Moran et al., 2017; Stöckel et al., 2017; Wunsch et al., 2017), and that altered motor planning may cause falls (Lord and Fitzpatrick, 2001; Lyon and Day, 2005; Robinovitch et al., 2013; Tisserand et al., 2016). Since fall is inherently linked to gravity, understanding how older adults adapt their motor planning to gravity is crucial.
Using arm reaching tasks, several studies have investigated gravity-related motor planning in young adults (Gentili et al., 2007; Le Seac’h and McIntyre, 2007; Crevecoeur et al., 2009; Gaveau et al., 2011, 2014, 2016; Bringoux et al., 2012; Sciutti et al., 2012; Yamamoto and Kushiro, 2014; Hondzinski et al., 2016; Yamamoto et al., 2016; Olesh et al., 2017; Gaveau et al., 2019; Yamamoto et al., 2019). A consistent outcome is the existence of direction-dependent arm kinematics in normal gravity, which progressively disappears in microgravity (Papaxanthis et al., 2005; Gaveau et al., 2016). Direction-dependent kinematics is demonstrated by shorter time to peak acceleration and time to peak velocity for upward than for downward movements, i.e., more abrupt acceleration and softer deceleration. Model simulations explain these direction-dependent kinematics, and their progressive disappearance under microgravity conditions, as an optimization process that saves muscle effort (Berret et al., 2008; Crevecoeur et al., 2009; Gaveau et al., 2014, 2016). Consequently, direction-dependent kinematics has been proposed to represent the signature of a motor planning strategy that optimally integrates gravity torque to save muscle effort. Recent electromyographic (EMG) analyses further support this concept (Gaveau et al., 2019).
Here, relying on the above-described robust findings, we investigated the motor planning of vertical arm movements in young and healthy older adults. We compared arm trajectories between upward and downward movements and between age-groups to test whether older adults produce trajectories that are similar to young adults. According to results suggesting that motor planning deteriorates during aging (Kanekar and Aruin, 2014; Kubicki et al., 2016; Casamento-Moran et al., 2017; Stöckel et al., 2017; Wunsch et al., 2017), one could predict that older adults present with a decreased capability to plan optimal arm movements that minimize muscle effort. Such impairment should be reflected by a decreased direction-dependence of arm kinematics in older adults compared to young ones. Alternatively, according to results showing that predictive mechanisms remain functional and are increasingly relied upon during aging, one could predict that directional asymmetries are preserved or even increased (Boisgontier and Nougier, 2013; Helsen et al., 2016; Wolpe et al., 2016; Hoellinger et al., 2017; Vandevoorde and Orban de Xivry, 2019).
Materials and Methods
Ethics and Participants
This study was carried out following legal requirements and international norms (Declaration of Helsinki, 1964) and approved by the French National ethics committee (2019-A01558-49). Eighteen young (14 males; mean age = 24 ± 3 years; mean weight = 70.5 ± 10.6 kg; mean initial gravity torque = 13.35 ± 2.7 N.m) and 17 healthy older adults (six males mean age = 70 ± 5 years; mean weight = 69.9 ± 11.8 kg; mean initial gravity torque = 12.7 ± 2.9 N.m) participated in this study after giving their written informed consent. All participants had normal or corrected to normal vision, were right-handed according to Edinburgh handedness inventory (Oldfield, 1971), and did not present any neurological or musculoskeletal deficiency.
Gravity torque is known to affect the direction dependence of arm kinematics. Increasing the amount of gravity torque increases the direction dependence (Gaveau et al., 2014, 2016). Therefore, if causing any effect, the small difference between the two age groups should cause a decrease in the direction dependence in older adults compared to young ones. A bilateral Student T-test did not reveal any group effect on the body weight nor on the initial gravity torque (weight, t = 0.177, p = 0.860, Cohen’s d = 0.059; torque, t = 0.667, p = 0.508, Cohen’s d = 0.225). Movement dynamics was therefore comparable between the two age groups.
Experimental Design
Our experimental devices and protocol were similar to those of previous studies investigating motor planning of arm movements in the gravity field (Gentili et al., 2007; Gaveau et al., 2011, 2014, 2016). We investigated single-degree-of-freedom arm movements to manipulate the effects of gravity (with movement direction) while keeping the rest of the movement dynamics constant (inertial forces). Single degree-of-freedom movements allow to specifically investigate gravity-related motor strategies. Participants were comfortably seated on a chair with their trunk in a vertical position. The target system (a curved steel bar with three targets fixed on it) was vertically aligned and placed in front of the participants’ right arm at a distance equal to the length of their fully extended arm plus two centimeters (Figure 1A). We horizontally aligned the central target (starting target) with the participants’ shoulder, while positioning the other two targets to imply a 30° upwards or a 30° downwards shoulder rotation. Starting from the central target, participants performed single-degree-of-freedom (rotation around the shoulder joint) upward and downward arm movements, as accurately as possible at two speeds. Previous work revealed that movement speed significantly influences the temporal organization of vertical arm movements (Gaveau and Papaxanthis, 2011). Therefore, to obtain movements of comparable durations between conditions and groups, automatic online analyses of movement duration were performed, and the results were used to provide verbal feedback to the participants. This feedback aimed at driving participants to perform reaching movements lasting about 350 ms (called fast speed below) and 550 ms (called slow speed below). Fast and slow trials were performed in a random block design, and upward and downward movements were randomly ordered within each block (12 trials × 4 conditions; 48 trials overall for each participant). Participants performed a few practice trials before the beginning of the experiment (~10 practice trials per experimental condition). At the beginning of each trial, they were invited to point at the central target (starting position, see Figure 1A). After a short period (~2 s), the experimenter verbally indicated the target (upward or downward) to reach. Participants were then allowed to carry out the movement without any constraint on their reaction time and to maintain their final finger position for about 2 s until a verbal signal informed them to relax their arm. In order to avoid muscle fatigue, we separated each trial by a short rest period (~15 s) and both blocks by a 5 min rest period.
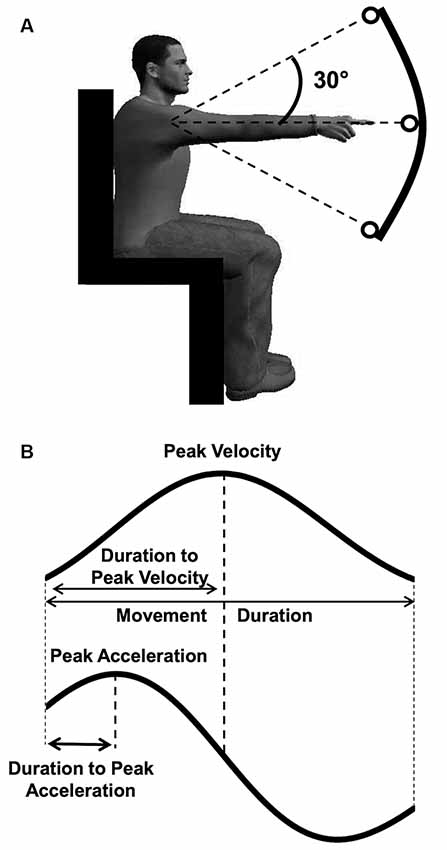
Figure 1. (A) Experimental setup and participant’s starting position. From this starting position, participants pointed to the upward or the downward target on separated trials. (B) Illustration of the parameters computed on the velocity and acceleration profiles.
We placed five reflective markers on the participants’ right shoulder (acromion), arm (middle of the humeral bone), elbow (lateral epicondyle), wrist (cubitus styloid process), and finger (nail of the index). We recorded markers’ position with an optoelectronic motion capture system (VICON system) that consisted of six cameras (sampling frequency: 100 Hz; precision <0.5 mm).
Data Analysis
Data were processed using custom programs written in Matlab (Mathworks, Natick, MA, USA). The tridimensional position (X, Y, Z) of markers was low-pass filtered with a digital third-order Butterworth filter (zero-phase distortion) at a cut-off frequency of 10 Hz. We derived these signals in order to compute three-dimensional velocity and acceleration profiles for each movement. Movement start and end was defined as the moment when finger velocity respectively went above or fell below 10% of the peak velocity value (Gaveau and Papaxanthis, 2011; Gaveau et al., 2014, 2016). Movement duration and amplitude were computed based on movement start and end. Peak velocity and peak acceleration were defined as maximal values of velocity and acceleration signals, respectively.
Here we focused on a single degree of freedom arm movement task to specifically investigate gravity-related motor planning. During such movements, no spatial features could differ between directions, speeds or ages. However, the temporal organization of movements can differ between conditions; i.e., participants can spend more or less time accelerating and decelerating their arm. Previous modeling studies have proposed that the temporal organization of vertical arm movements reveal an effort minimization strategy (Berret et al., 2008; Crevecoeur et al., 2009; Gaveau et al., 2014, 2016). Following results from previous studies, we quantified the temporal organization of each movement with two temporal parameters that are theoretically independent of the movement duration (see Figure 1B and Gaveau et al., 2011, 2014); (i) the relative duration to peak acceleration (rD-PA), defined as the duration to peak divided by movement duration; and (ii) the relative duration to peak velocity (rD-PV), defined as the duration to peak velocity divided by movement duration.
Statistical Analysis
We used STATISTICA 10 (StatSoft Inc., Tulsa, OK, USA) to perform all statistical analyses. All variables showed a normal distribution (Kolgomorov–Smirnov test). We carried out repeated measures variance analysis (three-way ANOVA) with three factors: age (Young vs. Older), speed (Fast and Slow), and direction (Upward vs. Downward). Scheffé tests were used for post hoc comparisons. In order to specifically compare directional asymmetries between groups in the same condition, we used pre-planned bilateral Student T-tests. The level of significance was set at p < 0.05 for all analyses.
Results
Participants accurately reached targets (average movement amplitude: 28.8 ± 1.1° for young and 28.7 ± 2.1° for older participants, see Table 1 for all values) with smoothed movement exhibiting single-peaked and bell-shaped velocity profiles (Kelso et al., 1979). Figure 2 qualitatively illustrates the mean position, velocity, and acceleration profiles recorded for each group and direction at the fast speed.
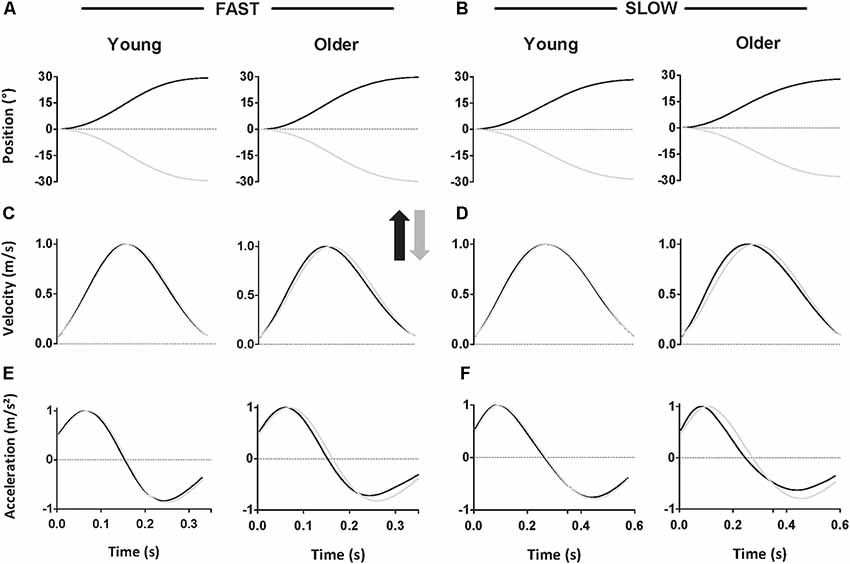
Figure 2. Mean position (A–B), velocity (C–D), and acceleration (E–F) profiles for each group and direction at fast (A,C,E) and slow (B,D,F) speed. Black traces represent upward movements, and grey traces represent downward movements. In each panel, left plots display young participants’ data, while right plots display older participants’ data.
Movement Duration
Because movement duration was previously shown to influence the temporal organization of arm movements (Gaveau and Papaxanthis, 2011), to ensure that it was similar between conditions, we performed the same statistical analysis on movement duration as on the other parameters. As required by task constraints, movement duration significantly varied with speed instructions (speed effect: F = 105.18; p < 0.001; = 0.76). Average movement durations were respectively 340 ± 40 ms and 575 ± 120 ms for fast and slow movements (Table 1). However, neither age (F = 0.27; p = 0.60; = 0.008) nor direction (F = 0.001; p = 0.97; < 0.001) effects were observed. Similarly, all interaction effects (age × direction, F = 0.39; p = 0.53; = 0.01; speed × age, F = 0.06; p = 0.81; = 0.001; speed × direction, F = 0.016; < 0.001; p = 0.90; age × speed × direction, F = 0.10; p = 0.92; < 0.001) were non-significant. These results show that movement duration was similar between groups and directions within each speed instruction.
Relative Duration to Peak Acceleration (rD-PA)
Figure 3A displays rD-PA for each group, speed, and direction (see also Table 1). rD-PA was smaller for upward than for downward movements (direction effect, F = 67.95; p < 0.001; = 0.67). Figure 3A also reveals that rD-PA was smaller for slow than for fast movements (speed effect, F = 40.64; p < 0.001; = 0.55). Of particular interest for the present study is the fact that the difference between upward and downward movements was larger in the older than in the young adults at both speeds. The ANOVA yielded a significant age × direction interaction effect (F = 4.41; p = 0.04; = 0.12) but no age × speed × direction interaction (F = 0.003; p = 0.95; < 0.001). Post hoc analyses on the group effect did not reach significance for the upward (p = 0.19; Cohen’s d = 0.49) nor for the downward direction (p = 0.99; Cohen’s d = 0.08). To further the analysis on the interaction effect, box-plot in Figure 3C presents the ratio of directional difference [(Down-Up)/Down], thereby illustrating the effect of age on direction-dependent kinematics. The directional ratio was significantly higher for older adults (t = 2.18; p = 0.04; Cohen’s d = 0.73).
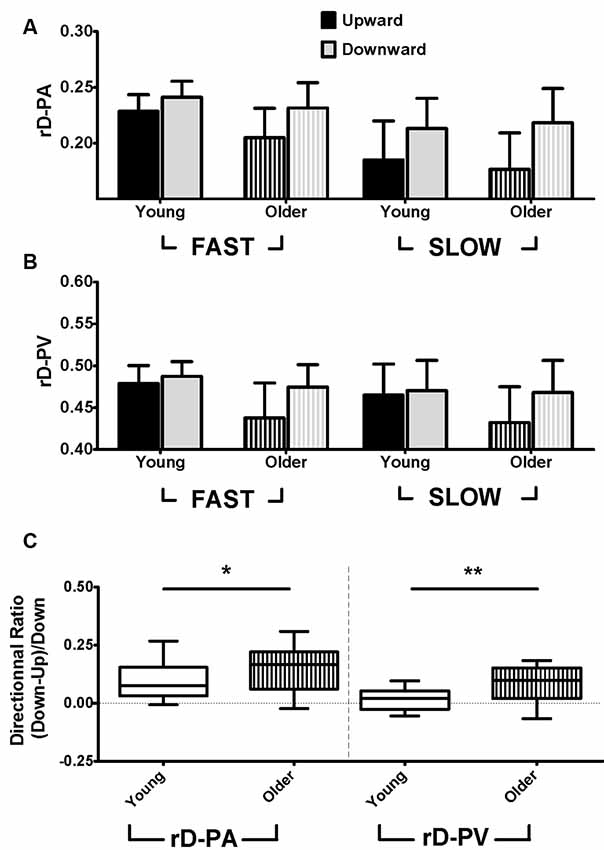
Figure 3. (A) Mean (± SD) relative duration to peak acceleration (rD-PA) for upward (black) and downward (gray) fast and slow movements. Solid bars represent young, and striped bars represent older participants. (B) Mean (± SD) relative duration to peak velocity (rD-PV) for upward (black) and downward (gray) fast and slow movements. Solid bars represent young, and striped bars represent older participants. (C) Box plots show directional ratio (computed for each participant as: (rD-PADown − rD-PAUp)/rD-PADown and (rD-PVDown − rD-PVUp)/rD-PVDown for young (empty boxes) and older (striped boxes) participants. Whiskers represent a 95% confidence interval. *p < 0.05, **p < 0.01.
Relative Duration to Peak Velocity (rD-PV)
Figure 3B displays rD-PV for each group, speed, and direction (see also Table 1). As for rD-PA, the relative duration to peak velocity was shorter for upward compared to downward movements (F = 20.21; p < 0.001; = 0.38) and for slow compared to fast movements (F = 4.98; p = 0.03; = 0.13; see Table 1). Also, the age × direction interaction yielded a significant effect (F = 9.21; p = 0.005; = 0.22), further supporting the increased directional asymmetry in older compared to young adults. Post hoc analyses on the group effect reached significance for the upward (p = 0.003; Cohen’s d = 1.02) but not for the downward direction (p = 0.89; Cohen’s d = 0.24). As for the rD-PA, the age × speed × direction interaction did not reach significance (F = 0.02; p = 0.90; < 0.001). Box-plot in Figure 3C presents the ratio of directional difference [(Down-Up)/Down] to illustrate the effect of age on direction-dependent kinematics. Directional ratio was significantly higher for older adults compared to young ones (t = 3.04; p = 0.004; Cohen’s d = 1.02).
Discussion
We investigated the motor planning of single-degree-of-freedom vertical arm movements in young and older adults. Precisely, we compared directional asymmetries (the difference between upwards and downwards) on relative durations to peak acceleration and peak velocity. Our results show increased directional asymmetries on both parameters in older participants compared to young ones.
In young adults, several studies have shown that single-degree-of-freedom arm movements exhibit directional asymmetries in the vertical plane. These asymmetries: (i) were consistently reported as a more abrupt acceleration phase for upward than for downward movements; (ii) do not exist in the horizontal plane (Gentili et al., 2007; Le Seac’h and McIntyre, 2007); (iii) progressively disappear in the vertical plane when participants are exposed to microgravity (Papaxanthis et al., 2005; Gaveau et al., 2016); (iv) were observed very early in the movements [before 70 ms after movement start (Gaveau and Papaxanthis, 2011; Gaveau et al., 2014)]; and (v) were explained by optimal control model simulations and EMG analyses as a motor strategy that minimizes muscle effort (Berret et al., 2008; Crevecoeur et al., 2009; Gaveau et al., 2014, 2016, 2019). Altogether, in young adults, previous results strongly suggest that direction-dependent kinematics represent the signature of a motor planning strategy that optimally integrates gravity torque to save muscle effort. The present results reveal that older adults also produce directional asymmetries in the vertical plane. Most importantly, these asymmetries have the same sign as those of young adults—a more abrupt acceleration for upward than for downward movements—and they emerge early in the movement—rD-PA was already different, which is about 70 ms after movement onset at the fastest speed. First, this reveals that older adults, similar to young adults, plan arm movements that are direction-dependent in the gravity field. Second, the present results suggest that optimal motor planning, and more specifically, the minimization of muscle effort, continue with healthy aging. Indeed, although the size of the directional asymmetry was shown larger in older compared to young adults, previous studies have demonstrated that the simple existence of the directional asymmetry—independently of its size—is sufficient to reveal an effort-related optimization process (Berret et al., 2008; Gaveau et al., 2016). Hereafter, building on recent experimental and modeling results, we discuss the increased direction-dependence of arm kinematics in older adults.
Studying motor planning within the optimal control framework, previous works explained human movement as the minimization of composite cost functions (for a recent review, see Berret et al., 2019). For example, minimizing a trade-off between effort and smoothness allowed to explain how to reach endpoint is selected during multi-articular arm movement tasks (Berret et al., 2011; Vu et al., 2016b). Using an effort and smoothness composite cost function, the model simulations from Gaveau et al. (2016) also demonstrated that increasing the weight of the effort cost produces an increase in directional asymmetries. Thus, the increased importance of effort during motor planning could explain the increased directional asymmetry observed in older adults. Indeed, aging is associated with a loss of muscle mass and strength (for a review, see Mitchell et al., 2012) that likely results in an increased perception of effort for daily-life tasks (McCloskey et al., 1974; Hess et al., 2016; Pageaux and Lepers, 2016).
Experimental and modeling results have also demonstrated that the size of the directional asymmetry is proportional to the gravity torque (Gaveau et al., 2014, 2016). Increasing the gravity torque either by moving body limbs of increasing mass (i.e., upper arm, forearm, and wrist) or by adding an external load on the limb both led to increased directional asymmetries in the vertical plane (Gaveau et al., 2014). Progressively varying movement direction relative to gravity caused a progressive variation of the temporal organization of arm movement such that arm kinematics and gravity torque linearly correlated with each other (Gaveau et al., 2016). Last, during exposure to microgravity, model simulations explained the progressive disappearance of directional asymmetries as the progressive decrease of the gravity value, i.e., a recalibration of the internal gravity model (Gaveau et al., 2016). Thus, the anticipation of an increased gravity torque could explain the increased directional asymmetry observed in older adults. Experiments on object weight perception in older adults have provided support for such an overestimation of gravity torque (Holmin and Norman, 2012; Parikh and Cole, 2012). Assuming that motor control is the result of two parallel processes, a forward model that produces accurate motor output and an optimal controller selecting the trajectories that minimize some hidden motor costs (Izawa et al., 2008), over-estimating gravity torque would lead to new optimal trajectories without impeding movement accuracy (Gaveau et al., 2011).
Sensory information about gravity is known to influence motor planning (Bringoux et al., 2012; Rousseau et al., 2016). With aging, several studies have provided evidence for sensory deterioration in visual (for review, see Saftari and Kwon, 2018), proprioceptive (Goble et al., 2009), and vestibular systems (Alberts et al., 2019), as well as their multisensory weighting (de Dieuleveult et al., 2017). The perception of gravity vertical also deteriorates with aging (Kobayashi et al., 2002; Baccini et al., 2014). One may, therefore, wonder whether an alternative hypothesis for increased directional asymmetry in older adults could be the elderly’s’ failure to measure gravity torque. In other words, one may expect older adults to apply the same motor plan for vertical and horizontal movements. This premise is implausible as it would produce either no directional asymmetry (Gentili et al., 2007; Le Seac’h and McIntyre, 2007)—should the forward model be accurate—or extensive end-point errors and directional asymmetries with an opposite sign—should the forward model be inaccurate. We observed none of those effects in the present study. Conversely, it is crucial to underline that we observed similar results for rD-PA and rD-PV. Also, we observed no interaction and small effect sizes of age × speed × direction, indicating that speed did not influence the age effect on directional asymmetries. Since rD-PA happens very early in the movement (about 70 ms after onset at fast speed) and rD-PV happens late in the movement (about 250 ms after onset at slow speed), our results suggest that no online feedback-driven correction was implemented to correct hypothetical motor planning errors.
The present results add to the existing literature suggesting that motor planning is modified with aging (Kanekar and Aruin, 2014; Kubicki et al., 2016; Casamento-Moran et al., 2017; Stöckel et al., 2017; Wunsch et al., 2017). Neuroscientists have first interpreted motor planning modifications as a deterioration of feedforward processes (proactive strategies) that urges older adults to favor feedback processes (reactive strategies). The most potent experimental support for this hypothesis is the general observation that movements become slower with aging (Buckles, 1993; Yan et al., 1998). However, older adults are not always slower than younger adults. Asking participants to reach, grasp and lift an object at their own pace, Hoellinger et al. (2017) found that older adults moved faster than young adults. Comparing their empirical results to model simulations, the authors suggested that older adults planned stronger feedforward forces in order to favor feedforward mechanisms over feedback ones. This hypothesis is rooted in results showing that the sensory system becomes noisier with aging (Desmedt and Cheron, 1980; Doherty et al., 1994; Stevens and Choo, 1996). Thus, to compensate for their noisy unreliable sensory system, older adults may favor feedforward over feedback mechanisms. This hypothesis is well-supported by recent results showing that sensory attenuation, a well-studied feedforward mechanism (Blakemore et al., 1998; Shergill et al., 2005; Pareés et al., 2014), increases with aging (Wolpe et al., 2016). Results of neurobiological studies suggesting that increased brain activations compensate for neuro-behavioral deficits to preserve motor performance in older adults could also support the hypothesis of increased reliance on feedforward mechanisms (Cabeza, 2002; Berchicci et al., 2012).
There are pieces of evidence suggesting that feedforward mechanisms remain functional with healthy aging and may even compensate for unreliable feedback mechanisms (Boisgontier and Nougier, 2013; Helsen et al., 2016; Wolpe et al., 2016; Hoellinger et al., 2017; Vandevoorde and Orban de Xivry, 2019). The result that older adults still plan direction-dependent arm movements in the gravity field further supports and extends this hypothesis. The present study also reveals that aging exacerbates the direction-dependence of arm kinematics. This result constitutes the first insight into the effect of aging on gravity-related motor planning, whose better understanding may benefit the prevention and rehabilitation of falls and fallers in older adults. Indeed, although we investigated single degree of freedom arm movements to isolate gravity effects here, it is essential to mention that directional asymmetries are also documented for multi-articular arm reaching, reaching to grasp, grasping, hand drawing, and whole-body sit-to-stand/stand-to-sit movements (Papaxanthis et al., 1998, 2003, 2005; Yamamoto and Kushiro, 2014). Future studies should attempt to extend the present findings to whole-body movement tasks and to disentangle sensory from motor planning modifications in older adults.
Data Availability Statement
The datasets generated for this study are available on request to the corresponding author.
Ethics Statement
The studies involving human participants were reviewed and approved by French National ethics committee (2019-A01558-49). Participants provided their written informed consent for taking part in this research.
Author Contributions
CP, FM, and JG conceived the experiment. GP and JG developed, performed the analysis and wrote the first draft. All authors edited the final manuscript.
Funding
This work was supported by the Institut National de la Santé et de la Recherche Médicale (INSERM), the Agence National de la Recherche (ANR, project MOTION ANR-14-CE30-0007-01) and the French “Investissements d’Avenir” program, project ISITE-BFC (contract ANR-15-IDEX-0003).
Conflict of Interest
The authors declare that the research was conducted in the absence of any commercial or financial relationships that could be construed as a potential conflict of interest.
Acknowledgments
We thank Cyril Sirandré and Yves Ballay for their technical support as well as Benjamin Pageaux and Bastien Berret for their helpful comments on this manuscript. Data, analytic methods, and study materials will be made available to other researchers upon request. This manuscript has been released as a Pre-Print at: Poirier et al. (2019) https://www.biorxiv.org/content/10.1101/821314v1.
References
Alberts, B. B. G. T., Selen, L. P. J., and Medendorp, W. P. (2019). Age-related reweighting of visual and vestibular cues for vertical perception. J. Neurophysiol. 121, 1279–1288. doi: 10.1152/jn.00481.2018
Baccini, M., Paci, M., Del Colletto, M., Ravenni, M., and Baldassi, S. (2014). The assessment of subjective visual vertical: comparison of two psychophysical paradigms and age-related performance. Atten. Percept. Psychophys 76, 112–122. doi: 10.3758/s13414-013-0551-9
Berchicci, M., Lucci, G., Pesce, C., Spinelli, D., and Di Russo, F. (2012). Prefrontal hyperactivity in older people during motor planning. NeuroImage 62, 1750–1760. doi: 10.1016/j.neuroimage.2012.06.031
Berret, B., Chiovetto, E., Nori, F., and Pozzo, T. (2011). Evidence for composite cost functions in arm movement planning: an inverse optimal control approach. PLoS Comput. Biol. 7:e1002183. doi: 10.1371/journal.pcbi.1002183
Berret, B., Darlot, C., Jean, F., Pozzo, T., Papaxanthis, C., and Gauthier, J. P. (2008). The inactivation principle: mathematical solutions minimizing the absolute work and biological implications for the planning of arm movements. PLoS Comput. Biol. 4:e1000194. doi: 10.1371/journal.pcbi.1000194
Berret, B., Delis, I., Gaveau, J., and Jean, F. (2019). “Optimality and modularity in human movement: from optimal control to muscle synergies,” in Biomechanics of Anthropomorphic Systems. Springer Tracts in Advanced Robotics, vol 124, eds G. Venture, J. P. Laumond and B. Watier (Cham: Springer), 105–133.
Blakemore, S.-J., Wolpert, D. M., and Frith, C. D. (1998). Central cancellation of self-produced tickle sensation. Nat. Neurosci. 1, 635–640. doi: 10.1038/2870
Boisgontier, M. P., and Nougier, V. (2013). Ageing of internal models: from a continuous to an intermittent proprioceptive control of movement. Age 35, 1339–1355. doi: 10.1007/s11357-012-9436-4
Bringoux, L., Blouin, J., Coyle, T., Ruget, H., and Mouchnino, L. (2012). Effect of gravity-like torque on goal-directed arm movements in microgravity. J. Neurophysiol. 107, 2541–2548. doi: 10.1152/jn.00364.2011
Buckles, V. D. (1993). “Age-related slowing,” in Sensorimotor Impairment in the Elderly, eds G. E. Stelmach and V. Hömberg (Dordrecht: Springer Netherlands), 73–87.
Cabeza, R. (2002). Hemispheric asymmetry reduction in older adults: the HAROLD model. Psychol. Aging 17, 85–100. doi: 10.1037/0882-7974.17.1.85
Casamento-Moran, A., Chen, Y. T., Lodha, N., Yacoubi, B., and Christou, E. A. (2017). Motor plan differs for young and older adults during similar movements. J. Neurophysiol. 117, 1483–1488. doi: 10.1152/jn.00640.2016
Crevecoeur, F., Thonnard, J.-L., and Lefèvre, P. (2009). Optimal integration of gravity in trajectory planning of vertical pointing movements. J. Neurophysiol. 102, 786–796. doi: 10.1152/jn.00113.2009
de Dieuleveult, A. L., Siemonsma, P. C., van Erp, J. B. F., and Brouwer, A.-M. (2017). Effects of aging in multisensory integration: a systematic review. Front. Aging Neurosci. 9:80. doi: 10.3389/fnagi.2017.00080
Desmedt, J. E., and Cheron, G. (1980). Somatosensory evoked potentials to finger stimulation in healthy octogenarians and in young adults: wave forms, scalp topography and transit times of pariental and frontal components. Electroencephalogr. Clin. Neurophysiol. 50, 404–425. doi: 10.1016/0013-4694(80)90007-3
Doherty, T. J., Komori, T., Stashuk, D. W., Kassam, A., and Brown, W. F. (1994). Physiological properties of single thenar motor units in the F-response of younger and older adults. Muscle Nerve 17, 860–872. doi: 10.1002/mus.880170805
Franklin, D. W., and Wolpert, D. M. (2011). Computational mechanisms of sensorimotor control. Neuron 72, 425–442. doi: 10.1016/j.neuron.2011.10.006
Gaveau, J., Berret, B., Angelaki, D. E., and Papaxanthis, C. (2016). Direction-dependent arm kinematics reveal optimal integration of gravity cues. Elife 5:e16394. doi: 10.7554/eLife.16394
Gaveau, J., Berret, B., Demougeot, L., Fadiga, L., Pozzo, T., and Papaxanthis, C. (2014). Energy-related optimal control accounts for gravitational load: comparing shoulder, elbow, and wrist rotations. J. Neurophysiol. 111, 4–16. doi: 10.1152/jn.01029.2012
Gaveau, J., Grospretre, S., Angelaki, D., and Papaxanthis, C. (2019). A cross-species neural integration of gravity for motor optimisation. bioRxiv 728857 [Preprint]. doi: 10.1101/728857
Gaveau, J., Paizis, C., Berret, B., Pozzo, T., and Papaxanthis, C. (2011). Sensorimotor adaptation of point-to-point arm movements after spaceflight: the role of internal representation of gravity force in trajectory planning. J. Neurophysiol. 106, 620–629. doi: 10.1152/jn.00081.2011
Gaveau, J., and Papaxanthis, C. (2011). The temporal structure of vertical arm movements. PLoS One 6:e22045. doi: 10.1371/journal.pone.0022045
Gentili, R., Cahouet, V., and Papaxanthis, C. (2007). Motor planning of arm movements is direction-dependent in the gravity field. Neuroscience 145, 20–32. doi: 10.1016/j.neuroscience.2006.11.035
Goble, D. J., Coxon, J. P., Wenderoth, N., Van Impe, A., and Swinnen, S. P. (2009). Proprioceptive sensibility in the elderly: degeneration, functional consequences and plastic-adaptive processes. Neurosci. Biobehav. Rev. 33, 271–278. doi: 10.1016/j.neubiorev.2008.08.012
Grisso, J. A., Schwarz, D. F., Wishner, A. R., Weene, B., Holmes, J. H., and Sutton, R. L. (1990). Injuries in an elderly inner-city population. J. Am. Geriatr. Soc. 38, 1326–1331. doi: 10.1111/j.1532-5415.1990.tb03456.x
Helsen, W. F., Van Halewyck, F., Levin, O., Boisgontier, M. P., Lavrysen, A., and Elliott, D. (2016). Manual aiming in healthy aging: does proprioceptive acuity make the difference? Age 38:45. doi: 10.1007/s11357-016-9908-z
Hess, T. M., Smith, B. T., and Sharifian, N. (2016). Aging and effort expenditure: the impact of subjective perceptions of task demands. Psychol. Aging 31, 653–660. doi: 10.1037/pag0000127
Hoellinger, T., McIntyre, J., Jami, L., Hanneton, S., Cheron, G., and Roby-Brami, A. (2017). A strategy of faster movements used by elderly humans to lift objects of increasing weight in ecological context. Neuroscience 357, 384–399. doi: 10.1016/j.neuroscience.2017.04.010
Holmin, J. S., and Norman, J. F. (2012). Aging and weight-ratio perception. PLoS One 7:e47701. doi: 10.1371/journal.pone.0047701
Hondzinski, J. M., Soebbing, C. M., French, A. E., and Winges, S. A. (2016). Different damping responses explain vertical endpoint error differences between visual conditions. Exp. Brain Res. 234, 1575–1587. doi: 10.1007/s00221-015-4546-8
Izawa, J., Rane, T., Donchin, O., and Shadmehr, R. (2008). Motor adaptation as a process of reoptimization. J. Neurosci. 28, 2883–2891. doi: 10.1523/JNEUROSCI.5359-07.2008
Kanekar, N., and Aruin, A. S. (2014). The effect of aging on anticipatory postural control. Exp. Brain Res. 232, 1127–1136. doi: 10.1007/s00221-014-3822-3
Kelso, J., Southard, D., and Goodman, D. (1979). On the nature of human interlimb coordination. Science 203, 1029–1031. doi: 10.1126/science.424729
Kobayashi, H., Hayashi, Y., Higashino, K., Saito, A., Kunihiro, T., Kanzaki, J., et al. (2002). Dynamic and static subjective visual vertical with aging. Auris Nasus Larynx 29, 325–328. doi: 10.1016/s0385-8146(02)00058-5
Kubicki, A., Fautrelle, L., Bourrelier, J., Rouaud, O., and Mourey, F. (2016). The early indicators of functional decrease in mild cognitive impairment. Front. Aging Neurosci. 8:193. doi: 10.3389/fnagi.2016.00193
Laughton, C. A., Slavin, M., Katdare, K., Nolan, L., Bean, J. F., Kerrigan, D. C., et al. (2003). Aging, muscle activity, and balance control: physiologic changes associated with balance impairment. Gait Posture 18, 101–108. doi: 10.1016/s0966-6362(02)00200-x
Le Seac’h, A. B., and McIntyre, J. (2007). Multimodal reference frame for the planning of vertical arms movements. Neurosci. Lett. 423, 211–215. doi: 10.1016/j.neulet.2007.07.034
Lord, S. R., and Fitzpatrick, R. C. (2001). Choice stepping reaction time: a composite measure of falls risk in older people. J. Gerontol. A Biol. Sci. Med. Sci. 56, M627–M632. doi: 10.1093/gerona/56.10.m627
Lyon, I. N., and Day, B. L. (2005). Predictive control of body mass trajectory in a two-step sequence. Exp. Brain Res. 161, 193–200. doi: 10.1007/s00221-004-2058-z
McCloskey, D. I., Ebeling, P., and Goodwin, G. M. (1974). Estimation of weights and tensions and apparent involvement of a “sense of effort”. Exp. Neurol. 42, 220–232. doi: 10.1016/0014-4886(74)90019-3
Mitchell, W. K., Williams, J., Atherton, P., Larvin, M., Lund, J., and Narici, M. (2012). Sarcopenia, dynapenia, and the impact of advancing age on human skeletal muscle size and strength; a quantitative review. Front. Physiol. 3:260. doi: 10.3389/fphys.2012.00260
Oldfield, R. C. (1971). The assessment and analysis of handedness: the Edinburgh inventory. Neuropsychologia 9, 97–113. doi: 10.1016/0028-3932(71)90067-4
Olesh, E. V., Pollard, B. S., and Gritsenko, V. (2017). Gravitational and dynamic components of muscle torque underlie tonic and phasic muscle activity during goal-directed reaching. Front. Hum. Neurosci. 11:474. doi: 10.3389/fnhum.2017.00474
Pageaux, B., and Lepers, R. (2016). Fatigue induced by physical and mental exertion increases perception of effort and impairs subsequent endurance performance. Front. Physiol. 7:587. doi: 10.3389/fphys.2016.00587
Papaxanthis, C., Dubost, V., and Pozzo, T. (2003). Similar planning strategies for whole-body and arm movements performed in the sagittal plane. Neuroscience 117, 779–783. doi: 10.1016/s0306-4522(02)00964-8
Papaxanthis, C., Pozzo, T., and McIntyre, J. (2005). Kinematic and dynamic processes for the control of pointing movements in humans revealed by short-term exposure to microgravity. Neuroscience 135, 371–383. doi: 10.1016/j.neuroscience.2005.06.063
Papaxanthis, C., Pozzo, T., Vinter, A., and Grishin, A. (1998). The representation of gravitational force during drawing movements of the arm. Exp. Brain Res. 120, 233–242. doi: 10.1007/s002210050397
Pareés, I., Brown, H., Nuruki, A., Adams, R. A., Davare, M., Bhatia, K. P., et al. (2014). Loss of sensory attenuation in patients with functional (psychogenic) movement disorders. Brain 137, 2916–2921. doi: 10.1093/brain/awu237
Parikh, P. J., and Cole, K. J. (2012). Handling objects in old age: forces and moments acting on the object. J. Appl. Physiol. 112, 1095–1104. doi: 10.1152/japplphysiol.01385.2011
Poirier, G., Papaxanthis, C., Mourey, F., and Gaveau, J. (2019). Motor planning of vertical arm movements in healthy older adults: does effort optimization persist with aging? bioRxiv 821314 [Preprint]. doi: 10.1101/821314
Robinovitch, S. N., Feldman, F., Yang, Y., Schonnop, R., Leung, P. M., Sarraf, T., et al. (2013). Video capture of the circumstances of falls in elderly people residing in long-term care: an observational study. Lancet 381, 47–54. doi: 10.1016/S0140-6736(12)61263-X
Rousseau, C., Papaxanthis, C., Gaveau, J., Pozzo, T., and White, O. (2016). Initial information prior to movement onset influences kinematics of upward arm pointing movements. J. Neurophysiol. 116, 1673–1683. doi: 10.1152/jn.00616.2015
Saftari, L. N., and Kwon, O.-S. (2018). Ageing vision and falls: a review. J. Physiol. Anthropol. 37:11. doi: 10.1186/s40101-018-0170-1
Sciutti, A., Demougeot, L., Berret, B., Toma, S., Sandini, G., Papaxanthis, C., et al. (2012). Visual gravity influences arm movement planning. J. Neurophysiol. 107, 3433–3445. doi: 10.1152/jn.00420.2011
Shergill, S. S., Samson, G., Bays, P. M., Frith, C. D., and Wolpert, D. M. (2005). Evidence for sensory prediction deficits in schizophrenia. Am. J. Psychiatry 162, 2384–2386. doi: 10.1176/appi.ajp.162.12.2384
Stevens, J. C., and Choo, K. K. (1996). Spatial acuity of the body surface over the life span. Somatosens. Mot. Res. 13, 153–166. doi: 10.3109/08990229609051403
Stöckel, T., Wunsch, K., and Hughes, C. M. L. (2017). Age-related decline in anticipatory motor planning and its relation to cognitive and motor skill proficiency. Front. Aging Neurosci. 9:283. doi: 10.3389/fnagi.2017.00283
Tisserand, R., Robert, T., Chabaud, P., Bonnefoy, M., and Chèze, L. (2016). Elderly fallers enhance dynamic stability through anticipatory postural adjustments during a choice stepping reaction time. Front. Hum. Neurosci. 10:613. doi: 10.3389/fnhum.2016.00613
Vandevoorde, K., and Orban de Xivry, J. J. (2019). Internal model recalibration does not deteriorate with age while motor adaptation does. Neurobiol. Aging 80, 138–153. doi: 10.1016/j.neurobiolaging.2019.03.020
Vu, V. H., Isableu, B., and Berret, B. (2016a). Adaptive use of interaction torque during arm reaching movement from the optimal control viewpoint. Sci. Rep. 6:38845. doi: 10.1038/srep38845
Vu, V. H., Isableu, B., and Berret, B. (2016b). On the nature of motor planning variables during arm pointing movement: compositeness and speed dependence. Neuroscience 328, 127–146. doi: 10.1016/j.neuroscience.2016.04.027
Wolpe, N., Ingram, J. N., Tsvetanov, K. A., Geerligs, L., Kievit, R. A., Henson, R. N., et al. (2016). Ageing increases reliance on sensorimotor prediction through structural and functional differences in frontostriatal circuits. Nat. Commun. 7:13034. doi: 10.1038/ncomms13034
Wong, A. L., Haith, A. M., and Krakauer, J. W. (2015). Motor planning. Neuroscientist 21, 385–398. doi: 10.1177/1073858414541484
World Health Organization, Ageing and Life Course Unit. (2008). WHO Global Report on Falls Prevention in Older Age. Geneva: World Health Organization.
Wunsch, K., Weigelt, M., and Stöckel, T. (2017). Anticipatory motor planning in older adults. J. Gerontol. B Psychol. Sci. Soc. Sci. 72, 373–382. doi: 10.1093/geronb/gbv078
Yamamoto, S., Fujii, K., Zippo, K., Kushiro, K., and Araki, M. (2019). The kinetic mechanisms of vertical pointing movements. Heliyon 5:e02012. doi: 10.1016/j.heliyon.2019.e02012
Yamamoto, S., and Kushiro, K. (2014). Direction-dependent differences in temporal kinematics for vertical prehension movements. Exp. Brain Res. 232, 703–711. doi: 10.1007/s00221-013-3783-y
Yamamoto, S., Shiraki, Y., Uehara, S., and Kushiro, K. (2016). Motor control of downward object-transport movements with precision grip by object weight. Somatosens. Mot. Res. 33, 130–136. doi: 10.1080/08990220.2016.1203304
Keywords: kinematics, aging, motor planning, effort, gravity, optimal control
Citation: Poirier G, Papaxanthis C, Mourey F and Gaveau J (2020) Motor Planning of Vertical Arm Movements in Healthy Older Adults: Does Effort Minimization Persist With Aging? Front. Aging Neurosci. 12:37. doi: 10.3389/fnagi.2020.00037
Received: 20 November 2019; Accepted: 04 February 2020;
Published: 25 February 2020.
Edited by:
Kelly J. Cole, Appalachian State University, United StatesReviewed by:
Pranav J. Parikh, University of Houston, United StatesNabin Koirala, Haskins Laboratories, United States
Copyright © 2020 Poirier, Papaxanthis, Mourey and Gaveau. This is an open-access article distributed under the terms of the Creative Commons Attribution License (CC BY). The use, distribution or reproduction in other forums is permitted, provided the original author(s) and the copyright owner(s) are credited and that the original publication in this journal is cited, in accordance with accepted academic practice. No use, distribution or reproduction is permitted which does not comply with these terms.
*Correspondence: Jeremie Gaveau, amVyZW1pZS5nYXZlYXVAdS1ib3VyZ29nbmUuZnI=
†ORCID: Gabriel Poirier orcid.org/0000-0002-4005-114X
Charalambos Papaxanthis orcid.org/0000-0003-1955-8269
France Mourey orcid.org/0000-0002-4526-6561
Jeremie Gaveau orcid.org/0000-0001-8827-1486