- 1Department of Geriatrics, The First Affiliated Hospital, China Medical University, Shenyang, China
- 2Department of Neurology, The First Affiliated Hospital, China Medical University, Shenyang, China
- 3Department of Neurology, People's Hospital of Liaoning Province, Shenyang, China
- 4Computational Systems Biology Laboratory, Department of Biochemistry and Molecular Biology and Institute of Bioinformatics, The University of Georgia, Athens, GA, United States
- 5Cancer Systems Biology Center, The China–Japan Union Hospital, Jilin University, Changchun, China
- 6Department of Cardiology, The Shengjing Affiliated Hospital, China Medical University, Shenyang, China
Background: This meta-analysis aimed to evaluate the relationship between serum uric acid (UA) and the risk of dementia and its subtypes.
Methods: Embase, PubMed, and Web of Science were searched from inception to July 2020. Random-effect models were employed to analyze the standard mean difference (SMD) with the corresponding 95% confidence intervals (CI).
Results: Twenty-three eligible studies involving 5,575 participants were identified. The overall results showed lower levels of UA in dementia relative to non-dementia controls [SMD = −0.32 (−0.64; −0.01) p = 0.04]. The subgroup analysis of the type of dementia demonstrated a significant association of UA with Alzheimer's disease (AD) [SMD = −0.58 (−1.02; −0.15) p = 0.009] and Parkinson's disease with dementia (PDD) [SMD = −0.33 (−0.52; −0.14) p = 0.001] but not with vascular dementia (VaD). The stratification analysis of the concentrations of UA revealed that the UA quartile 1–2 was negatively correlated with dementia and neurodegenerative subtypes (p < 0.05), whereas a positive correlation of UA quartile 4 with dementia was noted (p = 0.028). Additionally, the meta-regression analysis on confounders showed that not age, body mass index, diabetes mellitus, hypertension, or smoking but education (p = 0.003) exerted an influence of the UA in the risk estimate of dementia.
Conclusions: Low concentrations of UA (< 292 μmol/L or 4.91 mg/dL) is a potential risk factor for AD and PDD but not for VaD. The mechanism of different concentrations of the UA in dementia needs to be confirmed through further investigation.
Introduction
Dementia is a group of acquired clinical syndromes characterized by the progressive decline in cognition along with psychiatric and behavioral alterations of differing extents (Tari et al., 2019; Zhou et al., 2020). More than 50 million people worldwide suffer from the overload burden of finance due to this clinical entity, a number that is alarmingly expected to triple by 2050 (Kivipelto et al., 2020). The seriousness and urgency of the present situation are that no licensed drugs are available to cure any type of dementia, such as Alzheimer's disease (AD), Parkinson's disease with dementia (PDD), and vascular dementia (VaD) (Jennings et al., 2020). Incurable, however, does not mean untreatable. Approximately one-third of the cases of AD are partially due to modifiable risk factors, many of which are related to lifestyles (e.g., smoking, physical inactivity, midlife obesity, and hypertension) (Norton et al., 2014). Subsequently, attention to lifestyle interventions has increasingly concentrated on patients with diagnostic dementia and the general population at a high risk of developing dementia (Ngandu et al., 2015).
Serum uric acid (UA), regulated by diet and physical activities, is the final breakdown of purine nucleotides that plays a crucial role in human metabolism (So and Thorens, 2010; Viazzi et al., 2016). Observational studies have shown that hyperuricemia was a determinant of long-term hypertension (Bjornstad et al., 2015), possibly representing an association of UA with renal and cardiovascular diseases (Feig et al., 2008; Kanbay et al., 2013). Despite extensive estimates of the risk of dementia based on UA, the conclusion remained ambiguous. Several lines of evidence suggested UA as an index of redox homeostasis, which alleviated the presence of degenerative cascades in neurodegenerative diseases (e.g., AD, PD, frontotemporal dementia, progressive supranuclear palsy, and corticobasal degeneration) (Vannorsdall et al., 2008; Paganoni and Schwarzschild, 2017; Schirinzi et al., 2017). On the other hand, serum UA might aggravate cerebral ischemia and increase the risk of dementia (Vannorsdall et al., 2008). To address these conflicts, an integrated retrieve of literature was undertaken to summarize the population-based data on the correlation between UA and dementia, aiming to afford a quantitative assessment for the risk of dementia and its subtypes under different concentration of UA.
Materials and Methods
Inclusion and Exclusion Criteria
Published studies that met the following criteria were considered eligible: (1) determinant dementia in cases (such as AD, PDD, and VaD) had to be based on certain standardized diagnostic criteria, e.g., the National Institute of Neurological and Communicative Disorders and Stroke–Alzheimer's Disease and Related Disorders Association (NINCDS–ADRDA) (McKhann et al., 1984), the International Classification of Diseases (ICD)-10 criteria, and the Diagnostic and Statistical Manual of Mental Disorders (DSM)-III, -IV, or -V criteria (American Psychiatric Association, 1980, 1987, 1994, 2013), etc.; (2) participants with no dementia served as controls (including but not limited to healthy individuals and PD with non-dementia); (3) the concentration of serum UA in dementia and matched controls was available in each study; and (4) there were no restrictions of the study design (case-control, longitudinal, or mix of them). The exclusion criteria were as follows: (1) duplicate data or population from the same research center; (2) receiving UA therapy or anti-gout drugs; (3) subjects examined with renal failure dialysis, severe liver dysfunctions, or malignant diseases; (4) non-English publications; and (5) animal studies, case reports, letters, reviews, or conference abstracts.
Literature Search
Two reviewers retrieved the electronic databases of Embase (August 1973–June 2020), PubMed (September 1974–July 2020), and Web of Science (March 1992–July 2020), which followed the developed guidelines of the preferred reporting items for systematic reviews and meta-analyses (PRISMA) (Moher et al., 2009) (Supplementary Table 1). Search terms in the aforementioned databases were “uric acid”, “urate”, “hyperuricemia”, “dementia”, “cognitive”, “amentia”, and “Alzheimer”. The reference lists of included studies and relevant overviews were studied manually to minimize the omission of potentially eligible articles.
Data Extraction and Synthesis
The following information of selected studies was independently extracted by two investigators: first author, year, country, detection method, gender distribution, mean age, and levels of UA (Table 1). For discrepancies of the data, we negotiated with a third reviewer or consulted the authors of the original report. Other general characteristics, including body mass index (BMI), education, male gender, smoking, hypertension, and diabetes mellitus, were also summarized. As shown in Table 2, the association between general characteristics and the risk of dementia was evaluated.
Statistical Analysis
Software STATA version 15.0 and Review Manager 5.3 were used to conduct the meta-analyses. The effect size (ES) with a corresponding 95% confidence interval (CI) was identified by a z-test in which continuous and classified variables were measured by standard mean differences (SMD) and odds ratio (OR), respectively. The statistical heterogeneity between studies was assessed by the I2 test, with a value higher than 50% considering heterogeneity (Zintzaras and Ioannidis, 2005). A random-effect model was appropriate if there was substantial heterogeneity within the study populations (DerSimonian and Laird, 1986). Subgroup analyses on the type of dementia (AD, VaD, and PDD) and the concentrations of serum UA (quartile 1–4: < 262, 262–292, 292–316, > 316 μmol/L; or < 4.40, 4.40–4.91, 4.91–5.31, > 5.31 mg/dL) were carried out in levels of UA for the risk estimates of dementia. Meta-regression analyses were then applied to investigate how potential confounding factors affected the correlation of UA to dementia. A sensitivity analysis was conducted by omitting each study to assess its impact on the pooled ES. For continuous data with the mean difference as the effect index, Egger's linear regression test was selected, and p ≥ 0.05 suggested a low possibility of publication bias (Peters et al., 2006).
Results
Study Selection and Characteristics
A total of 1,202 articles were screened in the initial search, of which 96 studies were retained by inspecting titles and abstracts. Then, 73 articles were excluded through a comprehensive review of the full texts. Finally, 23 articles, which met the inclusion criteria, were included in the meta-analysis (for detailed steps, see Figure 1) (Maesaka et al., 1993; Tohgi et al., 1993; Foy et al., 1999; Polidori and Mecocci, 2002; Rinaldi et al., 2003; Pulido et al., 2005; Kim et al., 2006; Baldeiras et al., 2008; Cascalheira et al., 2009; Annanmaki et al., 2011; Cankurtaran et al., 2013; Cervellati et al., 2014; González-Aramburu et al., 2014; Al-khateeb et al., 2015; Hatanaka et al., 2015; Pellecchia et al., 2016; Pu et al., 2017; Tuven et al., 2017; Xu et al., 2017; Wang et al., 2018; Zuliani et al., 2018; Boccardi et al., 2020; Serdarevic et al., 2020). Figure 1 outlines a flowchart regarding the screening process of the inclusion of literature. The general characteristics of 23 studies, including 1,566 dementia patients and 4,009 non-dementia controls, are shown in Table 1. The pooled ES of baseline characteristics is summarized in Table 2. The SMD of each study and the overall studies in serum UA for the estimates of dementia risk are exhibited in Figure 2. Subgroup analyses were performed to identify the association between UA and dementia based on the classification of dementia (AD, PDD, and VaD) (Figure 3), concentrations of UA in patients with dementia (Figure 4), and neurodegenerative dementia (AD and PDD) (Figure 5). In the subgroup analysis of PDD, patients with PD without dementia served as the control group. Meta-regression analyses on age (Figure 6A), BMI (Figure 6B), diabetes mellitus (Figure 6C), education (Figure 6D), hypertension (Figure 6E), and smoking (Figure 6F) were conducted to evaluate the impact of these potential confounders on the ES of dementia in relation to UA. Data on physical activities and dietary habits were unavailable for the meta-regression analysis.
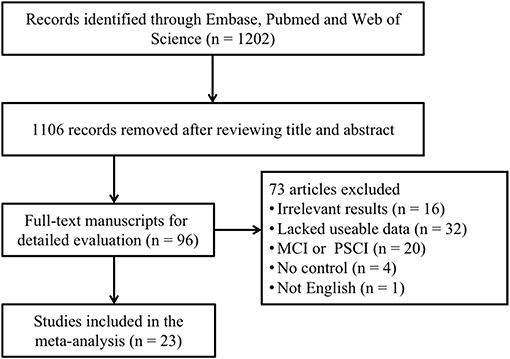
Figure 1. A flowchart of the screening of the study and the selection in the meta-analysis. MCI, mild cognitive impairment; PSCI, post-stroke cognitive impairment.
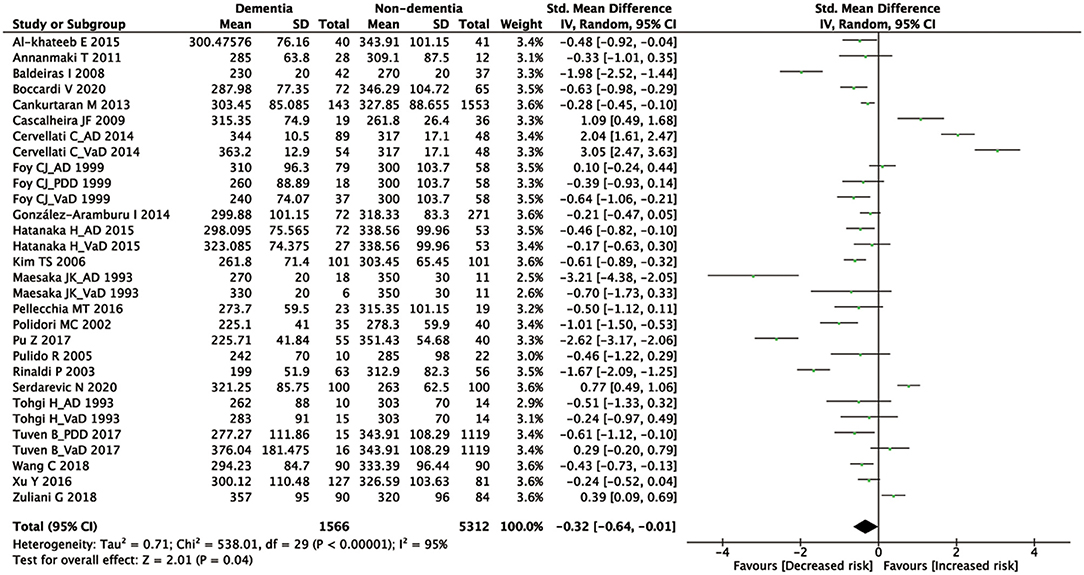
Figure 2. A forest plot of the SMD in the levels of UA between dementia and non-dementia. CI, confidence interval; SMD, standard mean difference; UA, uric acid.
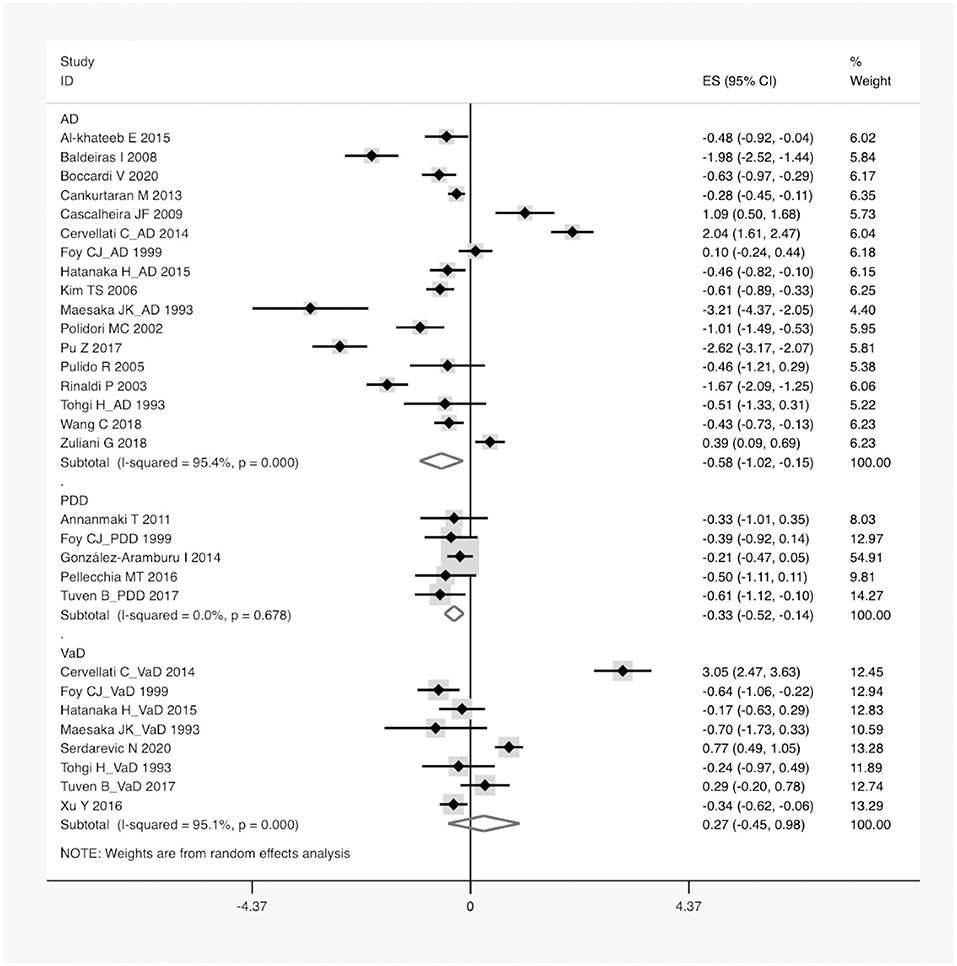
Figure 3. A forest plot of the subgroup analysis on the type of dementia in UA between dementia and non-dementia. AD, Alzheimer's disease; PDD, Parkinson's disease with dementia; VaD, vascular dementia; ES, effect size; CI, confidence interval; UA, uric acid.
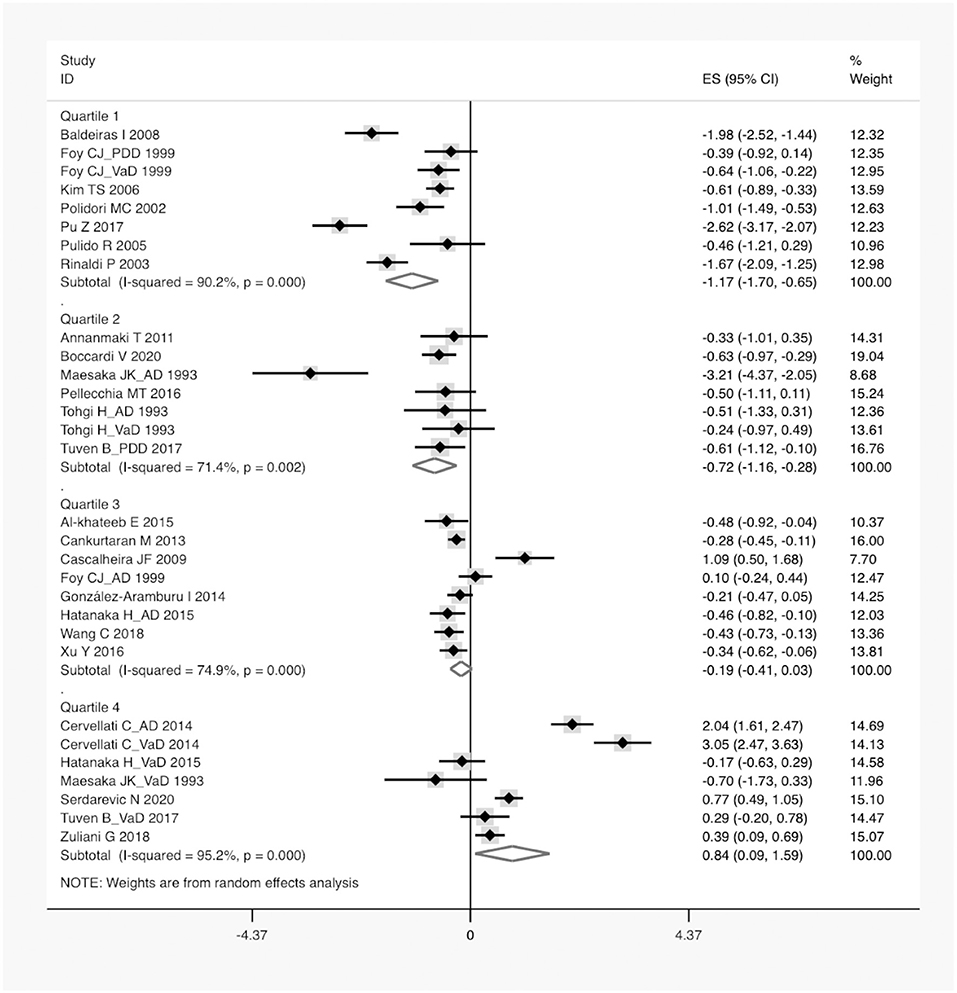
Figure 4. A forest plot of the subgroup analysis on the concentration of serum UA between dementia and non-dementia. ES, effect size; CI, confidence interval; UA, uric acid.
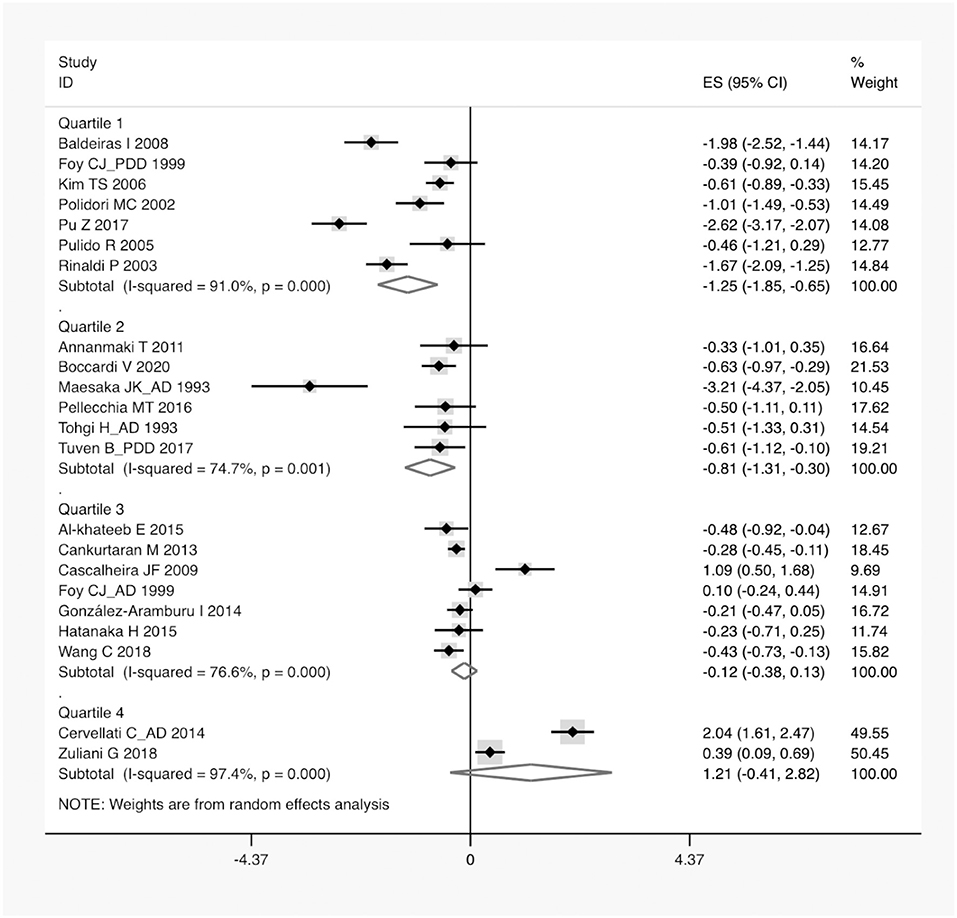
Figure 5. A forest plot of the subgroup analysis on the concentration of serum UA in neurodegenerative dementia compared with non-dementia. ES, effect size; CI, confidence interval; UA, uric acid.
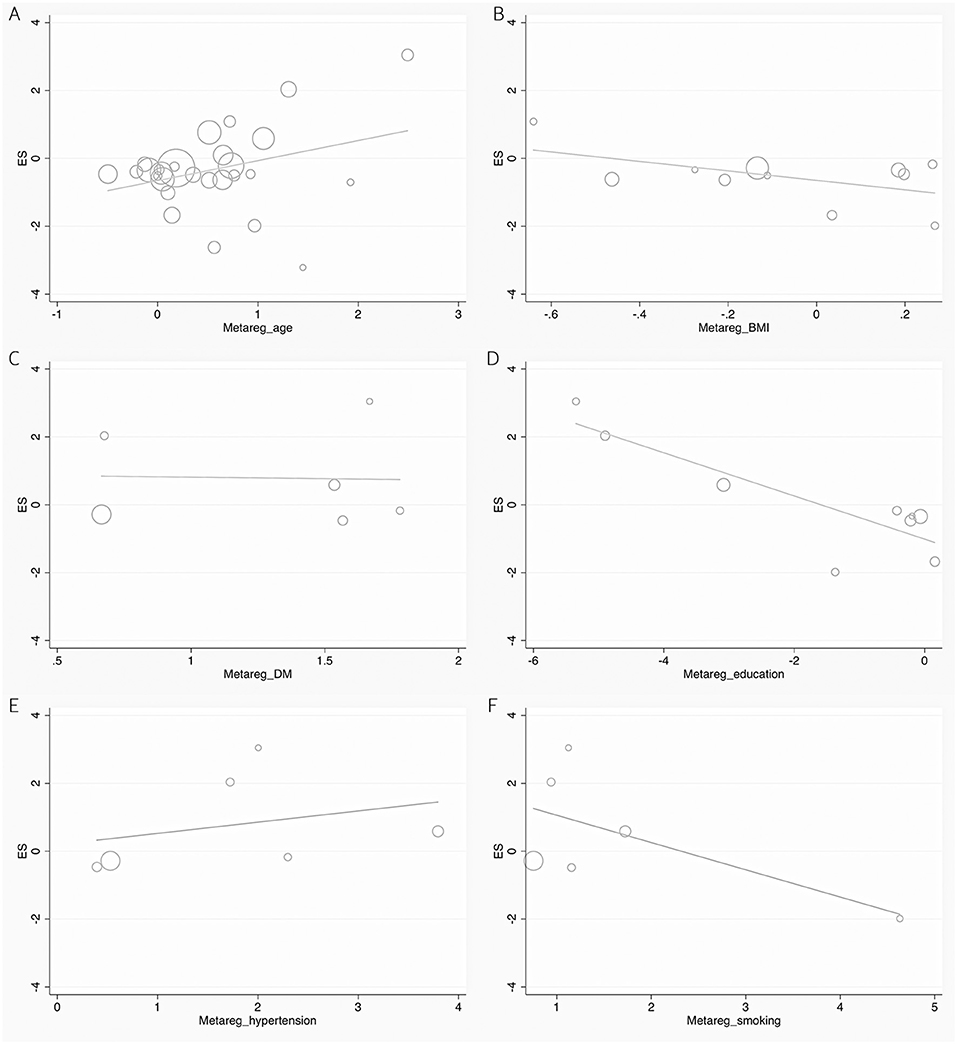
Figure 6. Forest plots of the meta-regression analyses on age (A), BMI (B), DM (C), education (D), hypertension (E), and smoking (F) in UA between dementia and non-dementia. BMI, body mass index; DM, diabetes mellitus; ES, effect size; UA, uric acid.
Meta-Analysis
According to the presence of heterogeneity (I2 = 95%, p < 0.01), the random-effect models were used to render the conservation of estimates by the weight disproportionation in small studies. The combined results of 23 studies showed lower levels of UA in patients with dementia compared with patients without dementia [SMD = −0.32 (−0.64; −0.01) p = 0.04] (Figure 2). As shown in Table 2, we found no statistic differences of the pooled weighted characteristics on BMI [SMD = −0.07 (−0.23; 0.10) p = 0.429], male gender [OR = 1.01 (0.80; 1.27) p = 0.933], smoking [OR = 1.08 (0.72; 1.62) p = 0.698], hypertension [OR = 1.32 (0.60; 2.91) p = 0.490], and diabetes mellitus [OR = 1.05 (0.69; 1.60) p = 0.806] between dementia and non-dementia controls; while older age [SMD = 0.51 (0.30; 0.71) p = 0.001] and lower educational attainment [SMD = −1.69 (−2.78; −0.60) p = 0.002] appeared in patients with dementia than at in controls with non-dementia. The subgroup analysis of the type of dementia (Figure 3) showed an association of the levels of UA with AD [SMD = −0.58 (−1.02; −0.15) p = 0.009] and PDD [SMD = −0.33 (−0.52; −0.14) p = 0.001] but not with VaD [SMD = 0.27 (−0.45; 0.98) p = 0.466]. The UA quartile 1 [SMD = −1.17 (−1.70; −0.65) p < 0.01], 2 [SMD = −0.72 (−1.16; −0.28) p = 0.02], and 4 [SMD = 0.84 (0.09; 1.59) p = 0.028] were significantly related to dementia, whereas no relationship was noted in UA quartile 3 [SMD = −0.19 (−0.41; 0.03) p = 0.093] (Figure 4). There was a negative correlation of UA quartile 1 [SMD = −1.25 (−1.85; −0.65) p < 0.01] and 2 [SMD = −0.81 (−1.31; −0.30) p = 0.02] with neurodegenerative dementia (Figure 5). The meta-regression analyses (Figure 6) revealed an impact of education (p = 0.003, Adj R-squared = 72.36%) on the relationship of UA with dementia; but age (p = 0.093, Adj R-squared = 10.20%), BMI (p = 0.087, Adj R-squared = 21.32%), diabetes mellitus (p = 0.952, Adj R-squared = −25.92%), hypertension (p = 0.576, Adj R-squared = −15.10%), and smoking (p = 0.167, Adj R-squared = 27.46%) exerted no influence on the relationship.
Sensitivity Analysis and Publication Bias
After removing one of the included studies at a time, a robust result of the pooled ES was presented in the sensitivity analysis (Supplementary Figure 1). The result of the Egger's regression analysis (p = 0.997) indicated no evidence of publication bias (Supplementary Figure 2).
Discussion
In the current meta-analysis, 23 studies, involving 5,575 participants, were generalized to evaluate the relationship between the levels of serum UA and the risk of dementia. In spite of substantial heterogeneity across studies, the pooled ES showed an increased risk of dementia in individuals with lower levels of UA. To the best of our knowledge, this is the first meta-analysis to quantitatively assess the risk of dementia by different gradients of concentrations of UA. Previously, a meta-analysis lacking sufficient statistical power had been conducted under the influence of confounders (Khan et al., 2016). Moreover, it differed from our study in that the post-stroke cognitive impairment (PSCI) and mild cognitive impairment (MCI) were enrolled in VaD and pooled dementia, respectively. Taking into account these limitations, our objective was to perform an updated overview and meta-analysis for the dose-response effects of serum UA in the risk of dementia and its subtypes.
The findings emerging from the subgroup analysis of the type of dementia demonstrated a direct and negative correlation between serum UA and AD, indicating that low levels of UA might be a risk factor of AD. As a major cause of dementia, AD was characterized by amyloid beta (Aβ), neurofibrillary tangles, and neuritic plaques (Ballard et al., 2011). These pathological hallmarks were observed to be associated with the oxidative stress (Desideri et al., 2017). Convincing evidence showed that the oxidative damage to enzymes of glycolysis, tricarboxylic acid cycle, and the biosynthesis of ATP contributed significantly to the pathogenesis of AD and its progression (Butterfield and Halliwell, 2019). UA, a powerful antioxidant and iron chelator, accounted for ~60% of the scavenging capacity of free radicals in the human body (Ames et al., 1981; Davies et al., 1986). In the case of subnormal levels of UA, the resultant accumulation of the oxidative stress enhanced Aβ-activated apoptosis, a process believed to underlie the development of AD (Desideri et al., 2017). By inhibiting the production of the reactive oxygen species, serum UA had neuroprotective implications in brain aging and cognitive impairment, as demonstrated in the Three-City Dijon cohort study (Latourte et al., 2018). In line with our results, two large population-based cohort studies presented a decreased risk of non-VaD in patients with gout (Hong et al., 2015; Lu et al., 2016).
The subgroup analysis of another neurodegenerative dementia, PDD, also exhibited lower concentrations of UA relative to controls with non-dementia. The involvement of low levels of UA has been reported not only in the onset of PD but also in established PD with worse motor symptoms (de Lau et al., 2005; Winquist et al., 2010). It is worth noting that patients with PD with non-dementia served as the control group. The pathological interference of PD on the metabolism of UA tended to be eliminated; thus, to draw the conclusion that low levels of UA were likely an independent causative factor of PDD. This was consistent with additional evidence that elevated levels of UA were a predictor of slow progression in non-motor symptoms of PD, including cognitive impairment (Moccia et al., 2015; Huang et al., 2018). The underlying mechanism of cognitive decline in PD was complex and likely involved several systems (Gratwicke et al., 2015). Among them, a possible interpretation was that the deposition of oxidants and iron in the substantia nigra of PD enhanced the oxidative stress, which impaired the dopaminergic projection from the substantia nigra to the cortex, leading to executive and attentional dysfunctions in PD (Dexter et al., 1989; Fahn and Cohen, 1992). Coincidentally, the antioxidant and iron scavenger traits of UA might provide natural neuroprotection against the dementing process in PD (Schlesinger and Schlesinger, 2008; Annanmaki et al., 2011).
Nevertheless, we found non-significant relationships of UA with VaD, which might account for the reduced ES of the risk of dementia when all subtypes of dementia merged. A plausible explanation was that the vulnerability of ischemic brain to the oxidative stress increased with the decrease in the levels of UA, leading to a series of VaD-related pathological reactions, such as free radical generation, lipid peroxidation, mitochondrial dysfunction, and excitatory toxicity (Sugawara and Chan, 2003; Allen and Bayraktutan, 2009; Amaro et al., 2015). The administration of serum UA has been shown to prevent neurons from excitotoxic insults in acute ischemic stroke, which contributed to the limitation of the infarct growth and an improvement in the behavioral dysfunction (Amaro et al., 2015). Conversely, several lines of evidence presented an interaction of high levels of UA with hypertension and metabolic syndrome, which synergistically aggravated atherosclerosis to yield VaD (Raffaitin et al., 2009; Richette et al., 2014; Borghi et al., 2015). Based on our findings, it was hypothesized that the pro-arteriosclerotic capacity of UA might counteract its antioxidant property, resulting in the ineffectiveness of UA in the development of VaD.
Furthermore, a linear dose-response correlation of serum UA with the risk of dementia was identified in this study. More specifically, lower levels of UA (<292 μmol/L) were negatively correlated with dementia and the subtypes of neurodegerative dementia, whereas a positive association of higher levels of UA (>316 μmol/L) with dementia was found after a smooth transition over a safe interval. This was consistent with previous studies that fluctuations in the concentrations of UA was linked to altered brain function and cognitive decline (Beydoun et al., 2016; Latourte et al., 2018; Tana et al., 2018). In the data of pooled weighted characteristics, the prevalence of dementia increased with an increase in age and lower educational attainment, in line with the epidemiological survey that nearly one in eight individuals suffered from dementia by the age of 80 (Wald et al., 2011). Further meta-regression analyses revealed that not age, BMI, smoking, hypertension, or diabetes mellitus but education exerted an impact on the relationship of UA with the risk of dementia. The sensitivity analysis showed no statistical difference in serum UA after the removal of one study at a time, suggesting the relatively robust ES of pooled results. Large cohort studies on serum UA (not limited to the concentration range in this study) in different types of dementia are required to validate the association between the levels of UA and the risk of dementia.
Limitations
Several limitations should be noted in our study. Substantial heterogeneity across studies was detected, partially attributed to different attainment of education. Given the inclusion of PD with non-dementia as controls, risk estimates for pooled dementia should be interpreted with caution. Since the individual data of the concentrations of UA were unavailable in each study, there were certain deviations for the subgroup analysis using the mean concentrations of UA. Other potential sources of heterogeneity might be derived from diversities in the cutoff values, detection methods, diagnostic criteria, underlying diseases, dietary habits, and physical activities, some of which have not been analyzed in this meta-analysis and are worthy of further investigation.
Conclusion
In aggregate, low levels of the concentration of UA (<292 μmol/L or 4.91 mg/dL) were a potential risk factor for AD and PDD rather than VaD. Additional in vivo or in vitro experiments are needed to elucidate the mechanisms by which changes in the levels of the concentration of UA are underlying causes of dementia.
Data Availability Statement
The raw data supporting the conclusions of this article will be made available by the authors without undue reservation.
Author Contributions
The study was conceived by MZ, CZ, and ZZ. The literature search and selection were conducted by SZ and XZ. The data were extracted and analyzed by YL, KK, YX, RZ, and HQ. The rough manuscript was drafted by MZ, CZ, and ZZ. All authors reviewed and approved the final version of the manuscript.
Funding
The study was supported by the National Natural Science Foundation of China (CZ, No. 81372104); the Natural Science Foundation of Liaoning Province (ZZ, No. 20180540150); the Shenyang Population and Health Technical Critical Special Project (CZ, No. F16-206-9-01); and the Program of the Distinguished Professor of Liaoning Province (CZ).
Conflict of Interest
The authors declare that the research was conducted in the absence of any commercial or financial relationships that could be construed as a potential conflict of interest.
Acknowledgments
All the authors of included original studies should be appreciated sincerely.
Supplementary Material
The Supplementary Material for this article can be found online at: https://www.frontiersin.org/articles/10.3389/fnagi.2021.625690/full#supplementary-material
Supplementary Table 1. The developed guidelines of preferred reporting items for systematic reviews and meta-analysis (PRISMA).
Supplementary Figure 1. A forest plot of a sensitivity analysis by omitting the given named study.
Supplementary Figure 2. A funnel plot of the Egger's test to detect the risk of publication bias in the meta-analysis.
References
Al-khateeb, E., Althaher, A., Al-khateeb, M., Al-Musawi, H., Azzouqah, O., Al-Shweiki, S., et al. (2015). Relation between uric acid and Alzheimer's disease in elderly Jordanians. J. Alzheimers Dis. 44, 859–865. doi: 10.3233/JAD-142037
Allen, C.L., and Bayraktutan, U. (2009). Oxidative stress and its role in the pathogenesis of ischaemic stroke. Int. J. Stroke 4, 461–470. doi: 10.1111/j.1747-4949.2009.00387.x
Amaro, S., Llull, L., Renú, A., Laredo, C., Perez, B., Vila, E., et al. (2015). Uric acid improves glucose-driven oxidative stress in human ischemic stroke. Ann. Neurol. 77, 775–783. doi: 10.1002/ana.24378
American Psychiatric Association (1980). Diagnostic and Statistical Manual of MentalDisorders, 3rd Edn. Washington, DC: American Psychiatric Association.
American Psychiatric Association (1987). Diagnostic and Statistical Manual of MentalDisorders, 3rd Edn. Washington, DC: American Psychiatric Association.
American Psychiatric Association (1994). Diagnostic and Statistical Manual of Mental Disorders: DSM-IV, 4th Edn. Washington, DC: American Psychiatric Association.
American Psychiatric Association (2013). Diagnostic and Statistical Manual of Mental Disorders, DSM-5, 5th Edn. Washington, DC: American Psychiatric Association. doi: 10.1176/appi.books.9780890425596
Ames, B.N., Cathcart, R., Schwiers, E., and Hochstein, P. (1981). Uric acid provides an antioxidant defense in humans against oxidant- and radical-caused aging and cancer: a hypothesis. Proc. Natl. Acad. Sci. U. S. A. 78, 6858–6862. doi: 10.1073/pnas.78.11.6858
Annanmaki, T., Pohja, M., Parviainen, T., Hakkinen, P., and Murros, K. (2011). Uric acid and cognition in Parkinson's disease: a follow-up study. Parkinsonism Relat. Disord. 17, 333–337. doi: 10.1016/j.parkreldis.2011.01.013
Baldeiras, I., Santana, I., Proença, M.T., Garrucho, M.H., Pascoal, R., Rodrigues, A., et al. (2008). Peripheral oxidative damage in mild cognitive impairment and mild Alzheimer's disease. J. Alzheimers Dis. 15, 117–128. doi: 10.3233/JAD-2008-15110
Ballard, C., Gauthier, S., Corbett, A., Brayne, C., Aarsland, D., and Jones, E. (2011). Alzheimer's disease. Lancet 377, 1019–1031. doi: 10.1016/S0140-6736(10)61349-9
Beydoun, M.A., Canas, J.-A., Dore, G.A., Beydoun, H.A., Rostant, O.S., Fanelli-Kuczmarski, M.T., et al. (2016). Serum uric acid and its association with longitudinal cognitive change among urban adults. J. Alzheimers Dis. 52, 1415–1430. doi: 10.3233/JAD-160028
Bjornstad, P., Lanaspa, M.A., Ishimoto, T., Kosugi, T., Kume, S., Jalal, D., et al. (2015). Fructose and uric acid in diabetic nephropathy. Diabetologia 58, 1993–2002. doi: 10.1007/s00125-015-3650-4
Boccardi, V., Carino, S., Marinelli, E., Lapenna, M., Caironi, G., Bianco, A.R., et al. (2020). Uric acid and late-onset Alzheimer's disease: results from the ReGAl 2.0 project. Aging Clin. Exp. Res. doi: 10.1007/s40520-020-01541-z. [Epub ahead of print].
Borghi, C., Rosei, E.A., Bardin, T., Dawson, J., Dominiczak, A., Kielstein, J.T., et al. (2015). Serum uric acid and the risk of cardiovascular and renal disease. J. Hypertens. 33:701. doi: 10.1097/HJH.0000000000000701
Butterfield, D.A., and Halliwell, B. (2019). Oxidative stress, dysfunctional glucose metabolism and Alzheimer disease. Nat. Rev. Neurosci. 20, 148–160. doi: 10.1038/s41583-019-0132-6
Cankurtaran, M., Yesil, Y., Kuyumcu, M.E., Oztürk, Z.A., Yavuz, B.B., Halil, M., et al. (2013). Altered levels of homocysteine and serum natural antioxidants links oxidative damage to Alzheimer's disease. J. Alzheimers Dis. 33, 1051–1058. doi: 10.3233/JAD-2012-121630
Cascalheira, J.F., João, S.S., Pinhanços, S.S., Castro, R., Palmeira, M., Almeida, S., et al. (2009). Serum homocysteine: interplay with other circulating and genetic factors in association to Alzheimer's type dementia. Clin. Biochem. 42, 783–790. doi: 10.1016/j.clinbiochem.2009.02.006
Cervellati, C., Romani, A., Seripa, D., Cremonini, E., Bosi, C., Magon, S., et al. (2014). Oxidative balance, homocysteine, and uric acid levels in older patients with Late Onset Alzheimer's Disease or Vascular Dementia. J. Neurol. Sci. 337, 156–161. doi: 10.1016/j.jns.2013.11.041
Davies, K.J., Sevanian, A., Muakkassah-Kelly, S.F., and Hochstein, P. (1986). Uric acid-iron ion complexes. A new aspect of the antioxidant functions of uric acid. Biochem. J. 235, 747–754. doi: 10.1042/bj2350747
de Lau, L.M.L., Koudstaal, P.J., Hofman, A., and Breteler, M.M.B. (2005). Serum uric acid levels and the risk of Parkinson disease. Ann. Neurol. 58, 797–800. doi: 10.1002/ana.20663
DerSimonian, R., and Laird, N. (1986). Meta-analysis in clinical trials. Control Clin. Trials 7, 177–188. doi: 10.1016/0197-2456(86)90046-2
Desideri, G., Gentile, R., Antonosante, A., Benedetti, E., Grassi, D., Cristiano, L., et al. (2017). Uric acid amplifies Aβ amyloid effects involved in the cognitive dysfunction/dementia: evidences from an experimental model in vitro. J. Cell Physiol. 232, 1069–1078. doi: 10.1002/jcp.25509
Dexter, D.T., Wells, F.R., Lees, A.J., Agid, F., Agid, Y., Jenner, P., et al. (1989). Increased nigral iron content and alterations in other metal ions occurring in brain in Parkinson's disease. J. Neurochem. 52, 1830–1836. doi: 10.1111/j.1471-4159.1989.tb07264.x
Fahn, S., and Cohen, G. (1992). The oxidant stress hypothesis in Parkinson's disease: evidence supporting it. Ann. Neurol. 32, 804–812. doi: 10.1002/ana.410320616
Feig, D.I., Kang, D.-H., and Johnson, R.J. (2008). Uric acid and cardiovascular risk. N. Engl. J. Med. 359, 1811–1821. doi: 10.1056/NEJMra0800885
Foy, C.J., Passmore, A.P., Vahidassr, M.D., Young, I.S., and Lawson, J.T. (1999). Plasma chain-breaking antioxidants in Alzheimer's disease, vascular dementia and Parkinson's disease. QJM 92, 39–45. doi: 10.1093/qjmed/92.1.39
González-Aramburu, I., Sánchez-Juan, P., Sierra, M., Fernández-Juan, E., Sánchez-Quintana, C., Berciano, J., et al. (2014). Serum uric acid and risk of dementia in Parkinson's disease. Parkinsonism Relat. Disord. 20, 637–639. doi: 10.1016/j.parkreldis.2014.02.023
Gratwicke, J., Jahanshahi, M., and Foltynie, T. (2015). Parkinson's disease dementia: a neural networks perspective. Brain 138, 1454–1476. doi: 10.1093/brain/awv104
Hatanaka, H., Hanyu, H., Fukasawa, R., Hirao, K., Shimizu, S., Kanetaka, H., et al. (2015). Differences in peripheral oxidative stress markers in Alzheimer's disease, vascular dementia and mixed dementia patients. Geriatr. Gerontol. Int. 15(Suppl.1), 53–58. doi: 10.1111/ggi.12659
Hong, J.-Y., Lan, T.-Y., Tang, G.-J., Tang, C.-H., Chen, T.-J., and Lin, H.-Y. (2015). Gout and the risk of dementia: a nationwide population-based cohort study. Arthrit. Res. Ther. 17:139. doi: 10.1186/s13075-015-0642-1
Huang, X., Ng, S.Y.-E., Chia, N.S.-Y., Acharyya, S., Setiawan, F., Lu, Z.H., et al. (2018). Serum uric acid level and its association with motor subtypes and non-motor symptoms in early Parkinson's disease: PALS study. Parkinsonism Relat. Disord. 55, 50–54. doi: 10.1016/j.parkreldis.2018.05.010
Jennings, A., Cunnane, S.C., and Minihane, A.M. (2020). Can nutrition support healthy cognitive ageing and reduce dementia risk? BMJ 369:m2269. doi: 10.1136/bmj.m2269
Kanbay, M., Segal, M., Afsar, B., Kang, D.-H., Rodriguez-Iturbe, B., and Johnson, R.J. (2013). The role of uric acid in the pathogenesis of human cardiovascular disease. Heart 99, 759–766. doi: 10.1136/heartjnl-2012-302535
Khan, A.A., Quinn, T.J., Hewitt, J., Fan, Y., and Dawson, J. (2016). Serum uric acid level and association with cognitive impairment and dementia: systematic review and meta-analysis. Age. 38:16. doi: 10.1007/s11357-016-9871-8
Kim, T.-S., Pae, C.-U., Yoon, S.-J., Jang, W.-Y., Lee, N.J., Kim, J.-J., et al. (2006). Decreased plasma antioxidants in patients with Alzheimer's disease. Int. J. Geriatr. Psychiatry 21, 344–348. doi: 10.1002/gps.1469
Kivipelto, M., Mangialasche, F., Snyder, H.M., Allegri, R., Andrieu, S., Arai, H., et al. (2020). World-Wide FINGERS Network: a global approach to risk reduction and prevention of dementia. Alzheimers Dement. 16, 1078–1094. doi: 10.1002/alz.12123
Latourte, A., Soumaré, A., Bardin, T., Perez-Ruiz, F., Debette, S., and Richette, P. (2018). Uric acid and incident dementia over 12 years of follow-up: a population-based cohort study. Ann. Rheum. Dis. 77, 328–335. doi: 10.1136/annrheumdis-2016-210767
Lu, N., Dubreuil, M., Zhang, Y., Neogi, T., Rai, S.K., Ascherio, A., et al. (2016). Gout and the risk of Alzheimer's disease: a population-based, BMI-matched cohort study. Ann. Rheum. Dis. 75, 547–551. doi: 10.1136/annrheumdis-2014-206917
Maesaka, J.K., Wolf-Klein, G., Piccione, J.M., and Ma, C.M. (1993). Hypouricemia, abnormal renal tubular urate transport, and plasma natriuretic factor(s) in patients with Alzheimer's disease. J. Am. Geriatr. Soc. 41, 501–506. doi: 10.1111/j.1532-5415.1993.tb01885.x
McKhann, G., Drachman, D., Folstein, M., Katzman, R., Price, D., and Stadlan, E.M. (1984). Clinical diagnosis of Alzheimer's disease: report of the NINCDS-ADRDA Work Group under the auspices of Department of Health and Human Services Task Force on Alzheimer's Disease. Neurology 34, 939–944. doi: 10.1212/WNL.34.7.939
Moccia, M., Picillo, M., Erro, R., Vitale, C., Longo, K., Amboni, M., et al. (2015). Presence and progression of non-motor symptoms in relation to uric acid in de novo Parkinson's disease. Eur. J. Neurol. 22, 93–98. doi: 10.1111/ene.12533
Moher, D., Liberati, A., Tetzlaff, J., and Altman, D.G. (2009). Preferred reporting items for systematic reviews and meta-analyses: the PRISMA statement. PLoS Med. 6:e1000097. doi: 10.1371/journal.pmed.1000097
Ngandu, T., Lehtisalo, J., Solomon, A., Levälahti, E., Ahtiluoto, S., Antikainen, R., et al. (2015). A 2 year multidomain intervention of diet, exercise, cognitive training, and vascular risk monitoring versus control to prevent cognitive decline in at-risk elderly people (FINGER): a randomised controlled trial. Lancet 385, 2255–2263. doi: 10.1016/S0140-6736(15)60461-5
Norton, S., Matthews, F.E., Barnes, D.E., Yaffe, K., and Brayne, C. (2014). Potential for primary prevention of Alzheimer's disease: an analysis of population-based data. Lancet Neurol. 13, 788–794. doi: 10.1016/S1474-4422(14)70136-X
Paganoni, S., and Schwarzschild, M.A. (2017). Urate as a marker of risk and progression of neurodegenerative disease. Neurotherapeutics 14, 148–153. doi: 10.1007/s13311-016-0497-4
Pellecchia, M.T., Savastano, R., Moccia, M., Picillo, M., Siano, P., Erro, R., et al. (2016). Lower serum uric acid is associated with mild cognitive impairment in early Parkinson's disease: a 4-year follow-up study. J. Neural. Transm. 123, 1399–1402. doi: 10.1007/s00702-016-1622-6
Peters, J.L., Sutton, A.J., Jones, D.R., Abrams, K.R., and Rushton, L. (2006). Comparison of two methods to detect publication bias in meta-analysis. JAMA 295, 676–680. doi: 10.1001/jama.295.6.676
Polidori, M.C., and Mecocci, P. (2002). Plasma susceptibility to free radical-induced antioxidant consumption and lipid peroxidation is increased in very old subjects with Alzheimer disease. J. Alzheimers Dis. 4, 517–522. doi: 10.3233/JAD-2002-4608
Pu, Z., Xu, W., Lin, Y., He, J., and Huang, M. (2017). Oxidative stress markers and metal ions are correlated with cognitive function in Alzheimer's disease. Am. J. Alzheimers Dis. Other Demen. 32, 353–359. doi: 10.1177/1533317517709549
Pulido, R., Jiménez-Escrig, A., Orensanz, L., Saura-Calixto, F., and Jiménez-Escrig, A. (2005). Study of plasma antioxidant status in Alzheimer's disease. Eur. J. Neurol. 12, 531–535. doi: 10.1111/j.1468-1331.2005.01000.x
Raffaitin, C., Gin, H., Empana, J.-P., Helmer, C., Berr, C., Tzourio, C., et al. (2009). Metabolic syndrome and risk for incident Alzheimer's disease or vascular dementia: the Three-City Study. Diabet. Care 32, 169–174. doi: 10.2337/dc08-0272
Richette, P., Perez-Ruiz, F., Doherty, M., Jansen, T.L., Nuki, G., Pascual, E., et al. (2014). Improving cardiovascular and renal outcomes in gout: what should we target? Nat. Rev. Rheumatol. 10, 654–661. doi: 10.1038/nrrheum.2014.124
Rinaldi, P., Polidori, M.C., Metastasio, A., Mariani, E., Mattioli, P., Cherubini, A., et al. (2003). Plasma antioxidants are similarly depleted in mild cognitive impairment and in Alzheimer's disease. Neurobiol. Aging 24, 915–919. doi: 10.1016/S0197-4580(03)00031-9
Schirinzi, T., Di Lazzaro, G., Colona, V.L., Imbriani, P., Alwardat, M., Sancesario, G.M., et al. (2017). Assessment of serum uric acid as risk factor for tauopathies. J. Neural. Transm. 124, 1105–1108. doi: 10.1007/s00702-017-1743-6
Schlesinger, I., and Schlesinger, N. (2008). Uric acid in Parkinson's disease. Mov. Disord. 23, 1653–1657. doi: 10.1002/mds.22139
Serdarevic, N., Stanciu, A.E., Begic, L., and Uncanin, S. (2020). Serum uric acid concentration in patients with cerebrovascular disease (ischemic stroke and vascular dementia). Med. Arch. 74, 95–99. doi: 10.5455/medarh.2020.74.95-99
So, A., and Thorens, B. (2010). Uric acid transport and disease. J. Clin. Invest. 120, 1791–1799. doi: 10.1172/JCI42344
Sugawara, T., and Chan, P.H. (2003). Reactive oxygen radicals and pathogenesis of neuronal death after cerebral ischemia. Antioxid Redox Signal 5, 597–607. doi: 10.1089/152308603770310266
Tana, C., Ticinesi, A., Prati, B., Nouvenne, A., and Meschi, T. (2018). Uric acid and cognitive function in older individuals. Nutrients 10:975. doi: 10.3390/nu10080975
Tari, A.R., Nauman, J., Zisko, N., Skjellegrind, H.K., Bosnes, I., Bergh, S., et al. (2019). Temporal changes in cardiorespiratory fitness and risk of dementia incidence and mortality: a population-based prospective cohort study. Lancet Public Health. 4, e565–e574. doi: 10.1016/S2468-2667(19)30183-5
Tohgi, H., Abe, T., Takahashi, S., and Kikuchi, T. (1993). The urate and xanthine concentrations in the cerebrospinal fluid in patients with vascular dementia of the Binswanger type, Alzheimer type dementia, and Parkinson's disease. J. Neural. Transm. Park Dis. Dement. Sect 6, 119–126. doi: 10.1007/BF02261005
Tuven, B., Soysal, P., Unutmaz, G., Kaya, D., and Isik, A.T. (2017). Uric acid may be protective against cognitive impairment in older adults, but only in those without cardiovascular risk factors. Exp. Gerontol. 89, 15–19. doi: 10.1016/j.exger.2017.01.002
Vannorsdall, T.D., Jinnah, H.A., Gordon, B., Kraut, M., and Schretlen, D.J. (2008). Cerebral ischemia mediates the effect of serum uric acid on cognitive function. Stroke 39, 3418–3420. doi: 10.1161/STROKEAHA.108.521591
Viazzi, F., Rebora, P., Giussani, M., Orlando, A., Stella, A., Antolini, L., et al. (2016). Increased serum uric acid levels blunt the antihypertensive efficacy of lifestyle modifications in children at cardiovascular risk. Hypertension 67, 934–940. doi: 10.1161/HYPERTENSIONAHA.115.06852
Wald, D.S., Kasturiratne, A., and Simmonds, M. (2011). Serum homocysteine and dementia: meta-analysis of eight cohort studies including 8,669 participants. Alzheimers Dement. 7, 412–417. doi: 10.1016/j.jalz.2010.08.234
Wang, C., Fei, G., Pan, X., Sang, S., Wang, L., Zhong, C., et al. (2018). High thiamine diphosphate level as a protective factor for Alzheimer's disease. Neurol. Res. 40, 658–665. doi: 10.1080/01616412.2018.1460704
Winquist, A., Steenland, K., and Shankar, A. (2010). Higher serum uric acid associated with decreased Parkinson's disease prevalence in a large community-based survey. Mov. Disord. 25, 932–936. doi: 10.1002/mds.23070
Xu, Y., Wang, Q., Cui, R., Lu, K., Liu, Y., and Zhao, Y. (2017). Uric acid is associated with vascular dementia in Chinese population. Brain Behav. 7:e00617. doi: 10.1002/brb3.617
Zhou, Z., Liang, Y., Zhang, X., Xu, J., Lin, J., Zhang, R., et al. (2020). Fibrinogen and risk of dementia: a systematic review and meta-analysis. Neurosci. Biobehav. Rev. 112, 353–360. doi: 10.1016/j.neubiorev.2020.02.022
Zintzaras, E., and Ioannidis, J.P.A. (2005). HEGESMA: genome search meta-analysis and heterogeneity testing. Bioinformatics 21, 3672–3673. doi: 10.1093/bioinformatics/bti536
Keywords: uric acid, dementia, Alzheimer's disease, risk factor, meta-analysis
Citation: Zhou Z, Zhong S, Liang Y, Zhang X, Zhang R, Kang K, Qu H, Xu Y, Zhao C and Zhao M (2021) Serum Uric Acid and the Risk of Dementia: A Systematic Review and Meta-Analysis. Front. Aging Neurosci. 13:625690. doi: 10.3389/fnagi.2021.625690
Received: 03 November 2020; Accepted: 29 January 2021;
Published: 25 February 2021.
Edited by:
Alessandro Martorana, University of Rome Tor Vergata, ItalyReviewed by:
Tommaso Schirinzi, University of Rome Tor Vergata, ItalyDiana Jalal, The University of Iowa, United States
Copyright © 2021 Zhou, Zhong, Liang, Zhang, Zhang, Kang, Qu, Xu, Zhao and Zhao. This is an open-access article distributed under the terms of the Creative Commons Attribution License (CC BY). The use, distribution or reproduction in other forums is permitted, provided the original author(s) and the copyright owner(s) are credited and that the original publication in this journal is cited, in accordance with accepted academic practice. No use, distribution or reproduction is permitted which does not comply with these terms.
*Correspondence: Mei Zhao, emhhb20xQHNqLWhvc3BpdGFsLm9yZw==; Chuansheng Zhao, Y3N6aGFvQGNtdS5lZHUuY24=