Female Rats Are Resistant to Cognitive, Motor and Dopaminergic Deficits in the Reserpine-Induced Progressive Model of Parkinson’s Disease
- 1Behavioral Neuroscience Laboratory, Department of Pharmacology, Universidade Federal de São Paulo, São Paulo, Brazil
- 2Memory and Cognition Studies Laboratory, Department of Psychology, Universidade Federal da Paraíba, João Pessoa, Brazil
- 3Behavioral and Evolutionary Neurobiology Laboratory, Department of Biosciences, Universidade Federal de Sergipe, Itabaiana, Brazil
Parkinson’s disease (PD) is the second most common neurodegenerative disease. The main symptoms are motor signs such as resting tremor and difficulty in initializing movements. Non-motor alterations, such as cognitive deficits, can precede the motor symptoms. PD is more frequent in men than women. The mechanisms related to this difference are not completely understood. There is evidence that females present distinct characteristics in dopaminergic function compared to males. While the severity of motor impairments is often compared between sexes, little is known about sex differences in the prodromal stage. Most animal models of PD present acute severe motor impairment, which precludes the study of non-motor symptoms. Our research group have proposed an adaptation of the classic reserpine protocol, using low doses in a chronic treatment. This method allows the observation of progressive motor impairment as well as premotor deficits. Here we investigate possible behavioral and neuronal sex differences in the effects of the repeated treatment with a low dose of reserpine in rats. Male and female Wistar rats received 10–15 injections of reserpine (0.1 mg/kg) or vehicle, on alternate days. We followed-up the estrous cycle phases and conducted motor and cognitive assessments (catalepsy, open field, oral movements and object recognition tests). The euthanasia occurred 48 h after the 10th or 15th injections, with the collection of blood for the quantification of sex hormones and brains for tyrosine hydroxylase (TH) immunohistochemistry in the substantia nigra pars compact (SNpc). Reserpine induced progressive catalepsy, involuntary oral movements and cognitive deficits in male rats. The behavioral effects of reserpine were attenuated (motor) or absent (cognitive) in females. Reserpine decreased TH immunoreactivity in males, but not in females. Estrogen levels in females negatively correlated with catalepsy duration. Our findings show that females present a delay and/or a prevention in the reserpine-induced motor alterations in the progressive PD model, compatible with the lower prevalence of this disease in women. Further, females were protected from the deficit in object recognition at the prodromal stage. The absence of reserpine-induce decrease in TH immunoreactivity suggests that differences in dopaminergic function/plasticity are related to this protection in female sex.
Introduction
Parkinson’s disease (PD) is the most common motor disorder and the second most common age-related neurodegenerative disease (Tysnes and Storstein, 2017). The disease is mainly characterized by motor features such as resting tremor, bradykinesia, rigidity, and postural instability (Moustafa et al., 2016), which are associated with loss of dopaminergic nigrostriatal neurons (Alexander, 2004).
PD also includes non-motor symptoms, such as autonomic dysfunctions, cognitive abnormalities, psychiatric symptoms (anxiety, depression), and sleep disorders, some of which may precede clinical diagnoses (Brown and Marsden, 1990; Chaudhuri and Schapira, 2009). Cognitive impairments are frequently reported in PD patients, particularly working and spatial memory deterioration (Gillies et al., 2004), attentional deficits (Benedetti et al., 2001), and recognition memory impairment (Wirdefeldt et al., 2011). Moreover, the cognitive impairments may be among the earliest symptoms in PD patients (Chaudhuri and Naidu, 2008).
The incidence of PD is 1.5–2.0 times higher in men than in women (Van Den Eeden et al., 2003; de Lau and Breteler, 2006; Wirdefeldt et al., 2011; Tysnes and Storstein, 2017). Furthermore, there is a delay in the symptoms onset in females, which is possibly related to a neuroprotective effect of estrogen on the nigrostriatal dopaminergic system (Gillies et al., 2004) or to sex differences in the physiology of dopaminergic neurotransmission (Haaxma et al., 2007). Clinical studies have demonstrated that the lowest severity of motor symptoms of PD in women was correlated with a higher period of estrogen exposure along lifetime. In addition, hormonal decrease or deprivation increases the risk of developing PD (Benedetti et al., 2001; Haaxma et al., 2007).
On the other hand, sex differences in prevalence and prognosis of non-motor symptoms in PD remain controversial. Non-motor symptoms can often reduce quality of life even more significantly than motor alterations (Todorova et al., 2014). Previous studies reported that women show higher predisposition to develop fatigue, constipation, pain, and depression, whereas male PD patients present more serious cognitive deficits (Scott et al., 2000; Solimeo, 2008; Martinez-Martin et al., 2012).
The mechanisms underlying sexual differences in PD, particularly regarding non-motor symptoms, have received little attention. In this regard, some authors pointed out that estrogen is neuroprotective. The protective effect of estradiol might be mediated by a suppressive effect on the dopamine transporter (DAT) function (Watson et al., 2006; McArthur et al., 2007). However, whether or not estradiol protects the dopaminergic pathways in experimental PD appears to vary according some experimental conditions (e.g., neurotoxin used, the severity of the lesion, the strain and species of rodent and the treatment regimen) as well as sex (McArthur et al., 2007). For example, in a previous study Bispo et al. (2019) have shown that ovariectomized females have decreased catalepsy compared to intact male and female rats, although showing a loss in TH + cells in SNpc.
The repeated low-dose reserpine protocol has been used as a model of progressive parkinsonism, showing phenomenological (progressive motor alterations) and construct (reduced dopaminergic parameters, increased oxidative stress, and augmented alpha-synuclein expression) similarities with PD (Fernandes et al., 2012; Santos et al., 2013; Leão et al., 2015, 2017, 2021; Ikram and Haleem, 2019). Unlike neurotoxin models, which lead to acute severe motor impairment, the low-dose repeated reserpine protocol allows the observation of progressive motor impairments as well as premotor deficits such as cognitive prejudice, anxiety and nociception alterations (Santos et al., 2013; Leal et al., 2019; Cintra et al., 2021; Dos Santos et al., 2021). This is of relevance when studying PD sex differences because women seem to have a later onset of the motor symptoms, while non-motor prodromic features are poorly understood. Thus, the present study aimed to evaluate sex differences on motor, cognitive, and neurochemical alterations in rats submitted to the low-dose reserpine-induced progressive model of PD.
Materials and Methods
Animals
Female (n = 40) and male (n = 20) Wistar rats (7–8 months old) were used in this study. All animals were housed in groups of 4 per cage (30 cm × 42 cm × 16 cm) under controlled airflow, acoustic isolation, and temperature at 22 ± 1°C with a 12 h light/12 h dark cycle (light on at 7:30 a.m.). All animals had free access to water and food. Animals used in this study were handled according to the Brazilian law for the use of animals in research (Law Number 11,794) and all procedures were approved by the local ethics committee (protocol number 3683250517). All efforts were made to minimize animal pain, suffering or discomfort and to reduce the number of animals used.
Drug Treatment and General Procedures
Reserpine (Res, Sigma Chemical Co., United States) was dissolved in glacial acetic acid (1%) and then diluted to the correct concentration with distilled water. The vehicle solution (Veh) consisted of the same amount of acetic acid and water as in the reserpine solution. Rats were randomly assigned to one of four groups: Female-Veh (n = 20), Male-Veh (n = 10), Female-Res (n = 20), and Male-Res (n = 10). Animals received subcutaneous injections of vehicle (Veh) or 0.1 mg/kg of reserpine (Res) at a volume of 1 mL/kg body weight, every 48 h for 20 or 30 days of treatment, completing 10 or 15 injections, respectively. Animals were euthanized 48 h after the 10th or 15th injections (Figure 1).
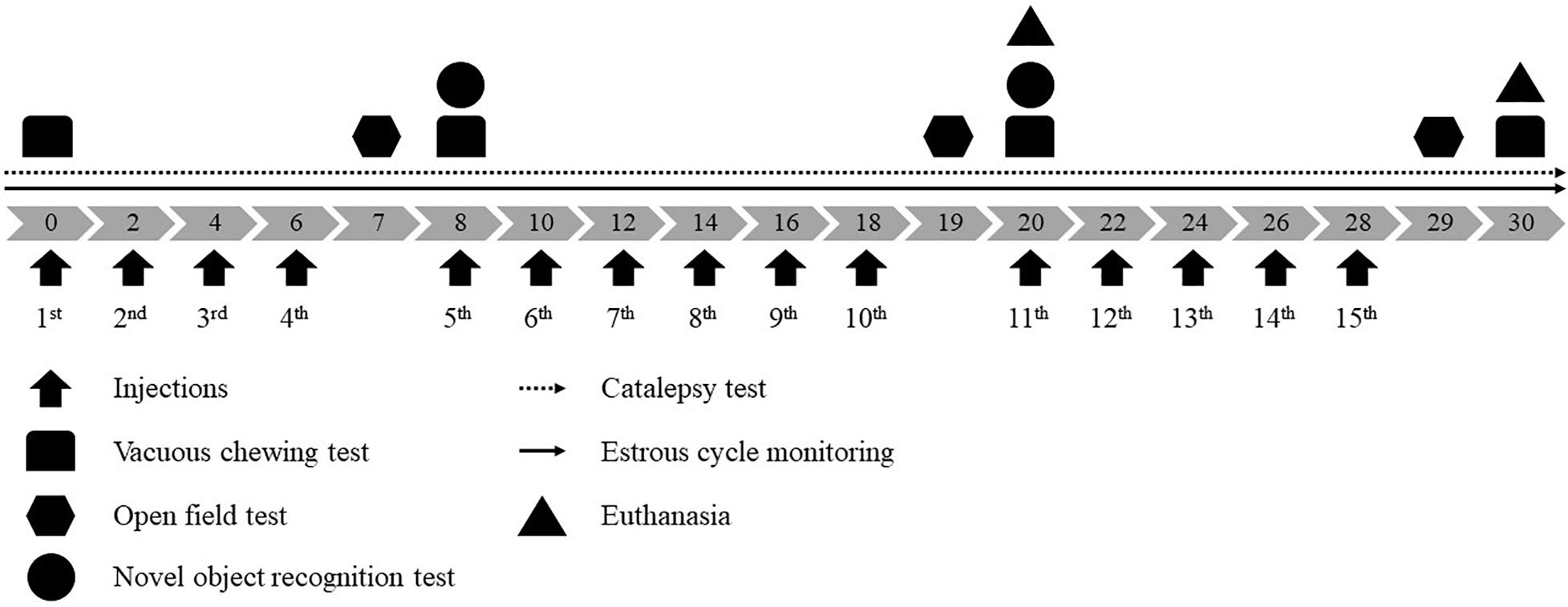
Figure 1. Experimental design. Gray arrows indicate days of treatment and black arrows pointed up indicate vehicle or 0.1 mg/kg reserpine s.c. injections.
Across treatment, animals were submitted to the following procedures: (1) estrous cycle monitoring (daily); (2) catalepsy test (before the first injection and daily across treatment); (3) assessment of oral movements before the first injection and 48 h after the 4th, 10th, and 15th injections; (4) evaluation of open field behavior 24 h after the 4th, 10th, and 15th injection; (5) novel object recognition test after the 4th and 10th. If the procedure was held in a treatment day, it was conducted before the injection (from 12:00 a.m. to 14:00 p.m.). Experimental design is shown in Figure 1.
In order to evaluate the estrous cycle, vaginal smears were collected by vaginal lavage using saline at room temperature. The vaginal smears collected were placed immediately on the glass slide, colored with methylene blue and analyzed under the optical microscope in 10× magnification, following the methods described by Marcondes et al. (2002).
Behavioral Tests
Catalepsy Test
The catalepsy behavior was assessed by placing the animal’s forepaws on a horizontal bar positioned 9 cm above the bench surface while the hind paws rested on the bench. The animals were free to move after they were placed in this position by the experimenter. Catalepsy was defined as an immobile posture (keeping both forepaws on the bar, see Supplementary Video 1). The latency to withdraw one or both forepaws from the bar was measured up to a maximum of 180 s (Sanberg et al., 1988). Three trials for animal in each observation day were carried out and the results were analyzed considering the mean value of these trials. In addition to the daily analysis of catalepsy curve, analyzes were performed in phases, considering means of 5 observations: basal (without injection), prodromal phase (1st∼5th), early motor phase (6th∼10th) and late motor phase (11th∼15th).
Vacuous Chewing Test
The animals were individually placed in a wire cage (20 × 20 × 15 cm). Mirrors were positioned under and behind it to allow behavioral quantification when animal faced away from the observer. The number of vacuous chewing movements (mouth openings in the vertical plane not directed toward physical material, see Supplementary Video 2) was measured continuously for 10 min.
Open Field Test
The animals were submitted to the open field in order to evaluate spontaneous locomotor activity. The apparatus was a circular arena (67 cm in diameter) surrounded by a 40 cm high wall, made of wood and painted black. Animals were placed in the center of the apparatus for free exploration for 5 min. Distance traveled in the whole arena (in meters) was evaluated. The sessions were recorded by a digital camera and the behavioral parameters were registered by Any-maze® (Stoelting. Co., IL, United States).
Novel Object Recognition Test
The novel object recognition (NOR) task was carried out in the same arena used in the open field test. In each day of test two pairs of objects were used randomly among animals. The objects used were all made of plastic material and filled with cement to ensure that animals could not displace them. The objects differed in height, width, color and shape. A previous experiment demonstrated that rats do not show spontaneous preference for any of these objects. In the training session, the animals were exposed to two copies of an object for 5 min. The same procedure was carried out 1 h later (test session), except that one of the objects was replaced for a new one. Different sets of objects were used on the 20th day (10th injection) to avoid a possible recognition of objects used on the 8th day (4th injections). The apparatus and objects were cleaned with a 5% alcohol solution after each behavioral session. The sessions were recorded by a digital camera and the behavioral parameters were registered by Any-maze®. The time spent exploring each object was measured in both sessions. Exploration behavior was defined by touching with forepaws or nose, sniffing and biting the objects. Only animals that showed total object exploration greater than 3 s during the training were included in the analysis. The discrimination index was calculated as exploration difference (old – new object)/total objects exploration (old + new object).
Tissue Processing and Tyrosine Hydroxylase Immunohistochemistry
Upon completion of behavioral procedures, animals were pre-anesthetized with fentanil (0.5 mg/kg, i.p.) and acepran (1.0 mg/kg, i.m.). After 5 min they were deeply anesthetized with an intraperitoneal injection of 9.0 mg/kg ketamine chloridate and xylazine and transcardially perfused with 200–250 mL phosphate-buffered saline (PBS), pH 7.4, containing 0.2% heparin, followed by 200 mL PBS with 4% paraformaldehyde 0.1 M. The brains were removed from the skull, post fixed in the same fixative solution and stored at 4°C. After 24 h, we transferred brains to a solution containing sucrose 30% 0.1 M PBS, at 4°C. Each brain was fixed in Tissue-Tek® (Sakura, Japan) at −20°C. Then, we serially sliced the brains in the coronal plane into 50 μm thick sections with a cryostat microtome (Leica, Germany) at a temperature of −20°C. Following tissue processing, we performed immunohistochemistry for TH, using a free-floating protocol. Sections were washed four times with PBS (pH 7.4) for 5 min each and consecutively washed with 0.03% H2O2 solution for 20 min to reduce endogenous peroxidase activity. For detection of TH, sections were incubated with rabbit anti-tyrosine hydroxylase polyclonal antibody (cat # AB152 Chemicon, United States, 1:5,000) diluted in triton x-100 0.4% and PBS with 2% albumin serum, for 18–24 h at room temperature. Afterward, sections were incubated with goat biotinylated anti-rabbit IgG (Vector Labs, United States, 1:1,000) diluted with triton x-100 0.4% NaCl and PBS for 2 h at room temperature, followed by washing steps, and incubated with avidin-biotin-peroxidase solution (ABC Elite kit, Vector Labs, Burlingame, United States) for another 2 h. The reaction was developed by adding of 3,3-diaminobenzidine (DAB—Sigma-Aldrich, United States) and 0.01% H2O2 0.1 M phosphate buffer solution for 3 min. Then, we left sections to dry, dehydrated in a graded alcohol series, cleared in xylene, and coverslipped with Entellan (Merck). All sections were immunostained concomitantly, to minimize possible background differences between samples. Sections were examined under brightfield illumination with an optical microscope (Nikon Eclipse 80i), attached with a motorized stage (MBF Bioscience) and CCD camera (Nikon, DXM-1200) to record images. In order to estimate the number of TH + cells in SNpc, we analyzed 8–12 sections of each animal, equally distributed at the rostral, medium and caudal levels of the structure. All TH + cells of SNpc on each section were registered. The mean of all measures was calculated, and the data were normalized by the respective control.
Estrogen Dosage
During the transcardiac perfusion, the blood was collected with a coagulation activator and separator gel. Posteriorly, it was centrifuged at 5,000 rpm for 10 min at 4°C, the pliers were stored at −80°C until the assays. The determination of estradiol concentrations in plasma was performing using electroimmunoluminescence method (Tiskievicz et al., 2011) in the Hermes Perdini Analysis Laboratory.
Data Analysis
Data normality and homogeneity of variances were tested by the Shapiro-Wilk and Levene’s tests, respectively. Behavioral data was grouped in two sets of analysis: animals that received 10 and 15 injections. The average of 2 days of catalepsy behavior, catalepsy phases, oral movements and locomotion in the open field were analyzed by two-way repeated-measure ANOVA followed by Sidak’s post hoc test. Discrimination indexes in NOR and percentage of occurrence of estrous cycle phases were analyzed by two-way ANOVA followed by Sidak’s post hoc test. Non-parametric data from TH + quantification and the hormonal levels were analyzed by the Mann-Whitney U-test in each group separately. In these cases, Bonferroni correction was carried out and alpha value was set at 0.025. Spearman’s rank correlation coefficient was used to investigate a possible association between hormonal levels and motor performance in the catalepsy test. Correlations in vehicle and reserpine groups were investigated separately, considering the different treatment durations. Results were expressed as mean ± SEM and p < 0.05 was considered to reflect significant differences.
Results
Catalepsy Test
A two-way repeated-measure ANOVA for all animals until the 10th injection of Veh or Res revealed significant effects of time [F(10, 560) = 61.014; p < 0.001], treatment (Veh and Res) [F(1, 56) = 16.740; p < 0.001], and interactions between time and treatment [F(10, 560) = 16.962; p < 0.001], time and sex (Male and Female) [F(10, 560) = 3.278; p = 0.021] and time, treatment and sex [F(10, 560) = 3.307; p = 0.021]. Another two-way repeated-measure ANOVA (only with animals that received 15 injections of Veh or Res) revealed significant effects of time [F(15, 390) = 15.918; p < 0.001], treatment [F(1, 26) = 22.356; p < 0.001], sex [F(1, 26) = 4.446; p = 0.045] and interactions between time and treatment [F(15, 390) = 10.951; p < 0.001] and sex and treatment [F(1, 26) = 4.605; p = 0.041]. The Sidak’s post hoc test revealed significant increases in the duration of catalepsy behavior in Male-Res group compared to Male-Veh from the 6th to the 15th measures. Female-Res group exhibited significant increases in the duration of catalepsy compared to Female-Veh in the 6th, 8th, 9th, 10th, and 15th measures. In addition, male-Res showed significant increases in the time on the bar compared to Female-Res on the 8th, 9th, 10th, 12th, and 13th measures (Figure 2A).
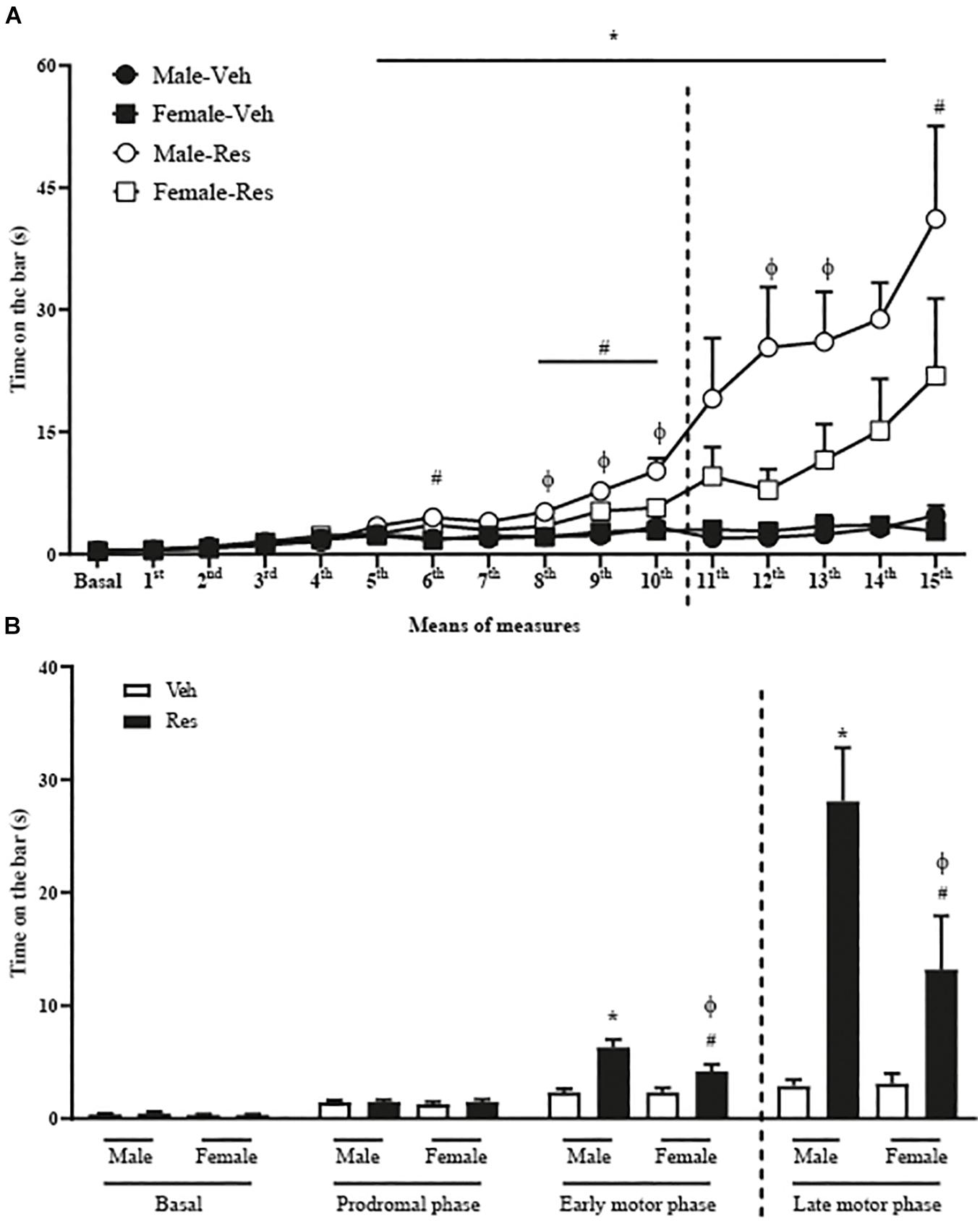
Figure 2. Effects of repeated treatment with 0.1 mg/kg reserpine (Res) or vehicle (Veh) on catalepsy behavior in female and male rats throughout the treatment (A) and grouped in phases (B) basal (without injection of Res or Veh), prodromal (1∼5 injections), early motor (6∼10 injections), and late motor (10∼15 injections). Dashed lines indicate the euthanasia of half the animals. *p < 0.05 comparing Male-Res and Male-Veh, #p < 0.05 comparing Female-Res and Female-Veh and ϕp < 0.05 comparing Male-Res and Female-Res (Two-way repeated-measure ANOVA followed by Sidak’s post hoc test). Values were expressed by mean ± SEM. Individual data are displayed in Supplementary Tables 1, 2).
We also analyzed the catalepsy progression in means of 5 injections in basal (without injections of Veh or Res), prodromal (1st∼5th injections), early motor (6th∼10th injections) and late motor (11th∼15th injections) phases. Two-way repeated-measure ANOVA (considering all animals until the 10th injection of Veh or Res) revealed significant effects of phases (basal, prodromal and early motor phase) [F(2, 112) = 122.805; p < 0.001], treatment (Veh or Res) [F(1, 56) = 17.028; p < 0.001], and interactions between time and treatment [F(2, 112) = 27.013; p < 0.001] and time, treatment and sex [F(2, 112) = 4.139; p = 0.039].
The analysis of the phases of catalepsy by two-way repeated-measure ANOVA (considering only animals that received 15 injections) showed significant effects of phases [F(3, 78) = 31.261; p < 0.001], treatment [F(1, 26) = 22.362; p < 0.001], sex [F(1, 26) = 4.556; p = 0.042], and interactions between phases and treatment [F(3, 78) = 20.288; p < 0.001] and sex and treatment [F(1, 26) = 4.635; p = 0.041]. The Sidak’s post hoc test revealed that Male-Res show increased time in catalepsy duration compared to Male-Veh and Female-Res on early and late motor phases (Figure 2B).
Vacuous Chewing Movements
A two-way repeated-measure ANOVA (considering all animals until the10th injection of Veh or Res) revealed significant effects of the interactions between time (basal, 4th and 10th injections) and treatment (Veh or Res) [F(2, 66) = 3.807; p = 0.027] and treatment and sex [F(1, 33) = 5.107; p = 0.031].
Another two-way repeated-measure ANOVA (considering only animals that received 15 injections) revealed a significant effect of time [F(3, 42) = 3.767; p = 0.018] and interaction between time and sex [F(3, 42) = 4.274; p < 0.010].
The Sidak’s post hoc test exhibited a significant effect of Res in Male animals in the 3rd and 4th measures (10th and 15th administrations, respectively). There was also a sex difference from the 2nd until the last measure (4th, 10th, and 15th injections). Reserpine-treated females did not show an increase in the vacuous chewing (Figure 3).
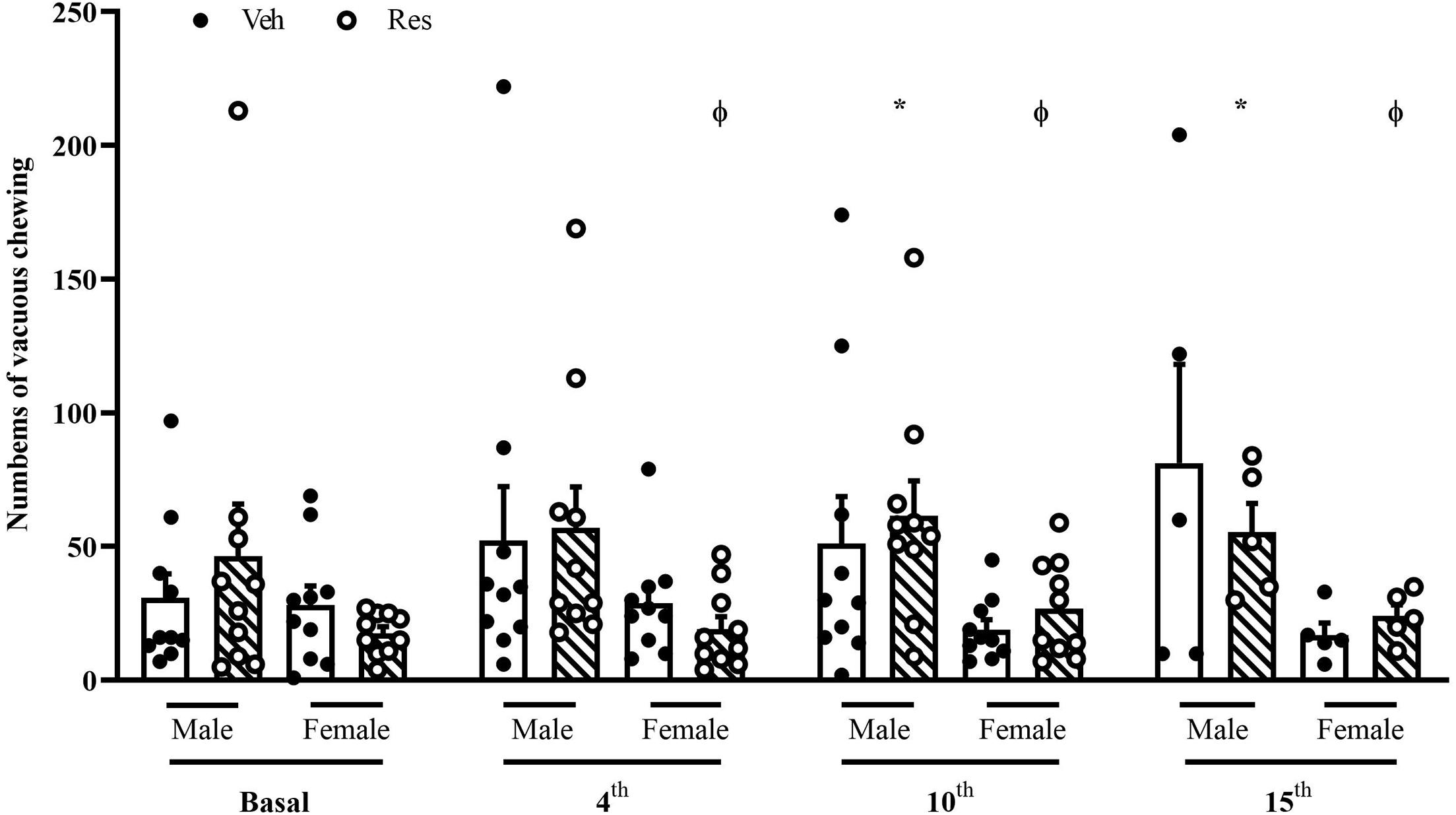
Figure 3. Effects of repeated treatment with 0.1 mg/kg reserpine (Res) or vehicle (Veh) on the vacuous chewing (VCM) behavior in female and male rats. *p < 0.05 comparing Res and Veh and ϕp < 0.05 comparing Male-Res and Female-Res (Two-way repeated-measure ANOVA followed by Sidak’s post hoc test). Values were expressed by mean ± SEM. Black and circles represent individual data of Veh and Res animals, respectively.
Open Field Test
A two-way repeated-measure ANOVA (considering all animals that went through OF 48 h after the 4th and 10th injections of Veh or Res) revealed significant effects of time [F(1, 36) = 47.821; p < 0.001], treatment [F(1, 36) = 6.378; p = 0.016], sex [F(1, 36) = 17.190; p < 0.001] and interaction between time and treatment [F(1, 36) = 9.571; p = 0.004].
Another two-way repeated-measure ANOVA (considering only animals that went through OF 48 h after the 4th, 10th, and 15th injections) exhibited significant effects of time [F(2, 32) = 64.913; p < 0.001], treatment [F(1, 16) = 22.256; p < 0.001], sex [F(1, 16) = 9.475; p = 0.007], and interaction between time and treatment [F(2, 32) = 34.727; p < 0.001] and time, treatment and sex [F(2, 32) = 8.018; P = 0.002].
The Sidak’s post hoc test revealed a significant increase of locomotion of Female-Veh in the three measures. In addition, 48 h after the 10th and 15th injections, the Sidak post hoc test showed Res decreased locomotion in both sexes (Figure 4).
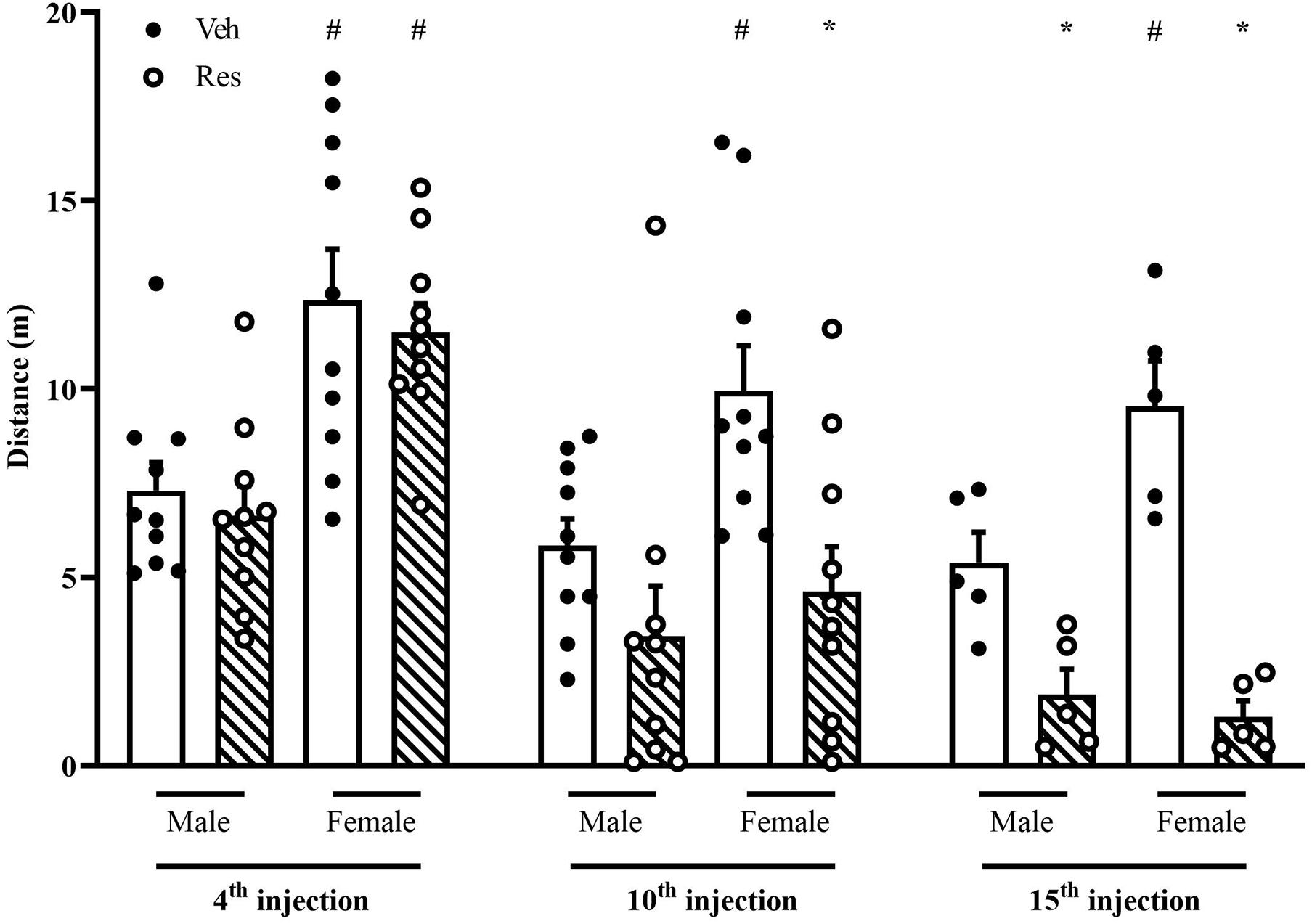
Figure 4. Effects of repeated treatment with 0.1 mg/kg reserpine (Res) or vehicle (Veh) on locomotion in the open field in female and male rats. *p < 0.05 comparing RES and Veh. #p < 0.05 comparing males and females with the same treatment (Two-way repeated-measure ANOVA followed by Sidak’s post hoc test). Values were expressed by mean ± SEM. Black and circles represent individual data of Veh and Res animals, respectively.
Novel Object Recognition
The NOR analysis included only animals that obtained a total exploration time greater than 3 s in the training phase. A two-way ANOVA for the discrimination index considering the first and second measures (48 h after the 4th and 10th injections of Veh or Res) exhibited a significant effect of the interaction between treatment and sex in the first (after the 4th injection of Veh or Res) [F(1, 34) = 4.302; p = 0.046] and second (after the 10th injection of Veh or Res) [F(1, 30) = 6.947; p = 0.013] measures. The Sidak’s post hoc test revealed significant effects of Res in Male in both tests. On the other hand, females did not show the same effect, and differed from males treated with Res (Figure 5).
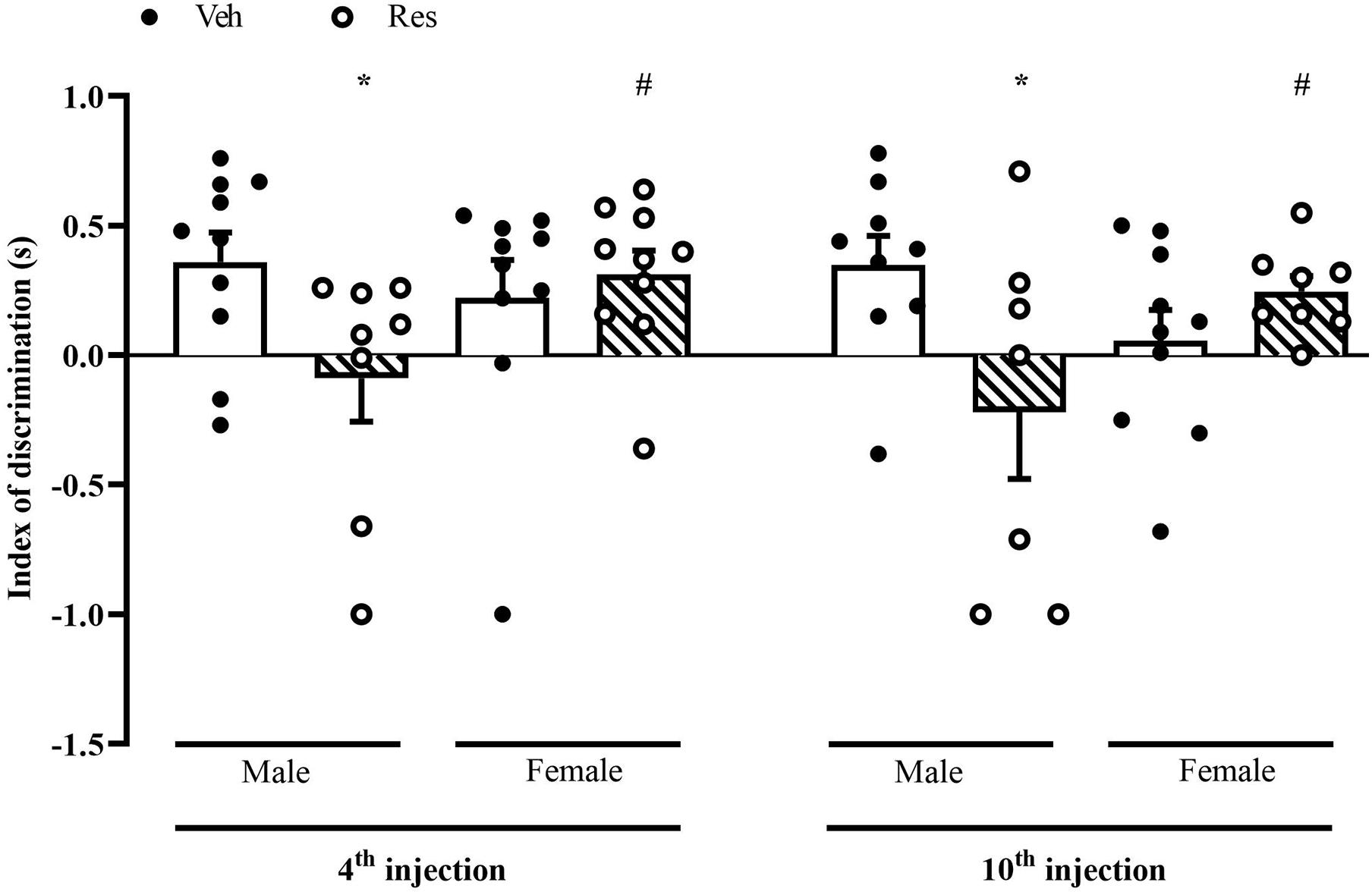
Figure 5. Effects of repeated treatment with of 0.1 mg/kg reserpine (Res) or vehicle (Veh) on object recognition memory in female and male rats. *p < 0.05 comparing Res and Veh #p < 0.05 comparing Male-Res and Female-Res (Two-way ANOVA followed by Sidak’s post hoc test). Values were expressed by mean ± SEM. Black and circles represent individual data of Veh and Res animals, respectively.
Tyrosine Hydroxylase Immunohistochemistry
Mann-Whitney U-test did not show differences in TH + levels between vehicle and reserpine for females and males after ten injections of Res (U = 13.000; p = 0.917, and U = 9.000; p = 0.905, respectively). On the other hand, fifteen injections of reserpine reduced TH expression of Male-Res when compared to Male-Veh (U = 0.000; p = 0.008). Female-Res did not show differences in TH expression compared with Female-Veh (U = 5.000; p = 0.286) (Figure 6).
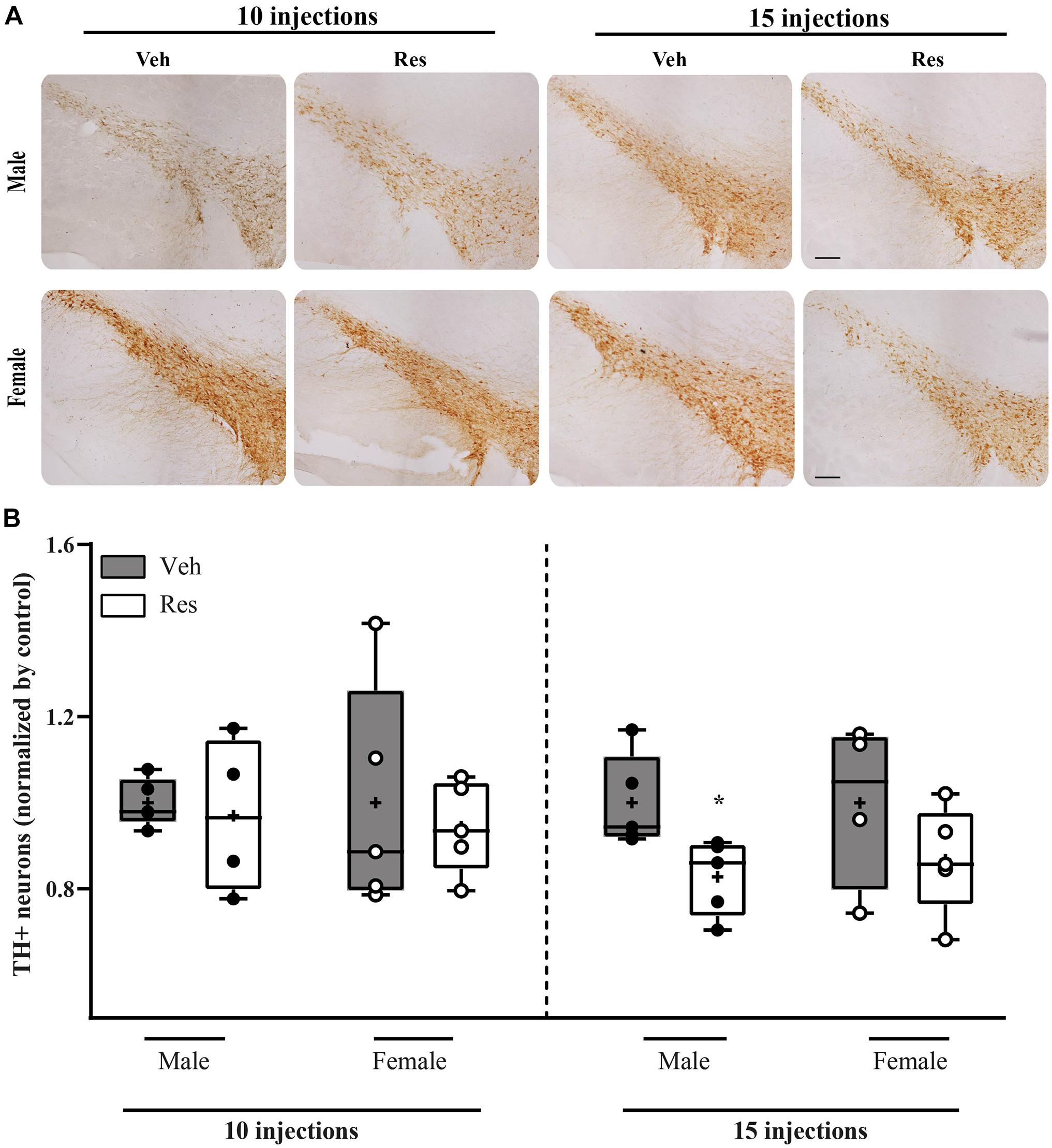
Figure 6. Effects of repeated treatment with 0.1 mg/kg reserpine (Res) or vehicle (Veh) on the number of TH immunoreactive cells in substantia nigra pars compacta in female and male rats after 10 or 15 injections. (A) Representative photomicrographs of brain coronal sections of substantia nigra pars compacta. Scale bar: 100 μm. (B) Quantification of TH immunoreactive cells. Horizontal lines in the middle of each box indicates the median TH levels, while the top and bottom borders of the box mark the 25th and 75th percentiles, respectively. Black and circles represent individual data of Veh and Res animals, respectively. *p < 0.05 comparing Res and Veh (Mann-Whitney U-test). Data of individual brain sections are displayed in Supplementary Table 3.
Evaluation of Estrous Cycle and Estradiol Levels
In order to verify the influence of the estrous cycle throughout the treatment, we monitored the estrous cycle phases throughout the protocol. The prevalence of each cycle phase in each day of the protocol was determined for each female group. A two-way ANOVA applied to the percentage of occurrence of the cycle stage between treatments showed only a significant effect of cycle phases [F(3, 240) = 42.194; p < 0.001], probably because the duration of each phase is physiologically different. There was a lower overall occurrence of the metaoestrous phase (Table 1 and Figure 7). There were no differences regarding treatment.
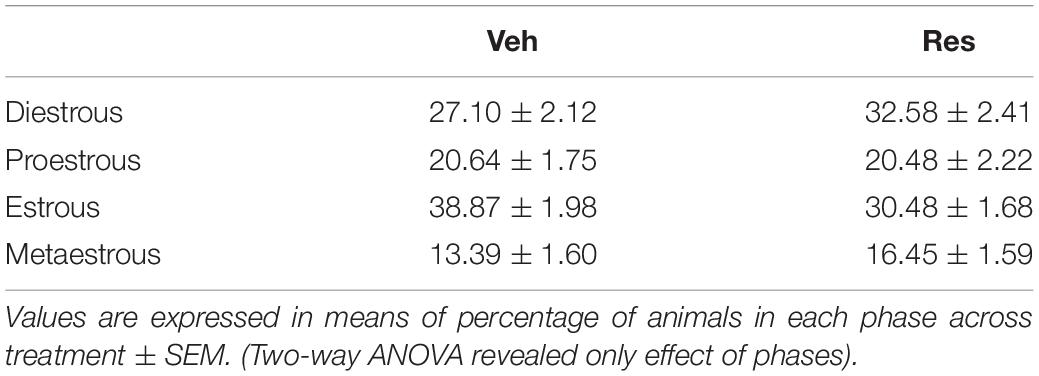
Table 1. Percentage of occurrence of each phase of the estrous cycle during treatment with 0.1 mg/kg reserpine (Res) or vehicle (Veh).
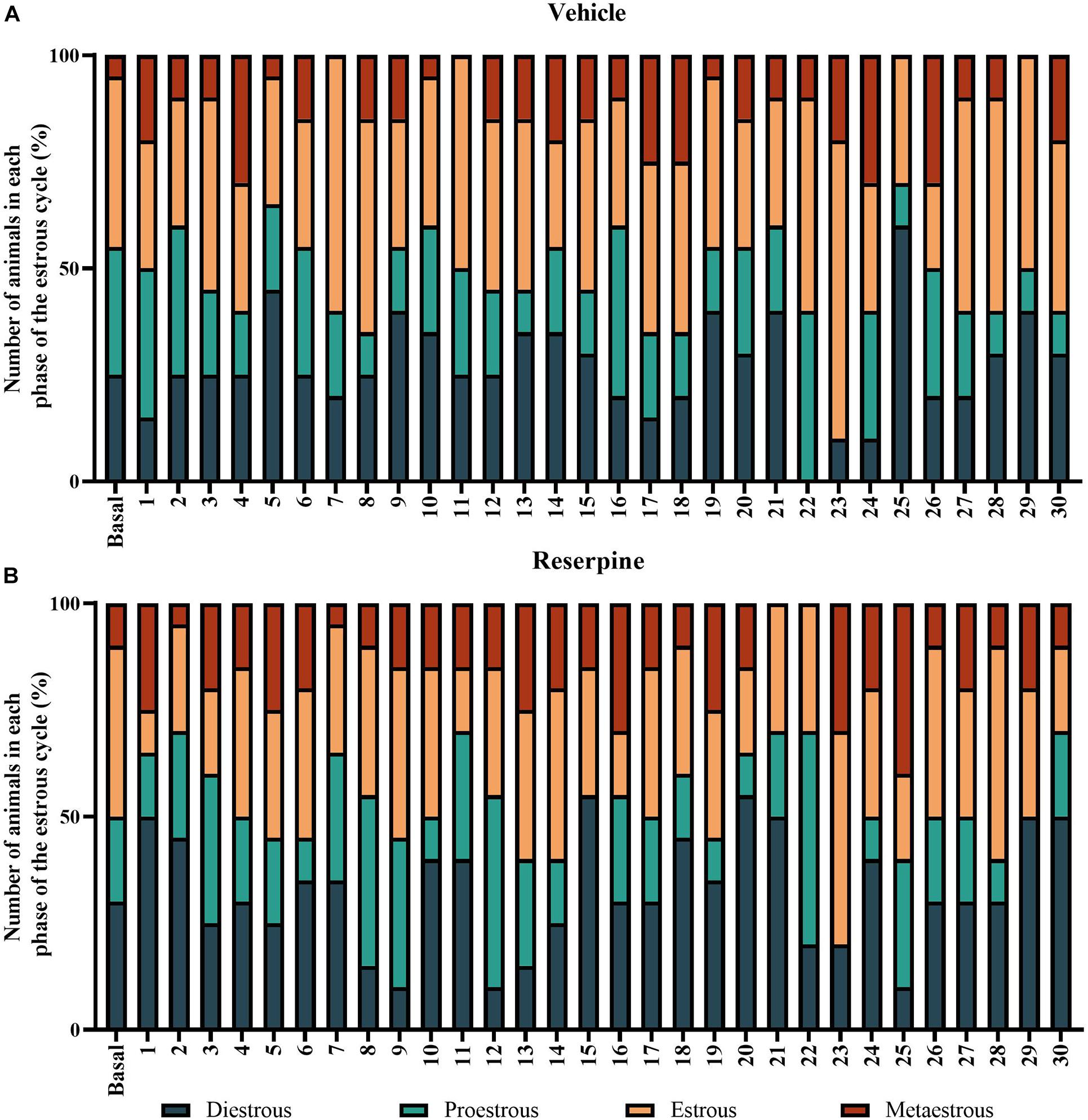
Figure 7. Prevalence of the phases of estrous cycle throughout the 30-day repeated treatment with vehicle (A) or 0.1 mg/kg reserpine (B) in female rats. Values were expressed by percentage of animals in each phase in each day of the protocol.
A non-parametric analysis (Mann-Whitney test) did not show differences in estrogen levels in vehicle and reserpine females after 10 or 15 injections (U = 12,000; p = 0.0916, and U = 16.000; p = 0.296, respectively, Table 2). A Spearman’s rank-order correlation was run to investigate a possible association between estradiol levels and motor performance (last catalepsy measure) for each group at both treatment lengths. There was a strong negative correlation between estradiol and catalepsy immobility for reserpine-treated group at the 15th injection [rs(6) = −0.928; p = 0.008], but not at the 10th injection [rs(5) = −0.500; p = 0.391] or vehicle-treated animals at the 10th [rs(5) = −0.564; p = 0.322] and 15th [rs(8) = 0.204; p = 0.629) injections (Figure 8).
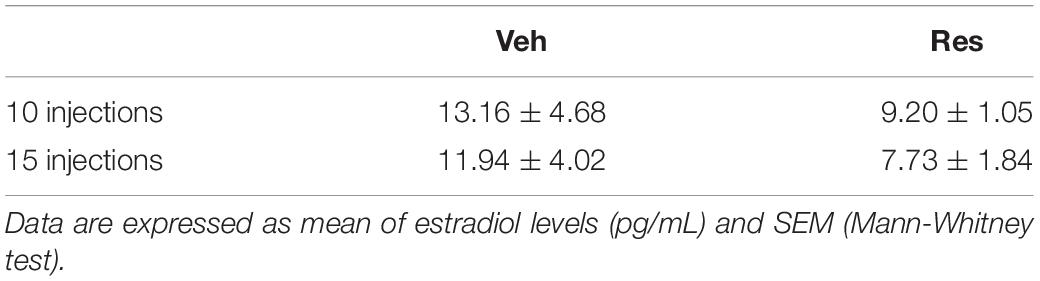
Table 2. Serum estradiol levels after 10 or 15 injections of repeated treatment with 0.1 mg/kg reserpine (Res) or vehicle (Veh) in female Wistar rats.
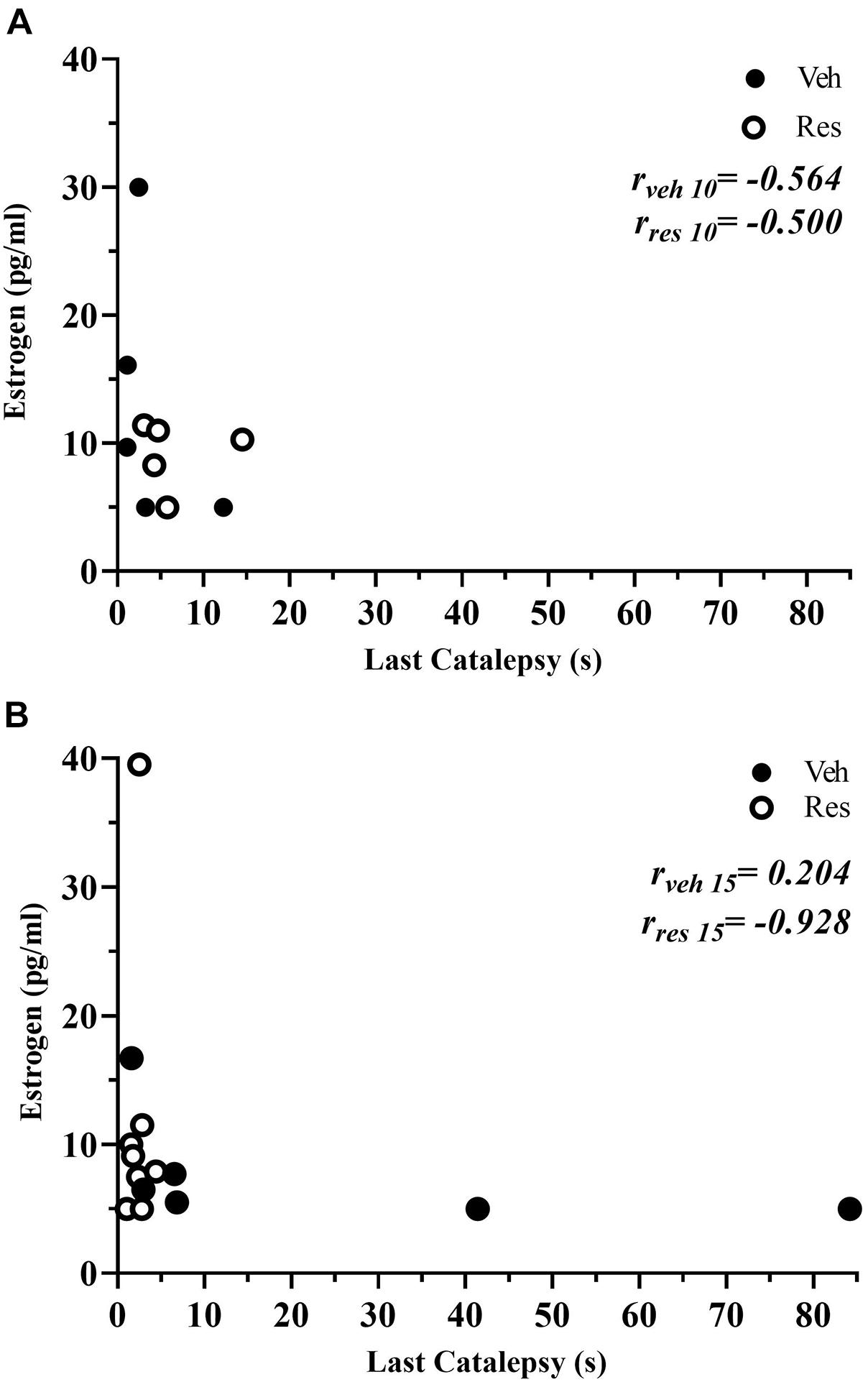
Figure 8. Scatterplots depicting the correlations between the serum estradiol levels and duration of catalepsy of vehicle and reserpine groups that received 10 (A) and 15 injections (B).
Discussion
In this work, we investigated sex differences in motor, cognitive and neurochemical alterations at different stages of repeated low-dose reserpine administration, a progressive animal model of PD (Santos et al., 2013; Leão et al., 2015, 2017; Peres et al., 2016; Campelo et al., 2017; Lins et al., 2018; Bispo et al., 2019).
Corroborating previous studies, we observed that reserpine treatment induced a progressive motor impairment in male rats (Fernandes et al., 2012; Santos et al., 2013; Leão et al., 2017; Lins et al., 2018; Bispo et al., 2019). Interestingly, male rats showed more susceptibility to these motor alterations than female rats. Indeed, our results showed that males had a greater increase in catalepsy duration and vacuous chewing movements when compared to females. Conversely, the decrement in open field spontaneous activity was similar for both sexes. Further, we observed a sex difference in the short-term memory task: only RES-treated male rats showed a reduction in the discrimination index, which was observed prior to the motor deficits onset, in the prodromal phase, and remained throughout the protocol. Moreover, the low-dose repeated reserpine administration caused a decrease in TH immunoreactivity in SNpc only in males. Decreased estrogen levels correlated with increased immobility in the catalepsy test. Overall, we showed sex differences in the development of repeated reserpine-induced parkinsonism, highlighting alterations that occur in non-motor and motor phases, which are poorly understood in terms of sex differences in PD.
Studies have investigated sex differences in the 6-OHDA (Cass et al., 2005; Field et al., 2006; Zarate et al., 2021) and MPTP (Ookubo et al., 2009; Antzoulatos et al., 2010) animal models of PD. However, these protocols promote acute brain injury and rapid instauration of severe motor impairment, which hinders the evaluation of differences in motor deficits progressiveness and non-motor assessments (Saunders-Pullman et al., 1999; Tsang et al., 2000; Datla et al., 2003). Our results showed that the low-dose reserpine injections induced a progressive motor deficit in both male and female rats. Our findings revealed a greater motor impairment in male rats, corroborating previous findings in which catalepsy onset occurred 48 h after 6–8 reserpine injections (Fernandes et al., 2012; Santos et al., 2013; Campelo et al., 2017; Leão et al., 2017). On the other hand, Res-treated females showed a delayed onset of motor deficits. This outcome corroborates a previous study conducted with male and female mice (Leão et al., 2015).
Besides the lower susceptibility to increased catalepsy, female rats were not susceptible to the oral dyskinesia (evaluated by the vacuous chewing movements) induced by repeated treatment with a low dose of reserpine, which was present in males. Previous studies shown increased VCM response in males with the same protocol used here (Fernandes et al., 2012; Peres et al., 2016; Leão et al., 2017). However, one prior study reported increased reserpine-induced VCM response in female mice (Silva et al., 2002) after an acute injection of 1.0 mg/kg reserpine. In this regard, these studies proposed several hypotheses to explain the mechanisms underlying VCM, including dopamine hypofunction. Notably, reserpine promotes dopamine depletion by VMAT2 inhibition and, possibly modulation of dopamine receptor expression in several brain areas. In addition, reserpine increases dopamine autooxidation and oxidative catabolism by MAO, leading to oxidative stress (Abílio et al., 2003; Fuentes et al., 2007; Reckziegel et al., 2013). Accordingly, oral dyskinesia is also related to oxidative stress and is attenuated by antioxidant compounds (Abílio et al., 2003). In this way, it is possible that female rats would have a greater protection against oxidative stress, since they showed higher antioxidant enzyme activity than that observed in males (Siani et al., 2017; Tenkorang et al., 2018; Varmazyar et al., 2019).
Concerning spontaneous locomotion in the open field, our results did not show a sex difference for locomotor deficits in the Res-treated rats. Despite both female groups (Veh and Res), 48 h after the 4th injection, presented a higher locomotor activity when compared with respective male groups, as reported before (Cronan et al., 1985; Ribeiro et al., 2010), our findings showed that both sexes significantly reduced locomotion throughout the experiment, probably due to habituation. Nevertheless, Res-treated rats, irrespective of sex, presented a significant decrease in motor activity 48 h after the 10th and 15th injections. In this regard, other studies have described similar effects for males (Santos et al., 2013; Leão et al., 2017; Lins et al., 2018; Bispo et al., 2019) and females (Bispo et al., 2019). Although there is a visually more pronounced reduction in locomotion in reserpine females than in males in the second open field session, statistical analysis did not show a significant interaction between time (repeated sessions) and sex. In addition, a comparison between percent of locomotion reduction between groups revealed only an effect of treatment (data not shown). The absence of a protection against Res-induced decrement in spontaneous locomotion in females may occur because this behavior does not depend purely on motor skills. Instead, it encompasses factors such as motivation to explore, anxiety-like behavior and habituation after repeated exposures, among others (Walsh and Cummins, 1976; Choleris et al., 2001).
Regarding non-motor alterations, which often appear before motor symptoms, cognitive deficits are highly prevalent in PD (Roheger et al., 2018). The progressive parkinsonism induced by low dose-reserpine is a valuable tool that enables the evaluation of non-motor impairment, including emotional and cognitive deficits, during early stages of parkinsonism development in rodents (Santos et al., 2013; Campelo et al., 2017; Leal et al., 2019). In a prior study, Santos et al. (2013) reported impaired short-term object recognition memory before motor deficits in male rats (48 h after the 4th injection of reserpine). In a similar way, our results showed that Res-treated male presented memory deficits 48 h after the 4th and 10th injections. Importantly, Res-treated female rats did not show object recognition memory deficits. The present study evaluated only one memory test. Thus, whether this sex difference is applicable for cognitive function as a whole remains to be investigated. Beneficial effects of estrogen on memory could be related to neuronal survival, neurotransmission modulation, increased synaptogenesis, and protection against oxidative stress damage (Behl et al., 1995; Maggi et al., 2004; Shulman, 2007; Matheus et al., 2016; Hsieh et al., 2017). In PD, clinical studies have reported that men are more susceptible to cognitive deficits, while in women there is a lower prevalence of cognitive impairment (Cereda et al., 2016; Reekes et al., 2020).
In our study, we showed a reduction in TH immunoreactivity in the substantia nigra 48 h after the 15th reserpine injection in males. However, female rats presented a lower susceptibility to the deleterious effects of reserpine on DA neurons, which reinforces the evidence regarding the protective effects of estrogen. A possible explanation concerning estrogen effects in females includes the neuroprotective effects on DA neurons (Hyman et al., 1991) as well as the maintenance of neural activity upregulated by BDNF (Cosgrove et al., 2007). Interestingly, a previous report from our group showed that repeated low-dose reserpine administration promotes a reduction in BDNF in the hippocampus of male mice (Campelo et al., 2017). Accordingly, the reduction of BDNF in the blood of PD patients of both sexes was demonstrated Wang et al. (2016) and a meta-analysis reported a more prominent reduction of BDNF in men (Rahmani et al., 2019).
It should be noted that the reserpine-induced reduction in TH could be related to a feedback inhibition of TH expression in DA neurons. In this respect, besides TH decrement in the nigrostriatal pathway, reserpine also causes increase in oxidative stress parameters (Fernandes et al., 2012; Silva-Martins et al., 2021), increase in alpha-synuclein expression (Leão et al., 2017), alterations in axonal ultrastructure (Leal et al., 2019) and increase of neuroinflammation parameters (unpublished data). However, despite the several indicators that repeated reserpine induces some extent of neuronal damage compatible with Parkinson’s disease, it is important to mention that the TH decrement was partially recovered after prolonged withdrawal (in male rats—Santos et al., 2013). Thus, the repeated reserpine model comprises important validations as an animal model of progressive parkinsonism, but the extent of neuronal damage and the presence of neuronal loss are yet to be determined. Regardless, our study shows a protection against behavioral impairment and TH decrement in female subjects, which can be related to estrogen neuroprotective effects such as anti-inflammatory, antioxidant, neurotrophic actions, among others (see Dluzen, 2000 for a review). Alternatively, there are sex differences in the basal physiology of dopaminergic neurotransmission, possibly mediated by estrogen (Watson et al., 2006; Haaxma et al., 2007; McArthur et al., 2007) which can counteract the effect of reserpine on dopamine turnover. Specifically, estradiol may increase dopamine release in the striatum (Becker, 1990; Morissette et al., 2008) and increase the DA turnover by modulating the DA transporter (DAT, McArthur et al., 2007).
Our results are in accordance with the lower incidence of PD in women (Gillies et al., 2014) and reinforces the valuable contribution of this model in evaluating different characteristics associated with PD, especially regarding sex differences in clinical motor and non-motor symptoms of the prodromal phase.
The results related to the estrous cycle evaluation revealed a counter-balanced distribution of the female rats across phases throughout the experimental design (Figure 7). As mentioned, several studies have suggested that sex hormones, mainly estrogen, play an essential role in these sex differences in animal models of PD. In this regard, after the 15th injection of the treatment regimen, we observed that reserpine-treated females present the estradiol levels inversely associated with motor performance in the catalepsy task. In other words, female rats with the lowest estradiol levels showed the highest duration of catalepsy. In contrast, for the vehicle group, we did not observe an association between hormonal levels and motor performance along the experimental protocol. These data corroborate previous studies showing that estrogen treatment (Saunders-Pullman et al., 1999; Tsang et al., 2000; Rajsombath et al., 2019) and prolonged natural exposure to endogenous estrogens (Saunders-Pullman et al., 1999) reduced the symptoms and risk of developing PD. In addition, physiological levels of estrogen, as well as exogenous reposition of this hormone protect females against nigrostriatal injury (Yu and Liao, 2000; Datla et al., 2003; Dluzen and Horstink, 2003). In the MPTP model, ovariectomized females mice showed a higher striatal DA depletion compared with gonadal intact females (Dluzen et al., 1996). In female rats, the replacement of estradiol levels reversed this DA depletion in studies using the 6-OHDA model (Murray et al., 2003), although the physiological mechanisms are not yet elucidated.
As mentioned, there is evidence that estrogens act as a neuroprotective factor for dopaminergic neurons in several animal models of PD, such as induced by 6-OHDA (Tamas et al., 2005) and MPTP (Ookubo et al., 2009; Antzoulatos et al., 2010). However, it has been reported that the effects of these neurotoxins may depend upon the specific stage of the estrous cycle the females are in when injected the toxin (Yu and Liao, 2000). For example, an extended loss of DA neurons occurs in phases of low estrogen levels (Yu and Liao, 2000). In this sense, due to the progressive nature of the PD model used here, our results have shown a protection against reserpine-induced alterations during the early stage in females, when there are still regular estrous cycles.
In summary, we report a sexually dimorphic profile of parkinsonism progression induced by reserpine, consistent with the clinical literature and acute animal models of PD using neurotoxins. We also showed a correlation between low levels of estrogen and increased catalepsy, suggesting a protective role of this hormone. However, further studies are required to elucidate the specific role of sex hormones on the behavior and neurochemical mechanisms related to sexual dimorphism in the development of reserpine-induced parkinsonism.
Data Availability Statement
The raw data supporting the conclusions of this article will be made available by the authors, without undue reservation.
Ethics Statement
The animal study was reviewed and approved by the Comissão de Ética no Uso de Animais—UNIFESP.
Author Contributions
AL conducted the experiments and data analysis. AL, YM, and RS wrote the manuscript. VB, DC, NG, LL-S, MB, MS, and GM participated in experiments, data collection, and data analysis. YM, JS, and RS designed the study. JS contributed in writing. RS coordinated the study. All authors contributed to the article and approved the submitted version.
Funding
This study was financed in part by the Coordenação de Aperfeiçoamento de Pessoal de Nível Superior—Brasil (CAPES, Finance Code 001) and the Fundação de Amparo à Pesquisa do Estado de São Paulo (FAPESP, grant 2017/26253-3). RS and JS were recipients of research fellowships from Conselho Nacional de Desenvolvimento Científico e Tecnológico (CNPq grants 303325/2017-8 and 308837/2020-7).
Conflict of Interest
The authors declare that the research was conducted in the absence of any commercial or financial relationships that could be construed as a potential conflict of interest.
Publisher’s Note
All claims expressed in this article are solely those of the authors and do not necessarily represent those of their affiliated organizations, or those of the publisher, the editors and the reviewers. Any product that may be evaluated in this article, or claim that may be made by its manufacturer, is not guaranteed or endorsed by the publisher.
Acknowledgments
We would like to thank Cleomar de Souza Ferreira and Claudenice Moreira dos Santos for capable technical assistance.
Supplementary Material
The Supplementary Material for this article can be found online at: https://www.frontiersin.org/articles/10.3389/fnagi.2021.757714/full#supplementary-material
References
Abílio, V. C., Araujo, C. C., Bergamo, M., Calvente, P. R., D’Almeida, V., Ribeiro Rde, A., et al. (2003). Vitamin E attenuates reserpine-induced oral dyskinesia and striatal oxidized glutathione/reduced glutathione ratio (GSSG/GSH) enhancement in rats. Prog. Neuropsychopharmacol. Biol. Psychiatry 27, 109–114. doi: 10.1016/s0278-5846(02)00340-8
Alexander, G. E. (2004). Biology of Parkinson’s disease: pathogenesis and pathophysiology of a multisystem neurodegenerative disorder. Dialog. Clin. Neurosci. 6, 259–280.
Antzoulatos, E., Jakowec, M. W., Petzinger, G. M., and Wood, R. I. (2010). Sex differences in motor behavior in the MPTP mouse model of Parkinson’s disease. Pharmacol. Biochem. Behav. 95, 466–472. doi: 10.1016/j.pbb.2010.03.009
Becker, J. B. (1990). Direct effect of 17 beta-estradiol on striatum: sex differences in dopamine release. Synapse 5, 157–164. doi: 10.1002/syn.890050211
Behl, C., Widmann, M., Trapp, T., and Holsboer, F. (1995). 17-beta estradiol protects neurons from oxidative stress-induced cell death in vitro. Biochem. Biophys. Res. Commun. 216, 473–482. doi: 10.1006/bbrc.1995.2647
Benedetti, M. D., Maraganore, D. M., Bower, J. H., McDonnell, S. K., Peterson, B. J., Ahlskog, J. E., et al. (2001). Hysterectomy, menopause, and estrogen use preceding Parkinson’s disease: an exploratory case-control study. Mov. Disord. 16, 830–837. doi: 10.1002/mds.1170
Bispo, J. M. M., Melo, J. E. C., Gois, A. M., Leal, P. C., Lins, L., Souza, M. F., et al. (2019). Sex differences in the progressive model of parkinsonism induced by reserpine in rats. Behav. Brain Res. 363, 23–29. doi: 10.1016/j.bbr.2019.01.041
Brown, R. G., and Marsden, C. D. (1990). Cognitive function in Parkinson’s disease: from description to theory. Trends Neurosci. 13, 21–29. doi: 10.1016/0166-2236(90)90058-i
Campelo, C. L. C., Santos, J. R., Silva, A. F., Dierschnabel, A. L., Pontes, A., Cavalcante, J. S., et al. (2017). Exposure to an enriched environment facilitates motor recovery and prevents short-term memory impairment and reduction of striatal BDNF in a progressive pharmacological model of parkinsonism in mice. Behav. Brain Res. 328, 138–148. doi: 10.1016/j.bbr.2017.04.028
Cass, W. A., Peters, L. E., and Smith, M. P. (2005). Reductions in spontaneous locomotor activity in aged male, but not female, rats in a model of early Parkinson’s disease. Brain Res. 1034, 153–161. doi: 10.1016/j.brainres.2004.12.009
Cereda, E., Cilia, R., Klersy, C., Siri, C., Pozzi, B., Reali, E., et al. (2016). Dementia in Parkinson’s disease: is male gender a risk factor? Parkinsonism Relat. Disord. 26, 67–72. doi: 10.1016/j.parkreldis.2016.02.024
Chaudhuri, K. R., and Naidu, Y. (2008). Early Parkinson’s disease and non-motor issues. J. Neurol. 255(Suppl. 5), 33–38. doi: 10.1007/s00415-008-5006-1
Chaudhuri, K. R., and Schapira, A. H. (2009). Non-motor symptoms of Parkinson’s disease: dopaminergic pathophysiology and treatment. Lancet Neurol. 8, 464–474. doi: 10.1016/S1474-4422(09)70068-7
Choleris, E., Thomas, A. W., Kavaliers, M., and Prato, F. S. (2001). A detailed ethological analysis of the mouse open field test: effects of diazepam, chlordiazepoxide and an extremely low frequency pulsed magnetic field. Neurosci. Biobehav. Rev. 25, 235–260. doi: 10.1016/s0149-7634(01)00011-2
Cintra, R. R., Lins, L., Medeiros, K., Souza, M. F., Gois, A. M., Bispo, J. M. M., et al. (2021). Nociception alterations precede motor symptoms in a progressive model of parkinsonism induced by reserpine in middle-aged rats. Brain Res. Bull. 171, 1–9. doi: 10.1016/j.brainresbull.2021.03.001
Cosgrove, K. P., Mazure, C. M., and Staley, J. K. (2007). Evolving knowledge of sex differences in brain structure, function, and chemistry. Biol. Psychiatry 62, 847–855. doi: 10.1016/j.biopsych.2007.03.001
Cronan, T., Conrad, J., and Bryson, R. (1985). Effects of chronically administered nicotine and saline on motor activity in rats. Pharmacol. Biochem. Behav. 22, 897–899. doi: 10.1016/0091-3057(85)90544-1
Datla, K. P., Murray, H. E., Pillai, A. V., Gillies, G. E., and Dexter, D. T. (2003). Differences in dopaminergic neuroprotective effects of estrogen during estrous cycle. Neuroreport 14, 47–50. doi: 10.1097/00001756-200301200-00009
de Lau, L. M. L., and Breteler, M. M. B. (2006). Epidemiology of Parkinson’s disease. Lancet Neurol. 5, 525–535. doi: 10.1016/S1474-4422(06)70471-9
Dluzen, D., and Horstink, M. (2003). Estrogen as neuroprotectant of nigrostriatal dopaminergic system: laboratory and clinical studies. Endocrine 21, 67–75. doi: 10.1385/endo:21:1:67
Dluzen, D. E. (2000). Neuroprotective effects of estrogen upon the nigrostriatal dopaminergic system. J. Neurocytol. 29, 387–399. doi: 10.1023/a:1007117424491
Dluzen, D. E., McDermott, J. L., and Liu, B. (1996). Estrogen alters MPTP-induced neurotoxicity in female mice: effects on striatal dopamine concentrations and release. J. Neurochem. 66, 658–666. doi: 10.1046/j.1471-4159.1996.66020658.x
Dos Santos, T. F. O., de, R. S. E., Bispo, J. M. M., de Souza, M. F., de Gois, A. M., Lins, L., et al. (2021). Balance alterations and reduction of pedunculopontine cholinergic neurons in early stages of parkinsonism in middle-aged rats. Exp. Gerontol. 145:111198. doi: 10.1016/j.exger.2020.111198
Fernandes, V. S., Santos, J. R., Leao, A. H., Medeiros, A. M., Melo, T. G., Izidio, G. S., et al. (2012). Repeated treatment with a low dose of reserpine as a progressive model of Parkinson’s disease. Behav. Brain Res. 231, 154–163. doi: 10.1016/j.bbr.2012.03.008
Field, E. F., Metz, G. A., Pellis, S. M., and Whishaw, I. Q. (2006). Sexually dimorphic postural adjustments during vertical behaviour are altered in a unilateral 6-OHDA rat model of Parkinson’s disease. Behav. Brain Res. 174, 39–48. doi: 10.1016/j.bbr.2006.07.004
Fuentes, P., Paris, I., Nassif, M., Caviedes, P., and Segura-Aguilar, J. (2007). Inhibition of VMAT-2 and DT-diaphorase induce cell death in a substantia nigra-derived cell line–an experimental cell model for dopamine toxicity studies. Chem. Res. Toxicol. 20, 776–783. doi: 10.1021/tx600325u
Gillies, G. E., Murray, H. E., Dexter, D., and McArthur, S. (2004). Sex dimorphisms in the neuroprotective effects of estrogen in an animal model of Parkinson’s disease. Pharmacol. Biochem. Behav. 78, 513–522. doi: 10.1016/j.pbb.2004.04.022
Gillies, G. E., Pienaar, I. S., Vohra, S., and Qamhawi, Z. (2014). Sex differences in Parkinson’s disease. Front. Neuroendocrinol. 35, 370–384. doi: 10.1016/j.yfrne.2014.02.002
Haaxma, C. A., Bloem, B. R., Borm, G. F., Oyen, W. J. G., Leenders, K. L., Eshuis, S., et al. (2007). Gender differences in Parkinson’s disease. J. Neurol. Neurosurg. Psychiatry 78, 819–824. doi: 10.1136/jnnp.2006.103788
Hsieh, M. H., Meng, W. Y., Liao, W. C., Weng, J. C., Li, H. H., Su, H. L., et al. (2017). Ceftriaxone reverses deficits of behavior and neurogenesis in an MPTP-induced rat model of Parkinson’s disease dementia. Brain Res. Bull. 132, 129–138. doi: 10.1016/j.brainresbull.2017.05.015
Hyman, C., Hofer, M., Barde, Y. A., Juhasz, M., Yancopoulos, G. D., Squinto, S. P., et al. (1991). BDNF is a neurotrophic factor for dopaminergic neurons of the substantia nigra. Nature 350, 230–232. doi: 10.1038/350230a0
Ikram, H., and Haleem, D. J. (2019). Repeated treatment with a low dose of reserpine as a progressive model of Parkinson’s dementia. Pak. J. Pharm. Sci. 32, 555–562.
Leal, P. C., Bispo, J. M. M., Lins, L., Souza, M. F., Gois, A. M., Moore, C., et al. (2019). Cognitive and anxiety-like impairments accompanied by serotonergic ultrastructural and immunohistochemical alterations in early stages of parkinsonism. Brain Res. Bull. 146, 213–223. doi: 10.1016/j.brainresbull.2019.01.009
Leão, A., Meurer, Y. S. R., Freitas, T. A., Medeiros, A. M., Abilio, V. C., Izidio, G. S., et al. (2021). Changes in the mesocorticolimbic pathway after low dose reserpine-treatment in Wistar and Spontaneously hypertensive rats (SHR): implications for cognitive deficits in a progressive animal model for Parkinson’s disease. Behav Brain Res. 410:113349. doi: 10.1016/j.bbr.2021.113349
Leão, A. H., Meurer, Y. S., da Silva, A. F., Medeiros, A. M., Campelo, C. L., Abilio, V. C., et al. (2017). Spontaneously hypertensive rats (SHR) are resistant to a reserpine-induced progressive model of Parkinson’s disease: differences in motor behavior, tyrosine hydroxylase and alpha-synuclein expression. Front. Aging Neurosci. 9:78. doi: 10.3389/fnagi.2017.00078
Leão, A. H., Sarmento-Silva, A. J., Santos, J. R., Ribeiro, A. M., and Silva, R. H. (2015). Molecular, neurochemical, and behavioral hallmarks of reserpine as a model for Parkinson’s disease: new perspectives to a long-standing model. Brain Pathol. 25, 377–390. doi: 10.1111/bpa.12253
Lins, L., Souza, M. F., Bispo, J. M. M., Gois, A. M., Melo, T. C. S., Andrade, R. A. S., et al. (2018). Carvacrol prevents impairments in motor and neurochemical parameters in a model of progressive parkinsonism induced by reserpine. Brain Res. Bull. 139, 9–15. doi: 10.1016/j.brainresbull.2018.01.017
Maggi, A., Ciana, P., Belcredito, S., and Vegeto, E. (2004). Estrogens in the nervous system: mechanisms and nonreproductive functions. Annu. Rev. Physiol. 66, 291–313. doi: 10.1146/annurev.physiol.66.032802.154945
Marcondes, F. K., Bianchi, F. J., and Tanno, A. P. (2002). Determination of the estrous cycle phases of rats: some helpful considerations. Braz. J. Biol. 62, 609–614. doi: 10.1590/s1519-69842002000400008
Martinez-Martin, P., Falup Pecurariu, C., Odin, P., van Hilten, J. J., Antonini, A., Rojo-Abuin, J. M., et al. (2012). Gender-related differences in the burden of non-motor symptoms in Parkinson’s disease. J. Neurol. 259, 1639–1647. doi: 10.1007/s00415-011-6392-3
Matheus, F. C., Rial, D., Real, J. I., Lemos, C., Ben, J., Guaita, G. O., et al. (2016). Decreased synaptic plasticity in the medial prefrontal cortex underlies short-term memory deficits in 6-OHDA-lesioned rats. Behav. Brain Res. 301, 43–54. doi: 10.1016/j.bbr.2015.12.011
McArthur, S., Murray, H. E., Dhankot, A., Dexter, D. T., and Gillies, G. E. (2007). Striatal susceptibility to a dopaminergic neurotoxin is independent of sex hormone effects on cell survival and DAT expression but is exacerbated by central aromatase inhibition. J. Neurochem. 100, 678–692. doi: 10.1111/j.1471-4159.2006.04226.x
Morissette, M., Al Sweidi, S., Callier, S., and Di Paolo, T. (2008). Estrogen and SERM neuroprotection in animal models of Parkinson’s disease. Mol. Cell Endocrinol. 290, 60–69. doi: 10.1016/j.mce.2008.04.008
Moustafa, A. A., Chakravarthy, S., Phillips, J. R., Gupta, A., Keri, S., Polner, B., et al. (2016). Motor symptoms in Parkinson’s disease: a unified framework. Neurosci. Biobehav. Rev. 68, 727–740. doi: 10.1016/j.neubiorev.2016.07.010
Murray, H. E., Pillai, A. V., McArthur, S. R., Razvi, N., Datla, K. P., Dexter, D. T., et al. (2003). Dose- and sex-dependent effects of the neurotoxin 6-hydroxydopamine on the nigrostriatal dopaminergic pathway of adult rats: differential actions of estrogen in males and females. Neuroscience 116, 213–222. doi: 10.1016/s0306-4522(02)00578-x
Ookubo, M., Yokoyama, H., Kato, H., and Araki, T. (2009). Gender differences on MPTP (1-methyl-4-phenyl-1,2,3,6-tetrahydropyridine) neurotoxicity in C57BL/6 mice. Mol. Cell Endocrinol. 311, 62–68. doi: 10.1016/j.mce.2009.07.011
Peres, F. F., Levin, R., Suiama, M. A., Diana, M. C., Gouvea, D. A., Almeida, V., et al. (2016). Cannabidiol prevents motor and cognitive impairments induced by reserpine in rats. Front. Pharmacol. 7:343. doi: 10.3389/fphar.2016.00343
Rahmani, F., Saghazadeh, A., Rahmani, M., Teixeira, A. L., Rezaei, N., Aghamollaii, V., et al. (2019). Plasma levels of brain-derived neurotrophic factor in patients with Parkinson disease: a systematic review and meta-analysis. Brain Res. 1704, 127–136. doi: 10.1016/j.brainres.2018.10.006
Rajsombath, M. M., Nam, A. Y., Ericsson, M., and Nuber, S. (2019). Female sex and brain-selective estrogen benefit alpha-synuclein tetramerization and the PD-like motor syndrome in 3K transgenic mice. J. Neurosci. 39, 7628–7640. doi: 10.1523/JNEUROSCI.0313-19.2019
Reckziegel, P., Peroza, L. R., Schaffer, L. F., Ferrari, M. C., de Freitas, C. M., Bürger, M. E., et al. (2013). Gallic acid decreases vacuous chewing movements induced by reserpine in rats. Pharmacol. Biochem. Behav. 104, 132–137. doi: 10.1016/j.pbb.2013.01.001
Reekes, T. H., Higginson, C. I., Ledbetter, C. R., Sathivadivel, N., Zweig, R. M., and Disbrow, E. A. (2020). Sex specific cognitive differences in Parkinson disease. NPJ Parkinsons Dis. 6:7. doi: 10.1038/s41531-020-0109-1
Ribeiro, A. M., Barbosa, F. F., Godinho, M. R., Fernandes, V. S., Munguba, H., Melo, T. G., et al. (2010). Sex differences in aversive memory in rats: possible role of extinction and reactive emotional factors. Brain Cogn. 74, 145–151. doi: 10.1016/j.bandc.2010.07.012
Roheger, M., Kalbe, E., and Liepelt-Scarfone, I. (2018). Progression of cognitive decline in Parkinson’s disease. J. Parkinsons Dis. 8, 183–193. doi: 10.3233/JPD-181306
Sanberg, P. R., Bunsey, M. D., Giordano, M., and Norman, A. B. (1988). The catalepsy test: its ups and downs. Behav. Neurosci. 102, 748–759. doi: 10.1037//0735-7044.102.5.748
Santos, J. R., Cunha, J. A., Dierschnabel, A. L., Campelo, C. L., Leao, A. H., Silva, A. F., et al. (2013). Cognitive, motor and tyrosine hydroxylase temporal impairment in a model of parkinsonism induced by reserpine. Behav. Brain Res. 253, 68–77. doi: 10.1016/j.bbr.2013.06.031
Saunders-Pullman, R., Gordon-Elliott, J., Parides, M., Fahn, S., Saunders, H. R., and Bressman, S. (1999). The effect of estrogen replacement on early Parkinson’s disease. Neurology 52, 1417–1421. doi: 10.1212/wnl.52.7.1417
Scott, B., Borgman, A., Engler, H., Johnels, B., and Aquilonius, S. M. (2000). Gender differences in Parkinson’s disease symptom profile. Acta Neurol. Scand. 102, 37–43. doi: 10.1034/j.1600-0404.2000.102001037.x
Shulman, L. M. (2007). Gender differences in Parkinson’s disease. Gender Med. 4, 8–18. doi: 10.1016/S1550-8579(07)80003-9
Siani, F., Greco, R., Levandis, G., Ghezzi, C., Daviddi, F., Demartini, C., et al. (2017). Influence of estrogen modulation on glia activation in a murine model of Parkinson’s disease. Front. Neurosci. 11:306. doi: 10.3389/fnins.2017.00306
Silva, R. H., Abílio, V. C., Torres-Leite, D., Bergamo, M., Chinen, C. C., Claro, F. T., et al. (2002). Concomitant development of oral dyskinesia and memory deficits in reserpine-treated male and female mice. Behav. Brain Res. 132, 171–177. doi: 10.1016/s0166-4328(01)00409-0
Silva-Martins, S., Beserra-Filho, J. I. A., Maria-Macêdo, A., Custódio-Silva, A. C., Soares-Silva, B., Silva, S. P., et al. (2021). Myrtenol complexed with β-cyclodextrin ameliorates behavioural deficits and reduces oxidative stress in the reserpine-induced animal model of Parkinsonism. Clin. Exp. Pharmacol. Physiol. doi: 10.1111/1440-1681.13563 Epub ahead of print.
Solimeo, S. (2008). Sex and gender in older adults’ experience of Parkinson’s disease. J. Gerontol. B Psychol. Sci. Soc. Sci. 63, S42–S48. doi: 10.1093/geronb/63.1.s42
Tamas, A., Lubics, A., Szalontay, L., Lengvari, I., and Reglodi, D. (2005). Age and gender differences in behavioral and morphological outcome after 6-hydroxydopamine-induced lesion of the substantia nigra in rats. Behav. Brain Res. 158, 221–229. doi: 10.1016/j.bbr.2004.09.002
Tenkorang, M. A., Snyder, B., and Cunningham, R. L. (2018). Sex-related differences in oxidative stress and neurodegeneration. Steroids 133, 21–27. doi: 10.1016/j.steroids.2017.12.010
Tiskievicz, F., Mallmann, E. S., Brenol, J. C., Xavier, R. M., and Spritzer, P. M. (2011). Prolactin, estradiol and anticardiolipin antibodies in premenopausal women with systemic lupus erythematosus: a pilot study. Rev. Bras. Reumatol. 51, 460–464.
Todorova, A., Jenner, P., and Ray Chaudhuri, K. (2014). Non-motor Parkinson’s: integral to motor Parkinson’s, yet often neglected. Pract. Neurol. 14, 310–322. doi: 10.1136/practneurol-2013-000741
Tsang, K. L., Ho, S. L., and Lo, S. K. (2000). Estrogen improves motor disability in parkinsonian postmenopausal women with motor fluctuations. Neurology 54, 2292–2298. doi: 10.1212/wnl.54.12.2292
Tysnes, O. B., and Storstein, A. (2017). Epidemiology of Parkinson’s disease. J. Neural Transm. (Vienna) 124, 901–905. doi: 10.1007/s00702-017-1686-y
Van Den Eeden, S. K., Tanner, C. M., Bernstein, A. L., Fross, R. D., Leimpeter, A., Bloch, D. A., et al. (2003). Incidence of Parkinson’s disease: variation by age, gender, and race/ethnicity. Am. J. Epidemiol. 157, 1015–1022. doi: 10.1093/aje/kwg068
Varmazyar, R., Noori-Zadeh, A., Rajaei, F., Darabi, S., and Bakhtiyari, S. (2019). 17 β-estradiol oxidative stress attenuation and autophagy-induced dopaminergic neuroprotection. Cell J. 21, 1–6. doi: 10.22074/cellj.2019.5799
Walsh, R. N., and Cummins, R. A. (1976). The open-field test: a critical review. Psychol. Bull. 83, 482–504.
Wang, Y., Liu, H., Zhang, B. S., Soares, J. C., and Zhang, X. Y. (2016). Low BDNF is associated with cognitive impairments in patients with Parkinson’s disease. Parkinsonism Relat. Disord. 29, 66–71. doi: 10.1016/j.parkreldis.2016.05.023
Watson, C. S., Alyea, R. A., Hawkins, B. E., Thomas, M. L., Cunningham, K. A., and Jakubas, A. A. (2006). Estradiol effects on the dopamine transporter - protein levels, subcellular location, and function. J. Mol. Signal. 1:5. doi: 10.1186/1750-2187-1-5
Wirdefeldt, K., Adami, H.-O., Cole, P., Trichopoulos, D., and Mandel, J. (2011). Epidemiology and etiology of Parkinson’s disease: a review of the evidence. Eur. J. Epidemiol. 26:1. doi: 10.1007/s10654-011-9581-6
Yu, L., and Liao, P. C. (2000). Sexual differences and estrous cycle in methamphetamine-induced dopamine and serotonin depletions in the striatum of mice. J. Neural Transm. (Vienna) 107, 419–427. doi: 10.1007/s007020070084
Zarate, S. M., Pandey, G., Chilukuri, S., Garcia, J. A., Cude, B., Storey, S., et al. (2021). Cytisine is neuroprotective in female but not male 6-hydroxydopamine lesioned parkinsonian mice and acts in combination with 17-β-estradiol to inhibit apoptotic endoplasmic reticulum stress in dopaminergic neurons. J. Neurochem. 157, 710–726. doi: 10.1111/jnc.15282
Keywords: parkinsonism, motor impairment, cognitive deficits, sexual dimorphism, tyrosine hydroxylase, estrogen
Citation: Lima AC, Meurer YSR, Bioni VS, Cunha DMG, Gonçalves N, Lopes-Silva LB, Becegato M, Soares MBL, Marinho GF, Santos JR and Silva RH (2021) Female Rats Are Resistant to Cognitive, Motor and Dopaminergic Deficits in the Reserpine-Induced Progressive Model of Parkinson’s Disease. Front. Aging Neurosci. 13:757714. doi: 10.3389/fnagi.2021.757714
Received: 12 August 2021; Accepted: 27 September 2021;
Published: 25 October 2021.
Edited by:
Arianna Maffei, Stony Brook University, United StatesReviewed by:
Sanjay Bhaskar Kasture, Pinnacle Biomedical Research Institute (PBRI), IndiaRahul Srinivasan, Texas A&M Health Science Center, United States
Copyright © 2021 Lima, Meurer, Bioni, Cunha, Gonçalves, Lopes-Silva, Becegato, Soares, Marinho, Santos and Silva. This is an open-access article distributed under the terms of the Creative Commons Attribution License (CC BY). The use, distribution or reproduction in other forums is permitted, provided the original author(s) and the copyright owner(s) are credited and that the original publication in this journal is cited, in accordance with accepted academic practice. No use, distribution or reproduction is permitted which does not comply with these terms.
*Correspondence: Regina H. Silva, reginahsilva@gmail.com; r.silva@unifesp.br