- 1Department of Physical Therapy and Assistive Technology, National Yang Ming Chiao Tung University, Taipei, Taiwan
- 2Division of Nerve Repair, Department of Neurosurgery, Taipei Veterans General Hospital, Taipei, Taiwan
- 3Division of General Neurology, Department of Neurology, Neurological Institute, Taipei Veterans General Hospital, Taipei, Taiwan
Background: Transcranial direct current stimulation (tDCS) is a non-invasive brain stimulation to modulate cortical activity for improving motor function. However, the information of tDCS stimulation on different brain regions for dual-task walking and cortical modulation in Parkinson’s disease (PD) has not yet been compared.
Objective: The objective of this study was to investigate the effects of different tDCS targets on dual-task gait performance and cortical activity in patients with PD.
Methods: A total of 36 participants were randomly assigned to primary motor cortex (M1) tDCS, dorsal lateral prefrontal cortex (DLPFC) tDCS, cerebellum tDCS, or Sham tDCS group. Each group received 20 min of tDCS stimulation, except for the Sham group. Gait performance was measured by the GAITRite system during dual-task walking and single walking. Corticomotor activity of the tibialis anterior (TA) was measured using transcranial magnetic stimulation (TMS). The functional mobility was assessed using the timed up and go (TUG) test.
Results: All participants showed no significant differences in baseline data. Following the one session of tDCS intervention, M1 (p = 0.048), DLPFC (p < 0.001), and cerebellum (p = 0.001) tDCS groups demonstrated significant improvements in dual-task gait speed compared with a pretest. The time × group interaction [F(3, 32) = 5.125, p = 0.005] was detected in dual-task walking speed. The post hoc Tukey’s test showed that the differences in gait speed were between the Sham tDCS group and the DLPFC tDCS group (p = 0.03). Moreover, DLPFC tDCS also increased the silent period (SP) more than M1 tDCS (p = 0.006) and Sham tDCS (p = 0.002).
Conclusion: The results indicate that DLPFC tDCS exerted the most beneficial effects on dual-task walking and cortical modulation in participants with PD.
Clinical trial registration: [http://www.thaiclinicaltrials.org/show/TCTR20200909005], Thai Clinical Trials Registry [TCTR20200909005].
Introduction
Parkinson’s disease (PD) is a degenerative neurological disease due to the loss of dopaminergic neurons in the substantia nigra pars compacta in basal ganglia (BG) (Lees et al., 2009). With impaired interactions among cortico-BG-cerebellar circuits, the deficits in gait performance are frequently seen in individuals with PD (Hausdorff et al., 1998). In addition to classical motor symptoms, cognitive symptoms are widely accepted as part of the clinical feature in individuals with PD. These motor and cognitive impairments increased their difficulties to perform complex daily activities, such as dual-task walking (i.e., responding to a cognitive demanding task while walking) (Benecke et al., 1986; Raffegeau et al., 2019). According to a meta-analysis, the gait speed and stride length decreased under the condition of dual-task walking as compared with single walking in people with PD (Raffegeau et al., 2019). These significant difficulties in dual-task walking may lead to increased disability, fall risks, and decreased quality of life in people with PD (Kelly et al., 2012). Therefore, how to improve the dual-task walking performance is crucial for people with PD.
In addition to deficits in cortico-BG-cerebellar circuits, 33 studies demonstrated abnormal activity in primary motor cortex (M1), dorsal lateral prefrontal cortex (DLPFC), and cerebellum during dual-task performance in patients with PD (Wu and Hallett, 2005; Nieuwhof et al., 2016; Al-Yahya et al., 2019). It has been suggested that abnormal plasticity within M1 reflects a loss of coordination among the BG, cerebellar, and cortical inputs and eventually causes motor impairments in PD (Gaspar et al., 1991; Kishore et al., 2014). The decreased M1 inhibition during resting was reported in people with PD by transcranial magnetic stimulation (TMS) (Rossini et al., 2015), which may be one of the compensations for the cortico-BG-cerebellar deficit. Furthermore, the pattern of hypo-activation between the cortical area and striatum was associated with gait impairment in PD (Shine et al., 2013). Therefore, modulating brain activities might be a strategy for motor improvement, especially the complex movement, such as dual-task walking in individuals with PD.
The transcranial direct current stimulation (tDCS) is a non-invasive technique to modulate cortical excitability (Sánchez-Kuhn et al., 2017). Anodal tDCS has been considered not only to alter cortical excitability but also to exert subcortical effects (Polanía et al., 2012). Fregni et al. (2006) indicated that a single session of M1 tDCS improved upper extremity performance. Moreover, Ferrucci et al. (2016) demonstrated that 5 sessions of cerebellum tDCS decreased the disease severity as indicated by the Unified Parkinson’s Disease Rating Scale (UPDRS). The previous study suggested that the lateral cerebellar region plays an important role in the complex motor task, such as dual-task walking (Ilg and Timmann, 2013). Furthermore, a recent review suggested that cerebellum may be the potential tDCS target area to improve the gait performance in people with PD (Potvin-Desrochers and Paquette, 2021). In contrast, as mentioned earlier, walking in daily activities demands interactions between motor and cognitive control, particularly, executive function (Yuan and Raz, 2014). The DLPFC has been recognized as the key area for executive function, and the relative DLPFC activations during gait can be demonstrated via dual-task walking (Collette et al., 2005; Beurskens et al., 2014). Evidence also showed that a single session of DLPFC tDCS improved balance and mobility (Manenti et al., 2014; Lattari et al., 2017). Taking together, the potential use of tDCS has been demonstrated for people with PD in neurorehabilitation. However, it is not known whether different tDCS targets would modulate the brain differently to result in different effects on motor performance. Therefore, this study aimed to compare the different tDCS targets in brain modulation and dual-task walking performance in individuals with PD.
Materials and Methods
Subjects
This study protocol was approved by the Institutional Review Board of Taipei Veterans General Hospital and Ministry of Health and Welfare. This trial was registered at https://www.clinicaltrials.in.th/(TCTR20200909005) and conformed to the CONSORT checklist. Participants who were diagnosed with idiopathic PD by neurologists (J-LF and H-LC) were recruited from Taipei Veterans General Hospital. The age, gender, duration since the diagnosis of PD, and medications were obtained from the detailed clinical interviews and medical charts. Inclusion criteria were as follows: (1) stages 1–3 on the Hoehn and Yahr scale, (2) ability to walk independently for at least 10 m without the use of walking aids, (3) stable medical condition, and (4) a score of ≥ 24 on the Mini-Mental State Examination (MMSE). Exclusion criteria were as follows: (1) history of diseases or conditions known to interfere with participating in this study (e.g., epilepsy or metal implants in the brain) and (2) history of using central nervous system medications other than for PD, e.g., antiepileptic or antidepressant drugs in recent months. In total, 51 individuals were identified as potential subjects. Of these, 36 participants provided informed consent for participation in this study (Figure 1).
Experimental Design
This study was a double-blinded, randomized, controlled trial with pre- and post-measurements. An individual who was not involved in this study selected the sealed envelopes to assign participants to one of the four groups (i.e., M1 tDCS group, DLPFC tDCS group, cerebellum tDCS group, and Sham tDCS group) by the block of 2 randomizations. The cortical activities followed by gait performance were measured before (pretest) the real (or Sham) tDCS by the assessor who was blinded to the group assignment (assessor blinded) (Figure 1). Participants were blinded to their group assignment (participants blinded) and received one session of real or Sham tDCS for 20 min according to the group assignment. After 20 min of tDCS, all participants were measured the cortical activities immediately after tDCS and gait performance for 30 min after tDCS. All interventions and assessments were carried out with patients in the “on” status.
Intervention
The stimulation was delivered by a current stimulator (Eldith DC Stimulator, NeuroConn, Germany) through a pair of 35 cm2 electrodes with a maximal output of 2 mA. The stimulation intensity was set to 2 mA for 20 min.
a. M1 tDCS group: The anode was placed over the M1 of the dominant hemisphere (C3 according to EEG 10/20 system), and the cathode was placed over the contralateral supraorbital ridge (Fregni et al., 2006).
b. DLPFC tDCS group: The anode was placed over the DLPFC of the dominant hemisphere (F3 according to EEG 10/20 system), and the cathode was placed over the contralateral supraorbital ridge (Fregni et al., 2006; Lattari et al., 2017).
c. Cerebellum tDCS group: The anode was placed 1 cm below and 2 cm lateral to the inion over the dominant hemisphere, and the cathode was placed over the contralateral supraorbital ridge (Ferrucci et al., 2015).
d. Sham tDCS group: The electrodes were positioned as described in the M1 tDCS group. However, the current was delivered only for the first 60 s, with a ramp up and ramp down for 30 s.
Outcome Measures
Primary Outcome Measures
The primary outcome of this study was dual-task walking performance measured by a GAITRite system (CIR system, Inc., Havertown, PA, United States) (Yang et al., 2019). The GAITRite system is 4.75 m long and 0.9 m wide, and the pressure-sensitive area of the walkway is 4.30 m long and 0.61 m wide. The dual-task walking was walking while performing serial subtracting by three, starting from a randomized 3-digit number at a comfortable speed. The walking trial was repeated three times with 60 s rest in between. The average of the three trials of each walking condition was used for data analysis. Gait parameters of interest were speed, cadence, stride time, stride length, and coefficients of variation (CV) of stride time and stride length. The formula of CV is standard deviation/mean × 100%. A lower CV value means a more consistent gait pattern (Yang et al., 2013). In addition, the dual-task cost (DTC) was calculated to indicate the dual-task interference. The formula of DTC was DTC = (dual-task walking speed – single-task walking speed)/single-task walking speed × 100% (Yang et al., 2019).
Secondary Outcome Measures
The secondary outcomes included corticomotor activity, single walking performance, and functional mobility.
Corticomotor Activity
The resting motor threshold (RMT), motor evoked potentials (MEPs), and silent period (SP) duration of the tibialis anterior (TA) elicited by TMS (Magstim 200 magnetic stimulator; Magstim Company, Whiteland, Dyfed, United Kingdom) were used to indicate the corticomotor activity. The MEPs of TA were recorded by an electromyographic (EMG) machine in response to TMS delivered through a double-cone coil placed on the M1 with participants lying supine wearing a fitted cap marked with a coordinate system (distance, 1 cm). The optimal scalp location (hot spot) was determined by moving the TMS stimulator over the scalp in 1-cm steps. Once the hot spot was identified, a single-pulse TMS was delivered to the location to determine the RMT, as the lowest stimulus intensity necessary to elicit MEPs greater than 0.05-mV peak-to-peak amplitude in at least 5 of 10 consecutive stimuli (Yang et al., 2013). The RMT was expressed as a percentage of maximum stimulator output, which reflects the excitability of motor cortex (Groppa et al., 2012). The MEPs were measured at an intensity of 120% RMT, and the peak-to-peak amplitudes of the MEPs of 10 trials were collected and averaged. The amplitude of MEPs is thought to represent the corticospinal excitability of the M1 (Groppa et al., 2012). Both RMT and MEP were considered to be mediated by the glutamatergic system indicated by the TMS-pharmacological study (Kapogiannis and Wassermann, 2008; Paulus et al., 2008). The SP duration was determined during isometric voluntary contraction of TA. Ten magnetic stimuli were applied at an intensity of 120% RMT, while the participant performed maximum of 20% voluntary contraction. The intensity used in the post-assessment was the same as that used in the pre-assessment. The SP duration was determined from the MEP onset to the recurrence of at least 50% of EMG background activity (Yang et al., 2013). The neurophysiological phenomenon of SP is thought to be due to inhibition mechanisms of the motor cortex mediated through the GABAergic system (Werhahn et al., 1999).
Single Walking Performance
The single walking performance was also measured using the GAITRite system. For a single walking performance, the participants walked at their comfortable speed without additional tasking. The average of the three trials was used for data analysis.
Timed Up and Go Test
The timed up and go (TUG) was used to evaluate the functional mobility. The participants were seated in a chair and were instructed to stand up, walk 3 m, turn around, walk back to the chair, and then sit down. We recorded the time needed to complete this task. A high reliability of this test has been documented in individuals with PD (Morris et al., 2001).
Statistical Analysis
All analyses were performed using the SPSS version 24.0. Descriptive statistics [mean ± standard deviation, frequency, or median (interquartile range)] were generated for all variables. The Shapiro–Wilk test was used to assess the normal distributions. The intergroup difference of baseline (pretest) data was analyzed by using the Kruskal–Wallis test and one-way ANOVA for continuous variables or χ2 test for nominal scales. Accordingly, the two-way repeated measures ANOVA (group × time) was used for intergroup comparisons of dual-task walking, single walking, and TUG performance, followed by the post hoc Tukey’s test with the Bonferroni correction, which multiplied the uncorrected p-values by 6 for multiple comparisons between four groups, if there was group × time effect. The post hoc paired t-test was used to examine significance between pre- and post-data if there was a significant time effect. The Kruskal–Wallis one-way ANOVA was used for intergroup comparisons of change values in corticomotor activity due to not being normally distributed, followed by the post hoc Mann–Whitney U tests with the Bonferroni correction. The intragroup difference was thus analyzed by the Wilcoxon signed-rank test. The change values of corticomotor activity were calculated by subtracting the baseline data from the post-intervention data. Statistical significance was set at p < 0.05. The sample size was calculated using the G-Power version 3.1.9.7. Although the effect size was 0.85 for tDCS in improving dual-task gait performance in patients with PD (Mishra and Thrasher, 2021), in this study, we chose a relatively smaller effect size of 0.518 according to the study by Kaski et al. (2014). The total sample size was required to be 36 (9 per group) with a power of 0.80 and a two-tailed alpha level of 0.05 to detect a difference in gait performance.
Results
A total of 51 patients were screened for the eligibility of participating in this study. As a result, 36 patients were included in this study and were randomly assigned to the M1 tDCS group (n = 9), DLPFC tDCS group (n = 9), cerebellum tDCS group (n = 9), or Sham tDCS group (n = 9). Participants received 20 min of tDCS according to their group assignment. None of them reported any adverse events or withdrew from this study (Figure 1). No significant differences between groups were found in baseline demographic characteristics (Table 1) and all outcome measures at the pre-intervention assessment.
Dual-Task Walking Performance
Table 2 shows the dual-task walking performance at pre- and post-intervention for 4 study groups. Regarding the dual-task gait parameters, there was no group effect [F(3, 32) = 2.237, p = 0.103] but a significant effect of time [F(3, 32) = 56.616, p < 0.001] and time × group interaction [F(3, 32) = 5.125, p = 0.005]. The post hoc Tukey’s test with the Bonferroni correction showed that the differences in gait speed were between the Sham tDCS group and the DLPFC tDCS group (p = 0.03) (Figure 2). The cadence showed no group effect [F(3, 32) = 1.723, p = 0.182) but a significant effect of time [F(3, 32) = 41.497, p < 0.001] and time × group interaction [F(3, 32) = 5.180, p = 0.005]. However, the post hoc Tukey’s test with the Bonferroni correction did not show any group difference in cadence. The stride time showed no group effect [F(3, 32) = 1.487, p = 0.237] but a significant effect of time [F(3, 32) = 9.628, p = 0.004) and time × group interaction [F(3, 32) = 3.649, p = 0.023]. However, the post hoc Tukey’s test with the Bonferroni correction did not show any group difference in stride time. The stride length showed no group effect [F(3, 32) = 2.267, p = 0.100] but a significant effect of time [F(3, 32) = 22.069, p < 0.001] and time × group interaction [F(3, 32) = 3.340, p = 0.031]. However, the post hoc Tukey’s test with the Bonferroni correction did not show any group difference in stride length.
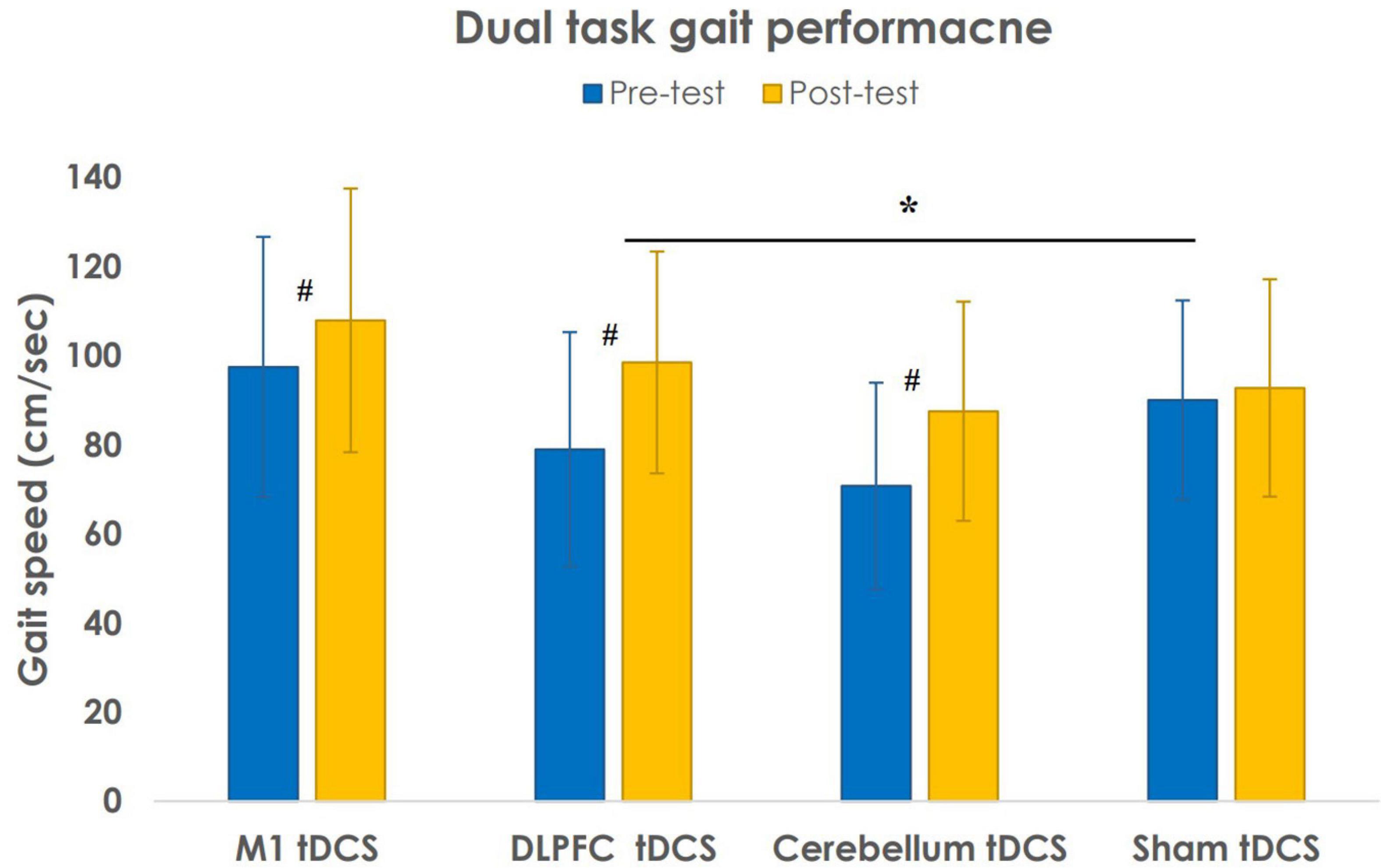
Figure 2. Results of gait speed during dual-task walking performance after different transcranial direct current stimulation (tDCS) stimulations. Data are presented as the mean ± SD. #P < 0.05: intragroup comparison. *P < 0.05: intergroup comparison.
Furthermore, two-way repeated ANOVA indicated several significant time effects. The post hoc paired t-test showed a significant increase in gait speed [t(8) = −6.963, p < 0.001], cadence [t(8) = −6.659, p < 0.001], and stride length [t(8) = −3.761, p = 0.006] and a decrease in stride time [t(8) = 4.600, p = 0.002] after DLPFC tDCS intervention. In addition, the post hoc paired t-tests indicated that patients in cerebellum tDCS group significantly increased in gait speed [t(8) = −5.231, p = 0.001], cadence [t(8) = −3.499, p = 0.008], and stride length [t(8) = −2.610, p = 0.031] and patients in M1 tDCS group significantly increased in gait speed [t(8) = −2.338, p = 0.048] and stride length [t(8) = −2.492, p = 0.037] after tDCS intervention.
Corticomotor Activity
Table 3 shows the cortical activity of M1 measured by the TMS before and after tDCS interventions. After the DLPFC tDCS stimulation, the SP of stimulating hemisphere increased significantly more than M1 tDCS (p = 0.038) and Sham tDCS (p = 0.001) (Figure 3). However, there was no significant difference in other groups. In contrast, the corticomotor activity of non-stimulating hemisphere did not change in this study.
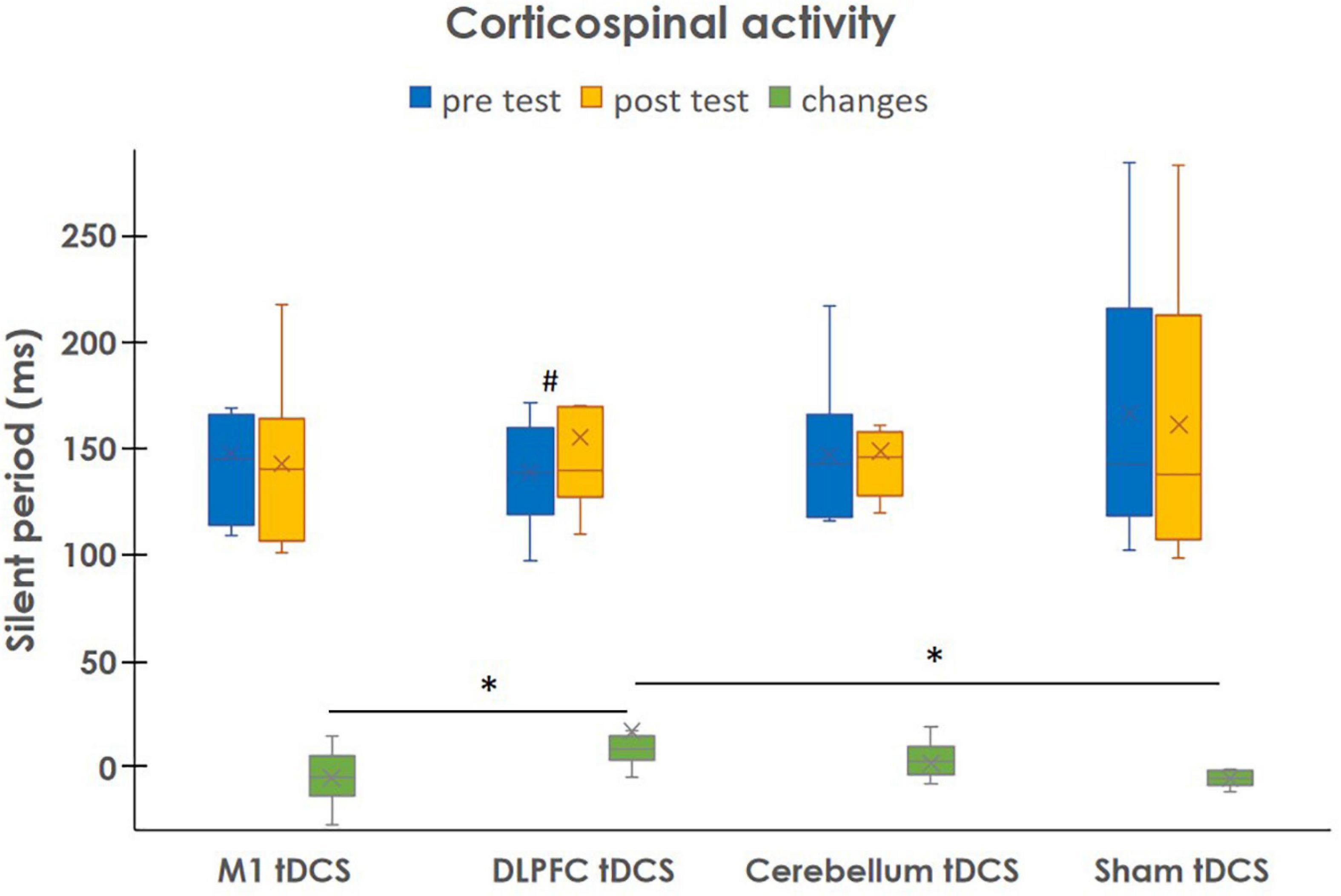
Figure 3. Changes in silent period of ipsilateral hemisphere relatively to the stimulating side after different tDCS stimulations. Data are presented as the median (interquartile range). #P < 0.05: intragroup comparison. *P < 0.05: intergroup comparison.
Single Walking Performance
Table 4 shows the single walking performance after different tDCS interventions. We found no significant time and group interaction for all gait parameters of single walking but a significant time effect. The post hoc paired t-test showed that a significant increase in gait speed [t(8) = −2.528, p = 0.035] and cadence [t(8) = −3.291, p = 0.011] after DLPFC tDCS intervention. In M1 tDCS group, the post hoc paired t-test showed a significant increase in stride length [t(8) = −3.315, p = 0.011] after tDCS intervention.
Timed Up and Go Performance
The results of TUG after different tDCS are shown in Table 4. We found no significant group effect [F(3, 32) = 0.289, p = 0.832], time effect [F(3, 32) = 0.006, p = 0.939], and group × time interactions [F(3, 32) = 1.174, p = 0.335]. In addition, there was no significant difference in intragroup comparisons.
Discussion
This randomized, double-blinded, controlled trial was the first study to compare the immediate neuromodulation effects of different tDCS targets on dual-task walking performance in individuals with PD. In this study, we found that only the tDCS on DLPFC increased cortical inhibition and exerted the most beneficial effects to improve dual-task walking in people with PD as compared with tDCS on M1 or Sham tDCS.
In this study, the improvements in dual-task walking coupled with increased SP duration were demonstrated after DLPFC tDCS. The change in dual-task gait speed was highly correlated with change in SP (Spearman’s correlation ρ = 0.733, p = 0.025). Wu et al. (2007) noted that shorter SP duration was associated with worse PD symptoms. We previously found the lengthening in SP duration and improvement of single walking performance after the combination of high-frequency repetitive transcranial magnetic stimulation (rTMS) and treadmill training in patients with PD (Yang et al., 2013). Fisher et al. (2008) demonstrated the SP lengthening associated with walking improvements after treadmill training and thus speculated SP lengthening could restore the normal motor processing in people with PD. Recent studies have provided the evidence for the potential of one session of DLPFC tDCS to enhance dopamine release in the striatum (Fonteneau et al., 2018; Fukai et al., 2019). A previous study also reported that dopaminergic treatment could prolong SP duration in patients with PD and suggested that SP may be modulated by the dopamine system (Nakashima et al., 1995). Furthermore, the recent study showed tDCS-induced dopamine release and GABA changes, which contributes to the phenomenon of SP (Bunai et al., 2021). Taking together, the beneficial motor effects of DLPFC tDCS may be related to dopamine release, therefore modulating the cortical inhibition in individuals with PD.
In contrast, dual tasking exacerbates the gait impairments in people with PD, suggesting the overloaded recruitment of prefrontal cortex (cognitive overloaded) under dual-task walking (Strouwen et al., 2015). DLPFC has been recognized as the key area for executive function which involves in many daily activities, especially dual-task walking (Lu et al., 2015). Therefore, we speculated that the improvements in dual-task walking after DLPFC tDCS may also be resulted from direct modulation of DLPFC. In this study, the participants walked faster by 24% under cognitive dual tasking after one session of DLPFC tDCS intervention. Mishra and Thrasher (2021) also reported that the increase of dual-task gait speed after a single session of DLPFC tDCS was more than Sham tDCS in patients with PD. Similarly, tDCS targeting the DLPFC has been reported to improve dual-task gait performance in older adults and people with stroke (Zhou et al., 2021). In contrast, we previously noted that the dual-task gait training for 12 sessions resulted in a 20% improvement of cognitive dual-task walking speed in people with PD (Yang et al., 2019). Therefore, DLPFC tDCS is an effective intervention to immediately improve dual-task walking ability for individuals with PD.
However, it should be mentioned that the DLPFC tDCS group did not improve significantly more in single walking and TUG performance as compared with other groups, although the DLPFC tDCS group showed a pre-post significant improvement in single walking. This may indicate that the dual-task walking performance is more sensitive to reflect the response to intervention, and single walking and TUG performance may need cumulative tDCS interventions for a significant improvement. In addition, it is interesting to note that the RMT and MEP did not change significantly after DLPFC tDCS. Studies have reported that RMT is normal and MEP is variable in people with PD (Lefaucheur, 2005; Rossini et al., 2015). However, decreased SP has been reported consistently in patients with PD (Wu et al., 2007). Therefore, measurement of SP may be a better indicator to reflect cortical activity changes during disease progression and in response to treatment than RMT and MEP in individuals with PD.
Regarding the cerebellum tDCS, Workman et al. (2020) did not observe a significant single-session effect in single walking performance in people with PD. However, Jayaram et al. (2012) found that one session of anodal cerebellum tDCS resulted in better adaptation on a split-belt treadmill than Sham tDCS in healthy subjects. This study noted that the cerebellum tDCS exerted a significant within-group improvement in dual-task walking but not in single walking and TUG performance. However, such within-group improvement did not couple with significant changes in SP duration. This result lent us to speculate the vestibulocerebellum pathways, which are majorly involved in posture and balance control (Purves et al., 2001), may be modulated by cerebellum tDCS, but this warrants further exploration.
It also drew our attention that one session of anodal tDCS over M1 did not improve the walking performance, and such results were consistent with the results reported by Verheyden et al. (2013). Although Fregni et al. (2006) demonstrated that M1 tDCS improves UPDRS motor scores, limited improvement in the UPDRS gait-related items was noted. Moreover, Schabrun et al. (2016) demonstrated that M1 tDCS did not enhance the effect of dual-task gait training and suggested that M1 tDCS may not be an effective application to improve dual-task walking in individuals with PD. Considering the results of previous studies and this study, it still needs more investigations to establish the beneficial effects of tDCS over M1 on walking performance in people with PD.
Some limitations should be mentioned regarding this study. First, the relatively small number of participants in each group may lead to type II error. The limited sample size and heterogeneity of patients with PD must be considered when generalizing the study results. Second, the included participants were with mild to moderate disease severity (Hoehn and Yahr stages I–III), and the outcomes were measured only at “on” status. Therefore, our findings may only be applicable to individuals with mild to moderate PD at “on” status. Third, we only investigated the post-intervention effects of single-session tDCS, but the maintenance effects or accumulative effects of tDCS are not known. Fourth, we applied 2 mA for all targets because the current with 2 mA was most commonly used in the previous studies, especially in the studies focusing on walking ability (Ferrucci et al., 2015; Liu et al., 2021). However, the best parameter for tDCS in different targets may be different. Therefore, the results may not represent the best effect of tDCS in these targets. Further studies may need to establish the best stimulating intensity of tDCS in various targets. Finally, it has been noted that a single session of DLPFC tDCS could improve cognitive function according to the results of a meta-analysis (Dedoncker et al., 2016). However, we did not measure cognitive performances in this study. Therefore, the cognitive improvement cannot be excluded from the beneficial effects of DLPFC tDCS.
Conclusion
The results suggest that one session of DLPFC tDCS can be recommended to improve dual-task walking. Further research is needed to explore the effects of multisessions of DLPFC tDCS.
Data Availability Statement
The raw data supporting the conclusions of this article will be made available by the authors, without undue reservation.
Ethics Statement
The studies involving human participants were reviewed and approved by Institutional Review Board of Taipei Veterans General Hospital. The patients/participants provided their written informed consent to participate in this study.
Author Contributions
P-LW and R-YW conceived and designed the experiments. P-LW performed the experiments. P-LW and Y-RY analyzed the data. J-LF and H-LC confirmed the medical diagnosis of subjects and recruited subjects. P-LW, Y-RY, and S-FH interpreted the data and prepared the manuscript. All authors approved the final manuscript for submission.
Funding
This work was supported by grants from the Ministry of Science and Technology, Taiwan (MOST-106-2314-B-010-037-MY3), and the National Health Research Institutes, Taiwan (NHRI-EX110-10913PI).
Conflict of Interest
The authors declare that the research was conducted in the absence of any commercial or financial relationships that could be construed as a potential conflict of interest.
Publisher’s Note
All claims expressed in this article are solely those of the authors and do not necessarily represent those of their affiliated organizations, or those of the publisher, the editors and the reviewers. Any product that may be evaluated in this article, or claim that may be made by its manufacturer, is not guaranteed or endorsed by the publisher.
Acknowledgments
We would like to thank the study participants.
Abbreviations
BG, basal ganglia; CV, coefficients of variation; DLPFC, dorsal lateral prefrontal cortex; DTC, dual-task cost; EMG, electromyography; M1, primary motor cortex; MEP, motor evoked potential; MMSE, Mini-Mental State Examination; PD, Parkinson’s disease; RMT, resting motor threshold; SP, silent period; TA, tibialis anterior; tDCS, transcranial direct current stimulation; TMS, transcranial magnetic stimulation; TUG, timed up and go test; UPDRS, Unified Parkinson’s Disease Rating Scale.
References
Al-Yahya, E., Mahmoud, W., Meester, D., Esser, P., and Dawes, H. (2019). Neural substrates of cognitive motor interference during walking; Peripheral and Central Mechanisms. Front. Hum. Neurosci. 12:536. doi: 10.3389/fnhum.2018.00536
Benecke, R., Rothwell, J. C., Dick, J. P., Day, B. L., and Marsden, C. D. (1986). Performance of simultaneous movements in patients with Parkinson’s disease. Brain 109, 739–757. doi: 10.1093/brain/109.4.739
Beurskens, R., Helmich, I., Rein, R., and Bock, O. (2014). Age-related changes in prefrontal activity during walking in dual-task situations: a fNIRS study. Int. J. Psychophysiol. 92, 122–128. doi: 10.1016/j.ijpsycho.2014.03.005
Bunai, T., Hirosawa, T., Kikuchi, M., Fukai, M., Yokokura, M., Ito, S., et al. (2021). tDCS-induced modulation of GABA concentration and dopamine release in the human brain: a combination study of magnetic resonance spectroscopy and positron emission tomography. Brain Stimul. 14, 154–160. doi: 10.1016/j.brs.2020.12.010
Collette, F., Olivier, L., Van der Linden, M., Laureys, S., Delfiore, G., Luxen, A., et al. (2005). Involvement of both prefrontal and inferior parietal cortex in dual-task performance. Brain Res Cogn Brain Res. 24, 237–51. doi: 10.1016/j.cogbrainres.2005.01.023
Dedoncker, J., Brunoni, A. R., Baeken, C., and Vanderhasselt, M. A. (2016). A Systematic Review and Meta-Analysis of the Effects of Transcranial Direct Current Stimulation (tDCS) Over the Dorsolateral Prefrontal Cortex in Healthy and Neuropsychiatric Samples: influence of Stimulation Parameters. Brain Stimul. 9, 501–517. doi: 10.1016/j.brs.2016.04.006
Ferrucci, R., Cortese, F., Bianchi, M., Pittera, D., Turrone, R., Bocci, T., et al. (2016). Cerebellar and motor cortical transcranial stimulation decrease levodopa-induced dyskinesias in Parkinson’s disease. Cerebellum 15, 43–47. doi: 10.1007/s12311-015-0737-x
Ferrucci, R., Cortese, F., and Priori, A. (2015). Cerebellar tDCS: how to do it. Cerebellum 14, 27–30. doi: 10.1007/s12311-014-0599-7
Fisher, B. E., Wu, A. D., Salem, G. J., Song, J., Lin, C. H., Yip, J., et al. (2008). The effect of exercise training in improving motor performance and corticomotor excitability in people with early Parkinson’s disease. Arch. Phys. Med. Rehabil. 89, 1221–1229. doi: 10.1016/j.apmr.2008.01.013
Fonteneau, C., Redoute, J., Haesebaert, F., Le Bars, D., Costes, N., Suaud-Chagny, M. F., et al. (2018). Frontal transcranial direct current stimulation induces dopamine release in the ventral striatum in human. Cereb. Cortex. 28, 2636–2646. doi: 10.1093/cercor/bhy093
Fregni, F., Boggio, P. S., Santos, M. C., Lima, M., Vieira, A. L., Rigonatti, S. P., et al. (2006). Noninvasive cortical stimulation with transcranial direct current stimulation in Parkinson’s disease. Mov. Disord. 21, 1693–1702. doi: 10.1002/mds.21012
Fukai, M., Bunai, T., Hirosawa, T., Kikuchi, M., Ito, S., Minabe, Y., et al. (2019). Endogenous dopamine release under transcranial direct-current stimulation governs enhanced attention: a study with positron emission tomography. Transl Psychiatry. 9:115. doi: 10.1038/s41398-019-0443-4
Gaspar, P., Duyckaerts, C., Alvarez, C., Javoy-Agid, F., and Berger, B. (1991). Alterations of dopaminergic and noradrenergic innervations in motor cortex in Parkinson’s disease. Ann. Neurol. 30, 365–374. doi: 10.1002/ana.410300308
Groppa, S., Oliviero, A., Eisen, A., Quartarone, A., Cohen, L. G., Mall, V., et al. (2012). A practical guide to diagnostic transcranial magnetic stimulation: report of an IFCN committee. Clin. Neurophysiol. 123, 858–882. doi: 10.1016/j.clinph.2012.01.010
Hausdorff, J. M., Cudkowicz, M. E., Firtion, R., Wei, J. Y., and Goldberger, A. L. (1998). Gait variability and basal ganglia disorders: stride-to-stride variations of gait cycle timing in Parkinson’s disease and Huntington’s disease. Mov. Disord. 13, 428–437. doi: 10.1002/mds.870130310
Ilg, W., and Timmann, D. (2013). Gait ataxia–specific cerebellar influences and their rehabilitation. Mov. Disord. 15, 1566–1575. doi: 10.1002/mds.25558
Jayaram, G., Tang, B., Pallegadda, R., Vasudevan, E. V., Celnik, P., and Bastian, A. (2012). Modulating locomotor adaptation with cerebellar stimulation. J. Neurophysiol. 107, 2950–2957. doi: 10.1152/jn.00645.2011
Kapogiannis, D., and Wassermann, E. M. (2008). Transcranial magnetic stimulation in Clinical Pharmacology. Cent. Nerv. Syst. Agents Med. Chem. 8, 234–240. doi: 10.2174/187152408786848076
Kaski, D., Dominguez, R. O., Allum, J. H., Islam, A. F., and Bronstein, A. M. (2014). Combining physical training with transcranial direct current stimulation to improve gait in Parkinson’s disease: a pilot randomized controlled study. Clin. Rehabil. 28, 1115–1124. doi: 10.1177/0269215514534277
Kelly, V. E., Eusterbrock, A. J., and Shumway-Cook, A. (2012). A review of dual-task walking deficits in people with Parkinson’s disease: motor and cognitive contributions, mechanisms, and clinical implications. Parkinsons Dis. 2012:918719. doi: 10.1155/2012/918719
Kishore, A., Meunier, S., and Popa, T. (2014). Cerebellar influence on motor cortex plasticity: behavioral implications for Parkinson’s disease. Front. Neurol. 5:68. doi: 10.3389/fneur.2014.00068
Lattari, E., Costa, S. S., Campos, C., de Oliveira, A. J., Machado, S., and Maranhao Neto, G. A. (2017). Can transcranial direct current stimulation on the dorsolateral prefrontal cortex improves balance and functional mobility in Parkinson’s disease? Neurosci Lett. 636, 165–169. doi: 10.1016/j.neulet.2016.11.019
Lees, A. J., Hardy, J., and Revesz, T. (2009). Parkinson’s disease. Lancet 373, 2055–2066. doi: 10.1016/S0140-6736(09)60492-X
Lefaucheur, J. P. (2005). Motor cortex dysfunction revealed by cortical excitability studies in Parkinson’s disease: influence of antiparkinsonian treatment and cortical stimulation. Clin. Neurophysiol. 116, 244–253. doi: 10.1016/j.clinph.2004.11.017
Liu, X., Liu, H., Liu, Z., Rao, J., Wang, J., Wang, P., et al. (2021). Transcranial Direct Current Stimulation for Parkinson’s Disease: a Systematic Review and Meta-Analysis. Front. Aging Neurosci. 28:746797. doi: 10.3389/fnagi.2021.746797
Lu, C. F., Liu, Y. C., Yang, Y. R., Wu, Y. T., and Wang, R. Y. (2015). Maintaining Gait Performance by Cortical Activation during Dual-Task Interference: a Functional Near-Infrared Spectroscopy Study. PLoS One 10:e0129390. doi: 10.1371/journal.pone.0129390
Manenti, R., Brambilla, M., Rosini, S., Orizio, I., Ferrari, C., Borroni, B., et al. (2014). Time up and go task performance improves after transcranial direct current stimulation in patient affected by Parkinson’s disease. Neurosci Lett. 580, 74–77. doi: 10.1016/j.neulet.2014.07.052
Mishra, R. K., and Thrasher, A. T. (2021). Transcranial direct current stimulation of dorsolateral prefrontal cortex improves dual-task gait performance in patients with Parkinson’s disease: a double blind, sham-controlled study. Gait Posture 84, 11–16. doi: 10.1016/j.gaitpost.2020.11.012
Morris, S., Morris, M. E., and Iansek, R. (2001). Reliability of measurements obtained with the Timed “Up & Go” test in people with Parkinson disease. Phys. Ther. 81, 810–818. doi: 10.1093/ptj/81.2.810
Nakashima, K., Wang, Y., Shimoda, M., Sakuma, K., and Takahashi, K. (1995). Shortened silent period produced by magnetic cortical stimulation in patients with Parkinson’s disease. J. Neurol. Sci. 130, 209–214. doi: 10.1016/0022-510x(95)00029-2
Nieuwhof, F., Reelick, M. F., Maidan, I., Mirelman, A., Hausdorff, J. M., Olde Rikkert, M. G., et al. (2016). Measuring prefrontal cortical activity during dual task walking in patients with Parkinson’s disease: feasibility of using a new portable fNIRS device. Pilot Feasibility Stud. 2:59. doi: 10.1186/s40814-016-0099-2
Paulus, W., Classen, J., Cohen, L. G., Large, C. H., Di Lazzaro, V., Nitsche, M., et al. (2008). State of the art: pharmacologic effects on cortical excitability measures tested by transcranial magnetic stimulation. Brain Stimul. 1, 151–163. doi: 10.1016/j.brs.2008.06.002
Polanía, R., Paulus, W., and Nitsche, M. A. (2012). Modulating cortico-striatal and thalamo-cortical functional connectivity with transcranial direct current stimulation. Hum. Brain Mapp. 33, 2499–2508. doi: 10.1002/hbm.21380
Potvin-Desrochers, A., and Paquette, C. (2021). Potential Non-invasive Brain Stimulation Targets to Alleviate Freezing of Gait in Parkinson’s Disease. Neuroscience 468, 366–376. doi: 10.1016/j.neuroscience.2021.05.037
Purves, D., Augustine, G. J., Fitzpatrick, D., Hall, W. C., LaMantia, A. S., McNamara, J. O., et al. (2001). Neuroscience. second ed. United States: Sinauer Associates.
Raffegeau, T. E., Krehbiel, L. M., Kang, N., Thijs, F. J., Altmann, L. J. P., Cauraugh, J. H., et al. (2019). A meta-analysis: Parkinson’s disease and dual-task walking. Parkinson. Relat. Disord. 62, 28–35. doi: 10.1016/j.parkreldis.2018.12.012
Rossini, P. M., Burke, D., Chen, R., Cohen, L. G., Daskalakis, Z., Di Iorio, R., et al. (2015). Non-invasive electrical and magnetic stimulation of the brain, spinal cord, roots and peripheral nerves: basic principles and procedures for routine clinical and research application. An updated report from an I.F.C.N. Committee. Clin. Neurophysiol. 126, 1071–1107. doi: 10.1016/j.clinph.2015.02.001
Sánchez-Kuhn, A., Pérez-Fernández, C., Cánovas, R., Flores, P., and Sánchez-Santed, F. (2017). Transcranial direct current stimulation as a motor neurorehabilitation tool: an empirical review. Biomed. Eng. Online 16:76. doi: 10.1186/s12938-017-0361-8
Schabrun, S. M., Lamont, R. M., and Brauer, S. G. (2016). Transcranial direct current stimulation to enhance dual-task gait training in Parkinson’s disease: a pilot RCT. PLoS One 11:e0158497. doi: 10.1371/journal.pone.0158497
Shine, J. M., Matar, E., Ward, P. B., Frank, M. J., Moustafa, A. A., Pearson, M., et al. (2013). Freezing of gait in Parkinson’s disease is associated with functional decoupling between the cognitive control network and the basal ganglia. Brain 136, 3671–3681. doi: 10.1093/brain/awt272
Strouwen, C., Molenaar, E. A., Münks, L., Keus, S. H., Bloem, B. R., Rochester, L., et al. (2015). Dual tasking in Parkinson’s disease: should we train hazardous behavior? Expert Rev. Neurother. 15, 1031–1039. doi: 10.1586/14737175.2015.1077116
Verheyden, G., Purdey, J., Burnett, M., Cole, J., and Ashburn, A. (2013). Immediate effect of transcranial direct current stimulation on postural stability and functional mobility in Parkinson’s disease. Mov. Disord. 28, 2040–2041. doi: 10.1002/mds.25640
Werhahn, K. J., Kunesch, E., Noachtar, S., Benecke, R., and Classen, J. (1999). Differential effects on motorcortical inhibition induced by blockade of GABA uptake in humans. J. Physiol. 517, 591–597. doi: 10.1111/j.1469-7793.1999.0591t.x
Workman, C. D., Fietsam, A. C., Uc, E. Y., and Rudroff, T. (2020). Cerebellar transcranial direct current stimulation in people with Parkinson’s disease: a pilot study. Brain Sci. 10, 96. doi: 10.3390/brainsci10020096
Wu, A. D., Petzinger, G. M., Lin, C. H., Kung, M., and Fisher, B. (2007). Asymmetric corticomotor excitability correlations in early Parkinson’s disease. Mov Disord. 22, 1587–1593. doi: 10.1002/mds.21565
Wu, T., and Hallett, M. (2005). A functional MRI study of automatic movements in patients with Parkinson’s disease. Brain 128, 2250–2259. doi: 10.1093/brain/awh569
Yang, Y. R., Cheng, S. J., Lee, Y. J., Liu, Y. C., and Wang, R. Y. (2019). Cognitive and motor dual task gait training exerted specific training effects on dual task gait performance in individuals with Parkinson’s disease: a randomized controlled pilot study. PLoS One 14:e0218180. doi: 10.1371/journal.pone.0218180
Yang, Y. R., Tseng, C. Y., Chiou, S. Y., Liao, K. K., Cheng, S. J., Lai, K. L., et al. (2013). Combination of rTMS and treadmill training modulates corticomotor inhibition and improves walking in Parkinson disease: a randomized trial. Neurorehabil. Neural. Repair. 27, 79–86.
Yuan, P., and Raz, N. (2014). Prefrontal cortex and executive functions in healthy adults: a meta-analysis of structural neuroimaging studies. Neurosci. Biobehav. Rev. 42, 180–192. doi: 10.1016/j.neubiorev.2014.02.005
Keywords: tDCS, different targets, single-session effects, dual-task gait, cortical activity, Parkinson’s disease
Citation: Wong P-L, Yang Y-R, Huang S-F, Fuh J-L, Chiang H-L and Wang R-Y (2022) Transcranial Direct Current Stimulation on Different Targets to Modulate Cortical Activity and Dual-Task Walking in Individuals With Parkinson’s Disease: A Double Blinded Randomized Controlled Trial. Front. Aging Neurosci. 14:807151. doi: 10.3389/fnagi.2022.807151
Received: 01 November 2021; Accepted: 10 January 2022;
Published: 07 February 2022.
Edited by:
Junhong Zhou, Harvard Medical School, United StatesReviewed by:
Dongning Su, Capital Medical University, ChinaOn-Yee Lo, Hinda and Arthur Marcus Institute for Aging Research and Harvard Medical School, United States
Copyright © 2022 Wong, Yang, Huang, Fuh, Chiang and Wang. This is an open-access article distributed under the terms of the Creative Commons Attribution License (CC BY). The use, distribution or reproduction in other forums is permitted, provided the original author(s) and the copyright owner(s) are credited and that the original publication in this journal is cited, in accordance with accepted academic practice. No use, distribution or reproduction is permitted which does not comply with these terms.
*Correspondence: Ray-Yau Wang, cnl3YW5nQG55Y3UuZWR1LnR3; orcid.org/0000-0002-8738-796X