Chemokines in patients with Alzheimer's disease: A meta-analysis
- 1School of Life and Pharmaceutical Sciences, Dalian University of Technology, Panjin, China
- 2Department of Cardiovascular Diseases, Civil Aviation General Hospital, Peking University, Beijing, China
Background: Alzheimer's disease (AD) is the most common neurodegenerative disease in elderly people. Many researches have reported that neuroinflammation is related to AD. Chemokines are a class of small cytokines that play important roles in cell migration and cell communication, which involved in neuroinflammation. Up to now there is no meta-analysis to explore the difference of chemokines between AD patients and healthy elderly individuals.
Method: We searched PubMed, Web of science, Cochrane library, EMBASE and Scopus databases from inception to January 2022. Data were extracted by two independent reviewers, and the Review Manager 5.3 was used for the meta-analysis.
Result: Thirty-two articles were included and analyzed. The total number of participants in the included study was 3,331. We found that the levels of CCL5 (SMD = 2.56, 95% CI: 1.91–3.21), CCL15 (SMD = 3.30, 95% CI: 1.48–5.13) and IP-10 (SMD = 3.88, 95% CI: 1.84–5.91) in the plasma of AD patients were higher than healthy people. MCP-1 protein (SMD = 0.67, 95% CI: 0.29–1.05) in the AD patients' CSF was higher than healthy controls.
Conclusion: These results suggested that chemokines may play an important role in AD. These findings could provide evidences for the diagnosis and treatment of AD.
Systematic review registration: https://www.crd.york.ac.uk/prospero/display_record.php?ID=CRD42021278736, identifier: CRD42021278736.
1. Introduction
It is estimated that more than 45 million people worldwide suffer from dementia, and the incidence is expected to triple by 2050 as the life expectancy increasing. Up to now, Alzheimer's disease (AD) is the most common type of dementia, accounting for almost 80% of dementia (Crous-Bou et al., 2017). In clinical practice, AD is mainly diagnosed by testing biomarkers including amyloid β-protein (Aβ) and phosphorylated tau (P-tau) (Scheltens et al., 2021). The detection of biomarkers in AD patients is mainly in the plasma and cerebrospinal fluid (CSF). Aβ1 − 42, total tau (T-tau), and p-tau in CSF are the keys to reflect AD pathophysiology (Blennow and Zetterberg, 2018). However, the pathological mechanism of AD is still not clear up to now. Therefore, the discovery of the new biomarker is important to the accurate diagnosis and treatment of AD. Recently, it has been shown that neuroinflammation play an important role in AD and is associated with high levels of inflammatory cytokines (Calsolaro and Edison, 2016; Ramirez et al., 2017; Weng et al., 2022). The CSF and plasma inflammatory cytokine levels in AD patients have attracted great interest in recent years (Chen et al., 2018).
Chemokines are low molecular weight chemotactic cytokines secreted by cells which are involved in neuroinflammation, and related to AD (Vilgelm and Richmond, 2019). They are divided into four families based on structure: CXC, CC, CX3C, and C chemokines. Chemokines exert intracellular signaling by binding to seven-transmembrane G protein-coupled receptors (Griffith et al., 2014; Nagarsheth et al., 2017). Most of the research on chemokines is related to cancer. In recent years, it has been found that chemokines are also involved in neuroinflammation, neuroendocrine, brain development, and thus play functional roles in the central nervous system (Reaux-Le Goazigo et al., 2013; Ghosh et al., 2021). In AD, chemokines play an important role in the development of Aβ plaques and neurofibrillary tangles (Martin and Delarasse, 2018). For example, chemokines and their receptors can promote AD pathology by inducing the production of Aβ (such as CXCR2 and CCR3), and also reduce the deposition of Aβ (such as CX3CR1, CXCR3, and CCR2), respectively. In addition, some chemokines (e.g., MCP−1) and receptors are also involved in tau phosphorylation (e.g., CX3CR1 and CCR3) (El Khoury et al., 2007; Bhaskar et al., 2010; Bakshi et al., 2011; Krauthausen et al., 2015; Zhu et al., 2017; Joly-Amado et al., 2020). These studies have shown that chemokines are closely related to AD. Chemokine levels in AD patients can be detected in the plasma and CSF. However, up to now there is no meta-analysis on chemokines between AD patients and healthy individuals.
In the present work, we aimed to investigate the changes of chemokine between AD patients and healthy controls. We reviewed the published articles, and analyzed the content of chemokines in the plasma and CSF of AD patients and healthy controls. Through the meta-analysis, we found that IP-10, CCL5 and CCL15 of the AD patients' plasma, and MCP-1 in the AD patients' CSF were higher than those of the control group. It could provide the potential biomarkers for AD diagnosis and treatment.
2. Methods
2.1. Search strategy
We searched PubMed (1995 to January 2022), EMBASE (1995 to January 2022), Web of science (1995 to January 2022), Scopus (1995 to January 2022), and Cochrane Library (1995 to January 2022) with the terms or medical subject terms (MeSH) of “Alzheimer's disease” and “Chemokines.” In order to avoid missing relevant studies from the database, all search results have been included.
2.2. Inclusion and exclusion criteria
The identified articles were reviewed carefully in order to qualify for this meta-analysis. All included trials met the following criteria: (1) articles were written in English; (2) participants in the studies were middle-aged and elderly people; (3) according to the fourth edition of the Mental Disorders Diagnostics and Statistics Manual (DSM-IV) and the National Association of Nervous and Communicative Disorders and Stroke/Alzheimer's Disease Institute of Standards (NINCDSADRDA), patients with AD and suspected AD were included (McKhann et al., 1984; Zulauf Logoz, 2014); (4) Studies had been published online by January 2022.
The criteria for the excluded studies were as follows: (1) systematic review or meta-analysis; (2) duplicate study; (3) animal or cell experiment; (4) review or letter; (5) without the full-text; (6) the samples were from brain tissues; (7) there were drug interventions in the process of the clinical trials.
2.3. Date extraction
Articles were screened according to inclusion and exclusion criteria. The following data were extracted from each article: first author name, country, publication year, number, gender, age, chemokines, and baseline MMSE. Meanwhile, we assessed the quality of included studies using the Newcastle-Ottawa Quality Assessment Scale (NOS) (Stang, 2010). Due to the inability of all chemokines involved in the current studies, we only analyzed the chemokines which are involved in the included studies in the present work.
2.4. Statistical analysis
This meta-analysis was performed using the Review Manager 5.3 software. We separated the sample sources into blood samples and CSF. The content of each chemokine was expressed in the form of mean ± standard deviation (SD). The correlation between chemokine content and AD was assessed by standardized mean difference (SMD) with 95% confidence interval (CI). Heterogeneity was assessed by using I2 tests and Q tests (Knopman et al., 2001; Higgins and Thompson, 2002; Zintzaras and Ioannidis, 2005). When the heterogeneity is large (I2 ≥ 50%, Q-test: p < 0.1), the random effect model is selected. Otherwise, the fixed effects model is selected (I2 ≤ 50%, Q-test: p > 0.1). In addition, publication bias was verified by funnel plots. When the funnel plot showed a symmetrical inverted triangle, the included articles were considered free of publication bias.
3. Results
3.1. Literature search findings
Five thousand six hundred sixty-eight studies were selected by the literature search, of which 57 papers were considered likely to meet the inclusion criteria after reviewing titles and abstracts. After further reading the full article, 32 studies were finally included in the meta-analysis (Figure 1). These studies were published from 2006 to 2020. The total number of participants in the included study was 3,331. In all studies, the control group referred to healthy individuals and the experimental group referred to AD patients.
3.2. Characteristics of the eligible studies
Characteristics of the included studies are shown in Table 1. Depending on the source of chemokines in the included studies, following analyses were also performed in both blood and CSF fractions. Seventeen studies detected chemokines from CSF and 23 studies detected chemokines from blood samples. In addition, there are eight articles detected chemokines in CSF and blood samples at the same time. The quality assessment was based on the Newcastle-Ottawa scale. NOS scores were shown in Table 1, and the quality scores of the included studies were all above five points.
3.3. Chemokines in the plasma
This meta-analysis was performed by Review Manager 5.3 software. In the 32 included studies, there were 23 studies including the data about blood samples. For blood samples, a total of 8 chemokines were analyzed. MCP-1, IL-8, MIP-1α, IP-10, MIP-1β, SDF-1α, CCL5, and CCL15 were included in the meta-analysis, respectively. Our meta-analysis showed that MCP-1 protein level (SMD = 0.39, 95% CI: −0.14 to 0.93), IL-8 protein level (SMD = 0.04, 95% CI: −0.59 to 0.66) and MIP-1α protein level (SMD = 1.82, 95% CI: −0.31 to 3.96) were not significantly different in blood samples between AD patients and healthy individuals (Figures 2A–C, respectively). The levels of IP-10 protein (SMD = 3.88, 95% CI: 1.84–5.91) were higher significantly in blood samples from AD patients than in those from healthy individuals (Figure 2D). Funnel plots were performed to detect the potential publication bias for meta-analysis of MCP-1, IL-8, MIP-1α and IP-10 protein level. The forest plots showed that the included studies for each chemokine analysis were distributed symmetrically, with less publication bias. Beyond that, we also analyzed the protein levels of MIP-1β, SDF-1α, CCL5, and CCL15 in the blood of AD patients and healthy people. We found that the levels of CCL5 (SMD = 2.56, 95% CI: 1.91–3.21) and CCL15 (SMD = 3.30, 95% CI: 1.48–5.13) in the plasma of AD patients were higher than those of healthy people, while the levels of MIP-1β (SMD = 0.32, 95% CI: −0.50 to 0.57) and SDF-1α (SMD = 0.03, 95% CI: −1.56 to 1.62) showed no significant difference (Table 2).
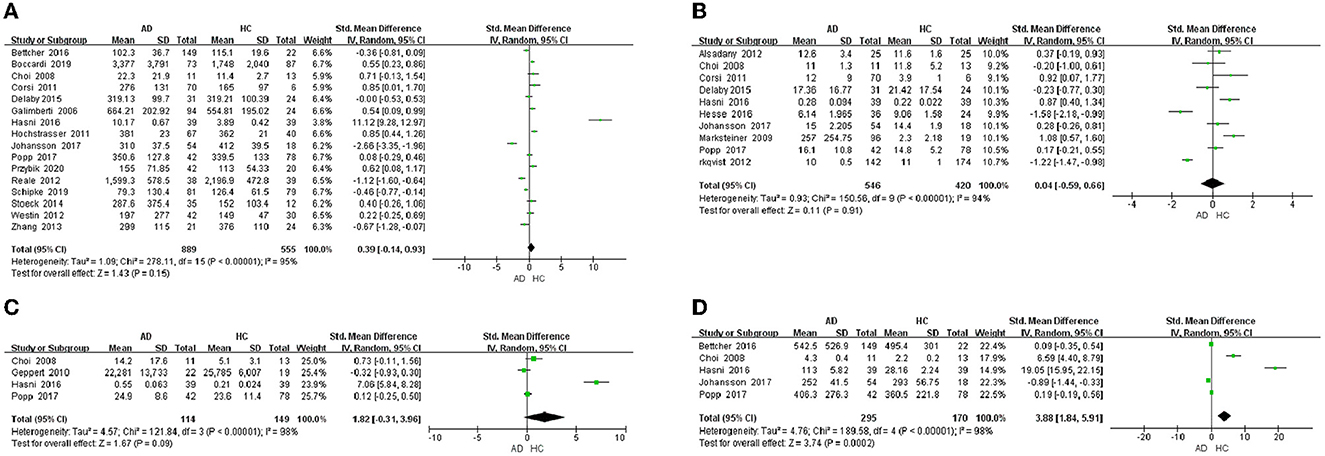
Figure 2. Chemokine levels in the plasma of AD patients. (A) MCP-1 protein content, (B) IL-8 protein content, (C) MIP-1α protein content, and (D) IP-10 protein content.
3.4. Chemokines in the CSF
In the 32 included studies, 17 studies were about the chemokines of CSF. In CSF samples, a total of 5 chemokines were analyzed. MCP-1, IL-8, IP-10, MIP-1β, and CX3CL1 were included in the meta-analysis, respectively. The outcomes showed that IP-10 protein level (SMD = −0.19, 95% CI: −0.63 to 0.24) and IL-8 protein level (SMD = 0.61, 95% CI: −0.26 to 1.48) were not significantly different in CSF samples from AD patients and healthy individuals (Figures 3A, B). And then, we found that the level of MCP-1 protein (SMD = 0.67, 95% CI: 0.29–1.05) in AD patients was higher than that in healthy controls (Figure 3C). Meanwhile, we analyzed the protein level of MIP-1β and CX3CL1. The outcomes displayed that MIP-1β (SMD = 0.92, 95% CI: −0.82 to 6.25) and CX3CL1 (SMD = −0.61, 95% CI: −2.11 to 0.89) showed no significant difference between AD patients and healthy controls (Table 3).
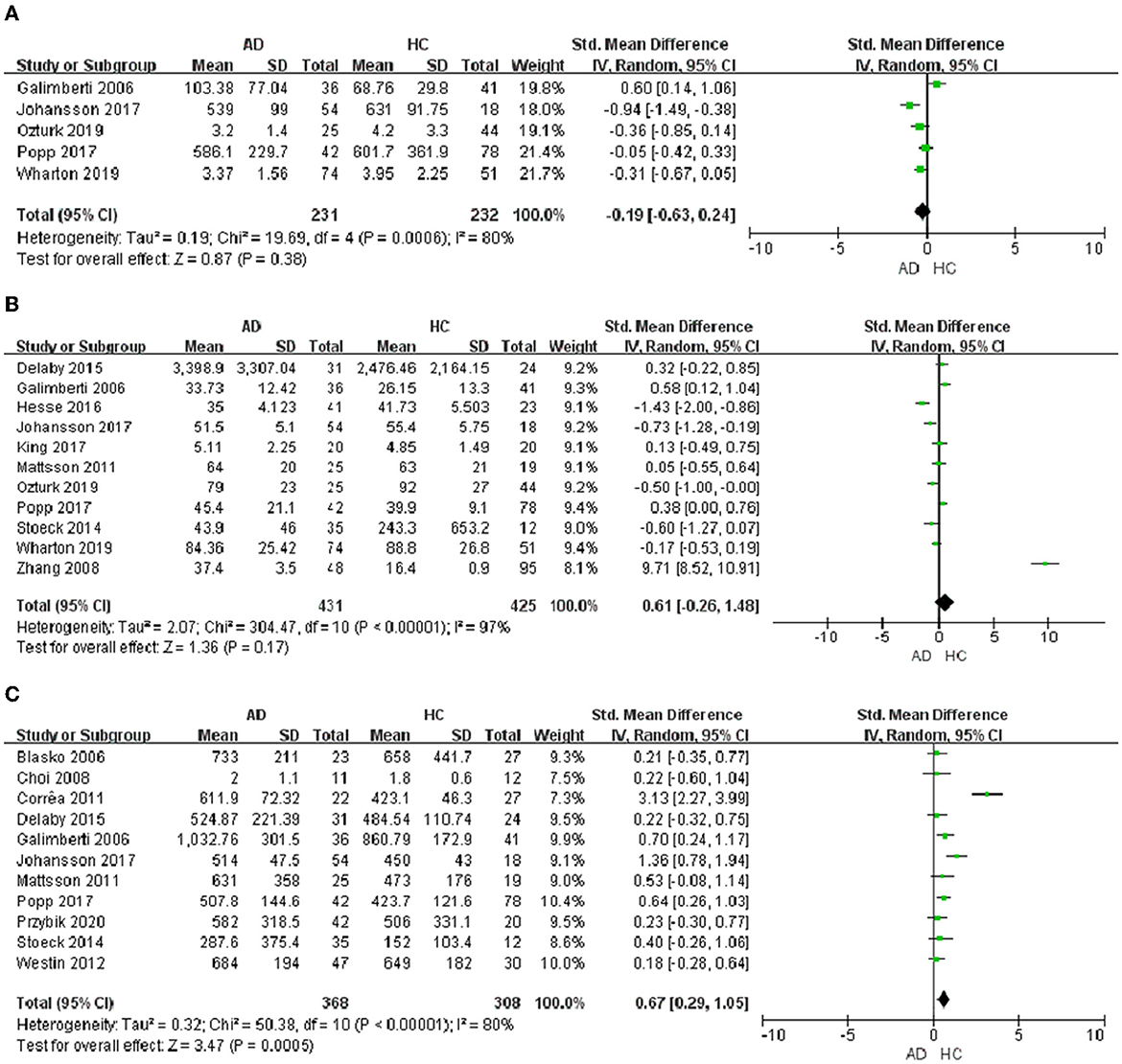
Figure 3. Chemokine levels in the CSF of AD patients. (A) IP-10 protein content, (B) IL-8 protein content, and (C) MCP-1 protein content.
Finally, all the SMD of chemokines between AD patients and healthy controls, and their 95% CIs were showed in the Figure 4.
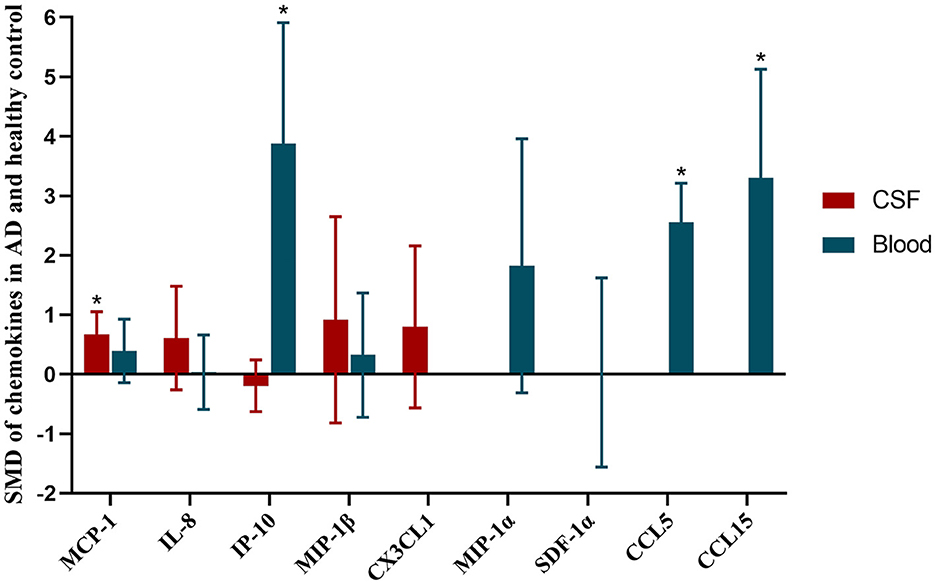
Figure 4. SMD of chemokines in AD and healthy control. Asterisks indicate significant significance between groups. Red histogram shows SMD between AD and healthy controls in CSF samples. Blue histogram shows SMD between AD and healthy controls in blood samples.
4. Discussion
To our knowledge, this is the first systematic review and meta-analysis to summarize the chemokines in the plasma and CSF between AD patients and healthy individuals. The chemokine system is involved in the brain microenvironment of AD patients (Azizi et al., 2014). Chemokines are important regulators of both the central and peripheral immune response, and play an important role in inflammatory processes of the brain. The chemokines in AD patients' plasma and CSF change significantly compared to healthy controls, which may be related to AD (Liu C. et al., 2014). Many studies have found that the chemokines regulate the infiltration of peripheral mononuclear cells into the AD brain, and/or the accumulation of Aβ deposition in microglia cells, and involved in tau phosphorylation (Zhu et al., 2017; Guedes et al., 2018). Therefore, chemokines and their receptors were involved in the occurrence and progression of AD (Liu C. et al., 2014; Wojcieszak et al., 2022). These results combined with the present work suggest that the chemokines may play an important role in AD.
In this meta-analysis, we detected higher protein concentrations of CCL5, IP-10, and CCL15 in the plasma of AD patients than in that of healthy people. Among them, the previous studies have shown that the expression of CCL5 is increased in the brain microenvironment circulation of AD patients (Chen et al., 2004; Sharma et al., 2007; Lumpkins et al., 2008; Tripathy et al., 2010). Li et al. (2023) have reported that the CCL5 gene is increased in the brain of AD patients and AD mice. In addition, soluble CCL5 could be activated by Aβ, which is related to AD. CCL5 was enhanced with Mn exposure inducing neuroinflammation which contributes to AD (Kirkley et al., 2017). As the receptor of CCL5, the expression of CCR5 is increased on some reactive microglia in AD patients compared to healthy controls (Xia et al., 1998). Furthermore, the IP-10 levels in the plasma of AD patients was up-regulated in the present work. However, IP-10 concentrations of plasma correlating positively with AD was controversial in the previous studies and other meta-analysis (Lai et al., 2017; Su et al., 2019). It was found that IP-10 which was co-localized with Aβ plaques was increased in aged brain of AD mice (Duan et al., 2008; Guedes et al., 2018). As the pro-inflammatory chemokine, IP-10 was up-regulated when mice were injected with Aβ (Lai et al., 2013). Besides, in the plasma, higher level of IP-10 were associated with the increased levels of CSF t-tau and p-tau (Bettcher et al., 2018). Therefore, the changes of IP-10 protein levels may reflect the progression of AD. In clinical trials, compared with the control group, the protein level of CCL15 in monocytes of AD patients was down-regulated significantly (Hochstrasser et al., 2011). As a potential target of AD, neurotrophin-3 has aroused the interest of AD researchers. Meanwhile some studies have found that CCL15 appears in a meta feature with neurotrophin-3 (Rocha De Paula et al., 2011). Therefore, we speculated that CCL15 might be related to AD. In the previous studies, it was reported that CCL15, CCL5, and IP-10 were involved in neuroinflammation (Ubogu et al., 2006; Hochstrasser et al., 2011; Pan et al., 2022). In the collection of clinical samples, blood sample is more readily obtained compared to CSF. The levels of IP-10, CCL5 and CCL15 which have been found to be significantly up-regulated in the plasma of AD patients may be the potential biomarkers for the clinical diagnosis of AD.
In the present work, the performance of the chemokines in the periphery was not the same as in CSF. One of the reasons is heterogeneity of the population and the different methods used in the research. In addition, cerebrospinal fluid is a colorless and transparent fluid existing in the ventricles and subarachnoid space. Owing to the blood-brain barrier, the content and the concentration of the proteins in the blood are quite different from that in the CSF (Stolp and Dziegielewska, 2009). The detection of biomarkers (e.g., Aβ levels) in CSF is important in the diagnosis and treatment of neurodegenerative diseases. For example, detection of Aβ1 − 42 and p-tau in CSF is a well-established method in AD diagnosis (Iwatsubo et al., 1994; Motter et al., 1995; Lleo et al., 2015; Olsson et al., 2016). Many studies have shown that chemokines in CSF could impact on tau hyperphosphorylation and the level of Aβ. In our study, it was found that the contents of MCP-1 in the CSF of AD patients was higher than that of healthy controls. MCP-1 (also called CCL2), a chemokine belonging to the CC family, is one of the chemokines for regulating monocyte/macrophage migration and infiltration (Sozzani et al., 1994). In recent years, many studies have found that MCP-1 play important roles in inflammation and related to AD (Chen et al., 2016; Wojcieszak et al., 2022). MCP-1 was expressed by microglia. It was found that MCP-1 could facilitate Aβ oligomer formation and induce neuronal loss by binding to CC-chemokine receptor 2 in pathology and physiology of AD (Britschgi and Wyss-Coray, 2007; Kiyota et al., 2009; Conductier et al., 2010). In the animal experiment, MCP-1 could accelerate Tau pathology together with glia activation (Joly-Amado et al., 2020). MCP-1 in HP resilient cases were up-regulated in AD patients compared to healthy individuals (Barroeta-Espar et al., 2019). In addition, elevated levels of MCP-1 in AD patients have also been found in other studies and the previous meta-analysis, which are consistent with our outcomes (Galimberti et al., 2006; Lee et al., 2018; Shen et al., 2019). CX3CL1/CX3CR1 is also related to AD. CX3CL1 was increased in blood-brain barrier of AD, as a potential biomarker in the early stages of AD (Verite et al., 2018). Meanwhile, CXC3CR1 deficiency increased the Aβ level, and aggravated tau pathology, inducing cognitive decline (Puntambekar et al., 2022). However, the CX3CL1 was not significantly different between AD and healthy controls in the present work. This may be due to the results of the included studies in the meta-analysis, and the differences in methodological inclusion criteria. Therefore, we concluded that MCP-1 protein in the CSF might be the potential biomarker of AD.
Previous studies have shown that MCP-1, IL-8 are elevated in AD patients' peripheral blood compared to healthy controls, which is inconsistent with our results (Lai et al., 2017; Shen et al., 2019; Su et al., 2019). This may be due to the methodological differences in the inclusion criteria, and the results of the new studies which have been published since the other meta-analysis.
The advantage of the meta-analysis was that the present work had included all of the chemokines which are involved in the included studies, to investigate the levels of chemokine in the plasma and CSF between AD patients and healthy individuals. Some studies have shown that these chemokines and receptors play a neuroprotective role in the brain. Overexpression of CCL5 may reduce the neurotoxicity induced by the infection of HIV, which was related to p38-MAPK and PI3K/Akt pathway (Mocchetti et al., 2013; Liu X. et al., 2014; Campbell et al., 2015). As for IP-10, it was slightly neuroprotective at 10 ng/ml when the mixed or pure neuronal cultures were also exposed to NMDA (Bruno et al., 2000). MCP-1/CCR2 was involved in neuronal survival through PI3K/Akt/NF-κB pathway (Yao et al., 2009). In addition, MCP-1 could reduce the extracellular level of glutamate and the apoptotic cell death to against NMDA toxicity in vitro (Bruno et al., 2000; Eugenin et al., 2003). However, many studies have found that these chemokines are involved in AD as pro-inflammatory cytokines. Therefore, we speculated that these chemokines may be the potential inflammatory biomarkers of AD. Further studies are needed to investigate the mechanism of these chemokines in the pathological process of AD and these chemokines correlate with the established biomarkers including Aβ and tau.
There are some limitations in our meta-analysis. First, we performed a risk assessment of the included articles using Review Manager 5.3 software, and one article was at risk of “selective publication.” There may be potential publication bias in the results. And then, some chemokines involved a small amount of studies, and the results may be biased due to the small amount of data. Finally, our search is limited to literatures published in English, which may cause some selection bias.
5. Conclusion
This meta-analysis showed that the progression of AD may be associated with the elevated concentrations of chemokines. The concentrations of IP-10, CCL5, and CCL15 were significantly increased in the plasma of AD patients. In the CSF of AD patients, we found that MCP-1 protein was significantly increased. The study could provide a new insight into the diagnosis and treatment of AD.
Data availability statement
The original contributions presented in the study are included in the article/supplementary material, further inquiries can be directed to the corresponding author/s.
Author contributions
WW, YH, and HW designed the study and wrote the manuscript. HW and YZ searched the databases, extracted the data, and analyzed the data. HW and LZ conducted the quality assessment of the included studies. All authors reviewed and approved the manuscript.
Funding
The following grants supported this research: the Fundamental Research Funds for Civil Aviation General Hospital (No. 202211) and the Fundamental Research Funds for the Central Universities (Nos. DUT21RC(4)003 and DUT22YG107).
Conflict of interest
The authors declare that the research was conducted in the absence of any commercial or financial relationships that could be construed as a potential conflict of interest.
Publisher's note
All claims expressed in this article are solely those of the authors and do not necessarily represent those of their affiliated organizations, or those of the publisher, the editors and the reviewers. Any product that may be evaluated in this article, or claim that may be made by its manufacturer, is not guaranteed or endorsed by the publisher.
References
Azizi, G., Khannazer, N., and Mirshafiey, A. (2014). The potential role of chemokines in Alzheimer's disease pathogenesis. Am. J. Alzheimers. Dis. Other Demen. 29, 415–425. doi: 10.1177/1533317513518651
Bakshi, P., Margenthaler, E., Reed, J., Crawford, F., and Mullan, M. (2011). Depletion of CXCR2 inhibits gamma-secretase activity and amyloid-beta production in a murine model of Alzheimer's disease. Cytokine 53, 163–169. doi: 10.1016/j.cyto.2010.10.008
Barroeta-Espar, I., Weinstock, L. D., Perez-Nievas, B. G., Meltzer, A. C., Siao Tick Chong, M., Amaral, A. C., et al. (2019). Distinct cytokine profiles in human brains resilient to Alzheimer's pathology. Neurobiol. Dis. 121, 327–337. doi: 10.1016/j.nbd.2018.10.009
Bettcher, B. M., Johnson, S. C., Fitch, R., Casaletto, K. B., Heffernan, K. S., Asthana, S., et al. (2018). Cerebrospinal fluid and plasma levels of inflammation differentially relate to CNS markers of Alzheimer's disease pathology and neuronal damage. J. Alzheimers. Dis. 62, 385–397. doi: 10.3233/JAD-170602
Bhaskar, K., Konerth, M., Kokiko-Cochran, O. N., Cardona, A., Ransohoff, R. M., and Lamb, B. T. (2010). Regulation of tau pathology by the microglial fractalkine receptor. Neuron 68, 19–31. doi: 10.1016/j.neuron.2010.08.023
Blennow, K., and Zetterberg, H. (2018). Biomarkers for Alzheimer's disease: current status and prospects for the future. J. Intern. Med. 284, 643–663. doi: 10.1111/joim.12816
Britschgi, M., and Wyss-Coray, T. (2007). Systemic and acquired immune responses in Alzheimer's disease. Int. Rev. Neurobiol. 82, 205–233. doi: 10.1016/S0074-7742(07)82011-3
Bruno, V., Copani, A., Besong, G., Scoto, G., and Nicoletti, F. (2000). Neuroprotective activity of chemokines against N-methyl-D-aspartate or beta-amyloid-induced toxicity in culture. Eur. J. Pharmacol. 399, 117–121. doi: 10.1016/S0014-2999(00)00367-8
Calsolaro, V., and Edison, P. (2016). Neuroinflammation in Alzheimer's disease: current evidence and future directions. Alzheimers. Dement. 12, 719–732. doi: 10.1016/j.jalz.2016.02.010
Campbell, L. A., Avdoshina, V., Day, C., Lim, S. T., and Mocchetti, I. (2015). Pharmacological induction of CCL5 in vivo prevents gp120-mediated neuronal injury. Neuropharmacology 92, 98–107. doi: 10.1016/j.neuropharm.2015.01.009
Chen, C. J., Chen, J. H., Chen, S. Y., Liao, S. L., and Raung, S. L. (2004). Upregulation of RANTES gene expression in neuroglia by Japanese encephalitis virus infection. J. Virol. 78, 12107–12119. doi: 10.1128/JVI.78.22.12107-12119.2004
Chen, W. W., Zhang, X., and Huang, W. J. (2016). Role of neuroinflammation in neurodegenerative diseases (Review). Mol. Med. Rep. 13, 3391–3396. doi: 10.3892/mmr.2016.4948
Chen, X., Hu, Y., Cao, Z., Liu, Q., and Cheng, Y. (2018). Cerebrospinal fluid inflammatory cytokine aberrations in Alzheimer's disease, Parkinson's disease and amyotrophic lateral sclerosis: a systematic review and meta-analysis. Front. Immunol. 9, 2122. doi: 10.3389/fimmu.2018.02122
Conductier, G., Blondeau, N., Guyon, A., Nahon, J. L., and Rovere, C. (2010). The role of monocyte chemoattractant protein MCP1/CCL2 in neuroinflammatory diseases. J. Neuroimmunol. 224, 93–100. doi: 10.1016/j.jneuroim.2010.05.010
Crous-Bou, M., Minguillon, C., Gramunt, N., and Molinuevo, J. L. (2017). Alzheimer's disease prevention: from risk factors to early intervention. Alzheimers. Res. Ther. 9, 71. doi: 10.1186/s13195-017-0297-z
Duan, R. S., Yang, X., Chen, Z. G., Lu, M. O., Morris, C., Winblad, B., et al. (2008). Decreased fractalkine and increased IP-10 expression in aged brain of APP(swe) transgenic mice. Neurochem. Res. 33, 1085–1089. doi: 10.1007/s11064-007-9554-z
El Khoury, J., Toft, M., Hickman, S. E., Means, T. K., Terada, K., Geula, C., et al. (2007). Ccr2 deficiency impairs microglial accumulation and accelerates progression of Alzheimer-like disease. Nat. Med. 13, 432–438. doi: 10.1038/nm1555
Eugenin, E. A., D'aversa, T. G., Lopez, L., Calderon, T. M., and Berman, J. W. (2003). MCP-1 (CCL2) protects human neurons and astrocytes from NMDA or HIV-tat-induced apoptosis. J. Neurochem. 85, 1299–1311. doi: 10.1046/j.1471-4159.2003.01775.x
Galimberti, D., Fenoglio, C., Lovati, C., Venturelli, E., Guidi, I., Corra, B., et al. (2006). Serum MCP-1 levels are increased in mild cognitive impairment and mild Alzheimer's disease. Neurobiol. Aging 27, 1763–1768. doi: 10.1016/j.neurobiolaging.2005.10.007
Ghosh, P., Singh, R., Ganeshpurkar, A., Pokle, A. V., Singh, R. B., Singh, S. K., et al. (2021). Cellular and molecular influencers of neuroinflammation in Alzheimer's disease: recent concepts & roles. Neurochem. Int. 151, 105212. doi: 10.1016/j.neuint.2021.105212
Griffith, J. W., Sokol, C. L., and Luster, A. D. (2014). Chemokines and chemokine receptors: positioning cells for host defense and immunity. Annu. Rev. Immunol. 32, 659–702. doi: 10.1146/annurev-immunol-032713-120145
Guedes, J. R., Lao, T., Cardoso, A. L., and El Khoury, J. (2018). Roles of microglial and monocyte chemokines and their receptors in regulating Alzheimer's disease-associated amyloid-beta and tau pathologies. Front. Neurol. 9, 549. doi: 10.3389/fneur.2018.00549
Higgins, J. P., and Thompson, S. G. (2002). Quantifying heterogeneity in a meta-analysis. Stat. Med. 21, 1539–1558. doi: 10.1002/sim.1186
Hochstrasser, T., Marksteiner, J., Defrancesco, M., Deisenhammer, E. A., Kemmler, G., and Humpel, C. (2011). Two blood monocytic biomarkers (CCL15 and p21) combined with the mini-mental state examination discriminate Alzheimer's disease patients from healthy subjects. Dement. Geriatr. Cogn. Dis. Extra 1, 297–309. doi: 10.1159/000330468
Iwatsubo, T., Odaka, A., Suzuki, N., Mizusawa, H., Nukina, N., and Ihara, Y. (1994). Visualization of A beta 42(43) and A beta 40 in senile plaques with end-specific A beta monoclonals: evidence that an initially deposited species is A beta 42(43). Neuron 13, 45–53. doi: 10.1016/0896-6273(94)90458-8
Joly-Amado, A., Hunter, J., Quadri, Z., Zamudio, F., Rocha-Rangel, P. V., Chan, D., et al. (2020). CCL2 Overexpression in the brain promotes glial activation and accelerates tau pathology in a mouse model of tauopathy. Front. Immunol. 11, 997. doi: 10.3389/fimmu.2020.00997
Kirkley, K. S., Popichak, K. A., Afzali, M. F., Legare, M. E., and Tjalkens, R. B. (2017). Microglia amplify inflammatory activation of astrocytes in manganese neurotoxicity. J. Neuroinflammation 14, 99. doi: 10.1186/s12974-017-0871-0
Kiyota, T., Yamamoto, M., Xiong, H., Lambert, M. P., Klein, W. L., Gendelman, H. E., et al. (2009). CCL2 accelerates microglia-mediated Abeta oligomer formation and progression of neurocognitive dysfunction. PLoS ONE 4, e6197. doi: 10.1371/journal.pone.0006197
Knopman, D. S., Dekosky, S. T., Cummings, J. L., Chui, H., Corey-Bloom, J., Relkin, N., et al. (2001). Practice parameter: diagnosis of dementia (an evidence-based review). Report of the Quality Standards Subcommittee of the American Academy of Neurology. Neurology 56, 1143–1153. doi: 10.1212/WNL.56.9.1143
Krauthausen, M., Kummer, M. P., Zimmermann, J., Reyes-Irisarri, E., Terwel, D., Bulic, B., et al. (2015). CXCR3 promotes plaque formation and behavioral deficits in an Alzheimer's disease model. J. Clin. Invest. 125, 365–378. doi: 10.1172/JCI66771
Lai, K. S. P., Liu, C. S., Rau, A., Lanctot, K. L., Kohler, C. A., Pakosh, M., et al. (2017). Peripheral inflammatory markers in Alzheimer's disease: a systematic review and meta-analysis of 175 studies. J. Neurol. Neurosurg. Psychiatry 88, 876–882. doi: 10.1136/jnnp-2017-316201
Lai, W., Wu, J., Zou, X., Xie, J., Zhang, L., Zhao, X., et al. (2013). Secretome analyses of Abeta(1-42) stimulated hippocampal astrocytes reveal that CXCL10 is involved in astrocyte migration. J. Proteome Res. 12, 832–843. doi: 10.1021/pr300895r
Lee, W. J., Liao, Y. C., Wang, Y. F., Lin, I. F., Wang, S. J., and Fuh, J. L. (2018). Plasma MCP-1 and cognitive decline in patients with Alzheimer's disease and mild cognitive impairment: a two-year follow-up study. Sci. Rep. 8, 1280. doi: 10.1038/s41598-018-19807-y
Li, X., Zhang, D. F., Bi, R., Tan, L. W., Chen, X., Xu, M., et al. (2023). Convergent transcriptomic and genomic evidence supporting a dysregulation of CXCL16 and CCL5 in Alzheimer's disease. Alzheimers. Res. Ther. 15, 17. doi: 10.1186/s13195-022-01159-5
Liu, C., Cui, G., Zhu, M., Kang, X., and Guo, H. (2014). Neuroinflammation in Alzheimer's disease: chemokines produced by astrocytes and chemokine receptors. Int. J. Clin. Exp. Pathol. 7, 8342–8355.
Liu, X., Shah, A., Gangwani, M. R., Silverstein, P. S., Fu, M., and Kumar, A. (2014). HIV-1 Nef induces CCL5 production in astrocytes through p38-MAPK and PI3K/Akt pathway and utilizes NF-kB, CEBP and AP-1 transcription factors. Sci. Rep. 4, 4450. doi: 10.1038/srep04450
Lleo, A., Cavedo, E., Parnetti, L., Vanderstichele, H., Herukka, S. K., Andreasen, N., et al. (2015). Cerebrospinal fluid biomarkers in trials for Alzheimer and Parkinson diseases. Nat. Rev. Neurol. 11, 41–55. doi: 10.1038/nrneurol.2014.232
Lumpkins, K., Bochicchio, G. V., Zagol, B., Ulloa, K., Simard, J. M., Schaub, S., et al. (2008). Plasma levels of the beta chemokine regulated upon activation, normal T cell expressed, and secreted (RANTES) correlate with severe brain injury. J. Trauma 64, 358–361. doi: 10.1097/TA.0b013e318160df9b
Martin, E., and Delarasse, C. (2018). Complex role of chemokine mediators in animal models of Alzheimer's Disease. Biomed. J. 41, 34–40. doi: 10.1016/j.bj.2018.01.002
McKhann, G., Drachman, D., Folstein, M., Katzman, R., Price, D., and Stadlan, E. M. (1984). Clinical diagnosis of Alzheimer's disease: report of the NINCDS-ADRDA Work Group under the auspices of Department of Health and Human Services Task Force on Alzheimer's Disease. Neurology 34, 939–944. doi: 10.1212/WNL.34.7.939
Mocchetti, I., Campbell, L. A., Harry, G. J., and Avdoshina, V. (2013). When human immunodeficiency virus meets chemokines and microglia: neuroprotection or neurodegeneration? J. Neuroimmune Pharmacol. 8, 118–131. doi: 10.1007/s11481-012-9353-4
Motter, R., Vigo-Pelfrey, C., Kholodenko, D., Barbour, R., Johnson-Wood, K., Galasko, D., et al. (1995). Reduction of beta-amyloid peptide42 in the cerebrospinal fluid of patients with Alzheimer's disease. Ann. Neurol. 38, 643–648. doi: 10.1002/ana.410380413
Nagarsheth, N., Wicha, M. S., and Zou, W. (2017). Chemokines in the cancer microenvironment and their relevance in cancer immunotherapy. Nat. Rev. Immunol. 17, 559–572. doi: 10.1038/nri.2017.49
Olsson, B., Lautner, R., Andreasson, U., Ohrfelt, A., Portelius, E., Bjerke, M., et al. (2016). CSF and blood biomarkers for the diagnosis of Alzheimer's disease: a systematic review and meta-analysis. Lancet Neurol. 15, 673–684. doi: 10.1016/S1474-4422(16)00070-3
Pan, R., Luo, S., Huang, Q., Li, W., Cai, T., Lai, K., et al. (2022). The associations of cerebrospinal fluid ferritin with neurodegeneration and neuroinflammation along the Alzheimer's disease continuum. J. Alzheimers. Dis. 88, 1115–1125. doi: 10.3233/JAD-220002
Puntambekar, S. S., Moutinho, M., Lin, P. B., Jadhav, V., Tumbleson-Brink, D., Balaji, A., et al. (2022). CX3CR1 deficiency aggravates amyloid driven neuronal pathology and cognitive decline in Alzheimer's disease. Mol. Neurodegener. 17, 47. doi: 10.1186/s13024-022-00545-9
Ramirez, A. I., De Hoz, R., Salobrar-Garcia, E., Salazar, J. J., Rojas, B., Ajoy, D., et al. (2017). The role of microglia in retinal neurodegeneration: Alzheimer's disease, Parkinson, and glaucoma. Front. Aging Neurosci. 9, 214. doi: 10.3389/fnagi.2017.00214
Reaux-Le Goazigo, A., Van Steenwinckel, J., Rostene, W., and Melik Parsadaniantz, S. (2013). Current status of chemokines in the adult CNS. Prog. Neurobiol. 104, 67–92. doi: 10.1016/j.pneurobio.2013.02.001
Rocha De Paula, M., Gomez Ravetti, M., Berretta, R., and Moscato, P. (2011). Differences in abundances of cell-signalling proteins in blood reveal novel biomarkers for early detection of clinical Alzheimer's disease. PLoS ONE 6, e17481. doi: 10.1371/journal.pone.0017481
Scheltens, P., De Strooper, B., Kivipelto, M., Holstege, H., Chetelat, G., Teunissen, C. E., et al. (2021). Alzheimer's disease. Lancet 397, 1577–1590. doi: 10.1016/S0140-6736(20)32205-4
Sharma, V., Mishra, M., Ghosh, S., Tewari, R., Basu, A., Seth, P., et al. (2007). Modulation of interleukin-1beta mediated inflammatory response in human astrocytes by flavonoids: implications in neuroprotection. Brain Res. Bull. 73, 55–63. doi: 10.1016/j.brainresbull.2007.01.016
Shen, X. N., Niu, L. D., Wang, Y. J., Cao, X. P., Liu, Q., Tan, L., et al. (2019). Inflammatory markers in Alzheimer's disease and mild cognitive impairment: a meta-analysis and systematic review of 170 studies. J. Neurol. Neurosurg. Psychiatry 90, 590–598. doi: 10.1136/jnnp-2018-319148
Sozzani, S., Zhou, D., Locati, M., Rieppi, M., Proost, P., Magazin, M., et al. (1994). Receptors and transduction pathways for monocyte chemotactic protein-2 and monocyte chemotactic protein-3. Similarities and differences with MCP-1. J. Immunol. 152, 3615–3622. doi: 10.4049/jimmunol.152.7.3615
Stang, A. (2010). Critical evaluation of the Newcastle-Ottawa scale for the assessment of the quality of nonrandomized studies in meta-analyses. Eur. J. Epidemiol. 25, 603–605. doi: 10.1007/s10654-010-9491-z
Stolp, H. B., and Dziegielewska, K. M. (2009). Review: role of developmental inflammation and blood-brain barrier dysfunction in neurodevelopmental and neurodegenerative diseases. Neuropathol. Appl. Neurobiol. 35, 132–146. doi: 10.1111/j.1365-2990.2008.01005.x
Su, C., Zhao, K., Xia, H., and Xu, Y. (2019). Peripheral inflammatory biomarkers in Alzheimer's disease and mild cognitive impairment: a systematic review and meta-analysis. Psychogeriatrics 19, 300–309. doi: 10.1111/psyg.12403
Tripathy, D., Thirumangalakudi, L., and Grammas, P. (2010). RANTES upregulation in the Alzheimer's disease brain: a possible neuroprotective role. Neurobiol. Aging 31, 8–16. doi: 10.1016/j.neurobiolaging.2008.03.009
Ubogu, E. E., Callahan, M. K., Tucky, B. H., and Ransohoff, R. M. (2006). Determinants of CCL5-driven mononuclear cell migration across the blood-brain barrier. Implications for therapeutically modulating neuroinflammation. J. Neuroimmunol. 179, 132–144. doi: 10.1016/j.jneuroim.2006.06.004
Verite, J., Page, G., Paccalin, M., Julian, A., and Janet, T. (2018). Differential chemokine expression under the control of peripheral blood mononuclear cells issued from Alzheimer's patients in a human blood brain barrier model. PLoS ONE 13, e0201232. doi: 10.1371/journal.pone.0201232
Vilgelm, A. E., and Richmond, A. (2019). Chemokines modulate immune surveillance in tumorigenesis, metastasis, and response to immunotherapy. Front. Immunol. 10, 333. doi: 10.3389/fimmu.2019.00333
Weng, S., Lai, Q. L., Wang, J., Zhuang, L., Cheng, L., Mo, Y., et al. (2022). The role of exosomes as mediators of neuroinflammation in the pathogenesis and treatment of Alzheimer's disease. Front. Aging Neurosci. 14, 899944. doi: 10.3389/fnagi.2022.899944
Wojcieszak, J., Kuczynska, K., and Zawilska, J. B. (2022). Role of chemokines in the development and progression of Alzheimer's disease. J. Mol. Neurosci. 72, 1929–1951. doi: 10.1007/s12031-022-02047-1
Xia, M. Q., Qin, S. X., Wu, L. J., Mackay, C. R., and Hyman, B. T. (1998). Immunohistochemical study of the beta-chemokine receptors CCR3 and CCR5 and their ligands in normal and Alzheimer's disease brains. Am. J. Pathol. 153, 31–37. doi: 10.1016/S0002-9440(10)65542-3
Yao, H., Peng, F., Dhillon, N., Callen, S., Bokhari, S., Stehno-Bittel, L., et al. (2009). Involvement of TRPC channels in CCL2-mediated neuroprotection against tat toxicity. J. Neurosci. 29, 1657–1669. doi: 10.1523/JNEUROSCI.2781-08.2009
Zhu, C., Xu, B., Sun, X., Zhu, Q., and Sui, Y. (2017). Targeting CCR3 to reduce amyloid-beta production, tau hyperphosphorylation, and synaptic loss in a mouse model of Alzheimer's disease. Mol. Neurobiol. 54, 7964–7978. doi: 10.1007/s12035-016-0269-5
Zintzaras, E., and Ioannidis, J. P. (2005). Heterogeneity testing in meta-analysis of genome searches. Genet. Epidemiol. 28, 123–137. doi: 10.1002/gepi.20048
Keywords: Alzheimer's disease, chemokines, meta-analysis, plasma, CSF
Citation: Wang H, Zong Y, Zhu L, Wang W and Han Y (2023) Chemokines in patients with Alzheimer's disease: A meta-analysis. Front. Aging Neurosci. 15:1047810. doi: 10.3389/fnagi.2023.1047810
Received: 18 September 2022; Accepted: 20 February 2023;
Published: 09 March 2023.
Edited by:
Bogdan O. Popescu, Carol Davila University of Medicine and Pharmacy, RomaniaReviewed by:
Panteleimon Giannakopoulos, University of Geneva, SwitzerlandEva Bagyinszky, Gachon University, Republic of Korea
Copyright © 2023 Wang, Zong, Zhu, Wang and Han. This is an open-access article distributed under the terms of the Creative Commons Attribution License (CC BY). The use, distribution or reproduction in other forums is permitted, provided the original author(s) and the copyright owner(s) are credited and that the original publication in this journal is cited, in accordance with accepted academic practice. No use, distribution or reproduction is permitted which does not comply with these terms.
*Correspondence: Weiyi Wang, xc_wangwy@163.com; Yanshuo Han, yanshuohan@dlut.edu.cn
†These authors have contributed equally to this work