- 1 The Division of Molecular Physiology and Neuroscience, Department of Neuroscience, Uppsala University, Uppsala, Sweden
- 2 The Division of Biological Research on Drug Dependence, Department of Pharmaceutical Bioscience, Uppsala University, Uppsala, Sweden
- 3 Institute of Biomedicine, Pharmacology, University of Helsinki, Finland
Alcohol dependence is a common chronic relapsing disorder. The development of alcohol dependence has been associated with changes in brain GABAA channel-mediated neurotransmission and plasticity. We have examined mRNA expression of the GABAA channel subunit genes in three brain regions in individuals with or without alcohol dependence using quantitative real-time PCR assay. The levels of selective GABAA channel subunit mRNAs were altered in specific brain regions in alcoholic subjects. Significant increase in the α1, α4, α5, β1, and γ1 subunit mRNAs in the hippocampal dentate gyrus region, and decrease in the β2 and δ subunit mRNAs in the orbitofrontal cortex were identified whereas no changes in the dorsolateral prefrontal cortex were detected. The data increase our understanding of the role of GABAA channels in the development of alcohol dependence.
Introduction
Beverages containing alcohol are commonly consumed in today’s societies and often abused. The brain is one of the main targets of alcohol. Long-term excessive consumption of alcohol can change the brain and lead to a variety of behavioral changes such as addiction and cognitive dysfunction (Harper, 1998). Magnetic resonance imaging studies have showed reduced hippocampal and prefrontal cortex volume of individuals suffering from alcohol dependence that may contribute to the cognitive deficit associated with chronic alcohol exposure (Jernigan et al., 1991; Sullivan et al., 1995). These aversive effects may be associated with direct and indirect actions of alcohol on various neurotransmitter and neuropeptide systems within the central nervous system (CNS; Harris et al., 2008; Vengeliene et al., 2008; Spanagel, 2009). Among those neurotransmitter receptors, a special focus has been on the association of alcohol action and alcoholism with γ-aminobutyric acid type A (GABAA) ion channels during the last 30 years. Many GABAA channel subunit genes have been suggested to be associated with human alcoholism (Korpi and Sinkkonen, 2006), but detailed mechanisms remain poorly known and are inconsistent between studies.
The GABAA channels are GABA-gated anion channels that predominantly mediate inhibitory neurotransmission within CNS. Each GABAA channel complex is formed by five homologous protein subunits and to date 19 GABAA channel subunits (α1–6, β1–3, γ1–3, δ, ε, θ, π, and ρ1–3) have been identified in mammals (Olsen and Sieghart, 2008). The various combinations of different subunits and associated proteins account for the diverse pharmacological and biophysical properties of the GABAA channel complex in the plasma membrane (Birnir and Korpi, 2007; Uusi-Oukari and Korpi, 2010). GABAA channels in neurons are present at synapses and extrasynaptic sites and mediate fast phasic inhibition and persistent tonic inhibition, respectively (Mody and Pearce, 2004; Semyanov et al., 2004; Lindquist and Birnir, 2006; Jin et al., 2011). Although many previous studies have shown that alcohol potentiates GABAA channels, how alcohol directly acts on GABAA channels remains unclear (Korpi et al., 2007). Alcohol action on GABAA channels depends on the concentration of alcohol and the channel subunits composition (Olsen et al., 2007). Some reports have demonstrated that low concentrations of alcohol (3–30 mM) can enhance the tonic inhibition mediated by extrasynaptic α4/6βδ GABAA channels but not the phasic inhibition mediated by γ2-containing synaptic GABAA channels (Sundstrom-Poromaa et al., 2002; Wallner et al., 2003; Wei et al., 2004; Borghese et al., 2006; Korpi et al., 2007; Baur et al., 2009). In contrast, high concentration of alcohol (>60 mM) directly modulates GABAA channels associated with phasic inhibition and the putative alcohol-binding site was identified in-between transmembrane domains 2 and 3 (Mihic et al., 1997). In addition to the acute alcohol effects on GABAA channels, chronic exposure to alcohol can affect GABAA channel functions via alterations in subunit expression, post-translational modification, localization, intracellular signaling, and neurosteroid response (Kumar et al., 2009). In rodent models, chronic alcohol administration differentially changes the expression levels of GABAA channel subunit mRNAs and proteins across various brain regions (Grobin et al., 2000; Liang et al., 2006; Sarviharju et al., 2006). However, alcohol-dependent disorders in humans are not fully mimicked by rodent models. Therefore, studies conducted on samples from post-mortem brains of individuals suffering from alcohol dependence may add important information and aid in understanding the mechanisms underlying human alcohol-dependent disorders.
In the present study, we performed quantitative real-time PCR (RT-qPCR) to investigate the expression of GABAA channel subunit mRNAs in the post-mortem hippocampal dentate gyrus, orbitofrontal, and dorsolateral prefrontal cortex (DL-PFC) of individuals suffering from alcohol dependence and have compared the results to brain samples from individuals without alcohol dependence.
Materials and Methods
Human Samples
Twenty-one human controls and 19 individuals suffering from chronic alcohol dependence were included in the study. All individuals were Caucasian males. The individuals suffering from alcoholism consumed ≥80 g alcohol per day during the majority of their adult lives, met the criteria for Diagnostic and Statistical Manual for Mental Disorders, fourth edition and National Health and Medical Research Council/World Health Organization and did not have liver cirrhosis, Wernicke–Korsakoff’s syndrome, or multi-drug abuse history. Individuals in the control group had either abstained from alcohol completely or were social drinkers who consumed ≤20 g of alcohol per day on average. Individuals in the control group were matched to individuals suffering from alcoholism by age and post-mortem interval (PMI). Post-mortem brain samples from hippocampal dentate gyrus (including both granule and molecular layer), orbitofrontal cortex (OFC; Brodmann’s area 47), and DL-PFC (Brodmann’s area 9) were collected at the New South Wales Tissue Resource Center (TRC), University of Sydney, Australia (http://sydney.edu.au/medicine/pathology/trc/index.php). The samples from all three brain regions were collected from the same donor in seven controls and ten individuals suffering from alcoholism. All samples were collected by qualified pathologists under full ethical clearance and with informed, written consent from the next of kin. The detailed demographic data for all subjects are given in Table A1 in Appendix.
Total RNA Isolation
Total RNA was isolated by using RNeasy Lipid Tissue Mini Kit (QIAGEN, MD, USA) or GenElute total RNA Miniprep (Sigma) and quantified with Nanodrop (Nanodrop Technologies, Inc.). The quality of RNA was evaluated by measuring RNA Quality Indicator (RQI) using Bio-Rad Experion (Bio-Rad Laboratories, Hercules, CA, USA) with Eukaryote Total RNA StdSens assay following the manufacturer’s manual. RQI is equivalent to RNA integrity number (RIN) from Agilent (Denisov et al., 2008). RNA samples with RQI values greater than 5 are generally considered as suitable for RT-qPCR (Fleige and Pfaffl, 2006; Fleige et al., 2006). In this study, samples with RQI less than 5 were not used for experiments. Average RQI of the samples was 7.29 ± 0.12 (mean ± SEM; 83% samples have RQI greater than 6) indicating high quality of isolated total RNA.
Quantitative Real-Time RT-PCR
Total RNA (250 ng) was reverse transcribed into cDNA in a 20 μl reaction mixture using Superscript III reverse transcriptase (Invitrogen). RT negative control was performed by omitting reverse transcriptase in the reaction in order to confirm no genomic DNA contamination in the isolated RNA. Real-time PCRs were performed in a 10 μl reaction mixture containing 4 μl cDNA (1 ng), 1 × PCR reaction buffer, 3 mM MgCl2, 0.3 mM dNTP, 1 × ROX reference dye, 0.8 U JumpStart Taq DNA polymerase (Sigma-Aldrich), 5 × SYBR Green I (Invitrogen), and 0.4 μM each of forward and reverse primers. The gene-specific primer pairs (primer sequences shown in Table A2 in Appendix) were designed using Primer Express Software version 3.0 (Applied Biosystems), synthesized by Invitrogen and further validated using BioBank cDNA from human brain (PrimerDesign). Amplification was performed in 384-well optical plates using the ABI PRISM 7900HT Sequence Detection System (Applied Biosystems) with an initial denaturation of 5 min at 95°C, followed by 45 cycles of 95°C for 15 s, 60°C for 30 s, and 72°C for 30 s. A melting curve was determined at the end of cycling to ensure the amplification of a single PCR product. Cycle threshold values (Ct) were determined with the SDS 2.3 and RQ Manager 1.2 softwares supplied with the instrument. The expression of each target gene relative to a normalization factor (geometric mean of two reference genes) was calculated with DataAssist v2.0 using the 2−ΔCt method as previously described (Schmittgen and Livak, 2008). Reference genes beta actin (ACTB) and ubiquitin C (UBC) for hippocampal dentate gyrus (average expression stability value M = 0.25), ribosomal large P0 (RPLP0) and ACTB for prefrontal cortex (average expression stability value M = 0.22), and phosphoglycerate kinase 1 (PGK1) and peptidylprolyl isomerase A (PPIA) for OFC (average expression stability value M = 0.125) were selected for normalization according to previously developed approach for analysis of reference genes (Johansson et al., 2007; Kuzmin et al., 2009; Bazov et al., 2011). As the expression of reference genes may vary between different brain regions of human alcoholics, it is of great importance to use validated stable reference genes for normalization.
Statistical Analysis
Statistical analysis was carried out using SigmaPlot and SigmaStat (Systat Software Inc., USA). Normality of data distribution was analyzed using Shapiro–Wilk normality test (see Table A3 in Appendix). The differences between groups were assessed by one-way ANOVA with Bonferroni post hoc test (normally distributed data) or non-parametric Kruskal–Wallis ANOVA on ranks with Dunn’s post hoc test (not normally distributed data). A general stepwise linear regression model was used to identify covariates (e.g., age and PMI). Variables with a significant association with group (controls and alcoholics) were included in the final statistical model as covariates. A significant level was set to p < 0.05.
Results
The demographic characteristics of individuals in this study are shown in Table 1 and Table A1 in Appendix. There was no significant difference in age, PMI, brain pH, RNA quality indicator, and proportions of smokers and non-smokers between individuals with or without alcohol dependence (Table 1).
Expression of the 19 GABAA channel subunit mRNAs (α1–6, β1–3, γ1–3, δ, ε, θ, π, ρ1–3) was quantified by RT-qPCR in the samples collected from the hippocampal dentate gyrus region (HP-DG), the orbitofronral cortex (OFC, Brodmann area 47), and the dorsolateral prefrontal cortex (DL-PFC, Brodmann area 9). Subunit genes that were not detected in any of the three brain regions were the π, ρ1, and ρ3 subunits.
Increased Levels of mRNAs for GABAA Channel Subunits α1, α4, α5, β1, and γ1 in the Hippocampal Dentate Gyrus Region of Alcoholics
In the hippocampal dentate gyrus region of individuals without alcohol dependence, high expression of α1, α2, α4, α5, β1, β2, and γ2, modest expression of α3, β3, γ1, γ3, δ, and θ, low expression of α6, ε, and ρ2 subunit mRNAs were detected (Figure 1). Interestingly, the mRNA levels of α1, α4, α5, β1, and γ1 subunits were significantly higher in the HP-DG of individuals suffering from alcohol dependence than in the controls. There was a 1.5-fold (α1), 1.6-fold (α4), 1.7-fold (α5), 2.1-fold (β1), and 2.3-fold (γ1) increase of mRNA in the hippocampal dentate gyrus region of individuals suffering from alcoholism as compared to those without alcohol dependence. Stepwise linear regression identified age, PMI, and brain pH as covariates for the α5 expression level. However, inclusion of these covariates in linear regression model did not affect the significant difference in α5 expression level between the two groups. The mRNA levels of other GABAA channel subunits did not differ between two groups (Figure 2).
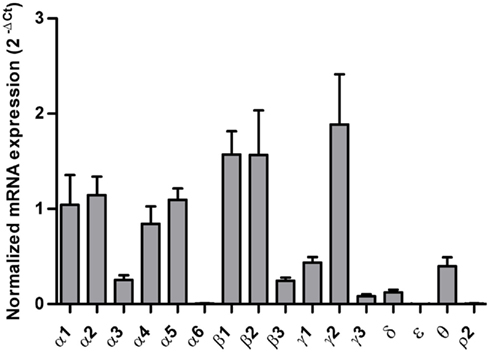
Figure 1. Expression of GABAA channel subunit mRNAs in the hippocampal dentate gyrus region from control subjects (n = 15). The mRNA level of each subunit was normalized to reference genes ACTB and UBC and presented as mean ± SE.
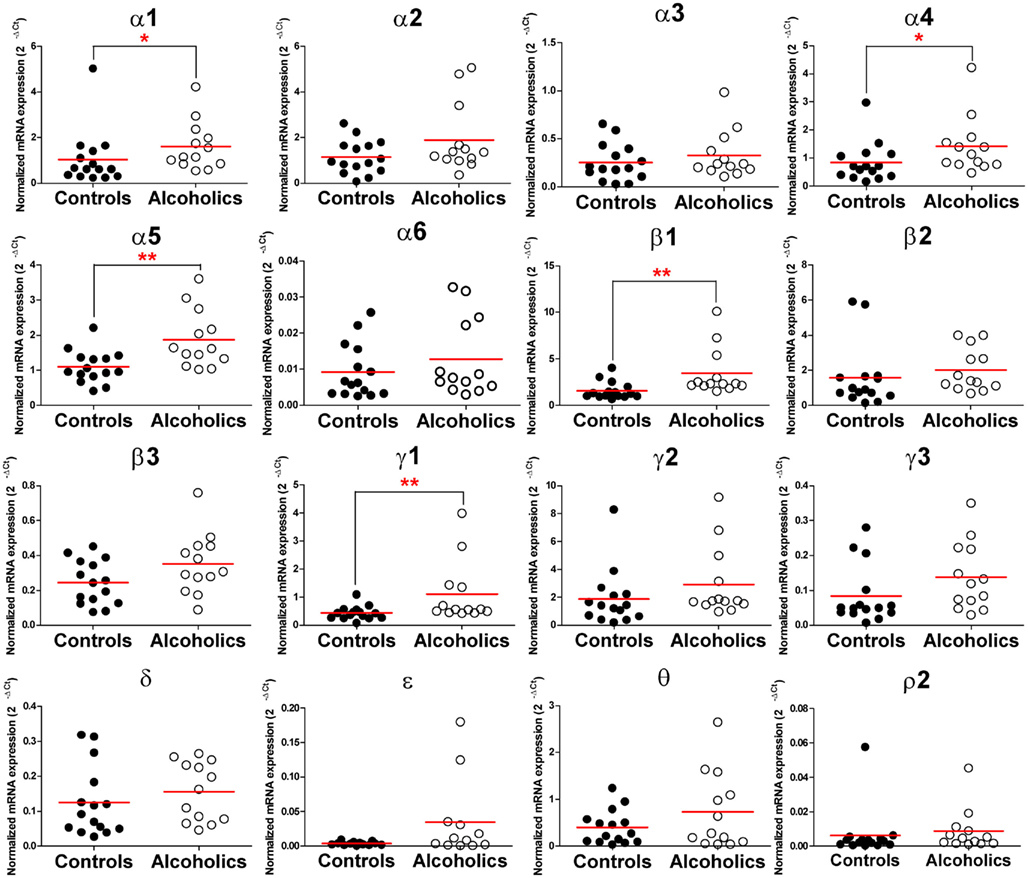
Figure 2. Expression of GABAA channel subunit mRNAs in the hippocampal dentate gyrus region of controls (•, n = 15) and alcoholics (◦, n = 13). Horizontal lines represented mean levels. Kruskal–Wallis ANOVA on ranks with Dunn’s post hoc test, α1, H(1, 28) = 4.39, p = 0.036; α2, H(1, 28) = 1.72, p = 0.19; α3, H(1, 28) = 0.73, p = 0.39; α4, H(1, 28) = 5.41, p = 0.02; α 5, H(1, 28) = 8.83, p = 0.003; α6, H(1, 28) = 1.17, p = 0.28; β1, H(1, 28) = 9.38, p = 0.002; β2, H(1, 28) = 2.68, p = 0.10; γ1, H(1, 28) = 7.02, p = 0.008; γ2, H(1, 28) = 2.83, p = 0.09; γ3, H(1, 28) = 3.31, p = 0.07; δ, H(1, 28) = 1.07, p = 0.3; ρ2, H(1, 28) = 1.97, p = 0.16; θ, H(1, 28) = 0.38, p = 0.53; ε, H(1, 28) = 2.32, p = 0.13. One way ANOVA with Bonferroni post hoc test, β3, df = 23, p = 0.06.
Decreased Expression of GABAA Channel Subunit β2 and δ mRNAs in the Orbitofrontal Cortex of Alcoholics
In the OFC of individuals without alcohol dependence, high expression of α1, α2, α4, β1, β2, and γ2, modest expression of α3, α5, β3, γ1, γ3, and δ, low expression of α6, ε, θ, and ρ2 subunit mRNAs were detected (Figure 3). Of particular interest is the result that the mRNA levels of the β2 and δ subunits were 26 and 47% lower, respectively, in individuals suffering from alcohol dependence as compared to those without alcohol dependence, while the mRNA expression of other subunits did not differ between the two groups (Figure 4). Stepwise linear regression identified age as a covariate for the β2 and δ expression level. However, inclusion of age as a covariate in linear regression model failed to affect the significant difference in the β2 and δ expression levels between the two groups.
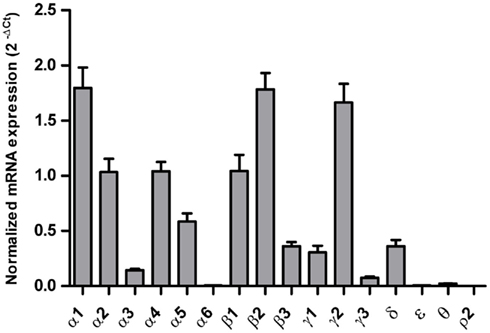
Figure 3. Expression of GABAA channel subunit mRNAs in the orbitolfrontal cortex of controls (n = 14). The mRNA level of each subunit was normalized to reference genes PPIA and PGK1 and presented as mean ± SE.
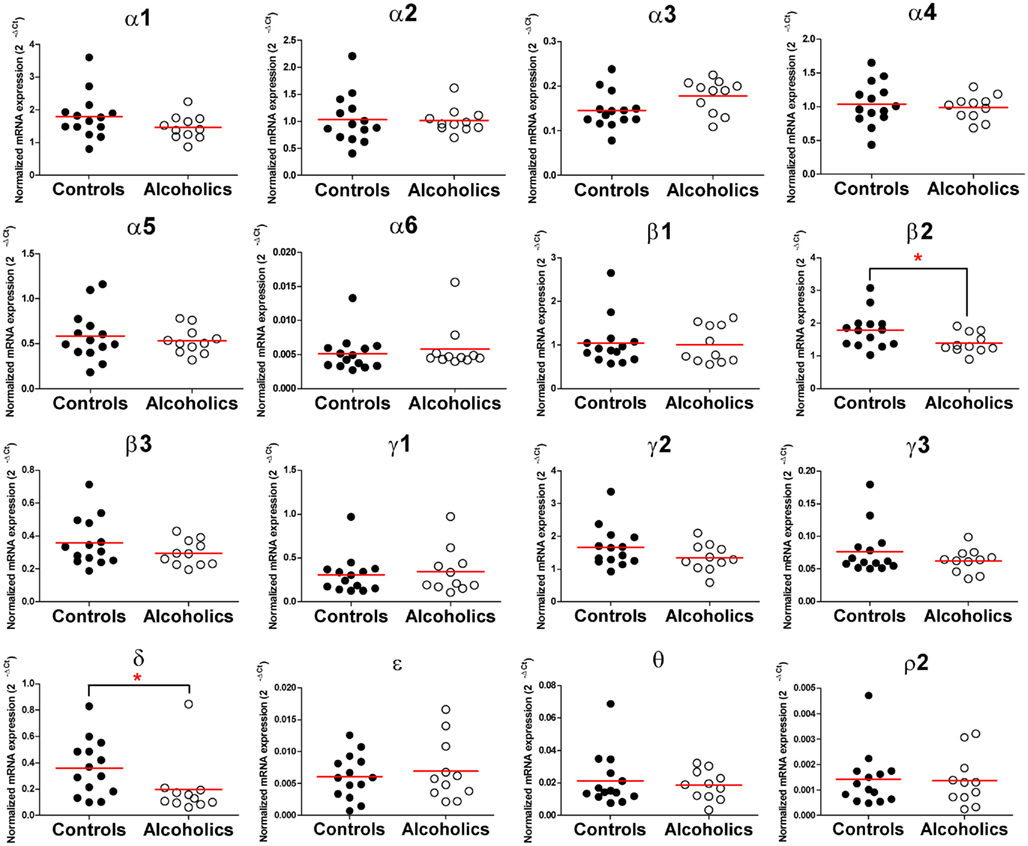
Figure 4. Expression of GABAA channel subunits mRNA in the orbitofrontal cortex of controls (•, n = 14) and alcoholics (◦, n = 11). Horizontal lines represented mean levels. One way ANOVA with Bonferroni post hoc test, α3, df = 23, p = 0.052; α4, df = 23, p = 0.61; α 5, df = 23, p = 0.55; β2, df = 23, p = 0.045; ε, df = 23, p = 0.06. Kruskal–Wallis ANOVA on ranks with Dunn’s post hoc test, α1, H(1, 25) = 2.03, p = 0.15; α2, H(1, 25) = 0.3, p = 0.58; α6, H(1, 25) = 0.51, p = 0.48; β1, H(1, 25) = 0.05, p = 0.83; β3, H(1, 25) = 1.09, p = 0.3; γ1, H(1, 25) = 0.15, p = 0.70; γ2, H(1, 25) = 1.59, p = 0.21; γ3, H(1, 25) = 0.15, p = 0.70; θ, H(1, 25) = 0.003, p = 0.96; ρ2, H(1, 25) = 0.003, p = 0.96; δ, H(1, 25) = 5.8, p = 0.016.
Unaltered Expression of GABAA Channel Subunit mRNAs in the Dorsolateral Prefrontal Cortex of Alcoholics
The mRNA expression profile of GABAA channel subunits in the DL-PFC in individuals without alcohol dependence closely resembled that observed in the OFC (Figures 3 and 5). Furthermore, no significant differences in the mRNA expression were observed for any of the subunits between alcoholics and non-alcoholic controls (Figure 6).
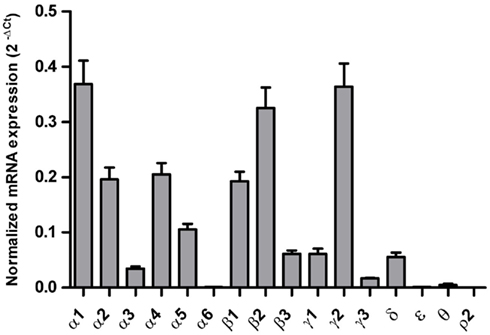
Figure 5. Expression of GABAA channel subunit mRNAs in the prefrontal cortex of controls (n = 15). The mRNA level of each subunit was normalized to reference genes ACTB and RPLP0 and presented as mean ± SE.
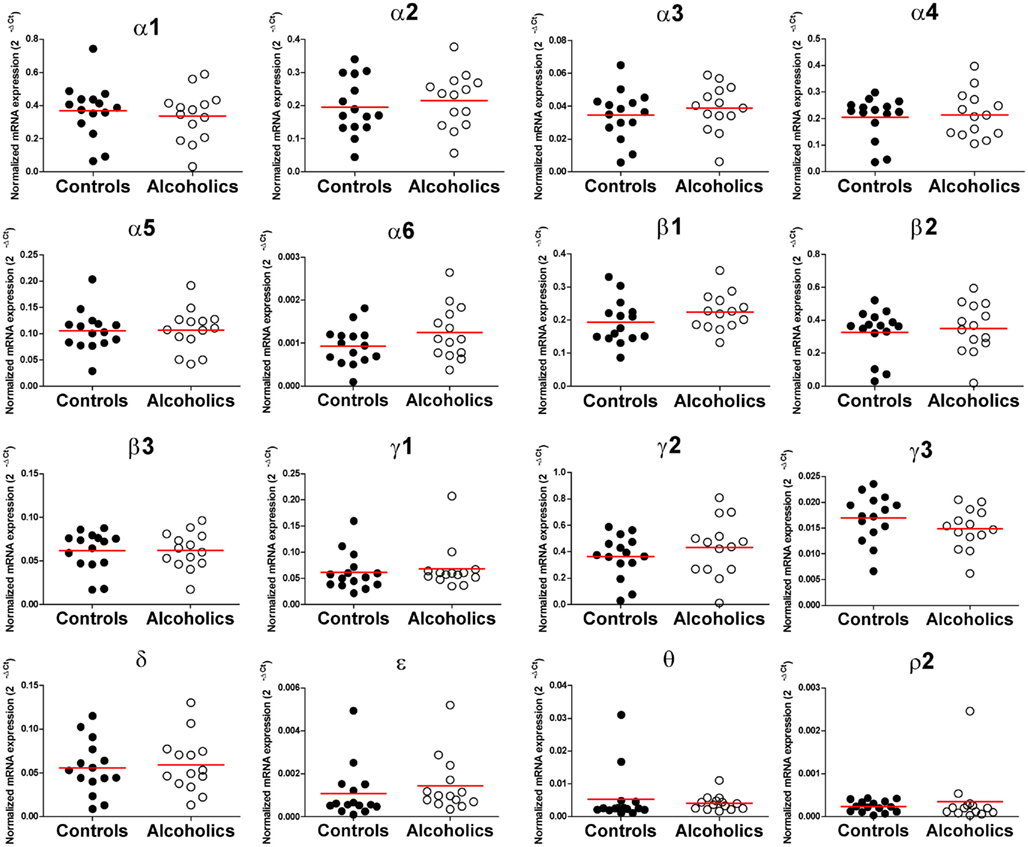
Figure 6. Expression of GABAA channel subunit mRNAs in the prefrontal cortex of controls (•, n = 15) and alcoholics (◦, n = 14). Horizontal lines represented mean levels. One way ANOVA with Bonferroni post hoc test, α1, df = 27, p = 0.58; α2, df = 27, p = 0.55; α3, df = 27, p = 0.45; α4, df = 27, p = 0.76; α5, df = 27, p = 0.93; α6, df = 27, p = 0.13; β1, df = 27, p = 0.19; β2, df = 27, p = 0.66; β3, df = 27, p = 0.97; γ2, df = 27, p = 0.36; γ3, df = 27, p = 0.19; δ, df = 27, p = 0.76; Kruskal–Wallis ANOVA on ranks with Dunn’s post hoc test, γ1, H(1, 29) = 0.69, p = 0.41; ε, H(1, 29) = 0.13; θ, H(1, 29) = 1.29, p = 0.26; ρ2, H(1, 29) = 0.62, p = 0.43.
Discussion
The expression of specific GABAA channel subunit mRNAs was altered in specific brain regions in individuals suffering from alcoholism. In particular, there was a significant increase of the α1, α4, α5, β1, and γ1 subunit mRNAs in the HP-DG; a decrease of the β2 and δ in the OFC, but no change of any subunit expression in the DL-PFC. These data complement previous expression studies in individuals suffering from alcohol dependence providing further evidence for long-term changes in the GABAA channels in the CNS induced by long-term alcohol consumption (Lewohl et al., 1997, 2001; Mitsyama et al., 1998; Thomas et al., 1998; Buckley and Dodd, 2004).
Gene expression profiling studies by, e.g., quantitative PCR using human autopsy brain tissue can be affected by many pre- and post-mortem factors such as age, gender, ethnicity, and PMI. In this study we have tried to minimize the differences between the two groups of individuals we have studied. All individuals included in the study were Caucasian males and the two groups were matched for parameters such as age, PMI, brain pH, RNA quality indicator value, and proportions of smokers and non-smokers. In addition, the sample size in each group was between 11 and 15 that falls within the group–size range providing rather reliable statistical estimation (Hynd et al., 2003).
GABAA channels are pentameric GABA-gated chloride channels. A change of subunit composition in the GABAA channel complex can directly affect its cellular and sub-cellular location as well as physiological and pharmacological properties of the channel, including ethanol sensitivity (Birnir and Korpi, 2007). Several studies have indicated that the expression of GABAA channel subunits differs at both the mRNA and protein levels between post-mortem brains from non-alcoholic individuals and alcoholics, although the results remain somewhat ambiguous (Lewohl et al., 1997, 2001; Dodd and Lewohl, 1998; Buckley et al., 2000, 2006; Buckley and Dodd, 2004). It has been shown that the expression of the GABAA channel subunit α1 mRNA but not protein was elevated in the superior frontal cortex of alcoholics (Lewohl et al., 1997; Dodd and Lewohl, 1998). Similarly, in our study α1 mRNA level was increased in the hippocampal dentate gyrus of alcoholic individuals. However, chronic alcohol administration to rodents decreased or did not change the α1 mRNA and protein levels in the cerebral cortex, cerebellum, or hippocampus (Uusi-Oukari and Korpi, 2010). This discrepancy in results between humans and rodents can potentially be attributed to the difference in, e.g., in metabolism or length of alcohol exposure, transcriptional regulation and animal models of alcohol dependence, in addition to the species difference.
GABAA channels containing the α4, α5, or the δ subunit are of particular interest. These subunits are parts of extrasynaptic GABAA channels that participate in generating tonic neuronal inhibition that decreases action potential frequency in neurons (Pavlov et al., 2009; Jin et al., 2011). Activation of GABAA channels containing these subunits is thought to have implications for cognitive function. In a rodent model for excessive alcohol consumption, repeated ethanol withdrawals or longer ethanol exposure increased the α4 subunit protein expression in the hippocampus (Matthews et al., 1998; Cagetti et al., 2003). This is in accordance with our data where the α4 mRNA expression was elevated in alcoholic individuals as compared to the non-alcoholic subjects. In the human hippocampus, the α5 subunit is abundant in the dentate gyrus molecular layer as well as in mid-CA1 regions (Howell et al., 2000; Wainwright et al., 2000; Rissman et al., 2003), whereas in the rodent hippocampus, α5-containing GABAA channels are only highly expressed in CA1 pyramidal neurons (Sperk et al., 1997). Genetic or pharmacological manipulation of α5-containing GABAA channels in mice modulates hippocampus-dependent learning (Crestani et al., 2002; Caraiscos et al., 2004; Martin et al., 2010; Prut et al., 2010). It is possible that GABAA α4 or α5 subunit-selective compounds may potentially be used for the treatment of alcohol-induced cognitive deficit.
Ethanol can induce the release of endogenous GABAergic neurosteroids that further enhance the GABA signaling system in neurons (Biggio et al., 2007). The sensitivity to neurosteroids is higher in γ1 subunit-containing GABAA channels than in γ2 subunit-containing GABAA channels (Puia et al., 1993). Chronic ethanol administration in rodents significantly increases the mRNA expression of γ1 subunit in the cerebral cortex (Devaud et al., 1995) and in the hippocampus (Cagetti et al., 2003), and similarly in our study, up-regulation of the γ1 subunit was observed in the HP-DG from alcoholic individuals. Therefore, increased sensitivity to neurosteroids of GABAA channels may be associated with the alcohol dependence.
Some of the genes encoding the human GABAA channel subunits are organized into clusters on chromosomes. Chromosome 4 contains four GABAA channel genes: GABRA2 (α2), GABRA4 (α4), GABRB1 (β1), and GABRG1 (γ1) (Reich et al., 1998). As the change in the gene regulation of one GABAA channel subunit may affect the transcription levels of other GABAA subunit genes in the same cluster (Uusi-Oukari et al., 2000; Steiger and Russek, 2004), it is not surprising to see the up-regulation of three of them (α4, β1, and γ1), in HP-DG of alcoholic individuals in our study. It will be worth using genetic mapping approach to study whether these GABAA subunit expressions are associated with specific gene polymorphisms and whether the regulation of transcription is similar for these subunit genes (Joyce, 2007). Since the α1, α4, α5, and β1 GABAA subunits are abundant in the hippocampal dentate gyrus, the increase in the expression of these subunits may have significant functional consequence in alcohol-induced cognitive impairment. Further studies are needed to determine the protein level of these altered subunits and assess their putative functional impact in human alcoholism.
Chronic alcohol consumption in humans has been shown to cause impairment of executive and cognitive functions which require normal prefrontal cortical function (Goldstein et al., 2004; Crego et al., 2010). Here we have examined the expression of GABAA channel subunits in the sub-regions of cortex, DL-PFC, and OFC from alcoholics. In the OFC of alcoholic individuals, the β2 and δ GABAA subunits were significantly decreased. Whether this decrease contributes to the impaired GABAergic function in the OFC reported in studies involving alcoholics remains to be determined (Volkow et al., 1993, 1997). In contrast, none of the GABAA subunits were changed in the DL-OFC of individuals suffering from alcohol dependence as compared to non-alcoholic individuals. This is in agreement with two microarray studies showing no change of any GABAA subunit mRNAs in the frontal cortex of alcoholic subjects (Mayfield et al., 2002; Flatscher-Bader et al., 2005).
In conclusion, we report brain area-specific selective changes in the mRNA expression of GABAA channel subunits in individuals suffering from alcohol dependence compared to control cases. It is of particular interest that several of the subunits that change with chronic alcohol consumption (e.g., α4, α5, and δ) are present in many extrasynaptic GABAA channels mediating tonic inhibition. As tonic inhibition has a significant role in determining baseline excitability of neurons this is perhaps not surprising but highlights the importance of GABAA channels located outside of synapses for drug effects.
Conflict of Interest Statement
The authors declare that the research was conducted in the absence of any commercial or financial relationships that could be construed as a potential conflict of interest.
Acknowledgments
We thank Karin Nygren for expert technical support. The study was supported by the Swedish Research Council (BB and GB), the Swedish Council for Working Life and Social Research (GB), Uppsala University, and Zhe Jin held a postdoctoral fellowship from the Swedish Association of Medical Research (SSMF). Brain tissues were provided by the New South Wales Tissue Resource Center at the University of Sydney supported by the National Health and Medical Research Council of Australia, National Institute of Alcohol Abuse and Alcoholism, and NSW Department of Health.
References
Baur, R., Kaur, K. H., and Sigel, E. (2009). Structure of alpha6 beta3 delta GABA(A) receptors and their lack of ethanol sensitivity. J. Neurochem. 111, 1172–1181.
Bazov, I., Kononenko, O., Watanabe, H., Kuntic, V., Sarkisyan, D., Taqi, M. M., Hussain, M. Z., Nyberg, F., Yakovleva, T., and Bakalkin, G. (2011). The endogenous opioid system in human alcoholics: molecular adaptations in brain areas involved in cognitive control of addiction. Addict. Biol.. doi: 10.1111/j.1369-1600.2011.00366.x. [Epub ahead of print].
Biggio, G., Concas, A., Follesa, P., Sanna, E., and Serra, M. (2007). Stress, ethanol, and neuroactive steroids. Pharmacol. Ther. 116, 140–171.
Birnir, B., and Korpi, E. R. (2007). The impact of sub-cellular location and intracellular neuronal proteins on properties of GABA(A) receptors. Curr. Pharm. Des. 13, 3169–3177.
Borghese, C. M., Storustovu, S., Ebert, B., Herd, M. B., Belelli, D., Lambert, J. J., Marshall, G., Wafford, K. A., and Harris, R. A. (2006). The delta subunit of gamma-aminobutyric acid type A receptors does not confer sensitivity to low concentrations of ethanol. J. Pharmacol. Exp. Ther. 316, 1360–1368.
Buckley, S. T., and Dodd, P. R. (2004). GABAA receptor beta subunit mRNA expression in the human alcoholic brain. Neurochem. Int. 45, 1011–1020.
Buckley, S. T., Eckert, A. L., and Dodd, P. R. (2000). Expression and distribution of GABAA receptor subtypes in human alcoholic cerebral cortex. Ann. N. Y. Acad. Sci. 914, 58–64.
Buckley, S. T., Foley, P. F., Innes, D. J., Loh El, W., Shen, Y., Williams, S. M., Harper, C. G., Tannenberg, A. E., and Dodd, P. R. (2006). GABA(A) receptor beta isoform protein expression in human alcoholic brain: interaction with genotype. Neurochem. Int. 49, 557–567.
Cagetti, E., Liang, J., Spigelman, I., and Olsen, R. W. (2003). Withdrawal from chronic intermittent ethanol treatment changes subunit composition, reduces synaptic function, and decreases behavioral responses to positive allosteric modulators of GABAA receptors. Mol. Pharmacol. 63, 53–64.
Caraiscos, V. B., Elliott, E. M., You-Ten, K. E., Cheng, V. Y., Belelli, D., Newell, J. G., Jackson, M. F., Lambert, J. J., Rosahl, T. W., Wafford, K. A., Macdonald, J. F., and Orser, B. A. (2004). Tonic inhibition in mouse hippocampal CA1 pyramidal neurons is mediated by alpha5 subunit-containing gamma-aminobutyric acid type A receptors. Proc. Natl. Acad. Sci. U.S.A. 101, 3662–3667.
Crego, A., Rodriguez-Holguin, S., Parada, M., Mota, N., Corral, M., and Cadaveira, F. (2010). Reduced anterior prefrontal cortex activation in young binge drinkers during a visual working memory task. Drug Alcohol Depend. 109, 45–56.
Crestani, F., Keist, R., Fritschy, J. M., Benke, D., Vogt, K., Prut, L., Bluthmann, H., Mohler, H., and Rudolph, U. (2002). Trace fear conditioning involves hippocampal alpha5 GABA(A) receptors. Proc. Natl. Acad. Sci. U.S.A. 99, 8980–8985.
Denisov, V., Strong, V., Walter, V., Gingrich, J., and Wintz, H. (2008). Development and validation of RQI: an RNA quality indicator for the experion automated electrophoresis system. BioRad technology note 5761.
Devaud, L. L., Smith, F. D., Grayson, D. R., and Morrow, A. L. (1995). Chronic ethanol consumption differentially alters the expression of gamma-aminobutyric acidA receptor subunit mRNAs in rat cerebral cortex: competitive, quantitative reverse transcriptase-polymerase chain reaction analysis. Mol. Pharmacol. 48, 861–868.
Dodd, P. R., and Lewohl, J. M. (1998). Cell death mediated by amino acid transmitter receptors in human alcoholic brain damage: conflicts in the evidence. Ann. N. Y. Acad. Sci. 844, 50–58.
Flatscher-Bader, T., Van Der Brug, M., Hwang, J. W., Gochee, P. A., Matsumoto, I., Niwa, S., and Wilce, P. A. (2005). Alcohol-responsive genes in the frontal cortex and nucleus accumbens of human alcoholics. J. Neurochem. 93, 359–370.
Fleige, S., and Pfaffl, M. W. (2006). RNA integrity and the effect on the real-time qRT-PCR performance. Mol. Aspects Med. 27, 126–139.
Fleige, S., Walf, V., Huch, S., Prgomet, C., Sehm, J., and Pfaffl, M. W. (2006). Comparison of relative mRNA quantification models and the impact of RNA integrity in quantitative real-time RT-PCR. Biotechnol. Lett. 28, 1601–1613.
Goldstein, R. Z., Leskovjan, A. C., Hoff, A. L., Hitzemann, R., Bashan, F., Khalsa, S. S., Wang, G. J., Fowler, J. S., and Volkow, N. D. (2004). Severity of neuropsychological impairment in cocaine and alcohol addiction: association with metabolism in the prefrontal cortex. Neuropsychologia 42, 1447–1458.
Grobin, A. C., Fritschy, J. M., and Morrow, A. L. (2000). Chronic ethanol administration alters immunoreactivity for GABA(A) receptor subunits in rat cortex in a region-specific manner. Alcohol. Clin. Exp. Res. 24, 1137–1144.
Harper, C. (1998). The neuropathology of alcohol-specific brain damage, or does alcohol damage the brain? J. Neuropathol. Exp. Neurol. 57, 101–110.
Harris, R. A., Trudell, J. R., and Mihic, S. J. (2008). Ethanol’s molecular targets. Sci. Signal. 1, re7.
Howell, O., Atack, J. R., Dewar, D., Mckernan, R. M., and Sur, C. (2000). Density and pharmacology of alpha5 subunit-containing GABA(A) receptors are preserved in hippocampus of Alzheimer’s disease patients. Neuroscience 98, 669–675.
Hynd, M. R., Lewohl, J. M., Scott, H. L., and Dodd, P. R. (2003). Biochemical and molecular studies using human autopsy brain tissue. J. Neurochem. 85, 543–562.
Jernigan, T. L., Butters, N., Ditraglia, G., Schafer, K., Smith, T., Irwin, M., Grant, I., Schuckit, M., and Cermak, L. S. (1991). Reduced cerebral grey matter observed in alcoholics using magnetic resonance imaging. Alcohol. Clin. Exp. Res. 15, 418–427.
Jin, Z., Jin, Y., Kumar-Mendu, S., Degerman, E., Groop, L., and Birnir, B. (2011). Insulin reduces neuronal excitability by turning on GABA(A) channels that generate tonic current. PLoS ONE 6, e16188. doi:10.1371/journal.pone.0016188
Johansson, S., Fuchs, A., Okvist, A., Karimi, M., Harper, C., Garrick, T., Sheedy, D., Hurd, Y., Bakalkin, G., and Ekstrom, T. J. (2007). Validation of endogenous controls for quantitative gene expression analysis: application on brain cortices of human chronic alcoholics. Brain Res. 1132, 20–28.
Joyce, C. J. (2007). In silico comparative genomic analysis of GABAA receptor transcriptional regulation. BMC Genomics 8, 203. doi:10.1186/1471-2164-8-203
Korpi, E. R., Debus, F., Linden, A. M., Malecot, C., Leppa, E., Vekovischeva, O., Rabe, H., Bohme, I., Aller, M. I., Wisden, W., and Luddens, H. (2007). Does ethanol act preferentially via selected brain GABAA receptor subtypes? the current evidence is ambiguous. Alcohol 41, 163–176.
Korpi, E. R., and Sinkkonen, S. T. (2006). GABA(A) receptor subtypes as targets for neuropsychiatric drug development. Pharmacol. Ther. 109, 12–32.
Kumar, S., Porcu, P., Werner, D. F., Matthews, D. B., Diaz-Granados, J. L., Helfand, R. S., and Morrow, A. L. (2009). The role of GABA(A) receptors in the acute and chronic effects of ethanol: a decade of progress. Psychopharmacology (Berl.) 205, 529–564.
Kuzmin, A., Bazov, I., Sheedy, D., Garrick, T., Harper, C., and Bakalkin, G. (2009). Expression of pronociceptin and its receptor is downregulated in the brain of human alcoholics. Brain Res. 1305(Suppl.), S80–S85.
Lewohl, J. M., Crane, D. I., and Dodd, P. R. (1997). Expression of the alpha 1, alpha 2 and alpha 3 isoforms of the GABAA receptor in human alcoholic brain. Brain Res. 751, 102–112.
Lewohl, J. M., Huygens, F., Crane, D. I., and Dodd, P. R. (2001). GABA(A) receptor alpha-subunit proteins in human chronic alcoholics. J. Neurochem. 78, 424–434.
Liang, J., Zhang, N., Cagetti, E., Houser, C. R., Olsen, R. W., and Spigelman, I. (2006). Chronic intermittent ethanol-induced switch of ethanol actions from extrasynaptic to synaptic hippocampal GABAA receptors. J. Neurosci. 26, 1749–1758.
Lindquist, C. E., and Birnir, B. (2006). Graded response to GABA by native extrasynaptic GABA receptors. J. Neurochem. 97, 1349–1356.
Martin, L. J., Zurek, A. A., Macdonald, J. F., Roder, J. C., Jackson, M. F., and Orser, B. A. (2010). Alpha5GABAA receptor activity sets the threshold for long-term potentiation and constrains hippocampus-dependent memory. J. Neurosci. 30, 5269–5282.
Matthews, D. B., Devaud, L. L., Fritschy, J. M., Sieghart, W., and Morrow, A. L. (1998). Differential regulation of GABA(A) receptor gene expression by ethanol in the rat hippocampus versus cerebral cortex. J. Neurochem. 70, 1160–1166.
Mayfield, R. D., Lewohl, J. M., Dodd, P. R., Herlihy, A., Liu, J., and Harris, R. A. (2002). Patterns of gene expression are altered in the frontal and motor cortices of human alcoholics. J. Neurochem. 81, 802–813.
Mihic, S. J., Ye, Q., Wick, M. J., Koltchine, V. V., Krasowski, M. D., Finn, S. E., Mascia, M. P., Valenzuela, C. F., Hanson, K. K., Greenblatt, E. P., Harris, R. A., and Harrison, N. L. (1997). Sites of alcohol and volatile anaesthetic action on GABA(A) and glycine receptors. Nature 389, 385–389.
Mitsuyama, H., Little, K. Y., Sieghart, W., Devaud, L. L., and Morrow, A. L. (1998). GABA(A) receptor alpha1, alpha4, and beta3 subunit mRNA and protein expression in the frontal cortex of human alcoholics. Alcohol. Clin. Exp. Res. 22, 815–822.
Mody, I., and Pearce, R. A. (2004). Diversity of inhibitory neurotransmission through GABA(A) receptors. Trends Neurosci. 27, 569–575.
Olsen, R. W., Hanchar, H. J., Meera, P., and Wallner, M. (2007). GABAA receptor subtypes: the “one glass of wine” receptors. Alcohol 41, 201–209.
Olsen, R. W., and Sieghart, W. (2008). International union of pharmacology LXX. Subtypes of gamma-aminobutyric acid(A) receptors: classification on the basis of subunit composition, pharmacology, and function. Update. Pharmacol. Rev. 60, 243–260.
Pavlov, I., Savtchenko, L. P., Kullmann, D. M., Semyanov, A., and Walker, M. C. (2009). Outwardly rectifying tonically active GABAA receptors in pyramidal cells modulate neuronal offset, not gain. J. Neurosci. 29, 15341–15350.
Prut, L., Prenosil, G., Willadt, S., Vogt, K., Fritschy, J. M., and Crestani, F. (2010). A reduction in hippocampal GABAA receptor alpha5 subunits disrupts the memory for location of objects in mice. Genes Brain Behav. 9, 478–488.
Puia, G., Ducic, I., Vicini, S., and Costa, E. (1993). Does neurosteroid modulatory efficacy depend on GABAA receptor subunit composition? Receptors Channels 1, 135–142.
Reich, T., Edenberg, H. J., Goate, A., Williams, J. T., Rice, J. P., Van Eerdewegh, P., Foroud, T., Hesselbrock, V., Schuckit, M. A., Bucholz, K., Porjesz, B., Li, T. K., Conneally, P. M., Nurnberger, J. I. Jr., Tischfield, J. A., Crowe, R. R., Cloninger, C. R., Wu, W., Shears, S., Carr, K., Crose, C., Willig, C., and Begleiter, H. (1998). Genome-wide search for genes affecting the risk for alcohol dependence. Am. J. Med. Genet. 81, 207–215.
Rissman, R. A., Mishizen-Eberz, A. J., Carter, T. L., Wolfe, B. B., De Blas, A. L., Miralles, C. P., Ikonomovic, M. D., and Armstrong, D. M. (2003). Biochemical analysis of GABA(A) receptor subunits alpha 1, alpha 5, beta 1, beta 2 in the hippocampus of patients with Alzheimer’s disease neuropathology. Neuroscience 120, 695–704.
Sarviharju, M., Hyytia, P., Hervonen, A., Jaatinen, P., Kiianmaa, K., and Korpi, E. R. (2006). Lifelong ethanol consumption and brain regional GABAA receptor subunit mRNA expression in alcohol-preferring rats. Alcohol 40, 159–166.
Schmittgen, T. D., and Livak, K. J. (2008). Analyzing real-time PCR data by the comparative C(T) method. Nat. Protoc. 3, 1101–1108.
Semyanov, A., Walker, M. C., Kullmann, D. M., and Silver, R. A. (2004). Tonically active GABA A receptors: modulating gain and maintaining the tone. Trends Neurosci. 27, 262–269.
Spanagel, R. (2009). Alcoholism: a systems approach from molecular physiology to addictive behavior. Physiol. Rev. 89, 649–705.
Sperk, G., Schwarzer, C., Tsunashima, K., Fuchs, K., and Sieghart, W. (1997). GABA(A) receptor subunits in the rat hippocampus I: immunocytochemical distribution of 13 subunits. Neuroscience 80, 987–1000.
Steiger, J. L., and Russek, S. J. (2004). GABAA receptors: building the bridge between subunit mRNAs, their promoters, and cognate transcription factors. Pharmacol. Ther. 101, 259–281.
Sullivan, E. V., Marsh, L., Mathalon, D. H., Lim, K. O., and Pfefferbaum, A. (1995). Anterior hippocampal volume deficits in nonamnesic, aging chronic alcoholics. Alcohol. Clin. Exp. Res. 19, 110–122.
Sundstrom-Poromaa, I., Smith, D. H., Gong, Q. H., Sabado, T. N., Li, X., Light, A., Wiedmann, M., Williams, K., and Smith, S. S. (2002). Hormonally regulated alpha(4)beta(2)delta GABA(A) receptors are a target for alcohol. Nat. Neurosci. 5, 721–722.
Thomas, G. J., Harper, C. G., and Dodd, P. R. (1998). Expression of GABA(A) receptor isoform genes in the cerebral cortex of cirrhotic and alcoholic cases assessed by S1 nuclease protection assays. Neurochem. Int. 32, 375–385.
Uusi-Oukari, M., Heikkila, J., Sinkkonen, S. T., Makela, R., Hauer, B., Homanics, G. E., Sieghart, W., Wisden, W., and Korpi, E. R. (2000). Long-range interactions in neuronal gene expression: evidence from gene targeting in the GABA(A) receptor beta2-alpha6-alpha1-gamma2 subunit gene cluster. Mol. Cell. Neurosci. 16, 34–41.
Uusi-Oukari, M., and Korpi, E. R. (2010). Regulation of GABA(A) receptor subunit expression by pharmacological agents. Pharmacol. Rev. 62, 97–135.
Vengeliene, V., Bilbao, A., Molander, A., and Spanagel, R. (2008). Neuropharmacology of alcohol addiction. Br. J. Pharmacol. 154, 299–315.
Volkow, N. D., Wang, G. J., Hitzemann, R., Fowler, J. S., Wolf, A. P., Pappas, N., Biegon, A., and Dewey, S. L. (1993). Decreased cerebral response to inhibitory neurotransmission in alcoholics. Am. J. Psychiatry 150, 417–422.
Volkow, N. D., Wang, G. J., Overall, J. E., Hitzemann, R., Fowler, J. S., Pappas, N., Frecska, E., and Piscani, K. (1997). Regional brain metabolic response to lorazepam in alcoholics during early and late alcohol detoxification. Alcohol. Clin. Exp. Res. 21, 1278–1284.
Wainwright, A., Sirinathsinghji, D. J., and Oliver, K. R. (2000). Expression of GABA(A) receptor alpha5 subunit-like immunoreactivity in human hippocampus. Brain Res. Mol. Brain Res. 80, 228–232.
Wallner, M., Hanchar, H. J., and Olsen, R. W. (2003). Ethanol enhances alpha 4 beta 3 delta and alpha 6 beta 3 delta gamma-aminobutyric acid type A receptors at low concentrations known to affect humans. Proc. Natl. Acad. Sci. U.S.A. 100, 15218–15223.
Wei, W., Faria, L. C., and Mody, I. (2004). Low ethanol concentrations selectively augment the tonic inhibition mediated by delta subunit-containing GABAA receptors in hippocampal neurons. J. Neurosci. 24, 8379–8382.
Appendix
Keywords: alcohol dependence, brain, GABAA channel, post-mortem
Citation: Jin Z, Bazov I, Kononenko O, Korpi ER, Bakalkin G and Birnir B (2012) Selective changes of GABAA channel subunit mRNAs in the hippocampus and orbitofrontal cortex but not in prefrontal cortex of human alcoholics. Front. Cell. Neurosci. 5:30. doi: 10.3389/fncel.2011.00030
Received: 12 October 2011;
Accepted: 14 December 2011;
Published online: 03 January 2012.
Edited by:
Enrico Cherubini, International School for Advanced Studies, ItalyReviewed by:
Istvan Mody, University of California Los Angeles, USARichard Olsen, University of California Los Angeles, USA
Copyright: © 2012 Jin, Bazov, Kononenko, Korpi, Bakalkin and Birnir. This is an open-access article distributed under the terms of the Creative Commons Attribution Non Commercial License, which permits non-commercial use, distribution, and reproduction in other forums, provided the original authors and source are credited.
*Correspondence: Zhe Jin and Bryndis Birnir, The Division of Molecular Physiology and Neuroscience, Department of Neuroscience, BMC, Box 593, Uppsala University, Uppsala, Sweden. e-mail:emhlLmppbkBuZXVyby51dS5zZQ==;YnJ5bmRpcy5iaXJuaXJAbmV1cm8udXUuc2U=