- Department of Engineering of Nervous and Muscular System, Nałęcz Institute of Biocybernetics and Biomedical Engineering, Polish Academy of Sciences, Warsaw, Poland
Introduction
Spike frequency adaptation (SFA) is defined as the decline in motoneuron (MN) firing rate during constant current injection. In classical study of Granit et al. (1963) this phenomenon was investigated in anesthetized animals through injecting MNs with long-lasting rectangular current steps. At least two phases of SFA have been observed in these experiments: the rapid initial phase and the slow (S) late phase (Kernell, 1965).
Historically, the first mechanism proposed to explain SFA was the summation of the medium afterhyperpolarization (Kernell, 1972; Kernell and Sjoholm, 1973; Baldissera et al., 1978). However, it has been shown that this mechanism may be responsible only for few initial MN interspike intervals (Powers et al., 1999). More recent studies of the possible mechanisms underlying late SFA have been usually conducted in vitro and often supported by computer simulations (e.g., Sawczuk et al., 1997; Zeng et al., 2005). In these experiments, a multitude of ion channels were blocked with appropriate pharmacological agents and the effects of blocking on SFA magnitude and/or time course were studied. With this type of protocol, certain mechanisms contributing to SFA were identified in non-MN cells. However, in MNs SFA appears to be such a robust phenomenon that blocking of any presumed mechanisms has no effect on its magnitude or time course. Thus, it was concluded that several membrane channels involved in generating rhythmic MN activity contribute to SFA. These mechanisms act together to ensure SFA stability: blocking one set of channels results in an increase in the contribution of the others (Powers et al., 1999; Zeng et al., 2005).
One piece of evidence supporting this theory of redundancy was presented by Goh et al. (1989) through experiments involving bullfrog sympathetic neurons. Under normal conditions, selective blockade of the delayed rectifier potassium current (IK) has no effect on SFA. However, SFA would be enhanced through blocking IK, when other IKs (including the calcium-dependent current IAHP) were blocked. Thus, the contribution of IK to MN firing patterns depends on the activity of other K+ currents.
Considerable part of the research on SFA was produced by Brownstone's lab (Miles et al., 2005; Brownstone, 2006). Recently, they reported the reversal of SFA during fictive locomotion of decerebrate cat (Brownstone et al., 2011). During the recent meeting of International Motoneurone Community in Sydney the title question of this paper was posed (Brownstone, 2012). The author explains further his concern, asking: “… is repetitive firing produced by current injected through the micropipette … informative about membrane currents during behaviour? Perhaps SFA is not present … during most motor behaviours?” These questions reflect the author's conviction that a role for late SFA has not been established yet.
Human MN studies offer a possibility to investigate intact MNs in their physiological environment. In our opinion, these studies provided enough evidence to answer the questions cited above. This evidence will be presented below.
Initial SFA
The initial SFA phase is present only when the MN starts firing in response to the intracellular rectangular current injection. Since such a rapid depolarization seems to be absolutely non-physiological, this phase is most likely a candidate to be an artefact. However, there is an analogy of rectangular current step in human experiments, when the experimental subject is asked to contract the muscle as quickly as possible (so-called ballistic contractions). This task evokes a sudden increase in synaptic inflow to MN pool, resulting in recruitment of a few MNs with initial firing rates of 60–120 imp/s, which quickly decrease thereafter. The time course of this decrease is similar to those observed in animal MNs in response to constant current injection (Figure 1A).
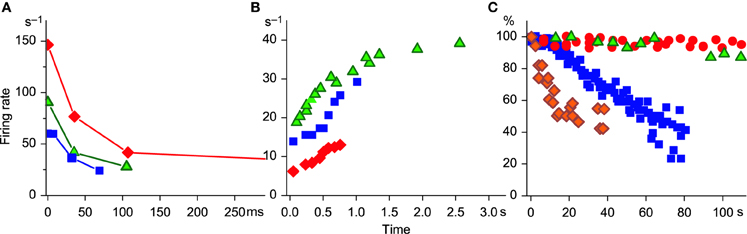
Figure 1. SFA time course. (A) Initial adaptation. Replotted data from Figure 6 of Granit et al. (1963): diamonds 10.2 nA, triangles, 5.7 nA, and Figure 4C (ballistic contraction) of Desmedt and Godaux (1977), squares. (B) Initial acceleration. Replotted data from Spielmann et al. (1993) (triangles, their Figure 3A) and from Desmedt and Godaux (1977), squares (Figure 4C, ramp contraction 12 kG/s); D2 (1.2 kG/s, Figure 4E); diamonds, unpublished data from a MN additionally recruited during sustained contraction. (C) Late adaptation. Squares, MN type F; circles, MN type S: replotted data from Spielmann et al. (1993) (their Figures 5A,B, respectively); diamonds, replotted data from Bigland-Ritchie et al. (1983a), (their Figure 4). Is kindly acknowledged; triangles, (Person and Kudina (1972), their Figure 3). The permission from Wiley and Sons and from Elsevier is kindly acknowledged. Note that the ordinate in (A) and (B) is scaled in cycles per second and in (C) in percent (data normalized by the initial value of firing rate); abscissa in (A) is scaled in milliseconds and in (B) and (C) in seconds.
The reaction of the MN to the rectangular current step was investigated by Ito and Oshima (1965). They showed that cat MNs respond to it with an abrupt change in membrane potential, which initially overshoots the target potential, peaks at approximately 15 ms after current onset and stabilizes around 100 ms. The magnitude of the overshoot increased with increasing current intensity. The time course of the initial SFA phase typically observed with rectangular current stimulation appears to follow changes in membrane voltage: the initial firing rate positively correlates with current step amplitude, and the steady level is reached at or shortly after 100 ms (Granit et al., 1963).
In contrast, during ramp contractions MN firing rate is gradually increasing until the contraction force reaches the target level (Desmedt and Godaux, 1977). Similar firing rate acceleration was observed in cat MNs, when the rectangular current step was applied extracellularly (Spielmann et al., 1993) and during sustained voluntary contractions in human MNs that were additionally recruited as muscle force gradually decreased (Person and Kudina, 1972; Piotrkiewicz et al., 1981) (see Figure 1B for comparison). The authors suggested that in this case the stimulation strength was progressively developing in the MN.
Yet another type of stimulation (intermediate between first two) was applied to cat MNs in the study of Baldissera et al. (1982), who investigated MN firing rate changes following “ramp and hold”-type current injections. The MN responded initially with a gradual increase in firing rate, followed by an overshoot with the peak occurring at approximately the time of transition between the ramp and the constant current, and stabilizing thereafter. The magnitude of this overshoot was positively correlated with the ramp slope and at the S ramps it virtually disappeared. It is conceivable that the transition from fast (F) ramp to steady current would generate a voltage overshoot analogous to that revealed by Ito and Oshima (1965).
Late SFA
Late SFA develops in MN over several minutes and does not depend on the type of excitation, but on the type of muscle fibers that the MN innervates. MNs supplying F muscle fibers have significantly higher initial firing rates and faster late adaptation (Kernell and Monster, 1982; Spielmann et al., 1993) than those supplying S fibers. Firing rate slowing, comparable to this adaptation phase, has also been observed in human MNs during sustained voluntary contractions (Person and Kudina, 1972; Bigland-Ritchie et al., 1983a). In these experiments, the classification of MN to F and S subtypes was not possible. However, it is commonly known that F-type MNs are preferentially recorded during maximal voluntary contractions (MVCs) and those recorded at low force levels usually belong to S-type. During MVC, the firing rate decline was faster for MNs firing at higher rates (Bigland-Ritchie et al., 1983a). According to the recent study by Oya et al. (2009), these MNs also have higher recruitment thresholds. In Figure 1C, the SFA time courses for cat and human MNs are compared. It is obvious that the plot of a high-threshold human MN from the study of Bigland-Ritchie et al. (1983a) is comparable to the plot of cat MN type F, whereas the plot of a human MN recorded during submaximal contraction (Person and Kudina, 1972) appears similar to the plot of MN type S (Spielmann et al., 1993).
The Role of SFA
The reason that SFA in MNs is such a robust phenomenon is presumably related to the unique role of MNs as the Sherrington's “final common pathway” leading to muscle contraction. For MNs to play this role, their properties must be properly matched to those of their muscle units. This matching is indeed observed (Kernell et al., 1999).
As a result of this matching, MN firing rate is adjusted to the requirements of motor task performance. A short initial ISI, for example, is beneficial for smoothness and speed of F contractions (Baldissera and Parmiggiani, 1975; Stein and Parmiggiani, 1979). However, initial SFA is necessary for prolonging the ISI to adjust the firing rate to muscle contractile properties. During precise manipulations, MNs fire in the range close to the maximum slope of the force-rate dependency (Kernell et al., 1983) that corresponds to the maximum tetanic potentiation (Piotrkiewicz and Celichowski, 2007). During sustained MVCs, the MN firing rate should lead to full tetanus of its muscle unit. Although, a MN is able to fire with rates exceeding those necessary for achieving full tetanus, firing at excessive rates is not compatible with optimal contraction control (Bigland-Ritchie et al., 1983a) and may even be destructive to muscle fibers [in Duchenne muscular dystrophy, the pathological process is presumably enhanced by the mismatch between the muscle fiber properties and MN firing rates (Vrbova, 1983)]. Muscle units gradually change their contractile properties during fatigue and become slower, which means that the full tetanus is reached at the lower rates. Thus, the SFA-related decline in the firing rate allows the proper fit between muscle and MN properties to be preserved (Bigland-Ritchie et al., 1983a,b). This concept was denominated “muscle wisdom” by Marsden et al. (1971) and is accepted by the majority of researchers as the rationale for SFA (Spielmann et al., 1993; Zeng et al., 2005; Nordstrom et al., 2007).
Initially, human MN rate slowing during sustained contractions was thought to be mediated by a peripheral reflex from the fatiguing muscle (Bigland-Ritchie et al., 1986; Woods et al., 1987). This hypothesis, however, was later tested and ruled out (Butler et al., 2003; McNeil et al., 2011), which led to the conclusion that for rate slowing intrinsic MN properties were most likely responsible. The same conclusion was reached in the earlier study (Johnson et al., 2004) in which human subjects were instructed to maintain the steady firing rate of a given MN during prolonged contraction. During this task, excitatory input to the MN was substantially increased, indicating that intrinsic MN properties were underlying the observed rate slowing.
Moreover, the match between MN and muscle fiber properties is preserved in normal aging. The firing rate slowing is related to increases in MN AHP duration (Piotrkiewicz et al., 2007, 2012), which corresponds to changes in muscle contractile properties (Frontera et al., 1997).
SFA has usually been studied in animal experiments in response to long-lasting rectangular current steps. The physiological analogue of this artificial condition may occur in extreme situations when the animal or human being is falling from a dangerous height so that survival depends on the ability to quickly catch possible support and to hold on to it long enough for rescue. In this case, SFA would help economize necessary forces.
However, there are certain motor control tasks in which the firing rate decline is not desirable, such as in breathing or locomotion. Rhythmic movements may be continued without fatigue for periods lasting much longer than sustained contractions of constant force. In this case, neuromodulators may reverse SFA through metabotropic pathways. This reversal has been recently observed during fictive locomotion (Brownstone et al., 2011).
In summary, there is adequate evidence that SFA is not an artefact, but rather a manifestation of “neuromuscular wisdom,” which assures proper functioning of the healthy neuromuscular system under all physiologic circumstances.
References
Baldissera, F., Campadelli, P., and Piccinelli, L. (1982). Neural encoding of input transients investigated by intracellular injection of ramp currents in cat alpha-motoneurones. J. Physiol. 328, 73–86.
Baldissera, F., Gustafsson, B., and Parmiggiani, F. (1978). Saturating summation of the afterhyperpolarization conductance in spinal motoneurones: a mechanism for ‘secondary range’ repetitive firing. Brain Res. 146, 69–82.
Baldissera, F., and Parmiggiani, F. (1975). Relevance of motoneuronal firing adaptation to tension development in the motor unit. Brain Res. 91, 315–320.
Bigland-Ritchie, B., Johansson, R., Lippold, O. C., Smith, S., and Woods, J. J. (1983a). Changes in motoneurone firing rates during sustained maximal voluntary contractions. J. Physiol. 340, 335–346.
Bigland-Ritchie, B., Johansson, R., Lippold, O. C., and Woods, J. J. (1983b). Contractile speed and EMG changes during fatigue of sustained maximal voluntary contractions. J. Neurophysiol. 50, 313–324.
Bigland-Ritchie, B. R., Dawson, N. J., Johansson, R. S., and Lippold, O. C. (1986). Reflex origin for the slowing of motoneurone firing rates in fatigue of human voluntary contractions. J. Physiol. 379, 451–459.
Brownstone, R. M. (2006). Beginning at the end: repetitive firing properties in the final common pathway. Prog. Neurobiol. 78, 156–172.
Brownstone, R. M. (2012). “Spike frequency adap-tation in motoneurons: is it an artefact?” in Abstracts of Conference Motoneurons and Beyond, 25. Available online: content/uploads/2011/11/abstracts.pdf
Brownstone, R. M., Krawitz, S., and Jordan, L. M. (2011). Reversal of the late phase of spike frequency adaptation in cat spinal motoneurons during fictive locomotion. J. Neurophysiol. 105, 1045–1050.
Butler, J. E., Taylor, J. L., and Gandevia, S. C. (2003). Responses of human motoneurons to corticospinal stimulation during maximal voluntary contractions and ischemia. J. Neurosci. 23, 10224–10230.
Desmedt, J. E., and Godaux, E. (1977). Ballistic contractions in man: characteristic recruitment pattern of single motor units of the tibialis anterior muscle. J. Physiol. 264, 673–693.
Frontera, W. R., Grimby, L., and Larsson, L. (1997). Firing rate of the lower motoneuron and contractile properties of its muscle fibers after upper motoneuron lesion in man. Muscle Nerve 20, 938–947.
Goh, J. W., Kelly, M. E. M., and Pennefather, P. S. (1989). Electrophysiological function of the delayed rectifier (IK) in bullfrog sympathetic ganglion neurones. Pflugers Arch. 413, 482–486.
Granit, R., Kernell, D., and Shortess, G. K. (1963). Quantitative aspects of repetitive firing of mammalian motoneurones, caused by injected currents. J. Physiol. 168, 911–931.
Ito, M., and Oshima, T. (1965). Electrical behaviour of the motoneurone membrane during intracellularly applied current steps. J. Physiol. 180, 607–635.
Johnson, K. V., Edwards, S. C., Van Tongeren, C., and Bawa, P. (2004). Properties of human motor units after prolonged activity at a constant firing rate. Exp. Brain Res. 154, 479–487.
Kernell, D. (1965). The adaptation and the relation between discharge frequency and current strength of cat lumbosacral motoneurones stimulated by long-lasting injected currents. Acta Physiol. Scand. 65, 65–73.
Kernell, D. (1972). The early phase of adaptation in repetitive impulse discharges of cat spinal motoneurones. Brain Res. 41, 184–186.
Kernell, D., Bakels, R., and Copray, J. C. (1999). Discharge properties of motoneurones: how are they matched to the properties and use of their muscle units? J. Physiol. Paris 93, 87–96.
Kernell, D., Eerbeek, O., and Verhey, B. A. (1983). Relation between isometric force and stimulus rate in cat's hindlimb motor units of different twitch contraction time. Exp. Brain Res. 50, 220–227.
Kernell, D., and Monster, A. W. (1982). Time course and properties of late adaptation in spinal motoneurones of the cat. Exp. Brain Res. 46, 191–196.
Kernell, D., and Sjoholm, H. (1973). Repetitive impulse firing: comparisons between neurone models based on ‘voltage clamp equations’ and spinal motoneurones. Acta Physiol. Scand. 87, 40–56.
Marsden, C. D., Meadows, J. C., and Merton, P. A. (1971). Isolated single motor units in human muscle and their rate of discharge during maximal voluntary effort. J. Physiol. 217, 12P–13P.
McNeil, C. J., Giesebrecht, S., Khan, S. I., Gandevia, S. C., and Taylor, J. L. (2011). The reduction in human motoneurone responsiveness during muscle fatigue is not prevented by increased muscle spindle discharge. J. Physiol. 589, 3731–3738.
Miles, G. B., Dai, Y., and Brownstone, R. M. (2005). Mechanisms underlying the early phase of spike frequency adaptation in mouse spinal motoneurones. J. Physiol. 566, 519–532.
Nordstrom, M. A., Gorman, R. B., Laouris, Y., Spielmann, J. M., and Stuart, D. G. (2007). Does motoneuron adaptation contribute to muscle fatigue? Muscle Nerve 35, 135–158.
Oya, T., Riek, S., and Cresswell, A. G. (2009). Recruitment and rate coding organisation for soleus motor units across entire range of voluntary isometric plantar flexions. J. Physiol. 587, 4737–4748.
Person, R. S., and Kudina, L. P. (1972). Discharge frequency and discharge pattern of human motor units during voluntary contraction of muscle. Electroencephalogr. Clin. Neurophysiol. 32, 471–483.
Piotrkiewicz, M., and Celichowski, J. (2007). Tetanic potentiation in motor units of rat medial gastrocnemius. Acta Neurobiol. Exp. 67, 35–42.
Piotrkiewicz, M., Kudina, L., Chen, J.-J. J., and Hausmanowa-Petrusewicz, I. (2012). Assessment of human motoneuron afterhyperpolarization duration in health and disease. Biocyb. Biomed. Eng. 32, 43–61.
Piotrkiewicz, M., Kudina, L., Mierzejewska, J., Jakubiec, M., and Hausmanowa-Petrusewicz, I. (2007). Age-related change in duration of afterhyperpolarization of human motoneurones. J. Physiol. 585, 483–490.
Piotrkiewicz, M., Lewandowski, A., and Zachara, J. (1981). “An investigation of temporal characteristics of motor unit action potential train,” in Biomechanics VIIB, eds Morecki, A., Fidelus, K., Kędzior, K., Wit, A. (Warsaw/Baltimore: PWN, University Park Press), 31–38.
Powers, R. K., Sawczuk, A., Musick, J. R., and Binder, M. D. (1999). Multiple mechanisms of spike-frequency adaptation in motoneurones. J. Physiol. Paris 93, 101–114.
Sawczuk, A., Powers, R. K., and Binder, M. D. (1997). Contribution of outward currents to spike-frequencyadaptation in hypoglossal motoneurons of the rat. J. Neurophysiol. 78, 2246–2253.
Spielmann, J. M., Laouris, Y., Nordstrom, M. A., Robinson, G. A., Reinking, R. M., and Stuart, D. G. (1993). Adaptation of cat motoneurons to sustained and intermittent extracellular activation. J. Physiol. 464, 75–120.
Stein, R. B., and Parmiggiani, F. (1979). Optimal motor patterns for activating mammalian muscle. Brain Res. 175, 372–376.
Vrbova, G. (1983). Duchenne dystrophy viewed as a disturbance of nerve-muscle interactions. Muscle Nerve 6, 671–675.
Woods, J. J., Furbush, F., and Bigland-Ritchie, B. (1987). Evidence for a fatigue-induced reflex inhibition of motoneuron firing rates. J. Neurophysiol. 58, 125–137.
Citation: Wilanowski G and Piotrkiewicz M (2012) Is spike frequency adaptation an artefact? Insight from human studies. Front. Cell. Neurosci. 6:50. doi: 10.3389/fncel.2012.00050
Received: 05 October 2011; Accepted: 12 October 2012;
Published online: 31 October 2012.
Edited by:
Dieter Wicher, Max Planck Institute for Chemical Ecology, GermanyReviewed by:
Dieter Wicher, Max Planck Institute for Chemical Ecology, GermanyCopyright © 2012 Wilanowski and Piotrkiewicz. This is an open-access article distributed under the terms of the Creative Commons Attribution License, which permits use, distribution and reproduction in other forums, provided the original authors and source are credited and subject to any copyright notices concerning any third-party graphics etc.
*Correspondence:bWFzaWFAaWJpYi53YXcucGw=