- 1Department of Gene Technology, Tallinn University of Technology, Tallinn, Estonia
- 2Biomedicum Functional Genomics Unit, Biomedicum Helsinki, University of Helsinki, Helsinki, Finland
- 3Institute of Biotechnology, University of Helsinki, Helsinki, Finland
Postnatal maturation of the neurons whose main phenotype and basic synaptic contacts are already established includes neuronal growth, refinement of synaptic contacts, final steps of differentiation, programmed cell death period (PCD) etc. In the sympathetic neurons, postnatal maturation includes permanent end of the PCD that occurs with the same time schedule in vivo and in vitro suggesting that the process could be genetically determined. Also many other changes in the neuronal maturation could be permanent and thus based on stable changes in the genome expression. However, postnatal maturation of the neurons is poorly studied. Here we compared the gene expression profiles of immature and mature sympathetic neurons using Affymetrix microarray assay. We found 1310 significantly up-regulated and 1151 significantly down-regulated genes in the mature neurons. Gene ontology analysis reveals up-regulation of genes related to neuronal differentiation, chromatin and epigenetic changes, extracellular factors and their receptors, and cell adhesion, whereas many down-regulated genes were related to metabolic and biosynthetic processes. We show that termination of PCD is not related to major changes in the expression of classical genes for apoptosis or cell survival. Our dataset is deposited to the ArrayExpress database and is a valuable source to select candidate genes in the studies of neuronal maturation. As an example, we studied the changes in the expression of selected genes Igf2bp3, Coro1A, Zfp57, Dcx, and Apaf1 in the young and mature sympathetic ganglia by quantitative PCR and show that these were strongly downregulated in the mature ganglia.
Introduction
Maturation of the neurons occurs when the differentiation is mainly completed and the contacts with synaptic targets are mostly established. It includes enlargement of cell soma, strengthening of cytoskeleton, elaboration and refinement of synaptic contacts and other final steps of differentiation. A remarkable event in the maturating neuronal populations is the programmed cell death (PCD) period, where many neurons die apoptotically, thereby determining the final number of neurons (Oppenheim, 1991). In the neurons that project out of the brain, such as sensory, autonomic and spinal motoneurons the final number is determined by the extent of trophic support provided by target-derived neurotrophic factors (NTFs) that rescue the neurons from apoptosis (Bibel and Barde, 2000; Huang and Reichardt, 2001). The PCD lasts during a relatively short time period and when it ends, the neurons are not apoptotic anymore and do not require trophic support for survival. Compared to the processes of the early neurogenesis, including the cell fate determination, migration, and early differentiation of the neurons during embryogenesis, the postnatal maturation of neurons is poorly studied.
Sympathetic neurons of the superior cervical ganglion (SCG) are one of the best studied models for neuronal development. In mouse, the neural crest-derived precursors form the SCG primordia between embryonic day (E) 11.5–14.5 (Nishino et al., 1999). Most of SCG neurons become postmitotic before birth of the animals (Hendry, 1977; Shi et al., 2008). The majority of SCG neurons are noradrenergic and the main neurotransmitter-synthesizing enzymes tyrosine hydroxylase and dopamine β hydroxylase are expressed already in the early sympathetic precursors (Pattyn et al., 1999). The neuritogenesis, target innervation and synaptogenesis of the sympathetic neurons begin in embryogenesis and continue after the birth (De Champlain et al., 1970; Schotzinger and Landis, 1990). During postnatal maturation, the somata of SCG neurons enlarge and synaptic contacts with the targets are refined. The PCD period in SCG neurons occurs during first two postnatal weeks when about 30% of the neurons die (Wright et al., 1983). Nerve growth factor (NGF), secreted by the targets of innervation in the limited amounts, rescues the neurons that get it (Harrington and Ginty, 2013) whereas neurons that remain without NGF die by unsuppressed apoptosis (Chang et al., 2002; Putcha and Johnson, 2004; Kristiansen et al., 2011; Kristiansen and Ham, 2014). The PCD of the SCG neurons ends by about second postnatal week and the neurons are then not dying any more in the absence of NGF (Kole et al., 2013). How the NGF-dependency disappears during maturation of SCG neurons is not known but it occurs with the same time schedule both in vivo and in the cultured neurons (Easton et al., 1997; Francis and Landis, 1999; Putcha et al., 2000; Glebova and Ginty, 2005; Young et al., 2011) and remains then a persistent trait, obviously via changes in genome activity. Such stable changes in gene expression could most probably be the basis for many other maturational events as well. However, while the early specification and differentiation of sympathetic neurons are extensively studied (Apostolova and Dechant, 2009; Cane and Anderson, 2009; Young et al., 2011), the postnatal maturation has deserved much less attention (Glebova and Ginty, 2005; Kole et al., 2013).
Here we set up on search for significantly changed genes during maturation of the SCG neurons. We compared by microarray gene expression profile of the immature (5 DIV—days in vitro) and mature (21 DIV) cultures of SCG neurons from newborn mice. Our dataset is deposited to the ArrayExpress database and is a valuable source to select candidate genes in the studies of neuronal maturation. As an example, we studied the changes in the expression of five genes that could be related to neuronal maturation, by quantitative PCR (qPCR) on the whole immature and mature SCG ganglia of mice and rats.
Materials and Methods
Neuronal Cultures
All procedures for animal use were approved by the Estonian Ethics Committee and University of Helsinki Laboratory Animal Centre (Protocol number KEK11-020). SCG neurons from newborn NMRI mice were prepared as described earlier (Yu et al., 2003; Hellman et al., 2011; Jakobson et al., 2012, 2013). The neurons were plated onto glass coverslips coated with type IV collagen (BD Biosciences, San Diego, CA, USA, #354233) and grown for 5 DIV (immature) or 21 DIV (mature) in the Neurobasal medium (Invitrogen Ltd, UK) containing B27 serum substitute (Invitrogen) and 30 ng/ml of NGF (PeproTech, Rocky Hill, NJ, USA; #450-01). The culture medium was changed twice a week. The neurons were harvested in phosphate-buffered saline, pelleted and dissolved in the RNeasy RLT lysis buffer (Qiagen, Venlo, Netherlands, #79216). Six independent cultures for mature neurons and three independent cultures for immature neurons (ganglia from 12 to 17 pups per culture) were prepared. The majority of the non-neuronal cells were removed by pre-plating step (Yu et al., 2003; Hellman et al., 2011; Jakobson et al., 2012, 2013) but some always remained and proliferated. The majority of these non-neuronal cells remained attached to the glass coverslips after collection of the cells for RNA extraction. However, to exclude genes of the non-neuronal cells from the analysis with certainty we also collected non-neuronal cells without neurons from the preplating step, that were grown on the non-coated dishes in Neurobasal/B27 medium without NGF for 5 DIV and 21 DIV, one sample for both. No neurons remained alive in these conditions. To verify that the neurons had indeed matured in vitro we tested whether the 21 DIV neurons have become independent of NGF for survival, a clear hallmark of the mature SCG neurons. The 5 DIV or 21 DIV cultures were washed three times with NGF-free medium and function-blocking anti-NGF antibodies (Chemicon MAB5260Z) were added. For comparison, the neurons were washed and re-supplemented with NGF. The original number of living neurons was counted under the inverted microscope immediately after NGF deprivation and again 5 days later. The experiment was repeated two times. On all repeats, all 21 DIV neurons remained alive for 5 additional DIV without or with NGF, as reported by others (Easton et al., 1997; Putcha et al., 2000) whereas the 5 DIV neurons died by NGF deprivation as predicted (Yu et al., 2003; Hellman et al., 2011; Mätlik et al., 2015) (not shown). Typical images are shown on Figure 1. The images were captured with inverted microscope (model DM-IRB; Leica, Germany) using HC PL FLUOTAR objective (10 × /0.30), and a 3CCD color video camera (model DXC-950P; Sony) under the control of Image-Pro Plus software version 3.0 (Media Cybernetics, Inc., Rockville, MD, USA).
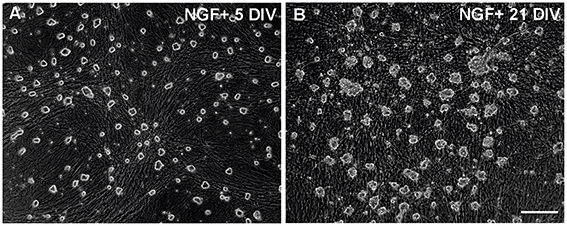
Figure 1. Neuronal cultures used for the microarray study. (A,B) Images of typical 5 DIV and 21 DIV cultures of the SCG neurons taken before cell collection. The 21 DIV cultures contain many non-neuronal cells but most of them remained attached to the glass surface after collection of the neurons. Scale bar, 100 μM.
Array Hybridization
RNeasy Kit (Qiagen) was utilized for the purification of total RNA. Cells were disrupted with lysis buffer and homogenized passing the lysate through 20-gauge needle. 70% ethanol was added and the sample was applied to an RNeasy spin column. The RNA bound the membrane of the column and the contaminants were washed away. Purified RNA was eluted in the water. Sample RNAs were analyzed for integrity and quality on Agilent Bioanalyzer 2100 (Agilent Technologies, Santa Clara, CA, USA). The labeling and hybridization were done using the Ambion WT Expression Kit (Ambion, Austin, TX, USA) and Affymetrix GeneChip WT Terminal Labeling Kit (Affymetrix, Santa Clara, CA, USA), following the manufacturer's instructions. The starting amount of total RNA was 50 ng. 15 μg of cRNA was used for single-stranded cDNA-synthesis. A total of 5.5 μg of single-stranded cDNA was fragmented and hybridized onto a Mouse Exon 1.0 ST Array (Affymetrix, Santa Clara, CA, USA) during 17-h incubation at 45°C. Immediately following hybridization, the array was processed using an automated protocol on the GeneChip® Fluidics Station 450, followed by scanning on a GeneChip® Scanner.
Microarray Data Analysis
Signal values were analyzed using Bioconductor (R). Re-mapped gene annotations from the Brainarray Custom chip description files were used (MoEx10stv1_Mm_ENSG, v.17.1.0, http://brainarray.mbni.med.umich.edu/Brainarray/), and additional gene information was extracted from EnsEMBL using Biomart-package. Robust Multichip Average (RMA) was used for background correction, quantile normalization and summarization of the signal values. Gene expression values were compared between the immature and mature neurons using the moderated t-statistic of limma-package. Benjamini-Hochberg (FDR) procedure was used for multiple testing correction. Genes with FDR adjusted p < 0.05 and fold-change >2 were considered significantly differentially expressed. List of these significantly up- and down-regulated genes were used for gene ontology enrichment analysis, which was performed with web-based DAVID software (http://david.abcc.ncifcrf.gov/) (Huang da et al., 2007).
Real-Time Quantitative PCR analysis
RNA from freshly prepared newborn, 14 days old and 21 days old mouse and Sprague Dawley rat SCG, as well as from total brain was purified with GeneElute Mammalian Total RNA Miniprep kit (Sigma, St. Louis, MO, USA). DNase treatment was done separately. RNA clean-up was done with the same RNA purification kit. cDNA synthesis was performed in 20 μl reaction containing 4 μl of 5x RT buffer (Solis Biodyne, Tartu, Estonia), 2.5 μl of 2.5 mM mix of 4 dNTPs (Solis BioDyne), 1 μl of Oligo (dT)18 primer (Thermo Fisher Scientific, Rockford, IL, USA), 0.2 μl of Random Hexamer Primer (Thermo Fisher Scientific), 0.5 μl of RiboLock RNase Inhibitor (Thermo Fisher Scientific), 0.5 μl of 200 U/μl MMLV Revertase RNase H minus (Solis BioDyne) and 2 μg of RNA in 11.3 μl water. cDNA was synthesized at 37°C for 90 min and kept thereafter for 5 min at 95°C to inactivate the polymerase. The whole reaction mixture was diluted 2x with RNase-free water to standardize the cDNA amount as 1 μg of RNA in 20 μl of cDNA synthesis reaction. The quality of cDNA synthesis was estimated by PCR for cyclophilin G expression.
qPCR analysis was done using Roche Light Cycler 480 II equipment (Roche, Penzberg, Germany). Ten μl reaction mixture contained 5 μl of LightCycler 480 SYBR Green I Master mix (Roche), 10 μM forward and reverse primers (0.5 μl each), 0.8 μl of cDNA (RT mix) and the rest of the volume constituted with RNase-free water. Each reaction was run in triplicates and includes the enzyme activation step 95°C for 4 min continued for 40 times with the following steps: 95°C for 10 s, 56°C for 10 s, 72°C for 10 s. Each run was finished with the melting curve. Neuron-specific Neurofilament H (Nefh) was used as the endogenous control gene. Nefh appears over threshold at Cp 24–25 in our qPCR conditions in all runs. The laboratory golden standard was the 1:1 mixture of RNAs prepared from the newborn and 21 day whole brains. This mixture, representing all the genes analyzed by qPCR, was used as calibrator in every run. Primer sequences are listed in Supplementary file S2. Three or four independent experiments were performed. In each repeat, all genes were analyzed simultaneously on the same PCR run. The means were statistically analyzed with one-way ANOVA followed by Tukey-Kramer Multiple Comparisons Test using GraphPad InStat 3 program (GraphPad Software, Inc., CA, USA).
Results
Microarray Profiling of Gene Expression in Immature and Mature Sympathetic Neurons
To reveal the genes that are differently expressed in the immature and mature SCG neurons the biotin-labeled single-stranded cDNA samples were hybridized to the Affymetrix GeneChips® Mouse Exon 1.0 ST microarrays. To assess the quality of data, all experimental groups: immature 5 DIV neurons (three repeats) mature 21 DIV neurons (six repeats), 5 DIV non-neuronal cells (one repeat) and 21 DIV non-neuronal cells (one repeat) were subjected to principle component analysis (PCA) and hierarchical clustering. The PCA-plot (Figure 2) shows that immature neurons, mature neurons and non-neuronal cells cluster separately from each other but the repeats within these groups cluster together. No obvious outliers were detected. The same conclusions were drawn from hierarchical clustering (not shown).
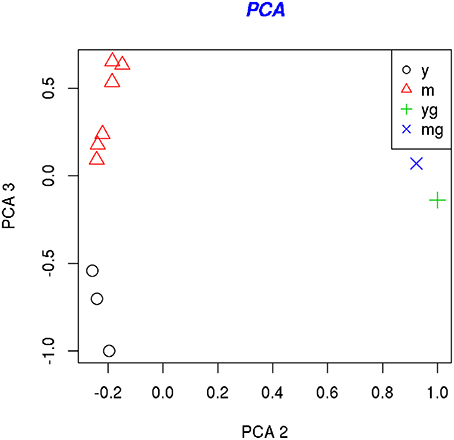
Figure 2. PCA analysis of the samples. PCA-plots show the location of the samples along the first and second principle component (left) and along the second and third principle components (right). The second principal component separates young (5 DIV) (yg) and mature (21 DIV) (mg) glial cell samples from the neuronal samples, whereas the third principal component separates the young (5 DIV) (y) and mature (21 DIV) (m) neuronal samples.
The significance of differential expression between 5 DIV and 21 DIV neuronal samples, as well as between 5 DIV and 21 DIV non-neuronal samples was assessed using the empirical Bayes moderated t-statistics followed by p-value adjustment with false discovery rate (FDR) approach. Importantly, the genes that changed significantly in the non-neuronal cells during 3-week culture almost completely differed from those that changed during 3-week maturation of neurons (not shown). We therefore conclude that the small number of non-neuronal cells remaining in the neuronal samples after cell collection could not significantly affect the interpretation of array data.
In the neuronal samples, of about 26,800 genes analyzed, 2461 changed with adjusted p < 0.05, 1310 of these being up-regulated and 1151 down-regulated. Table 1 shows the number of differentially expressed genes with increasingly stringent cut-off parameters. 39 genes were up-regulated and 30 genes down-regulated more than log 1.5-fold with adjusted p < 0.01. These genes are listed in the Tables 2, 3, respectively. Data of the whole array are available from the ArrayExpress database (http://www.ebi.ac.uk/arrayexpress) under accession number E-MTAB-3221.
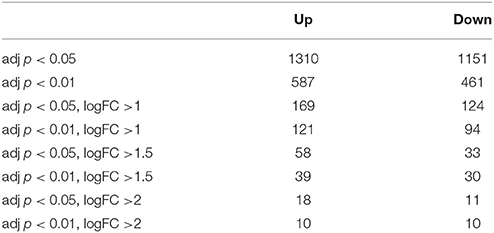
Table 1. Number of significantly up-or down-regulated genes using different adjusted p-values and log fold changes (logFC).
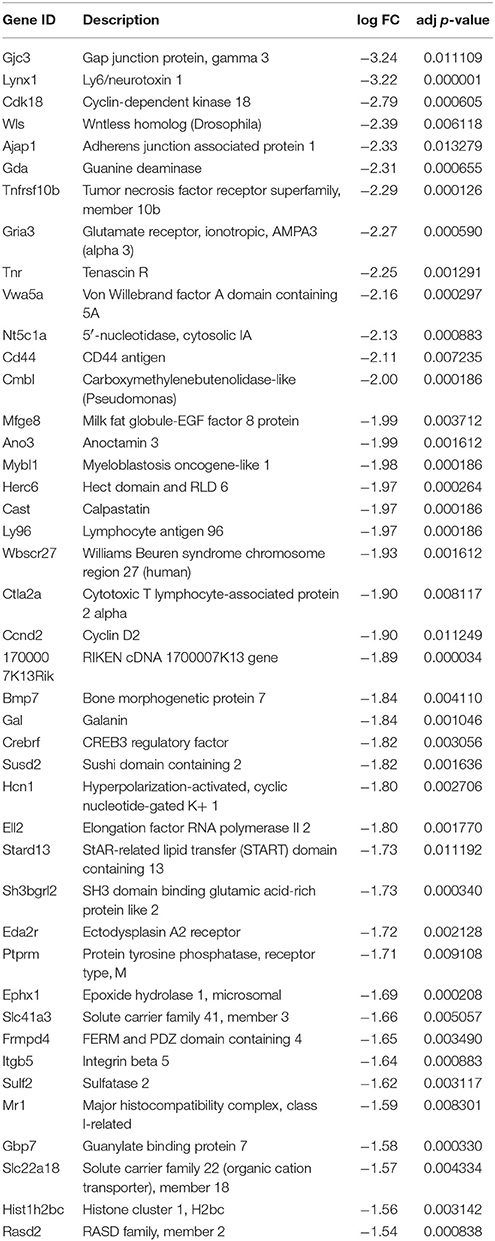
Table 2. Most significantly up-regulated genes in the 21 DIV compared to 5 DIV SCG neurons (adjusted p < 0.01, log fold change >1.5).
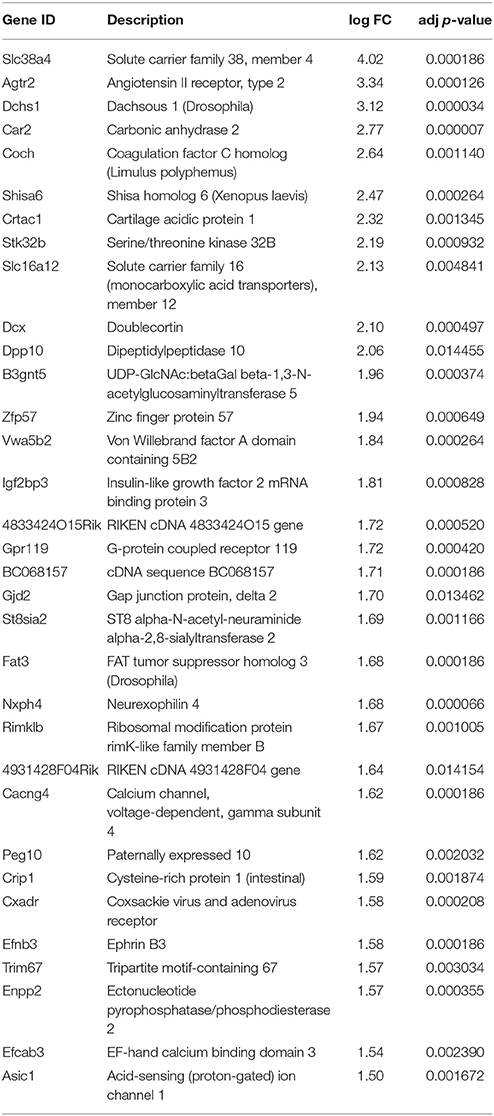
Table 3. Most significantly down-regulated genes in the 21 DIV compared to 5 DIV SCG neurons (adjusted p < 0.01, log fold change >1.5).
There were no significant changes in the genes for classical markers of sympathetic neurons such as th (tyrosine hydroxylase), ddc (dopa decarboxylase), dbh (dopamine beta hydroxylase), npy (neuropeptide Y), Eno2 (neuron-specific enolase), and Slc18a2 (vesicular monoamine transporter 2). We conclude that the main sympathetic phenotype is already developed by the first postnatal week.
Gene Ontology Analysis
To reveal the potential functional trends in our data, differentially expressed genes with adjusted p > 0.05 were associated with Gene Ontology (GO) terms using web-based DAVID software (http://david.abcc.ncifcrf.gov/) (Huang da et al., 2007). Up- and down-regulated genes were analyzed separately. The most abundantly enriched GO-terms among up- and down-regulated genes are presented on Tables 4, 5, respectively. The full cluster analysis is presented in the Supplementary file S1. Of note, many significantly up-regulated GO-terms were related to the neurons, confirming purity of our neuronal samples. As shown in Table 4, the most potently up-regulated GO-terms during SCG neuronal maturation were associated with neuronal differentiation, including the neurites, synapses, ion channels, neurotransmitters etc.
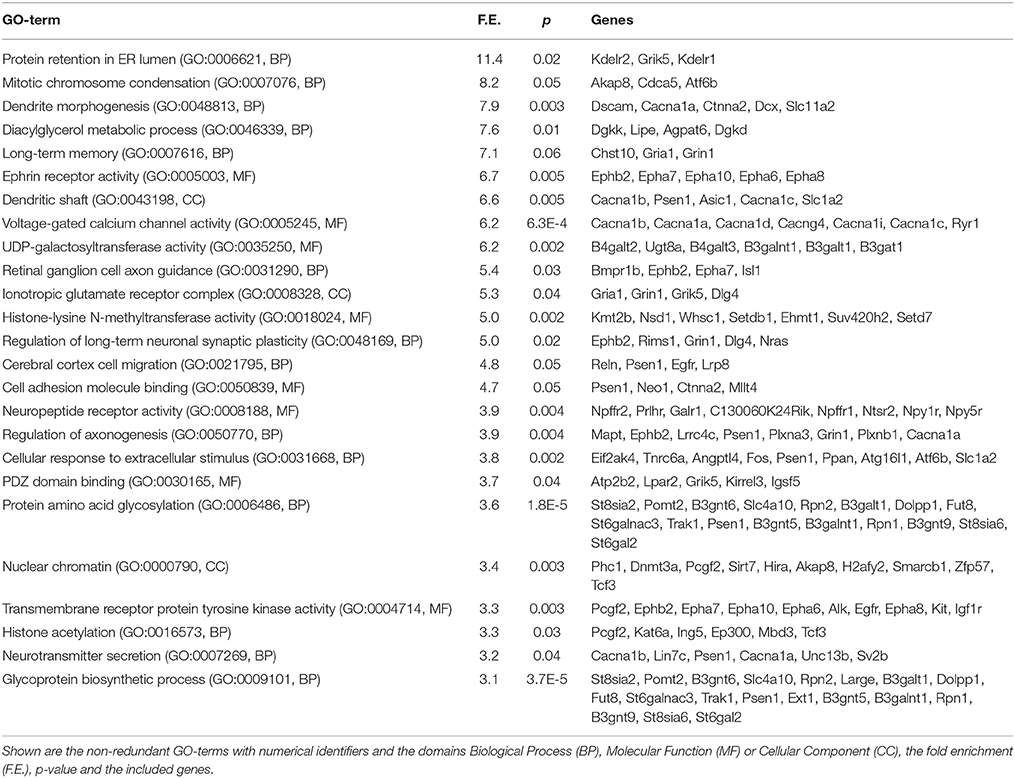
Table 4. Most significantly enriched GO-terms from the genes up-regulated during maturation of the SCG neurons.
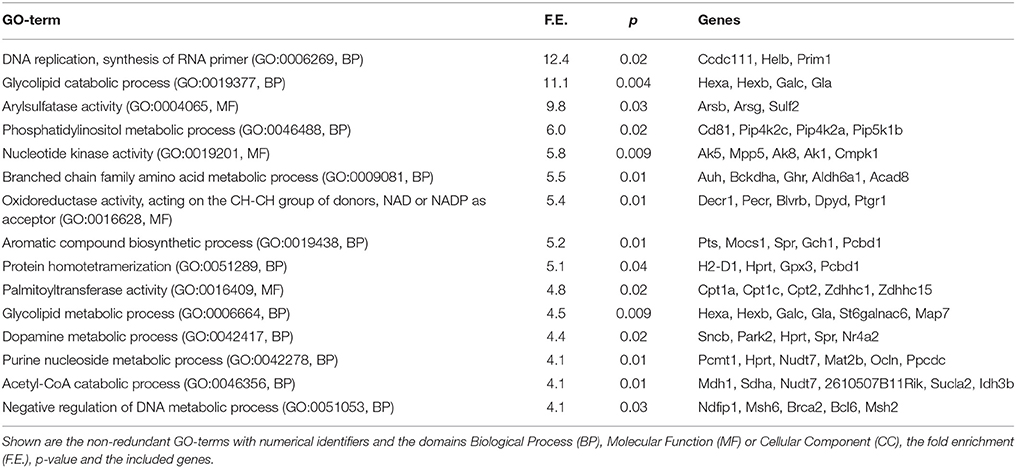
Table 5. Most significantly enriched GO-terms from the genes down-regulated during maturation of the SCG neurons.
The GO-terms related to epigenetic repression of the genome and transcription were among the most significantly up-regulated ones, including the genes involved in chromosome condensation (Akap8, Cdca5), nuclear chromatin (Phc1, Dnmt3a, Pcgf2, Sirt7, Hira, H2afy2, Zfp57), histone acetylation (Pcgf2, Kat6a) and histone methyltransferases (Kmt2b, Nsd1, Whsc1, Setdb1, Ehmt1, Suv420h2). However, some genes related to transcriptional repression (histone deacetylases Hdac8, Hdac9, Hdac11) were down-regulated and some transcriptional activators (Setd7, Kat6a, Ing5, Ep300) up-regulated.
Functional categories related to cell surface receptors (several Eph receptors, Alk, Egfr, Igf1r) and their signaling (cellular response to extracellular stimulus, transmembrane receptor protein tyrosine kinase activity, PZD domain binding) were also significantly enriched among the up-regulated genes. Also, the cell adhesion-related GO-terms were up-regulated during maturation, including the genes Psen1 (presenilin 1), Neo1 (neogenin1), Ctnna2 (catenin, alpha2), Mllt4, Reln (reelin). In contrast, many down-regulated GO-terms appeared to be involved in the various metabolic and biosynthetic processes (Table 5).
Analysis of the Cell Death- and Survival-Related Genes
The ending of PCD is a remarkable event during maturation of the SCG neurons. It is genetically determined (Easton et al., 1997; Francis and Landis, 1999; Putcha et al., 2000; Glebova and Ginty, 2005; Young et al., 2011) but very little is known about its mechanisms and the involved genes (Kole et al., 2011). We therefore paid special attention to the changes in the genes related to cell death and survival. Gene ontology cluster analysis (Supplementary file S1) revealed the GO-terms related to apoptosis and cell death among the down-regulated genes (Table 6) but not among up-regulated genes.
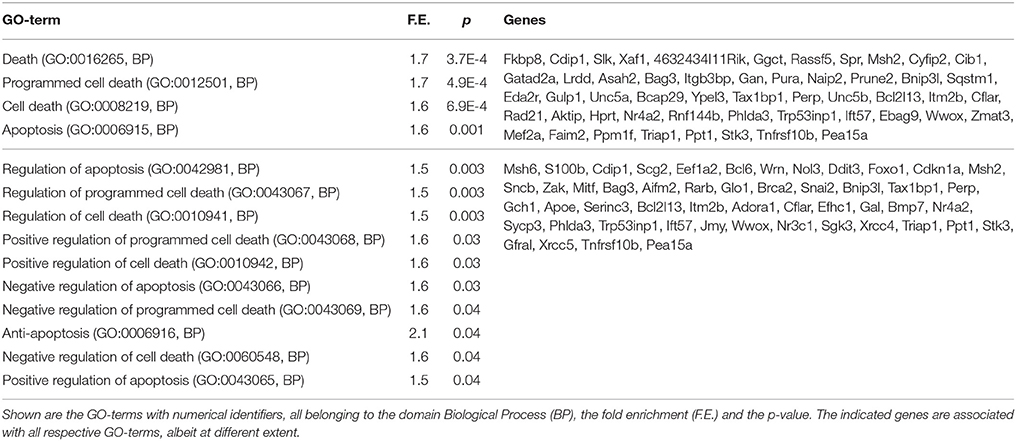
Table 6. GO-terms related to cell death or apoptosis that were significantly down-regulated during maturation of the SCG neurons.
We analyzed changes in the genes known to be essential in the survival or death of the sympathetic or other neurons. Only few of those were significantly changed. Genes for the neurotrophic factors related to sympathetic neurons Ngf, Bdnf, Ntf3 (NT3), Gdnf, Nrtn, Artn and their respective receptors Ntrk2 (TrkB), Ntrk3 (TrkC), Ret, Ngfr (p75NTR), Gfra1, Gfra1, and Gfra3 were not significantly changed during neuronal maturation. Surprisingly, the gene for NGF receptor Ntrk1 (TrkA) was missing from the array. However, activated (phosphorylated) TrkA was shown to be more stable in the mature than immature sympathetic neurons (Tsui-Pierchala and Ginty, 1999) that could contribute to the insensitivity of mature neurons to NGF for survival. We therefore checked the genes reported to be associated with TrkA, such as Appl1 and Gipc1 (Lin et al., 2006), Slc9a5 (Diering et al., 2013), Ccm2 (Harel et al., 2009), Stk25 (Costa et al., 2012), Kidins220 (ARMS) (Sniderhan et al., 2008), Ptpn6 (SHP1) (Marsh et al., 2003), Jakmip2 (Necc2) (Díaz-Ruiz et al., 2013), Pincher (Shao et al., 2002), and Cox (cytochrome oxidase) genes (Vaughn and Deshmukh, 2008). All these genes were not significantly changed, suggesting that regulation of the activity of TrkA could not be the main cause why the mature neurons survive without NGF. However, the levels of Ptpn11, encoding for the phosphatase SHP2 and reported to regulate positively the signaling of TrkB receptors (Araki et al., 2000; Takai et al., 2002), had slightly increased in the mature neurons (Table 4). The genes reported to interact with p75NTR (Roux and Barker, 2002) such as Sort1 (sortilin), Traf6, Maged1 (NRAGE), Zfp110 (NRIF), Ngfrap1 (NADE), and Ndn (Necdin) were also not significantly changed. In conclusion, the independence of the mature SCG neurons from NTFs for survival seems not to be caused by changes in the levels of NTFs, their receptors and known receptor-interacting proteins.
Among the genes for the core apoptotic machinery proteins, including Bcl-2 family members, caspases, Birc (IAP) family members, but also death receptors, their ligands and the proteins of death-inducing signaling complex (DISC) only few changed significantly upon neuronal maturation (Table 7). The proapoptotic protein Bax is critical for the PCD of SCG neurons (Putcha et al., 1999), as in its absence or upon its blockage the neurons do not die by NGF deprivation (Deckwerth et al., 1996; Aalto et al., 2007). As Bax protein is present but not activated by NGF deprivation in the mature SCG neurons (Easton et al., 1997; Putcha et al., 2000; Kole et al., 2011) we analyzed the reported genes for Bax-interacting proteins, such as Xrcc6 (Ku70) (Sawada et al., 2003), mt-Rnr2 (humanin) (Guo et al., 2003), all Ywha (14-3-3) genes (Samuel et al., 2001), Sh3glb1 (Bif-1, endophilin B1) (Wang et al., 2014), Pin1 (Shen et al., 2009), Clu (clusterin) (Zhang et al., 2005), Npm1 (nucleophosmin) (Kerr et al., 2007), Cfl1 (cofilin) (Simonishvili et al., 2013), Tmbim genes (Rojas-Rivera and Hetz, 2014), and Nelfb (Cobra-1) (Ichim et al., 2013) and found that these were not significantly changed during neuronal maturation (except slight increase in Tmbim1). Only few genes for classical cytoplasmic kinases whose activity could promote cell survival changed significantly during neuronal maturation (Table 7), whereas, e.g., Map3k11 (MLK3), Mapk8 (Jnk1), Mapk9 (Jnk2), Akt1, and Akt2, but also phosphatase Dusp1 (Mkp1) (Kristiansen et al., 2010) remained unchanged. Thus, the levels of the members of core apoptotic machinery or classical survival-promoting kinases are not drastically changed during SCG maturation. The mRNA levels of several transcription factors reported to be related to neuronal maturation or apoptosis, such as Trp73 (p73) (Walsh et al., 2004), Trp53 (p53) (Vaughn and Deshmukh, 2007), Jun (Easton et al., 1997; Ham et al., 2000) did not change significantly upon neuronal maturation. However, Nr1d1 (Chomez et al., 2000), Bcl6 (Otaki et al., 2010), Foxo1 (Gilley et al., 2003), and Klf9 (Lebrun et al., 2013) transcripts were all significantly up-regulated in the mature neurons.
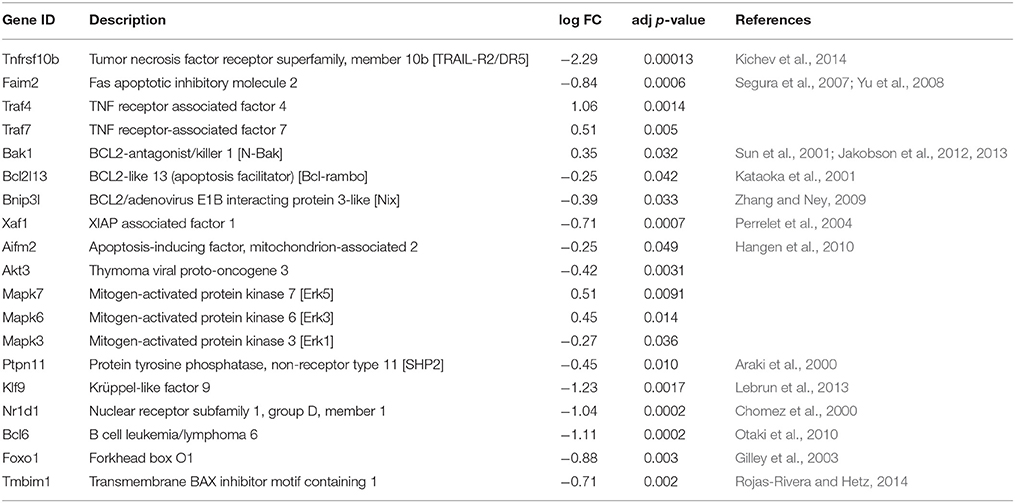
Table 7. Genes related to apoptosis or cell survival that changed significantly during SCG neuronal maturation.
We were surprised to find that core apoptotic machinery was basically not changed in the neurons whose PCD was over. To confirm this finding we analyzed the array data also with Gene Set Enrichment Analysis (GSEA) another web-based method that uses the whole gene sets to interpret the array data and is therefore useful to test specific hypotheses (Supplementary files S3, S4). No gene sets related to apoptosis and cell death were revealed in the young neurons, whereas 11 were found in the mature neurons that, however had very high normalized p-values and FDR's (Supplementary file S4) and are most likely false positives. Thus, also GSEA did not reveal significant changes in the classical apoptotic genes in the mature neurons.
qPCR Analysis of Selected Genes in the Immature and Mature Sympathetic Ganglia Isolated from Mouse and Rat
As an example of the usage of array data we selected five genes of interest: Igf2bp3, Coro1A, Zfp57, Dcx, and Apaf (Table 8) that could potentially be involved in the neuronal maturation and whose expression changes were also revealed by the microarray. Expression of these genes was analyzed by qPCR in the immature and mature ganglia from two species—mouse and rat. To gain insight whether gene expression changes obtained from in vitro cultures reflect the situation in vivo, total RNA from freshly prepared whole ganglia from the newborn, 2 and 3 weeks old mice and rats were analyzed. The data were normalized to the Nefh (neurofilament heavy polypeptide) gene that is expressed only in the neurons and whose expression level did not change significantly in the SCG during the analyzed time points (see the Materials and Methods).
The results are presented on Figure 3. The mRNAs for Igf2bp3, Coro1A, Zpf57, and Dcx were drastically reduced by second week of postnatal development in both animal species, and very low levels of these genes persisted by third postnatal week. All these genes were also significantly down-regulated in the microarray assay (Table 8). Apaf1 mRNA was also significantly down-regulated in the mature neurons as reported by others (Wright et al., 2007), although the change was less significant in the microarray. Thus, the changes in these selected genes determined by microarray in the cultured neurons mostly matched the changes determined by qPCR from the whole ganglia.
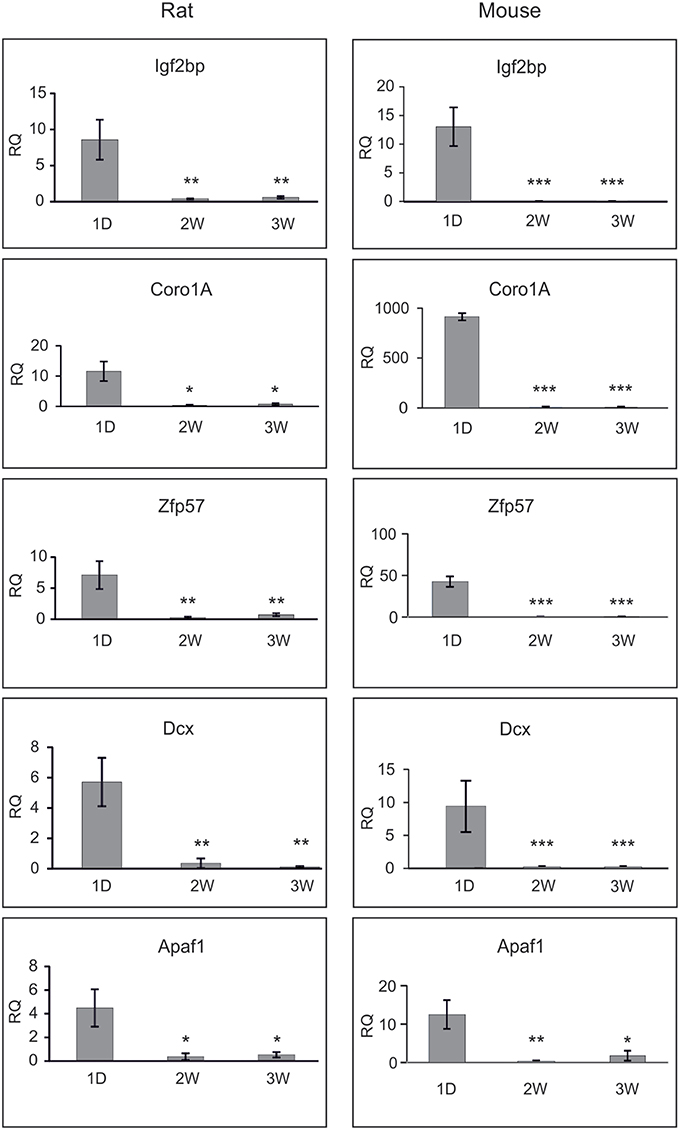
Figure 3. qPCR analysis of the selected genes during maturation of rat SCG. Shown are the relative mRNA quantifies (RQ) from newborn (1D), 2 weeks (2W) and 3 weeks (3W) old rat and mouse SCG, normalized to the levels of Nefh and calibrated against the laboratory golden standard. Three independent analyses (except four for rat Apaf1) were performed and the means were compared with one-way ANOVA followed by Tukey-Kramer Multiple Comparisons Test. Shown are the means ± S.E.M. *p < 0.05; **p > 0.01, ***p > 0.001. The null hypothesis was rejected at p < 0.05.
Discussion
In this study we compared the gene expression profiles of immature and mature sympathetic neurons to get first insight of the poorly studied processes of maturation, including the control of PCD (Kole et al., 2013; Lebrun et al., 2013). To our knowledge, the current analysis is the first one performed on the immature and mature SCG neurons. Many changes that occur during neuronal maturation are permanent, suggesting stable changes in the gene expression. Therefore, our analysis, deposited to the public database, could be a valuable source in the studies of neuronal maturation.
The majority of mouse and rat SCG neurons exit the cell cycle before birth (Hendry, 1977; Shi et al., 2008). Thus, in the neonatal mouse ganglia most of the dividing cells are glial progenitors that give rise to the satellite cells, except a small population of the neurons that became postmitotic during first postnatal week (Shi et al., 2008). The neuritogenesis, target innervation and synaptogenesis of the sympathetic neurons also begin in the embryogenesis but occur mostly during postnatal maturation (De Champlain et al., 1970; Schotzinger and Landis, 1990). This correlates well with our GO analysis showing enrichment of the GO terms related to neurite maturation, synaptic plasticity, neuronal migration, ion channels and neurotransmitter receptors in the mature neurons. Also, the main known markers for the sympathetic neurons (th, ddc, dbh, npy, Eno2, Slc18a2) did not change significantly during maturation, showing that the basic sympathetic phenotype was already established in the mice by birth.
Many GO-terms concerning the modification of chromatin and regulation of transcription were significantly up-regulated showing that the postnatal maturation of the sympathetic ganglia involves epigenetic regulation of gene expression that could be the last steps of terminal differentiation. Most of the genes in these GO-terms have been related to chromatin condensation and gene repression, suggesting that in the mature neurons, chromatin is more repressed than in the immature ones. However, some transcriptional repressors were down-regulated and some transcriptional activators up-regulated, suggesting that some genes are also activated during maturation. The GO-terms related to cell surface receptors, their signaling and cell adhesion molecules were also significantly enriched in the mature neurons, suggesting that extracellular signaling and increased cell adhesion play important role in the maturation of SCG neurons. The most significantly down-regulated GO-terms included genes that are related to the metabolic and biosynthetic processes. It is difficult to conclude from the GO-terms the details of the metabolic changes and we therefore conclude only that the metabolic activity is high in the neonatal SCG neurons but could decrease during their maturation.
The end of PCD is a remarkable event in the postnatal maturation of the SCG neurons that occurs largely via intrinsically determined genetic events rather than the extracellular signals, as it appears in the dissociated cultures with similar time schedule as in the whole ganglia in vivo (Easton et al., 1997; Francis and Landis, 1999; Putcha et al., 2000; Glebova and Ginty, 2005; Young et al., 2011). We therefore paid special attention to the genes related to cell survival and death. Individual checking of the classical genes for apoptosis or cell survival did not reveal major changes in their levels during neuronal maturation, except Apaf1 that was clearly reduced, as also reported by others (Wright et al., 2007). This conclusion was supported by alternative GSEA method that also did not reveal significant changes in the apoptotic and cell death related gene sets. Active killing of the neurons via neurotrophin receptor p75NTR (Deppmann et al., 2008; Hempstead, 2014) or dependence receptors such as Ret (Bordeaux et al., 2000) or TrkC (Tauszig-Delamasure et al., 2007) but also TrkA (Nikoletopoulou et al., 2010) could contribute to neuronal PCD. However, these receptors and the proteins known to associate with them were not significantly down-regulated in the mature SCG neurons and might not be the major mechanism of the NGF-independency. Low levels of Apaf1 (Wright et al., 2007), this study, and repression of transcripts for some Bax-activating BH3-only proteins (Bim, DP5/Hrk, PUMA) by microRNAs in the mature SCG neurons (Kole et al., 2011) certainly contribute to their independence of NGF for survival. However, additional yet to be discovered genes/proteins could be involved in the termination of PCD that is a major process crucially changing the status of the neuron (Kole et al., 2013). Our cluster analysis revealed significant enrichment of the GO-terms related to cell death and apoptosis among down-regulated genes, but not up-regulated genes, suggesting general suppression of the apoptotic program. Notably the apoptotic functions of most of the genes associated with these GO-terms are currently poorly characterized. We propose that the end of PCD is mediated by yet to be found genes whose products control the activity of apoptotic machinery.
Our microarray data offer a good choice to select the candidate genes for the further studies of neuronal maturation. In this study we selected some genes of interest and studied their expression changes in the immature and mature SCG by qPCR. The selected genes changed significantly during neuronal maturation, were revealed by the gene ontology analysis and could potentially participate in this process, but their role in the cell death and neuronal maturation is currently poorly studied. The genes were analyzed in vivo by using neuronal marker gene Nefh as a reference to overcome the confounding effect of non-neuronal cells present in the ganglia but mainly removed from the neuronal cultures used for the microarray analysis. The expression changes of the selected genes analyzed from the in vitro cultures by microassay and from the whole ganglia by qPCR principally matched. We want to stress, however, that such match is not quaranteed, as the culture conditions may change gene expression. E.g., much smaller down-regulation of the Apaf1 gene was revealed by microarray than by qPCR in our study. How the culture conditions affect gene expression in the SCG neurons is currently poorly known and warrants further studies.
Igf2bp3 (insulin-like growth factor 2 mRNA binding protein 3) is an RNA-binding protein, involved in the RNA synthesis, metabolism and stability in different cells including the neurons (Mori et al., 2001). Coro1A, a cytoskeleton binding protein, was shown to bind and regulate TrkA-NGF-containing signaling endosome and thereby increase the survival of the SCG neurons (Suo et al., 2014). We analyzed Dcx (doublecortin), a microtubule-associated protein expressed by migrating immature neurons but down-regulated during neuronal maturation (Francis et al., 1999; Gleeson et al., 1999), and found that it was down-regulated also in the mature SCG. Down-regulation of the mRNA for Apaf1 (apoptotic peptidase activating factor 1) in the mature SCG (Wright et al., 2007) was confirmed here as well. Two transcription factors were selected for further analysis. Zfp57 (zinc finger protein 57) is related to genomic methylation imprinting (Zuo et al., 2012). Our analysis of genes expressed differently in the immature and mature sympathetic neurons could provide a good candidate gene list for further studies addressing the genetic program governing the maturation of neurons.
Author Contributions
MR and JP carried out the preparation of the ganglia and real-time qPCR experiments. ML performed analysis of the array data. UA designed the experiments and wrote the manuscript. All authors read and approved the final manuscript.
Conflict of Interest Statement
The authors declare that the research was conducted in the absence of any commercial or financial relationships that could be construed as a potential conflict of interest.
Acknowledgments
We are indebted to Congjun Zheng for the help in the culture of the neurons. We thank Marianna Školnaja and Tatjana Tanvel for taking care of the laboratory animals and Jaan-Olle Andressoo for critical reading of the manuscript. Biomedicum Functional Genomics Unit, University of Helsinki is acknowledged for RNA extraction, microarray hybridization and data analysis. This study was financed by the European Union through the European Social Fund (Mobilitas grant MTT84), Estonian Research Council grant PUT110 and Academy of Finland program 11186236 (Finnish Centre of Excellence Program 2008–2013).
Supplementary Material
The Supplementary Material for this article can be found online at: http://journal.frontiersin.org/article/10.3389/fncel.2016.00066
Abbreviations
NGF, nerve growth factor; SCG, superior cervical ganglion; PCD, programmed cell death; NTF, neurotrophic factor; FDR, false discovery rate; PCA, principle component analysis; GO, gene ontology; RT, reverse transcriptase; qPCR, quantitative polymerase chain reaction; DIV, days in vitro.
References
Aalto, A. P., Sarin, L. P., van Dijk, A. A., Saarma, M., Poranen, M. M., Arumäe, U., et al. (2007). Large-scale production of dsRNA and siRNA pools for RNA interference utilizing bacteriophage phi6 RNA-dependent RNA polymerase. RNA 13, 422–429. doi: 10.1261/rna.348307
Apostolova, G., and Dechant, G. (2009). Development of neurotransmitter phenotypes in sympathetic neurons. Auton. Neurosci. 151, 30–38. doi: 10.1016/j.autneu.2009.08.012
Araki, T., Yamada, M., Ohnishi, H., Sano, S., Uetsuki, T., and Hatanaka, H. (2000). Shp-2 specifically regulates several tyrosine-phosphorylated proteins in brain-derived neurotrophic factor signaling in cultured cerebral cortical neurons. J. Neurochem. 74, 659–668. doi: 10.1046/j.1471-4159.2000.740659.x
Bibel, M., and Barde, Y. A. (2000). Neurotrophins: key regulators of cell fate and cell shape in the vertebrate nervous system. Genes Dev. 14, 2919–2937. doi: 10.1101/gad.841400
Bordeaux, M. C., Forcet, C., Granger, L., Corset, V., Bidaud, C., Billaud, M., et al. (2000). The RET proto-oncogene induces apoptosis: a novel mechanism for Hirschsprung disease. EMBO J. 19, 4056–4063. doi: 10.1093/emboj/19.15.4056
Cane, K. N., and Anderson, C. R. (2009). Generating diversity: mechanisms regulating the differentiation of autonomic neuron phenotypes. Auton. Neurosci. 151, 17–29. doi: 10.1016/j.autneu.2009.08.010
Chang, L. K., Putcha, G. V., Deshmukh, M., and Johnson, E. M. Jr. (2002). Mitochondrial involvement in the point of no return in neuronal apoptosis. Biochimie 84, 223–231. doi: 10.1016/S0300-9084(02)01372-X
Chomez, P., Neveu, I., Mansén, A., Kiesler, E., Larsson, L., Vennström, B., et al. (2000). Increased cell death and delayed development in the cerebellum of mice lacking the rev-erbA(alpha) orphan receptor. Development 127, 1489–1498.
Costa, B., Kean, M. J., Ast, V., Knight, J. D., Mett, A., Levy, Z., et al. (2012). STK25 protein mediates TrkA and CCM2 protein-dependent death in pediatric tumor cells of neural origin. J. Biol. Chem. 287, 29285–29289. doi: 10.1074/jbc.C112.345397
De Champlain, J., Malmfors, T., Olson, L., and Sachs, C. (1970). Ontogenesis of peripheral adrenergic neurons in the rat: pre- and postnatal observations. Acta Physiol. Scand. 80, 276–288. doi: 10.1111/j.1748-1716.1970.tb04791.x
Deckwerth, T. L., Elliott, J. L., Knudson, C. M., Johnson, E. M. Jr., Snider, W. D., and Korsmeyer, S. J. (1996). BAX is required for neuronal death after trophic factor deprivation and during development. Neuron 17, 401–411. doi: 10.1016/S0896-6273(00)80173-7
Deppmann, C. D., Mihalas, S., Sharma, N., Lonze, B. E., Niebur, E., and Ginty, D. D. (2008). A model for neuronal competition during development. Science 320, 369–373. doi: 10.1126/science.1152677
Díaz-Ruiz, A., Rabanal-Ruiz, Y., Trávez, A., Gracia-Navarro, F., Cruz-García, D., Montero-Hadjadje, M., et al. (2013). The long coiled-coil protein NECC2 is associated to caveolae and modulates NGF/TrkA signaling in PC12 cells. PLoS ONE 8:e73668. doi: 10.1371/journal.pone.0073668
Diering, G. H., Numata, Y., Fan, S., Church, J., and Numata, M. (2013). Endosomal acidification by Na+/H+ exchanger NHE5 regulates TrkA cell-surface targeting and NGF-induced PI3K signaling. Mol. Biol. Cell 24, 3435–3448. doi: 10.1091/mbc.E12-06-0445
Easton, R. M., Deckwerth, T. L., Parsadanian, A. S., and Johnson, E. M. Jr. (1997). Analysis of the mechanism of loss of trophic factor dependence associated with neuronal maturation: a phenotype indistinguishable from Bax deletion. J. Neurosci. 17, 9656–9666.
Francis, F., Koulakoff, A., Boucher, D., Chafey, P., Schaar, B., Vinet, M. C., et al. (1999). Doublecortin is a developmentally regulated, microtubule-associated protein expressed in migrating and differentiating neurons. Neuron 23, 247–256. doi: 10.1016/S0896-6273(00)80777-1
Francis, N. J., and Landis, S. C. (1999). Cellular and molecular determinants of sympathetic neuron development. Annu. Rev. Neurosci. 22, 541–566. doi: 10.1146/annurev.neuro.22.1.541
Gilley, J., Coffer, P. J., and Ham, J. (2003). FOXO transcription factors directly activate bim gene expression and promote apoptosis in sympathetic neurons. J. Cell Biol. 162, 613–622. doi: 10.1083/jcb.200303026
Glebova, N. O., and Ginty, D. D. (2005). Growth and survival signals controlling sympathetic nervous system development. Annu. Rev. Neurosci. 28, 191–222. doi: 10.1146/annurev.neuro.28.061604.135659
Gleeson, J. G., Lin, P. T., Flanagan, L. A., and Walsh, C. A. (1999). Doublecortin is a microtubule-associated protein and is expressed widely by migrating neurons. Neuron 23, 257–271. doi: 10.1016/S0896-6273(00)80778-3
Guo, B., Zhai, D., Cabezas, E., Welsh, K., Nouraini, S., Satterthwait, A. C., et al. (2003). Humanin peptide suppresses apoptosis by interfering with Bax activation. Nature 423, 456–461. doi: 10.1038/nature01627
Ham, J., Eilers, A., Whitfield, J., Neame, S. J., and Shah, B. (2000). c-Jun and the transcriptional control of neuronal apoptosis. Biochem. Pharmacol. 60, 1015–1021. doi: 10.1016/S0006-2952(00)00372-5
Hangen, E., De Zio, D., Bordi, M., Zhu, C., Dessen, P., Caffin, F., et al. (2010). A brain-specific isoform of mitochondrial apoptosis-inducing factor: AIF2. Cell Death Differ. 17, 1155–1166. doi: 10.1038/cdd.2009.211
Harel, L., Costa, B., Tcherpakov, M., Zapatka, M., Oberthuer, A., Hansford, L. M., et al. (2009). CCM2 mediates death signaling by the TrkA receptor tyrosine kinase. Neuron 63, 585–591. doi: 10.1016/j.neuron.2009.08.020
Harrington, A. W., and Ginty, D. D. (2013). Long-distance retrograde neurotrophic factor signalling in neurons. Nat. Rev. Neurosci. 14, 177–187. doi: 10.1038/nrn3253
Hellman, M., Arumäe, U., Yu, L. Y., Lindholm, P., Peranen, J., Saarma, M., et al. (2011). Mesencephalic astrocyte-derived neurotrophic factor (MANF) has a unique mechanism to rescue apoptotic neurons. J. Biol. Chem. 286, 2675–2680. doi: 10.1074/jbc.M110.146738
Hempstead, B. L. (2014). Deciphering proneurotrophin actions. Handb. Exp. Pharmacol. 220, 17–32. doi: 10.1007/978-3-642-45106-5_2
Hendry, I. A. (1977). Cell division in the developing sympathetic nervous system. J. Neurocytol. 6, 299–309. doi: 10.1007/BF01175193
Huang, E. J., and Reichardt, L. F. (2001). Neurotrophins: roles in neuronal development and function. Annu. Rev. Neurosci. 24, 677–736. doi: 10.1146/annurev.neuro.24.1.677
Huang, D. W., Sherman, B. T., Tan, Q., Collins, J. R., Alvord, W. G., Roayaei, J., et al. (2007). The DAVID Gene Functional Classification Tool: a novel biological module-centric algorithm to functionally analyze large gene lists. Genome Biol. 8:R183. doi: 10.1186/gb-2007-8-9-r183
Ichim, G., Genevois, A. L., Ménard, M., Yu, L. Y., Coelho-Aguiar, J. M., Llambi, F., et al. (2013). The dependence receptor TrkC triggers mitochondria-dependent apoptosis upon Cobra-1 recruitment. Mol. Cell 51, 632–646. doi: 10.1016/j.molcel.2013.08.021
Jakobson, M., Jakobson, M., Llano, O., Palgi, J., and Arumäe, U. (2013). Multiple mechanisms repress N-Bak mRNA translation in the healthy and apoptotic neurons. Cell Death Dis. 4:e777. doi: 10.1038/cddis.2013.297
Jakobson, M., Lintulahti, A., and Arumäe, U. (2012). mRNA for N-Bak, a neuron-specific BH3-only splice isoform of Bak, escapes nonsense-mediated decay and is translationally repressed in the neurons. Cell Death Dis. 3:e269. doi: 10.1038/cddis.2012.4
Kataoka, T., Holler, N., Micheau, O., Martinon, F., Tinel, A., Hofmann, K., et al. (2001). Bcl-rambo, a novel Bcl-2 homologue that induces apoptosis via its unique C-terminal extension. J. Biol. Chem. 276, 19548–19554. doi: 10.1074/jbc.M010520200
Kerr, L. E., Birse-Archbold, J. L., Short, D. M., McGregor, A. L., Heron, I., Macdonald, D. C., et al. (2007). Nucleophosmin is a novel Bax chaperone that regulates apoptotic cell death. Oncogene 26, 2554–2562. doi: 10.1038/sj.onc.1210044
Kichev, A., Rousset, C. I., Baburamani, A. A., Levison, S. W., Wood, T. L., Gressens, P., et al. (2014). Tumor necrosis factor-related apoptosis-inducing ligand (TRAIL) signaling and cell death in the immature central nervous system after hypoxia-ischemia and inflammation. J. Biol. Chem. 289, 9430–9439. doi: 10.1074/jbc.M113.512350
Kole, A. J., Annis, R. P., and Deshmukh, M. (2013). Mature neurons: equipped for survival. Cell Death Dis. 4:e689. doi: 10.1038/cddis.2013.220
Kole, A. J., Swahari, V., Hammond, S. M., and Deshmukh, M. (2011). miR-29b is activated during neuronal maturation and targets BH3-only genes to restrict apoptosis. Genes Dev. 25, 125–130. doi: 10.1101/gad.1975411
Kristiansen, M., and Ham, J. (2014). Programmed cell death during neuronal development: the sympathetic neuron model. Cell Death Differ. 21, 1025–1035. doi: 10.1038/cdd.2014.47
Kristiansen, M., Hughes, R., Patel, P., Jacques, T. S., Clark, A. R., and Ham, J. (2010). Mkp1 is a c-Jun target gene that antagonizes JNK-dependent apoptosis in sympathetic neurons. J. Neurosci. 30, 10820–10832. doi: 10.1523/JNEUROSCI.2824-10.2010
Kristiansen, M., Menghi, F., Hughes, R., Hubank, M., and Ham, J. (2011). Global analysis of gene expression in NGF-deprived sympathetic neurons identifies molecular pathways associated with cell death. BMC Genomics 12:551. doi: 10.1186/1471-2164-12-551
Lebrun, C., Avci, H. X., Wehrlé, R., Doulazmi, M., Jaudon, F., Morel, M. P., et al. (2013). Klf9 is necessary and sufficient for Purkinje cell survival in organotypic culture. Mol. Cell. Neurosci. 54, 9–21. doi: 10.1016/j.mcn.2012.11.010
Lin, D. C., Quevedo, C., Brewer, N. E., Bell, A., Testa, J. R., Grimes, M. L., et al. (2006). APPL1 associates with TrkA and GIPC1 and is required for nerve growth factor-mediated signal transduction. Mol. Cell. Biol. 26, 8928–8941. doi: 10.1128/MCB.00228-06
Marsh, H. N., Dubreuil, C. I., Quevedo, C., Lee, A., Majdan, M., Walsh, G. S., et al. (2003). SHP-1 negatively regulates neuronal survival by functioning as a TrkA phosphatase. J. Cell Biol. 163, 999–1010. doi: 10.1083/jcb.200309036
Mätlik, K., Yu, L. Y., Eesmaa, A., Hellman, M., Lindholm, P., Peränen, J., et al. (2015). Role of two sequence motifs of mesencephalic astrocyte-derived neurotrophic factor in its survival-promoting activity. Cell Death Dis. 6:e2032. doi: 10.1038/cddis.2015.371
Mori, H., Sakakibara, S., Imai, T., Nakamura, Y., Iijima, T., Suzuki, A., et al. (2001). Expression of mouse igf2 mRNA-binding protein 3 and its implications for the developing central nervous system. J. Neurosci. Res. 64, 132–143. doi: 10.1002/jnr.1060
Nikoletopoulou, V., Lickert, H., Frade, J. M., Rencurel, C., Giallonardo, P., Zhang, L., et al. (2010). Neurotrophin receptors TrkA and TrkC cause neuronal death whereas TrkB does not. Nature 467, 59–63. doi: 10.1038/nature09336
Nishino, J., Mochida, K., Ohfuji, Y., Shimazaki, T., Meno, C., Ohishi, S., et al. (1999). GFR alpha3, a component of the artemin receptor, is required for migration and survival of the superior cervical ganglion. Neuron 23, 725–736. doi: 10.1016/S0896-6273(01)80031-3
Oppenheim, R. W. (1991). Cell death during development of the nervous system. Annu. Rev. Neurosci. 14, 453–501. doi: 10.1146/annurev.ne.14.030191.002321
Otaki, J. M., Hatano, M., Matayoshi, R., Tokuhisa, T., and Yamamoto, H. (2010). The proto-oncogene BCL6 promotes survival of olfactory sensory neurons. Dev. Neurobiol. 70, 424–435. doi: 10.1002/dneu.20786
Pattyn, A., Morin, X., Cremer, H., Goridis, C., and Brunet, J. F. (1999). The homeobox gene Phox2b is essential for the development of autonomic neural crest derivatives. Nature 399, 366–370. doi: 10.1038/20700
Perrelet, D., Perrin, F. E., Liston, P., Korneluk, R. G., MacKenzie, A., Ferrer-Alcon, M., et al. (2004). Motoneuron resistance to apoptotic cell death in vivo correlates with the ratio between X-linked inhibitor of apoptosis proteins (XIAPs) and its inhibitor, XIAP-associated factor 1. J. Neurosci. 24, 3777–3785. doi: 10.1523/JNEUROSCI.0413-04.2004
Putcha, G. V., Deshmukh, M., and Johnson, E. M. Jr. (1999). BAX translocation is a critical event in neuronal apoptosis: regulation by neuroprotectants, BCL-2, and caspases. J. Neurosci. 19, 7476–7485.
Putcha, G. V., Deshmukh, M., and Johnson, E. M. Jr. (2000). Inhibition of apoptotic signaling cascades causes loss of trophic factor dependence during neuronal maturation. J. Cell Biol. 149, 1011–1018. doi: 10.1083/jcb.149.5.1011
Putcha, G. V., and Johnson, E. M. Jr. (2004). Men are but worms: neuronal cell death in C elegans and vertebrates. Cell Death Differ. 11, 38–48. doi: 10.1038/sj.cdd.4401352
Rojas-Rivera, D., and Hetz, C. (2014). TMBIM protein family: ancestral regulators of cell death. Oncogene. 34, 269–280. doi: 10.1038/onc.2014.6
Roux, P. P., and Barker, P. A. (2002). Neurotrophin signaling through the p75 neurotrophin receptor. Prog. Neurobiol. 67, 203–233. doi: 10.1016/S0301-0082(02)00016-3
Samuel, T., Weber, H. O., Rauch, P., Verdoodt, B., Eppel, J. T., McShea, A., et al. (2001). The G2/M regulator 14-3-3sigma prevents apoptosis through sequestration of Bax. J. Biol. Chem. 276, 45201–45206. doi: 10.1074/jbc.M106427200
Sawada, M., Sun, W., Hayes, P., Leskov, K., Boothman, D. A., and Matsuyama, S. (2003). Ku70 suppresses the apoptotic translocation of Bax to mitochondria. Nat. Cell Biol. 5, 320–329. doi: 10.1038/ncb950
Schotzinger, R. J., and Landis, S. C. (1990). Acquisition of cholinergic and peptidergic properties by sympathetic innervation of rat sweat glands requires interaction with normal target. Neuron 5, 91–100. doi: 10.1016/0896-6273(90)90037-G
Segura, M. F., Sole, C., Pascual, M., Moubarak, R. S., Perez-Garcia, M. J., Gozzelino, R., et al. (2007). The long form of Fas apoptotic inhibitory molecule is expressed specifically in neurons and protects them against death receptor-triggered apoptosis. J. Neurosci. 27, 11228–11241. doi: 10.1523/JNEUROSCI.3462-07.2007
Shao, Y., Akmentin, W., Toledo-Aral, J. J., Rosenbaum, J., Valdez, G., Cabot, J. B., et al. (2002). Pincher, a pinocytic chaperone for nerve growth factor/TrkA signaling endosomes. J. Cell Biol. 157, 679–691. doi: 10.1083/jcb.200201063
Shen, Z. J., Esnault, S., Schinzel, A., Borner, C., and Malter, J. S. (2009). The peptidyl-prolyl isomerase Pin1 facilitates cytokine-induced survival of eosinophils by suppressing Bax activation. Nat. Immunol. 10, 257–265. doi: 10.1038/ni.1697
Shi, H., Cui, H., Alam, G., Gunning, W. T., Nestor, A., Giovannucci, D., et al. (2008). Nestin expression defines both glial and neuronal progenitors in postnatal sympathetic ganglia. J. Comp. Neurol. 508, 867–878. doi: 10.1002/cne.21719
Simonishvili, S., Jain, M. R., Li, H., Levison, S. W., and Wood, T. L. (2013). Identification of Bax-interacting proteins in oligodendrocyte progenitors during glutamate excitotoxicity and perinatal hypoxia-ischemia. ASN Neuro 5:e00131. doi: 10.1042/AN20130027
Sniderhan, L. F., Stout, A., Lu, Y., Chao, M. V., and Maggirwar, S. B. (2008). Ankyrin-rich membrane spanning protein plays a critical role in nuclear factor-kappa B signaling. Mol. Cell. Neurosci. 38, 404–416. doi: 10.1016/j.mcn.2008.04.001
Sun, Y. F., Yu, L. Y., Saarma, M., Timmusk, T., and Arumae, U. (2001). Neuron-specific Bcl-2 homology 3 domain-only splice variant of Bak is anti-apoptotic in neurons, but pro-apoptotic in non-neuronal cells. J. Biol. Chem. 276, 16240–16247. doi: 10.1074/jbc.M010419200
Suo, D., Park, J., Harrington, A. W., Zweifel, L. S., Mihalas, S., and Deppmann, C. D. (2014). Coronin-1 is a neurotrophin endosomal effector that is required for developmental competition for survival. Nat. Neurosci. 17, 36–45. doi: 10.1038/nn.3593
Takai, S., Yamada, M., Araki, T., Koshimizu, H., Nawa, H., and Hatanaka, H. (2002). Shp-2 positively regulates brain-derived neurotrophic factor-promoted survival of cultured ventral mesencephalic dopaminergic neurons through a brain immunoglobulin-like molecule with tyrosine-based activation motifs/Shp substrate-1. J. Neurochem. 82, 353–364. doi: 10.1046/j.1471-4159.2002.00960.x
Tauszig-Delamasure, S., Yu, L. Y., Cabrera, J. R., Bouzas-Rodriguez, J., Mermet-Bouvier, C., Guix, C., et al. (2007). The TrkC receptor induces apoptosis when the dependence receptor notion meets the neurotrophin paradigm. Proc. Natl. Acad. Sci. U.S.A. 104, 13361–13366. doi: 10.1073/pnas.0701243104
Tsui-Pierchala, B. A., and Ginty, D. D. (1999). Characterization of an NGF-P-TrkA retrograde-signaling complex and age-dependent regulation of TrkA phosphorylation in sympathetic neurons. J. Neurosci. 19, 8207–8218.
Vaughn, A. E., and Deshmukh, M. (2007). Essential postmitochondrial function of p53 uncovered in DNA damage-induced apoptosis in neurons. Cell Death Differ. 14, 973–981. doi: 10.1038/sj.cdd.4402084
Vaughn, A. E., and Deshmukh, M. (2008). Glucose metabolism inhibits apoptosis in neurons and cancer cells by redox inactivation of cytochrome c. Nat. Cell Biol. 10, 1477–1483. doi: 10.1038/ncb1807
Walsh, G. S., Orike, N., Kaplan, D. R., and Miller, F. D. (2004). The invulnerability of adult neurons: a critical role for p73. J. Neurosci. 24, 9638–9647. doi: 10.1523/JNEUROSCI.1299-04.2004
Wang, D. B., Uo, T., Kinoshita, C., Sopher, B. L., Lee, R. J., Murphy, S. P., et al. (2014). Bax interacting factor-1 promotes survival and mitochondrial elongation in neurons. J. Neurosci. 34, 2674–2683. doi: 10.1523/JNEUROSCI.4074-13.2014
Wright, K. M., Smith, M. I., Farrag, L., and Deshmukh, M. (2007). Chromatin modification of Apaf-1 restricts the apoptotic pathway in mature neurons. J. Cell Biol. 179, 825–832. doi: 10.1083/jcb.200708086
Wright, L. L., Cunningham, T. J., and Smolen, A. J. (1983). Developmental neuron death in the rat superior cervical sympathetic ganglion: cell counts and ultrastructure. J. Neurocytol. 12, 727–738. doi: 10.1007/BF01258147
Young, H. M., Cane, K. N., and Anderson, C. R. (2011). Development of the autonomic nervous system: a comparative view. Auton. Neurosci. 165, 10–27. doi: 10.1016/j.autneu.2010.03.002
Yu, L. Y., Jokitalo, E., Sun, Y. F., Mehlen, P., Lindholm, D., Saarma, M., et al. (2003). GDNF-deprived sympathetic neurons die via a novel nonmitochondrial pathway. J. Cell Biol. 163, 987–997. doi: 10.1083/jcb.200305083
Yu, L. Y., Saarma, M., and Arumäe, U. (2008). Death receptors and caspases but not mitochondria are activated in the GDNF- or BDNF-deprived dopaminergic neurons. J. Neurosci. 28, 7467–7475. doi: 10.1523/JNEUROSCI.1877-08.2008
Zhang, H., Kim, J. K., Edwards, C. A., Xu, Z., Taichman, R., and Wang, C. Y. (2005). Clusterin inhibits apoptosis by interacting with activated Bax. Nat. Cell Biol. 7, 909–915. doi: 10.1038/ncb1291
Zhang, J., and Ney, P. A. (2009). Role of BNIP3 and NIX in cell death, autophagy, and mitophagy. Cell Death Differ. 16, 939–946. doi: 10.1038/cdd.2009.16
Zuo, X., Sheng, J., Lau, H. T., McDonald, C. M., Andrade, M., Cullen, D. E., et al. (2012). Zinc finger protein ZFP57 requires its co-factor to recruit DNA methyltransferases and maintains DNA methylation imprint in embryonic stem cells via its transcriptional repression domain. J. Biol. Chem. 287, 2107–2118. doi: 10.1074/jbc.M111.322644
Keywords: sympathetic neurons, maturation, microarray, programmed cell death, nerve growth factor
Citation: Raba M, Palgi J, Lehtivaara M and Arumäe U (2016) Microarray Analysis Reveals Increased Transcriptional Repression and Reduced Metabolic Activity but Not Major Changes in the Core Apoptotic Machinery during Maturation of Sympathetic Neurons. Front. Cell. Neurosci. 10:66. doi: 10.3389/fncel.2016.00066
Received: 06 December 2015; Accepted: 01 March 2016;
Published: 16 March 2016.
Edited by:
James Francis Curtin, Dublin Institute of Technology, IrelandReviewed by:
Elsa Fabbretti, University of Nova Gorica, SloveniaEva Benito, Deutsches Zentrum Für Neurodegenerative Erkrankungen, Germany
Copyright © 2016 Raba, Palgi, Lehtivaara and Arumäe. This is an open-access article distributed under the terms of the Creative Commons Attribution License (CC BY). The use, distribution or reproduction in other forums is permitted, provided the original author(s) or licensor are credited and that the original publication in this journal is cited, in accordance with accepted academic practice. No use, distribution or reproduction is permitted which does not comply with these terms.
*Correspondence: Urmas Arumäe, dXJtYXMuYXJ1bWFlQHR0dS5lZQ==