- 1Department of Anatomy and Cell Biology, University of Pennsylvania, Philadelphia, PA, United States
- 2Department of Orthodontics, University of Pennsylvania, Philadelphia, PA, United States
- 3Department of Pharmacology and Chemical Biology, Pittsburgh University, Pittsburgh, PA, United States
- 4Department of Ophthalmology, University of Pennsylvania, Philadelphia, PA, United States
- 5Department of Physiology, University of Pennsylvania, Philadelphia, PA, United States
Inflammatory responses play a key role in many neural pathologies, with localized signaling from the non-immune cells making critical contributions. The NLRP3 inflammasome is an important component of innate immune signaling and can link neural insult to chronic inflammation. The NLRP3 inflammasome requires two stages to contribute: priming and activation. The priming stage involves upregulation of inflammasome components while the activation stage results in the assembly and activation of the inflammasome complex. The priming step can be rate limiting and can connect insult to chronic inflammation, but our knowledge of the signals that regulate NLRP3 inflammasome priming in sterile inflammation is limited. This study examined the link between mechanical strain and inflammasome priming in neural systems. Transient non-ischemic elevation of intraocular pressure increased mRNA for inflammasome components IL-1β, NLRP3, ASC, and CASP1 in rat and mouse retinas. The elevation was greater 1 day after the insult, with the rise in IL-1β most pronounced. The P2X7 receptor was implicated in the mechanosensitive priming of IL-1β mRNA in vivo, as the antagonist Brilliant Blue G (BBG) blocked the increased expression, the agonist BzATP mimicked the pressure-dependent rise in IL-1β, and the rise was absent in P2X7 knockout mice. In vitro measurements from optic nerve head astrocytes demonstrated an increased expression of IL-1β following stretch or swelling. This increase in IL-1β was eliminated by degradation of extracellular ATP with apyrase, or by the block of pannexin hemichannels with carbenoxolone, probenecid, or 10panx1 peptide. The rise in IL-1β expression was also blocked by P2X7 receptor antagonists BBG, A839977 or A740003. The rise in IL-1β was prevented by blocking transcription factor NFκB with Bay 11-7082, while the swelling-dependent fall in NFκB inhibitor IκB-α was reduced by A839977 and in P2X7 knockout mice. In summary, mechanical trauma to the retina primed NLRP3 inflammasome components, but only if there was ATP release through pannexin hemichannels, and autostimulation of the P2X7 receptor. As the P2X7 receptor can also trigger stage two of inflammasome assembly and activation, the P2X7 receptor may have a central role in linking mechanical strain to neuroinflammation.
Introduction
Mechanical trauma can induce complex pathological changes to neural tissue via inflammation (Corps et al., 2015; Heppner et al., 2015). While recruitment of immune cells to the injured region can contribute, localized inflammatory signaling between glia and neurons can also initiate or enhance inflammatory damage. The NLRP3 inflammasome is a key component of the localized innate immune system, leading to the cleavage and release of pro-inflammatory cytokines (Rathinam et al., 2012), and it has been implicated in neural disorders associated with mechanical strain or elevated pressure (Walsh et al., 2014), including traumatic brain injury, encephalitis, and glaucoma (Kaushik et al., 2012; Liu et al., 2013; Chi et al., 2014, 2015).
The involvement of the NLRP3 inflammasome is a two-step process. In the first stage, referred to as the priming step, expression of inflammasome components such as pro-IL-1β and NLRP3 is increased at the transcriptional and translational level (Mariathasan et al., 2006; Patel et al., 2017). This priming stage can be the rate-limiting step in inflammatory responses and may connect the initial insult to chronic inflammation. During the second stage, inflammasome components are assembled and activated, turning on caspase 1 which subsequently catalyzes the maturation of cytokines IL-1β and IL-18 (Stutz et al., 2009). This later step has been linked to efflux of K+ through the P2X7 purinergic receptor (Mariathasan et al., 2006; Petrilli et al., 2007; Bernier, 2012; Karmakar et al., 2016), even for activation associated with lysosomal rupture (Muñoz-Planillo et al., 2013), and can be mimicked by the K+ ionophore nigericin (Perregaux and Gabel, 1994). While activation of the NLRP3 inflammasome has been the subject of intense investigation (e.g., Guo et al., 2015; Yilmaz and Lee, 2015; Freeman and Ting, 2016), the signals leading to inflammasome priming are less well understood. Standard models attribute priming to microbial molecules or other toll-like receptor agonists that are rarely detected in sterile neural environments.
The central role of aberrant purinergic signaling in the neuropathology triggered by mechanical strain has been outlined for the retina (Mitchell et al., 2009). In astrocytes isolated from the optic nerve head, moderate strain leads to a release of ATP through pannexin hemichannels (Beckel et al., 2014). This released ATP then autostimulates P2X7 receptors on these astrocytes to regulate cytoplasmic Ca2+ and other physiological responses. Expression of pannexins is increased in vitro by cell stretch and in vivo in a model of chronic intraocular pressure (IOP) elevation, consistent with a role for ATP release in the neural response to sustained mechanical strain. Elevated extracellular ATP was confirmed in primate, rat, and mouse models of chronic IOP elevation (Lu et al., 2015) and detected in the eyes of humans with chronic glaucoma (Li et al., 2011).
This study asks whether extracellular ATP release through pannexins and autostimulation of the P2X7 receptor are involved in the priming of the NLRP3 inflammasome. The data are consistent with a role for the P2X7 receptor in priming IL-1β and NLRP3 in retina following activation of NFκB in optic nerve head astrocytes. This identifies a new pathway for priming the inflammasome in sterile neural environments subject to mechanical strain.
Materials and Methods
Animal Care and Use
All procedures were performed in strict accordance with the National Research Council’s “Guide for the Care and Use of Laboratory Animals” and were approved by the University of Pennsylvania Institutional Animal Care and Use Committee (IACUC). All animals were housed in temperature-controlled rooms on a 12:12 light:dark cycle with food and water ad libitum. Long–Evans and Sprague Dawley rats (Harlan Laboratories/Envigo, Frederick, MD, United States), and mice (C57BL/6J wild type and P2X7-/- knock out) of both sexes were utilized. Both the wild type C57BL/6J and the P2X7-/- B6.129P2-P2rx7tm1Gab/J Pfizer mice were from Jackson Laboratories (Bar Harbor, ME, United States). Tg-MyocY 437H mice exhibiting a moderate rise in IOP were received as a gift from Zode et al. (2011). Previous characterization of the mice in our laboratory confirmed the moderate, sustained elevation of IOP and loss of peripheral ganglion cells in Tg-MyocY 437H mice (Lu et al., 2015).
Controlled Elevation of IOP Model
The IOP was elevated in adult Sprague-Dawley rats as previously reported (Lu et al., 2017) based on the Control Elevation of IOP (CEI) protocol developed by Morrison et al. (2010, 2014). This procedure separates the effects of increased ocular pressure from cell death to enable a focus specifically on the consequences of mechanical strain. Pressures were selected so that retinal blood flow was maintained and ischemia avoided; studies suggest this protocol leads to minimal loss of neurons (Crowston et al., 2015; Lu et al., 2017).
After receiving 2 mg/kg meloxicam, rats were deeply anesthetized with 1.5% isoflurane or intraperitoneal injection of ketamine (80 mg/kg) and xylazine (10 mg/kg). After administration of proparacaine (0.5%) and tropicamide (0.5–1%), one eye was cannulated with a 27-gauge shielded wing needle (Becton Dickinson, NJ) inserted into the anterior chamber, connected to a 20 ml syringe filled with sterile phosphate buffered saline (PBS). IOP was increased to 50–60 mmHg by elevating the reservoir to the appropriate height; blood flow through the retina was maintained throughout to avoid ischemic complications. Slightly higher pressures were needed with isoflurane. The contralateral eye without cannulation served as a normotensive control. After 4 h, IOP was returned to normal, the needle removed and 0.3% gentamicin ointment or 0.5% erythromycin applied to the cornea. In rat experiments, tissue was obtained as soon as the IOP was returned to baseline. Experiments on mice were performed using procedures similar to those described elsewhere (Crowston et al., 2015). Mice were anesthetized with 1.5% isoflurane, and IOP was increased to 60 mmHg for 4 h, pressure was returned to normal, the needle removed and 0.5% erythromycin applied to the cornea. Mice were either sacrificed immediately or 22 h later to examine the time dependence of gene upregulation.
Intravitreous Injection
Intravitreal injections were performed in rat eyes under a dissecting microscope using a micropipette connected to a microsyringe (Drummond Scientific Co., Broomall, PA, United States) as described (Hu et al., 2010). A glass pipette filled with P2X7 receptor antagonist Brilliant Blue G (BBG, 0.8%) dissolved in saline was passed through the superior nasal region of sclera into the vitreous cavity, ∼1 mm from the limbus, with a total volume of 5 μl injected over a 30 s time period. The antagonist was delivered 1–3 days before IOP elevation. C57BL/6J wild type mice were injected with either P2X7 receptor agonist BzATP (2 μl, 250 μM) or sterile saline.
Astrocyte Cell Culture
Primary rat optic nerve head astrocyte cultures were grown based on a protocol modified from Mandal et al. (2009). The optic nerve proximal to the sclera, defined as the optic nerve head, was obtained from rat pups PD3-5 of both genders; rat astrocytes grew much more efficiently when obtained from neonatal material. This optic nerve head tissue was digested for 1 h using 0.25% trypsin (Invitrogen), with periodic trituration to create a cell suspension. Cells were washed once with Dulbecco’s modified Eagle’s medium (DMEM)/F12 containing 10% of fetal bovine serum (FBS), re-suspended in DMEM/F12, 10% FBS, 1% penicillin/streptomycin, and 25 ng/mL epidermal growth factor (#E4127, Sigma–Aldrich) plated on 35 mm culture dishes and grown at 37°C, 5.5% CO2. Cultures were found to contain >99% astrocytes, as defined by glial fibrillary acidic protein (GFAP) immunofluorescence staining. Cells were generally used at passages 2–5. Mouse optic nerve head astrocyte tissue was collected from 3-month-old C57BL/6J and P2X7-/- mice of both genders using a similar protocol but with only 35 min of trypsin incubation; mouse neonates were not used as the tissues were too small to be handled appropriately.
Swelling Isolated Astrocytes
Rat and mouse optic nerve head astrocytes were subcultured onto plastic 6-well plates and grown until confluent. Cells were incubated in control isotonic solution containing (in mM) 105 NaCl, 5 KCl, 4 NaHEPES, 6 HEPES acid, 1.3 CaCl2, 5 glucose, 5 NaHCO3 and 60 mannitol, pH 7.4 or in solution made 30% hypotonic solution by addition of dH20, for 4 h in the tissue culture incubator. Cells were pretreated with inhibitors Bay 11-7082 (4 μM), Brilliant blue G (BBG, 10 μM), A839977 (50 nM, Tocris Bioscience), A740003 (5 μM, Tocris Bioscience), carbenoxolone (10 μM, #C4790), probenecid (1 mM, #P8761) or 10Panx1 and scrambled peptide (100 μM, #3348 and #3708, respectively, Tocris Bioscience) for 1 h before adding test solutions. RNA was extracted immediately after the 4 h treatment.
In Vitro Stretch Experiments
Isolated rat optic nerve head astrocytes were plated on silicone substrates coated with collagen 1 (Flexcell biaxial six-well plate #BF-3001C, Flexcell International Corp.) for 6–7 days until confluent. After replacing medium with isotonic solution, cells were exposed to square strain of 16% at 0.3 Hz for 4 h in the tissue culture incubator using a vacuum with the Flexcell FX-5000 Tension System (Flexcell International Corp.). Control cells were cultured on similar plates and kept in the same incubator without stretch. RNA was extracted immediately after the 4 h stretch period.
Quantitative PCR
RNA was extracted from astrocytes or retinas by homogenizing in 1 ml TRIzol reagent (Invitrogen), with total RNA purified using a RNeasy mini kit (#79254, Qiagen, Inc., Germantown, MD, United States). RNA concentration and purity were assessed using a Nanodrop spectrophotometer (Thermo Scientific). cDNA was synthesized from 1 μg of total RNA per reaction using the High Capacity cDNA Reverse Transcription Kit (#4368814, Applied Biosystems) at 25°C for 10 min, 37°C for 120 min and terminated at 85°C for 5 min. The Quantitative Polymerase Chain Reaction (qPCR) was performed using SYBR Green and the 7300 RealTimePCR system (Applied Biosystems Corp.), starting with 50°C for 2 min and 95°C for 10 min, followed by 40 cycles at 95°C for 15 s and 60°C for 1 min, and concluding with 15 s at 95°C, 60°C for 1 min and 95°C for 15 s to ensure a single product on melting curves; 0.5 μl of cDNA was used per well, except for in vitro analysis of IL-1β in which 1 μl was used. All data were analyzed using the ΔΔCt approach as described previously (Coffey et al., 2014). Primers used are described in Table 1.
For the PCR gel used in genotyping, RNA was extracted from confluent wild type and P2X7-/- mouse optic nerve head astrocytes and converted to cDNA as above. PCR amplification reaction included 10 μl REDExtract-N-Amp PCR Reaction mixture (#XNATS, Sigma–Aldrich) with 4 μl of the cDNA, 2 μl H2O and 2 μl of the P2X7 receptor primer (10 μM) (Table 1). cDNA was denatured at 94°C for 3 min, followed by 35 PCR cycles. Each consists of three steps: 94°C for 45 s, 65°C for 1 min, and 72°C for 1 min. Final extension was set at 72°C for 10 min. PCR products were detected by 1% (w/v) agarose gels using a 100 base pair DNA Ladder (#15628-019, Invitrogen).
Immunocytochemistry
Astrocytes were grown to 80% of confluence, fixed with 4% formaldehyde for 20 min at 25°C, permeabilized with 0.1% Triton X-100 (Bio-Rad, United States) for 15 min then blocked with 20% Superblock (Thermo Fisher Scientific Inc.) in PBS with Tween 20 (Bio-Rad, United States) (PBS/T) for 1 h. Coverslips were incubated with anti-GFAP monoclonal antibody (#MABH360, 1:250: Chemicon Int.) overnight at 4°C, followed by donkey anti-mouse IgG Alexa-Fluor 488 for 60 min (#A21202, 1:500; Invitrogen). For pannexin 1 (panx1), cells were incubated with goat polyclonal (#ab124131, 1:200, Abcam), followed by rabbit anti-goat 594 nm; over 80% of astrocytes stained positive for panx1. For P2X7 receptor staining, cells were incubated overnight with rabbit polyclonal (#APR-008, 1:100, Alomone), followed by donkey anti-rabbit IgG Alexa Fluor 488 for 1 h (#A21206, 1:500, Invitrogen); over 80% of astrocytes stained positive for P2X7. Cells were incubated with Alexa Fluor 568 Phalloidin (#A12380, 1:100; Invitrogen) for 15 min. After incubation with DAPI (#D9542, Sigma–Aldrich) for 10 min, coverslips were washed and mounted using SlowFade Gold anti-fade media (Invitrogen). Images were acquired using a Nikon Eclipse microscope (Nikon, United States) and Image-Pro software (Media Cybernetics). ImageJ (Schindelin et al., 2015) was used to subtract background, modify intensity and combine pseudocolored images, with parallel processing for all images. Mouse astrocytes were stained with the same anti-GFAP monoclonal antibody used for rat cells.
For retinal sections, mice were transcardially perfused with 4% formaldehyde in PBS. After enucleation, eyes were fixed with 4% formaldehyde overnight then incubated in 30% sucrose for 2 h. Eyes were embedded in OCT compound (Tissue-Tek #62550-1), cryosectioned at 9 μm and mounted on Colorfrost plus slides (Thermo Fisher #9991001). Sections were fixed with 4% formaldehyde for 10 min, permeabilized with 0.1% Triton-X-100 for 10 min, then blocked with 20% Superblock with PBS/T plus 10% donkey serum. Sections were incubated in 5 μg/ml IL-1β primary antibody (goat polyclonal antibody #AF-401-NA R&D systems, Lot #NP2715111) and anti-GFAP monoclonal antibody (#MABH360, 1:250: Chemicon Int.) overnight at 4°C, followed by secondary donkey anti-goat Alexa555-conjugated antibody (#A21432) and donkey anti-mouse IgG Alexa Fluor 488 for 60 min (#A21202, 1:500; Invitrogen). Images were obtained as described above.
Immunoblots
Immunoblots were processed as described (Guha et al., 2012; Coffey et al., 2014). In brief, whole retinas or astrocytes grown on six well-plates were washed twice with cold PBS and lysed in RIPA buffer containing 50 mM Tris-HCl, 150 mM NaCl, protease inhibitor cocktail (Complete; Roche Diagnostics, Germany), 1% Triton X-100, 0.1% SDS, and 10% glycerol. Sonicated samples were centrifuged (14,000 g, 10 min, 4°C) and quantified using a BCA Protein Assay (Pierce/Thermo Fisher). Protein was separated using conventional SDS-PAGE, and processed standard immunoblot protocols (Lu et al., 2015). Polyvinylidene difluoride blots were incubated with 0.25 μg/ml IL-1β goat polyclonal antibody (#AF-401-NA R&D systems, Lot #NP2715111), IκBα rabbit polyclonal antibody (#9242, 1:1000; Cell Signaling) overnight at 4°C, followed by donkey anti-goat IgG-HRP (1:5000; #sc-2020, Santa Cruz Biotechnology) or rabbit IgG, HRP-linked whole antibody from donkey (1:5000, #NA934, Amersham Bioscience Corp.). Blots were developed using the chemiluminescence detection (ECL detection system; Amersham Biosciences Corp.) and visualized with the ImageQuant LAS 4100 imager and Image Quant software (GE Healthcare Life Sciences).
ATP Measurement
Rat astrocytes cells were grown to confluence in a 96-well assay plate (#3610, Corning Inc.). Growth medium was replaced with isotonic solution and cells were allowed to recover for 30 min at 37°C before measurements were taken. A bioluminescent luciferin/luciferase assay was used to measure ATP levels (Reigada et al., 2005). The luciferin/luciferase mix (#FLAAM, Sigma–Aldrich) was stored frozen as a stock solution with 450 μl of control solution/50 μl of dH2O per vial and diluted 50-fold in isotonic solution. Each well contained 20 μl of the assay with 50 μl isotonic base and 30 μl of either isotonic, dH2O to achieve 30% hypotonic solution, dH2O with apyrase (1 U/ml, A6535; Sigma–Aldrich) or 10 μM carbenoxolone. Isotonic solution was removed from the cells, and prepared mixes were added to the cells carefully, then ATP was quantified using the Luminoskan Ascent luminometer (Thermo Fisher), integrating over 200 ms and sampling in succession every 10 s for 18 min at 25°C. The ATP release was calculated at different time points indicated and a standard curve transformed the arbitrary light units to ATP concentration, then normalized to mean levels for isotonic solution.
Data Analysis
Data are reported as mean ± SEM. Analysis was performed in a masked fashion whenever possible. Statistical analysis used a 1-way ANOVA with post hoc tests, or paired t-test when appropriate. Analysis was performed on ranks when data were not normally distributed. Results with p < 0.05 were considered significant.
Results
Priming of Inflammasome Genes after Elevation of IOP
We first examined whether mechanical strain primed inflammasome components in vivo. IOP was unilaterally elevated in rats to between 50 and 60 mmHg for 4 h using a variant of the Controlled Elevation of IOP (CEI) procedure. RNA was extracted from the retina of treated and contralateral control eyes soon after return of IOP to baseline, and qPCR was used to compare expression of genes associated with the NLRP3 inflammasome. There was a significant elevation in the expression of mRNA for IL-1β, NLRP3, Interleukin-1 converting enzyme/caspase1 gene (CASP1) and Apoptosis-Associated Speck-Like Protein Containing CARD (ASC), but not in expression of cytokine IL-18 (Figure 1A).
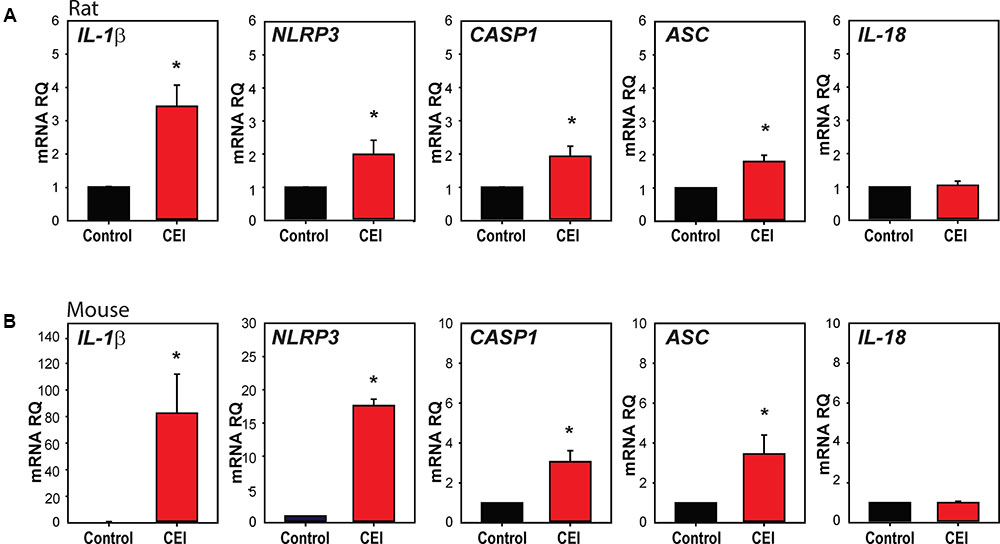
FIGURE 1. Transient elevation of intraocular pressure primes inflammasome genes in rat and mouse retina. (A) Increased expression of inflammasome-associated genes in rat retina after controlled elevation of IOP (CEI). RNA was extracted from the retina soon after IOP returned to baseline following an elevation to 50–60 mmHg for 4 h. The IOP rise led to increased expression of IL-1β (∗p = 0.004, n = 10), NLRP3 (∗p = 0.045, n = 10), CASP1 (∗p = 0.014, n = 10), and ASC (∗p = 0.008, n = 5) as compared to contralateral control eye. There was no detectable rise in IL-18 (n = 5). (B) Mouse retina exposed to CEI showed increased expression of IL-1β (∗p = 0.049, n = 5), NLRP3 (∗p < 0.001, n = 3), CASP1 (∗p = 0.021, n = 3) and ASC (∗p = 0.029, n = 4), but not IL-18 (n = 4). Note the scale difference for IL-1β. RNA from retina was extracted 22 h after returning IOP to baseline from an elevation to 60 mmHg for 4 h.
The procedure was also performed in mice to determine whether the response occurred across species. In material extracted shortly after IOP was returned to baseline, IL-1β was elevated moderately (Supplementary Figure S1A). Expression was substantially increased in material extracted 22 h after IOP returned to baseline, with IL-1β, NLRP3, CASP1, and ASC levels elevated significantly (Figure 1B). The increased expression was greatest for IL-1β, with mRNA levels increasing over 80-fold. At neither time point did the CEI procedure elevate message for the pro-apoptotic marker BAX (Supplementary Figure S1B), consistent with the lack of cell death found previously with this procedure (Crowston et al., 2015).
Expression was also examined in retinas from Tg-MyocY 437H mice; these mice had a sustained, moderate elevation in IOP of 15.5 ± 0.5 mmHg, as compared to 12.2 ± 1.0 in wild type controls at 14–18 months, similar to the IOP difference measured previously at 8 months (Lu et al., 2015). Expression of IL-1β mRNA was increased in retinas from Tg-MyocY 437H mice compared to littermate controls (Supplementary Figure S1C), but the rise in NLRP3 or CASP1 was not significant.
Inflammasome Priming at Protein Level
Given that the elevation of IL-1β was substantially greater than that of other inflammasome components, further efforts were focused on this cytokine. Immunoblots were performed to probe for pro-IL-1β protein to confirm the mRNA results. Expression of 31 kDa pro-IL-1β protein was significantly elevated in mouse eyes when examined 22 h after IOP elevation (Figures 2A,B). Immunohistochemistry was used to localize the rise in IL-1β induced by IOP elevation. Staining for IL-1β was low under control conditions, but increased substantially in eyes exposed to IOP elevation (Figure 2C and Supplementary Figure S2). The increased staining was greatest in the nerve fiber bundle of the retina and throughout the optic nerve. Closer inspection of the staining pattern in the optic nerve head showed increased expression of IL-1β in bands through the optic nerve head that colocalized with GFAP, suggesting IL-1β expression was increased in optic nerve head astrocytes.
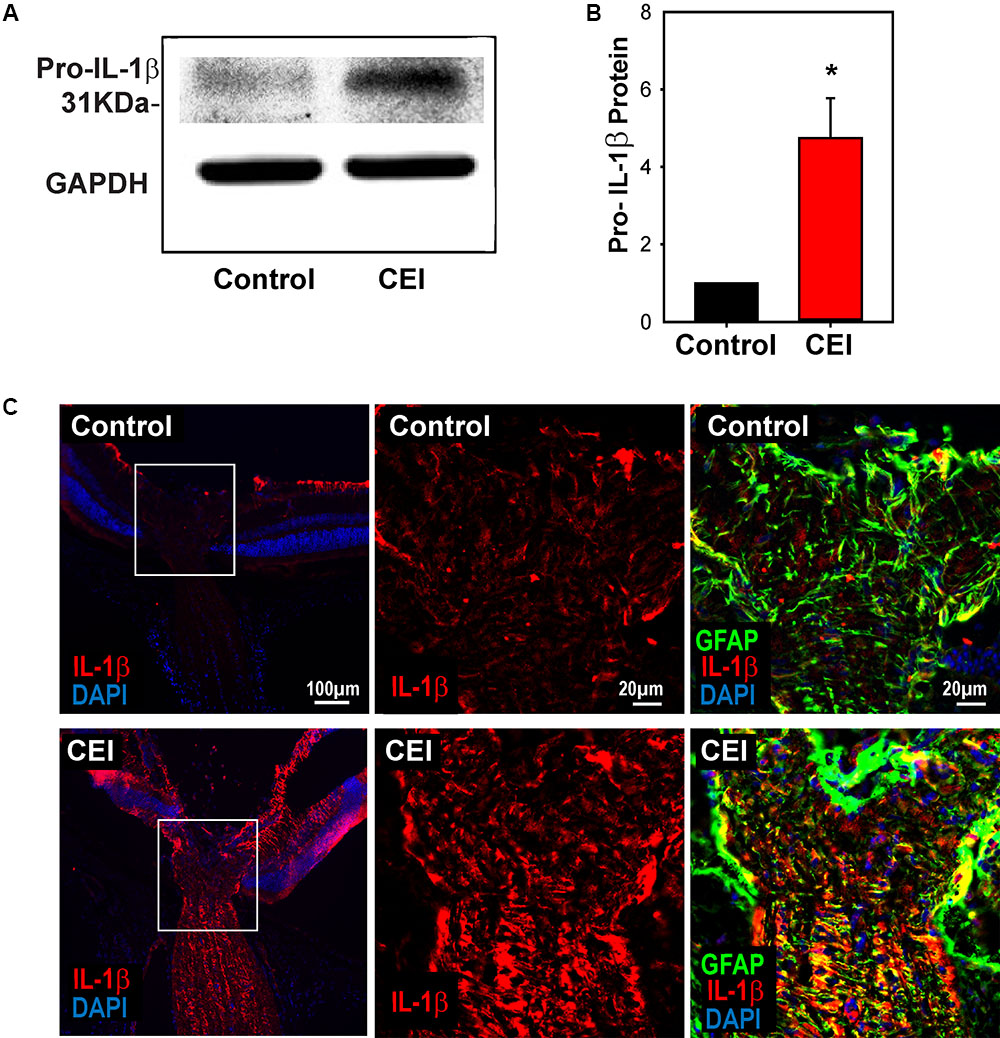
FIGURE 2. Elevation of IL-1β at the protein level. (A) Representative immunoblots from mouse whole retina lysates probed for pro-IL-1β, at the expected 31 kDa size. Protein levels increased in eyes subject to controlled elevation of IOP (CEI) to 60 mmHg for 4 h and sacrificed 22 h after IOP returned to baseline, as compared to the contralateral non-pressurized control eye. Levels of housekeeping protein GAPDH (37 kDa) were similar between conditions. (B) Summary of relative protein expression in response to IOP elevation, as quantified with densitometry and normalized to GAPDH levels (∗p = 0.006, n = 5). (C) Immunohistochemistry sections of mouse retina stained for IL-1β (red), GFAP (green) and with the nuclear stain DAPI (blue). The top row shows representative images from the non-pressurized eye, while the bottom row is from a contralateral eye exposed to the CEI procedure as in “(A)”. Increased staining for IL-1β was apparent in the nerve fiber layer, optic nerve and to a lesser extent throughout the retina (left). Higher magnification of the boxed area shows horizontal bands stained for IL-1β throughout the optic nerve head (center). These bands colocalize with GFAP (right), consistent with optic nerve head astrocytes.
P2X7 Receptor Is Involved in IL-1β Priming In Vivo
We hypothesized that the increased expression of following IOP elevation might relate to the release of ATP and autostimulation of P2X7 receptors found in optic nerve head astrocytes exposed to mechanical strain (Beckel et al., 2014). This theory was supported by recent findings implicating the P2X7 receptor in the mechanosensitive upregulation of cytokines IL-3 (Lim et al., 2016) and IL-6 (Lu et al., 2017) in the retina. To determine if the P2X7 receptor was involved in IOP-sensitive priming of IL-1β, the P2X7 receptor antagonist BBG (0.8%) was injected intravitreally 1–3 days before the IOP rise, then retinas were collected 22 h after the IOP returned to baseline. Pretreatment with BBG prevented the upregulation of IL-1β mRNA triggered by IOP elevation (Figure 3A).
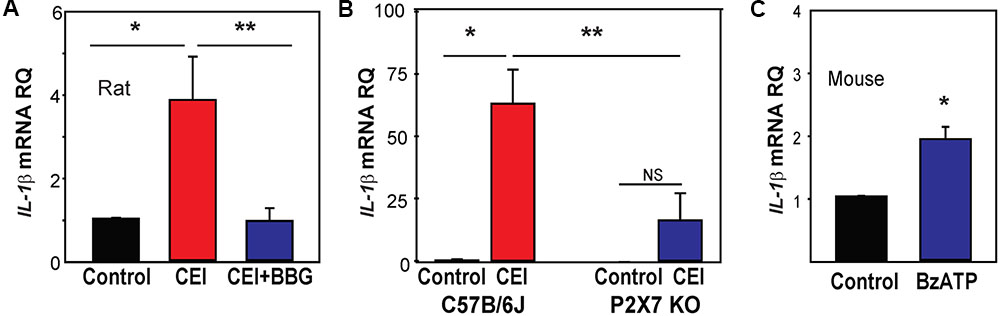
FIGURE 3. Involvement of the P2X7 receptor in inflammasome priming in vivo. (A) The pressure-dependent rise in IL-1β mRNA in rat retinas exposed to moderate elevation of IOP (CEI) was not present following injection of the P2X7 receptor antagonist BBG. Data are expressed as relative gene expression in the non-pressurized (Control) vs pressurized retina for eyes injected with 0.8% BBG (CEI + BBG) or saline (CEI) 1–3 days before the elevation of IOP to 50 mmHg for 4 h (n = 4–5. ∗p < 0.05 vs. saline pressurized). (B) In C57BL/6J mice, the CEI procedure increased retina levels of IL-1β mRNA relative to contralateral untreated eyes (∗p = 0.018). In P2X7 knockout mice, the elevation in IOP did not significantly (NS) increase levels of IL-1β. Levels of IL-1β mRNA in pressurized eyes of P2X7 knockout mice were significantly less than in wild type pressurized eyes (∗∗p = 0.036). Data are expressed as gene expression of untreated eyes (Control) relative to pressurized eyes (CEI). Retina including optic nerve head was extracted 22 h after returning IOP to baseline from an elevation to 60 mmHg for 4 h (n = 4). (C) Intravitreal injection of P2X7 agonist BzATP was sufficient to increase levels IL-1β mRNA in mouse retina when extracted 24 h after injection. Data are expressed as relative gene expression of contralateral non-injected eye (Control) vs. injected eye (BzATP; ∗p < 0.01, n = 3).
P2X7 receptor involvement was examined further by evaluating IL-1β mRNA levels in P2X7-/- mice. Elevation of IOP significantly increased IL-1β mRNA levels in control C57BL/6J mouse eyes, but not P2X7-/- mice (Figure 3B). A similar reduction was observed in levels of NLRP3 in tissue from the P2X7-/- mice (Supplementary Figure S3A). P2X7 receptor stimulation was itself sufficient to increase IL-1β expression; the P2X7 agonist BzATP was injected intravitreally in C57BL/6J mice with sterile saline injected into the contralateral eye as a control. Retinas collected 1 day after the injections showed that BzATP significantly increased the expression of IL-1β (Figure 3C). The effect of BzATP on other inflammasome genes was much smaller (Supplementary Figure S3B). Together, the data implied the P2X7 receptor was priming IL-1β in response to mechanical strain in vivo.
Mechanical Strain Primes Inflammasome Genes in Isolated Astrocytes
The optic nerve head has been identified as a focal center of mechanical strain that accompanies IOP elevation (Burgoyne et al., 2005; Downs et al., 2008), with optic nerve head astrocytes involved in several signaling pathways implicated in glaucomatous pathology (Hernandez, 2000; Morgan, 2000; Tehrani et al., 2016). Mild stretch to optic nerve head astrocytes leads to a release of ATP and autostimulation of P2X7 receptors (Beckel et al., 2014), and the immunohistochemical staining in Figure 2C indicated an increase in IL-1β in optic nerve head astrocytes. As such, the mechanosensitive priming of IL-1β and the contribution of the P2X7 receptor to this priming was examined further in isolated optic nerve head astrocytes.
Primary rat optic nerve head astrocytes were plated on a silicon sheet and subjected to 16% strain at 0.3 Hz for 4 h. Cells subjected to this stretch protocol looked identical to control cells on a macroscopic level, with very similar patterns of actin staining (Figure 4A). The level of IL-1β mRNA was significantly increased in stretched cells (Figure 4B). Expression of IL-1β was also elevated by applying strain to the cells by swelling in a 30% hypotonic solution (Figure 4C). The rise in other inflammasome genes induced by swelling astrocytes was variable, with small increases in NLRP3, ASC, and IL-18, but not CASP1 (Supplementary Figure S4).
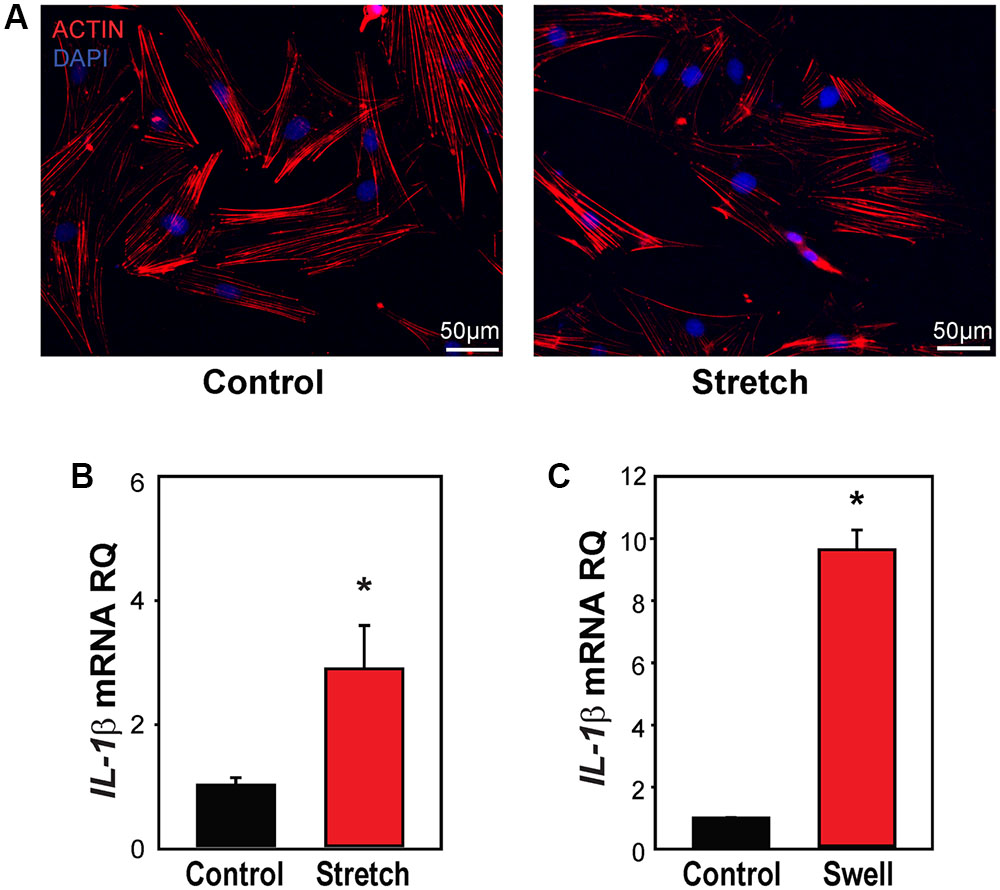
FIGURE 4. Mechanical strain primes IL-1β in optic nerve head astrocytes. (A) Astrocytes plated on silicon substrates and fixed after 4 h in control conditions (left, Control) or after 16% cyclical strain at 0.3 Hz (right, Stretch). Staining for actin with phalloidin (red) showed no obvious changes to the cytoskeleton. (B) Application of 16% cyclical strain for 4 h increased expression of IL-1β mRNA (n = 5, ∗p < 0.03). (C) Astrocytes exposed to moderate swelling induced by 30% hypotonicity (Swell) showed increased expression of IL-1β mRNA relative to untreated cells maintained in isotonic solution (Control; n = 3, ∗p = 0.009).
ATP Release through Pannexin Channels Required for Mechanosensitive Priming of IL-1β in Astrocytes
The measurements of IL-1β mRNA from optic nerve head astrocytes in vitro allowed further investigation of the mechanisms linking mechanical strain to IL-1β upregulation. First, the ability of astrocytes to release ATP when swollen, and of the soluble ectoATPase apyrase to prevent the extracellular elevation in ATP was confirmed (Figures 5A,B). The ability of apyrase to prevent the swelling-induced rise in IL-1β expression supported a role for extracellular ATP in this pathway (Figure 5C).
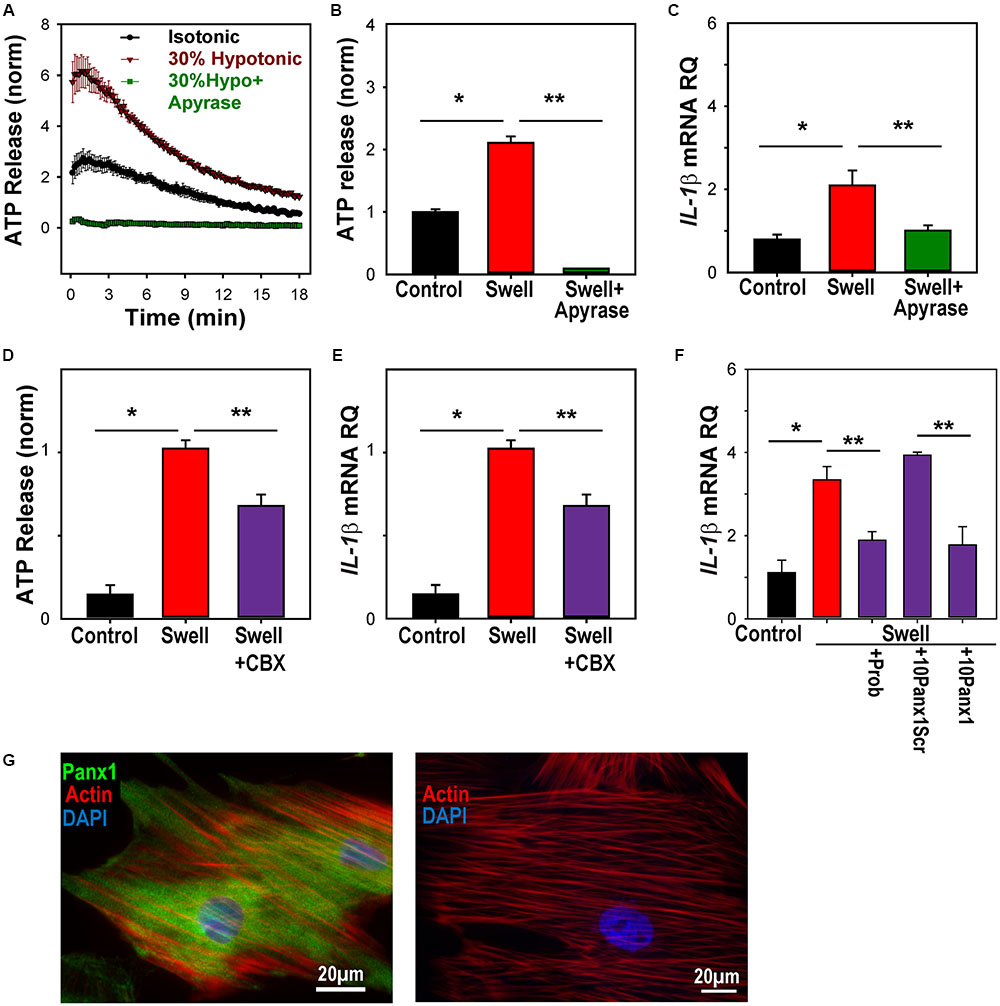
FIGURE 5. ATP release through pannexin channels required for mechanosensitive priming of IL-1β in astrocytes. (A) Swelling rat astrocytes in hypotonic solution led to a release of ATP into the extracellular medium, as detected by the luciferin/luciferase assay. The ATP hydrolase apyrase (1 U/ml) substantially reduced the response. Symbols represent mean ± SEM, n = 10. (B) Quantification of extracellular ATP levels 18 min after exposure to solutions (∗Swell vs. Control isotonic, p < 0.05, ∗∗ Swell vs Swell+Apyrase, p < 0.05, n = 10). (C) Swelling astrocytes in the presence of apyrase also prevented the rise in IL-1β mRNA (∗p = 0.02 Swell vs control, ∗∗p = 0.03 Swell vs Swell+Apyrase, n = 3). (D) The swelling-induced release of ATP was inhibited by pannexin channel blocker carbenoxolone (CBX, 10 μM, ∗p < 0.05, Swell vs. control, ∗∗p < 0.05 Swell vs. Swell+CBX, n = 20, normalized to swell). (E) The swelling-induced rise in IL-1β mRNA was also inhibited by 10 μM carbenoxolone (∗p < 0.05, Swell vs. control, ∗∗p < 0.05 Swell vs Swell+CBX, n = 7, normalized to swell). (F) Pannexin blocker probenecid (Prob, 1 mM) reduced the swelling-induced rise of IL-1β in astrocytes (p = 0.029). The specific peptide blocker 10Panx1 (100 μM) reduced the expression of IL-1β as compared to the scrambled peptide control (10panxscr, ∗p = 0.003). Swelling alone raised IL-1β (p = 0.03, n = 3 for all). (G) Left: Astrocytes stained for panx1 (green), actin (red) and DAPI (blue). Right: No signal was detected in the absence of panx1 antibody.
Previous work suggests that pannexin hemichannels are a conduit for the mechanosensitive release of ATP from these cells (Beckel et al., 2014). Carbenoxolone is reported to be relatively specific for pannexin channels at 10 μM (Bruzzone et al., 2005), and this concentration led to a moderate, but significant reduction in the swelling-induced release of ATP (Figure 5D). This concentration of carbenoxolone reduced the rise in IL-1β mRNA expression by a similar amount (Figure 5E). The swelling-induced rise in IL-1β was also blocked by blocked by probenecid and the peptide blocker 10panx1, while the scrambled peptide control had no effect on expression (Figure 5F). The expression of pannexin 1 in these astrocytes (Figure 5G), combined with the reduction by three pannexin blockers, implicated pannexins in the IL-1β response.
P2X7 Receptor Necessary and Sufficient for Mechanosensitive Priming of IL-1β In Vitro
ATP released after swelling can autostimulate astrocytes, with P2X7 antagonists blocking the rise in cytoplasmic Ca2+ induced by swelling (Beckel et al., 2014). To determine whether this autostimulation contributed to the priming of IL-1β, the effect of P2X7 antagonists on the swelling-dependent rise in IL-1β was examined. The expression of the P2X7 receptor in astrocytes was confirmed using immunocytochemistry (Figure 6A). Three P2X7 antagonists, BBG, A839977, and A740003, significantly prevented the mechanosensitive IL-1β priming in optic nerve astrocytes (Figure 6B). To support this pharmacological identification of the P2X7 receptor, experiments were pursued on astrocytes isolated from C57Bl/6J mice and P2X7-/- mice. PCR confirmed the absence of the P2X7 receptor message in astrocytes obtained from knockout mice while immunocytochemistry supported the absence of P2X7 protein (Supplementary Figures S5A,B). The increased in expression of IL-1β mRNA after 4 h of swelling was significantly lower in astrocytes from the P2X7-/- mice as compared to the C57BL/6J mice (Figure 6C). Treatment of astrocytes with the P2X7 receptor agonist BzATP was sufficient to upregulate IL-1β mRNA (Figure 6D). Similar results were found with NLRP3; the response was reduced in astrocytes from P2X7-/- mice (Supplementary Figure S5C), while addition of BzATP induced a significant, albeit small, rise in NLRP3 (Supplementary Figure S5D).
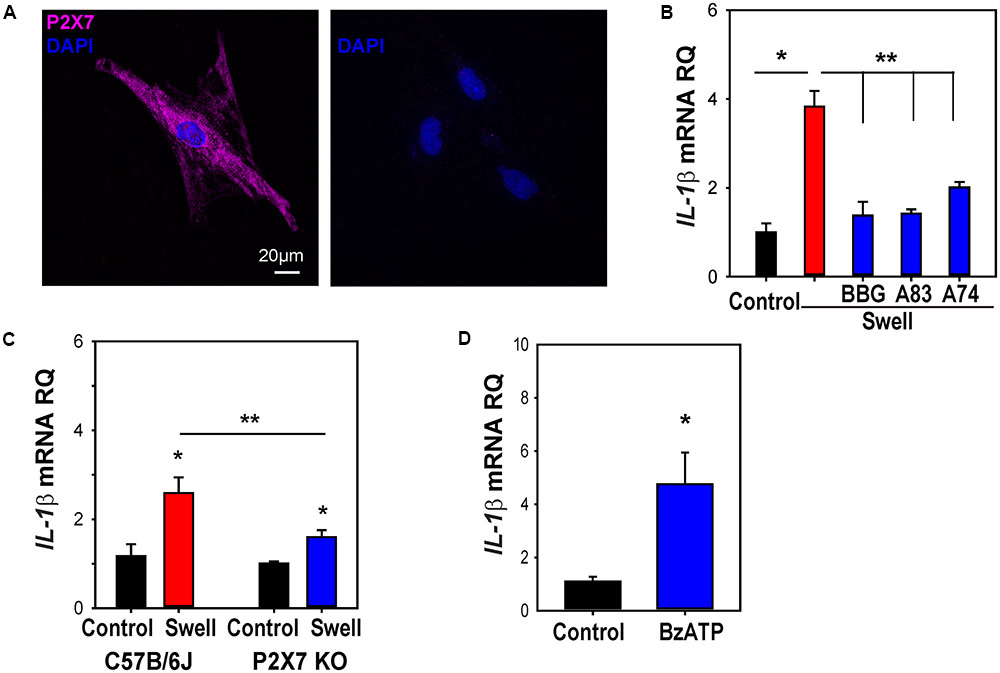
FIGURE 6. P2X7 receptor involved in priming of IL-1β in astrocytes. (A) Immunocytochemistry showing expression of the P2X7R in cultured optic nerve head astrocytes (left). No signal was detected in the absence of the primary antibody (right). (B) The swelling-induced rise in IL-1β mRNA was inhibited by P2X7R antagonists BBG (10 μM), A839977 (50 nM) and A740003 (5 μM). Cells were pretreated with drugs for 1 h before swelling (∗p < 0.001 Swell vs. control, ∗∗p < 0.001 Swell vs. Swell+drugs, n = 4). (C) The swelling-induced rise in IL-1β was reduced in astrocytes from P2X7-/- mice as compared to C57BL/6J mice. Data are expressed relative to the matched control group (∗p < 0.01, ∗∗p = 0.026, n = 6). (D) Application of BzATP (400 μM) for 4 h increased IL-1β expression (∗p < 0.01, n = 7).
NFκB Is Involved in Priming of NLRP3 and IL-1β after Mechanical Strain
While many different transcription factors could be involved in the upregulation of IL-1β, we focused on the contribution of NFκB, as it is linked to the transcription of inflammasome genes including IL-1β (Cogswell et al., 1994) and can be activated by P2X7 stimulation (Liu et al., 2011). The NFκB inhibitor Bay 11-7082 prevented the swelling-induced upregulation of IL-1β in rat astrocytes (Figure 7A). Upregulation of NLRP3 was similarly blocked by Bay 11-7082 (Supplementary Figure S6).
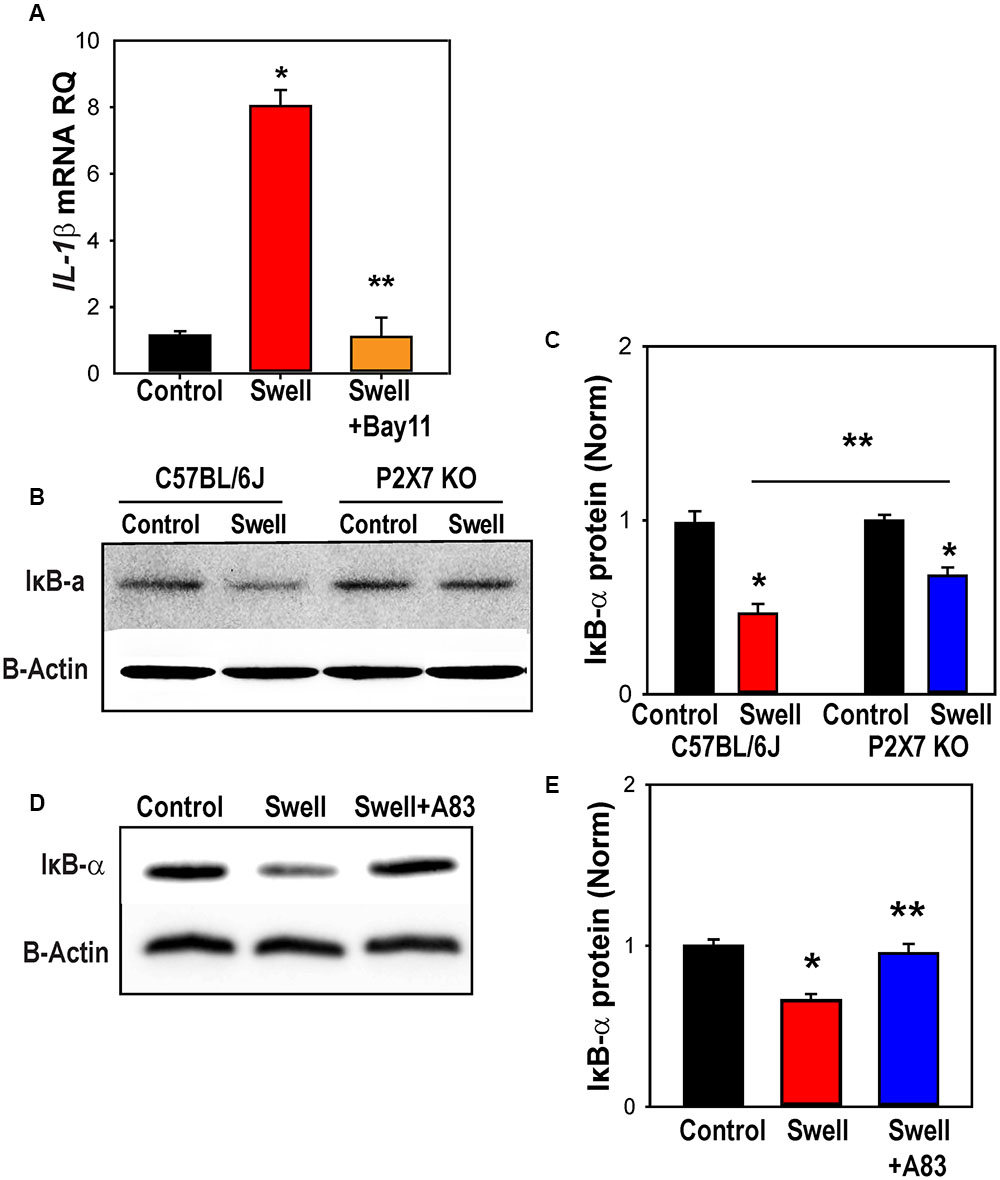
FIGURE 7. NFκB is involved in inflammasome priming after mechanical strain. (A) NFκB inhibitor Bay11-7082 (Bay11, 4 μM) prevented IL-1β upregulation in rat astrocytes. Bay11-7082 was present for 1 h before and during the 4 h swelling (∗p < 0.001 Control vs. Swell, ∗∗p < 0.001, Swell vs. Swell+Bay11; n = 4). (B) Representative immunoblots from mouse optic nerve head astrocyte lysates from control C57BL/6J and P2X7-/- mice probed for IκB-α (39 kDa) and housekeeping protein β-actin (42 kDa). Expression of IκB-α was reduced following 4 h of swelling in control astrocytes, consistent with the activation of NFκb. (C) Summary of relative IκB-α protein expression from experiments illustrated in panel B quantified with densitometry. The effect of swelling on IκB-α was significantly less in astrocytes from P2X7-/- mice (∗p < 0.001 Swell vs. Control C57BL/6J, ∗p = 0.011 Swell vs. Control P2X7-/-, ∗∗p = 0.038 Swell C57BL/6J vs. Swell P2X7-/-; n = 3). (D) Representative immunoblots from mouse optic nerve head astrocyte lysates from control mice probed for IκB-α (39 kDa) and housekeeping protein β-actin (42 kDa). The reduction in IκB-α triggered by swelling was reduced in the presence of P2X7R antagonist A839977 (100 nM). (E) Mean densitometry values for IκB-α protein expression from immunoblots like those in “(D)” (∗p = 0.002, ∗∗p = 0.004; n = 4).
To confirm a role for NFκB in the transcriptional changes, levels of nuclear factor of kappa light polypeptide gene enhancer in B-cells inhibitor, alpha (IκBα) in extracts from control and swollen astrocytes from C57BL/6J were probed with immunoblots. The reduction in Iκbα levels corresponds to activation of NFκB (Finco and Baldwin, 1995). Swelling reduced levels Iκbα in astrocytes from control mice, but not in cells from P2X7-/- mice (Figure 7B). Quantification showed the reduction in Iκbα induced by swelling was significantly less in astrocytes from P2X7-/- mice (Figure 7C). The P2X7 receptor antagonist A839977 also reduced the ability of swelling to activate IκBα (Figures 7D,E), supporting a role for the P2X7 receptor in the swelling-dependent activation of NFκB.
Discussion
This study suggests that mechanical strain can increase expression of certain components of the NLRP3 inflammasome in neural tissue and identifies a role for ATP release and the P2X7 receptor in this priming. The cytokine IL-1β was linked through this pathway most strongly, with supportive evidence for upregulation of NLRP3. Given that priming is the initial step in NLRP3 inflammasome involvement, this study implicates a role for the P2X7 receptor in linking mechanical strain to innate immune responses in neural tissues.
Role of Purinergic Signaling
Evidence linking the P2X7 receptor with priming of IL-1β comes from in vivo and in vitro assays of mRNA and protein. The P2X7 antagonist BBG prevented the rise in IL-1β expression in vivo in rat retinas exposed to a transient rise in IOP. The rise in IL-1β following transient IOP increase was significantly less in P2X7-/- mice as compared to control, while the rise in IL-1β expression following intravitreal injection of P2X7 agonist BzATP suggests receptor stimulation is sufficient to increase IL-1β expression.
In vitro work using isolated astrocytes provides additional support and implicates the P2X7 receptor more specifically, with the use of more selective antagonists A839977 and A740003 (Honore et al., 2006, 2009), in addition to BBG. The pressure-induced rise in IL-1β expression was prevented by these agents and was not present in P2X7-/- mice, while the P2X7 agonist BzATP was sufficient to elevate IL-1β. Together, the combined evidence from pharmacological and genetic methods, and in both isolated astrocytes and whole retina, strongly implicates a role for the P2X7 receptor in priming of IL-1β. The identification of a P2X7 receptor contribution in both rats and mice suggests receptor involvement may be widespread, particularly given the differences in the receptor across these species (Donnelly-Roberts et al., 2009).
Involvement of extracellular ATP in IL-1β priming was supported by the ability of the soluble ectoATPase apyrase to block gene upregulation. The ability of pannexin channel blockers carbenoxolone, probenecid and the 10panx1 peptide to prevent a rise in IL-1β strongly implicates the release of ATP through the hemichannel in priming, as these drugs inhibited the ATP release induced by astrocyte swelling (Beckel et al., 2014). The partial block by carbenoxolone is consistent, as this concentration shows specificity for pannexins over connexins while not providing a complete block, although it may also reflect a contribution from an alternative efflux mechanism. Overall, these studies suggest a model in which mechanical strain leads to release of ATP through pannexin hemichannels, autostimulation of the P2X7 receptor and subsequent priming of IL-1β (Figure 8).
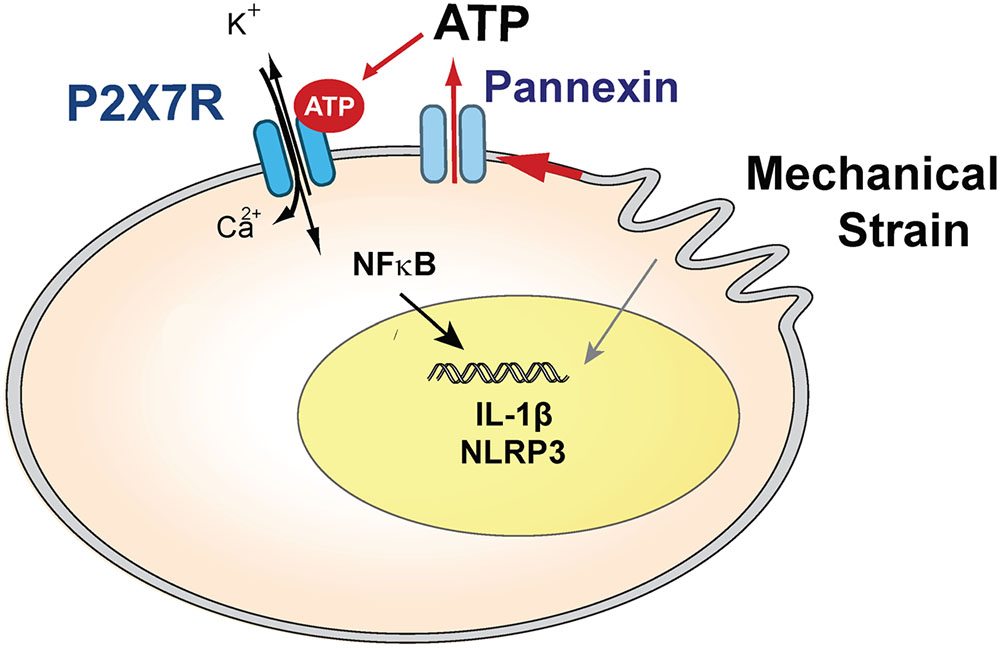
FIGURE 8. Model summarizing the hypothesized role of the P2X7 receptor in the priming of inflammasome genes after mechanical strain, which leads to ATP release through pannexin hemichannels. Extracellular ATP autostimulates P2X7 receptors, leading to NFκB activation and transcriptional elevation of IL-1β and NLRP3 in optic nerve head astrocytes. Swelling may also activate inflammasome genes through other pathways.
Transcription Factors and Gene Variation
The transcription factor NFκB was implicated in the upregulation of IL-1β and NLRP3 in astrocytes. Increased expression of both genes in swollen astrocytes was blocked by NFκB antagonist Bay 11-7082. The P2X7 receptor was implicated by data showing the swelling-dependent decreased in NFκB inhibitor Iκbα was reduced in astrocytes from P2X7-/- mice and by the P2X7 receptor antagonist A839977. Elevation of hydrostatic pressure leads to translocation of NFκB to the nucleus in retinal astrocytes (Sappington and Calkins, 2006), while NFκB regulates transcription of NLRP3 and IL-1β in other cells (Cogswell et al., 1994; Lawrence, 2009; Boaru et al., 2015). The P2X7 receptor has been shown to activate NFκB through contact with MyD88 in HEK cells (Liu et al., 2011). This makes the activation of NFκB by P2X7 a likely route to connect mechanical strain with increased expression of IL-1β and NLRP3. While the residual activation in astrocytes from P2X7-/- mice may reflect the involvement of other pathways, the presence of P2X7 splice variants may provide additional possibilities (Masin et al., 2012).
The increase in IL-1β in response to mechanical strain was particularly consistent, observed both in rat and mouse in vivo models, and in cultured astrocytes from rat and mouse tissues; the increase in the 31 kDa pro-form in immunoblots confirmed this on a protein level. While expression of NLRP3, CASP1, ASC, and IL-18 were all increased by some model of mechanical strain, the effects in these genes were less consistent. Some of this variation may have been time-dependent, as the expression of most genes was substantially larger in mouse retina 22 h after IOP elevation was returned to baseline. The diverse responses to swelling and stretching were not unexpected given that IL-1β, IL-18, NLRP3, CASP1, and ASC are all regulated by a different combination of transcription factors. Regardless, the priming of IL-1β and NLRP3 may be rate limiting in inflammasome activation, as CASP1 and IL-18 are constitutively expressed in monocytes and epithelial cells (Thornberry et al., 1992; Dinarello, 2007).
Contribution of Astrocytes
The P2X7 receptor was implicated in priming IL-1β in both in vivo experiments, where material from the entire retina was analyzed, and during the in vitro experiments using isolated optic nerve head astrocytes. These optic nerve head astrocytes make up a small proportion of retinal material, however, and increased staining for IL-1β in various parts of the retina after elevated IOP suggests additional cell types may contribute to the retinal response. For example, our staining was consistent with increased expression in Muller glial cells. Neuronal involvement is also likely; the increased staining mentioned above is supported by recent results showing increased IL-1β expression in isolated retinal ganglion cells exposed to stretch (Lim et al., 2016); these neurons release ATP and autostimulate their P2X7 receptors, suggesting a parallel pathway may be involved (Xia et al., 2012). However, the optic nerve head is a focal center of mechanical strain in the glaucomatous eye (Burgoyne et al., 2005), and astrocytes from patients showed morphological changes before marked loss of retinal ganglion cells (Lye-Barthel et al., 2013). The astrocytes express mechanosensitive channels (Choi et al., 2015) and contribute to the inflammatory response in glaucomatous eyes (Johnson and Morrison, 2009). As such, the identification of the P2X7 receptor linking mechanical strain to inflammasome priming in optic nerve head astrocytes is particularly relevant.
ATP as Endogenous Trigger Linking Mechanical Strain to Inflammation in Neural Tissues
Inflammation has emerged as a critical component of chronic neurodegeneration, with the NLRP3 inflammasome a major contributor (Freeman and Ting, 2016). While priming of the NLRP3 inflammasome traditionally has been attributed to stimulation of toll-like receptors (Patel et al., 2017), these receptors are primarily activated by pathogens, and the endogenous triggers linking neural insult to inflammasome priming are largely unknown.
Our identification of the P2X7 receptor as a trigger for NLRP3 inflammasome priming in the retina builds on evidence linking mechanical strain to aberrant purinergic signaling in the retina and allows this endogenous trigger to be placed in a physiological context. Extracellular ATP is elevated after increased IOP in bovine mouse, rat, primate and human samples (Zhang et al., 2007; Reigada et al., 2008; Lu et al., 2015). Stimulation of P2X7 receptors can damage retinal ganglion cells in vitro and in vivo (Zhang et al., 2005; Hu et al., 2010), and ATP release through pannexin hemichannels following mechanical strain can autostimulate P2X7 receptors on optic nerve head astrocytes (Beckel et al., 2014). As pannexin hemichannels are upregulated by prolonged stretch in vitro and in vivo, this provides a source of the sustained extracellular ATP found in the chronic glaucoma models. The present study suggests this may also provide a mechanism for chronic priming of inflammasome genes.
The P2X7 receptor is traditionally known for its ability to activate the NLRP3 inflammasome following the efflux of K+ through the open channel (Katsnelson et al., 2015). The present study identifies a novel role for the P2X7 receptor in the priming of IL-1β and NLRP3. The ability of one receptor to mediate both steps of inflammasome involvement identifies a potentially central role for purinergic signaling in the link between mechanical strain and innate inflammation in neural tissues. Future studies focused on the contributions of the P2X7 receptor to inflammasome activation following its role in priming will clarify how this “double punch” impacts the inflammatory state of the retina.
The P2X7 receptor is implicated in multiple forms of neural degeneration (Sanderson et al., 2014; Sperlagh and Illes, 2014). Neuronal loss after axotomy was reduced in P2X7 knock out mice (Nadal-Nicolas et al., 2016) while the receptor is also implicated in Alzheimer’s disease (Miras-Portugal et al., 2015). Activation of the P2X7 receptor was implicated in a study of traumatic brain injury, with expression of IL-1β reduced in knock out mice (Kimbler et al., 2012). The present study identifies a role of the receptor in priming of IL-1β and other genes involved with the NLRP3 inflammasome, suggesting a central role in the inflammasome activation.
Portions of this work have previously been presented in abstract form (Lu et al., 2013; Albalawi et al., 2016; Mitchell et al., 2016, 2017).
Availability of Data and Materials
All readily reproducible materials described in the manuscript, including new software, databases and all relevant raw data will be freely available to any scientist wishing to use them.
Author Contributions
FA helped design the study, carried out experiments on elevation of IOP in mice and rats and experiments on isolated astrocytes. WL helped design the study and developed the protocols for in vivo experiments and carried out some rat IOP experiments, JB and JL performed in vitro experiments on rat optic nerve head astrocytes. SM helped in the writing of the manuscript. CM conceived of the study, and participated in its design and coordination and helped to draft the manuscript. All authors read, edited and approved of the final manuscript.
Funding
This work is supported by grants from the NIH EY015537 and EY013434 and core grant EY001583 (CM), NIH DK106115 (JB), Jody Sack Fund (WL) and King Saud bin Abdulaziz University for Health Sciences (FA). These funding bodies had no direct role in the design of the study and collection, analysis, and interpretation of data or in writing the manuscript.
Conflict of Interest Statement
The authors declare that the research was conducted in the absence of any commercial or financial relationships that could be construed as a potential conflict of interest.
Acknowledgments
The authors would like to thank Mary Leonard of the University of Pennsylvania Biomedical Art and Design group for assistance with the artwork. The authors would like to thank Gulab Zode and Val Sheffield for the Tg-MyocY437H mice.
Supplementary Material
The Supplementary Material for this article can be found online at: http://journal.frontiersin.org/article/10.3389/fncel.2017.00227/full#supplementary-material
References
Albalawi, F., Lu, W., Lim, J., and Mitchell, C. H. (2016). The role of P2X7R in priming the NLRP3 inflammasome after mechanical strain. FASEB J. 30, 745.
Beckel, J. M., Argall, A. J., Lim, J. C., Xia, J., Lu, W., Coffey, E. E., et al. (2014). Mechanosensitive release of adenosine 5′-triphosphate through pannexin channels and mechanosensitive upregulation of pannexin channels in optic nerve head astrocytes: a mechanism for purinergic involvement in chronic strain. Glia 62, 1486–1501. doi: 10.1002/glia.22695
Bernier, L. P. (2012). Purinergic regulation of inflammasome activation after central nervous system injury. J. Gen. Physiol. 140, 571–575. doi: 10.1085/jgp.201210875
Boaru, S. G., Borkham-Kamphorst, E., Van de Leur, E., Lehnen, E., Liedtke, C., and Weiskirchen, R. (2015). NLRP3 inflammasome expression is driven by NF-kappaB in cultured hepatocytes. Biochem. Biophys. Res. Commun. 458, 700–706. doi: 10.1016/j.bbrc.2015.02.029
Bruzzone, R., Barbe, M. T., Jakob, N. J., and Monyer, H. (2005). Pharmacological properties of homomeric and heteromeric pannexin hemichannels expressed in Xenopus oocytes. J. Neurochem. 92, 1033–1043. doi: 10.1111/j.1471-4159.2004.02947.x
Burgoyne, C. F., Downs, J. C., Bellezza, A. J., Suh, J. K., and Hart, R. T. (2005). The optic nerve head as a biomechanical structure: a new paradigm for understanding the role of IOP-related stress and strain in the pathophysiology of glaucomatous optic nerve head damage. Prog. Retin. Eye Res. 24, 39–73. doi: 10.1016/j.preteyeres.2004.06.001
Chi, W., Chen, H., Li, F., Zhu, Y., Yin, W., and Zhuo, Y. (2015). HMGB1 promotes the activation of NLRP3 and caspase-8 inflammasomes via NF-kappaB pathway in acute glaucoma. J. Neuroinflammation 12, 137. doi: 10.1186/s12974-015-0360-2
Chi, W., Li, F., Chen, H., Wang, Y., Zhu, Y., Yang, X., et al. (2014). Caspase-8 promotes NLRP1/NLRP3 inflammasome activation and IL-1beta production in acute glaucoma. Proc. Natl. Acad. Sci. U.S.A. 111, 11181–11186. doi: 10.1073/pnas.1402819111
Choi, H. J., Sun, D., and Jakobs, T. C. (2015). Astrocytes in the optic nerve head express putative mechanosensitive channels. Mol. Vis. 21, 749–766.
Coffey, E. E., Beckel, J. M., Laties, A. M., and Mitchell, C. H. (2014). Lysosomal alkalization and dysfunction in human fibroblasts with the Alzheimer’s disease-linked presenilin 1 A246E mutation can be reversed with cAMP. Neuroscience 263, 111–124. doi: 10.1016/j.neuroscience.2014.01.001
Cogswell, J. P., Godlevski, M. M., Wisely, G. B., Clay, W. C., Leesnitzer, L. M., Ways, J. P., et al. (1994). NF-kappa B regulates IL-1 beta transcription through a consensus NF-kappa B binding site and a nonconsensus CRE-like site. J. Immunol. 153, 712–723.
Corps, K. N., Roth, T. L., and McGavern, D. B. (2015). Inflammation and neuroprotection in traumatic brain injury. JAMA Neurol. 72, 355–362. doi: 10.1001/jamaneurol.2014.3558
Crowston, J. G., Kong, Y. X., Trounce, I. A., Dang, T. M., Fahy, E. T., Bui, B. V., et al. (2015). An acute intraocular pressure challenge to assess retinal ganglion cell injury and recovery in the mouse. Exp. Eye Res. 141, 3–8. doi: 10.1016/j.exer.2015.03.006
Dinarello, C. A. (2007). Historical insights into cytokines. Eur. J. Immunol. 37(Suppl. 1), S34–S45. doi: 10.1002/eji.200737772
Donnelly-Roberts, D. L., Namovic, M. T., Han, P., and Jarvis, M. F. (2009). Mammalian P2X7 receptor pharmacology: comparison of recombinant mouse, rat and human P2X7 receptors. Br. J. Pharmacol. 157, 1203–1214. doi: 10.1111/j.1476-5381.2009.00233.x
Downs, J. C., Roberts, M. D., and Burgoyne, C. F. (2008). Mechanical environment of the optic nerve head in glaucoma. Optom. Vis. Sci. 85, 425–435. doi: 10.1097/OPX.0b013e31817841cb
Finco, T. S., and Baldwin, A. S. (1995). Mechanistic aspects of NF-κB regulation: the emerging role of phosphorylation and proteolysis. Immunity 3, 263–272. doi: 10.1016/1074-7613(95)90112-4
Freeman, L. C., and Ting, J. P. (2016). The pathogenic role of the inflammasome in neurodegenerative diseases. J. Neurochem. 136, 29–38. doi: 10.1111/jnc.13217
Guha, S., Baltazar, G. C., Tu, L. A., Liu, J., Lim, J. C., Lu, W., et al. (2012). Stimulation of the D5 dopamine receptor acidifies the lysosomal pH of retinal pigmented epithelial cells and decreases accumulation of autofluorescent photoreceptor debris. J. Neurochem. 122, 823–833. doi: 10.1111/j.1471-4159.2012.07804.x
Guo, H., Callaway, J. B., and Ting, J. P. (2015). Inflammasomes: mechanism of action, role in disease, and therapeutics. Nat. Med. 21, 677–687. doi: 10.1038/nm.3893
Heppner, F. L., Ransohoff, R. M., and Becher, B. (2015). Immune attack: the role of inflammation in Alzheimer disease. Nat. Rev. Neurosci. 16, 358–372. doi: 10.1038/nrn3880
Hernandez, M. R. (2000). The optic nerve head in glaucoma: role of astrocytes in tissue remodeling. Prog. Retin. Eye Res. 19, 297–321. doi: 10.1016/S1350-9462(99)00017-8
Honore, P., Donnelly-Roberts, D., Namovic, M., Zhong, C., Wade, C., Chandran, P., et al. (2009). The antihyperalgesic activity of a selective P2X7 receptor antagonist, A-839977, is lost in IL-1alphabeta knockout mice. Behav. Brain Res. 204, 77–81. doi: 10.1016/j.bbr.2009.05.018
Honore, P., Donnelly-Roberts, D., Namovic, M. T., Hsieh, G., Zhu, C. Z., Mikusa, J. P., et al. (2006). A-740003 [N-(1-{[(cyanoimino)(5-quinolinylamino) methyl]amino}-2,2-dimethylpropyl)-2-(3,4-dimethoxyphenyl)acetamide], a novel and selective P2X7 receptor antagonist, dose-dependently reduces neuropathic pain in the rat. J. Pharmacol. Exp. Ther. 319, 1376–1385. doi: 10.1124/jpet.106.111559
Hu, H., Lu, W., Zhang, M., Zhang, X., Argall, A. J., Patel, S., et al. (2010). Stimulation of the P2X7 receptor kills rat retinal ganglion cells in vivo. Exp. Eye Res. 91, 425–432. doi: 10.1016/j.exer.2010.06.017
Johnson, E. C., and Morrison, J. C. (2009). Friend or foe? Resolving the impact of glial responses in glaucoma. J. Glaucoma 18, 341–353. doi: 10.1097/IJG.0b013e31818c6ef6
Karmakar, M., Katsnelson, M. A., Dubyak, G. R., and Pearlman, E. (2016). Neutrophil P2X7 receptors mediate NLRP3 inflammasome-dependent IL-1beta secretion in response to ATP. Nat. Commun. 7:10555. doi: 10.1038/ncomms10555
Katsnelson, M. A., Rucker, L. G., Russo, H. M., and Dubyak, G. R. (2015). K+ efflux agonists induce NLRP3 inflammasome activation independently of Ca2+ signaling. J. Immunol. 194, 3937–3952. doi: 10.4049/jimmunol.1402658
Kaushik, D. K., Gupta, M., Kumawat, K. L., and Basu, A. (2012). NLRP3 inflammasome: key mediator of neuroinflammation in murine Japanese encephalitis. PLoS ONE 7:e32270. doi: 10.1371/journal.pone.0032270
Kimbler, D. E., Shields, J., Yanasak, N., Vender, J. R., and Dhandapani, K. M. (2012). Activation of P2X7 promotes cerebral edema and neurological injury after traumatic brain injury in mice. PLoS ONE 7:e41229. doi: 10.1371/journal.pone.0041229
Lawrence, T. (2009). The nuclear factor NF-kappaB pathway in inflammation. Cold Spring Harb. Perspect. Biol. 1:a001651. doi: 10.1101/cshperspect.a001651
Li, A., Zhang, X., Zheng, D., Ge, J., Laties, A. M., and Mitchell, C. H. (2011). Sustained elevation of extracellular ATP in aqueous humor from humans with primary chronic angle-closure glaucoma. Exp. Eye Res. 93, 528–533. doi: 10.1016/j.exer.2011.06.020
Lim, J. C., Lu, W., Beckel, J. M., and Mitchell, C. H. (2016). Neuronal release of cytokine IL-3 triggered by mechanosensitive autostimulation of the P2X7 receptor is neuroprotective. Front. Cell. Neurosci. 10:270. doi: 10.3389/fncel.2016.00270
Liu, H. D., Li, W., Chen, Z. R., Hu, Y. C., Zhang, D. D., Shen, W., et al. (2013). Expression of the NLRP3 inflammasome in cerebral cortex after traumatic brain injury in a rat model. Neurochem. Res. 38, 2072–2083. doi: 10.1007/s11064-013-1115-z
Liu, Y., Xiao, Y., and Li, Z. (2011). P2X7 receptor positively regulates MyD88-dependent NF-kappaB activation. Cytokine 55, 229–236. doi: 10.1016/j.cyto.2011.05.003
Lu, W., Albalawi, F., Lim, J. C., Beckel, J. M., and Mitchell, C. H. (2017). The P2X7 receptor links mechanical strain to cytokine IL-6 upregulation and release in neurons and astrocytes. J. Neurochem. 141, 436–448. doi: 10.1111/jnc.13998
Lu, W., Beckel, J., Lim, J., Zode, G., Sheffield, V., Laties, A., et al. (2013). Elevation of IOP triggers responses from cytokines IL-6 and IL-1β; involvement of both optic nerve head astrocytes and retinal ganglion cells. Invest. Ophthalmol. Vis. Sci. 54, 784.
Lu, W., Hu, H., Sévigny, J., Gabelt, B. T., Kaufman, P. L., Johnson, E. C., et al. (2015). Rat, mouse, and primate models of chronic glaucoma show sustained elevation of extracellular ATP and altered purinergic signaling in the posterior eye. Invest. Ophthalmol. Vis. Sci. 56, 3075–3083. doi: 10.1167/iovs.14-15891
Lye-Barthel, M., Sun, D., and Jakobs, T. C. (2013). Morphology of astrocytes in a glaucomatous optic nerve. Invest. Ophthalmol. Vis. Sci. 54, 909–917. doi: 10.1167/iovs.12-10109
Mandal, A., Shahidullah, M., Delamere, N. A., and Teran, M. A. (2009). Elevated hydrostatic pressure activates sodium/hydrogen exchanger-1 in rat optic nerve head astrocytes. Am. J. Physiol. Cell Physiol. 297, C111–C120. doi: 10.1152/ajpcell.00539.2008
Mariathasan, S., Weiss, D. S., Newton, K., McBride, J., O’Rourke, K., Roose-Girma, M., et al. (2006). Cryopyrin activates the inflammasome in response to toxins and ATP. Nature 440, 228–232. doi: 10.1038/nature04515
Masin, M., Young, C., Lim, K., Barnes, S. J., Xu, X. J., Marschall, V., et al. (2012). Expression, assembly and function of novel C-terminal truncated variants of the mouse P2X7 receptor: re-evaluation of P2X7 knockouts. Br. J. Pharmacol. 165, 978–993. doi: 10.1111/j.1476-5381.2011.01624.x
Miras-Portugal, M. T., Diaz-Hernandez, J. I., Gomez-Villafuertes, R., Diaz-Hernandez, M., Artalejo, A. R., and Gualix, J. (2015). Role of P2X7 and P2Y2 receptors on alpha-secretase-dependent APP processing: control of amyloid plaques formation “in vivo” by P2X7 receptor. Comput. Struct. Biotechnol. J. 13, 176–181. doi: 10.1016/j.csbj.2015.02.005
Mitchell, C. H., Albalawi, F., Lim, J., and Lu, W. (2016). Priming of the NLRP3 inflammasome in optic nerve head astrocytes by mechanical strain and stimulation of the P2X7 receptor. Invest. Ophthalmol. Vis. Sci. 57:3009.
Mitchell, C. H., Albalawi, F., and Lu, W. (2017). Increased IOP primes the NLRP3 inflammasome and increases IL-1β levels. Invest. Ophthalmol. Vis. Sci. 58:851.
Mitchell, C. H., Lu, W., Hu, H., Zhang, X., Reigada, D., and Zhang, M. (2009). The P2X7 receptor in retinal ganglion cells: a neuronal model of pressure-induced damage and protection by a shifting purinergic balance. Purinergic Signal. 5, 241–249. doi: 10.1007/s11302-009-9142-6
Morgan, J. E. (2000). Optic nerve head structure in glaucoma: astrocytes as mediators of axonal damage. Eye 14(Pt 3B), 437–444. doi: 10.1038/eye.2000.128
Morrison, J. C., Cepurna, W. O., Doser, T. A., Dyck, J. A., and Johnson, E. C. (2010). A short interval of controlled elevation of IOP (CEI) reproduces early chronic glaucoma model optic nerve head (ONH) gene expression responses. Invest. Ophthalmol. Vis. Sci. 51, 5216.
Morrison, J. C., Choe, T. E., Cepurna, W. O., and Johnson, E. C. (2014). Optic nerve head (ONH) gene expression responses to elevated intraocular pressure (IOP), anesthesia and anterior chamber cannulation in the CEI (Controlled Elevation of IOP) model of IOP-induced optic nerve injury. Invest. Ophthalmol. Vis. Sci. 55, 2402.
Muñoz-Planillo, R., Kuffa, P., Martínez-Colón, G., Smith, B. L., Rajendiran, T. M., and Núñez, G. (2013). K+ efflux is the common trigger of NLRP3 inflammasome activation by bacterial toxins and particulate matter. Immunity 38, 1142–1153. doi: 10.1016/j.immuni.2013.05.016
Nadal-Nicolas, F. M., Galindo-Romero, C., Valiente-Soriano, F. J., Barbera-Cremades, M., deTorre-Minguela, C., Salinas-Navarro, M., et al. (2016). Involvement of P2X7 receptor in neuronal degeneration triggered by traumatic injury. Sci. Rep. 6:38499. doi: 10.1038/srep38499
Patel, M. N., Carroll, R. G., Galván-Peña, S., Mills, E. L., Olden, R., Triantafilou, M., et al. (2017). Inflammasome priming in sterile inflammatory disease. Trends Mol. Med. 23, 165–180. doi: 10.1016/j.molmed.2016.12.007
Perregaux, D., and Gabel, C. A. (1994). Interleukin-1 beta maturation and release in response to ATP and nigericin. Evidence that potassium depletion mediated by these agents is a necessary and common feature of their activity. J. Biol. Chem. 269, 15195–15203.
Petrilli, V., Papin, S., Dostert, C., Mayor, A., Martinon, F., and Tschopp, J. (2007). Activation of the NALP3 inflammasome is triggered by low intracellular potassium concentration. Cell Death Differ. 14, 1583–1589. doi: 10.1038/sj.cdd.4402195
Rathinam, V. A., Vanaja, S. K., and Fitzgerald, K. A. (2012). Regulation of inflammasome signaling. Nat. Immunol. 13, 333–342. doi: 10.1038/ni.2237
Reigada, D., Lu, W., Zhang, M., and Mitchell, C. H. (2008). Elevated pressure triggers a physiological release of ATP from the retina: possible role for pannexin hemichannels. Neuroscience 157, 396–404. doi: 10.1016/j.neuroscience.2008.08.036
Reigada, D., Lu, W., Zhang, X., Friedman, C., Pendrak, K., McGlinn, A., et al. (2005). Degradation of extracellular ATP by the retinal pigment epithelium. Am. J. Physiol. Cell Physiol. 289, C617–C624. doi: 10.1152/ajpcell.00542.2004
Sanderson, J., Dartt, D. A., Trinkaus-Randall, V., Pintor, J., Civan, M. M., Delamere, N. A., et al. (2014). Purines in the eye: recent evidence for the physiological and pathological role of purines in the RPE, retinal neurons, astrocytes, Müller cells, lens, trabecular meshwork, cornea and lacrimal gland. Exp. Eye Res. 127, 270–279. doi: 10.1016/j.exer.2014.08.009
Sappington, R. M., and Calkins, D. J. (2006). Pressure-induced regulation of IL-6 in retinal glial cells: involvement of the ubiquitin/proteasome pathway and NFkappaB. Invest. Ophthalmol. Vis. Sci. 47, 3860–3869. doi: 10.1167/iovs.05-1408
Schindelin, J., Rueden, C. T., Hiner, M. C., and Eliceiri, K. W. (2015). The ImageJ ecosystem: an open platform for biomedical image analysis. Mol. Reprod. Dev. 82, 518–529. doi: 10.1002/mrd.22489
Sperlagh, B., and Illes, P. (2014). P2X7 receptor: an emerging target in central nervous system diseases. Trends Pharmacol. Sci. 35, 537–547. doi: 10.1016/j.tips.2014.08.002
Stutz, A., Golenbock, D. T., and Latz, E. (2009). Inflammasomes: too big to miss. J. Clin. Invest. 119, 3502–3511. doi: 10.1172/JCI40599
Tehrani, S., Davis, L., Cepurna, W. O., Choe, T. E., Lozano, D. C., Monfared, A., et al. (2016). Astrocyte structural and molecular response to elevated intraocular pressure occurs rapidly and precedes axonal tubulin rearrangement within the optic nerve head in a rat model. PLoS ONE 11:e0167364. doi: 10.1371/journal.pone.0167364
Thornberry, N. A., Bull, H. G., Calaycay, J. R., Chapman, K. T., Howard, A. D., Kostura, M. J., et al. (1992). A novel heterodimeric cysteine protease is required for interleukin-1 beta processing in monocytes. Nature 356, 768–774. doi: 10.1038/356768a0
Walsh, J. G., Muruve, D. A., and Power, C. (2014). Inflammasomes in the CNS. Nat. Rev. Neurosci. 15, 84–97. doi: 10.1038/nrn3638
Xia, J., Lim, J. C., Lu, W., Beckel, J. M., Macarak, E. J., Laties, A. M., et al. (2012). Neurons respond directly to mechanical deformation with pannexin-mediated ATP release and autostimulation of P2X7 receptors. J. Physiol. 590, 2285–2304. doi: 10.1113/jphysiol.2012.227983
Yilmaz, O., and Lee, K. L. (2015). The inflammasome and danger molecule signaling: at the crossroads of inflammation and pathogen persistence in the oral cavity. Periodontol. 2000, 69, 83–95. doi: 10.1111/prd.12084
Zhang, X., Li, A., Ge, J., Reigada, D., Laties, A. M., and Mitchell, C. H. (2007). Acute increase of intraocular pressure releases ATP into the anterior chamber. Exp. Eye Res. 85, 637–643. doi: 10.1016/j.exer.2007.07.016
Zhang, X., Zhang, M., Laties, A. M., and Mitchell, C. H. (2005). Stimulation of P2X7 receptors elevates Ca2+ and kills retinal ganglion cells. Invest. Ophthalmol. Vis. Sci. 46, 2183–2191. doi: 10.1167/iovs.05-0052
Keywords: IL-1β, astrocytes, glaucoma, pannexin, ATP release, NFκB, caspase 1, NLRP3
Citation: Albalawi F, Lu W, Beckel JM, Lim JC, McCaughey SA and Mitchell CH (2017) The P2X7 Receptor Primes IL-1β and the NLRP3 Inflammasome in Astrocytes Exposed to Mechanical Strain. Front. Cell. Neurosci. 11:227. doi: 10.3389/fncel.2017.00227
Received: 04 April 2017; Accepted: 19 July 2017;
Published: 08 August 2017.
Edited by:
Valery I. Shestopalov, University of Miami, United StatesReviewed by:
Stefania Ceruti, Università degli Studi di Milano, ItalyElsa Fabbretti, University of Nova Gorica, Slovenia
Copyright © 2017 Albalawi, Lu, Beckel, Lim, McCaughey and Mitchell. This is an open-access article distributed under the terms of the Creative Commons Attribution License (CC BY). The use, distribution or reproduction in other forums is permitted, provided the original author(s) or licensor are credited and that the original publication in this journal is cited, in accordance with accepted academic practice. No use, distribution or reproduction is permitted which does not comply with these terms.
*Correspondence: Claire H. Mitchell, Y2htQHVwZW5uLmVkdQ==