- 1Department of Pharmacology, Universidad de Extremadura, Cáceres, Spain
- 2ARADyAL Network, Instituto de Salud Carlos III, Madrid, Spain
- 3Department of Psychiatry, Universidad de Extremadura, Badajoz, Spain
- 4Research Laboratory, Instituto de Investigación Biomédica de Málaga, Regional University Hospital of Malaga, UMA, Malaga, Spain
- 5Section of Neurology, Hospital Universitario del Sureste, Madrid, Spain
Background: The Gamma-aminobutyric acid type A receptor (GABA-A receptor) is affected by ethanol concentrations equivalent to those reached during social drinking. At these concentrations, ethanol usually causes impairment in reaction and motor times in most, but not all, individuals.
Objectives: To study the effect of GABA-A receptor variability in motor and reaction times, and the effect of low ethanol doses.
Methods: Two hundred and fifty healthy subjects received one single dose of 0.5 g/Kg ethanol per os. Reaction and motor times were determined before ethanol challenge (basal), and when participants reached peak ethanol concentrations. We analyzed all common missense polymorphisms described in the 19 genes coding for the GABA-A receptor subunits by using TaqMan probes.
Results: The GABRA6 rs4454083 T/C polymorphisms were related to motor times, with individuals carrying the C/C genotype having faster motor times, both, at basal and at peak ethanol concentrations. The GABRA4 rs2229940 T/T genotype was associated to faster reaction times and with lower ethanol effects, determined as the difference between basal reaction time and reaction time at peak concentrations. All these associations remained significant after correction for multiple comparisons. No significant associations were observed for the common missense SNPs GABRB3 rs12910925, GABRG2 rs211035, GABRE rs1139916, GABRP rs1063310, GABRQ rs3810651, GABRR1 rs12200969 or rs1186902, GABRR2 rs282129, and GABRR3 rs832032.
Conclusions: This study provides novel information supporting a role of missense GABA-A receptor polymorphisms in reaction time, motor time and effects of low ethanol doses in vivo.
Introduction
The Gamma-aminobutyric acid type A receptor (GABA-A receptor) is a ligand-gated ion channel that plays a key role in the inhibition of neurotransmission because, when activated by GABA, it causes neuronal hyperpolarization. The activation of the GABA-A receptor by ethanol has long been well-known (Hunt, 1983; Kulonen, 1983; Leidenheimer and Harris, 1992; Aguayo et al., 2002; Chester and Cunningham, 2002; Lobo and Harris, 2008; Kumar et al., 2009b; Zuo et al., 2017), and it is related to the motor effects of ethanol. Independent studies have shown that ethanol exposure modulates neuronal function by potentiating GABA-A effects, whereas ethanol effects are blocked by GABA-A antagonists such as bicuculline, Ro15-1788, or picrotoxin (Mehta and Ticku, 1988; Ticku, 1989, 1990; Allan et al., 1991; Miczek et al., 1997). Moreover, GABA-A antagonists seem to reduce ethanol self-administration (Chester and Cunningham, 2002; Holtyn et al., 2017) and GABA system has been proposed as a therapeutic target for alcoholism therapy (Caputo and Bernardi, 2010; Liang and Olsen, 2014; Long et al., 2017; Prisciandaro et al., 2017). In addition, chronic ethanol exposure modifies the expression of some GABA-A receptors subunits (Wallner et al., 2014; Wang et al., 2014; Bekdash and Harrison, 2015; Ortiz et al., 2015; Bohnsack et al., 2016, 2017; Carlson et al., 2016), thus suggesting the involvement of GABA-A in ethanol tolerance and dependence (Mhatre and Ticku, 1993; Grobin et al., 2000; Davies, 2003; Kumar et al., 2004; Blednov et al., 2017; Caputo et al., 2017; Chandler et al., 2017; Lieberman et al., 2017; Lindemeyer et al., 2017; McCabe et al., 2017). Other effects induced by chronic ethanol exposure on GABA-A are changes in receptor function, phosphorylation, synaptic localization and changes in the receptor subunit composition (Kumar et al., 2004; Follesa et al., 2006; Weiner and Valenzuela, 2006; Enoch, 2008).
Human GABA-A receptors are pentamers, composed of a combination of 19 different subunits, namely, six GABRα (GABRα1 to GABRα6), three GABRβ (GABRβ1 to GABRβ3), three GABRγ (GABRγ1 to GABRγ3), one each of GABRδ, GABRε, GABRπ and GABRθ (GABRQ) and three GABRρ (GABRρ1 to GABRρ3). The major isoform consists of α1, β2, and γ2 subunits, but many other combinations exist, and it is widely accepted that the subunit combination plays a key role in the function of these receptors (Olsen and Sieghart, 2008, 2009; Sigel and Steinmann, 2012; Martenson et al., 2017). Some subunits and some combinations seem to be more sensitive to ethanol effects than others. It has been shown that highest sensitivity to ethanol is observed with the combination GABRα4 + GABRβ3 + GABRδ and GABRα6 + GABRβ3 + GABRδ (Hanchar et al., 2004). Receptors with such particular subunit combinations are affected by low ethanol concentrations (Wallner et al., 2006; Lovinger and Homanics, 2007), although these findings are not free of controversy (Borghese and Harris, 2007; Botta et al., 2007; Jia et al., 2007; Korpi et al., 2007; Mody et al., 2007; Olsen et al., 2007; Santhakumar et al., 2007).
The response to ethanol in vivo is very variable and many factors, besides variability in ethanol metabolism, modify ethanol response. Sex differences in the sensitivity of GABA-A receptors have been shown (Finn et al., 2010) but, interestingly, it has been reported that most of the interindividual variability in the response to ethanol depends on inheritance (Matsushita and Higuchi, 2014). This suggest that genetic variations may play a relevant role in ethanol effects.
In animal models, alteration in the response to ethanol has been related to amino-acid changes in some GABA-A receptor subunits (Loh and Ball, 2000), and it has been suggested that inter- individual variability in the motor impairment caused by low doses of ethanol are related to genetic variability in genes coding for GABA-A receptor subunits (Korpi, 1994; Wallner et al., 2006). For instance, in animal models, a mutation in the GABRα6 subunit has been associated with motor impairment and ethanol sensitivity (Wallner et al., 2006). Information about the putative role of GABA-A genetic variations in ethanol effects in humans, however, is scarce.
The 19 human genes coding for GABA-A receptor subunits contain several common missense polymorphisms (Table 1). Some of the amino acid substitutions have been classified as deleterious according to Sorting Intolerant From Tolerant (SIFT) (Kumar et al., 2009a) and/or Polymorphism Phenotyping v2 (PolyPhen-2) prediction (Adzhubei et al., 2010). In a previous study we observed that a relevant percentage of the population, when exposed to low ethanol doses, did not diminish their reaction time and motor time, but rather were not significantly affected or it even improved their performance (Martinez et al., 2010). The reason for this dimorphism in the response to ethanol is unknown, although we demonstrated that it is not related to ethanol pharmacokinetics (Martinez et al., 2010). This raises the question of whether pharmacodynamic mechanisms, or genetic predisposition, may be involved in such inter-individual variability to ethanol response. In this study, we investigated whether common missense GABR polymorphisms are modifiers of response to low doses of ethanol in vivo, with the focus on effects on motor and reaction times.
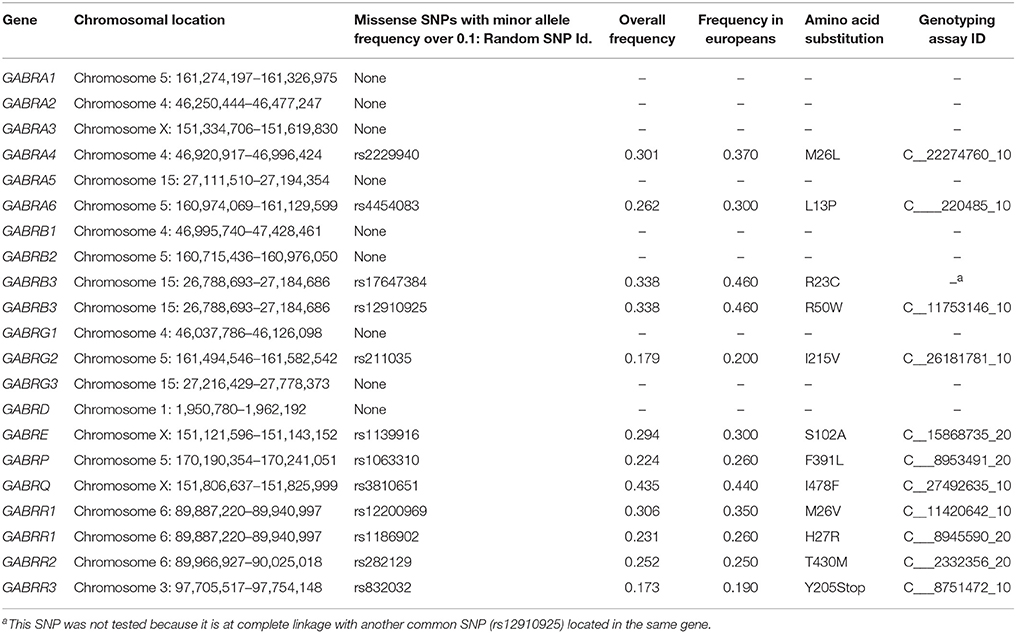
Table 1. Common stop and missense SNPs in GABR genes and allele frequencies according the 1,000 genomes database.
Methods
This study was performed on a group of individuals involved in a previous study on the effect of variability of genes coding for ethanol-metabolizing enzymes in ethanol pharmacokinetics and effects (Martinez et al., 2010). The study group consisted of 250 healthy individuals (66 men and 184 women), with a mean age equal to 22.5 ± 6.2 years. All were Spanish Caucasian individuals recruited at the Medical School of the University of Extremadura (Badajoz, Spain) among students and staff. The inclusion criteria included absence of ethanol consumption, absence of consumption of drugs with known effects in the nervous system, as well as illicit drugs 7 days before the study. We recorded data on age, sex, smoking, and drinking habits. Signed informed consent was obtained for all participants. The protocol of the study was approved by the Ethics Committee of the University Hospital Infanta Cristina (Badajoz, Spain).
Ethanol Challenge and Determination of Ethanol Effects
The design of ethanol challenge and the pharmacokinetic analysis is described elsewhere (Martinez et al., 2010). In brief, participants started the challenge at the same hour, after overnight fasting. Participants received 0.5 g/Kg ethanol per os as liquor containing 17% ethanol. In order to determine when the peak concentration was reached, breath samples were measured every 15 min by using a breath analyzer Alcotest-7710 (Dräger Safety, Spain). Pharmacokinetic determinations were carried out until ethanol levels reached values lower than 0.02 g/L in air, or during 5 h.
After training, participants carried out tests designed to determine reaction time and motor time by using the Vienna Test System 5.20 (Dr. G. Schuhfried GMBH 2003, Austria). Each participant carried out one test just before the ethanol challenge and then one test every 30 min. Each time, 16 measurements of reaction time and motor time were carried out, and the average values for these determinations were used for further analyses. Test takers had their finger on a gold button, moving it to press a black button when they perceived a determined visual stimuli. Reaction time is defined as the time lapsed between displaying the stimulus and removing the finger from the gold button, and motor time is defined as the time lapsed between removing the finger from the gold button until the black button is pressed. To assess ethanol effect on the reaction times and in the motor times, we compared these parameters at basal conditions (the average of the results before ethanol administration and after ethanol concentration in breath were lower than 0.05 g/L) with those obtained at peak ethanol concentration.
Genotyping
Genomic DNA was obtained from venous blood as described elsewhere. The selection of the non-synonymous GABRR polymorphisms analyzed was made according to the expected allele frequencies in the population study previously reported in public databases such as the public 1,000 genomes database1 The study was designed to investigate all non-synonymous and/or nonsense SNPs with minor allele frequencies (MAF) higher than 0.1 for individuals of European descent, for the 19 GABA-A receptor genes described in Table 1. SNPs with lower MAF were not included in the study because the statistical power would be insufficient to obtain conclusive evidence. According to the 1,000 genomes database, 12 SNPs fulfilled the selection criteria. However, two GABRB3 SNPs, namely rs12910925 and rs17647384, are at complete linkage, and therefore only the former was included in the study. Genotyping was carried out in triplicate using SNP TaqMan assays (Life Technologies S.A., Alcobendas, Madrid, Spain). Further details on Assay ID are provided in Table 1.
Statistical Analyses
Statistical analyses were carried out by using the IBM SPSS Statistical Package Version 22 (International Business Machines Corporation, Madrid, Spain). Intergroup comparison values were calculated by using the T-test when appropriate. After normality test, multiple comparison of means was carried out by using the Kruskal–Wallis test for independent samples, because most parameters did not follow a normal distribution. Multiple testing correction was carried out by means of the False Discovery Rate (FDR) procedure (Benjamini and Hochberg, 1995). Haplotypes were calculated as described elsewhere (Mateos-Munoz et al., 2016). The Hardy–Weinberg equilibrium was analyzed through the DeFinetti procedure (http://ihg.gsf.de/cgi-bin/hw/hwa1.pl).
Results
GABRA genotyping results stratified by sex are summarized in Table 2. The genotypes and allele frequencies observed for the SNP analyzed are consistent with those reported elsewhere for healthy Spanish individuals (Garcia-Martin et al., 2011a,b, 2017) and with those reported in public databases2 All SNPs were at Hardy-Weinberg's equilibrium with the exception of the SNPs GABRE rs1139916 and GABRQ rs3810651, which correspond to genes located in the X chromosome (as shown in Table 1). These two SNPs were at Hardy-Weinberg's equilibrium in women (Pearson's goodness-of-fit chi-square P = 0.361 and P = 0.707, respectively), but not in men, as is to be expected in genes located on chromosome X.
The ethanol challenge was designed to obtain low peak concentrations, comparable to the ethanol concentrations reached during social drinking, that is, between two and four drinks depending on body weight (one drink being equal to 14 g of pure ethanol). In the population studied, peak concentrations in breath ranged from 0.19 to 0.65 g/L (average 0.42 ± 0.09 g/L) and peak concentrations were reached in the range 15 to 90 min after ethanol ingestion (mean 37.0 ± 16.8 min). We described previously in this study group that no statistically significant associations of reaction or motor times with peak concentration or drinking habits were observed, but average motor values, both, basal, and peak motor times, were shorter in men than in women (Martinez et al., 2010), and the statistical association remained significant after FDR correction for multiple testing (Pc = 0.030 for basal motor time and Pc = 0.012 for peak motor time). The finding that motor times are faster in men than in women was reported previously in another study involving different participants (Jimenez-Jimenez et al., 2011).
In order to test the putative effect of the GABR genotypes analyzed on reaction and motor times, we stratified the population according to the 11 SNPs studied. Table 3 shows the distribution of the reaction times according to GABR genotypes. Basal reaction time, peak reaction time, and the difference (peak time minus basal time) were shorter among individuals carrying the GABRA4 rs2229940 T/T genotype, as compared to individuals carrying at least one non-mutated gene. The K–W test remains significant for peak reaction time and the difference (peak time minus basal time), even after correction for multiple comparison. In addition, the T-test revealed significant differences when comparing individuals with the rs2229940 T/T genotype with individuals carrying at least one non-mutated gene: P = 0.031, P < 0.001 and P = 0.001 for basal, peak and the difference in reaction time, respectively. This implies that individuals with GABRA4 receptors with a leucine in position 26 react faster, and are less sensitive to ethanol, as compared with individuals that have a methionine instead. The percentage of variation of the basal functional response was 14.97 ± 15.23 for individuals with the G/G genotype, 14.07 ± 13.04 for individuals with the G/T genotype, and 6.51 ± 14.34 for individuals with the T/T genotype (K–W test P = 0.005). Another significant effect was observed in the difference in the reaction time for individuals with GABRR1 rs12200969 C/C genotype (crude P = 0.017; Table 3). The percentage of variation of the basal functional response was 15.60 ± 14.91 for individuals with the T/T genotype, 10.49 ± 13.14 for individuals with the T/C genotype, and 10.92 ± 14.20 for individuals with the C/C genotype (K–W test P = 0.017). However, in this case the K–W test did not remain significant after correction for multiple comparisons, and nor did the T-test when individuals were compared with the rs12200969 C/C genotype with individuals carrying at least one non-mutated gene: P = 0.068.
Table 4 shows the distribution of the motor times according to GABR genotypes. Basal motor time and peak motor time were shorter among individuals carrying the GABRA6 rs4454083 C/C genotype, as compared with individuals carrying at least one non-mutated gene. The K–W test remains significant for both motor times after correction for multiple comparisons. In addition, the T-test reveals very significant differences when individuals with the rs2229940 T/T genotype were compared, with individuals carrying at least one non-mutated gene: P < 0.001 and P = 0.001 for basal and peak motor time, respectively. This implies that individuals with GABRA6 receptors with a proline in the position 13 instead of leucine have faster motor times. In contrast, no statistically significant effects related to ethanol (that is, the difference between basal motor time minus peak motor time) were observed. The percentage of variation of the basal functional response was 8.38 ± 17.15 for individuals with the T/T genotype, 6.84 ± 17.94 for individuals with the T/C genotype, and 8.99 ± 23.56 for individuals with the C/C genotype (K–W test P = 0.746). No other associations with motor times were identified when other GABR genotypes were analyzed.
Table 5 shows the haplotype analysis results for the whole study group. Due to the high frequency of variant GABR alleles, no common haplotypes were observed. The most common haplotype had a frequency of about 6%, and rare haplotypes (with frequencies lower than 1%) accounted for almost 50% of all haplotypes identified. For that reason, and in order to refine the putative genetic associations, the effect of inferred haplotypes on reaction and motor times was studied for the SNPs that displayed any association in Tables 3, 4, the SNPs associated to the most common GABA-A receptor configuration and the SNPs associated to the subunit combinations that are the most sensitive to ethanol. Table 6 shows the association of haplotypes, adjusted by sex, with reaction and motor times. Several statistically significant associations were identified, even after FDR correction for multiple comparisons. However, some haplotypes caused modest changes in reaction or motor times. In addition, some haplotypes that caused larger changes are extremely rare. Table 6 was sorted by type of effect, then statistical significance and then effect intensity. Only two particular effects corresponded unambiguously to specific variant haplotype distributions, as compared to the reference haplotype GTCGT. These are the haplotype GCTGC (corresponding to variant alleles in GABRA6 and GABRB3), which is related to slower basal movement time, and the haplotype TTTAC (corresponding to variant alleles in GABRA4, GABRB3, GABRG2, and GABRR1), which is related to faster peak movement time. These two haplotypes, however, are extremely rare.
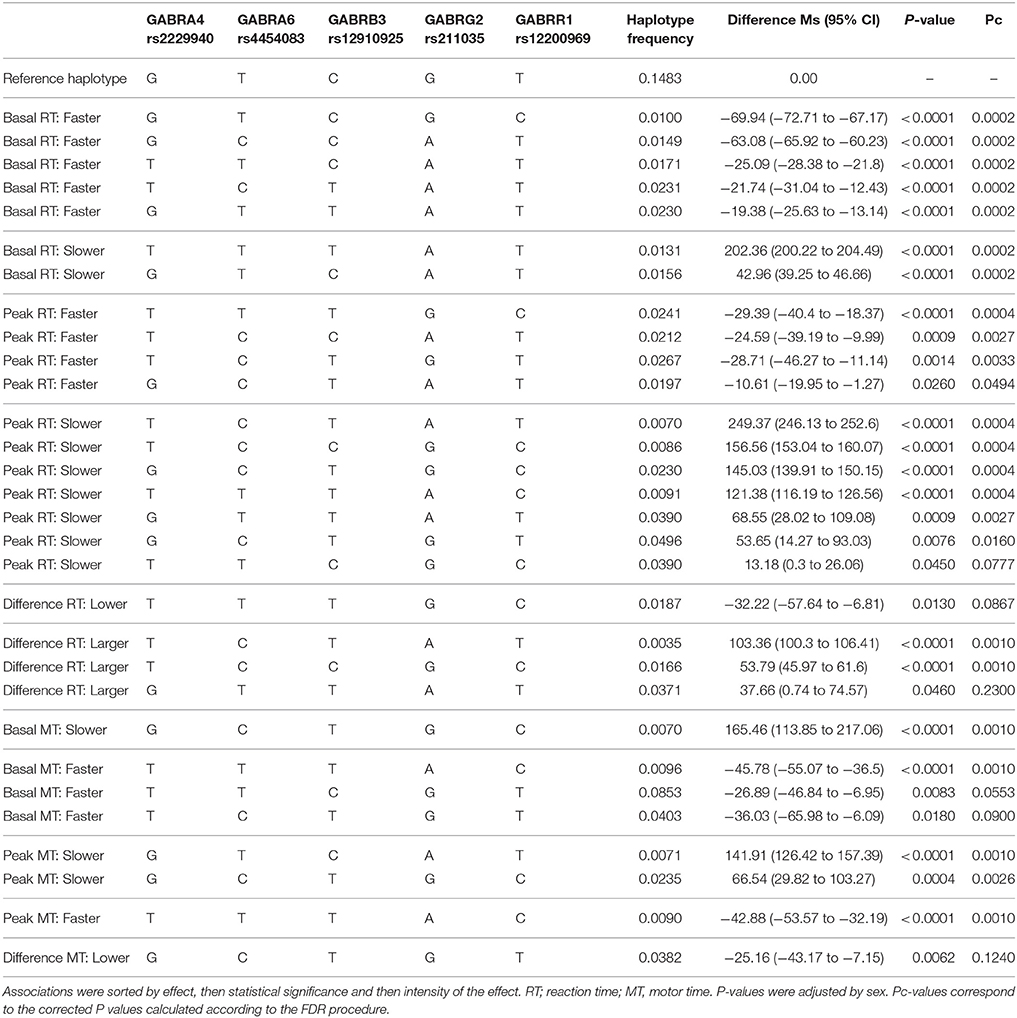
Table 6. Statistically significant interactions of selected GABR haplotypes with reaction and motor times.
Discussion
Genetic variation in GABR genes has been related to ethanol effects in animal models, with controversial findings (Hanchar et al., 2004; Wallner et al., 2006; Borghese and Harris, 2007; Botta et al., 2007; Jia et al., 2007; Korpi et al., 2007; Lovinger and Homanics, 2007; Mody et al., 2007; Olsen et al., 2007; Santhakumar et al., 2007). This study analyzes for the first time the effect of common human GABR missense polymorphisms in reaction time, motor time and low-dose ethanol effects in vivo. Human GABR genes showed a modest inter-individual variability as compared to other genes. No common missense or stop polymorphisms have been described for GABRA1, 2, 3, and 5. In contrast, the genes GABRA4 and GABRA6 have one common missense polymorphism each. These genes encode subunits that have been reported to be related to low-dose ethanol effects (Hanchar et al., 2004; Wallner et al., 2006; Lovinger and Homanics, 2007) and, indeed, one of these polymorphisms is associated in this study to inter-individual differences in reaction times and in ethanol effects (GABRA4 rs2229940), whereas the other one is related to inter-individual differences in the motor times (GABRA6 rs4454083) although not to ethanol effects. In both cases, variant subunits are associated with faster times, which is consistent with a functional impairment in inhibitory receptors, such as GABA-A receptors. The amino acid substitution caused by the GABRA4 polymorphism rs2229940 is predicted as deleterious by using SIFT and as benign by using PolyPhen, and it was reported to display a marginal association with nicotine dependence (Agrawal et al., 2008, 2009). Predictions for the GABRA6 polymorphism are also deleterious with SIFT, with low confidence, possibly damaging with PolyPhen, but no clinical associations have been yet described.
Besides GABRα4 and GABRα6, the GABA-A subunit combinations containing GABRβ3 and GABRδ are in the model proposed by Hanchar et al. as the primary targets for ethanol (Hanchar et al., 2004). GABRB3 has two common missense polymorphisms (Table 1) that are at complete linkage, and that in the present study are not associated to reaction times, motor times or ethanol effects (Tables 3, 4). As for GABRD, no common missense SNPs have been described (Table 1). Hence, among ethanol-sensitive subunit combinations, the strongest candidates to play a role in the genetic variability of ethanol effects are GABRα4 and GABRα6.
The rest of the common missense GABR gene variations did not show in this study a major association with reaction times, motor times, or ethanol effects (Tables 3, 4). Regarding the putative clinical associations for these polymorphisms, the GABRG2 SNP designated as rs211035 was previously investigated in relation to epilepsy with negative results (Dixit et al., 2016). It has been proposed that the GABRE SNP designated as rs1139916 affects the binding of extracellular ligands, and it has been associated with migraine risk in women, with carriers of variant alleles being at decreased risk (Quintas et al., 2013). Our own findings confirm this observation (García-Martín et al., 2018). In addition, a weak association of this SNP with some types of breast cancer has been identified in an exome sequencing study (Li et al., 2015). The GABRP rs1063310 SNP has been analyzed as a putative predisposing factor for autism, although no significant association was identified (Ma et al., 2005). Association of the GABRQ SNP rs3810651 with response to antidepressants has been claimed in a single study carried out on Chinese individuals (Pu et al., 2013). This SNP has also been analyzed as a putative risk factor for essential tremor, with negative findings (Garcia-Martin et al., 2011a). A putative association of heterozygosity (T/A genotype) with increased risk of developing migraine has been described (Quintas et al., 2013), although these findings could not be replicated by our group (García-Martín et al., 2018). GABRR1 SNPs rs1186902 and rs12200969 were analyzed as putative risk factors related to ethanol dependence, but no association was identified (Xuei et al., 2010). Conversely, the GABRR2 SNP rs282129 was significantly related to ethanol dependence (Xuei et al., 2010), and recently it has been claimed to be related to cognitive ability (Ma et al., 2017).
In summary, we identified for the first time functional consequences in vivo of two common missense GABR polymorphisms. These concern the subunits GABRα4 and GABRα6, which have been reported to be part of sensitive subunit combinations to ethanol. It could be speculated that GABA-A receptors containing such subunits could possibly cause selective changes in reaction and motor time, respectively, by being expressed in different brain regions. Interesting findings regarding a differential effect of extrasynaptic and synaptic GABA inhibition with similar GABA concentrations have been reported (Mooney et al., 2017), and these findings raise evidences supporting effects related to receptor location. The findings regarding the effect of these polymorphisms on reaction times and movement times may have additional clinical implications in movement disorders: Patients with essential tremor and Parkinson's disease have slower reaction times and/or motor times as compared to healthy subjects (Montgomery et al., 2000; Jimenez-Jimenez et al., 2010). In patients with restless syndrome motor impairment exists too, although it is restricted to some movements (Jimenez-Jimenez et al., 2009). GABR alteration may be related to the clinical presentation of movement disorders: for instance, increased expression of GABRα4 has been described in tremor rats (Mao et al., 2011), and our own findings suggest that the GABRA4 rs2229940 T/T genotype is related to younger age at onset of restless legs syndrome (Jiménez-Jiménez et al. submitted). In the light of the findings obtained in the present study, it would be interesting to verify in further studies whether the GABRA4 rs2229940 and the GABRA6 rs4454083 polymorphisms, isolated or combined, are related to clinical presentation, and particularly to severity, reaction time or motor impairment, in patients suffering from movement disorders.
Author Contributions
EG-M, JA: conception and design of the work, interpretation of data, drafting the work, final approval of the version to be published and agreement to be accountable for all aspects of the work in ensuring that questions related to the accuracy or integrity of any part of the work are appropriately investigated and resolved. MR, SG, LR-S: Data acquisition and analysis, interpretation of data for the work, revising critically the work for important intellectual content, final approval of the version to be published and agreement to be accountable for all aspects of the work in ensuring that questions related to the accuracy or integrity of any part of the work are appropriately investigated and resolved. JC-G, JP, HA-N, FJ-J: Interpretation of data for the work, revising critically the work for important intellectual content, final approval of the version to be published and agreement to be accountable for all aspects of the work in ensuring that questions related to the accuracy or integrity of any part of the work are appropriately investigated and resolved.
Funding
We are grateful to Prof. James McCue for assistance in language editing. This work was supported in part by Grants PI15/00303, and ARADyAL RD16/0006/0001 and RD16/0006/0004 from Fondo de Investigación Sanitaria, Instituto de Salud Carlos III, Spain, IB16170 and GR15026 from Junta de Extremadura, Spain. Financed in part with FEDER funds from the European Union. JC-G is a researcher from the Miguel Servet Program (Ref CP14/00034), and JP from the Sara Borrell Program (Ref CD14/00242).
Conflict of Interest Statement
The authors declare that the research was conducted in the absence of any commercial or financial relationships that could be construed as a potential conflict of interest.
Footnotes
1. ^http://phase3browser.1000genomes.org/Homo_sapiens/Info/Index.
2. ^http://phase3browser.1000genomes.org/Homo_sapiens/Info/Index.
References
Adzhubei, I. A., Schmidt, S., Peshkin, L., Ramensky, V. E., Gerasimova, A., Bork, P., et al. (2010). A method and server for predicting damaging missense mutations. Nat. Methods 7, 248–249. doi: 10.1038/nmeth0410-248
Agrawal, A., Pergadia, M. L., Balasubramanian, S., Saccone, S. F., Hinrichs, A. L., Saccone, N. L., et al. (2009). Further evidence for an association between the gamma-aminobutyric acid receptor A, subunit 4 genes on chromosome 4 and Fagerstrom Test for Nicotine Dependence. Addiction 104, 471–477. doi: 10.1111/j.1360-0443.2008.02445.x
Agrawal, A., Pergadia, M. L., Saccone, S. F., Hinrichs, A. L., Lessov-Schlaggar, C. N., Saccone, N. L., et al. (2008). Gamma-aminobutyric acid receptor genes and nicotine dependence: evidence for association from a case-control study. Addiction 103, 1027–1038. doi: 10.1111/j.1360-0443.2008.02236.x
Aguayo, L. G., Peoples, R. W., Yeh, H. H., and Yevenes, G. E. (2002). GABA(A) receptors as molecular sites of ethanol action. Direct or indirect actions? Curr. Top. Med. Chem. 2, 869–885. doi: 10.2174/1568026023393426
Allan, A. M., Burnett, D., and Harris, R. A. (1991). Ethanol-induced changes in chloride flux are mediated by both GABA(A) and GABA(B) receptors. Alcohol. Clin. Exp. Res. 15, 233–237. doi: 10.1111/j.1530-0277.1991.tb01862.x
Bekdash, R. A., and Harrison, N. L. (2015). Downregulation of Gabra4 expression during alcohol withdrawal is mediated by specific microRNAs in cultured mouse cortical neurons. Brain Behav. 5:e00355. doi: 10.1002/brb3.355
Benjamini, Y., and Hochberg, Y. (1995). Controlling the false discovery rate: a practical and powerful approach to multiple testing. J. R. Stat. Soc. Ser. B 57, 289–300.
Blednov, Y. A., Borghese, C. M., Ruiz, C. I., Cullins, M. A., Da Costa, A., Osterndorff-Kahanek, E. A., et al. (2017). Mutation of the inhibitory ethanol site in GABAA rho1 receptors promotes tolerance to ethanol-induced motor incoordination. Neuropharmacology 123, 201–209. doi: 10.1016/j.neuropharm.2017.06.013
Bohnsack, J. P., Carlson, S. L., and Morrow, A. L. (2016). Differential regulation of synaptic and extrasynaptic alpha4 GABA(A) receptor populations by protein kinase A and protein kinase C in cultured cortical neurons. Neuropharmacology 105, 124–132. doi: 10.1016/j.neuropharm.2016.01.009
Bohnsack, J. P., Patel, V. K., and Morrow, A. L. (2017). Ethanol exposure regulates Gabra1 expression via histone deacetylation at the promoter in cultured cortical neurons. J. Pharmacol. Exp. Ther. 363, 1–11. doi: 10.1124/jpet.117.242446
Borghese, C. M., and Harris, R. A. (2007). Studies of ethanol actions on recombinant delta-containing gamma-aminobutyric acid type A receptors yield contradictory results. Alcohol 41, 155–162. doi: 10.1016/j.alcohol.2007.03.006
Botta, P., Radcliffe, R. A., Carta, M., Mameli, M., Daly, E., Floyd, K. L., et al. (2007). Modulation of GABAA receptors in cerebellar granule neurons by ethanol: a review of genetic and electrophysiological studies. Alcohol 41, 187–199. doi: 10.1016/j.alcohol.2007.04.004
Caputo, F., and Bernardi, M. (2010). Medications acting on the GABA system in the treatment of alcoholic patients. Curr. Pharm. Des. 16, 2118–2125. doi: 10.2174/138161210791516468
Caputo, F., Ciminelli, B. M., Jodice, C., Blasi, P., Vignoli, T., Cibin, M., et al. (2017). Alcohol use disorder and GABAB receptor gene polymorphisms in an Italian sample: haplotype frequencies, linkage disequilibrium and association studies. Ann. Hum. Biol. 44, 384–388. doi: 10.1080/03014460.2017.1287307
Carlson, S. L., Bohnsack, J. P., Patel, V., and Morrow, A. L. (2016). Regulation of extrasynaptic GABAA alpha4 receptors by ethanol-induced protein kinase, A., but not protein kinase C activation in cultured rat cerebral cortical neurons. J. Pharmacol. Exp. Ther. 356, 148–156. doi: 10.1124/jpet.115.228056
Chandler, C. M., Overton, J. S., Rüedi-Bettschen, D., and Platt, D. M. (2017). GABAA receptor subtype mechanisms and the abuse-related effects of ethanol: genetic and pharmacological evidence. Handb Exp Pharmacol. doi: 10.1007/164_2017_80. [Epub ahead of print].
Chester, J. A., and Cunningham, C. L. (2002). GABA(A) receptor modulation of the rewarding and aversive effects of ethanol. Alcohol 26, 131–143. doi: 10.1016/S0741-8329(02)00199-4
Davies, M. (2003). The role of GABAA receptors in mediating the effects of alcohol in the central nervous system. J. Psychiatry Neurosci. 28, 263−274.
Dixit, A. B., Banerjee, J., Ansari, A., Tripathi, M., and Chandra, S. P. (2016). Mutations in GABRG2 receptor gene are not a major factor in the pathogenesis of mesial temporal lobe epilepsy in Indian population. Ann. Indian Acad. Neurol. 19, 236–241. doi: 10.4103/0972-2327.182304
Enoch, M. A. (2008). The role of GABA(A) receptors in the development of alcoholism. Pharmacol. Biochem. Behav. 90, 95–104. doi: 10.1016/j.pbb.2008.03.007
Finn, D. A., Beckley, E. H., Kaufman, K. R., and Ford, M. M. (2010). Manipulation of GABAergic steroids: sex differences in the effects on alcohol drinking- and withdrawal-related behaviors. Horm. Behav. 57, 12–22. doi: 10.1016/j.yhbeh.2009.07.002
Follesa, P., Biggio, F., Talani, G., Murru, L., Serra, M., Sanna, E., et al. (2006). Neurosteroids, GABAA receptors, and ethanol dependence. Psychopharmacology 186, 267–280. doi: 10.1007/s00213-005-0126-0
García-Martín, E., Esguevillas, G., Serrador, M., Alonso-Navarro, H., Navacerrada, F., Amo, G., et al. (2018). Gamma-aminobutyric acid (GABA) receptors GABRA4, GABRE, and GABRQ gene polymorphisms and risk for migraine. J. Neural Transm. (Vienna). doi: 10.1007/s00702-017-1834-4. [Epub ahead of print].
García-Martín, E., Martínez, C., Alonso-Navarro, H., Benito-Leon, J., Lorenzo-Betancor, O., Pastor, P., et al. (2011a). Gamma-aminobutyric acid GABRA4, GABRE, and GABRQ receptor polymorphisms and risk for essential tremor. Pharmacogenet. Genomics 21, 436–439. doi: 10.1097/FPC.0b013e328345bec0
García-Martín, E., Martínez, C., Alonso-Navarro, H., Benito-Leon, J., Lorenzo-Betancor, O., Pastor, P., et al. (2011b). Gamma-aminobutyric acid (GABA) receptor rho (GABRR) polymorphisms and risk for essential tremor. J. Neurol. 258, 203–211. doi: 10.1007/s00415-010-5708-z
García-Martín, E., Martínez, C., Serrador, M., Alonso-Navarro, H., Navacerrada, F., Esguevillas, G., et al. (2017). Gamma-aminobutyric acid (Gaba) receptors rho (Gabrr) gene polymorphisms and risk for migraine. Headache 57, 1118–1135. doi: 10.1111/head.13122
Grobin, A. C., Papadeas, S. T., and Morrow, A. L. (2000). Regional variations in the effects of chronic ethanol administration on GABA(A) receptor expression: potential mechanisms. Neurochem. Int. 37, 453–461. doi: 10.1016/S0197-0186(00)00058-9
Hanchar, H. J., Wallner, M., and Olsen, R. W. (2004). Alcohol effects on gamma-aminobutyric acid type A receptors: are extrasynaptic receptors the answer? Life Sci. 76, 1–8. doi: 10.1016/j.lfs.2004.05.035
Holtyn, A. F., Tiruveedhula, V. V., Stephen, M. R., Cook, J. M., and Weerts, E. M. (2017). Effects of the benzodiazepine GABAA alpha1-preferring antagonist 3-isopropoxy-beta-carboline hydrochloride (3-ISOPBC) on alcohol seeking and self-administration in baboons. Drug Alcohol Depend. 170, 25–31. doi: 10.1016/j.drugalcdep.2016.10.036
Hunt, W. A. (1983). The effect of ethanol on GABAergic transmission. Neurosci. Biobehav. Rev. 7, 87–95. doi: 10.1016/0149-7634(83)90009-X
Jia, F., Pignataro, L., and Harrison, N. L. (2007). GABAA receptors in the thalamus: alpha4 subunit expression and alcohol sensitivity. Alcohol 41, 177–185. doi: 10.1016/j.alcohol.2007.03.010
Jiménez-Jiménez, F. J., Calleja, M., Alonso-Navarro, H., Rubio, L., Navacerrada, F., Pilo-de-la-Fuente, B., et al. (2011). Influence of age and gender in motor performance in healthy subjects. J. Neurol. Sci. 302, 72–80. doi: 10.1016/j.jns.2010.11.021
Jiménez-Jiménez, F. J., Rubio, L., Alonso-Navarro, H., Calleja, M., Pilo-de-la-Fuente, B., Plaza-Nieto, J. F., et al. (2010). Impairment of rapid repetitive finger movements and visual reaction time in patients with essential tremor. Eur. J. Neurol. 17, 152–159. doi: 10.1111/j.1468-1331.2009.02784.x
Jimenez-Jimenez, F. J., Rubio, L., Calleja, M., Alonso-Navarro, H., Turpin-Fenoll, L., Plaza-Nieto, J. F., et al. (2009). Motor performance in patients with restless legs syndrome. Mov. Disord. 24, 1656–1661. doi: 10.1002/mds.22650
Korpi, E. R. (1994). Role of GABAA receptors in the actions of alcohol and in alcoholism: recent advances. Alcohol Alcohol. 29, 115–129.
Korpi, E. R., Debus, F., Linden, A. M., Malécot, C., Leppä, E., Vekovischeva, O., et al. (2007). Does ethanol act preferentially via selected brain GABAA receptor subtypes? The current evidence is ambiguous. Alcohol 41, 163–176. doi: 10.1016/j.alcohol.2007.03.007
Kumar, P., Henikoff, S., and Ng, P. C. (2009a). Predicting the effects of coding non-synonymous variants on protein function using the SIFT algorithm. Nat. Protoc. 4, 1073–1081. doi: 10.1038/nprot.2009.86
Kumar, S., Fleming, R. L., and Morrow, A. L. (2004). Ethanol regulation of gamma-aminobutyric acid A receptors: genomic and nongenomic mechanisms. Pharmacol. Ther. 101, 211–226. doi: 10.1016/j.pharmthera.2003.12.001
Kumar, S., Porcu, P., Werner, D. F., Matthews, D. B., Diaz-Granados, J. L., Helfand, R. S., et al. (2009b). The role of GABA(A) receptors in the acute and chronic effects of ethanol: a decade of progress. Psychopharmacology 205, 529–564. doi: 10.1007/s00213-009-1562-z
Leidenheimer, N. J., and Harris, R. A. (1992). Acute effects of ethanol on GABAA receptor function: molecular and physiological determinants. Adv. Biochem. Psychopharmacol. 47, 269–279.
Li, Y., Wang, X., Vural, S., Mishra, N. K., Cowan, K. H., and Guda, C. (2015). Exome analysis reveals differentially mutated gene signatures of stage, grade and subtype in breast cancers. PLoS ONE 10:e0119383. doi: 10.1371/journal.pone.0119383
Liang, J., and Olsen, R. W. (2014). Alcohol use disorders and current pharmacological therapies: the role of GABA(A) receptors. Acta Pharmacol. Sin. 35, 981–993. doi: 10.1038/aps.2014.50
Lieberman, R., Kranzler, H. R., Levine, E. S., and Covault, J. (2017). Examining the effects of alcohol on GABAA receptor mRNA expression and function in neural cultures generated from control and alcohol dependent donor induced pluripotent stem cells. Alcohol 66, 45–53. doi: 10.1016/j.alcohol.2017.08.005
Lindemeyer, A. K., Shen, Y., Yazdani, F., Shao, X. M., Spigelman, I., Davies, D. L., et al. (2017). alpha2 subunit-containing GABAA receptor subtypes are upregulated and contribute to alcohol-induced functional plasticity in the rat hippocampus. Mol. Pharmacol. 92, 101–112. doi: 10.1124/mol.116.107797
Lobo, I. A., and Harris, R. A. (2008). GABA(A) receptors and alcohol. Pharmacol. Biochem. Behav. 90, 90–94. doi: 10.1016/j.pbb.2008.03.006
Loh, E. W., and Ball, D. (2000). Role of the GABA(A)beta2, GABA(A)alpha6, GABA(A)alpha1 and GABA(A)gamma2 receptor subunit genes cluster in drug responses and the development of alcohol dependence. Neurochem. Int. 37, 413–423. doi: 10.1016/S0197-0186(00)00054-1
Long, D., Long, B., and Koyfman, A. (2017). The emergency medicine management of severe alcohol withdrawal. Am. J. Emerg. Med. 35, 1005–1011. doi: 10.1016/j.ajem.2017.02.002
Lovinger, D. M., and Homanics, G. E. (2007). Tonic for what ails us? High-affinity GABAA receptors and alcohol. Alcohol 41, 139–143. doi: 10.1016/j.alcohol.2007.03.008
Ma, D. Q., Whitehead, P. L., Menold, M. M., Martin, E. R., Ashley-Koch, A. E., Mei, H., et al. (2005). Identification of significant association and gene-gene interaction of GABA receptor subunit genes in autism. Am. J. Hum. Genet. 77, 377–388. doi: 10.1086/433195
Ma, Z., Niu, B., Shi, Z., Li, J., Wang, J., Zhang, F., et al. (2017). Genetic polymorphism of GABRR2 modulates individuals' general cognitive ability in healthy Chinese Han people. Cell. Mol. Neurobiol. 37, 93–100. doi: 10.1007/s10571-016-0347-2
Mao, X., Ma, P., Cao, D., Sun, C., Ji, Z., Min, D., et al. (2011). Altered expression of GABAA receptors (alpha4, gamma2 subunit), potassium chloride cotransporter 2 and astrogliosis in tremor rat hippocampus. Brain Res. Bull. 86, 373–379. doi: 10.1016/j.brainresbull.2011.09.002
Martenson, J. S., Yamasaki, T., Chaudhury, N. H., Albrecht, D., and Tomita, S. (2017). Assembly rules for GABAA receptor complexes in the brain. Elife 6:e30826. doi: 10.7554/eLife.27443
Martínez, C., Galván, S., Garcia-Martin, E., Ramos, M. I., Gutierrez-Martin, Y., and Agundez, J. A. (2010). Variability in ethanol biodisposition in whites is modulated by polymorphisms in the ADH1B and ADH1C genes. Hepatology 51, 491–500. doi: 10.1002/hep.23341
Mateos-Muñoz, B., García-Martín, E., Torrejón, M. J., Devesa-Medina, M. J., Esguevillas, G., Cardenas, M. C., et al. (2016). GC gene polymorphism and unbound serum retinol-binding protein 4 are related to the risk of insulin resistance in patients with chronic hepatitis C: a prospective cross-sectional study. Medicine 95:e3019. doi: 10.1097/MD.0000000000003019
Matsushita, S., and Higuchi, S. (2014). Genetic differences in response to alcohol. Handb. Clin. Neurol. 125, 617–627. doi: 10.1016/B978-0-444-62619-6.00036-7
McCabe, W. A., Way, M. J., Ruparelia, K., Knapp, S., Ali, M. A., Anstee, Q. M., et al. (2017). Genetic variation in GABRbeta1 and the risk for developing alcohol dependence. Psychiatr. Genet. 27, 110–115. doi: 10.1097/YPG.0000000000000169
Mehta, A. K., and Ticku, M. K. (1988). Ethanol potentiation of GABAergic transmission in cultured spinal cord neurons involves gamma-aminobutyric acidA-gated chloride channels. J. Pharmacol. Exp. Ther. 246, 558–564.
Mhatre, M. C., and Ticku, M. K. (1993). Alcohol: effects on GABAA receptor function and gene expression. Alcohol Alcohol. Suppl. 2, 331–335. doi: 10.1007/978-1-4615-2470-0_16
Miczek, K. A., DeBold, J. F., van Erp, A. M., and Tornatzky, W. (1997). Alcohol, GABAA-benzodiazepine receptor complex, and aggression. Recent Dev. Alcohol. 13, 139–171.
Mody, I., Glykys, J., and Wei, W. (2007). A new meaning for “Gin & Tonic”: tonic inhibition as the target for ethanol action in the brain. Alcohol 41, 145–153. doi: 10.1016/j.alcohol.2007.03.009
Montgomery, E. B. Jr., Baker, K. B., Lyons, K., and Koller, W. C. (2000). Motor initiation and execution in essential tremor and Parkinson's disease. Mov. Disord. 15, 511–515. doi: 10.1002/1531-8257(200005)15:3<511::AID-MDS1014>3.0.CO;2-R
Mooney, R. A., Cirillo, J., and Byblow, W. D. (2017). GABA and primary motor cortex inhibition in young and older adults: a multimodal reliability study. J. Neurophysiol. 118, 425–433. doi: 10.1152/jn.00199.2017
Olsen, R. W., and Sieghart, W. (2008). International Union of Pharmacology. LXX. Subtypes of gamma-aminobutyric acid(A) receptors: classification on the basis of subunit composition, pharmacology, and function. Update. Pharmacol. Rev. 60, 243–260. doi: 10.1124/pr.108.00505
Olsen, R. W., and Sieghart, W. (2009). GABA A receptors: subtypes provide diversity of function and pharmacology. Neuropharmacology 56, 141–148. doi: 10.1016/j.neuropharm.2008.07.045
Olsen, R. W., Hanchar, H. J., Meera, P., and Wallner, M. (2007). GABAA receptor subtypes: the “one glass of wine” receptors. Alcohol 41, 201–209. doi: 10.1016/j.alcohol.2007.04.006
Ortiz, V., Giachero, M., Espejo, P. J., Molina, V. A., and Martijena, I. D. (2015). The effect of Midazolam and Propranolol on fear memory reconsolidation in ethanol-withdrawn rats: influence of d-cycloserine. Int. J. Neuropsychopharmacol. 18:pyu082. doi: 10.1093/ijnp/pyu082
Prisciandaro, J. J., Tolliver, B. K., Prescot, A. P., Brenner, H. M., Renshaw, P. F., Brown, T. R., et al. (2017). Unique prefrontal GABA and glutamate disturbances in co-occurring bipolar disorder and alcohol dependence. Transl. Psychiatry 7:e1163. doi: 10.1038/tp.2017.141
Pu, M., Zhang, Z., Xu, Z., Shi, Y., Geng, L., Yuan, Y., et al. (2013). Influence of genetic polymorphisms in the glutamatergic and GABAergic systems and their interactions with environmental stressors on antidepressant response. Pharmacogenomics 14, 277–288. doi: 10.2217/pgs.13.1
Quintas, M., Neto, J. L., Pereira-Monteiro, J., Barros, J., Sequeiros, J., Sousa, A., et al. (2013). Interaction between gamma-aminobutyric acid A receptor genes: new evidence in migraine susceptibility. PLoS ONE 8:e74087. doi: 10.1371/journal.pone.0074087
Santhakumar, V., Wallner, M., and Otis, T. S. (2007). Ethanol acts directly on extrasynaptic subtypes of GABAA receptors to increase tonic inhibition. Alcohol 41, 211–221. doi: 10.1016/j.alcohol.2007.04.011
Sigel, E., and Steinmann, M. E. (2012). Structure, function, and modulation of GABA(A) receptors. J. Biol. Chem. 287, 40224–40231. doi: 10.1074/jbc.R112.386664
Ticku, M. K. (1989). Ethanol and the benzodiazepine-GABA receptor-ionophore complex. Experientia 45, 413–418. doi: 10.1007/BF01952022
Ticku, M. K. (1990). Alcohol and GABA-benzodiazepine receptor function. Ann. Med. 22, 241–246. doi: 10.3109/07853899009148934
Wallner, M., Hanchar, H. J., and Olsen, R. W. (2006). Low dose acute alcohol effects on GABA A receptor subtypes. Pharmacol. Ther. 112, 513–528. doi: 10.1016/j.pharmthera.2006.05.004
Wallner, M., Hanchar, H. J., and Olsen, R. W. (2014). Alcohol selectivity of beta3-containing GABAA receptors: evidence for a unique extracellular alcohol/imidazobenzodiazepine Ro15-4513 binding site at the alpha+beta- subunit interface in alphabeta3delta GABAA receptors. Neurochem. Res. 39, 1118–1126. doi: 10.1007/s11064-014-1243-0
Wang, S., Luo, Y., Feng, A., Li, T., Yang, X., Nofech-Mozes, R., et al. (2014). Ethanol induced impairment of glucose metabolism involves alterations of GABAergic signaling in pancreatic beta-cells. Toxicology 326, 44–52. doi: 10.1016/j.tox.2014.10.005
Weiner, J. L., and Valenzuela, C. F. (2006). Ethanol modulation of GABAergic transmission: the view from the slice. Pharmacol. Ther. 111, 533–554. doi: 10.1016/j.pharmthera.2005.11.002
Xuei, X., Flury-Wetherill, L., Dick, D., Goate, A., Tischfield, J., Nurnberger, J. Jr., et al. (2010). GABRR1 and GABRR2, encoding the GABA-A receptor subunits rho1 and rho2, are associated with alcohol dependence. Am. J. Med. Genet. B Neuropsychiatr. Genet. 153B, 418–427. doi: 10.1002/ajmg.b.30995
Keywords: GABA-A receptors, ethanol, polymorphism, single nucleotide, reaction time, movement time
Citation: García-Martín E, Ramos MI, Cornejo-García JA, Galván S, Perkins JR, Rodríguez-Santos L, Alonso-Navarro H, Jiménez-Jiménez FJ and Agúndez JAG (2018) Missense Gamma-Aminobutyric Acid Receptor Polymorphisms Are Associated with Reaction Time, Motor Time, and Ethanol Effects in Vivo. Front. Cell. Neurosci. 12:10. doi: 10.3389/fncel.2018.00010
Received: 09 November 2017; Accepted: 08 January 2018;
Published: 31 January 2018.
Edited by:
Antonio Gambardella, Magna Græcia University, ItalyReviewed by:
Enrico Sanna, Università degli Studi di Cagliari, ItalyDavid F. Werner, Binghamton University (SUNY), United States
Copyright © 2018 García-Martín, Ramos, Cornejo-García, Galván, Perkins, Rodríguez-Santos, Alonso-Navarro, Jiménez-Jiménez and Agúndez. This is an open-access article distributed under the terms of the Creative Commons Attribution License (CC BY). The use, distribution or reproduction in other forums is permitted, provided the original author(s) and the copyright owner are credited and that the original publication in this journal is cited, in accordance with accepted academic practice. No use, distribution or reproduction is permitted which does not comply with these terms.
*Correspondence: José A. G. Agúndez, amFndW5kZXpAdW5leC5lcw==