- 1Neuroscience Graduate Program, Cumming School of Medicine, University of Calgary, Calgary, AB, Canada
- 2Hotchkiss Brain Institute, Cumming School of Medicine, University of Calgary, Calgary, AB, Canada
- 3Mathison Centre for Mental Health Research and Education, Cumming School of Medicine, University of Calgary, Calgary, AB, Canada
- 4Department of Cell Biology and Anatomy, Cumming School of Medicine, University of Calgary, Calgary, AB, Canada
- 5Department of Psychiatry, Cumming School of Medicine, University of Calgary, Calgary, AB, Canada
- 6Snyder Institute for Chronic Diseases, Cumming School of Medicine, University of Calgary, Calgary, AB, Canada
- 7Department of Physiology and Pharmacology, Cumming School of Medicine, University of Calgary, Calgary, AB, Canada
- 8Department of Psychiatry, Weill Cornell Medical College, New York, NY, United States
Cannabinoids, including cannabis derived phytocannabinoids and endogenous cannabinoids (endocannabinoids), are typically considered anti-inflammatory. One such endocannabinoid is N-arachidonoylethanolamine (anandamide, AEA), which is metabolized by fatty acid amide hydrolase (FAAH). In humans, there is a loss of function single nucleotide polymorphism (SNP) in the FAAH gene (C385A, rs324420), that leads to increases in the levels of AEA. Using a mouse model with this SNP, we investigated how this SNP affects inflammation in a model of inflammatory bowel disease. We administered 2,4,6-trinitrobenzene sulfonic acid (TNBS) intracolonically, to adult male FAAH SNP mice and examined colonic macroscopic tissue damage and myeloperoxidase activity, as well as levels of plasma and amygdalar cytokines and chemokines 3 days after administration, at the peak of colitis. We found that mice possessing the loss of function alleles (AC and AA), displayed no differences in colonic damage or myeloperoxidase activity compared to mice with wild type alleles (CC). In contrast, in plasma, colitis-induced increases in interleukin (IL)-2, leukemia inhibitory factor (LIF), monocyte chemoattractant protein (MCP)-1, and tumor necrosis factor (TNF) were reduced in animals with an A allele. A similar pattern was observed in the amygdala for granulocyte colony stimulating factor (G-CSF) and MCP-1. In the amygdala, the mutant A allele led to lower levels of IL-1α, IL-9, macrophage inflammatory protein (MIP)-1β, and MIP-2 independent of colitis—providing additional understanding of how FAAH may serve as a regulator of inflammatory responses in the brain. Together, these data provide insights into how FAAH regulates inflammatory processes in disease.
Introduction
Cannabinoids typically confer anti-inflammatory effects and provide protection against peripheral inflammatory illnesses, such as inflammatory bowel diseases (IBD) (Picardo et al., 2019; Osafo et al., 2021). Phytocannabinoids, such as Δ9-tetrahydrocannabinol, derived from the Cannabis sativa plant, exert their biological effects through interactions with the endocannabinoid (eCB) system. The eCB system is composed of the lipid signaling molecules, N-arachidonoylethanolamine/anandamide (AEA) and 2-arachidonylglycerol (2-AG); their respective primary biosynthetic [N-acylphospitidyl ethanolamine-phospholipase D (NAPE-PLD) for AEA and diacylglycerol lipase (DAGL) for 2-AG] and metabolizing enzymes [fatty acid amide hydrolase (FAAH) for AEA and monoacylglycerol lipase (MAGL) for 2-AG]; and two receptors, CB1 and CB2 (Katona and Freund, 2012). While both cannabinoid receptors are expressed on immune cells (Munro et al., 1993) and largely act to suppress inflammatory processes, CB2 is the receptor primarily responsible for the regulation of immune function while CB1 is the primary receptor in the brain. The specific mechanisms by which eCBs exert their anti-inflammatory effects include the inhibition of cell proliferation and migration, as well as through suppression of cytokine production (Parolaro, 1999; Nagarkatti et al., 2009).
Numerous studies show that elevating eCB signaling in chronic inflammatory diseases, such as IBD, is protective (Massa et al., 2004; Engel et al., 2008; Marques et al., 2008; Storr et al., 2010). Mice with genetic deletions of cannabinoid receptors have increased susceptibility to IBD (Massa et al., 2004; Storr et al., 2008a,b, 2009; Engel et al., 2010) and collagen-induced arthritis (Kinsey et al., 2011). Alternately, pharmacological inhibition or genetic deletion of FAAH, pharmacological inhibition of MAGL or activation of CB1 improves chemically induced colitis in rodents (Massa et al., 2004; Alhouayek et al., 2011; Fichna et al., 2014; Sałaga et al., 2014; Sasso et al., 2015; Shamran et al., 2017). Furthermore, in neurological inflammatory conditions, including excitotoxic injury (Marsicano et al., 2003), experimental autoimmune encephalitis (EAE) (Walter and Stella, 2004; Rossi et al., 2010; Pryce and Baker, 2012), neurodegenerative diseases (Rossi et al., 2010; Sánchez and García-Merino, 2012; Vázquez et al., 2015), head injury (Panikashvili et al., 2006; Tchantchou et al., 2014), aging (Marchalant et al., 2008), and ischemia (Hayakawa et al., 2008), there are neuroprotective effects of eCBs, primarily through reducing the expression of proinflammatory cytokines.
Inhibiting the degradation of AEA through the administration of FAAH inhibitors is typically anti-inflammatory. FAAH inhibitors or genetic deletion of Faah results in decreased responses to lipopolysaccharide (LPS) administration (Naidu et al., 2007) and reduced inflammation in a carrageenan model of acute inflammation (Holt et al., 2005), both via a CB2 receptor dependent mechanism. Increasing AEA signaling in vivo or in vitro, reduces levels of proinflammatory cytokines and other inflammatory mediators, such as nitric oxide, and increases anti-inflammatory cytokines (Puffenbarger et al., 2000; Chang et al., 2001; Facchinetti et al., 2003; Ortega-Gutiérrez et al., 2005; Tham et al., 2007; Correa et al., 2009, 2010). This also occurs within the central nervous system, where FAAH inhibition reduces the expression of LPS-induced proinflammatory cytokines in the hypothalamus (Kerr et al., 2012).
The amygdala is a brain region that is influenced by inflammation, with different inflammatory stimuli [i.e., rodent models of bacterial infection, systemic cytokine administration or disease models for multiple sclerosis (MS), colitis and arthritis] elevating excitatory neurotransmission within subnuclei of the amygdala, such as the basolateral nucleus (BLA), which is related to associated increases in anxiety-like behavior (Han and Neugebauer, 2004; Porcher et al., 2004; Welch et al., 2005; Chen et al., 2013; Jain et al., 2015; Acharjee et al., 2018; Li et al., 2018; Munshi and Rosenkranz, 2018; Zheng et al., 2021). Furthermore, elevated indices of amygdala activation are also observed in patients with gastrointestinal diseases, including, irritable bowel syndrome, and IBD (Naliboff and Mayer, 2006; Labus et al., 2009, 2013; Agostini et al., 2011; Fukudo and Kanazawa, 2011; Hubbard et al., 2011; Tillisch et al., 2011; Bao et al., 2015; Icenhour et al., 2015; Weaver et al., 2016; Fan et al., 2019). We previously showed in a rodent colitis model that the amygdalar eCB system is altered, and that these changes contribute to anxiety-like behavior in rats (Vecchiarelli et al., 2021). Given that FAAH inhibition ameliorates colitis-induced anxiety, and has the ability to suppress inflammatory processes, it remains possible that one mechanism by which elevated AEA signaling in the amygdala could reduce inflammation-associated anxiety is via the suppression of inflammatory cytokines.
Recently, a knock-in mouse model was developed for the common human single nucleotide polymorphism (SNP) mutation in FAAH (C385A; rs324420) (Dincheva et al., 2015). Individuals with this SNP have FAAH in which a conserved (C) proline (AA129) is substituted with a (A) threonine, which makes FAAH more susceptible to proteolytic degradation (Dincheva et al., 2015). In A allele carriers, there are reduced FAAH protein levels and activity, as well as an increase in AEA levels (Dincheva et al., 2015; Mayo et al., 2020). There is little known about the effect of this SNP on inflammatory processes. Given that inhibiting FAAH is anti-inflammatory, these FAAH C385A SNP mice represent an ideal model system to investigate the effects of elevating AEA on gut inflammation, peripheral and amygdala inflammatory levels in a manner that may shed light on disease vulnerability for humans who possess this SNP. Based on the established role of AEA signaling, inflammation and disease, we hypothesize that reduced FAAH activity (as seen in A carriers) will improve gut inflammation and reduce circulating and amygdalar cytokines.
Materials and Methods
Animals
These studies utilized young adult (7–9 week old), male FAAH C385A mice (Dincheva et al., 2015). These mice were derived from the line previously generated (Dincheva et al., 2015) and back crossed on a C57/Bl6J strain for over twenty generations. Mice were maintained in a specified pathogen free facility and obtained from in-house breeding, which crossed heterozygous males and heterozygous females. This breeding resulted in the following genotypes, all of which were utilized: wild type (CC), heterozygous (AC), and homozygous (AA) for the mutation. Genotyping was performed at the Hotchkiss Brain Institute Molecular Core Facility. Both the AC and AA groups exhibit reduced FAAH activity and increased AEA levels (Dincheva et al., 2015; Mayo et al., 2020). A carriers (i.e., AC and AA groups), as in human studies (Hariri et al., 2009; Dincheva et al., 2015; Gärtner et al., 2019; Lazary et al., 2019), were collapsed (collectively referred to as A, whereas CC are referred to as C) for analysis, as the magnitude of FAAH activity and AEA level changes are similar in both the AA and AC groups (Dincheva et al., 2015). Mice were kept on an 12:12 h light-dark cycle and had ad libitum access to food and water. All experiments were performed during the light phase. All experiments were approved by the Health Sciences Animal Care Committee of the University of Calgary and followed guidelines from the Canadian Council on Animal Care.
Colitis Induction
Under brief isoflurane anesthesia, mice received an intrarectal bolus (approximately 3 cm proximal to the anus) of 2,4,6-trinitrobenzene sulfonic acid (TNBS) [Millipore Sigma, Darmstadt, Germany, #92822; 4 mg; 50% (vol/vol) in ethanol/water], via a plastic cannula. Control animals received the same volume of saline. Body weight was monitored daily. Analysis took place 3 days after TNBS administration, when inflammatory responses peak (Elson et al., 1996).
Macroscopic Tissue Damage
Colons were removed and washed with ice-cold physiological saline (0.9%) and cut open longitudinally and macroscopically scored blindly for damage and inflammation. These scores were based on the presence or absence of adhesions and diarrhea and the degree of ulceration similar to those previously reported (Riazi et al., 2008; Vecchiarelli et al., 2021).
Myeloperoxidase Activity
Following macroscopic tissue damage assessment, a sample of colon was excised, snap frozen, and stored at −80°C for later use in a myeloperoxidase (MPO) activity assay, as previously described (Sigalet et al., 2010; Vecchiarelli et al., 2021). Briefly, samples were homogenized in hexadecyltrimethylammonium bromide (HTAB; Sigma-Aldrich, Darmstadt, Germany, #H5882) in potassium phosphate buffer, homogenized using a 5 mm stainless-steel bead (Qiagen, Hilden, Germany, #69989) and TissueLyser LT bead homogenizer (Qiagen) for 10 min at 5 Hz and centrifuged for 10 min at 15871 × g at 4°C. Supernatant was added to hydrogen peroxide and o-dianisidine dihydrochloride in a 96 well-plate. Absorbance was measured at 450 nm, three times over 1 min (SpectraMax M Plate Reader, Molecular Devices, San Jose, CA, United States). MPO was expressed in milliunits per gram of wet tissue, 1 unit being the quantity of enzyme able to convert 1 μmol of H2O2 to water in 1 min at room temperature. Units of MPO activity per minute were calculated from a standard curve using purified peroxidase enzyme (Sigma-Aldrich, #M6908).
Cytokine/Chemokine Multiplex Assay (Plasma and Amygdala)
As previously described (Vecchiarelli et al., 2016), plasma was separated from trunk blood following collection by centrifugation for 20 min at 10,000 × g at 4°C. Plasma was aliquoted and stored at −80°C. Amygdalae were micro-dissected (Gray et al., 2015; Vecchiarelli et al., 2016, 2021) and stored at −80°C prior to processing for cytokine ELISAs. Samples were placed in 10 μL/mg of homogenization buffer [150 mM sodium chloride, 2.5 mm magnesium chloride, 5 mg/500 mL aprotinin and cOmplete™ protease inhibitors (Millipore Sigma, #11836145001)]. Tissue homogenization occurred with mechanical disruption using a 5 mm stainless steel bead and TissueLyser LT bead homogenizer for 2 min at 50 Hz. Homogenized samples were run through a 0.22 μm spin filter tube (Millipore Sigma, #UFC30GVNB) for 4 min at 12,000 g at 4°C. Total protein quantification was assayed using a Pierce bicinchoninic acid (BCA) assay according to the manufacturer’s protocol (Thermo Fisher Scientific, Waltham, MA, United States, #23225). Samples were diluted to a final protein concentration of 500 μg/mL.
Eotaxin [C-C motif chemokine ligand (CCL)11], granulocyte colony stimulating factor (G-CSF), granulocyte monocyte colony stimulating factor (GM-CSF), interferon (IFN)γ, interleukin (IL)-1α, IL-1β, IL-2, IL-3, IL-4, IL-5, IL-6, IL-7, IL-9, IL-10, IL-12 (p40), IL-12 (p70), IL-13, IL-15, IL-17A, IFNγ inducible protein (IP)-10 (C-X-C motif chemokine ligand 10; CXCL10), KC (CXCL1), leukemia inhibitory factor (LIF), LPS-induced CXC (LIX), monocyte chemoattractant protein (MCP)-1 (CCL2), macrophage colony stimulating factor (M-CSF), monokine induced by IFNγ (MIG), monocyte inflammatory protein (MIP)-1α (CCL3), MIP-1β (CCL4), MIP-2 (CXCL2), regulated on activation normal T cell expressed and secreted (RANTES; CCL5), TNF, and vascular endothelial growth factor (VEGF) were assayed using a Millipore Milliplex Mouse 32-Plex Cytokine- and Chemokine-Array (Millipore Sigma, #MCYTMAG-70K-PX32) by Eve Technologies (Calgary, AB, Canada). The dotted line in each graph in the results represented the limit of detection for each assay and if values were not detected, the minimum standard was utilized instead.
Statistics
Statistics were carried out using Prism v9 (GraphPad, San Diego, CA, United States, RRID:SCR_002798). Outliers were removed using the ROUT method (Motulsky and Brown, 2006), set to a 1% threshold, in the software, as previously described (Vecchiarelli et al., 2016). All data were comparisons between two independent variables, therefore two-way analysis of variance (ANOVA)s were performed. For all ANOVA analyses, interactions and main effects were reported, and relevant comparisons were performed using Fisher’s Least Significant Difference (LSD) tests. F-values and p-values are reported in Supplementary Table 1. Data are presented as mean ± standard error of the mean (SEM). p < 0.05 was considered statistically significant and p < 0.1 was noted.
Results
The Fatty Acid Amide Hydrolase Single Nucleotide Polymorphism Had No Impact on Macroscopic Damage or Gut Inflammation
Colitis was associated with an increased macroscopic tissue damage (Figure 1A), but this was unaffected by the genotype of the mice. Similarly, increased MPO activity was observed in mice with colitis (Figure 1B), but again there was no effect of genotype.
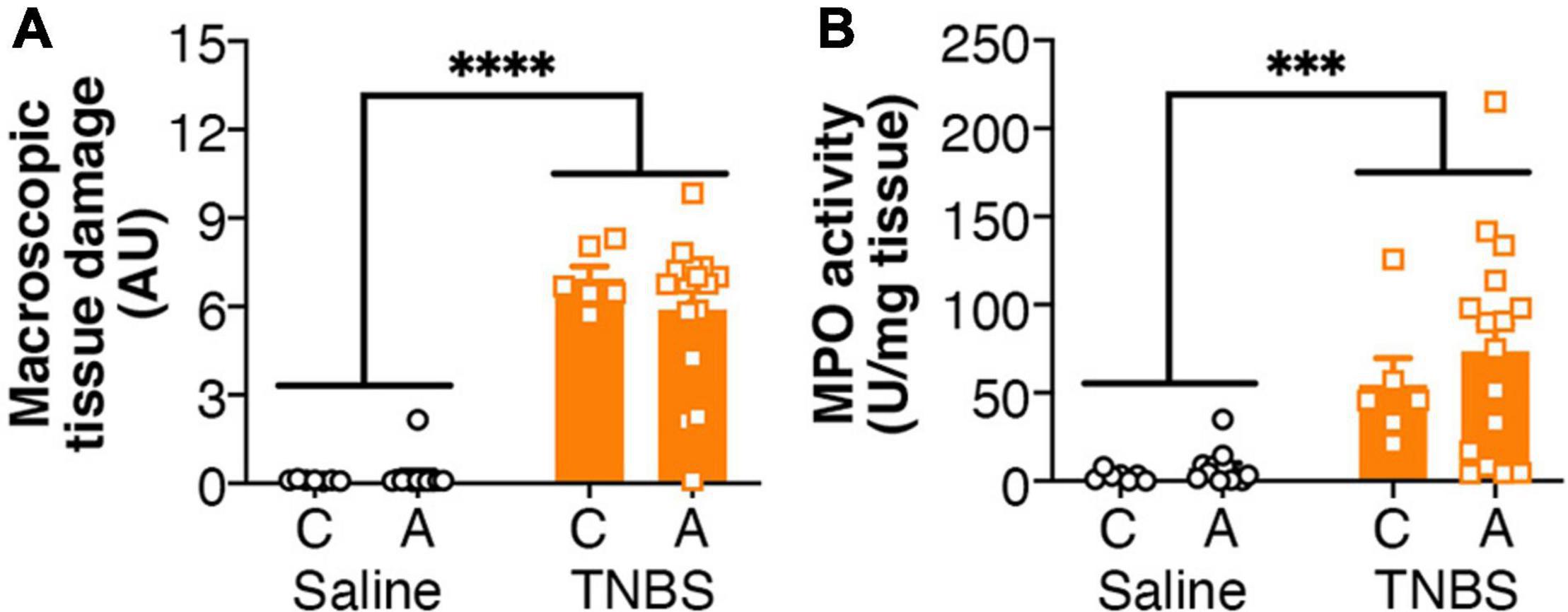
Figure 1. Colitis is not altered by FAAH genotype. Colitis significantly increased (A) macroscopic tissue damage and (B) MPO activity, but there was no effect of genotype, or interaction between the genotype and colitis. n = 6–16/group. ∗∗∗p < 0.001, ∗∗∗∗p < 0.0001, main effect of colitis. Saline = left pair, black bars with circles. TNBS = right pair, orange bars with squares. In each pair, the left bar is the C allele group (CC genotype) and the right bar is the A allele group (AC/AA genotypes).
Genetic Variants of Fatty Acid Amide Hydrolase Influenced Colitis-Induced Alterations in Circulating Cytokines and Chemokines
Both colitis and genotype influenced plasma cytokine levels (Figure 2). For IL-2 and LIF (Figures 2A,B), inflammation-induced increases were reversed with an A genotype, whereas for MCP-1 and TNF (Figures 2C,D), colitis-induced increases were attenuated with an A genotype. For RANTES (Figure 2E), in the colitic mice, animals with an A allele had higher levels than the C allele. For IL-1α, LIX and M-CSF (Figures 2F–H), both colitis and possessing an A allele led to reductions, however, there was no interaction or additive effects between the two conditions. G-CSF, GM-CSF, IL-6, IL-15, IP-10, KC, MIP-1α, and MIP-1β (Figures 2I–P) levels were increased in animals with colitis. Conversely, MIG and MIP-2 (Figures 2Q,R) levels were decreased in animals with colitis. There were no significant changes in in Eotaxin, IFNγ, IL-1β, IL-3, IL-4, IL-5, IL-7, IL-9, IL-10, IL-12p40, 1L-12p70, IL-13, IL-17A, and VEGF levels (Figures 2S–AF) from either colitis or the FAAH SNP.
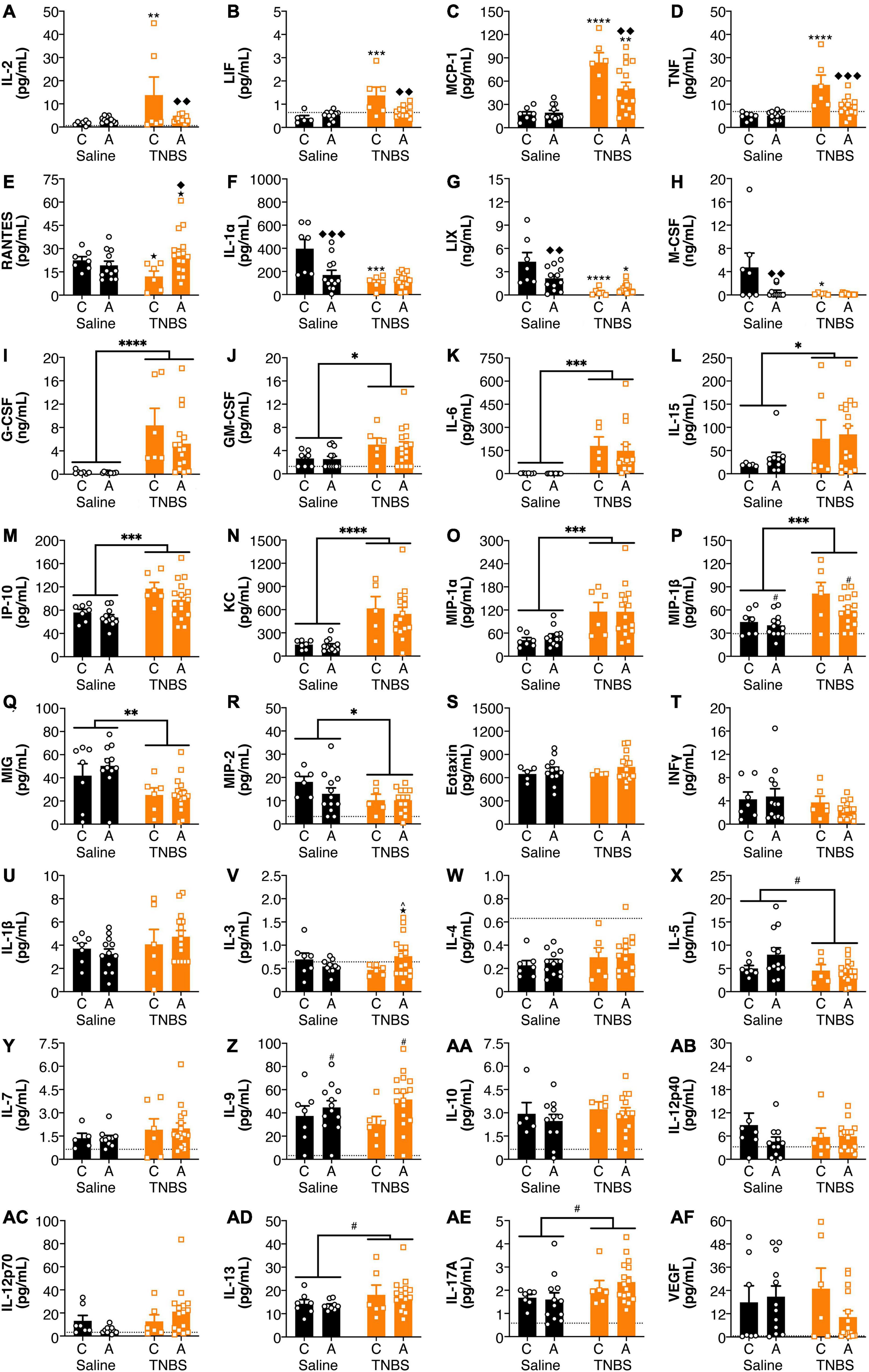
Figure 2. FAAH SNP altered colitis-induced changes in plasma cytokines and chemokines. Having an A allele mitigated colitis-induced increases in (A) IL-2, (B) LIF, (C) MCP-1, and (D) TNF and reductions in (E) RANTES. Both possessing an A allele or colitis reduced levels of (F) IL-1α, (G) LIX, and (H) M-CSF, but there was not an additive effect of both conditions. Colitis increased levels of (I) G-CSF, (J) GM-CSF, (K) IL-6, (L) IL-15, (M) IP-10, (N) KC, (O) MIP-1α and (P) MIP-1β and reduced levels of (Q) MIG and (R) MIP-2. There were no significant changes in (S) Eotaxin, (T) IFNγ, (U) IL-1β, (V) IL-3, (W) IL-4, (X) IL-5, (Y) IL-7, (Z) IL-9, (AA) IL-10, (AB) IL-12p40, (AC) IL-12p70, (AD) IL-13, (AE) IL-17A, and (AF) VEGF. n = 4–16/group. ★p < 0.1, *p < 0.05, **p < 0.01, ***p < 0.001, ****p < 0.0001, saline vs. TNBS of same genotype. ^p < 0.1, ◆p < 0.05, ◆◆p < 0.01, ◆◆◆p < 0.001, C vs. A of same condition (saline or TNBS). #p < 0.1, ∗p < 0.05, ∗∗p < 0.01, ∗∗∗p < 0.001, ∗∗∗∗p < 0.0001 main effect of colitis (lines) or genxotype (symbols above bars). Saline = left pair, black bars with circles. TNBS = right pair, orange bars with squares. In each pair, the left bar is the C allele group (CC genotype) and the right bar is the A allele group (AC/AA genotypes). Dotted lines indicate minimal detection threshold for the analyte and was included in graphs where values were below threshold or the threshold value was used.
Genetic Variants of Fatty Acid Amide Hydrolase Influenced Colitis-Induced Changes in Amygdalar Cytokines and Chemokines
There were effects of both colitis and the FAAH SNP on amygdala cytokine and chemokine levels (Figure 3). There was a reversal of colitis-induced changes in amygdala levels of G-CSF and MCP-1 with an A allele (Figures 3A,B). IL-5 levels (Figure 3C) were lower in the amygdala of animals possessing an A allele following colitis than either the A allele saline group or the C allele colitis group. Colitis increased amygdalar levels of Eotaxin, IL-13 and KC (Figures 3D–F); whereas levels of IL-12p70 and VEGF (Figures 3G,H) were reduced in animals with colitis. Possessing an A allele led to decreased levels of IL-1α, IL-9, MIP-1β, and MIP-2 (Figures 3I–L). There were no significant changes in the amygdala in levels of GM-CSF, IFNγ, IL-1β, IL-2, IL-3, IL-4, IL-6, IL-7, IL-10, IL-12p40, IL-15, IL-17A, IP-10, LIF, M-CSF, MIG, MIP-1α, RANTES, and TNFα (Figures 3M–AE). There were no detectable levels of LIX in the amygdala (data not shown).
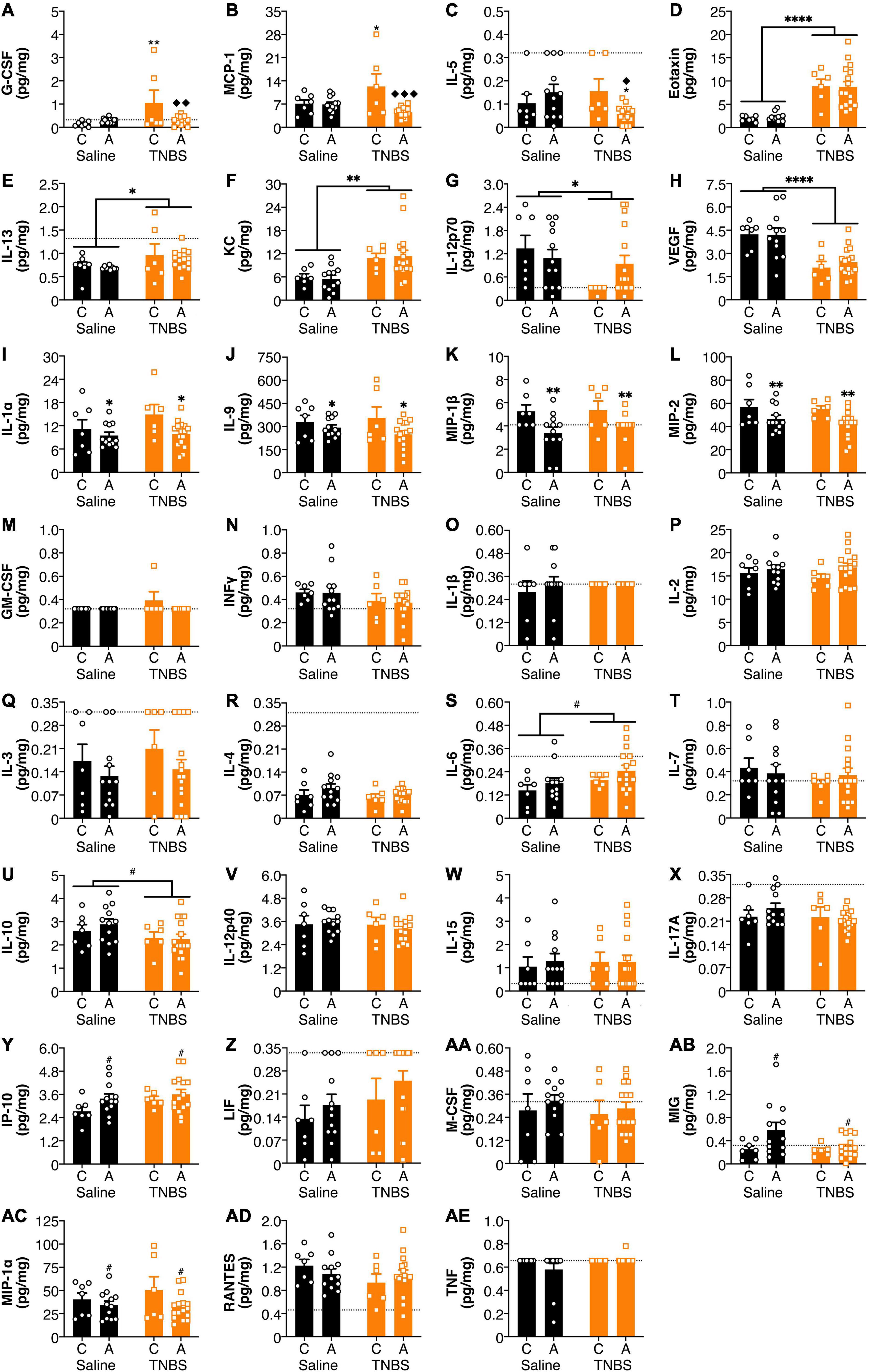
Figure 3. FAAH SNP altered colitis-induced changes in amygdala cytokines and chemokines. Having an A allele mitigated colitis induced increases in (A) G-CSF, (B) MCP-1, and (C) IL-5. Colitis increased levels (D) Eotaxin, (E) IL-13, and (F) KC and reduced levels of (G) IL-12p70 and (H) VEGF. Possessing an A allele led to lower levels of (I) IL-1α, (J) IL-9, (K) MIP-1β, and (L) MIP-2. There were no significant changes in (M) GM-CSF, (N) INFγ, (O) IL-1β, (P) IL-2, (Q) IL-3, (R) IL-4, (S) IL-6, (T) IL-7, (U) IL-10, (V) IL-12p40, (W) IL-15, (X) IL-17A, (Y) IP-10, (Z) LIF, (AA) M-CSF, (AB) MIG, (AC) MIP-1α, (AD) RANTES, and (AE) TNF. n = 5–16/group. *p < 0.05, **p < 0.01, saline vs. TNBS of same genotype. ◆p < 0.05, ◆◆p < 0.01, ◆◆◆p < 0.001, C vs. A of same condition (saline or TNBS). #p < 0.1, ∗p < 0.05, ∗∗p < 0.01, ∗∗∗∗p < 0.0001 main effect of colitis (lines) or genotype (symbols above bars). Saline = left pair, black bars with circles. TNBS = right pair, orange bars with squares. In each pair, the left bar is the C allele group (CC genotype) and the right bar is the A allele group (AC/AA genotypes). Dotted lines indicate minimal detection threshold for the analyte and was included in graphs where values were below threshold or the threshold value was used.
Discussion
The role of the common SNP of FAAH in inflammatory diseases is not well understood. We examined how genetic variants of FAAH could influence the peripheral and neuroinflammatory response in colitis in a recently developed mouse model expressing the human SNP of FAAH (Dincheva et al., 2015). Three days after the induction of colitis corresponds to the peak of inflammation (Elson et al., 1996), and thus we chose to examine this time point. We found that there was no effect of genotype on macroscopic tissue damage or MPO activity in the inflamed colon. Interestingly, however, genetic reduction of FAAH activity significantly attenuated or fully reversed inflammation-induced increases of plasma IL-2, LIF, MCP-1, and TNF; as well as amygdala G-CSF and MCP-1 levels. FAAH reduction in the amygdala leads to reduced IL-1α, IL-9, MIP-1β, and MIP-2 levels.
Many of the colitis-induced cytokines that we found to be influenced by the FAAH SNP (e.g., IL-2, LIF, MCP-1, and TNF), have been found to be regulated by FAAH inhibition in other studies. FAAH inhibition or boosting AEA levels inhibits IL-2 (Rockwell and Kaminski, 2004; Kaplan et al., 2005; Rockwell et al., 2008; Cencioni et al., 2010; Patsenker et al., 2015; Zajkowska et al., 2020), inhibits LIF release (Maccarrone et al., 2001; Maccarrone and Wenger, 2005), and reduces MCP-1 levels (Nakajima et al., 2006; Özdemir et al., 2014; Rivera et al., 2018; Selvaraj et al., 2019; Zhang et al., 2020; Hermes et al., 2021). There are many reports showing that FAAH inhibition or increases in AEA levels reduces TNF levels (Nakajima et al., 2006; Bátkai et al., 2007; Naidu et al., 2007; Tham et al., 2007; Roche et al., 2008; Rettori et al., 2012; Nader et al., 2014; Özdemir et al., 2014; Patsenker et al., 2015; Petrosino et al., 2018; Rivera et al., 2018; Selvaraj et al., 2019; Shang et al., 2020). Following a wide range of inflammatory stimuli, FAAH inhibition or increasing AEA levels also leads to a reduction in IFNγ, IL-1β, IL-6, IL-8, among others (Nakajima et al., 2006; Rettori et al., 2012; Özdemir et al., 2014; Patsenker et al., 2015; Chiurchiù et al., 2016; Petrosino et al., 2018; Rivera et al., 2018; Selvaraj et al., 2019; Zhang et al., 2020). This is, to the best of our knowledge, the first report of FAAH/AEA’s influence on G-CSF levels. Our work is consistent with these previous reports and is the first to report an immunomodulatory effect of this SNP, which reduces FAAH activity, including in the context of central cytokine changes in a model of peripheral inflammatory disease. However, it is unclear why reducing FAAH activity does not attenuate colitis-induced increases in IL-6, given what others have shown. We only measure one time point, and it is possible that without a time course analysis, we are missing subtle dynamics as to the effects of both colitis, FAAH genotype and their combination on cytokine expression levels, both peripherally and centrally.
Intriguingly, our results show no changes in colonic macroscopic tissue damage and MPO activity, but do show the ability of the FAAH SNP to attenuate some changes in plasma cytokines/chemokines. Therefore, it seems unlikely that the attenuation of some plasma cytokines altered with colitis in mice with an A SNP is due to changes in their production in the colon. As such, it seems likely that the elevated levels of anandamide seen in this FAAH SNP may act distal to the colon, likely directly on immune cells in the circulation or resident in the brain, to dampen the release of immunomodulatory cytokines.
Given that we have previously demonstrated that elevations of FAAH within the amygdala following the induction of colitis were associated with the development of anxiety (Vecchiarelli et al., 2021), which in peripheral inflammatory contexts is known to be driven by inflammatory cytokines within the amygdala proper (Chen et al., 2013), these data also suggest that a potential mechanism by which elevated AEA signaling may be able to dampen inflammation-associated anxiety is via a suppression of inflammatory cytokine levels within the amygdala.
FAAH reductions ameliorate colitis-induced increases in circulating IL-2, LIF, MCP-1, and TNF, which implies that FAAH activity 3 days after TNBS administration may contribute to T cell differentiation, proliferation and migration, as well as monocyte trafficking in this model, perhaps by altering levels of AEA. Faah expression has been shown to be increased in the proximal colon in this model of inflammation (Storr et al., 2008a), indicating that colitis may alter FAAH at this time point, thus suggesting that the loss of function FAAH SNP we are exploring in the current study may confer some immunomodulatory benefit by reducing the impacts of this upregulation of FAAH. We also see that at baseline FAAH activity indirectly regulates other inflammatory molecules in the amygdala, particularly IL-9, MIP-1β, and MIP-2, which stimulate mast cells and neutrophils (Ren et al., 2010; Rojas-Zuleta and Sanchez, 2017), as well as IL-1α, contributing to our understanding of AEA as a potential modulator of inflammatory responses. To the best of our knowledge, we are the first to demonstrate that FAAH inhibition regulates IL-1α, IL-9, MIP-1β, and MIP-2 levels.
These effects on cytokine levels are perhaps not only due to alterations in the levels of AEA caused by the FAAH SNP. FAAH hydrolyzes other fatty acid ethanolamides including palmitoylethanolamide (PEA) and oleoylethanolamide (OEA), which have anti-inflammatory effects (Pontis et al., 2016). OEA inhibits MCP-1 levels (Montecucco et al., 2015; Antón et al., 2017; Zhao et al., 2018) and also reduces TNF levels (Sayd et al., 2014; Chen et al., 2015; Xu et al., 2016; Antón et al., 2017). PEA reduces MCP-1 levels (D’Aloia et al., 2021; Fusco et al., 2021) and TNF levels (Costa et al., 2008; De Filippis et al., 2009; Hoareau et al., 2009; Cerrato et al., 2010; Di Paola et al., 2012, 2016; Sayd et al., 2014; Impellizzeri et al., 2015; Rahimi et al., 2015; Orefice et al., 2016; Britti et al., 2017; Gugliandolo et al., 2017; D’amico et al., 2019; Puglia et al., 2020; Ardizzone et al., 2021; D’Aloia et al., 2021). PEA reduces inflammation in human colonic tissue (from patients with IBD, colon cancer and appendicitis), including levels of IL-6, IL-8, IL-17A, MCP-1, and GM-CSF (Couch et al., 2017). There are no reported effects of PEA or OEA on IL-2, LIF, or G-CSF, although this does not rule out any effects in our model in these cytokines being attributed to either PEA or OEA. Given that PEA and OEA are not only metabolized by FAAH, but also have immunomodulatory effects, it is possible that the effects we observed are not limited to AEA and the endocannabinoid system, but are a concerted network effect produced by the elevations of all of these fatty acid amides acting in tandem.
In the human literature, there are very few reports examining the relationship between the FAAH SNP (C385A) and inflammatory diseases. In one study, in MS patients who received IFNγ (resulting in a condition called flu-like syndrome), possessing the A allele of this SNP did not alter any of the syndrome symptoms measured, including general malaise, muscle pain, chills, weakness and the need to take non-steroidal anti-inflammatory drugs (Buttari et al., 2017). Additionally, one report investigated this SNP in relation to IBD (Storr et al., 2009). They find that there are no differences in the frequency, and thus potentially, susceptibility, of this genotype among controls, or patients with ulcerative colitis or Crohn’s disease (for which TNBS administration is a model) (Storr et al., 2009). They do show, however, that in patients with Crohn’s disease, being homozygous for the A allele leads to a more severe phenotype, including fistulas and extra-intestinal manifestations (Storr et al., 2009). In ulcerative colitis patients, being homozygous for the A allele is associated with an earlier disease onset (Storr et al., 2009). Interestingly, patients with type two diabetes carrying an A allele (C385A) have higher TNF levels (de Luis et al., 2010), indicating for some diseases, this allele may not always confer protective effects. Inflammation can increase AEA levels (Liu et al., 2003; Doenni et al., 2016; Grill et al., 2019), and therefore, it is possible that in the context of a reduction in FAAH activity, as seen in this genotype, there is even more AEA. Greater AEA and other mediators may act on the transient receptor potential vanilloid type 1 (TRPV1) or other receptors to drive inflammation or inflammatory damage. While we did not see any exacerbation of the primary disease pathology itself by the FAAH SNP, we did look at an early time point to align with the peak of the acute phase of colitis, so it remains possible that this SNP could have deleterious effects on the disease progression under more long-term or chronic conditions.
In summary, in mice with acute colitis, increased plasma and amygdala cytokine levels are regulated by the activity of FAAH. Reductions in FAAH activity are able to reverse some of the peripheral and central inflammatory changes, particularly in those that drive monocyte and CD4+ T cell activation and migration. Since there were no effects of the genotype on colitis, these effects are likely mediated by the actions of elevated AEA (or other fatty acid amides) signaling to circulating or resident immune cells. Our data will inform future approaches to the personalized treatment of individuals with FAAH SNPs and may contribute to an understanding of disease outcome and progression, potentially providing treatment insights for these individuals.
Data Availability Statement
The raw data supporting the conclusions of this article will be made available by the authors, without undue reservation.
Ethics Statement
The animal study was reviewed and approved by the Health Sciences Animal Care Committee of the University of Calgary and followed guidelines from the Canadian Council on Animal Care.
Author Contributions
HV, CK, QP, KS, and MH designed the experiments. HV, RA, CH, VC, MM, and AN performed the experiments. FL contributed experimental tools. HV analyzed the data and prepared the figures. HV and MH wrote the manuscript with input from all authors. QP, KS, and MH obtained funding for the work and provided oversight and supervision.
Funding
This work was supported by grants from the Canadian Institutes of Health Research (CIHR; FDN333950 to MH; FDN148380 to KS; PJT159454 to QP, MH, KS); Alberta Innovates Health Solutions (AIHS) CRIO Project 201200828 to QP, KS. HV received stipend funding from CIHR (Vanier CGS), the University of Calgary (UofC; Killam Pre-doctoral Laureate), AIHS and Branch Out Neurological Foundation (BONF); RA received stipend funding from the Mathison Centre for Mental Health Research and Education; CH received funding from a UofC Eyes High Scholarship and Hotchkiss Brain Institute Postdoctoral Fellowship; VC received a studentship from UofC; MM received fellowship support from AIHS and CIHR; AN received stipend funding from the UofC Mathison Centre Graduate Recruitment Scholarship in Mental Health and Cumming School of Medicine Graduate Scholarship. MH was the recipient of a Tier II Canada Research Chair. KS was the Crohn’s and Colitis Canada Chair in Inflammatory Bowel Disease Research. Funding agencies had no influence on the design, execution or publishing of this work.
Conflict of Interest
KS has been an advisor to Arena Pharmaceuticals and has received a research grant from Abalone Inc. MH was a scientific advisor for Sophren Therapeutics, Jazz Pharmaceuticals and Lundbeck.
The remaining authors declare that the research was conducted in the absence of any commercial or financial relationships that could be construed as a potential conflict of interest.
Publisher’s Note
All claims expressed in this article are solely those of the authors and do not necessarily represent those of their affiliated organizations, or those of the publisher, the editors and the reviewers. Any product that may be evaluated in this article, or claim that may be made by its manufacturer, is not guaranteed or endorsed by the publisher.
Acknowledgments
We acknowledge that this work was conducted on the traditional territories of the people of the Treaty 7 region in Southern Alberta, which includes the Blackfoot Confederacy (comprising the Siksika, Piikani, and Kainai First Nations), as well as the Tsuut’ina First Nation, and the Stoney Nakoda (including the Chiniki, Bearspaw, and Wesley First Nations). The City of Calgary is also home to Métis Nation of Alberta, Region III. We acknowledge the work of the University of Calgary Health Sciences Animal Research Centre for breeding and care of the mice, particularly Krista Jensen and Brittany Munro. Genotyping was performed by Dr. Frank Visser, Hotchkiss Brain Institute Molecular Core Facility. Aspects of this work appear in the following doctoral thesis by HV: “Investigating the Role of Central Endocannabinoids and Inflammation in Comorbid Anxiety-Like Behavior and Colitis,” the University of Calgary, 2020.
Supplementary Material
The Supplementary Material for this article can be found online at: https://www.frontiersin.org/articles/10.3389/fncel.2021.764706/full#supplementary-material
References
Acharjee, S., Verbeek, M., Gomez, C. D., Bisht, K., Lee, B., Benoit, L., et al. (2018). Reduced microglial activity and enhanced glutamate transmission in the basolateral amygdala in early CNS autoimmunity. J. Neurosci. 38, 9019–9033. doi: 10.1523/JNEUROSCI.0398-18.2018
Agostini, A., Filippini, N., Cevolani, D., Agati, R., Leoni, C., Tambasco, R., et al. (2011). Brain functional changes in patients with ulcerative colitis: a functional magnetic resonance imaging study on emotional processing. Inflamm. Bowel Dis. 17, 1769–1777. doi: 10.1002/ibd.21549
Alhouayek, M., Lambert, D. M., Delzenne, N. M., Cani, P. D., and Muccioli, G. G. (2011). Increasing endogenous 2-arachidonoylglycerol levels counteracts colitis and related systemic inflammation. FASEB J. 25, 2711–2721. doi: 10.1096/fj.10-176602
Antón, M., Alén, F., Gómez de Heras, R., Serrano, A., Pavón, F. J., Leza, J. C., et al. (2017). Oleoylethanolamide prevents neuroimmune HMGB1/TLR4/NF-kB danger signaling in rat frontal cortex and depressive-like behavior induced by ethanol binge administration. Addict. Biol. 22, 724–741. doi: 10.1111/adb.12365
Ardizzone, A., Fusco, R., Casili, G., Lanza, M., Impellizzeri, D., Esposito, E., et al. (2021). Effect of ultra-micronized-palmitoylethanolamide and acetyl-l-carnitine on experimental model of inflammatory pain. Int. J. Mol. Sci. 22:1967. doi: 10.3390/ijms22041967
Bao, C. H., Liu, P., Liu, H. R., Wu, L. Y., Shi, Y., Chen, W. F., et al. (2015). Alterations in brain grey matter structures in patients with crohn’s disease and their correlation with psychological distress. J. Crohns Colitis 9, 532–540. doi: 10.1093/ecco-jcc/jjv057
Bátkai, S., Rajesh, M., Mukhopadhyay, P., Haskó, G., Liaudet, L., Cravatt, B. F., et al. (2007). Decreased age-related cardiac dysfunction, myocardial nitrative stress, inflammatory gene expression, and apoptosis in mice lacking fatty acid amide hydrolase. Am. J. Physiol. Heart Circ. Physiol. 293, H909–H918. doi: 10.1152/ajpheart.00373.2007
Britti, D., Crupi, R., Impellizzeri, D., Gugliandolo, E., Fusco, R., Schievano, C., et al. (2017). A novel composite formulation of palmitoylethanolamide and quercetin decreases inflammation and relieves pain in inflammatory and osteoarthritic pain models. BMC Vet. Res. 13:229. doi: 10.1186/s12917-017-1151-z
Buttari, F., Zagaglia, S., Marciano, L., Albanese, M., Landi, D., Nicoletti, C. G., et al. (2017). TRPV1 polymorphisms and risk of interferon β-induced flu-like syndrome in patients with relapsing-remitting multiple sclerosis. J. Neuroimmunol. 305, 172–174. doi: 10.1016/j.jneuroim.2017.02.007
Cencioni, M. T. Chiurchiú, V., Catanzaro, G., Borsellino, G., Bernardi, G., Battistini, L., et al. (2010). Anandamide suppresses proliferation and cytokine release from primary human T-lymphocytes mainly via CB2 receptors. PLoS One 5. doi: 10.1371/journal.pone.0008688
Cerrato, S., Brazis, P., della Valle, M. F., Miolo, A., and Puigdemont, A. (2010). Effects of palmitoylethanolamide on immunologically induced histamine. PGD2 and TNFalpha release from canine skin mast cells. Vet. Immunol. Immunopathol. 133, 9–15. doi: 10.1016/j.vetimm.2009.06.011
Chang, Y. H., Lee, S. T., and Lin, W. W. (2001). Effects of cannabinoids on LPS-stimulated inflammatory mediator release from macrophages: involvement of eicosanoids. J. Cell. Biochem. 81, 715–723. doi: 10.1002/jcb.1103
Chen, C.-X., Yang, L.-C., Xu, X.-D., Wei, X., Gai, Y.-T., Peng, L., et al. (2015). Effect of propane-2-sulfonic acid octadec-9-enyl-amide on the expression of adhesion molecules in human umbilical vein endothelial cells. Eur. J. Pharmacol. 756, 15–21. doi: 10.1016/j.ejphar.2015.03.014
Chen, J., Song, Y., Yang, J., Zhang, Y., Zhao, P., Zhu, X.-J., et al. (2013). The contribution of TNF-α in the amygdala to anxiety in mice with persistent inflammatory pain. Neurosci. Lett. 541, 275–280. doi: 10.1016/j.neulet.2013.02.005
Chiurchiù, V., Rapino, C., Talamonti, E., Leuti, A., Lanuti, M., Gueniche, A., et al. (2016). Anandamide suppresses proinflammatory T cell responses in vitro through Type-1 cannabinoid receptor-mediated mTOR inhibition in human keratinocytes. J. Immunol. 197, 3545–3553. doi: 10.4049/jimmunol.1500546
Correa, F., Docagne, F., Mestre, L., Clemente, D., Hernangómez, M., Loría, F., et al. (2009). A role for CB2 receptors in anandamide signalling pathways involved in the regulation of IL-12 and IL-23 in microglial cells. Biochem. Pharmacol. 77, 86–100. doi: 10.1016/j.bcp.2008.09.014
Correa, F., Hernangómez, M., Mestre, L., Loría, F., Spagnolo, A., Docagne, F., et al. (2010). Anandamide enhances IL-10 production in activated microglia by targeting CB(2) receptors: roles of ERK1/2, JNK, and NF-kappaB. Glia 58, 135–147. doi: 10.1002/glia.20907
Costa, B., Comelli, F., Bettoni, I., Colleoni, M., and Giagnoni, G. (2008). The endogenous fatty acid amide, palmitoylethanolamide, has anti-allodynic and anti-hyperalgesic effects in a murine model of neuropathic pain: involvement of CB(1), TRPV1 and PPARgamma receptors and neurotrophic factors. Pain 139, 541–550. doi: 10.1016/j.pain.2008.06.003
Couch, D. G., Tasker, C., Theophilidou, E., Lund, J. N., and O’Sullivan, S. E. (2017). Cannabidiol and palmitoylethanolamide are anti-inflammatory in the acutely inflamed human colon. Clin. Sci. 131, 2611–2626. doi: 10.1042/CS20171288
D’Aloia, A., Molteni, L., Gullo, F., Bresciani, E., Artusa, V., Rizzi, L., et al. (2021). Palmitoylethanolamide Modulation of Microglia Activation: characterization of Mechanisms of Action and Implication for Its Neuroprotective Effects. Int. J. Mol. Sci. 22:3054. doi: 10.3390/ijms22063054
D’amico, R., Fusco, R., Gugliandolo, E., Cordaro, M., Siracusa, R., Impellizzeri, D., et al. (2019). Effects of a new compound containing palmitoylethanolamide and baicalein in myocardial ischaemia/reperfusion injury in vivo. Phytomedicine 54, 27–42. doi: 10.1016/j.phymed.2018.09.191
De Filippis, D., D’Amico, A., Cinelli, M. P., Esposito, G., Di Marzo, V., and Iuvone, T. (2009). Adelmidrol, a palmitoylethanolamide analogue, reduces chronic inflammation in a carrageenin-granuloma model in rats. J. Cell. Mol. Med. 13, 1086–1095. doi: 10.1111/j.1582-4934.2008.00353.x
de Luis, D. A., Sagrado, M. G., Aller, R., Izaola, O., Conde, R., and Romero, E. (2010). C358A missense polymorphism of the endocannabinoid degrading enzyme fatty acid amide hydrolase (FAAH) and insulin resistance in patients with diabetes mellitus type 2. Diabetes Res. Clin. Pract. 88, 76–80. doi: 10.1016/j.diabres.2009.12.019
Di Paola, R., Cordaro, M., Crupi, R., Siracusa, R., Campolo, M., Bruschetta, G., et al. (2016). Protective effects of ultramicronized palmitoylethanolamide (PEA-um) in myocardial ischaemia and reperfusion injury in vivo. Shock 46, 202–213. doi: 10.1097/SHK.0000000000000578
Di Paola, R., Impellizzeri, D., Torre, A., Mazzon, E., Cappellani, A., Faggio, C., et al. (2012). Effects of palmitoylethanolamide on intestinal injury and inflammation caused by ischemia-reperfusion in mice. J. Leukoc. Biol. 91, 911–920. doi: 10.1189/jlb.0911485
Dincheva, I., Drysdale, A. T., Hartley, C. A., Johnson, D. C., Jing, D., King, E. C., et al. (2015). FAAH genetic variation enhances fronto-amygdala function in mouse and human. Nat. Commun. 6:6395. doi: 10.1038/ncomms7395
Doenni, V. M., Gray, J. M., Song, C. M., Patel, S., Hill, M. N., and Pittman, Q. J. (2016). Deficient adolescent social behavior following early-life inflammation is ameliorated by augmentation of anandamide signaling. Brain Behav. Immun. 58, 237–247. doi: 10.1016/j.bbi.2016.07.152
Elson, C. O., Beagley, K. W., Sharmanov, A. T., Fujihashi, K., Kiyono, H., Tennyson, G. S., et al. (1996). Hapten-induced model of murine inflammatory bowel disease: mucosa immune responses and protection by tolerance. J Immunol 157, 2174–2185.
Engel, M. A., Kellermann, C. A., Burnat, G., Hahn, E. G., Rau, T., and Konturek, P. C. (2010). Mice lacking cannabinoid CB1-CB2-receptors or both receptors show increased susceptibility to trinitrobenzene sulfonic acid (TNBS)-induced colitis. J. Physiol. Pharmacol. 61, 89–97.
Engel, M. A., Kellermann, C. A., Rau, T., Burnat, G., Hahn, E. G., and Konturek, P. C. (2008). Ulcerative colitis in AKR mice is attenuated by intraperitoneally administered anandamide. J. Physiol. Pharmacol. 59, 673–689.
Facchinetti, F., Del Giudice, E., Furegato, S., Passarotto, M., and Leon, A. (2003). Cannabinoids ablate release of TNFalpha in rat microglial cells stimulated with lypopolysaccharide. Glia 41, 161–168. doi: 10.1002/glia.10177
Fan, Y., Bao, C., Wei, Y., Wu, J., Zhao, Y., Zeng, X., et al. (2019). Altered functional connectivity of the amygdala in Crohn’s disease. Brain Imaging Behav. 14, 2097–2106. doi: 10.1007/s11682-019-00159-8
Fichna, J., Bawa, M., Thakur, G. A., Tichkule, R., Makriyannis, A., McCafferty, D.-M., et al. (2014). Cannabinoids alleviate experimentally induced intestinal inflammation by acting at central and peripheral receptors. PLoS One 9:e109115. doi: 10.1371/journal.pone.0109115
Fukudo, S., and Kanazawa, M. (2011). Gene, environment, and brain-gut interactions in irritable bowel syndrome. J. Gastroenterol. Hepatol. 26(Suppl. 3) 110–115. doi: 10.1111/j.1440-1746.2011.06631.x
Fusco, R., Siracusa, R., Gugliandolo, E., Peritore, A. F., D’Amico, R., Cordaro, M., et al. (2021). Micro Composite Palmitoylethanolamide/Rutin Reduces Vascular Injury through Modulation of the Nrf2/HO-1 and NF-kB Pathways. Curr. Med. Chem. 28, 6287–6302. doi: 10.2174/0929867328666210329120213
Gärtner, A., Dörfel, D., Diers, K., Witt, S. H., Strobel, A., and Brocke, B. (2019). Impact of FAAH genetic variation on fronto-amygdala function during emotional processing. Eur. Arch. Psychiatry Clin. Neurosci. 269, 209–221. doi: 10.1007/s00406-018-0944-9
Gray, J. M., Vecchiarelli, H. A., Morena, M., Lee, T. T. Y., Hermanson, D. J., Kim, A. B., et al. (2015). Corticotropin-releasing hormone drives anandamide hydrolysis in the amygdala to promote anxiety. J. Neurosci. 35, 3879–3892. doi: 10.1523/JNEUROSCI.2737-14.2015
Grill, M., Högenauer, C., Blesl, A., Haybaeck, J., Golob-Schwarzl, N., Ferreirós, N., et al. (2019). Members of the endocannabinoid system are distinctly regulated in inflammatory bowel disease and colorectal cancer. Sci. Rep. 9:2358. doi: 10.1038/s41598-019-38865-4
Gugliandolo, E., Fusco, R., Biundo, F., D’Amico, R., Benedetto, F., Di Paola, R., et al. (2017). Palmitoylethanolamide and Polydatin combination reduces inflammation and oxidative stress in vascular injury. Pharmacol. Res. 123, 83–92. doi: 10.1016/j.phrs.2017.06.014
Han, J. S., and Neugebauer, V. (2004). Synaptic plasticity in the amygdala in a visceral pain model in rats. Neurosci. Lett. 361, 254–257. doi: 10.1016/j.neulet.2003.12.027
Hariri, A. R., Gorka, A., Hyde, L. W., Kimak, M., Halder, I., Ducci, F., et al. (2009). Divergent effects of genetic variation in endocannabinoid signaling on human threat- and reward-related brain function. Biol. Psychiatry 66, 9–16. doi: 10.1016/j.biopsych.2008.10.047
Hayakawa, K., Mishima, K., Irie, K., Hazekawa, M., Mishima, S., Fujioka, M., et al. (2008). Cannabidiol prevents a post-ischemic injury progressively induced by cerebral ischemia via a high-mobility group box1-inhibiting mechanism. Neuropharmacology 55, 1280–1286. doi: 10.1016/j.neuropharm.2008.06.040
Hermes, D. J., Yadav-Samudrala, B. J., Xu, C., Paniccia, J. E., Meeker, R. B., Armstrong, M. L., et al. (2021). GPR18 drives FAAH inhibition-induced neuroprotection against HIV-1 Tat-induced neurodegeneration. Exp. Neurol. 341:113699. doi: 10.1016/j.expneurol.2021.113699
Hoareau, L., Buyse, M., Festy, F., Ravanan, P., Gonthier, M.-P., Matias, I., et al. (2009). Anti-inflammatory effect of palmitoylethanolamide on human adipocytes. Obesity (Silver Spring) 17, 431–438. doi: 10.1038/oby.2008.591
Holt, S., Comelli, F., Costa, B., and Fowler, C. J. (2005). Inhibitors of fatty acid amide hydrolase reduce carrageenan-induced hind paw inflammation in pentobarbital-treated mice: comparison with indomethacin and possible involvement of cannabinoid receptors. Br. J. Pharmacol. 146, 467–476. doi: 10.1038/sj.bjp.0706348
Hubbard, C. S., Labus, J. S., Bueller, J., Stains, J., Suyenobu, B., Dukes, G. E., et al. (2011). Corticotropin-releasing factor receptor 1 antagonist alters regional activation and effective connectivity in an emotional-arousal circuit during expectation of abdominal pain. J. Neurosci. 31, 12491–12500. doi: 10.1523/JNEUROSCI.1860-11.2011
Icenhour, A., Langhorst, J., Benson, S., Schlamann, M., Hampel, S., Engler, H., et al. (2015). Neural circuitry of abdominal pain-related fear learning and reinstatement in irritable bowel syndrome. Neurogastroenterol. Motil. 27, 114–127. doi: 10.1111/nmo.12489
Impellizzeri, D., Ahmad, A., Bruschetta, G., Di Paola, R., Crupi, R., Paterniti, I., et al. (2015). The anti-inflammatory effects of palmitoylethanolamide (PEA) on endotoxin-induced uveitis in rats. Eur. J. Pharmacol. 761, 28–35. doi: 10.1016/j.ejphar.2015.04.025
Jain, P., Hassan, A. M., Koyani, C. N., Mayerhofer, R., Reichmann, F., Farzi, A., et al. (2015). Behavioral and molecular processing of visceral pain in the brain of mice: impact of colitis and psychological stress. Front. Behav. Neurosci. 9:177. doi: 10.3389/fnbeh.2015.00177
Kaplan, B. L. F., Ouyang, Y., Herring, A., Yea, S. S., Razdan, R., and Kaminski, N. E. (2005). Inhibition of leukocyte function and interleukin-2 gene expression by 2-methylarachidonyl-(2′-fluoroethyl)amide, a stable congener of the endogenous cannabinoid receptor ligand anandamide. Toxicol. Appl. Pharmacol. 205, 107–115. doi: 10.1016/j.taap.2004.09.013
Katona, I., and Freund, T. F. (2012). Multiple functions of endocannabinoid signaling in the brain. Annu. Rev. Neurosci. 35, 529–558. doi: 10.1146/annurev-neuro-062111-150420
Kerr, D. M., Burke, N. N., Ford, G. K., Connor, T. J., Harhen, B., Egan, L. J., et al. (2012). Pharmacological inhibition of endocannabinoid degradation modulates the expression of inflammatory mediators in the hypothalamus following an immunological stressor. Neuroscience 204, 53–63. doi: 10.1016/j.neuroscience.2011.09.032
Kinsey, S. G., Naidu, P. S., Cravatt, B. F., Dudley, D. T., and Lichtman, A. H. (2011). Fatty acid amide hydrolase blockade attenuates the development of collagen-induced arthritis and related thermal hyperalgesia in mice. Pharmacol. Biochem. Behav. 99, 718–725. doi: 10.1016/j.pbb.2011.06.022
Labus, J. S., Hubbard, C. S., Bueller, J., Ebrat, B., Tillisch, K., Chen, M., et al. (2013). Impaired emotional learning and involvement of the corticotropin-releasing factor signaling system in patients with irritable bowel syndrome. Gastroenterology 145, 1253–1261. doi: 10.1053/j.gastro.2013.08.016
Labus, J. S., Naliboff, B. D., Berman, S. M., Suyenobu, B., Vianna, E. P., Tillisch, K., et al. (2009). Brain networks underlying perceptual habituation to repeated aversive visceral stimuli in patients with irritable bowel syndrome. Neuroimage 47, 952–960. doi: 10.1016/j.neuroimage.2009.05.078
Lazary, J., Eszlari, N., Juhasz, G., and Bagdy, G. (2019). A functional variant of CB2 receptor gene interacts with childhood trauma and FAAH gene on anxious and depressive phenotypes. J. Affect. Disord. 257, 716–722. doi: 10.1016/j.jad.2019.07.083
Li, Y., Missig, G., Finger, B. C., Landino, S. M., Alexander, A. J., Mokler, E. L., et al. (2018). Maternal and early postnatal immune activation produce dissociable effects on neurotransmission in mPFC-amygdala circuits. J. Neurosci. 38, 3358–3372. doi: 10.1523/JNEUROSCI.3642-17.2018
Liu, J., Batkai, S., Pacher, P., Harvey-White, J., Wagner, J. A., Cravatt, B. F., et al. (2003). Lipopolysaccharide induces anandamide synthesis in macrophages via CD14/MAPK/phosphoinositide 3-kinase/NF-kappaB independently of platelet-activating factor. J. Biol. Chem. 278, 45034–45039. doi: 10.1074/jbc.M306062200
Maccarrone, M., and Wenger, T. (2005). Effects of cannabinoids on hypothalamic and reproductive function. Handb. Exp. Pharmacol. 168, 555–571. doi: 10.1007/3-540-26573-2_18
Maccarrone, M., Valensise, H., Bari, M., Lazzarin, N., Romanini, C., and Finazzi-Agrò, A. (2001). Progesterone up-regulates anandamide hydrolase in human lymphocytes: role of cytokines and implications for fertility. J. Immunol. 166, 7183–7189. doi: 10.4049/jimmunol.166.12.7183
Marchalant, Y., Cerbai, F., Brothers, H. M., and Wenk, G. L. (2008). Cannabinoid receptor stimulation is anti-inflammatory and improves memory in old rats. Neurobiol. Aging 29, 1894–1901. doi: 10.1016/j.neurobiolaging.2007.04.028
Marques, C. P., Cheeran, M. C.-J., Palmquist, J. M., Hu, S., Urban, S. L., and Lokensgard, J. R. (2008). Prolonged microglial cell activation and lymphocyte infiltration following experimental herpes encephalitis. J. Immunol. 181, 6417–6426. doi: 10.4049/jimmunol.181.9.6417
Marsicano, G., Goodenough, S., Monory, K., Hermann, H., Eder, M., Cannich, A., et al. (2003). CB1 cannabinoid receptors and on-demand defense against excitotoxicity. Science 302, 84–88. doi: 10.1126/science.1088208
Massa, F., Marsicano, G., Hermann, H., Cannich, A., Monory, K., Cravatt, B. F., et al. (2004). The endogenous cannabinoid system protects against colonic inflammation. J. Clin. Invest. 113, 1202–1209. doi: 10.1172/JCI19465
Mayo, L. M., Asratian, A., Lindé, J., Holm, L., Nätt, D., Augier, G., et al. (2020). Protective effects of elevated anandamide on stress and fear-related behaviors: translational evidence from humans and mice. Mol. Psychiatry 25, 993–1005. doi: 10.1038/s41380-018-0215-1
Montecucco, F., Lenglet, S., Quercioli, A., Burger, F., Thomas, A., Lauer, E., et al. (2015). Gastric bypass in morbid obese patients is associated with reduction in adipose tissue inflammation via N-oleoylethanolamide (OEA)-mediated pathways. Thromb. Haemost. 113, 838–850. doi: 10.1160/TH14-06-0506
Motulsky, H. J., and Brown, R. E. (2006). Detecting outliers when fitting data with nonlinear regression - a new method based on robust nonlinear regression and the false discovery rate. BMC Bioinformatics 7:123. doi: 10.1186/1471-2105-7-123
Munro, S., Thomas, K. L., and Abu-Shaar, M. (1993). Molecular characterization of a peripheral receptor for cannabinoids. Nature 365, 61–65. doi: 10.1038/365061a0
Munshi, S., and Rosenkranz, J. A. (2018). Effects of peripheral immune challenge on in vivo firing of basolateral amygdala neurons in adult male rats. Neuroscience 390, 174–186. doi: 10.1016/j.neuroscience.2018.08.017
Nader, J., Rapino, C., Gennequin, B., Chavant, F., Francheteau, M., Makriyannis, A., et al. (2014). Prior stimulation of the endocannabinoid system prevents methamphetamine-induced dopaminergic neurotoxicity in the striatum through activation of CB2 receptors. Neuropharmacology 87, 214–221. doi: 10.1016/j.neuropharm.2014.03.014
Nagarkatti, P., Pandey, R., Rieder, S. A., Hegde, V. L., and Nagarkatti, M. (2009). Cannabinoids as novel anti-inflammatory drugs. Future Med. Chem. 1, 1333–1349. doi: 10.4155/fmc.09.93
Naidu, P. S., Varvel, S. A., Ahn, K., Cravatt, B. F., Martin, B. R., and Lichtman, A. H. (2007). Evaluation of fatty acid amide hydrolase inhibition in murine models of emotionality. Psychopharmacology 192, 61–70. doi: 10.1007/s00213-006-0689-4
Nakajima, Y., Furuichi, Y., Biswas, K. K., Hashiguchi, T., Kawahara, K., Yamaji, K., et al. (2006). Endocannabinoid, anandamide in gingival tissue regulates the periodontal inflammation through NF-kappaB pathway inhibition. FEBS Lett. 580, 613–619. doi: 10.1016/j.febslet.2005.12.079
Naliboff, B. D., and Mayer, E. A. (2006). Brain imaging in IBS: drawing the line between cognitive and non-cognitive processes. Gastroenterology 130, 267–270. doi: 10.1053/j.gastro.2005.11.034
Orefice, N. S., Alhouayek, M., Carotenuto, A., Montella, S., Barbato, F., Comelli, A., et al. (2016). Oral palmitoylethanolamide treatment is associated with reduced cutaneous adverse effects of interferon-β1a and circulating proinflammatory cytokines in relapsing-remitting multiple sclerosis. Neurotherapeutics 13, 428–438. doi: 10.1007/s13311-016-0420-z
Ortega-Gutiérrez, S., Molina-Holgado, E., and Guaza, C. (2005). Effect of anandamide uptake inhibition in the production of nitric oxide and in the release of cytokines in astrocyte cultures. Glia 52, 163–168. doi: 10.1002/glia.20229
Osafo, N., Yeboah, O. K., and Antwi, A. O. (2021). Endocannabinoid system and its modulation of brain, gut, joint and skin inflammation. Mol. Biol. Rep. 48, 3665–3680. doi: 10.1007/s11033-021-06366-1
Özdemir, B., Shi, B., Bantleon, H. P., Moritz, A., Rausch-Fan, X., and Andrukhov, O. (2014). Endocannabinoids and inflammatory response in periodontal ligament cells. PLoS One 9:e107407. doi: 10.1371/journal.pone.0107407
Panikashvili, D., Shein, N. A., Mechoulam, R., Trembovler, V., Kohen, R., Alexandrovich, A., et al. (2006). The endocannabinoid 2-AG protects the blood-brain barrier after closed head injury and inhibits mRNA expression of proinflammatory cytokines. Neurobiol. Dis. 22, 257–264. doi: 10.1016/j.nbd.2005.11.004
Parolaro, D. (1999). Presence and functional regulation of cannabinoid receptors in immune cells. Life Sci. 65, 637–644.
Patsenker, E., Sachse, P., Chicca, A., Gachet, M. S., Schneider, V., Mattsson, J., et al. (2015). Elevated levels of endocannabinoids in chronic hepatitis C may modulate cellular immune response and hepatic stellate cell activation. Int. J. Mol. Sci. 16, 7057–7076. doi: 10.3390/ijms16047057
Petrosino, S., Verde, R., Vaia, M., Allarà, M., Iuvone, T., and Di Marzo, V. (2018). Anti-inflammatory properties of cannabidiol, a nonpsychotropic cannabinoid, in experimental allergic contact dermatitis. J. Pharmacol. Exp. Ther. 365, 652–663. doi: 10.1124/jpet.117.244368
Picardo, S., Kaplan, G. G., Sharkey, K. A., and Seow, C. H. (2019). Insights into the role of cannabis in the management of inflammatory bowel disease. Therap. Adv. Gastroenterol. 12:1756284819870977. doi: 10.1177/1756284819870977
Pontis, S., Ribeiro, A., Sasso, O., and Piomelli, D. (2016). Macrophage-derived lipid agonists of PPAR-α as intrinsic controllers of inflammation. Crit. Rev. Biochem. Mol. Biol. 51, 7–14. doi: 10.3109/10409238.2015.1092944
Porcher, C., Sinniger, V., Juhem, A., Mouchet, P., and Bonaz, B. (2004). Neuronal activity and CRF receptor gene transcription in the brains of rats with colitis. Am. J. Physiol. Gastrointest. Liver Physiol. 287, G803–G814. doi: 10.1152/ajpgi.00135.2004
Pryce, G., and Baker, D. (2012). Potential control of multiple sclerosis by cannabis and the endocannabinoid system. CNS Neurol. Disord. Drug Targets 11, 624–641. doi: 10.2174/187152712801661310
Puffenbarger, R. A., Boothe, A. C., and Cabral, G. A. (2000). Cannabinoids inhibit LPS-inducible cytokine mRNA expression in rat microglial cells. Glia 29, 58–69.
Puglia, C., Santonocito, D., Ostacolo, C., Maria Sommella, E., Campiglia, P., Carbone, C., et al. (2020). Ocular formulation based on palmitoylethanolamide-loaded nanostructured lipid carriers: technological and pharmacological profile. Nanomaterials (Basel) 10:287. doi: 10.3390/nano10020287
Rahimi, A., Faizi, M., Talebi, F., Noorbakhsh, F., Kahrizi, F., and Naderi, N. (2015). Interaction between the protective effects of cannabidiol and palmitoylethanolamide in experimental model of multiple sclerosis in C57BL/6 mice. Neuroscience 290, 279–287. doi: 10.1016/j.neuroscience.2015.01.030
Ren, M., Guo, Q., Guo, L., Lenz, M., Qian, F., Koenen, R. R., et al. (2010). Polymerization of MIP-1 chemokine (CCL3 and CCL4) and clearance of MIP-1 by insulin-degrading enzyme. EMBO J. 29, 3952–3966. doi: 10.1038/emboj.2010.256
Rettori, E., De Laurentiis, A., Zorrilla Zubilete, M., Rettori, V., and Elverdin, J. C. (2012). Anti-inflammatory effect of the endocannabinoid anandamide in experimental periodontitis and stress in the rat. Neuroimmunomodulation 19, 293–303. doi: 10.1159/000339113
Riazi, K., Galic, M. A., Kuzmiski, J. B., Ho, W., Sharkey, K. A., and Pittman, Q. J. (2008). Microglial activation and TNFalpha production mediate altered CNS excitability following peripheral inflammation. Proc. Natl. Acad. Sci. U.S.A. 105, 17151–17156. doi: 10.1073/pnas.0806682105
Rivera, P., Fernández-Arjona, M. D. M., Silva-Peña, D., Blanco, E., Vargas, A., López-Ávalos, M. D., et al. (2018). Pharmacological blockade of fatty acid amide hydrolase (FAAH) by URB597 improves memory and changes the phenotype of hippocampal microglia despite ethanol exposure. Biochem. Pharmacol. 157, 244–257. doi: 10.1016/j.bcp.2018.08.005
Roche, M., Kelly, J. P., O’Driscoll, M., and Finn, D. P. (2008). Augmentation of endogenous cannabinoid tone modulates lipopolysaccharide-induced alterations in circulating cytokine levels in rats. Immunology 125, 263–271. doi: 10.1111/j.1365-2567.2008.02838.x
Rockwell, C. E., and Kaminski, N. E. (2004). A cyclooxygenase metabolite of anandamide causes inhibition of interleukin-2 secretion in murine splenocytes. J. Pharmacol. Exp. Ther. 311, 683–690. doi: 10.1124/jpet.104.065524
Rockwell, C. E., Raman, P., Kaplan, B. L. F., and Kaminski, N. E. (2008). A COX-2 metabolite of the endogenous cannabinoid, 2-arachidonyl glycerol, mediates suppression of IL-2 secretion in activated Jurkat T cells. Biochem. Pharmacol. 76, 353–361. doi: 10.1016/j.bcp.2008.05.005
Rojas-Zuleta, W. G., and Sanchez, E. (2017). “IL-9: function, sources, and detection,” in Th9 Cells: Methods and Protocols Methods in Molecular Biology, ed. R. Goswami (New York, NY: Springer), 21–35. doi: 10.1007/978-1-4939-6877-0_2
Rossi, S., Bernardi, G., and Centonze, D. (2010). The endocannabinoid system in the inflammatory and neurodegenerative processes of multiple sclerosis and of amyotrophic lateral sclerosis. Exp. Neurol. 224, 92–102. doi: 10.1016/j.expneurol.2010.03.030
Sałaga, M., Mokrowiecka, A., Zakrzewski, P. K., Cygankiewicz, A., Leishman, E., Sobczak, M., et al. (2014). Experimental colitis in mice is attenuated by changes in the levels of endocannabinoid metabolites induced by selective inhibition of fatty acid amide hydrolase (FAAH). J. Crohns Colitis 8, 998–1009. doi: 10.1016/j.crohns.2014.01.025
Sánchez, A. J., and García-Merino, A. (2012). Neuroprotective agents: cannabinoids. Clin. Immunol. 142, 57–67. doi: 10.1016/j.clim.2011.02.010
Sasso, O., Migliore, M., Habrant, D., Armirotti, A., Albani, C., Summa, M., et al. (2015). Multitarget fatty acid amide hydrolase/cyclooxygenase blockade suppresses intestinal inflammation and protects against nonsteroidal anti-inflammatory drug-dependent gastrointestinal damage. FASEB J. 29, 2616–2627. doi: 10.1096/fj.15-270637
Sayd, A., Antón, M., Alén, F., Caso, J. R., Pavón, J., Leza, J. C., et al. (2014). Systemic administration of oleoylethanolamide protects from neuroinflammation and anhedonia induced by LPS in rats. Int. J. Neuropsychopharmacol. 18:pyu111. doi: 10.1093/ijnp/pyu111
Selvaraj, P., Wen, J., Tanaka, M., and Zhang, Y. (2019). Therapeutic effect of a novel fatty acid amide hydrolase inhibitor pf04457845 in the repetitive closed head injury mouse model. J. Neurotrauma 36, 1655–1669. doi: 10.1089/neu.2018.6226
Shamran, H., Singh, N. P., Zumbrun, E. E., Murphy, A., Taub, D. D., Mishra, M. K., et al. (2017). Fatty acid amide hydrolase (FAAH) blockade ameliorates experimental colitis by altering microRNA expression and suppressing inflammation. Brain Behav. Immun. 59, 10–20. doi: 10.1016/j.bbi.2016.06.008
Shang, Y., Hao, Q., Jiang, K., He, M., and Wang, J. (2020). Discovery of heterocyclic carbohydrazide derivatives as novel selective fatty acid amide hydrolase inhibitors: design, synthesis and anti-neuroinflammatory evaluation. Bioorg. Med. Chem. Lett. 30:127118. doi: 10.1016/j.bmcl.2020.127118
Sigalet, D. L., Wallace, L., De Heuval, E., and Sharkey, K. A. (2010). The effects of glucagon-like peptide 2 on enteric neurons in intestinal inflammation. Neurogastroenterol. Motil. 22:1318-e350. doi: 10.1111/j.1365-2982.2010.01585.x
Storr, M. A., Keenan, C. M., Emmerdinger, D., Zhang, H., Yüce, B., Sibaev, A., et al. (2008a). Targeting endocannabinoid degradation protects against experimental colitis in mice: involvement of CB1 and CB2 receptors. J. Mol. Med. (Berl) 86, 925–936. doi: 10.1007/s00109-008-0359-6
Storr, M. A., Yüce, B., Andrews, C. N., and Sharkey, K. A. (2008b). The role of the endocannabinoid system in the pathophysiology and treatment of irritable bowel syndrome. Neurogastroenterol. Motil. 20, 857–868. doi: 10.1111/j.1365-2982.2008.01175.x
Storr, M., Emmerdinger, D., Diegelmann, J., Pfennig, S., Ochsenkühn, T., Göke, B., et al. (2010). The cannabinoid 1 receptor (CNR1) 1359 G/A polymorphism modulates susceptibility to ulcerative colitis and the phenotype in Crohn’s disease. PLoS One 5:e9453. doi: 10.1371/journal.pone.0009453
Storr, M., Emmerdinger, D., Diegelmann, J., Yüce, B., Pfennig, S., Ochsenkühn, T., et al. (2009). The role of fatty acid hydrolase gene variants in inflammatory bowel disease. Aliment. Pharmacol. Ther. 29, 542–551. doi: 10.1111/j.1365-2036.2008.03910.x
Tchantchou, F., Tucker, L. B., Fu, A. H., Bluett, R. J., McCabe, J. T., Patel, S., et al. (2014). The fatty acid amide hydrolase inhibitor PF-3845 promotes neuronal survival, attenuates inflammation and improves functional recovery in mice with traumatic brain injury. Neuropharmacology 85, 427–439. doi: 10.1016/j.neuropharm.2014.06.006
Tham, C.-S., Whitaker, J., Luo, L., and Webb, M. (2007). Inhibition of microglial fatty acid amide hydrolase modulates LPS stimulated release of inflammatory mediators. FEBS Lett. 581, 2899–2904. doi: 10.1016/j.febslet.2007.05.037
Tillisch, K., Mayer, E. A., and Labus, J. S. (2011). Quantitative meta-analysis identifies brain regions activated during rectal distension in irritable bowel syndrome. Gastroenterology 140, 91–100. doi: 10.1053/j.gastro.2010.07.053
Vázquez, C., Tolón, R. M., Grande, M. T., Caraza, M., Moreno, M., Koester, E. C., et al. (2015). Endocannabinoid regulation of amyloid-induced neuroinflammation. Neurobiol. Aging 36, 3008–3019. doi: 10.1016/j.neurobiolaging.2015.08.003
Vecchiarelli, H. A., Gandhi, C. P., Gray, J. M., Morena, M., Hassan, K. I., and Hill, M. N. (2016). Divergent responses of inflammatory mediators within the amygdala and medial prefrontal cortex to acute psychological stress. Brain Behav. Immun. 51, 70–91. doi: 10.1016/j.bbi.2015.07.026
Vecchiarelli, H. A., Morena, M., Keenan, C. M., Chiang, V., Tan, K., Qiao, M., et al. (2021). Comorbid anxiety-like behavior in a rat model of colitis is mediated by an upregulation of corticolimbic fatty acid amide hydrolase. Neuropsychopharmacology 46, 992–1003. doi: 10.1038/s41386-020-00939-7
Walter, L., and Stella, N. (2004). Cannabinoids and neuroinflammation. Br. J. Pharmacol. 141, 775–785. doi: 10.1038/sj.bjp.0705667
Weaver, K. R., Sherwin, L. B., Walitt, B., Melkus, G. D., and Henderson, W. A. (2016). Neuroimaging the brain-gut axis in patients with irritable bowel syndrome. World J. Gastrointest. Pharmacol. Ther. 7, 320–333. doi: 10.4292/wjgpt.v7.i2.320
Welch, M. G., Welch-Horan, T. B., Anwar, M., Anwar, N., Ludwig, R. J., and Ruggiero, D. A. (2005). Brain effects of chronic IBD in areas abnormal in autism and treatment by single neuropeptides secretin and oxytocin. J. Mol. Neurosci. 25, 259–274. doi: 10.1385/JMN:25:3:259
Xu, X., Guo, H., Jing, Z., Yang, L., Chen, C., Peng, L., et al. (2016). N-Oleoylethanolamine reduces inflammatory cytokines and adhesion molecules in TNF-α-induced human umbilical vein endothelial cells by activating CB2 and PPAR-α. J. Cardiovasc. Pharmacol. 68, 280–291. doi: 10.1097/FJC.0000000000000413
Zajkowska, Z., Borsini, A., Nikkheslat, N., Russell, A., Romano, G. F., Tomassi, S., et al. (2020). Differential effect of interferon-alpha treatment on AEA and 2-AG levels. Brain Behav. Immun. 90, 248–258. doi: 10.1016/j.bbi.2020.08.024
Zhang, F., Özdemir, B., Nguyen, P. Q., Andrukhov, O., and Rausch-Fan, X. (2020). Methanandamide diminish the Porphyromonas gingivalis lipopolysaccharide induced response in human periodontal ligament cells. BMC Oral Health 20:107. doi: 10.1186/s12903-020-01087-6
Zhao, R., Zhou, H., Huang, L., Xie, Z., Wang, J., Gan, W.-B., et al. (2018). Neuropathic pain causes pyramidal neuronal hyperactivity in the anterior cingulate cortex. Front. Cell Neurosci. 12:107. doi: 10.3389/fncel.2018.00107
Keywords: endocannabinoids, colitis, cytokines, amygdala, inflammation
Citation: Vecchiarelli HA, Aukema RJ, Hume C, Chiang V, Morena M, Keenan CM, Nastase AS, Lee FS, Pittman QJ, Sharkey KA and Hill MN (2021) Genetic Variants of Fatty Acid Amide Hydrolase Modulate Acute Inflammatory Responses to Colitis in Adult Male Mice. Front. Cell. Neurosci. 15:764706. doi: 10.3389/fncel.2021.764706
Received: 25 August 2021; Accepted: 25 October 2021;
Published: 22 November 2021.
Edited by:
Antonio J. Herrera, Universidad de Sevilla, SpainReviewed by:
Matthew K. Ross, Mississippi State University, United StatesZunyi Wang, University of Wisconsin-Madison, United States
Copyright © 2021 Vecchiarelli, Aukema, Hume, Chiang, Morena, Keenan, Nastase, Lee, Pittman, Sharkey and Hill. This is an open-access article distributed under the terms of the Creative Commons Attribution License (CC BY). The use, distribution or reproduction in other forums is permitted, provided the original author(s) and the copyright owner(s) are credited and that the original publication in this journal is cited, in accordance with accepted academic practice. No use, distribution or reproduction is permitted which does not comply with these terms.
*Correspondence: Haley A. Vecchiarelli, aGFsZXl2ZWNjaGlhcmVsbGlAdXZpYy5jYQ==; Matthew N. Hill, bW5oaWxsQHVjYWxnYXJ5LmNh
†These authors share senior authorship