- 1Division of Otolaryngology—Head and Neck Surgery, Department of Surgery, Faculty of Medicine, The University of British Columbia, Vancouver, BC, Canada
- 2Faculty of Medicine, University of Aberdeen, Aberdeen, United Kingdom
- 3Division of Otolaryngology—Head and Neck Surgery, Gordon and Leslie Diamond Health Care Centre, Vancouver General Hospital, Vancouver, BC, Canada
Introduction: Sensorineural hearing loss (SNHL) is a prevalent sensory deficit presenting commonly as age-related hearing loss. Other forms of SNHL include noise-induced and sudden SNHL. Recent evidence has pointed to oxidative stress as a common pathogenic pathway in most subtypes of acquired SNHL. MicroRNAs (miRNAs) are small non-coding RNA sequences that suppress target mRNA expression and affect downstream processes. Many studies have shown that miRNAs are integral biomolecules in hypoxia-adaptive responses. They also promote apoptosis in response to oxidative stress resulting in SNHL. Our hypothesis is that miRNAs are involved in the pathophysiological responses to hypoxia and oxidative stress that result in SNHL. This study reviews the evidence for hypoxia-adaptive miRNAs (hypoxamiRs) in different types of acquired SNHL and focuses on miRNAs involved in hypoxia driven SNHL.
Methods: Electronic bibliographic databases PubMed, Ovid MEDLINE, Ovid EMBASE, and Web of Science Core Collection were searched independently by two investigators for articles published in English from the inception of individual databases to the end of July 2020. The text word or medical subject heading searches of all fields, titles, abstracts, or subject headings depending on the database were undertaken with combinations of the words “microRNAs”, “hypoxia”, “hypoxamiRs”, “oxidative stress”, “ischemia” and “hearing loss”. The reference lists of studies meeting the inclusion criteria were searched to identify additional relevant studies. The inclusion criteria included relevant clinical studies with human subjects, animals, and in vitro experiments. The risk of bias was assessed using the Cochrane risk of bias assessment tool for human studies and the Systematic Review Center for Laboratory animal Experimentation (SYRCLE) a risk of bias assessment tool for animal model and in vitro studies.
Results: A total of 15 primary articles were selected for full text screening after excluding duplicates, reviews, retracted articles, and articles not published in English. All nine articles meeting the study inclusion criteria were from animal or in vitro model studies and were assessed to be at low risk of bias. miRNAs miR-34a and miR-29b were reported to be involved in SNHL in inner ear cell models exposed to oxidative stress. Signaling pathways Sirtuin 1/peroxisome proliferator-activated receptor gamma coactivator-1-alpha (SIRT1/PGC-1α), SIRT1/p53, and SIRT1/hypoxia-inducible factor 1-alpha (HIF-1α) were identified as underlying pathways involved in acquired SNHL.
Conclusion: There is evidence that miR-34a and -29b are involved in hypoxia-driven and other causes of oxidative stress-related acquired SNHL. Further studies are required to determine if these findings are clinically applicable.
Introduction
Background
Hearing loss has been ranked as the fifth leading cause of years lived with disability, higher than other chronic diseases such as diabetes, dementia, and chronic obstructive pulmonary disease (Global Burden of Disease Study 2013 Collaborators, 2015). At the societal level, hearing loss has a significant economic impact and is estimated to cost over 750 billion USD globally (World Health Organization, 2017). Sensorineural hearing loss (SNHL) an increasingly prevalent sensory deficit estimated to affect 1.57 billion people, presents most commonly as age-related hearing loss (ARHL) which affects up to 62.1% of individuals 50 years and older worldwide (GBD 2019 Hearing Loss Collaborators, 2021).
The clinical presentation of SNHL can differ depending on the specific form of SNHL. However, hearing loss and tinnitus are ubiquitous symptoms, and in conditions which also affect the vestibular apparatus, vertigo can occur. ARHL is believed to be due to the accumulation of reactive oxygen species (ROS) within hair cells that eventually leads to cell death as part of normal aging (Riva et al., 2007). Aside from ARHL, other forms of acquired SNHL include sudden SNHL, and noise-induced SNHL. The mechanisms underlying sudden SNHL are not fully understood, however, the most accepted mechanism is vascular compromise which suggests that a sudden disruption of the circulation that supplies the cochlea leads to ischemia and associated hypoxic-cell death (Kim et al., 2016). Noise-induced SNHL usually occurs because of irreversible cumulative acoustic trauma to cochlear hair cells, leading to cell death.
Acquired SNHL and hypoxia/oxidative stress-related miRNAs
The ear is comprised of an outer, middle, and inner component. The inner ear houses the main sensory organ responsible for the special sense of hearing. This sensory organ is called the organ of Corti and it is located within the cochlea. Damage to the sensory cells of the organ of Corti or of the vestibulocochlear nerve (cranial nerve 8) that carries signals to the brain, results in SNHL. The damage could be induced by direct trauma, vascular compromise leading to cellular hypoxia, or secondary to the ototoxic effects of various drugs. The sensitivity of the cochlear vasculature to circulating inflammatory factors places the ear at considerable risk of damage (Trune and Nguyen-Huynh, 2012). Tissue hypoxia secondary to ischemia results in oxidative stress directly through reduced aerobic metabolic mitochondrial electron transport chain activity partly due to inhibition of cytochrome oxidase (Raedschelders et al., 2012) and through mitochondrial dysfunction secondary to increased intra-mitochondrial calcium accumulation (Starkov et al., 2004). More oxygen electron transfer under these conditions occurs by multiple single electron transfer steps resulting in an increase in free oxygen radicals. Furthermore, reperfusion following ischemia leads to reactive oxygen species (ROS) generation overwhelming ROS degradation capacity (Raedschelders et al., 2012). Oxidative stress and high levels of ROS which occur in ARHL (Fujimoto and Yamasoba, 2014), sudden SNHL, and noise trauma induced SNHL suggest a common oxidative stress pathogenetic pathway in acquired SNHL. Oxidative stress is associated with ROS production which, in high concentrations, leads to cellular apoptosis and hearing loss. Despite the significant impact on individuals and society, effective treatments for SNHL beyond electro-mechanical devices namely hearing aids or cochlear implants are mostly lacking (Nunez et al., 2020). Changes in miRNA expression in response to hypoxia exert an effect on cells by regulating the function of genes involved in signaling pathways (Gupta et al., 2018). A link between miRNAs and oxidative stress is described in other ischemia associated conditions such as ischemic strokes, and giant cell arteritis (Croci et al., 2016; Devaux et al., 2016; Chen et al., 2017) with miRNAs being proposed as diagnostic or prognostic biomarkers.
miRNAs are endogenous, short regulatory RNA molecules, that modulate target gene expression at the post-translational level by guiding the RNA-induced silencing complex (RISC) to miRNA target sites in the 3’ untranslated region of mRNAs, leading to mRNA degradation or the inhibition of translation (Bartel, 2004). miRNAs are expressed in normal inner ear cells, and play an essential role in their development, differentiation, and survival (Friedman et al., 2009; Ushakov et al., 2013). Changes in miRNA expression have been, well documented in animal models of ARHL (Xiong et al., 2015; Xue et al., 2016) and shown to induce mitochondrial dysfunction and hair cell loss via downregulation of Sirtuin 1/ peroxisome proliferator-activated receptor-gamma coactivator 1α (SIRT1/PGC-1α) signaling in aged mice. SIRT1 regulates intracellular oxidative stress by the deacetylation of its substrates including PGC-1α, a transcriptional co-regulator that binds to numerous transcription factors to promote mitochondrial biogenesis and oxidative metabolism (Someya et al., 2010). Hence, it is reasonable to explore the evidence for changes in miRNAs associated with SNHL. Studies have documented the role of miRNAs in the cochlea in response to hypoxic stress. Following ROS production in the inner ear, changes in miRNAs alter mRNA expression; specifically, upregulation of miR-29a, miR-200c, and miR-17 occurs which in turn, downregulates insulin-like growth factor 1 (IGF-1), alters (IGF-1) mediated signaling and contributes to hearing loss (Ushakov et al., 2013).
Many studies have shown that specific miRNAs (hypoxamiRs) are integral biomolecules in hypoxia-adaptive responses (Gupta et al., 2018). Devaux et al. (2016) found that the level of circulating miR-124-3p after cardiac arrest and cardiomyocyte ischemia was associated with survival outcome in patients who experienced out-of-hospital cardiac arrest. A number of hypoxamiRs have been proposed as diagnostic or prognostic markers namelymiR-223 in stroke (Chen et al., 2017), let-7e, miR-15b, -16, -20b, -25, -26b, -27b, -28–5p, -126, -195, -335, and -363 in peripheral arterial disease (Stather et al., 2013), and miR-342, -191 and -510 in diabetes mellitus (Hezova et al., 2010).
Objective
Therefore, this review aims to identify SNHL related hypoxia-associated miRNAs (hypoxamiRs) with a view to facilitating the development of new SNHL diagnostic, prognostic and therapeutic tools.
Methods
Electronic search strategy
The electronic bibliographic databases PubMed, Ovid MEDLINE, Ovid EMBASE, and Web of Science Core Collection were searched for articles published in English from the inception of the database to the end of July 2020. A text word or MeSH terms search of all fields, titles, abstracts, or subject headings depending on the database was undertaken with combinations of the words “microRNAs”, “hypoxia”, “hypoxamiRs”, “oxidative stress”, “ischemia” and “hearing loss”. The reference lists of studies meeting the inclusion criteria were further searched to identify additional relevant studies.
Article selection
Two investigators (SS and MN) independently screened the titles and abstracts yielded by the search to select full text articles that matched the purpose of this review. Primary articles investigating ARHL, sudden SNHL, noise-, chemical-, ototoxic drug- or neurotoxic drug-induced hearing loss, or other forms of acquired SNHL such as: published clinical studies, case series, and case reports with human participants including adults, children, or neonates who had documented SNHL based on otoacoustic emission, brain stem evoked audiometry, or masked bone conduction pure tone audiometry tests were included. Additionally, published experimental studies on mammalian animal models and in in vitro cell models were included. All types of review articles, non-full text articles, books, conference abstracts, letters, surveys, articles not published in the English language, studies lacking a priori experimental design, and primary studies on non-mammals (example: avian, amphibians) were excluded.
Human participant studies where exposure to hypoxia or ischemia/oxidative stress was documented by objective measures, such as altered plasma glutathione, thioredoxin or clinical measures such as the APGAR score and studies including patients diagnosed with clinical conditions affecting oxygen saturation levels were targeted for inclusion. Likewise, animal model and in vitro cell studies, where hypoxia or tissue ischemia was evidenced by production of ROS or expression of hypoxia-associated genes (e.g., HIF-1α, CDKN1B), and or induced by physiological or pharmacological approaches were included. Human studies without evidence of exposure to hypoxia or tissue ischemia that is oxygen saturation levels of 95%–100% and better, and those studying conditions without an ischemia-driven pathophysiology were excluded. Animal model and in vitro cell model studies carried out under normoxic conditions or where there was no ROS production or expression of hypoxia-associated genes were also excluded.
Any disagreements on the inclusion of individual studies were resolved by the majority opinion in consultation with the senior investigator (DN).
Data extraction
Two investigators (SS, MN) extracted data from the included studies using standard data extraction forms. For the human participants, animal model, and in vitro cell model studies: publication year, authors, study design, type of SNHL investigated, sample size, mean ages of treatment and control group subjects, methods of hypoxia induction, reagents used to induce hypoxia, dosage, exposure time, samples targeted for miRNA extraction, techniques used to determine miRNA expression levels, validation methods, reported differentially expressed miRNAs, confirmed or predicted target genes, and gene enrichment or functional enrichment pathway analysis findings were recorded.
Study investigators undertook data extraction independently. Discrepancies and inadequacies in the data extracted by individual investigators were resolved in consultation with the senior investigators (PW or DN). If relevant data was not reported in an article, the article’s corresponding author was contacted twice to obtain the data.
Outcomes
The primary outcome was the identification of microRNAs involved in different types of acquired SNHL in response to hypoxic, oxidative stress, or ischemic insults (hypoxamiRs). A hypoxamiR was defined for this review as a miRNA that was differentially expressed based on qPCR when compared with controls at a statistically significant (p < 0.05) level, or with a minimum 2-fold intergroup difference; or via other validated methods such as Western blot, reporter assay, sequencing; or similar objective methods. The secondary outcomes were the predicted and validated target gene(s), target genes enriched or functionally enriched pathways, and the suggested underlying molecular targets or pathological actions of the hypoxamiRs. HypoxamiR target genes were similarly defined.
Assessment of study quality and risk of bias
The quality of evidence and risk of bias were assessed independently by three investigators (SS, MN, and DN). The Systematic Review Center for Laboratory animal Experimentation (SYRCLE; Hooijmans et al., 2014) tool based on the Cochrane risk of bias tool adjusted for aspects of bias that play a specific role in animal intervention studies was used for animal studies. It was also used for in vitro cell model studies, as there is currently no widely accepted standardized risk of bias tool for in vitro studies. The SYRCLE tool assesses selection bias (related to sequence generation, baseline characteristics, allocation concealment), performance bias, detection bias, attrition bias, reporting bias, and other sources of bias. The overall score of high, low, or indeterminate based on the SYRCLE risk of bias tool was decided by majority opinion. A high-quality study was defined as one with a low risk of bias in all the assessed domains.
Data presentation
A narrative synthesis was planned. The Cochrane Collaboration’s Review Manager version 5.3 was used to generate Preferred Reporting Items for Systematic Reviews and Meta-Analysis (PRISMA) flow diagram of the results of the electronic searches and to visualize the risk of bias assessments (Mcguinness and Higgins, 2021).
Results
A total of 130 records were identified by database searches. One additional article was found by searching the reference list of included articles. A total of 101 article abstracts were screened after duplicates were removed. A total of 87 that failed to meet study inclusion criteria were excluded. The full text of the remaining 14 articles was reviewed and a further five were excluded for failing to meet the inclusion criteria. The remaining nine articles (Tables 1, 2) formed the basis of this review (Figure 1).
The nine studies included consisted of, two in vitro studies, one animal study, and six that included findings from both in vitro cell and animal studies. Four investigated ototoxic drug-induced SNHL, four investigated age-related hearing loss, and one investigated diabetes-related hearing loss. The study characteristics of the included in vitro cell model studies are summarized in Table 1 and the animal model studies in Table 2.
The risk of bias was found to be unclear due to insufficient information or low in all articles using the SYRCLE tool. No article had areas that were deemed to be at a high risk of bias (Figure 2).
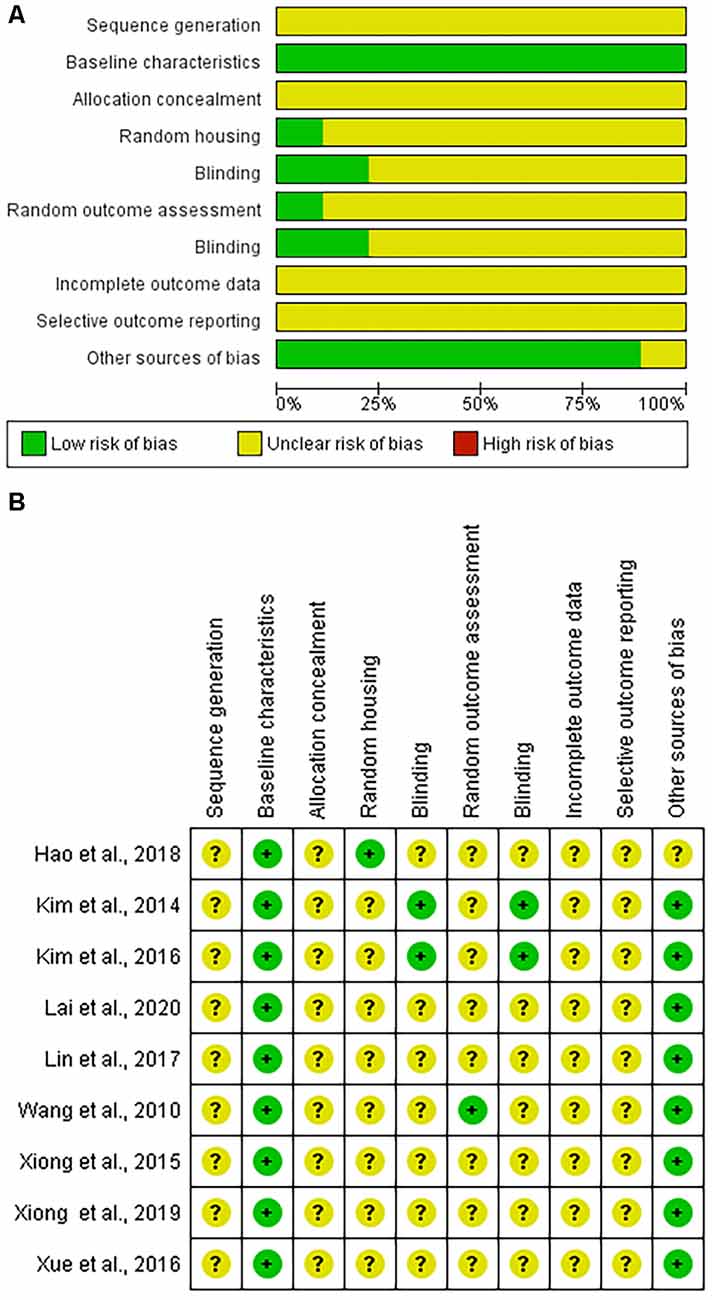
Figure 2. Systematic Review Center for Laboratory animal Experimentation (SYRCLE) risk of bias assessment. (A) Graphic representation summarizing the assessment of the nine included articles. (B) Summary of individual article assessments by SYRCLE domain.
Discussion
The mediating role of miRNAs and ROS during oxidative stress
States of cellular stress, such as hypoxia or oxidative stress, are associated with increased levels of ROS (Raedschelders et al., 2012; Lushchak, 2014). Temporary and milder ROS release may lead to adaptive mechanisms facilitating cellular survival (Lushchak, 2014). However, prolonged ROS over-production and accumulation, such as in drug-induced ototoxicity or aging (Riva et al., 2007), can trigger maladaptive processes such as apoptosis or autophagy that lead to cellular damage and death.
There is now accumulating evidence to suggest that miRNAs play a role in mediating the signaling between excessive ROS production and the deleterious effects on cellular health and survival (Subramanian and Steer, 2010). Therefore, Wang et al. (2010) investigated the miRNA profile of the organ of corti cell damage because of oxidative stress by using increasing concentrations of the potent oxidant t-BHP to induce the release of ROS in HEI-OC1 cells. Following this treatment, the degree of ROS production and cell viability were correlated with miRNA and mRNA expression levels. They found that the expression levels of 35 miRNAs were upregulated and 40 downregulated while the transcription of 2,686 mRNAs were altered. Network analysis revealed miRNA changes consistent with activation of the pro-apoptotic MAPK and JNK signaling pathway (Wang et al., 2003) and inhibition of neuronal protection by downregulation of IGF-1 signaling. Specifically, MAPK signaling pathway miRNAs miR-383, -208a, -377, -181d, -377, and -874 and IGF-1 signaling pathway miRNAs miR-29a, -17, and -200c were responsible for oxidative stress induced cochlea cell death. The authors propose that drugs targeting the MAPK/JNK pathway may have anti-apoptotic effects of relevance to the treatment of SNHL.
Cisplatin ototoxicity and hypoxic stress mediated hair cell damage
Cisplatin is a chemotherapeutic agent used to treat solid tumors (Cohen and Lippard, 2001). It has been associated with progressive hearing loss. The mechanism through which this occurs is not completely understood.
The study by Kim et al. (2014) demonstrated that the ototoxic mechanisms of cisplatin involve a p53 mediated upregulation of poly (ADP-ribose) transferase (PARP)-1 and miR-34a. This leads to a decrease in Nicotinamide adenine dinucleotide (NAD+) levels resulting in decreased Sirtuin 1 (SIRT1). A combination of mouse models and in vitro HEI-OC1 cell lines in this study showed that cisplatin induced oxidative stress that damaged hair cell DNA and hyperactivated PARP-1. PARP-1 activation at modest levels is important for DNA repair, however, excess PARP-1 activity leads to depletion of the co-factor NAD+ (Goodwin et al., 1978). Normally, SIRT1 would consume NAD+ to mediate a range of processes, including DNA repair. However, depleted NAD+ led to reduced SIRT1 activity and DNA repair mechanisms. Moreover, while it had been previously shown that cisplatin treatment led to inhibition of p53 expression that resulted in cytotoxicity, further downstream effects of p53 inhibition were not fully known. This study identified that reduced p53 levels led to overexpression of miR-34a. The increased miRNA-34a then suppressed SIRT1 translation. The reduced SIRT1 activity due to depleted NAD+ pool and translational block by miR-34a led to impaired DNA repair mechanisms resulting in hair cell damage and ototoxicity. Furthermore, Kim et al. (2014) obtained evidence that p53 silencing RNA transfection of HEI-OC1 cells reduced miR-34a overexpression and restored SIRT1 expression in cisplatin treated cells. However, no miR-34a silencing experiments were undertaken so there was no direct evidence that SIRT1 expression is controlled by miR-34a, though it is strongly implied.
Potential novel ototoxicity treatments targeting oxidative stress
Kin et al.’s initial and follow-on studies identified that recovery of the available NAD+ pool by administration of Dunnione an antifungal and anti-tumor drug that is a substrate of antioxidant flavoprotein NADH: quinone oxidoreductase 1 (NQO1) ameliorated the ototoxic effects of cisplatin (Kim et al., 2014, 2016). Gentamicin ototoxicity associated oxidative stress was demonstrated in HEI-OC1 cells cultured under non-permissive conditions by an increase in Malondialdehyde (MDA) and a reduction in superoxide dismutase (SOD), both markers of oxidative stress (Ho et al., 2013). The addition of mouse inner ear stem cell derived exosomes to gentamicin treated HEI-OC1 cell cultures significantly reversed changes in MDA and SOD levels (Lai et al., 2020). These changes were accompanied by increased inner ear cell survival. Lai et al. (2020) studied the effect of exosomes derived from mouse inner ear stem cells (IESC-ex) on gentamicin toxicity in HEI-OC1 cells for evidence that miR-182-5p/FOXO3 was the pathway through which the exosomes were exerting their effect. They added increasing concentrations of IESC-ex to gentamicin-treated non-permissive cultured HEI-OC1 cells and identified a dose-dependant: reduction in gentamicin-induced oxidative stress, downregulation of miR-182-5p, upregulation of FOXO3 mRNA, and protein expression. It was further determined that exosomes derived from miR-182-5p transfected IESCs exacerbated these effects compared with exosomes derived from negative control transfected IESCs and the standard IESC derived exosomes. While no miR-182-5p silencing experiments were undertaken, Lai et al.’s (2020) findings suggest that a miR-182-5p/FOXO3 pathway is involved in gentamicin-induced ototoxicity.
miRNA induced SIRTI reduction a common pathway in oxidative stress and diabetes mediated hair cell loss in ARHL
Xiong et al. (2015, 2019) studied the effects of miR-34a/SIRT1/p53 expression and hair cell death in ARHL. The authors studied the effects of oxidative stress on C57BL/6 mice and cultured HEI-OC1 cells. Auditory brainstem response thresholds increased at all tested frequencies with age, related to cochlear inner and outer hair cell loss. Overexpression of miR-34a inhibited SIRT1 causing increased p53 acetylation and hair cell apoptosis, thus causing hearing loss. Similarly, Xue et al. (2016, 2022) studied the effects of miR-29b on SIRT1/PGC-1α expression in C57BL/6 mice and HEI-OC1 cells in relation to AHL pathogenesis. The authors noted greater mitochondrial dysfunction in the cochleae of aged C75BL/6 mice and decreased hair cell count secondary to hair cell apoptosis. They identified that SIRT1 and PGC-1α expression was decreased in the cochlea of aged mice compared to young mice. Furthermore, miR-29b mimic transfection of HEI-OC1 cells suppressed SIRT1/PGC-1α levels whilst transfection with a miR-29b inhibitor upregulated the expression of SIRT1/PGC-1α. Therefore, the authors established that miR-29b overexpression leads to hair cell loss and mitochondrial dysfunction through the downregulation of SIRT1/PGC-1α signaling with aging.
Hao et al. (2019) investigated the association between a single nucleotide polymorphism of the myocardial ischemia associated transcript (MIAT) which is a long noncoding RNA in ARHL affected humans. The relationship between MIAT, SIRT1, and miR-29b in neuroblastoma cell lines was studied in young and aged C57BL/6 mice. They corroborated Xue et al.’s (2016) finding that PGC-1α/SIRT1 was decreased in aged mice and the frequency of cochlear mitochondrial dysfunction increased. They identified that an allelic polymorphism of MIAT increased the risk of ARHL in humans. Furthermore, MIAT modulated the effect of miR-29b on SIRT1 supporting a possible MIAT/miR-29b/PGC-1α/SIRT1signaling pathway in hair cell apoptosis and mitochondrial dysfunction in ARHL.
Homozygous leptin receptor deficient mice (db/db) that are known to be a good model of human type 2 diabetes (Burke et al., 2017) were compared to heterozygous controls by Lin et al. (2017). The db/db mice demonstrated cochlea hair cell loss, hearing impairment, elevated miR-34a, elevated mRNA levels of Hypoxia inducible factor (HIF), and reduced SIRT1 mRNA. miR-34a mimics had an identical effect on HEI-OC1 cells cultured under high glucose conditions, i.e., SIRT1 decreased and this was associated with increased HIF levels and HEI-OC1 cellular apoptosis. These findings provide further evidence for miRNAs exerting a deleterious effect on hearing via suppression of SIRT1 in association with a hypoxia/ischemia related co-factor.
Potential novel treatments targeting cochlea hair cell depleting oxidative stress
Xiong et al. (2019) discovered that PGC-1α/NRF1/NRF2/TFAM, mitochondrial biogenesis-related proteins, were upregulated in aged C57BL/6 mice suggesting increased mitochondrial biogenesis in the aging cochlea. PINK1/Parkin signaling, characteristic of mitophagy, was also found to be increased. These changes were associated with an increase in mitochondrial mass but reduction in mitochondrial function, suggesting an accumulation of dysfunctional mitochondria with age. The authors concluded that resveratrol, a SIRT1 activator, counteracted the effects of cochlea aging by increasing mitophagy, attenuating increased mitochondrial biogenesis, and lead to improved mitochondrial function. miR-34 a deficient knock down mice were also found to exhibit reduced cochlear outer hair cells with age though less so than SIRT1 transgenic mice. Xiong et al.’s (2019) findings suggest that novel pharmaco therapies may have a role in slowing ARHL progression.
Evidence from clinical practice and other sources relevant to hypoxamiRs in SNHL
The abrupt onset of sudden SNHL and the higher occurrence in metabolic syndrome is similar to acute myocardial infarction and stroke. This supports the ischemic theory of etiology in SSNHL. Autoimmune SNHL also typically presents with sudden hearing deterioration but differs from SSNHL because the episodes are recurrent and there is accompanying evidence of autoimmune disease. ARHL and NIHL the more predominant forms of SNHL occur gradually over years making it difficult to separate out any effect of hypoxic stress-related miRNAs from other causative factors. Therefore, studies of SSNHL and autoimmune SNHL are more likely to reveal information on hypoxia and hypoxic stress miRNAs in SNHL.
miR-132 and -195 were among eight differentially expressed miRNAs (DEMs) in the serum of SSNHL patients (Nunez et al., 2020). They have not so far been classified as hypoxamiRs however bioinformatic analysis reveals that they target RAF1 (proto-oncogene, serine/threonine kinase) which initiates the MAPK pathway. The MAPK signaling pathway, HIF, and VEGF are targets of identified hypoxamiRs (Gupta et al., 2018) supporting a hypoxic causal link in SSNHL. A JNK inhibitor (D-JNKI-1), a synthetic peptide was the active intervention agent in a phase 3 SSNHL clinical trial (ClinicalTrials.gov Identifier: NCT02561091). JNK1 is a member of the MAPK family, and while the outcome of the trial is not publicly available it means that therapeutic interventions targeting the MAPK pathway are feasible. miR-34a a hypoxamiR identified in this review as a SIRT1 inhibitor (Xiong et al., 2015, 2019) was differentially expressed in SSNHL patients’ plasma. Li et al. (2017) also predicted that MAPK pathway genes are likely targets of the 24 DEMS they identified in SSNHL patients. Furthermore, experimental induction of SSNHL by infusing lipopolysaccharides into the cochlea of guinea pigs reduced cochlear expression of apoptosis-inhibition genes Bcl-2 and Bcl-xl. Bcl-xl is linked by bioinformatic analysis to miR-204-5p an established hypoxamiR (Xie et al., 2021). Thus, there is complementary evidence from several sources suggesting that hypoxamiRs acting upon MAPK signaling pathways are important in the pathogenesis of SSNHL.
MAPK and vascular endothelial growth factor (VEGF) also play an important role in the innate immune system working through toll-like receptor (TLR) and interleukin-1β (IL-1β) receptor. MiRs -146 and -155 both target the common TL/IL-1 adaptor molecule myeloid differentiation primary response protein 88 (MYD88) as well as individually targeting TL and IL receptors and so suppressing the immune response. These miRs in turn are targeted by downstream MYD88 responsive molecules suggesting a miRNA/IL-1β/TL feedback mechanism fine tuning the innate immune response (Virtue et al., 2012). While the mechanisms leading to auto-immune SNHL are poorly understood. Pathak et al. (2011) found that auto-immune SNHL non-steroid responsive patients have higher levels of IL-1β and matrix metalloproteinase-9 (mmP-9) than responders. The higher levels of IL-1β and mmP-9 were reversed by an IL receptor agonist. The cause for IL-1β dysregulation is unknown but given the involvement of miRNAs -146, and -155 in an IL-1β feedback loop they are certainly promising candidates for further study.
Limitations
Many included studies except Wang et al.’s (2010) study investigated the role of single selected miRNAs predominantly miR-34a and miR-29b in different types of acquired SNHL using animal models and/or in vitro cell models, thus precluding meta-analysis. No studies were identified that investigated the role of hypoxamiRs specifically in humans based on this review’s inclusion criteria reflecting a lack of human subject studies in this area.
Author contributions
Review idea conception: DN and SS. Drafting the protocol: SS, PW, and DN. Study selection: SS, MN, and DN. Extracting data from studies: SS, MN, and PW. Synthesis and drafting the final review: SS, MN, PW, and DN. All authors contributed to the article and approved the submitted version.
Funding
This review study is funded by a University of British Columbia, Faculty of Medicine, Summer Student Research Program (SSRP) award to SS, Pacific Otolaryngology Foundation, Rotary Club of Vancouver Hearing Foundation, and Vancouver Coastal Research Institute grants to DN.
Conflict of Interest
The authors declare that the research was conducted in the absence of any commercial or financial relationships that could be construed as a potential conflict of interest.
Publisher’s Note
All claims expressed in this article are solely those of the authors and do not necessarily represent those of their affiliated organizations, or those of the publisher, the editors and the reviewers. Any product that may be evaluated in this article, or claim that may be made by its manufacturer, is not guaranteed or endorsed by the publisher.
Acknowledgments
University of British Columbia library resources supported the review.
References
Bartel, D. P. (2004). Micrornas: genomics, biogenesis, mechanism and function. Cell 116, 281–297. doi: 10.1016/s0092-8674(04)00045-5
Burke, S. J., Batdorf, H. M., Burk, D. H., Noland, R. C., Eder, A. E., Boulos, M. S., et al. (2017). Db/db mice exhibit features of human type 2 diabetes that are not present in weight-matched c57bl/6j mice fed a western diet. J. Diabetes Res. 2017:8503754. doi: 10.1155/2017/8503754
Croci, S., Zerbini, A., Boiardi, L., Muratore, F., Bisagni, A., Nicoli, D., et al. (2016). MicroRNA markers of inflammation and remodelling in temporal arteries from patients with giant cell arteritis. Ann. Rheum. Dis. 75, 1527–1533. doi: 10.1136/annrheumdis-2015-207846
Chen, Y., Song, Y., Huang, J., Qu, M., Zhang, Y., Geng, J., et al. (2017). Increased circulating exosomal mirna-223 is associated with acute ischemic stroke. Front. Neurol. 8:57. doi: 10.3389/fneur.2017.00057
Cohen, S. M., and Lippard, S. J. (2001). Cisplatin: from dna damage to cancer chemotherapy. Prog. Nucleic Acid Res. Mol. Biol. 67, 93–130. doi: 10.1016/s0079-6603(01)67026-0
Devaux, Y., Dankiewicz, J., Salgado-Somoza, A., Stammet, P., Collignon, O., Gilje, P., et al. (2016). Association of circulating microrna-1243p levels with outcomes after out-of-hospital cardiac arrest: a substudy of a randomized clinical trial. JAMA Cardiol. 1, 305–313. doi: 10.1001/jamacardio.2016.0480
Friedman, L. M., Dror, A. A., Mor, E., Tenne, T., Toren, G., Satoh, T., et al. (2009). Micrornas are essential for development and function of inner ear hair cells in vertebrates. Proc. Natl. Acad. Sci. U S A 106, 7915–7920. doi: 10.1073/pnas.0812446106
Fujimoto, C., and Yamasoba, T. (2014). Oxidative stresses and mitochondrial dysfunction in age-related hearing loss. Oxid. Med. Cell Longev. 2014: 582849.. doi: 10.1155/2014/582849
Global Burden of Disease Study 2013 Collaborators (2015). Global, regional and national incidence, prevalence and years lived with disability for 301 acute and chronic diseases and injuries in 188 countries, 1990-2013: a systematic analysis for the global burden of disease study 2013. Lancet 386, 743–800. doi: 10.1016/S0140-6736(15)60692-4
GBD 2019 Hearing Loss Collaborators (2021). Hearing loss prevalence and years lived with disability: findings from the global burden of disease study 2019. Lancet 397, 996–1009. doi: 10.1016/S0140-6736(21)00516-X
Goodwin, P. M., Lewis, P. J., Davies, M. I., Skidmore, C. J., and Shall, S. (1978). The effect of gamma radiation and neocarzinostatin on nad and atp levels in mouse leukaemia cells. Biochim. Biophys. Acta 543, 576–582. doi: 10.1016/0304-4165(78)90312-4
Gupta, A., Sugadev, R., Sharma, Y. K., Yahmad, Y., and Khurana, P. (2018). Role of mirnas in hypoxia-related disorders. J. Biosci. 43, 739–749. doi: 10.1007/s12038-018-9789-7
Hao, S., Wang, L., Zhao, K., Zhu, X., and Ye, F. (2019). Rs1894720 polymorphism in miat increased susceptibility to age-related hearing loss by modulating the activation of mir-29b/strt1/pgc-1 signaling. J. Cell. Biochem. 120, 4975–4986. doi: 10.1002/jcb.27773
Hezova, R., Slaby, O., Faltejskova, P., Mikulkova, Z., Buresova, I., Raja, K. R., et al. (2010). Microrna-342, microrna-191 and microrna-510 are differentially expressed in t regulatory cells of type 1 diabetic patients. Cell. Immunol. 260, 70–74. doi: 10.1016/j.cellimm.2009.10.012
Ho, E., Karimi Galougahi, K., Liu, C. C., Bhindi, R., and Figtree, G. A. (2013). Biological markers of oxidative stress: applications to cardiovascular research and practice. Redox Biol. 1, 483–491. doi: 10.1016/j.redox.2013.07.006
Hooijmans, C. R., Rovers, M. M., De Vries, R. B., Leenaars, M., Ritskes-Hoitinga, M., and Langendam, M. W. (2014). Syrcle’s risk of bias tool for animal studies. BMC Med. Res. Methodol. 14:43. doi: 10.1186/1471-2288-14-43
Kim, H. J., Oh, G. S., Shen, A., Lee, S. B., Choe, S. K., Kwon, K. B., et al. (2014). Augmentation of nad(+) by nqo1 attenuates cisplatin-mediated hearing impairment. Cell Death Dis. 5:e1292. doi: 10.1038/cddis.2014.255
Kim, C., Sohn, J. H., Jang, M. U., Hong, S. K., Lee, J. S., Kim, H. J., et al. (2016). Ischemia as a potential etiologic factor in idiopathic unilateral sudden sensorineural hearing loss: analysis of posterior circulation arteries. Hear. Res. 331, 144–151. doi: 10.1016/j.heares.2015.09.003
Lai, R., Cai, C., Wu, W., Hu, P., and Wang, Q. (2020). Exosomes derived from mouse inner ear stem cells attenuate gentamicin-induced ototoxicity in vitro through the mir-182-5p/foxo3 axis. J. Tissue Eng. Regen. Med. 14, 1149–1156. doi: 10.1002/term.3089
Li, Q., Peng, X., Huang, H., Li, J., Wang, F., and Wang, J. (2017). Rna sequencing uncovers the key micrornas potentially contributing to sudden sensorineural hearing loss. Medicine (Baltimore) 96:e8837. doi: 10.1097/MD.0000000000008837
Lin, Y., Shen, J., Li, D., Ming, J., Liu, X., Zhang, N., et al. (2017). Mir-34a contributes to diabetes-related cochlear hair cell apoptosis via strt1/hif-1 signaling. Gen. Comp. Endocrinol. 246, 63–70. doi: 10.1016/j.ygcen.2017.02.017
Lushchak, V. I. (2014). Free radicals, reactive oxygen species, oxidative stress and its classification. Chem. Biol. Interact. 224, 164–175. doi: 10.1016/j.cbi.2014.10.016
Mcguinness, L. A., and Higgins, J. (2021). Risk-of-bias visualization (robvis): an r package and shiny web app for visualizing risk-of-bias assessments. Res. Synthesis Methods 12, 55–61. doi: 10.1002/jrsm.1411
Nunez, D. A., Wijesinghe, P., Nabi, S., Yeh, D., and Garnis, C. (2020). Micrornas in sudden hearing loss. Laryngoscope 130, E416–E422. doi: 10.1002/lary.28327
Pathak, S., Goldofsky, E., Vivas, E. X., Bonagura, V. R., and Vambutas, A. (2011). Il-1 is overexpressed and aberrantly regulated in corticosteroid nonresponders with autoimmune inner ear disease. J. Immunol. 186, 1870–1879. doi: 10.4049/jimmunol.1002275
Raedschelders, K., Ansley, D. M., and Chen, D. D. (2012). The cellular and molecular origin of reactive oxygen species generation during myocardial ischemia and reperfusion. Pharmacol. Ther. 133, 230–255. doi: 10.1016/j.pharmthera.2011.11.004
Riva, C., Donadieu, E., Magnan, J., and Lavieille, J. P. (2007). Age-related hearing loss in cd/1 mice is associated to ros formation and hif target proteins up-regulation in the cochlea. Exp. Gerontol. 42, 327–336. doi: 10.1016/j.exger.2006.10.014
Someya, S., Yu, W., Hallows, W. C., Xu, J., Vann, J. M., Leeuwenburgh, C., et al. (2010). Sirt3 mediates reduction of oxidative damage and prevention of age-related hearing loss under caloric restriction. Cell 143, 802–812. doi: 10.1016/j.cell.2010.10.002
Starkov, A. A., Chinopoulos, C., and Fiskum, G. (2004). Mitochondrial calcium and oxidative stress as mediators of ischemic brain injury. Cell Calcium 36, 257–264. doi: 10.1016/j.ceca.2004.02.012
Stather, P. W., Sylvius, N., Wild, J. B., Choke, E., Sayers, R. D., and Bown, M. J. (2013). Differential microrna expression profiles in peripheral arterial disease. Circ. Cardiovasc. Genet. 6, 490–497. doi: 10.1161/circgenetics.111.000053
Subramanian, S., and Steer, C. J. (2010). Micrornas as gatekeepers of apoptosis. J. Cell. Physiol. 223, 289–298. doi: 10.1002/jcp.22066
Trune, D. R., and Nguyen-Huynh, A. (2012). Vascular pathophysiology in hearing disorders. Semin. Hear. 33, 242–250. doi: 10.1055/s-0032-1315723
Ushakov, K., Rudnicki, A., and Avraham, K. B. (2013). Micrornas in sensorineural diseases of the ear. Front. Mol. Neurosci. 6:52. doi: 10.3389/fnmol.2013.00052
Virtue, A., Wang, H., and Yang, X. F. (2012). Micrornas and toll-like receptor/interleukin-1 receptor signaling. J. Hematol. Oncol. 5:66. doi: 10.1186/1756-8722-5-66
Wang, Z., Liu, Y., Han, N., Chen, X., Yu, W., Zhang, W., et al. (2010). Profiles of oxidative stress-related microrna and mrna expression in auditory cells. Brain Res. 1346, 14–25. doi: 10.1016/j.brainres.2010.05.059
Wang, J., Van De Water, T. R., Bonny, C., De Ribaupierre, F., Puel, J. L., and Zine, A. (2003). A peptide inhibitor of c-jun n-terminal kinase protects against both aminoglycoside and acoustic trauma-induced auditory hair cell death and hearing loss. J. Neurosci. 23, 8596–8607. doi: 10.1523/JNEUROSCI.23-24-08596.2003
World Health Organization (2017). Global costs of unaddressed hearing loss and cost-effectiveness of interventions: a WHO report, 2017. London, UK: IWA Publishing.
Xie, L., Zhou, Q., Chen, X., Du, X., Liu, Z., Fei, B., et al. (2021). Elucidation of the hdac2/Sp1/mir-2045p/Bcl-2axis as a modulator of cochlear apoptosis via in vivo/in vitro models of acute hearing loss. Mol. Ther. Nucleic Acids 23, 1093–1109. doi: 10.1016/j.omtn.2021.01.017
Xiong, H., Chen, S., Lai, L., Yang, H., Xu, Y., Pang, J., et al. (2019). Modulation of mir-34a/strt1 signaling protects cochlear hair cells against oxidative stress and delays age-related hearing loss through coordinated regulation of mitophagy and mitochondrial biogenesis. Neurobiol. Aging 79, 30–42. doi: 10.1016/j.neurobiolaging.2019.03.013
Xiong, H., Pang, J., Yang, H., Dai, M., Liu, Y., Ou, Y., et al. (2015). Activation of mir-34a/sirt1/p53 signaling contributes to cochlear hair cell apoptosis: implications for age-related hearing loss. Neurobiol. Aging 36, 1692–1701. doi: 10.1016/j.neurobiolaging.2014.12.034
Xue, T., Wei, L., Zha, D., Qiu, J., Chen, F., Qiao, L., et al. (2016). Mir-29b overexpression induces cochlear hair cell apoptosis through the regulation of strt1/pgc-1 signaling: implications for age-related hearing loss. Int. J. Mol. Med. 38, 1387–1394. doi: 10.3892/ijmm.2016.2735
Keywords: hearing loss, microRNAs, hypoxia, ischemia, oxidative stress, hypoxamiRs
Citation: Safabakhsh S, Wijesinghe P, Nunez M and Nunez DA (2022) The role of hypoxia-associated miRNAs in acquired sensorineural hearing loss. Front. Cell. Neurosci. 16:916696. doi: 10.3389/fncel.2022.916696
Received: 09 April 2022; Accepted: 20 June 2022;
Published: 05 August 2022
Edited by:
Jordi Llorens, University of Barcelona, SpainReviewed by:
Peter S. Steyger, Creighton University, United StatesIsabel Varela-Nieto, Spanish National Research Council (CSIC), Spain
Copyright © 2022 Safabakhsh, Wijesinghe, Nunez and Nunez. This is an open-access article distributed under the terms of the Creative Commons Attribution License (CC BY). The use, distribution or reproduction in other forums is permitted, provided the original author(s) and the copyright owner(s) are credited and that the original publication in this journal is cited, in accordance with accepted academic practice. No use, distribution or reproduction is permitted which does not comply with these terms.
*Correspondence: Desmond A. Nunez, ZGVzbW9uZC5udW5lekB1YmMuY2E=