- 1Department of Psychiatry, TN Medical College and BYL Nair Charitable Hospital, Mumbai, India
- 2Department of Psychiatry, National Institute of Mental Health and Neurosciences, Bengaluru, India
The current review provides an overview of the existing literature on multimodal transcranial magnetic stimulation, and functional magnetic resonance imaging (TMS/fMRI) studies in individuals with schizophrenia and discusses potential future avenues related to the same. Multimodal studies investigating pathophysiology have explored the role of abnormal thalamic reactivity and have provided further evidence supporting the hypothesis of schizophrenia as a disorder of aberrant connectivity and cortical plasticity. Among studies examining treatment, low-frequency rTMS for the management of persistent auditory verbal hallucinations (AVH) was the most studied. While multimodal TMS/fMRI studies have provided evidence of involvement of local speech-related and distal networks on stimulation of the left temporoparietal cortex, current evidence does not suggest the superiority of fMRI based neuronavigation over conventional methods or of active rTMS over sham for treatment of AVH. Apart from these, preliminary findings suggest a role of rTMS in treating deficits in neurocognition, social cognition, and self-agency. However, most of these studies have only examined medication-resistant symptoms and have methodological concerns arising from small sample sizes and short treatment protocols. That being said, combining TMS with fMRI appears to be a promising approach toward elucidating the pathophysiology of schizophrenia and could also open up a possibility toward developing personalized treatment for its persistent and debilitating symptoms.
Introduction
Schizophrenia is a severe mental illness characterized by positive (such as delusions, hallucinations), negative (anhedonia, asociality), cognitive (such as working memory deficits) symptom clusters. It has a life-time prevalence of around 1% and typically begins in late adolescence or early adulthood, leading to substantial disability, morbidity and mortality. While the exact pathophysiology of the illness remains elusive, schizophrenia is generally considered to be caused by a combination of genetic liability and environmental influences.
Current pharmacological strategies primarily focus on improving positive symptoms, with little or no effect on the negative and cognitive symptoms. Furthermore, the medications are effective in 1 only 50% of the cases, thus creating a need for newer strategies to target not only resistant positive symptoms, but also the other symptom clusters (de Araújo et al., 2012). Transcranial magnetic stimulation (TMS) is a neuromodulatory technique that acts via electromagnetic induction to generate an electric current in the superficial layers of the cortex. Single or paired-pulse TMS can be used as a neurophysiological probe to understand brain functions (Polanía et al., 2018). With these paradigms, TMS can have an excellent temporal resolution to the order of milliseconds (Bolognini and Ro, 2010). Further, when given repetitively in trains, rTMS can have differential effects by causing excitatory or inhibitory changes depending on the stimulation pattern and the cortical state (Wagner et al., 2007). These perturbation effects can cause plastic changes lasting 30–45 min and can be used to enhance or disrupt the underlying cortical networks. TMS over a target area causes effects in the underlying target areas and remote anatomically and functionally interconnected regions. Hence, TMS has limited spatial resolution when used on its own unless combined with an imaging modality (Wagner et al., 2007).
In contrast, functional magnetic resonance imaging (fMRI) offers the advantage of having a high spatial resolution. When combined with TMS as a neurophysiological probe, fMRI can be used to confirm the findings of ‘virtual lesions’ created using TMS (Pascual-Leone et al., 2000). Similarly, we can measure TMS-induced disruption of one node in a brain network on other distant nodes to yield brain connectivity metrics (Wagner et al., 2007). While fMRI has a weaker temporal resolution than EEG, it has an added advantage due to its ability to detect and monitor activity changes across larger and deeper areas such as subcortical structures (Siebner et al., 2009).
Electrophysiological and neurobiological research in the last two decades has demonstrated schizophrenia to be a disconnection syndrome involving widespread neuronal networks (Maran et al., 2016; Li et al., 2019; Mehta et al., 2019). However, these studies were based on individual applications of investigational techniques and were primarily correlational. Combining existing investigational techniques allows us to overcome their individual shortcomings and pave way for better understanding of pathophysiology of psychiatric illnesses. For example, combining TMS with EEG (TMS/EEG) can allow for simultaneous perturbation and measurement of neurophysiological correlates of cortical functioning in schizophrenia (Vittala et al., 2020). Using this technique, studies have demonstrated evidence of dysfunction in the frontal thalamocortical circuits in general and impaired cortical connectivity in the dorsolateral prefrontal cortex (DLPFC) in particular as compared to healthy controls using the single pulse paradigm (Li et al., 2021). Similarly, it is now possible to perform concurrent TMS/fMRI to interfere with specifically targeted networks and examining the cortical- and behavioral-level after-effects. fMRI exploits neurovascular coupling and can easily map TMS-evoked neuronal activity with high spatial resolution while providing a whole-brain coverage. However, owing to significant technical challenges, the concurrent TMS/fMRI setup currently exists in only a few specialized labs worldwide. The TMS coil has to be devoid of ferromagnetic material like other equipment in the MR environment. The TMS stimulator either has to be kept inside a shielded metal cabinet or outside the MR room, to which the coil then has to be connected using a waveguide. Additionally, a low-pass filter is necessary to filter out external high-frequency noise (Bungert et al., 2012a). Conventional MR radiofrequency (RF) coils pose obvious constraints on the positioning of the TMS coil. To surmount this issue, single-channel transmit/receive (Tx/Rx) volume ‘bird-cage’ coils can be used, which provide an adequate opening for optimal positioning of the TMS coil (Bestmann et al., 2003). However, these are single-channel RF coils which are insufficient for performing modern parallel multiband imaging sequences. Hence, some labs now use commercially available or custom-made flexible multichannel surface RF coil arrays (Wang et al., 2017; Oh et al., 2019) which can easily accommodate MR compatible TMS coils and also provide high-quality images for a concurrent TMS/fMRI study. To stabilize the TMS coil in a fixed position for the entire duration of the fMRI recording, customized MR compatible coil holders are required (Bestmann et al., 2003; Moisa et al., 2009).
The presence of a TMS coil between the subject’s head and the RF coil leads to local field inhomogeneities causing significant limitations in the signal-to-noise ratio (SNR). To overcome this, thinner MR compatible 7-channel surface RF-coil arrays have been developed, which can be mounted directly below the TMS coil. These novel arrays have been shown to achieve a five-fold rise in SNR at 3 cm depth underneath the TMS coil as compared to the bird-cage coils (Navarro de Lara et al., 2015, 2017). However, this arrangement can cause a reduction in the effective stimulation intensity and pose difficulties in reaching suprathreshold intensities in subjects with high motor threshold.
Use of concurrent TMS/fMRI can lead to static artifacts due to the presence of TMS coil on the magnetic field of the scanner, or cause dynamic artifacts during the actual discharging of the TMS coil. The field inhomogeneities caused by the TMS coil itself can be reduced using shimming techniques before image acquisition (Bungert et al., 2012b). Apart from these, eddy currents created by the changing MR fields in the copper windings of the TMS coil can be prevented using MR compatible TMS coils. Tiny leakage currents generated in the capacitors inside the TMS device can transmit through the coil, causing image artifacts (Weiskopf et al., 2009). These can be minimized using actively controlled high-voltage relay-diode systems to electrically insulate the TMS coil from the stimulator until immediately before and after each TMS pulse or by using built-in leakage filters (Weiskopf et al., 2009). Dynamic artifacts caused by the TMS pulse on the RF pulse can be prevented by setting precise time intervals between the two techniques (Bestmann et al., 2003; Navarro de Lara et al., 2017).
Clearly, setting up and running an adequately accurate system of acquiring concurrent TMS/fMRI data is contingent upon strong multidisciplinary technological expertise, timely quality control evaluations and a liberal financial support to acquire and maintain such state-of-the-art equipment. Once these aforementioned challenges have been met, TMS/fMRI can be used to effectively to make causal inferences based on a specific hypothesis. For example, based on the evidence of abnormal activity in the subgenual anterior cingulate cortex (sgACC) in individuals with depression, a study by Vink et al. (2018) assessed for the propagation of TMS-induced activity to sgACC after stimulation of left dorsolateral prefrontal cortex (DLPFC). Combined TMS/fMRI approaches facilitate a better understanding of brain physiology in general and psychiatric illnesses like schizophrenia in particular by overcoming the shortcomings of either technique alone. The following review provides an overview of the existing literature on multimodal TMS/fMRI in individuals with schizophrenia and potential future avenues.
Materials and Methods
We conducted a systematic review was based on the recommended PRISMA guidelines1 using the PubMed electronic database. We searched for all publications whose titles or abstracts contained the following terms: magnetic resonance imaging OR functional MRI OR FMRI AND Transcranial magnetic stimulation OR TMS AND schizophrenia OR psychosis. We established the following inclusion criteria: (a) Experiments recruiting individuals with a diagnosis of schizophrenia, (b) use of single-/paired-pulse/repetitive TMS, and (c) use of resting-state or task-based fMRI. We included all kinds of publications such as case-control and open-label studies, case reports, and conference abstracts. Review articles and experiments which explored physiological processes in otherwise healthy subjects using TMS/fMRI were excluded. Both the authors conducted the searches and the selection process independently.
For the selected titles, full-text articles were retrieved, and reference lists of each were searched for additional publications. In case of incomplete or missing information, the corresponding author of the included studies were contacted. The initial search strategy yielded 53 results; after applying the selection criteria, 30 studies were included in the review based on both authors’ consensus. These were then categorized as those exploring schizophrenia pathophysiology (n = 6 studies) and those exploring treatment of schizophrenia (n = 24 studies). Figure 1 describes the flow diagram of the selection/inclusion process followed in this review.
Results
Multimodal TMS/fMRI Studies Exploring the Pathophysiology of Schizophrenia
A summary of all the 6 studies investigating the pathophysiology of schizophrenia has been presented in Table 1. These can be understood as those exploring cortical connectivity and those exploring cortical reactivity. Three of these experiments have utilized interleaved (concurrent) TMS/fMRI, while the rest have used it in a sequential or offline fashion by obtaining independent measurements and then correlating the two.
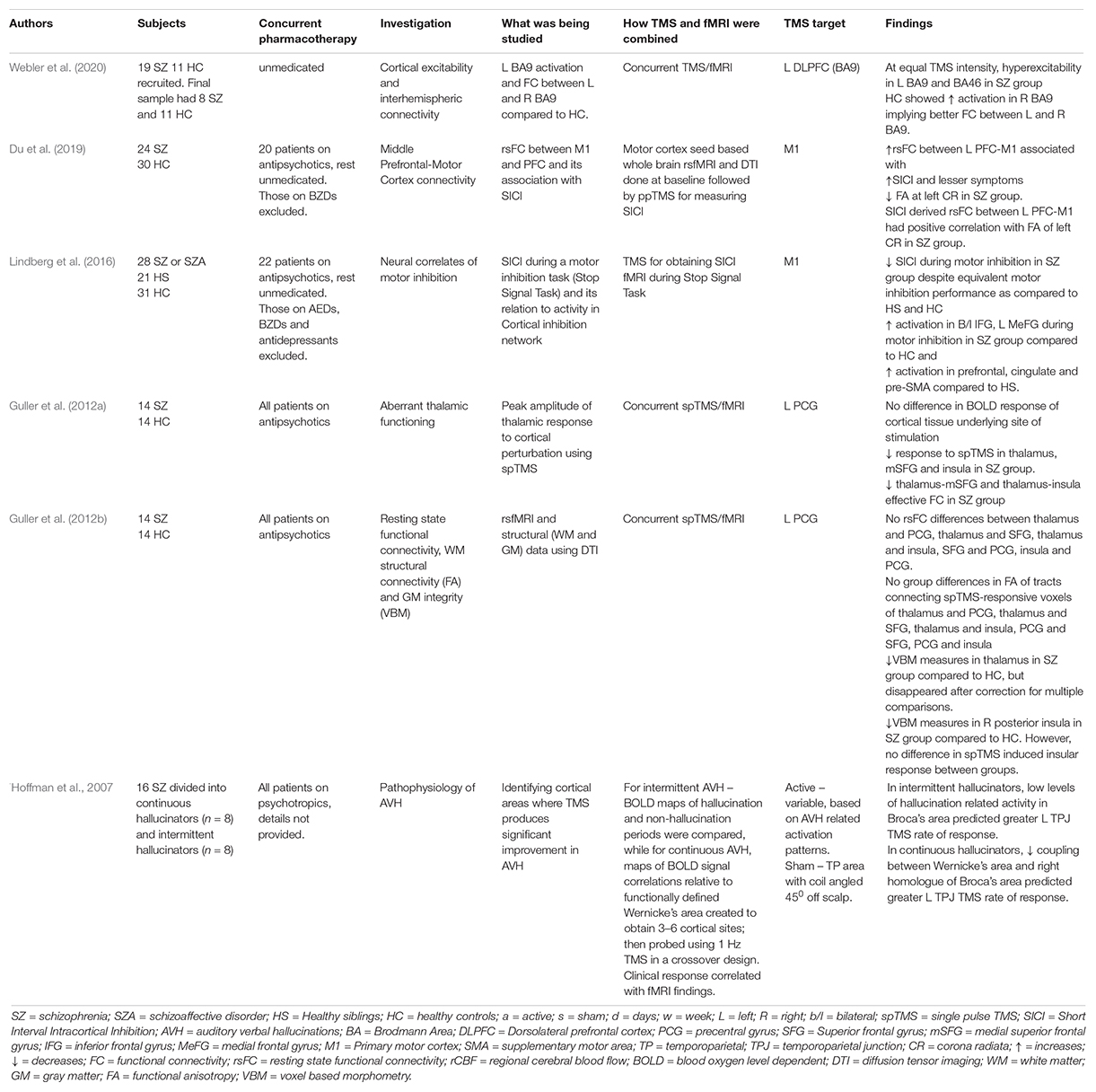
Table 1. Technical aspects and principal findings of TMS/fMRI studies exploring pathophysiology of schizophrenia.
Cortical Connectivity
Single-pulse TMS (spTMS) to the precentral gyrus has been utilized with concurrent fMRI to measure response in synaptically connected regions (thalamus, medial superior frontal cortex, insula) in a case-control study (Guller et al., 2012a). Schizophrenia patients showed reduced activation in the thalamus, medial superior frontal cortex, and insula response to spTMS to the precentral gyrus. Functional connectivity analyses revealed weaker thalamus-medial superior frontal cortex and thalamus-insula connectivity in patients, thereby demonstrating aberrant thalamic connectivity in schizophrenia (Guller et al., 2012a). In an extension of the experiment, resting state functional connectivity (rsFC), white matter (WM) structural connectivity, and gray matter (GM) integrity were assessed in the same subjects using DTI (Guller et al., 2012b). The study found impaired effective connectivity (measured using spTMS/fMRI) but normal functional connectivity (measured using resting state fMRI or rsfMRI) in schizophrenia patients and failed to find any WM or GM abnormalities that could explain the aberrant functional thalamic connectivity.
Cortical Reactivity
Short Interval Intracortical Inhibition (SICI) is a paired-pulse TMS paradigm that is known to be mediated by GABAA receptors (Kujirai et al., 1993). Previous literature has consistently demonstrated SICI to be deficient in individuals with schizophrenia, implying a reduction in intracortical GABAergic neurotransmission (Radhu et al., 2013). A case-control study was conducted by Lindberg et al. to assess neural correlates of motor inhibition using concurrent fMRI/TMS. The study utilized a Stop Signal Task (SST) as a measure of volitional motor inhibition and the rapidity of inhibition process was estimated for each subject (labeled Stop Signal Reaction Time, SSRT). Simultaneously, motor evoked potentials (measure of cortical excitability) and SICI (measure of motor inhibition) were recorded during the stop-go task of the SST. Following this, fMRI data during motor inhibition was recorded using a modified version of the SST. The study demonstrated that despite having an equal motor inhibition performance on the SST, fMRI showed greater prefrontal and premotor activation in schizophrenia during the inhibition task than controls (Lindberg et al., 2016). This task-related modulation of SICI was notably higher in subjects who showed less inhibition-related activity in pre-SMA and cingulate motor area, providing direct evidence of task-related deficiency of SICI modulation. Another case-control study performed measurements of SICI, followed by seed-based whole-brain functional connectivity (FC) using the SICI stimulation site and diffusion tensor imaging (Du et al., 2019). Higher resting-state left prefrontal-motor cortex functional connectivity, accompanied by a higher functional anisotropy of left corona radiata was found to predict less inhibitory deficits (or higher SICI), implying that a top-down prefrontal influence might partly mediate the inhibitory deficits in the motor cortex in schizophrenia.
A recent case-control study by Webler et al. (2020) assessed for prefrontal excitability and interhemispheric functional connectivity using concurrent TMS/fMRI in schizophrenia patients and compared them with healthy controls. In both groups, resting motor threshold (RMT) was estimated at baseline and the left-sided DLPFC (Brodmann area 9) was then stimulated using 35 triplet TMS pulses at 100 ms apart (10Hz) at 0, 80, 100, and 120% of RMT in a randomized order. Simultaneously, fMRI was performed to assess for activation patterns in bilateral BA 9 and neighboring BA46. The study found that schizophrenia patients showed hyperexcitability in left-sided BA9 and BA46 compared to healthy controls for equal TMS intensity. Also, on stimulating the left BA9, healthy controls showed increased right-sided BA9 activity compared to schizophrenia patients, thereby demonstrating impaired interhemispheric connectivity in the patients (Webler et al., 2020).
Pathophysiology of Auditory Verbal Hallucinations
Apart from these studies, one study has investigated auditory verbal hallucinations (AVH) using TMS/fMRI (Hoffman et al., 2007). The study aimed to identify cortical sites where treatment with rTMS produced significant reduction in AVH and then assess statistical relationship between clinical response and fMRI changes in these regions. For this study, patients of schizophrenia with resistant AVH were divided into continuous or intermittent hallucinators. For intermittent hallucinators, BOLD activation maps comparing hallucination and non-hallucination periods were generated by using a behavioral task to demarcate onset and offset of each hallucination event. In continuous hallucinators, functionally defined Wernicke’s area was delineated in each case using the activation patterns generated while listening to external speech. Correlations between BOLD signal time course in Wernicke’s area, and other regions were used to map functional coupling to the former. In both groups, activation maps for AVH were then created around Wernicke’s area and 3–6 cortical sites for each case were identified. These were then probed using 1-Hz (16 min, once daily for 3 days) and sham rTMS using a crossover design. To the site producing greatest clinical benefit, 3 more days of active rTMS was administered after unmasking. The study demonstrated that temporoparietal areas of the dominant hemisphere were involved in experience of AVH and rTMS to these areas produced greater rates of improvement as compared to anterior temporal sites and sham stimulation. The study also demonstrated involvement of inferior frontal regions in the pathophysiology of AVH as suggested by higher levels of coactivation involving inferior frontal and temporoparietal areas during hallucination periods and a robust negative correlation between temporoparietal rTMS response and hallucination-related activation/coupling involving inferior frontal regions.
Multimodal TMS/fMRI Studies Exploring the Treatment of Schizophrenia
A summary of all the studies exploring the treatment of schizophrenia has been presented in Supplementary Table 1. Additional details of the rTMS treatment-related parameters and outcome measures used in the studies have been provided in Table 2. Among positive symptoms of schizophrenia, the management of treatment-resistant AVH has been explored the most. These multimodal studies have either utilized fMRI for target localization (neuronavigation) or the comparison of pre-and post-treatment functional connectivity changes or both.
Hallucinations
Most studies have utilized block design fMRI for target localization using language tasks to create individualized cortical targets of auditory processing areas for treatment using rTMS. This is based on the hypothesis that abnormalities in the speech/language network underlie the pathophysiology of AVH in schizophrenia (Hoffman et al., 1999; Gavrilescu et al., 2010; Oertel-Knöchel et al., 2014). Others have utilized the event-related/symptom capture fMRI paradigm to create individualized activation maps for target localization (Sommer et al., 2007; Slotema et al., 2011; de Weijer et al., 2014). The second group of multimodal studies has utilized fMRI to assess whether rTMS leads to functional connectivity changes in the areas implicated in AVH and whether these changes correlate with clinical improvement. Based on the existing literature, the efficacy of fMRI-guided rTMS over sham for AVH has not clearly been established (Schönfeldt-Lecuona et al., 2004; Slotema et al., 2011; de Weijer et al., 2014; Paillère-Martinot et al., 2017).
Similarly, studies directly comparing fMRI guided and non-guided (10/20 EEG system based) treatment of AVH has also not found any superiority of the former over the latter (Sommer et al., 2007; Slotema et al., 2011). However, there has been evidence from some sham-controlled studies that active rTMS to the temporoparietal junction affects local speech-related network as well as its connections to distal networks (Vercammen et al., 2010; Bais et al., 2017). The first study demonstrated increased connectivity between left temporoparietal junction (TPJ) and right insula secondary to active treatment (Vercammen et al., 2010). The second study compared the effects of left TPJ, bilateral TPJ, and sham stimulation on inner speech-related brain networks (Bais et al., 2017). It showed that active rTMS to the left or bilateral TPJ areas resulted in a weaker network contribution of the left supramarginal gyrus to the bilateral frontotemporal network, which was hypothesized to a reduced likelihood of speech intrusions. However, only left TPJ stimulation led to stronger network contributions of right superior temporal gyrus to functional areas involved in attention and cognitive control, hinting toward the possible superiority of left TPJ stimulation to bilateral TPJ stimulation.
Apart from AVH, management of treatment resistant coenesthetic hallucinations has also been explored in a single case study. Based on activity in bilateral somatosensory cortices (SSC) during active hallucinations using data-driven analyses, the patient was administered 10 days of neuronavigated 1Hz rTMS over SSC, with which the frequency and intensity of coenesthetic hallucinations decreased (Jardri et al., 2008). However, sham stimulation of the same site was not tried prior to the active stimulation.
Negative Symptoms
Only three studies have specifically examined efficacy of rTMS for treating negative symptoms using multimodal TMS/fMRI approach (Dlabac-de Lange et al., 2015; Basavaraju et al., 2019; Brady et al., 2019). The earliest of these, a double-blind randomized sham controlled trial (RCT), examined the effect of 3 weeks of 10 Hz rTMS to bilateral DLPFC (located using EEG 10–20 system) on frontal brain activation in patients with negative symptoms of schizophrenia, as measured by fMRI during the Tower of London (ToL) task (Dlabac-de Lange et al., 2015). The study demonstrated an increased activity in the right DLPFC and right medial frontal gyrus in the active arm, which was accompanied by significant improvement in negative symptoms as compared to the sham arm. The second RCT employed a different approach by using rsfMRI to identify functional connectivity correlates of negative symptoms (Brady et al., 2019). The study found the functional connectivity breakdown between the right DLPFC and the midline cerebellar node in the default network as the most significant predictor of negative symptom severity in a network discovery cohort. Five days of twice daily cerebellar intermittent theta burst stimulation (iTBS) led to improvement in negative symptoms and this was associated with the reversal of functional dysconnectivity in an independent cohort. However, a subsequent sham controlled RCT of 5 days of twice daily iTBS to cerebellar vermis demonstrated a significant but equal improvement in negative symptoms in both active and sham groups at the end of treatment and at 6-week follow-up (Basavaraju et al., 2019). Nevertheless, only the active TMS group showed a significant engagement of the cerebellar-prefrontal resting-state functional connectivity.
Cognitive Symptoms
Two studies have examined the effect of rTMS on cognition in schizophrenia using task-based fMRI (Prikryl et al., 2012; Guse et al., 2013). Both of these have assessed for improvement in performance of working memory (WM) tasks (verbal fluency and letter 2-back) along with changes in neuronal activation during task-based fMRI using a double-blind sham-controlled design. The first trial found equal improvement in WM task performance in both arms and failed to show any differences in task-based activation in either groups (Prikryl et al., 2012). The second trial also utilized an additional healthy control arm to compare baseline and post-treatment scores with schizophrenia patients. The study did not find any differences in WM task-based activation between schizophrenia patients and healthy controls after 3 weeks of 10Hz rTMS (110% RMT, ITI 30 s, 1000 stimuli per session) or surprisingly, even at baseline (Guse et al., 2013).
Social Cognition
Only one sham controlled study has indirectly assessed the role of rTMS in social cognition in schizophrenia using multimodal approach (Liemburg et al., 2018). The RCT primarily assessed for activity changes in the prefrontal cortex during an ambiguous socio-emotional processing (Wall of Faces) task at baseline and compared with those after 3 weeks of 10Hz rTMS to bilateral DLPFC. It demonstrated a reduction in task-based activation in frontal, parietal and striatal regions, which they hypothesized to be possibly secondary to more effective processing in the prefrontal brain networks secondary to active treatment.
Agency
The role of rTMS in self-agency has been examined in a case study of childhood-onset schizophrenia who had persistent self-awareness impairments along with resistant AVH (Jardri et al., 2009). Based on abnormal activation in the right inferior parietal lobule (IPL) and related self-awareness network during self-agency related tasks (collision paradigm for motor-agency, block design experiment for speech awareness, and two scales for source monitoring), the patient was administered 10 days of 1Hz rTMS to the right TPJ. There was an improvement in the performance of self-other discrimination tasks associated with increased activity in the right IPL. However, there was no improvement in AVH until the patient was also administered a course of 1Hz rTMS to left TPJ, suggesting a functional dissociation between self-agency and hallucinations related networks.
Discussion
The studies reviewed here illustrate the variety of concurrent TMS/fMRI experiments that have been conducted in patients with schizophrenia. These include isolated case reports, open-label and randomized control trials. The studies exploring treatment have assessed for effects of rTMS on hallucinations, negative symptoms, neurocognition, social cognition, and agency, while the studies exploring pathophysiology have in general looked at altered cortical excitability or connectivity in schizophrenia as compared to healthy controls.
Studies Exploring Pathophysiology
The findings of multimodal studies evaluating cortical reactivity are different from that of prior research. While the study by Lindberg et al. (2016) did demonstrate a task-related deficiency in SICI during motor inhibition, this was associated with increased motor inhibition-related processing in the prefrontal and premotor areas. Previous studies have, in general, shown decreased prefrontal activation response inhibition tasks in schizophrenia patients (Kaladjian et al., 2007; Hughes et al., 2012).
The experiments on cortical connectivity demonstrated impaired effective connectivity between the thalamus and insula and thalamus and superior frontal gyrus, thereby implicating thalamic abnormalities in the pathogenesis of schizophrenia (Guller et al., 2012a,b). This is in line with previous neuropathological and neuroimaging research that has demonstrated thalamic dysfunction in schizophrenia (Clinton and Meador-Woodruff, 2004; Harms et al., 2007). An important point worth mentioning here is that while functional connectivity primarily provides an index of coactivation of two or more brain regions, it does not give any information as to the causal or primary contribution of one area over the other. In contrast, TMS-fMRI can help to infer causal influences of one brain region over the other via effective connectivity (Friston, 2011). This could help understand the heterogeneity from rs-fMRI studies by creating a better characterization of intra and inter-individual variability, thus paving the way for a more tailor-made or personalized approach toward treatment using rTMS. Findings of altered prefrontal interhemispheric connectivity in the study by Weber et al. (Webler et al., 2020) parallel those in previous TMS studies on motor cortex, which have demonstrated transcallosal inhibition abnormalities in patients of schizophrenia (Boroojerdi et al., 1999; Fitzgerald et al., 2002). Previous structural neuroimaging studies have also pointed toward corpus callosum impairments in schizophrenia (Foong et al., 2000; Keshavan et al., 2002).
Left TPJ is probably the commonest targeted area in treating persistent AVH using various non-invasive brain stimulation modalities. While other areas such as the inferior frontal gyrus (IFG; Broca’s area or its right homologous region) might be considered as potential targets for treating AVH based on the activation patterns during AVH, the exploratory study by Hoffman et al. (2007) demonstrated no improvement in delivering rTMS to these areas and also underscored the importance of left-sided TPJ stimulation for treatment of AVH.
An important point worth mentioning here is that a variety of psychotropics, including antidepressants, antipsychotics, mood stabilizers and benzodiazepines can have an effect on TMS measures of cortical excitability (Ziemann et al., 2015). Antiepileptic mood stabilizers are known cause an increase in values in RMT while BZDs are known to increase SICI. Antipsychotics such as haloperidol have also been noted to decrease SICI. Similarly, concurrent administration of psychotropics can also have effects on TMS measures of cortical plasticity. Antipsychotics like Haloperidol and Sulpiride have been shown to suppress plasticity induced by various NIBS methods. Mood stabilizers such as lamotrigine can reduce LTP-like plasticity. Whereas, SSRIs such as citalopram have also been shown to promote LTP-like plasticity and abolish LTD-like plasticity. These effects have in general been shown to persist and normalize only after withdrawal of the drug. While conducting studies that explore pathophysiology of schizophrenia, there are obvious difficulties in recruiting patients who are unmedicated and in acute phase of psychosis. It is not surprising that subjects in all studies in the current review barring one (Webler et al., 2020) were on antipsychotics at the time of assessment. Some of these studies excluded those on mood stabilizers and benzodiazepines at the time of assessment, while others had subjects who were on stable doses of benzodiazepines, mood stabilizers and antidepressants at the time of assessment. When compared to health controls who are essentially drug free, it is expected that the findings related to cortical excitability and plasticity will be altered to an extent by concurrent administration of psychotropics.
Studies Exploring Treatment
Functional magnetic resonance imaging-based target localization for rTMS offers a promising approach toward providing personalized therapy for various symptom domains of schizophrenia. However, current research has not proved unequivocally whether this approach is superior to the conventional 10/20 EEG based system. Most studies have been conducted on patients with medication-resistant symptoms and are plagued by methodological concerns stemming from small sample sizes and shorter duration of treatment/number of pulses. Moreover, the cost-effectiveness of such a treatment in clinical settings in terms of time, money, and workforce also needs to be considered.
There are also particular concerns with regards to fMRI-based neuronavigated rTMS, which merit a mention. Target localization for AVH in current multimodal studies has been performed using event related fMRI or block design fMRI, both of which are types of task-based fMRI. The main caveat with task-based fMRI is that the demonstration of functional connectivity between two regions does not imply a causal relationship or even a direct connection between the said regions. Furthermore, block design paradigm of fMRI for AVH is based on the concept that areas related to language processing are the same ones involved in the pathogenesis of AVH, which need not be necessary. Similarly, targets determined using both block-based and event-related fMRI can include structures that are deeper and even inaccessible to TMS. Target localization for negative symptoms has been performed using seed based rsfMRI, which at best can provide only indirect measurements of neural activity. In comparison to this, using concurrent TMS/fMRI might help in a more efficient localization of target by allowing to observe immediate response to local perturbation and also provide direct proof of target engagement (Windischberger, 2019; Bergmann et al., 2021).
Furthermore, considering that psychiatric illnesses involve abnormalities in complex neural networks, it seems too simplistic and reductionistic to expect that stimulation or inhibition of a single area will improve symptoms. Recent research in obsessive-compulsive disorder and depression has shown that a deeper and broader area of stimulation targeting subcortical regions using deep TMS may be a better alternative to the focal cortical stimulation using the F8 coil (Tendler et al., 2016; Lusicic et al., 2018). It has been suggested that deep-TMS might be more helpful due to targeting more widespread networks, thus questioning the need for functional imaging (Tendler et al., 2016).
The utility of rTMS is limited by its depth of penetration, making it possible to target only superficial cortical structures. However, combining fMRI with TMS also enables us to examine the effects of stimulating superficial cortical structures on deeper connections. This has been utilized in studies in healthy subjects to understand various aspects of brain physiology. For example, Zito et al. (2020) found the network-related sense of agency in healthy subjects to be amenable to inhibition by low-frequency rTMS. Another study by Hermiller et al. (2020) attempted theta burst stimulation to the hippocampal network targeted location in the parietal cortex during concurrent fMRI while performing a memory task and demonstrated increased activity of the targeted hippocampus during scene encoding and subsequently increased recollection. Such insights obtained from studies in healthy subjects could help understand physiological mechanisms and plan future experiments in patients with schizophrenia.
The current review has certain limitations. The main objective of this review was to discuss concurrent TMS/fMRI studies in schizophrenia. Hence, studies in normal healthy individuals that have investigated physiological mechanisms that might be aberrant in schizophrenia (for example, potential pathways for AVH, sense of agency) were excluded from this study. Also, this review has only examined studies published in the English language and may have missed out studies published in non-English languages. Most studies that have been reviewed here have not utilized interleaved TMS/fMRI, possibly due to the aforementioned technical and methodological challenges. Future studies using TMS/fMRI will require further optimization of these challenges while also using proper sham conditions to improve the quality of the studies. Correspondingly, further technical refinements in the entire process of concurrent TMS/fMRI are necessary so that these can be easily replicated across different centers. In conclusion, there is a definitive role of experiments combining TMS and fMRI in schizophrenia. Larger and adequately powered multicentric trials employing combined TMS/fMRI are needed to get consistent and reliable results. Such multimodal techniques appear to be a promising approach in elucidating the pathophysiology of schizophrenia and could also open up a possibility toward the development of a personalized approach toward treatment of its debilitating symptoms.
Author Contributions
SB and UM performed the literature review. SB prepared the first draft. UM supervised and SB edited the manuscript. Both authors contributed to the article and approved the submitted version.
Conflict of Interest
The authors declare that the research was conducted in the absence of any commercial or financial relationships that could be construed as a potential conflict of interest.
Publisher’s Note
All claims expressed in this article are solely those of the authors and do not necessarily represent those of their affiliated organizations, or those of the publisher, the editors and the reviewers. Any product that may be evaluated in this article, or claim that may be made by its manufacturer, is not guaranteed or endorsed by the publisher.
Supplementary Material
The Supplementary Material for this article can be found online at: https://www.frontiersin.org/articles/10.3389/fnhum.2021.662976/full#supplementary-material
Footnotes
References
Bais, L., Liemburg, E., Vercammen, A., Bruggeman, R., Knegtering, H., and Aleman, A. (2017). Effects of low frequency rTMS treatment on brain networks for inner speech in patients with schizophrenia and auditory verbal hallucinations. Prog. Neuro-Psychopharmacol. Biol. Psychiatry 78, 105–113. doi: 10.1016/j.pnpbp.2017.04.017
Basavaraju, R., Ithal, D., Thanki, M., Hr, A., Thirthalli, J., Pascual-Leone, A., et al. (2019). T79. INTERMITTENT THETA BURST STIMULATION OF CEREBELLAR VERMIS IN SCHIZOPHRENIA: IMPACT ON NEGATIVE SYMPTOMS AND BRAIN CONNECTIVITY. Schizophr. Bull. 45:S234. doi: 10.1093/schbul/sbz019.359
Bergmann, T. O., Varatheeswaran, R., Hanlon, C. A., Madsen, K. H., Thielscher, A., and Siebner, H. R. (2021). Concurrent TMS-fMRI for causal network perturbation and proof of target engagement. NeuroImage 237:118093. doi: 10.1016/j.neuroimage.2021.118093
Bestmann, S., Baudewig, J., Siebner, H. R., Rothwell, J. C., and Frahm, J. (2003). Subthreshold high-frequency TMS of human primary motor cortex modulates interconnected frontal motor areas as detected by interleaved fMRI-TMS. NeuroImage 20, 1685–1696. doi: 10.1016/j.neuroimage.2003.07.028
Bolognini, N., and Ro, T. (2010). Transcranial Magnetic Stimulation: Disrupting Neural Activity to Alter and Assess Brain Function. J. Neurosci. 30, 9647–9650. doi: 10.1523/JNEUROSCI.1990-10.2010
Boroojerdi, B., Töpper, R., Foltys, H., and Meincke, U. (1999). Transcallosal inhibition and motor conduction studies in patients with schizophrenia using transcranial magnetic stimulation. Br. J. Psychiatry 175, 375–379. doi: 10.1192/bjp.175.4.375
Brady, R. O., Gonsalvez, I., Lee, I., Öngür, D., Seidman, L. J., Schmahmann, J. D., et al. (2019). Cerebellar-Prefrontal Network Connectivity and Negative Symptoms in Schizophrenia. Am. J. Psychiat. 176, 512–520. doi: 10.1176/appi.ajp.2018.18040429
Briend, F., Leroux, E., Delcroix, N., Razafimandimby, A., Etard, O., and Dollfus, S. (2017). Impact of rTMS on functional connectivity within the language network in schizophrenia patients with auditory hallucinations. Schizoph. Res. 189, 142–145. doi: 10.1016/j.schres.2017.01.049
Bungert, A., Chambers, C. D., Long, E., and Evans, C. J. (2012a). On the importance of specialized radiofrequency filtering for concurrent TMS/MRI. J. Neurosci. Methods 210, 202–205. doi: 10.1016/j.jneumeth.2012.07.023
Bungert, A., Chambers, C. D., Phillips, M., and Evans, C. J. (2012b). Reducing image artefacts in concurrent TMS/fMRI by passive shimming. NeuroImage 59, 2167–2174. doi: 10.1016/j.neuroimage.2011.10.013
Clinton, S. M., and Meador-Woodruff, J. H. (2004). Thalamic dysfunction in schizophrenia: neurochemical, neuropathological, and in vivo imaging abnormalities. Schizophrenia Res. 69, 237–253. doi: 10.1016/j.schres.2003.09.017
de Araújo, A. N., de Sena, E. P., de Oliveira, I. R., and Juruena, M. F. (2012). Antipsychotic agents: efficacy and safety in schizophrenia. Drug Healthc. Patient Saf. 4, 173–180. doi: 10.2147/DHPS.S37429
de Weijer, A. D., Sommer, I. E. C., Lotte Meijering, A., Bloemendaal, M., Neggers, S. F. W., Daalman, K., et al. (2014). High frequency rTMS; a more effective treatment for auditory verbal hallucinations? Psychiat. Res. 224, 204–210. doi: 10.1016/j.pscychresns.2014.10.007
Dlabac-de Lange, J. J., Liemburg, E. J., Bais, L., Renken, R. J., Knegtering, H., and Aleman, A. (2015). Effect of rTMS on brain activation in schizophrenia with negative symptoms: A proof-of-principle study. Schizophrenia Res. 168, 475–482. doi: 10.1016/j.schres.2015.06.018
Du, X., Choa, F.-S., Chiappelli, J., Wisner, K. M., Wittenberg, G., Adhikari, B., et al. (2019). Aberrant Middle Prefrontal-Motor Cortex Connectivity Mediates Motor Inhibitory Biomarker in Schizophrenia. Biol. Psychiatry 85, 49–59. doi: 10.1016/j.biopsych.2018.06.007
Fitzgerald, P. B., Brown, T. L., Daskalakis, Z. J., deCastella, A., and Kulkarni, J. A. (2002). study of transcallosal inhibition in schizophrenia using transcranial magnetic stimulation. Schizophrenia Res. 56, 199–209. doi: 10.1016/S0920-9964(01)00222-5
Fitzgerald, P. B., Sritharan, A., Benitez, J., Daskalakis, Z. J., Jackson, G., Kulkarni, J., et al. (2007). preliminary fMRI study of the effects on cortical activation of the treatment of refractory auditory hallucinations with rTMS. Psychiatry Res. 155, 83–88. doi: 10.1016/j.pscychresns.2006.12.011
Foong, J., Maier, M., Clark, C. A., Barker, G. J., Miller, D. H., and Ron, M. A. (2000). Neuropathological abnormalities of the corpus callosum in schizophrenia: a diffusion tensor imaging study. J. Neurol. Neurosurg. Psychiatry 68, 242–244. doi: 10.1136/jnnp.68.2.242
Friston, K. J. (2011). Functional and Effective Connectivity: A Review. Brain Connectiv. 1, 13–36. doi: 10.1089/brain.2011.0008
Gavrilescu, M., Rossell, S., Stuart, G. W., Shea, T. L., Innes-Brown, H., Henshall, K., et al. (2010). Reduced connectivity of the auditory cortex in patients with auditory hallucinations: a resting state functional magnetic resonance imaging study. Psychol. Med. 40, 1149–1158. doi: 10.1017/S0033291709991632
Giesel, F. L., Mehndiratta, A., Hempel, A., Hempel, E., Kress, K. R., Essig, M., et al. (2012). Improvement of auditory hallucinations and reduction of primary auditory area’s activation following TMS. Eur. J. Radiol. 81, 1273–1275. doi: 10.1016/j.ejrad.2011.03.002
Guller, Y., Ferrarelli, F., Shackman, A. J., Sarasso, S., Peterson, M. J., Langheim, F. J., et al. (2012a). Probing thalamic integrity in schizophrenia using concurrent transcranial magnetic stimulation and functional magnetic resonance imaging. Archi. Gener. Psychiatry 69, 662–671. doi: 10.1001/archgenpsychiatry.2012.23
Guller, Y., Tononi, G., and Postle, B. R. (2012b). Conserved functional connectivity but impaired effective connectivity of thalamocortical circuitry in schizophrenia. Brain Connectiv. 2, 311–319. doi: 10.1089/brain.2012.0100
Guse, B., Falkai, P., Gruber, O., Whalley, H., Gibson, L., Hasan, A., et al. (2013). The effect of long-term high frequency repetitive transcranial magnetic stimulation on working memory in schizophrenia and healthy controls—A randomized placebo-controlled, double-blind fMRI study. Behav. Brain Res. 237, 300–307. doi: 10.1016/j.bbr.2012.09.034
Harms, M. P., Wang, L., Mamah, D., Barch, D. M., Thompson, P. A., and Csernansky, J. G. (2007). Thalamic Shape Abnormalities in Individuals with Schizophrenia and Their Nonpsychotic Siblings. J. Neurosci. 27, 13835–13842. doi: 10.1523/JNEUROSCI.2571-07.2007
Hermiller, M. S., Chen, Y. F., Parrish, T. B., and Voss, J. L. (2020). Evidence for Immediate Enhancement of Hippocampal Memory Encoding by Network-Targeted Theta-Burst Stimulation during Concurrent fMRI. J. Neurosci. 40, 7155–7168. doi: 10.1523/JNEUROSCI.0486-20.2020
Hoffman, R. E., Hampson, M., Wu, K., Anderson, A. W., Gore, J. C., Buchanan, R. J., et al. (2007). Probing the Pathophysiology of Auditory/Verbal Hallucinations by Combining Functional Magnetic Resonance Imaging and Transcranial Magnetic Stimulation. Cereb. Cortex 17, 2733–2743. doi: 10.1093/cercor/bhl183
Hoffman, R. E., Rapaport, J., Mazure, C. M., and Quinlan, D. M. (1999). Selective speech perception alterations in schizophrenic patients reporting hallucinated “voices.”. Am. J. Psychiatry 156, 393–399. doi: 10.1176/ajp.156.3.393
Homan, P., Kindler, J., Hauf, M., Hubl, D., and Dierks, T. (2012). Cerebral blood flow identifies responders to transcranial magnetic stimulation in auditory verbal hallucinations. Transl. Psychiatry 2:e189. doi: 10.1038/tp.2012.114
Hughes, M. E., Fulham, W. R., Johnston, P. J., and Michie, P. T. (2012). Stop-signal response inhibition in schizophrenia: Behavioural, event-related potential and functional neuroimaging data. Biol. Psychol. 89, 220–231. doi: 10.1016/j.biopsycho.2011.10.013
Jardri, R., Delevoye-Turrell, Y., Lucas, B., Pins, D., Bulot, V., Delmaire, C., et al. (2009). Clinical practice of rTMS reveals a functional dissociation between agency and hallucinations in schizophrenia. Neuropsychologia 47, 132–138. doi: 10.1016/j.neuropsychologia.2008.08.006
Jardri, R., Lucas, B., Delevoye-Turrell, Y., Delmaire, C., Delion, P., Thomas, P., et al. (2007). An 11-year-old boy with drug-resistant schizophrenia treated with temporo-parietal rTMS. Mol. Psychiatry 12:320. doi: 10.1038/sj.mp.4001968
Jardri, R., Pins, D., and Thomas, P. A. (2008). case of fMRI-guided rTMS treatment of coenesthetic hallucinations. Am. J. Psychiatry 165, 1490–1491. doi: 10.1176/appi.ajp.2008.08040504
Kaladjian, A., Jeanningros, R., Azorin, J.-M., Grimault, S., Anton, J.-L., and Mazzola-Pomietto, P. (2007). Blunted activation in right ventrolateral prefrontal cortex during motor response inhibition in schizophrenia. Schizophrenia Res. 97, 184–193. doi: 10.1016/j.schres.2007.07.033
Keshavan, M. S., Diwadkar, V. A., Harenski, K., Rosenberg, D. R., Sweeney, J. A., and Pettegrew, J. W. (2002). Abnormalities of the corpus callosum in first episode, treatment naive schizophrenia. J. Neurol. Neurosurg. Psychiatry 72, 757–760. doi: 10.1136/jnnp.72.6.757
Kindler, J., Homan, P., Jann, K., Federspiel, A., Flury, R., Hauf, M., et al. (2013). Reduced neuronal activity in language-related regions after transcranial magnetic stimulation therapy for auditory verbal hallucinations. Biol. Psychiatry 73, 518–524. doi: 10.1016/j.biopsych.2012.06.019
Kujirai, T., Caramia, M. D., Rothwell, J. C., Day, B. L., Thompson, P. D., Ferbert, A., et al. (1993). Corticocortical inhibition in human motor cortex. J. Physiol. 471, 501–519. doi: 10.1113/jphysiol.1993.sp019912
Li, S., Hu, N., Zhang, W., Tao, B., Dai, J., Gong, Y., et al. (2019). Dysconnectivity of Multiple Brain Networks in Schizophrenia: A Meta-Analysis of Resting-State Functional Connectivity. Front. Psychiatry 10:00482. doi: 10.3389/fpsyt.2019.00482
Li, X., Honda, S., Nakajima, S., Wada, M., Yoshida, K., Daskalakis, Z. J., et al. (2021). Research to Elucidate the Pathophysiological Neural Bases in Patients with Schizophrenia: A Systematic Review. J. Personalized Med. 11:388. doi: 10.3390/jpm11050388
Liemburg, E. J., Dlabac-De Lange, J. J., Bais, L., Knegtering, H., and Aleman, A. (2018). Effects of bilateral prefrontal rTMS on brain activation during social-emotional evaluation in schizophrenia: A double-blind, randomized, exploratory study. Schizophrenia Res. 202, 210–211. doi: 10.1016/j.schres.2018.06.051
Lindberg, P. G., Térémetz, M., Charron, S., Kebir, O., Saby, A., Bendjemaa, N., et al. (2016). Altered cortical processing of motor inhibition in schizophrenia. Cortex J. Devoted Study Nerv. Syst. Behav. 85, 1–12. doi: 10.1016/j.cortex.2016.09.019
Lusicic, A., Schruers, K. R., Pallanti, S., and Castle, D. J. (2018). Transcranial magnetic stimulation in the treatment of obsessive-compulsive disorder: current perspectives. Neuropsychiatr. Dis. Treat. 14, 1721–1736. doi: 10.2147/NDT.S121140
Maïza, O., Hervé, P.-Y., Etard, O., Razafimandimby, A., Montagne-Larmurier, A., and Dollfus, S. (2013). Impact of repetitive transcranial magnetic stimulation (rTMS) on brain functional marker of auditory hallucinations in schizophrenia patients. Brain Sci. 3, 728–743. doi: 10.3390/brainsci3020728
Maran, M., Grent-‘t-Jong, T., and Uhlhaas, P. J. (2016). Electrophysiological insights into connectivity anomalies in schizophrenia: a systematic review. Neuropsychiatr. Electrophysiol. 2:6. doi: 10.1186/s40810-016-0020-5
Mehta, U. M., Naik, S. S., Thanki, M. V., and Thirthalli, J. (2019). Investigational and Therapeutic Applications of Transcranial Magnetic Stimulation in Schizophrenia. Curr. Psychiatry Rep. 21:89. doi: 10.1007/s11920-019-1076-2
Moisa, M., Pohmann, R., Ewald, L., and Thielscher, A. (2009). New coil positioning method for interleaved transcranial magnetic stimulation (TMS)/functional MRI (fMRI) and its validation in a motor cortex study. J. Magn. Reson. Imaging 29, 189–197. doi: 10.1002/jmri.21611
Montagne-Larmurier, A., Etard, O., Razafimandimby, A., Morello, R., and Dollfus, S. (2009). Two-day treatment of auditory hallucinations by high frequency rTMS guided by cerebral imaging: a 6 month follow-up pilot study. Schizoph. Res. 113, 77–83. doi: 10.1016/j.schres.2009.05.006
Navarro de Lara, L. I., Tik, M., Woletz, M., Frass-Kriegl, R., Moser, E., Laistler, E., et al. (2017). High-sensitivity TMS/fMRI of the Human Motor Cortex Using a Dedicated Multichannel MR Coil. NeuroImage 150, 262–269. doi: 10.1016/j.neuroimage.2017.02.062
Navarro de Lara, L. I., Windischberger, C., Kuehne, A., Woletz, M., Sieg, J., Bestmann, S., et al. (2015). A novel coil array for combined TMS/fMRI experiments at 3 T. Magn. Reson. Med. 74, 1492–1501. doi: 10.1002/mrm.25535
Oertel-Knöchel, V., Knöchel, C., Matura, S., Stäblein, M., Prvulovic, D., Maurer, K., et al. (2014). Association between symptoms of psychosis and reduced functional connectivity of auditory cortex. Schizophrenia Res. 160, 35–42. doi: 10.1016/j.schres.2014.10.036
Oh, H., Kim, J. H., and Yau, J. M. E. P. I. (2019). distortion correction for concurrent human brain stimulation and imaging at 3T. J. Neurosci. Methods 327:108400. doi: 10.1016/j.jneumeth.2019.108400
Paillère-Martinot, M.-L., Galinowski, A., Plaze, M., Andoh, J., Bartrés-Faz, D., Bellivier, F., et al. (2017). Active and placebo transcranial magnetic stimulation effects on external and internal auditory hallucinations of schizophrenia. Acta Psychiatrica Scand. 135, 228–238. doi: 10.1111/acps.12680
Pascual-Leone, A., Walsh, V., and Rothwell, J. (2000). Transcranial magnetic stimulation in cognitive neuroscience–virtual lesion, chronometry, and functional connectivity. Curr. Opin. Neurobiol. 10, 232–237. doi: 10.1016/s0959-4388(00)00081-7
Polanía, R., Nitsche, M. A., and Ruff, C. C. (2018). Studying and modifying brain function with non-invasive brain stimulation. Nat. Neurosci. 21, 174–187. doi: 10.1038/s41593-017-0054-4
Prikryl, R., Mikl, M., Prikrylova Kucerová, H., Ustohal, L., Kasparek, T., Marecek, R., et al. (2012). Does repetitive transcranial magnetic stimulation have a positive effect on working memory and neuronal activation in treatment of negative symptoms of schizophrenia? Neuro Endocrinol. Lett. 33, 90–97.
Radhu, N., de Jesus, D. R., Ravindran, L. N., Zanjani, A., Fitzgerald, P. B., and Daskalakis, Z. J. A. (2013). meta-analysis of cortical inhibition and excitability using transcranial magnetic stimulation in psychiatric disorders. Clin. Neurophysiol. 124, 1309–1320. doi: 10.1016/j.clinph.2013.01.014
Schönfeldt-Lecuona, C., Grön, G., Walter, H., Büchler, N., Wunderlich, A., Spitzer, M., et al. (2004). Stereotaxic rTMS for the treatment of auditory hallucinations in schizophrenia. Neuroreport 15, 1669–1673. doi: 10.1097/01.wnr.0000126504.89983.ec
Siebner, H. R., Bergmann, T. O., Bestmann, S., Massimini, M., Johansen-Berg, H., Mochizuki, H., et al. (2009). Consensus paper: combining transcranial stimulation with neuroimaging. Brain Stimul. 2, 58–80. doi: 10.1016/j.brs.2008.11.002
Slotema, C. W., Blom, J. D., de Weijer, A. D., Diederen, K. M., Goekoop, R., Looijestijn, J., et al. (2011). Can low-frequency repetitive transcranial magnetic stimulation really relieve medication-resistant auditory verbal hallucinations? Negative results from a large randomized controlled trial. Biol. Psychiatry 69, 450–456. doi: 10.1016/j.biopsych.2010.09.051
Sommer, I. E. C., de Weijer, A. D., Daalman, K., Neggers, S. F., Somers, M., Kahn, R. S., et al. (2007). Can fMRI-guidance improve the efficacy of rTMS treatment for auditory verbal hallucinations? Schizophrenia Res. 93, 406–408. doi: 10.1016/j.schres.2007.03.020
Tendler, A., Barnea Ygael, N., Roth, Y., and Zangen, A. (2016). Deep transcranial magnetic stimulation (dTMS) – beyond depression. Expert Rev. Med. Dev. 13, 987–1000. doi: 10.1080/17434440.2016.1233812
Vercammen, A., Knegtering, H., Liemburg, E. J., den Boer, J. A., and Aleman, A. (2010). Functional connectivity of the temporo-parietal region in schizophrenia: effects of rTMS treatment of auditory hallucinations. J. Psychiatric Res. 44, 725–731. doi: 10.1016/j.jpsychires.2009.12.011
Vink, J. J. T., Mandija, S., Petrov, P. I., van den Berg, C. A. T., Sommer, I. E. C., and Neggers, S. F. W. A. (2018). novel concurrent TMS−fMRI method to reveal propagation patterns of prefrontal magnetic brain stimulation. Hum. Brain Mapp. 39, 4580–4592. doi: 10.1002/hbm.24307
Vittala, A., Murphy, N., Maheshwari, A., and Krishnan, V. (2020). Understanding Cortical Dysfunction in Schizophrenia With TMS/EEG. Front. Neurosci. 14:00554. doi: 10.3389/fnins.2020.00554
Wagner, T., Valero-Cabre, A., and Pascual-Leone, A. (2007). Noninvasive human brain stimulation. Annu. Rev. Biomed. Eng. 9, 527–565. doi: 10.1146/annurev.bioeng.9.061206.133100
Wang, W.-T., Xu, B., and Butman, J. A. (2017). Improved SNR for combined TMS-fMRI: A support device for commercially available body array coil. J. Neurosci. Methods 289, 1–7. doi: 10.1016/j.jneumeth.2017.06.020
Webler, R. D., Hamady, C., Molnar, C., Johnson, K., Bonilha, L., Anderson, B. S., et al. (2020). Decreased interhemispheric connectivity and increased cortical excitability in unmedicated schizophrenia: A prefrontal interleaved TMS fMRI study. Brain Stimulat. 13, 1467–1475. doi: 10.1016/j.brs.2020.06.017
Weiskopf, N., Josephs, O., Ruff, C. C., Blankenburg, F., Featherstone, E., Thomas, A., et al. (2009). Image artifacts in concurrent transcranial magnetic stimulation (TMS) and fMRI caused by leakage currents: Modeling and compensation. J. Magn. Reson. Imaging 29, 1211–1217. doi: 10.1002/jmri.21749
Windischberger, C. (2019). Verifying successful brain stimulation by concurrent TMS/fMRI. Brain Stimulat. Translat. Clin. Res. Neuromodulat. 12:502. doi: 10.1016/j.brs.2018.12.643
Ziemann, U., Reis, J., Schwenkreis, P., Rosanova, M., Strafella, A., Badawy, R., et al. (2015). TMS and drugs revisited 2014. Clin. Neurophysiol. 126, 1847–1868. doi: 10.1016/j.clinph.2014.08.028
Zito, G. A., Anderegg, L. B., Apazoglou, K., Müri, R. M., Wiest, R., Holtforth, M., et al. (2020). Transcranial magnetic stimulation over the right temporoparietal junction influences the sense of agency in healthy humans. JPN 2020, 271–278. doi: 10.1503/jpn.190099
Zöllner, R., Hübener, A.-F., Dannlowski, U., Kircher, T., Sommer, J., and Zavorotnyy, M. (2020). Theta-burst stimulation for auditory-verbal hallucination in very-late-onset schizophrenia-like psychosis-a functional magnetic resonance imaging case study. Front. Psychiatry 11:294. doi: 10.3389/fpsyt.2020.00294
Keywords: brain connectivity, concurrent TMS/fMRI, simultaneous TMS-fMRI, psychosis, neuroplasticity, treatment resistance, causal inferences
Citation: Baliga SP and Mehta UM (2021) A Review of Studies Leveraging Multimodal TMS-fMRI Applications in the Pathophysiology and Treatment of Schizophrenia. Front. Hum. Neurosci. 15:662976. doi: 10.3389/fnhum.2021.662976
Received: 02 February 2021; Accepted: 21 June 2021;
Published: 02 August 2021.
Edited by:
Nivethida Thirugnanasambandam, National Brain Research Centre (NBRC), IndiaReviewed by:
Andrea Guerra, Sapienza University of Rome, ItalyHyuntaek Oh, Baylor College of Medicine, United States
Copyright © 2021 Baliga and Mehta. This is an open-access article distributed under the terms of the Creative Commons Attribution License (CC BY). The use, distribution or reproduction in other forums is permitted, provided the original author(s) and the copyright owner(s) are credited and that the original publication in this journal is cited, in accordance with accepted academic practice. No use, distribution or reproduction is permitted which does not comply with these terms.
*Correspondence: Urvakhsh Meherwan Mehta, dXJ2YWtoc2hAZ21haWwuY29t