- 1College of Marine Life Sciences, Ocean University of China, Qingdao, China
- 2Shandong Key Laboratory of Behavioral Medicine, School of Mental Health, Jining Medical University, Jining, China
- 3Shandong Collaborative Innovation Center for Diagnosis, Treatment & Behavioral Interventions of Mental Disorders, Institute of Mental Health, Jining Medical University, Jining, China
- 4Center of Evidence-Based Medicine, Jining Medical University, Jining, China
- 5Department of Neurology, Affiliated Hospital of Jining Medical University, Jining Medical University, Jining, China
Alzheimer's disease (AD) is the most common type of neurodegenerative disease leading to dementia in the elderly. Increasing evidence indicates that folate plays an important role in the pathogenesis of AD. To investigate the role of folate deficiency/possible deficiency in the risk of AD and the benefical effect of sufficient folate intake on the prevention of AD, a systematic review and meta-analysis were performed. The Web of Science, PubMed, CENTRAL, EBSCO, CNKI, CQVIP, and Wanfang databases were searched. The analysis of cross-sectional studies showed that the standardized mean difference (SMD) was −0.60 (95% confidence interval (CI): −0.65, −0.55), indicating that plasma/serum folate level is lower in AD patients than that in controls. Moreover, the combined odds ratio (OR) of case-control studies was 0.96 (95% CI: 0.93, 0.99), while the combined ORs were 0.86 (95% CI: 0.46, 1.26) and 1.94 (95% CI: 1.02, 2.86) in populations with normal levels of folate (≥13.5 nmol/L) and folate deficiency/possible deficiency (<13.5 nmol/L), respectively. In addition, the risk ratio (RR) of the cohort studies was 1.88 (95% CI: 1.20, 2.57) in populations with folate deficiency/possible deficiency. Furthermore, when the intake of folate was equal to or higher than the recommended daily allowance, the combined RR and hazard ratio (HR) were 0.44 (95% CI: 0.18, 0.71) and 0.76 (95% CI: 0.52, 0.99), respectively. These results indicate that folate deficiency/possible deficiency increases the risk for AD, while sufficient intake of folate is a protective factor against AD.
Introduction
Alzheimer's disease (AD) is the most common type of neurodegenerative disease leading to dementia in the elderly. A progressive memory loss and deterioration of other cognitive functions are the main clinical manifestations, while extraneuronal neuritic plaques, intraneuronal neurofibrillary tangles, and neuronal loss are the neuropathological hallmarks of AD (Hebert et al., 2013; Bakota and Brandt, 2016; Mantzavinos and Alexiou, 2017; Li et al., 2018). According to the age of onset, AD is classified into early-onset AD (EOAD) and late-onset AD (LOAD). Compared with EOAD (onset before age 65), LOAD (onset after age 65) accounts for 95% or more of AD cases (Alzheimer's Association, 2012). With the rapid increase of the aging population worldwide, over 50 million people were living with dementia globally in 2019 and the number is said to increase to 152 million by 2050 (Alzheimer's Disease International, 2019). The total cost for dementia was about 1 trillion US dollars in 2019 and it will be doubled by 2030 (Alzheimer's Disease International, 2019). AD accounts for 60–80% of dementia. Preventing or delaying the onset of AD is a priority as there is no effective treatment for AD.
Increasing evidence has indicated that dietary patterns and nutrition are implicated in the pathogenesis of AD (Otaegui-Arrazola et al., 2014). Thus, healthy diet and the balance of nutrients including vitamins are key factors in AD prevention. For example, marginal vitamin A deficiency promotes Aβ generation, the major component of neuritic plaques, and subsequent cognitive deficits (Zeng et al., 2017). Increasing evidence suggests that folate, an essential vitamin, plays an important role in AD development (Jheng et al., 2016; Tian et al., 2016; Robinson et al., 2018; Guo et al., 2019). The normal range of plasma/serum folate ranges from 13.5 nmol/L to 45.3 nmol/L. Folate deficiency and possible deficiency are defined when the level of plasma/serum folate is <6.8 nmol/L and 13.5 nmol/L, respectively (WHO, 2015). Previous studies showed that low folate level is not only associated with specific domains of cognitive functioning, e.g., episodic recall and recognition (Wahlin et al., 1996; Hassing et al., 1999; Nurk et al., 2005; De Lau et al., 2007), but also associated with all types of dementia, including vascular dementia and AD (Clarke et al., 1998; Ebly et al., 1998; Morris, 2003; Zhuo et al., 2011; Douaud et al., 2013; Cascalheira et al., 2015). In addition, folate/folic acid supplementation is beneficial to the improvement of cognitive functions in aged subjects and cases of mild cognitive impairment (Fioravanti et al., 1997; Morris, 2003; Durga et al., 2007; De Jager et al., 2012; Ma et al., 2019). Moreover, deprivation of folate increases tau phosphorylation, the major component of neurofibrillary tangles (Chan and Shea, 2006). However, there has not been a meta-analysis study to investigate the association between folate deficiency/possible deficiency and the risk of AD, as well as the benefical effect of sufficient folate intake on the prevention of AD.
This study aims to investigate the role of folate deficiency/possible deficiency in the risk of AD and the benefical effect of sufficient folate intake on the prevention of AD in addition to updating the association between plasma/serum folate levels and AD. We designed and performed this systematic review and meta-analysis to evaluate the difference of folate levels between AD patients and healthy controls, the association of folate deficiency/possible deficiency with AD risk, and the effect of sufficient folate intake on the prevention of AD.
Materials and Methods
Protocol and Registration
This systematic review and meta-analysis were conducted in accordance with the Meta-analysis Of Observational Studies in Epidemiology (MOOSE) (Stroup et al., 2000) statement published in 2009. The study protocol was developed before this review and was registered at PROSPERO with the registration number CRD42020173072.
Search Strategy and Selection Criteria
To find publications of the association between folate and AD, two authors independently performed a systematic literature search in four English databases (Web of Science, PubMed, CENTRAL, EBSCO), and three Chinese databases (CNKI, CQVIP, and Wanfangdata). Boolean search techniques were carried out in full text, i.e., (Folic acid OR Folate OR vitamin B9 OR Vitamin Bc OR pteroylglutamic acid OR R factor OR MTHFR OR methyltetrahydrofolate reductase) AND (dementia OR Alzheimer). In addition, the references of identified publications were also screened by two independent authors. Only studies on AD, not other types of dementia [e.g., vascular dementia (VAD)], were included for the systematic review and meta-analysis in this study although both “dementia” and “Alzheimer” were included in the search terms list in order to avoid missing any study related to AD. The searching process was completed on December 31, 2019.
Inclusion and Exclusion Criteria
The inclusion criteria were: (1) cross-sectional studies, case-control studies, or longitudinal studies with primary data collection; (2) studies conducted in the general population; (3) outcomes of individuals with AD were determined by clinicians' diagnosis according to the Diagnostic and Statistical Manual of Mental Disorders (DSM) criteria, the Consortium to Establish a Registry for Alzheimer's Disease (CERAD) criteria, the National Institute of Neurological and Communicative Disorders and Stroke and Alzheimer's Disease and Related Disorders Association (NINCDS-ADRDA) criteria or pathological criteria; (4) studies that provided sufficient statistical data to calculate the combined effect sizes; and (5) studies in any language and publication period.
The following types of studies were excluded: (1) studies with non-primary data such as opinion articles, editorials, letters to the editor, and comments; (2) animal studies; (3) qualitative studies; 4) dissertations; and (5) studies that could not provide sufficient statistical data.
Quality Evaluation and Data Extraction
The Newcastle-Ottawa Scale (NOS, scores range from 0 to 9) was used to assess the quality and risk of bias (Stang, 2010). Studies with a NOS score of 6 or more were included for the meta-analysis. All discrepancies were resolved by discussion with a third author.
Two authors independently extracted the following data from all articles: first author's name, publication year, country of survey, number of cases and controls, mean age of participants, the mean and standard deviation of folate or odds ratio (OR) or risk ratio (RR) or hazard ratio (HR) and their 95% confidence intervals (CI).
The standardized mean difference (SMD) is used as a summary statistic in meta-analysis when the studies all assess the same outcome, but measure it in a variety of ways. The SMD expresses the size of the intervention effect in each study relative to the between-participant variability in outcome measurements observed in that study (Cochrane Training, 2020). In the current study, SMD was used to calculate differences of mean folate levels between people who did and did not suffer from AD. SMD and corresponding 95% CIs of plasma/serum folate were calculated based on the sample size, mean, and SD. Median and range were also used to estimate mean and SD (Hozo et al., 2005). 95% CI was transformed from SD through the formula as follows: 95% CI = mean ± 1.96 SD. The 25th and 75th percentiles were transformed to SD through the following formula: SD = Norm IQR = (P75–P25) ×0.7413 (IQR: inter-quartile range; P75: 75th percentile; P25: 25th percentile). SMD <0 represents a folate level that is lower in the AD group compared with that in the control group.
Measures of relative effect express the expected outcome in one group relative to that in the other. RR is the ratio of the risk of an event in the two groups, whereas OR is the ratio of the odds of an event. For both measures a value of 1 indicates that the estimated effects are the same for both interventions, while a value <1 might indicate a beneficial effect of an experimental intervention. The most appropriate way of summarizing time-to-event data is to use methods of survival analysis and express the intervention effect as a HR. Hazard is similar in notion to risk, but is subtly different in that it measures instantaneous risk and may change continuously (Cochrane Training, 2020). In the current study, OR, RR, and HR were used to indicate the beneficial effect (<1) or detrimental effect (>1) of different folate levels and amount of folate intake.
Data Synthesis and Statistical Analysis
All statistical analyses were performed using Stata 15.0. The pooled effect size (SMD/OR/RR/HR) and its 95% CI were reported. A two-sided p <0.05 was considered statistically significant.
Different models were used based on heterogeneity tests. The heterogeneity of the included studies was evaluated using Higgins I2 test. The random-effect model was used if I2 >50%, which was marked in the forest plots. The fixed-effect model was used if I2 ≤ 50%, which was not marked in the forest plots.
Funnel plots and an Egger's test were used to investigate the potential publication bias. The Egger's test was only conducted when six or more studies were included.
Sensitivity Analysis
When the heterogeneity was high (I2 >50%), sensitivity analysis was conducted to evaluate the stability of the outcome. Sensitivity analysis was performed by excluding an individual study at one time. A two-sided p <0.05 was considered statistically significant. The random-effect model was used. A subgroup meta-analysis was further conducted for the combined index of AD based on the level of plasma/serum folate and the daily intake of folate.
Results
Characteristics of Included Studies
A total of 3,672 publications (including 3,370 in English and 302 in Chinese) related to AD in the general population were initially identified from the databases. Sixty-two publications were included in this systematic review and 59 of them were included for further meta-analysis (Figure 1). Fifty-six and three articles were published in English and Chinese, respectively. Forty publications only included cross-sectional studies and eight publications only included cohort studies, respectively. Ten publications included both cross-sectional and case-control studies, while one publication included both cross-sectional and cohort studies. The studies were conducted in European countries (33 publications), Asian countries (14 publications), American countries (10 publications), and Oceania countries (2 publications), respectively.
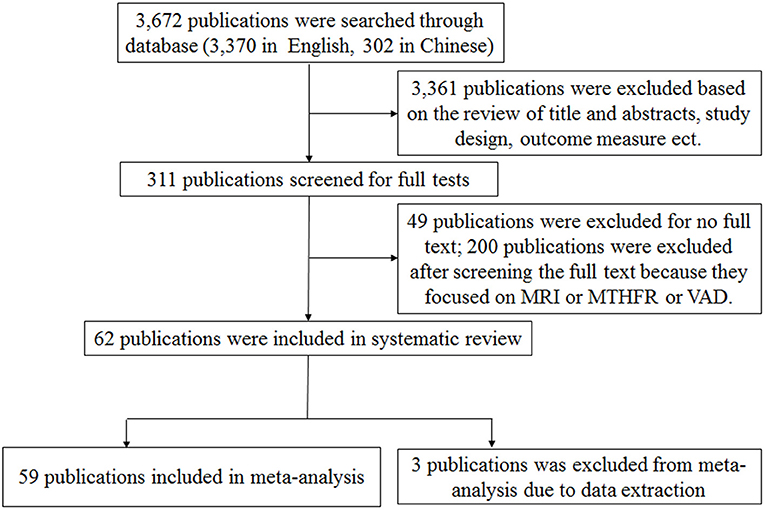
Figure 1. Flow diagram of the process used to breakdown the retrieved publications into publications suitable for meta-analysis.
Each subgroup of the analysis was considered as an independent study according to the methods previously used (Moazzen et al., 2018). In total, 52 cross-section studies, 14 case-control studies, and 16 cohort studies were included for meta-analysis. The sample size ranged from 24 to 965.
The Level of Plasma/Serum Folate Is Lower in AD Patients: Results From Cross-Sectional Studies
Fifty-two cross-sectional studies covering a total of 3,496 AD patients and 4,318 controls were included in the meta-analysis. The summary of these studies is shown in Table 1. The level of plasma/serum folate was lower in AD patients than that in the controls, with an SMD of −0.60 (95% CI: −0.65, −0.55) (Figure 2A).
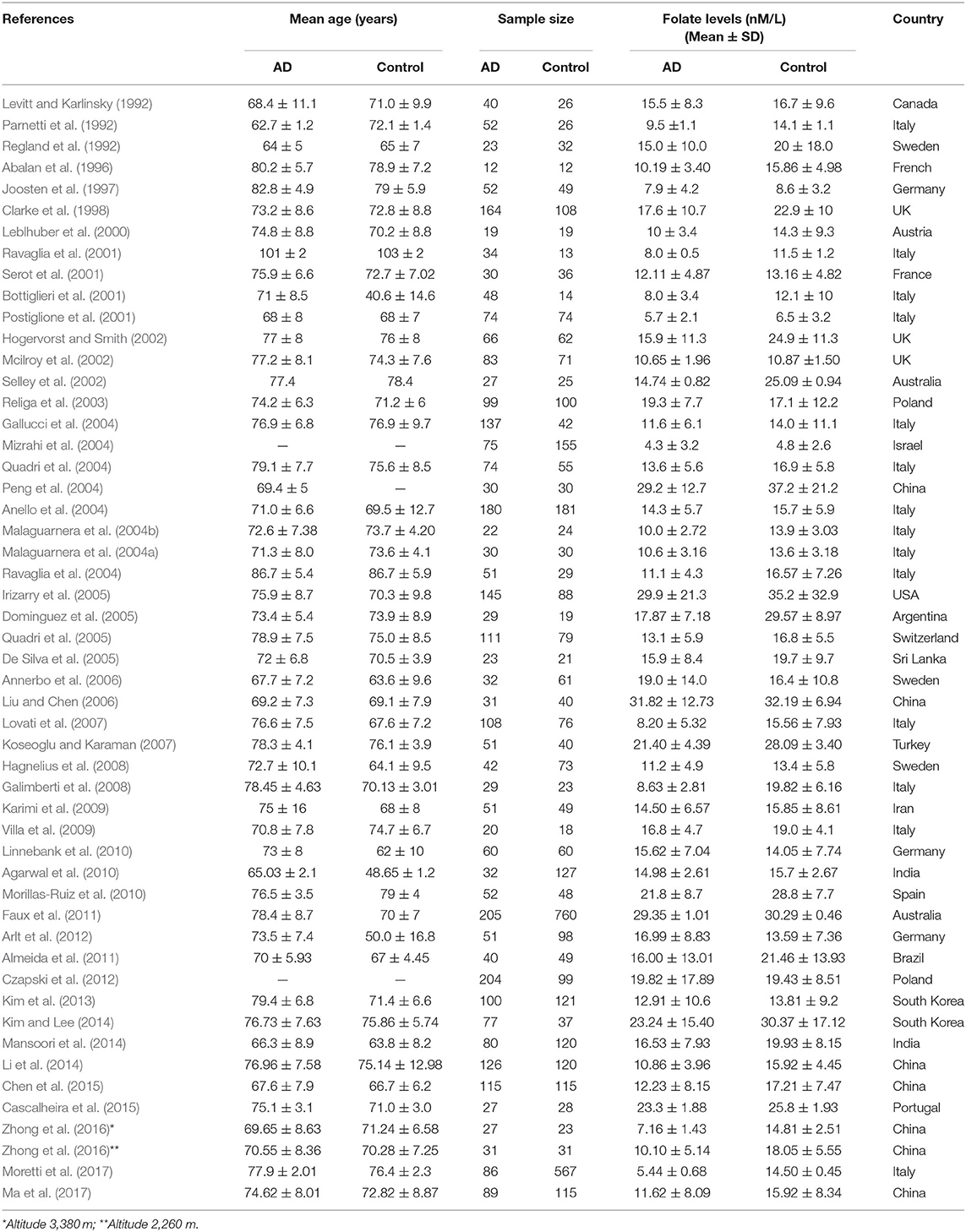
Table 1. Summary of studies of folate levels (nM/L) among AD patients and healthy controls in 52 cross-sectional studies.
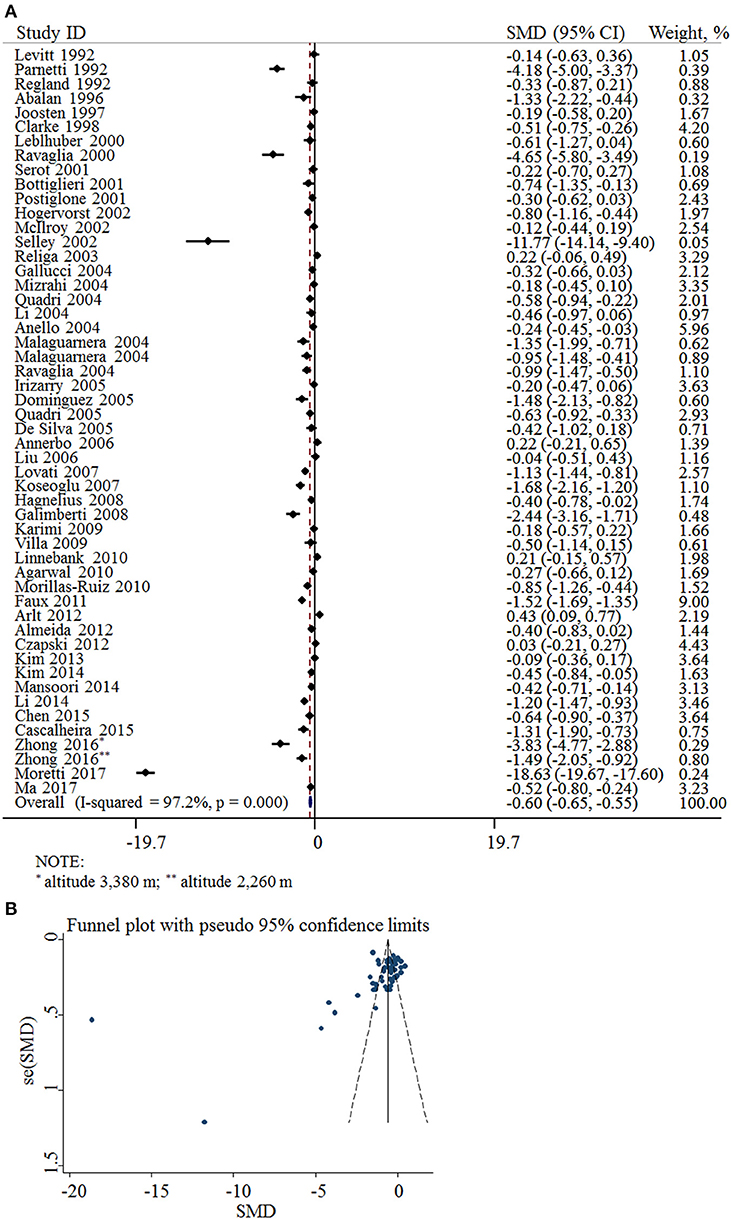
Figure 2. SMD analysis of the plasma/serum folate levels between AD and controls. (A) Pooled estimate of SMD and 95% CI of folate levels among AD patients and controls; (B) Funnel plot for publication bias of SMD.
The funnel plots appeared to be asymmetrical (Figure 2B). Moreover, Egger's test was performed. The P-value was 0.004, indicating there was potential publication bias across all included studies.
Folate Defiency/Possible Deficiency Is Associated With the Risk for AD: Results From Case-Control Studies
Fifteen case-control studies involving 971 AD patients and 1,059 controls were included in the meta-analysis (Table 2). The sample size ranged from 27 to 181. Eight studies were conducted in European countries and seven studies were conducted in Asian countries.
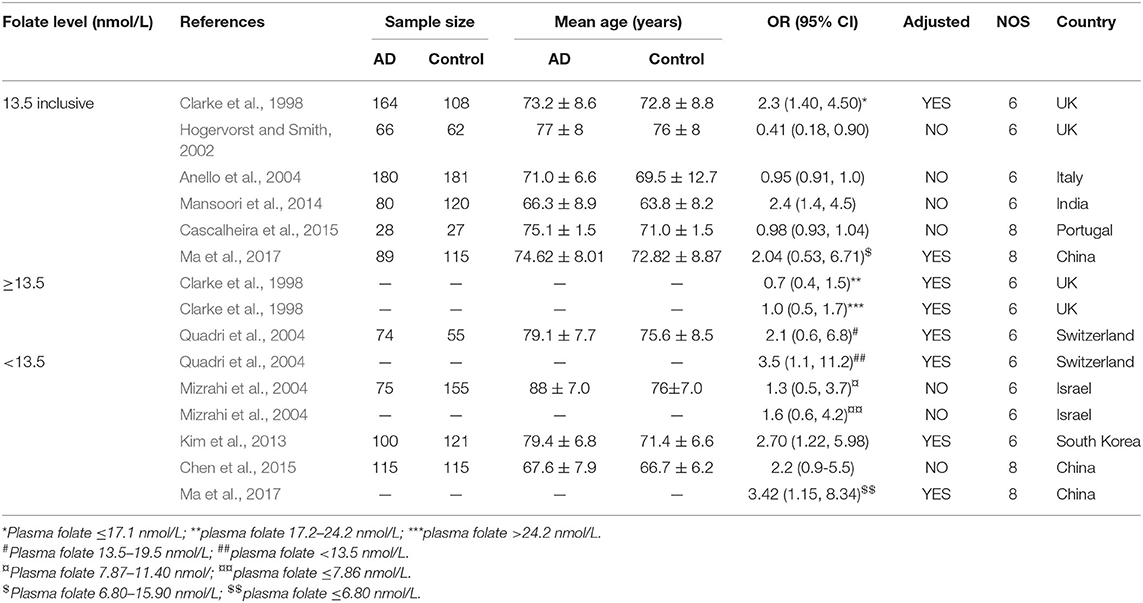
Table 2. Summary of studies regarding the association between folate level and the risk of AD in 14 case-control studies.
The combined OR was 0.96 with 95% CI (0.93, 0.99) (Figure 3A). According to the level of plasma/serum folate, all individuals were further divided into two subgroups, folate deficiency/possible deficiency group and normal group with folate level <13.5 and ≥13.5 nmol/L, respectively (WHO, 2015). In the folate deficiency/possible deficiency group, the combined OR was 1.94 (95% CI: 1.02, 2.86) (Figure 3B). However, the combined OR was 0.86 (95% CI: 0.46, 1.26) in the normal group (Figure 3B). The above data indicated that folate deficiency/possible deficiency is correlated with AD risk. It suggested that folate deficiency/possible deficiency may increase the risk for AD.
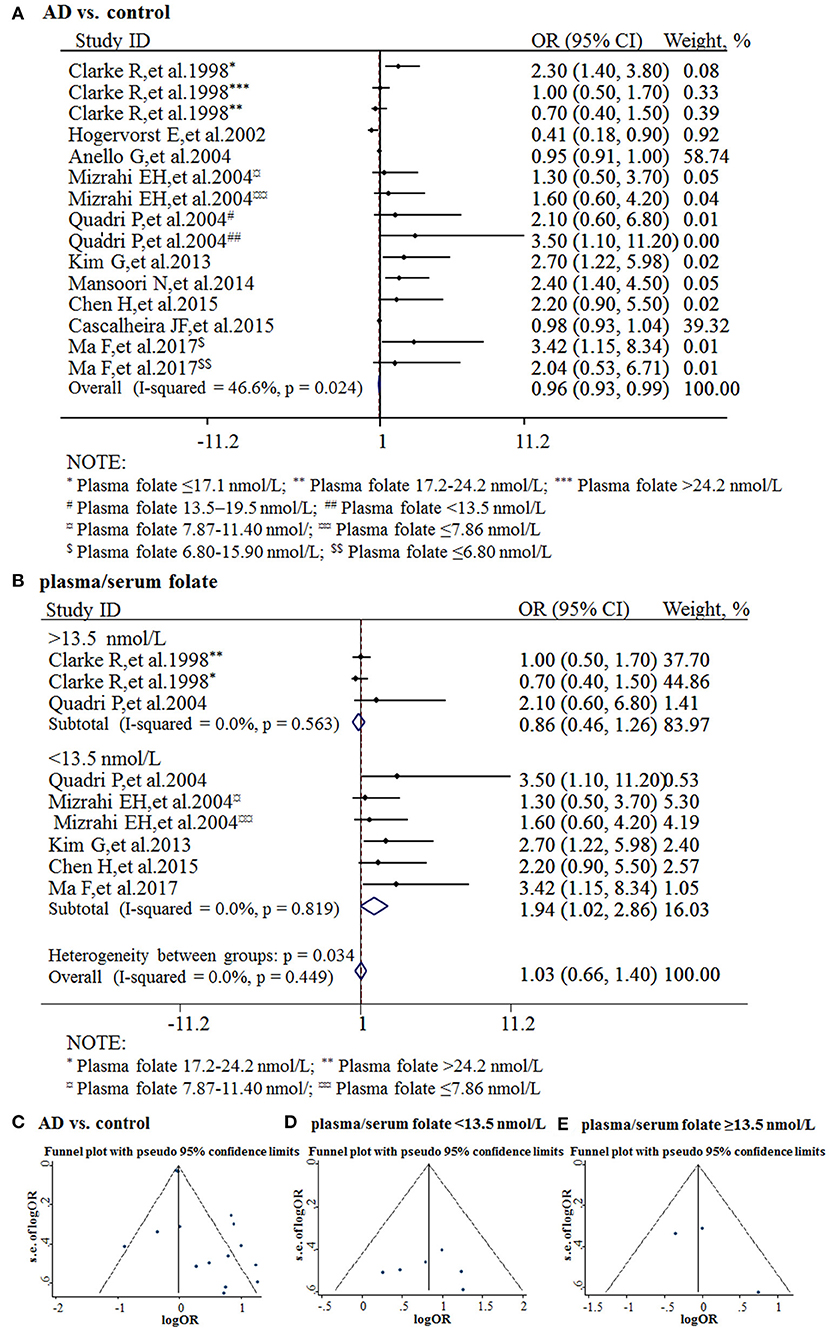
Figure 3. Meta-analysis for the associations between folate levels and risk of AD. (A) Combined folate OR of AD patients; (B) Combined OR in the folate deficiency/possible deficiency group and normal group; (C) Funnel plot for publication bias of AD vs. control; (D) Funnel plot for publication bias of the folate deficiency/possible deficiency group; (E) Funnel plot for publication bias of the normal folate group.
The funnel plots appeared to be symmetrical and all studies were within the 95% CIs, visually indicating there was no publication bias (Figures 3C–E). An Egger's test was further performed to evaluate publication bias. The P-value was 0.011 in all studies, indicating there was publication bias, language bias, inflated estimates, and/or a lack of publications with opposite results. Moreover, the P-value was 0.957 in the subgroup of folate deficiency/possible deficiency, indicating there was no publication bias in this subgroup.
Folate Defiency/Possible Deficiency Increases the Risk for AD: Results From Cohort Studies
Five studies from 2001 to 2017 were included in the meta-analysis (Table 3). Sample size ranged from 190 to 816. Two studies were conducted in Canada, and three in Italy, Sweden, and Switzerland, respectively. All the cohort studies presenting the folate levels of participants were divided into the folate deficiency/possible deficiency group and the normal group with folate level <13.5 and ≥13.5 nmol/L, respectively. In the folate deficiency/possible deficiency group, the combined RR was 1.88 (95% CI: 1.20, 2.57) (Figure 4A). The data indicated that folate deficiency/possible deficiency increases the risk for AD.

Table 3. Summary of studies regarding the association between folate level and the risk of AD in five cohort studies.
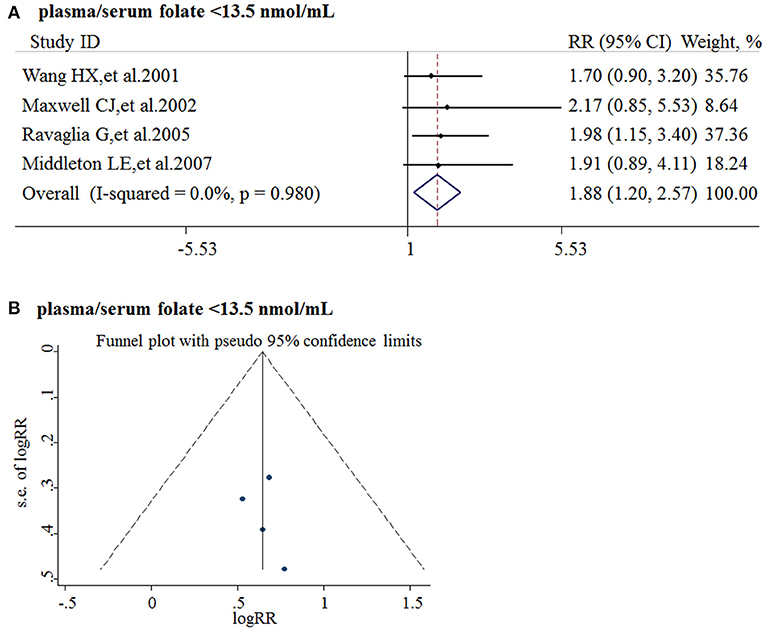
Figure 4. Meta-analysis for the associations between folate levels and risk of AD in general population. (A) Combined RR of AD in the folate deficiency/possible deficiency group; (B) Funnel plot for publication bias.
Publication bias was analyzed by funnel plots. The funnel plots appeared to be symmetrical and all studies were within the 95% CIs, visually indicating there was no publication bias (Figure 4B).
Sufficient Intake of Folate Reduces the Risk for AD: Results From Cohort Studies
Eleven cohort studies published from 2005 to 2009 were included in the meta-analysis (Table 4). Sample size ranged from 192 to 727. All studies were conducted in the USA.
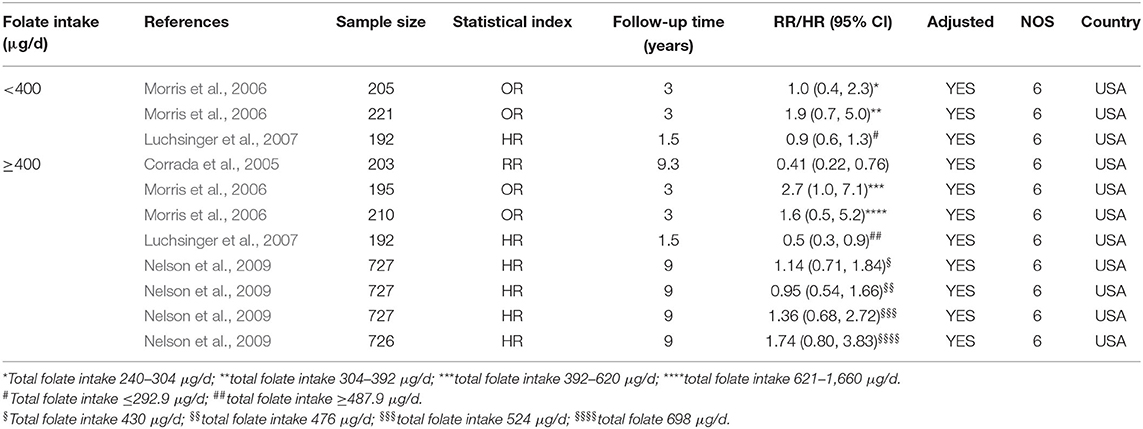
Table 4. Summary of studies regarding the association between folate intake and the risk of AD in 11 cohort studies.
All the 11 cohort studies were included in the meta-analysis to examine the association between folate intake and AD in the elderly. The combined RR was 0.50 (95% CI: 0.25, 0.76). As the recommended daily allowance of folate is 400 μg, a daily intake of 400 μg of folate was used as the cut-off to define the two subgroups (Benoist, 2008). When the daily intake of folate was <400 μg, the combined RR and HR were 1.15 (95% CI: 0.28, 2.02) and 0.9 (95% CI: 0.6, 1.3), respectively (Figure 5A). When the daily intake of folate was equal to or higher than 400 μg, the combined RR and HR were 0.44 (95% CI: 0.18, 0.71) and 0.76 (95% CI: 0.52, 0.99), respectively (Figures 5A,B). It indicated that sufficient folate intake (i.e., ≥400 μg/d) is a protective factor for AD, which significantly reduces the risk for AD.
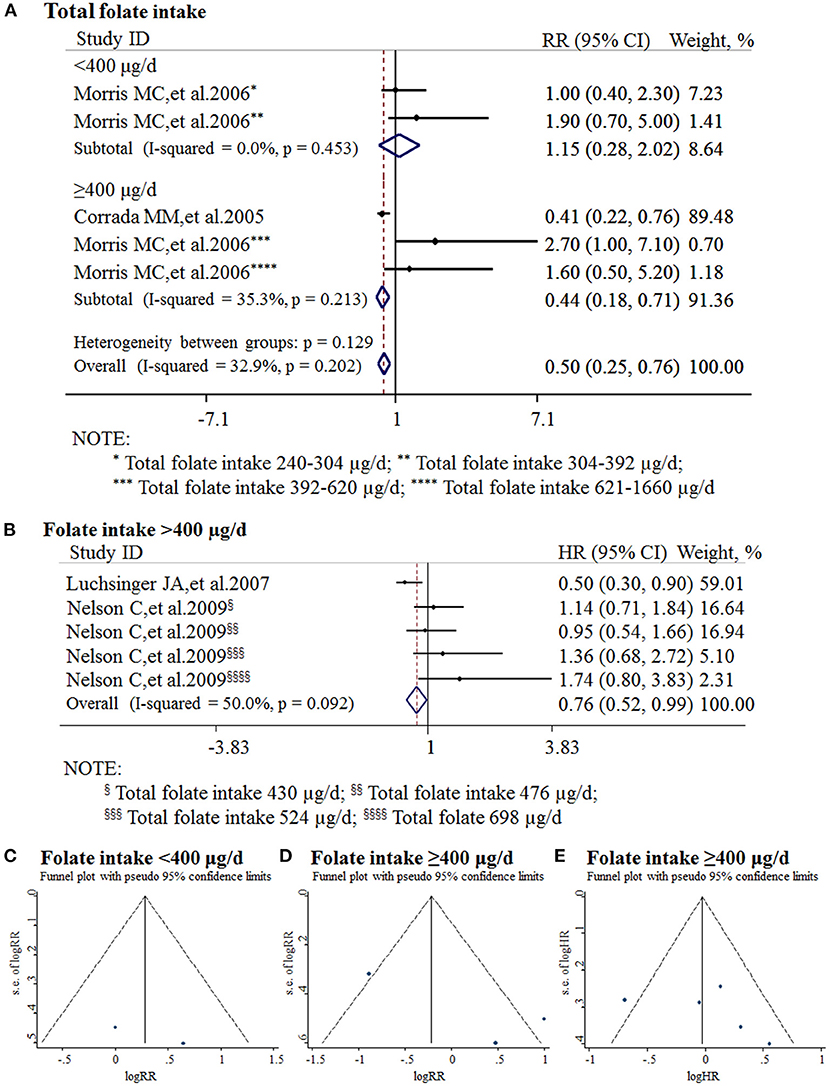
Figure 5. Meta-analysis for the associations between daily intake of folate and the risk of AD in the general population. (A) Combined RR of AD with insufficient and sufficient folate intake, respectively; (B) Combined HR of AD with sufficient folate intake; (C) Funnel plot for publication bias of insufficient folate intake; (D) Funnel plot for publication bias of sufficient folate intake (combined RR); (E) Funnel plot for publication bias of sufficient folate intake (combined HR).
Publication bias was analyzed by funnel plots. The funnel plots appeared to be symmetrical and all studies were within the 95% CIs, visually indicating there was no publication bias (Figures 5C–E).
Discussion
The present analyses were designed to comprehensively evaluate the associations between AD and folate levels. The pooled results showed that the folate level of AD patients was lower compared with that of healthy controls. Moreover, the deficiency/possible deficiency of folate (<13.5 nmol/L) increases the risk for AD. Importantly, sufficient daily intake of folate (≥400 μg/d) reduces the risk of AD occurence.
A meta-analysis was performed to explore the association between folate and Alzheimer's disease based on studies published before January, 2014 (Shen and Ji, 2015). Compared with the previous one, our study has the following distinguished characteristics. First, inclusion criteria of this study was restricted to AD, but not all forms of dementia. It might minimize the heterogeneity of different studies and make the conclusion more convincing. Moreover, cohort studies were included in addition to case-control studies. It indicated that folate deficiency/possible deficiency may have a causal effect on AD development. In addition, subgroup analysis was performed to evaluate the association between folate and AD. It definitely minimized the heterogeneity between the two populations, i.e., folate within normal range and folate deficiency/possible deficiency. Thus, our study highly indicated that folate deficiency/possible deficiency is a risk factor for AD. Importantly, the combined effect sizes of daily intake of folate and AD were first analyzed in this study. It indicated that sufficient daily intake of folate significantly reduces the risk for AD. Furthermore, the included studies were updated and cross-sectional studies were also included. For example, 52 cross-sectional studies covering 3,496 AD patients and 4,318 controls were included in the current study. The increase of the sample size makes the difference between AD and controls more reliable.
There were some limitations in this study. First, the different classification criteria, different categories of demographic characteristics, and different follow-up periods of the included studies may affect the pooled effects. Moreover, most of the studies were from North America, Europe, and Asia, with limited data from Africa and South America. The regional effect might need to be considered. Furthermore, more long-term follow-up studies were needed to confirm the preventive effect of sufficient folate intake on AD.
Although the mechanism of folate protecting against AD is not clear, there are plausible explanations for the association between AD and folate levels. First, folate has important biological activities, such as anti-oxidative stress, which counteracts AD development (Alonso et al., 2001; Agnati et al., 2007; De Felice et al., 2007; Zhang et al., 2009). Secondly, folate participates in the DNA methylation process as a donor of methyl, while DNA methylation plays a crucial role in aging and AD pathogenesis (Zhang et al., 2009; Smith and Lunnon, 2017). In addition, folate regulates the expression of both β-secretase and γ-secretase, theses two key secretases contribute to Aβ generation and neruitic plaque formation (Fuso et al., 2007; Ly et al., 2013; Wang et al., 2017; Zhang et al., 2020). Moreover, folate inhibits tau phosphorylation and subsequent neurofibrillary tangle formation by indirectly regulating the activity of protein phosphatase cyclin-dependent kinase and glycogen synthase kinase (Sontag and Sontag, 2014). Furthermore, folate might also be implicated in AD by regulating the level of homocysteine (Hcy) as it is a co-factor of Hcy metabolism (Zhang et al., 2009; Mccaddon and Miller, 2015; Smith and Refsum, 2016; Smith et al., 2018).
Conclusions
AD patients had lower levels of folate than healthy controls. Folate deficiency/possible deficiency may increase the risk for AD. Sufficient daily intake of folate may reduce the risk of AD occurrence. Trials have already shown that folic acid supplementation can slow cognitive decline and brain atrophy in patients with mild cognitive impairment (De Jager et al., 2012; Douaud et al., 2013; Ma et al., 2019). These findings indicated that sufficient folate intake is preventive against AD. Randomized controlled trials are needed to verify the causality of sufficient folate intake or folic acid supplementation and AD prevention.
Author Contributions
YW designed the study. XZ, GB, and YY searched for and screened the literature. XZ, GB, and YY extracted the data independently. XZ, DL, GB, and YL conducted the meta-analysis constructions. XZ, GB, and YY analyzed the data and wrote this manuscript. XL, YL, and YW revised the manuscript. All authors reviewed and proved the manuscript.
Funding
This study was funded by the National Natural Science Foundation of China (NSFC) grant 81771147 to YW, grant 81901391 to YL, and grant 31701247 to XL.
Conflict of Interest
The authors declare that the research was conducted in the absence of any commercial or financial relationships that could be construed as a potential conflict of interest.
References
Abalan, F., Zittoun, J., Boutami, C., Dugrais, G., Manciet, G., Decamps, A., et al. (1996). Plasma, red cell, and cerebrospinal fluid folate in Alzheimer's disease. Encephale 22, 430–434.
Agarwal, R., Chhillar, N., Kushwaha, S., Singh, N. K., and Tripathi, C. B. (2010). Role of vitamin B(12), folate, and thyroid stimulating hormone in dementia: a hospital-based study in north Indian population. Ann. Indian Acad. Neurol. 13, 257–262. doi: 10.4103/0972-2327.74193
Agnati, L. F., Genedani, S., Leo, G., Forni, A., Woods, A. S., Filaferro, M., et al. (2007). Abeta peptides as one of the crucial volume transmission signals in the trophic units and their interactions with homocysteine. Physiological implications and relevance for Alzheimer's disease. J. Neural Transm. 114, 21–31. doi: 10.1007/s00702-006-0564-9
Almeida, C. C., Brentani, H. P., Forlenza, O. V., and Diniz, B. S. (2011). Serum folic acid is reduced in patients with Alzheimer's disease. Arch. Clin. Psychiat. 39, 90–93. doi: 10.1590/S0101-60832012000300004
Alonso, A., Zaidi, T., Novak, M., Grundke-Iqbal, I., and Iqbal, K. (2001). Hyperphosphorylation induces self-assembly of tau into tangles of paired helical filaments/straight filaments. Proc. Natl. Acad. Sci. U. S. A. 98, 6923–6928. doi: 10.1073/pnas.121119298
Alzheimer's Association (2012). 2012 Alzheimer's disease facts and figures. Alzheimers Dement. 8, 131–168. doi: 10.1016/j.jalz.2012.02.001
Alzheimer's Disease International (2019). The World Alzheimer Report 2019: Attitudes to dementia. Available online at: https://www.alzint.org/resource/world-alzheimer-report-2019/ (accessed October 16, 2020).
Anello, G., Guéant-Rodríguez, R.-M., Bosco, P., Guéant, J.-L., Romano, A., Namour, B., et al. (2004). Homocysteine and methylenetetrahydrofolate reductase polymorphism in Alzheimer's disease. Neuroreport 15, 859–861. doi: 10.1097/00001756-200404090-00025
Annerbo, S., Wahlund, L.-O., and Lökk, J. (2006). The significance of thyroid-stimulating hormone and homocysteine in the development of Alzheimer's disease in mild cognitive impairment: a 6-year follow-up study. Am. J. Alzheimers Dis. 21, 182–188. doi: 10.1177/1533317506289282
Arlt, S., Schwedhelm, E., Kolsch, H., Jahn, H., Linnebank, M., Smulders, Y., et al. (2012). Dimethylarginines, homocysteine metabolism, and cerebrospinal fluid markers for Alzheimer's disease. J. Alzheimers. Dis. 31, 751–758. doi: 10.3233/JAD-2012-112138
Bakota, L., and Brandt, R. (2016). Tau biology and tau-directed therapies for Alzheimer's dieases. Drugs 76, 301–313. doi: 10.1007/s40265-015-0529-0
Benoist, B. D. (2008). Conclusions of a WHO Technical Consultation on folate and vitamin B12 deficiencies. Food Nutr. Bull. 29, S238–244. doi: 10.1177/15648265080292S129
Bottiglieri, T., Parnetti, L., Arning, E., Ortiz, T., Amici, S., Lanari, A., et al. (2001). Plasma total homocysteine levels and the C677T mutation in the methylenetetrahydrofolate reductase (MTHFR) gene: a study in an Italian population with dementia. Mech. Ageing Dev. 122, 2013–2023. doi: 10.1016/S0047-6374(01)00307-4
Cascalheira, J. F., Goncalves, M., Barroso, M., Castro, R., Palmeira, M., Serpa, A., et al. (2015). Association of the transcobalamin II gene 776C –> G polymorphism with Alzheimer's type dementia: dependence on the 5, 10-methylenetetrahydrofolate reductase 1298A –> C polymorphism genotype. Ann. Clin. Biochem. 52, 448–455. doi: 10.1177/0004563214561770
Chan, A., and Shea, T. B. (2006). Dietary and genetically-induced oxidative stress alter tau phosphorylation: influence of folate and apolipoprotein E deficiency. J. Alzheimers. Dis. 9, 399–405. doi: 10.3233/JAD-2006-9405
Chen, H., Liu, S., Ji, L., Wu, T., Ma, F., Ji, Y., et al. (2015). Associations between Alzheimer's Disease and blood homocysteine, vitamin B12, and folate: a case-control study. Curr. Alzheimer Res. 12, 88–94. doi: 10.2174/1567205012666141218144035
Clarke, R., Smith, A. D., Jobst, K. A., Refsum, H., Sutton, L., and Ueland, P. M. (1998). Folate, vitamin b12, and serum total homocysteine levels in confirmed Alzheimer Disease. JAMA Neurol. 55, 1449–1455. doi: 10.1001/archneur.55.11.1449
Cochrane Training (2020). Cochrane Handbook for Systematic Reviews of Interventions 10.4.3. Available online at: https://training.cochrane.org/handbook/current/chapter-10#section-10-4-3 (accessed October 16, 2020).
Corrada, M. M., Kawas, C. H., Hallfrisch, J., Muller, D., and Brookmeyer, R. (2005). Reduced risk of Alzheimer's disease with high folate intake: the baltimore longitudinal study of aging. Alzheimers Dement. 1, 11–18. doi: 10.1016/j.jalz.2005.06.001
Czapski, G. A., Maruszak, A., Styczyńska, M., Źekanowski, C., Safranow, K., and Strosznajder, J. B. (2012). Association between plasma biomarkers, CDK5 polymorphism and the risk of Alzheimer's disease. Acta. Neurobiol. Exp. 72, 397–411.
De Felice, F. G., Velasco, P. T., Lambert, M. P., Viola, K., Fernandez, S. J., Ferreira, S. T., et al. (2007). Abeta oligomers induce neuronal oxidative stress through an N-methyl-D-aspartate receptor-dependent mechanism that is blocked by the Alzheimer drug memantine. J. Biol. Chem. 282, 11590–11601. doi: 10.1074/jbc.M607483200
De Jager, C. A., Oulhaj, A., Jacoby, R., Refsum, H., and Smith, A. D. (2012). Cognitive and clinical outcomes of homocysteine-lowering B-vitamin treatment in mild cognitive impairment: a randomized controlled trial. Int. J. Geriatr. Psychiatry 27, 592–600. doi: 10.1002/gps.2758
De Lau, L. M., Refsum, H., Smith, A. D., Johnston, C., and Breteler, M. B. (2007). Plasma folate concentration and cognitive performance: Rotterdam Scan Study. Am. J. Clin. Nutr. 86, 728–734. doi: 10.1093/ajcn/86.3.728
De Silva, H. A., Gunatilake, S. B., Johnston, C., Warden, D., and Smith, A. D. (2005). Medial temporal lobe atrophy, apolipoprotein genotype, and plasma homocysteine in Sri Lankan patients with Alzheimer's disease. Exp. Aging Res. 31, 345–354. doi: 10.1080/03610730590948221
Dominguez, R. O., Marschoff, E. R., Guareschi, E. M., Famulari, A. L., Pagano, M. A., Serra, J. A., et al. (2005). Homocysteine, vitamin B 12 and folate in Alzheimer's and vascular dementias: the paradoxical effect of the superimposed type II diabetes mellitus condition. Clin. Chim. Acta 359, 163–170. doi: 10.1016/j.cccn.2005.03.049
Douaud, G., Refsum, H., De Jager, C. A., Jacoby, R., Nichols, T. E., Smith, S. M., et al. (2013). Preventing Alzheimer's disease-related gray matter atrophy by B-vitamin treatment. Proc. Natl. Acad. Sci. U. S. A. 110, 9523–9528. doi: 10.1073/pnas.1301816110
Durga, J., Boxtel, M. V. P., Schouten, E. G., Kok, F. J., Jolles, J., Katan, M. B., et al. (2007). Effect of 3-year folic acid supplementation on cognitive function in older adults in the FACIT trial: a randomised, double blind, controlled trial. Lancet 369, 208–216. doi: 10.1016/S0140-6736(07)60109-3
Ebly, E. M., Schaefer, J. P., Campbell, N. R., and Hogan, D. B. (1998). Folate status, vascular disease and cognition in elderly Canadians. Age Ageing 27, 485–491. doi: 10.1093/ageing/27.4.485
Faux, N. G., Ellis, K. A., Porter, L., Fowler, C. J., Laws, S. M., Martins, R. N., et al. (2011). Homocysteine, vitamin B12, and folic acid levels in Alzheimer's disease, mild cognitive impairment, and healthy elderly: baseline characteristics in subjects of the Australian Imaging Biomarker Lifestyle study. J. Alzheimers Dis. 27, 909–922. doi: 10.3233/JAD-2011-110752
Fioravanti, M., Ferrario, E., Massaia, M., Cappa, G., Rivolta, G., Grossi, E., et al. (1997). Low folate levels in the cognitive decline of elderly patients and the efficacy of folate as a treatment for improving memory deficits. Arch. Gerontol. Geriat. 26, 1–13. doi: 10.1016/S0167-4943(97)00028-9
Fuso, A., Cavallaro, R. A., Zampelli, A., D' Anselmi, F., and Scarpa, S. (2007). Gamma-Secretase is differentially modulated by alterations of homocysteine cycle in neuroblastoma and glioblastoma cells. J. Alzheimers Dis. 11, 275–290. doi: 10.3233/JAD-2007-11303
Galimberti, G., Conti, E., Zini, M., Piazza, F., Fenaroli, F., Isella, V., et al. (2008). Post-methionine load test: a more sensitive tool to reveal hyperhomocysteinemia in Alzheimer patients? Clin. Biochem. 41, 914–916. doi: 10.1016/j.clinbiochem.2008.03.015
Gallucci, M., Zanardo, A., De Valentin, L., and Vianello, A. (2004). Homocysteine in Alzheimer disease and vascular dementia. Arch. Gerontol. Geriatr. 38, 195–200. doi: 10.1016/j.archger.2004.04.027
Guo, J., Ni, S., Li, Q., Wang, J. Z., and Yang, Y. (2019). Folate/vitamin b alleviates hyperhomocysteinemia-induced alzheimer-like pathologies in rat retina. Neurosci. Bull. 35, 325–335. doi: 10.1007/s12264-018-0293-8
Hagnelius, N. O., Wahlund, L. O., and Nilsson, T. K. (2008). CSF/serum folate gradient: physiology and determinants with special reference to dementia. Dement. Geriatr. Cogn. 25, 516–523. doi: 10.1159/000129696
Hassing, L., Wahlin Winblad, B., and Bäckman, L. (1999). Further evidence on the effects of vitamin B12 and folate levels on episodic memory functioning: a population-based study of healthy very old adults. Biol. Psychiat. 45, 1472–1480. doi: 10.1016/S0006-3223(98)00234-0
Hebert, L. E., Weuve, J., Scherr, P. A., and Evans, D. A. (2013). Alzheimer disease in the United States (2010-2050) estimated using the 2010 census. Neurology 80, 1778–1783. doi: 10.1212/WNL.0b013e31828726f5
Hogervorst, E., and Smith, A. D. (2002). The interaction of serum folate and estradiol levels in Alzheimer's disease. NeuroEndocrinol. Lett. 23, 155–160.
Hozo, S. P., Djulbegovic, B., and Hozo, I. (2005). Estimating the mean and variance from the median, range, and the size of a sample. BMC Med. Res. Methodol. 5:13. doi: 10.1186/1471-2288-5-13
Irizarry, M. C., Gurol, M. E., Raju, S., Diaz-Arrastia, R., Locascio, J. J., Tennis, M., et al. (2005). Association of homocysteine with plasma amyloid beta protein in aging and neurodegenerative disease. Neurology 65, 1402–1408. doi: 10.1212/01.wnl.0000183063.99107.5c
Jheng, Y., Chen, T. F., Chou, C. Y., Chiu, M. J., Hsieh, R. H., Wang, C. C., et al. (2016). Effects of folic acid supplementation in Alzheimer diseases-associated mitochondrial pathology and genomic expression profiles in the 3xTg-AD mice model. FASEB J. 30. Abstract retrieved from Abstracts in Embase (Embase Identification Number (PUI) L72319904).
Joosten, E., Lesaffre, E., Riezler, R., Ghekiere, V., Dereymaeker, L., Pelemans, W., et al. (1997). Is metabolic evidence for vitamin b-12 and folate deficiency more frequent in elderly patients with Alzheimer's Disease? J. Gerontol. A Biol. Sci. Med. Sci. 52, 76–79. doi: 10.1093/gerona/52A.2.M76
Karimi, F., Haghighi, A. B., and Petramfar, P. (2009). Serum levels of homocysteine, vitamin b12, and folic acid in patients with Alzheimer's Disease. Iran. J. Med. Sci. 34, 181–185.
Kim, G., Kim, H., Kim, K. N., Son, J. I., Kim, S. Y., Tamura, T., et al. (2013). Relationship of cognitive function with B vitamin status, homocysteine, and tissue factor pathway inhibitor in cognitively impaired elderly: a cross-sectional survey. J. Alzheimers. Dis. 33, 853–862. doi: 10.3233/JAD-2012-121345
Kim, H., and Lee, K. J. (2014). Serum homocysteine levels are correlated with behavioral and psychological symptoms of Alzheimer's disease. Neuropsych. Dis. Treat. 10, 1887–1896. doi: 10.2147/NDT.S68980
Koseoglu, E., and Karaman, Y. (2007). Relations between homocysteine, folate and vitamin B12 in vascular dementia and in Alzheimer disease. Clin. Biochem. 40, 859–863. doi: 10.1016/j.clinbiochem.2007.04.007
Leblhuber, F., Walli, J. S., Wagner-Jauregg, L.-N., Austria, L., Atner-Dworzak, E., Vrecko, K., et al. (2000). Hyperhomocysteinemia in dementia. J. Neural Transm. 107, 1469–1474. doi: 10.1007/s007020070010
Levitt, A. J., and Karlinsky, H. (1992). Folate, vitamin B12 and cognitive impairment in patients with Alzheimer's disease. Acta Psychiat. Scand. 86, 301–305. doi: 10.1111/j.1600-0447.1992.tb03270.x
Li, K., Wei, Q., Liu, F. F., Hu, F., Xie, A. J., Zhu, L. Q., et al. (2018). Synaptic dysfunction in Alzheimer's Disease: Aβ, tau, and epigenetic alterations. Mol. Neurobiol. 55, 3021–3032. doi: 10.1007/s12035-017-0533-3
Li, Q., Chen, Z., Zhang, S.-Z., Ma, L., Zhang, L., Wu, H., et al. (2014). Change of plasma levels hemocysteine and folate and vitamin B12 in patients with Alzheimer's disease. Chin. J. Gen. Prac. 12, 9–11. doi: 10.16766/j.cnki.issn.1674-4152.2014.01.066
Linnebank, M., Popp, J., Smulders, Y., Smith, D., Semmler, A., Farkas, M., et al. (2010). S-adenosylmethionine is decreased in the cerebrospinal fluid of patients with Alzheimer's disease. Neurodegener. Dis. 7, 373–378. doi: 10.1159/000309657
Liu, J.-H., and Chen, Z.-L. (2006). Observation of serum cholesterol, IL-6 and VitB12 levels in Alzheimer's Disease or vascular dementia patients. J. Jiangsu Univ. Med. Ed. 16, 48–52. doi: 10.13312/j.issn.1671-7783.2006.01.015
Lovati, C., Galimberti, D., Pomati, S., Capiluppi, E., Dolci, A., Scapellato, L., et al. (2007). Serum folate concentrations in patients with cortical and subcortical dementias. Neurosci. Lett. 420, 213–216. doi: 10.1016/j.neulet.2007.04.060
Luchsinger, J. A., Tang, M. X., Miller, J., Green, R., and Mayeux, R. (2007). Relation of higher folate intake to lower risk of Alzheimer disease in the elderly. Arch. Neurol. 64, 86–92. doi: 10.1001/archneur.64.1.86
Ly, P. T., Wu, Y., Zou, H., Wang, R., Zhou, W., Kinoshita, A., et al. (2013). Inhibition of GSK3beta-mediated BACE1 expression reduces Alzheimer-associated phenotypes. J. Clin. Invest. 123, 224–235. doi: 10.1172/JCI64516
Ma, F., Wu, T., Zhao, J., Ji, L., Song, A., Zhang, M., et al. (2017). Plasma homocysteine and serum folate and vitamin b12 levels in mild cognitive impairment and Alzheimer's Disease: a case-control study. Nutrients 9:725. doi: 10.3390/nu9070725
Ma, F., Zhou, X., Li, Q., Zhao, J., Song, A., An, P., et al. (2019). Effects of folic acid and Vitamin B12, alone and in combination on cognitive function and inflammatory factors in the elderly with mild cognitive impairment: a single-blind experimental design. Curr. Alzheimer Res. 16, 622–632. doi: 10.2174/1567205016666190725144629
Malaguarnera, M., Bella, R., Alagona, G., Ferri, R., Carnemolla, A., and Pennisi, G. (2004a). Helicobacter pylori and Alzheimer's disease: a possible link. Eur. J. Intern. Med. 15, 381–386. doi: 10.1016/j.ejim.2004.05.008
Malaguarnera, M., Ferri, R., Bella, R., Alagona, G., Carnemolla, A., and Pennisi, G. (2004b). Homocysteine, vitamin B12 and folate in vascular dementia and in Alzheimer disease. Clin. Chem. Lab. Med. 42, 1032–1035. doi: 10.1515/CCLM.2004.208
Mansoori, N., Tripathi, M., Alam, R., Luthra, K., Sharma, S., Lakshmy, R., et al. (2014). Serum folic acid and RFC A80G polymorphism in Alzheimer's disease and vascular dementia. Am. J. Alzheimers Dis. 29, 38–44. doi: 10.1177/1533317513505131
Mantzavinos, V., and Alexiou, A. (2017). Biomarkers for Alzheimer's disease diagnosis. Curr. Alzheimer Res. 14, 1149–1154. doi: 10.2174/1567205014666170203125942
Maxwell, C. J., Hogan, D. B., and Ebly, E. M. (2002). Serum folate levels and subsequent adverse cerebrovascular outcomes in elderly persons. Dement. Geriatr. Cogn. 13, 225–234. doi: 10.1159/000057701
Mccaddon, A., and Miller, J. W. (2015). Assessing the association between homocysteine and cognition: reflections on Bradford Hill, meta-analyses, and causality. Nutr. Rev. 73, 723–735. doi: 10.1093/nutrit/nuv022
Mcilroy, S. P., Dynan, K. B., Lawson, J. T., Patterson, C. C., and Passmore, A. P. (2002). Moderately elevated plasma homocysteine, methylenetetrahydrofolate reductase genotype, and risk for stroke, vascular dementia, and Alzheimer disease in Northern Ireland. Stroke 33, 2351–2356. doi: 10.1161/01.STR.0000032550.90046.38
Middleton, L. E., Kirkland, S. A., Maxwell, C. J., Hogan, D. B., and Rockwood, K. (2007). Exercise: a potential contributing factor to the relationship between folate and dementia. J. Am. Geriatr. Soc. 55, 1095–1098. doi: 10.1111/j.1532-5415.2007.01238.x
Mizrahi, E. H., Bowirrat, A., Jacobsen, D. W., Korczyn, A. D., Traore, F., Petot, G. J., et al. (2004). Plasma homocysteine, vitamin B12 and folate in Alzheimer's patients and healthy Arabs in Israel. J. Neurol. Sci. 227, 109–113. doi: 10.1016/j.jns.2004.08.011
Moazzen, S., Dolatkhah, R., Tabrizi, J. S., Shaarbafi, J., Alizadeh, B. Z., De Bock, G. H., et al. (2018). Folic acid intake and folate status and colorectal cancer risk: a systematic review and meta-analysis. Clin. Nutr. 37, 1926–1934. doi: 10.1016/j.clnu.2017.10.010
Moretti, R., Caruso, P., Dal Ben, M., Conti, C., Gazzin, S., and Tiribelli, C. (2017). Vitamin D, homocysteine, and folate in subcortical vascular dementia and Alzheimer dementia. Front. Aging Neurosci. 9:169. doi: 10.3389/fnagi.2017.00169
Morillas-Ruiz, J. M., Rubio-Perez, J. M., Albaladejo, M. D., Zafrilla, P., Parra, S., and Vidal-Guevara, M. L. (2010). Effect of an antioxidant drink on homocysteine levels in Alzheimer's patients. J. Neurol. Sci. 299, 175–178. doi: 10.1016/j.jns.2010.08.050
Morris, M. C., Evans, D. A., Schneider, J. A., Tangney, C. C., Bienias, J. L., and Aggarwal, N. T. (2006). Dietary folate and vitamins B-12 and B-6 not associated with incident Alzheimer's disease. J. Alzheimers. Dis. 9, 435–443. doi: 10.3233/JAD-2006-9410
Morris, M. S. (2003). Homocysteine and Alzheimer's disease. Lancet Neurol. 2, 425–428. doi: 10.1016/S1474-4422(03)00438-1
Nelson, C., Wengreen, H. J., Munger, R. G., and Corcoran, C. D. (2009). Dietary folate, vitamin B-12, vitamin B-6 and incident Alzheimer's disease: the cache county memory, health and aging study. J. Nutr. Health Aging 13, 899–905. doi: 10.1007/s12603-009-0249-9
Nurk, E., Refsum, H., Tell, G. S., Engedal, K., Vollset, S. E., Ueland, P. M., et al. (2005). Plasma total homocysteine and memory in the elderly: the Hordaland Homocysteine Study. Ann. Neurol. 58, 847–857. doi: 10.1002/ana.20645
Otaegui-Arrazola, A., Amiano, P., Elbusto, A., Urdaneta, E., and Martinez-Lage, P. (2014). Diet, cognition, and Alzheimer's disease: food for thought. Eur. J. Nutr. 53, 1–23. doi: 10.1007/s00394-013-0561-3
Parnetti, L., Mecocci, P., Reboldi, G. P., Santucci, C., Brunetti, M., Gaiti, A., et al. (1992). Platelet MAO-B activity and vitamin B12 in old age dementias. Mol. Chem. Neuropathol. 16, 23–32. doi: 10.1007/BF03159958
Peng, H., Li, F.-M., and Ye, N. (2004). Correlations of Alzheimer disease with vitamin B12 and homocysteine. Chin. J. Clin. Rehabil. 8, 6210–6211. doi: 10.3321/j.issn:1673-8225.2004.28.030
Postiglione, A., Milan, G., Ruocco, A., Gallotta, G., Guiotto, G., and Di Minno, G. (2001). Plasma folate, vitamin B12, and total homocysteine and homozygosity for the C677T mutation of the 5,10-methylene tetrahydrofolate reductase gene in patients with Alzheimer's dementia. Gerontology 47, 324–329. doi: 10.1159/000052822
Quadri, P., Fragiacomo, C., Pezzati, R., Zanda, E., Forloni, G., Tettamanti, M., et al. (2004). Homocysteine, folate, and vitamin B-12 in mild cognitive impairment, Alzheimer disease, and vascular dementia. Am. J. Clin. Nutr. 80, 114–122. doi: 10.1093/ajcn/80.1.114
Quadri, P., Fragiacomo, C., Pezzati, R., Zanda, E., Tettamanti, M., and Lucca, U. (2005). Homocysteine and B vitamins in mild cognitive impairment and dementia. Clin. Chem. Lab. Med. 43, 1096–1100. doi: 10.1515/CCLM.2005.191
Ravaglia, G., Forti, P., Maioli, F., Bianchi, G., Martelli, M., Talerico, T., et al. (2004). Plasma amino acid concentrations in patients with amnestic mild cognitive impairment or Alzheimer disease. Am. J. Clin. Nutr. 80, 483–488. doi: 10.1093/ajcn/80.2.483
Ravaglia, G., Forti, P., Maioli, F., Vettori, C., Grossi, G., Bargossi, A. M., et al. (2001). Elevated plasma homocysteine levels in centenarians are not associated with cognitive impairment. Mech. Ageing Dev. 121, 251–261. doi: 10.1016/S0047-6374(00)00221-9
Ravaglia, G., Paola, F., Fabiola, M., Mabel, M., Lucia, S., Nicoletta, B., et al. (2005). Homocysteine and folate as risk factors for dementia and Alzheimer disease. Am. J. Clin. Nutr. 82, 636–643. doi: 10.1093/ajcn/82.3.636
Regland, B., Abrahamsson, L., Blennow, K., Gottfries, C. G., and Wallin, A. (1992). Vitamin B12 in CSF: reduced CSF/serum B12 ratio in demented men. Acta Neurol. Scand. 85, 276–281. doi: 10.1111/j.1600-0404.1992.tb04044.x
Religa, D., Styczynska, M., Peplonska, B., Gabryelewicz, T., Pfeffer, A., Chodakowska, M., et al. (2003). Homocysteine, apolipoproteine E and methylenetetrahydrofolate reductase in Alzheimer's disease and mild cognitive impairment. Dement. Geriatr. Cogn. 16, 64–70. doi: 10.1159/000070677
Robinson, N., Grabowski, P., and Rehman, I. (2018). Alzheimer's disease pathogenesis: is there a role for folate? Mech. Ageing Dev. 174, 86–94. doi: 10.1016/j.mad.2017.10.001
Selley, M. L., Closea, D. R., and Sternb, S. E. (2002). The effect of increased concentrations of homocysteine on the concentration of (E)-4-hydroxy-2-nonenal in the plasma and cerebrospinal fluid of patients with Alzheimer's disease. Neurobiol. Aging 23, 383–388. doi: 10.1016/S0197-4580(01)00327-X
Serot, J. M., Christmann, D., Dubost, T., Béné, M. C., and Faure, G. C. (2001). CSF-folate levels are decreased in late-onset AD patients. J. Neural Transm. 108, 93–99. doi: 10.1007/s007020170100
Shen, L., and Ji, H. F. (2015). Associations between homocysteine, folic acid, vitamin B12 and Alzheimer's disease: insights from meta-analyses. J. Alzheimers Dis. 46, 777–790. doi: 10.3233/JAD-150140
Smith, A. D., and Refsum, H. (2016). Homocysteine, B vitamins, and cognitive impairment. Ann. Rev. Nutr. 36, 211–239. doi: 10.1146/annurev-nutr-071715-050947
Smith, A. D., Refsum, H., Bottiglieri, T., Fenech, M., Hooshmand, B., Mccaddon, A., et al. (2018). Homocysteine and dementia: an international consensus statement. J. Alzheimers Dis. 62, 561–570. doi: 10.3233/JAD-171042
Smith, R. G., and Lunnon, K. (2017). DNA modifications and Alzheimer's disease. Adv. Exp. Med. Biol. 978, 303–319. doi: 10.1007/978-3-319-53889-1_16
Sontag, J. M., and Sontag, E. (2014). Protein phosphatase 2A dysfunction in Alzheimer's disease. Front. Mol. Neurosci. 7:16. doi: 10.3389/fnmol.2014.00016
Stang, A. (2010). Critical evaluation of the Newcastle-Ottawa scale for the assessment of the quality of nonrandomized studies in meta-analyses. Eur. J. Epidemiol. 25, 603–605. doi: 10.1007/s10654-010-9491-z
Stroup, D. F., Berlin, J. A., Morton, S. C., Olkin, I., Williamson, G. D., Rennie, D., et al. (2000). Meta-analysis of observational studies in epidemiology: a proposal for reporting. Meta-analysis Of Observational Studies in Epidemiology (MOOSE) group. J. Am. Med. Assoc. 283, 2008–2012. doi: 10.1001/jama.283.15.2008
Tian, T., Bai, D., Li, W., Huang, G. W., and Liu, H. (2016). Effects of folic acid on secretases involved in Aβ deposition in APP/PS1 mice. Nutrients 8:556. doi: 10.3390/nu8090556
Villa, P., Bosco, P., Ferri, R., Perri, C., Suriano, R., Costantini, B., et al. (2009). Fasting and post-methionine homocysteine levels in Alzheimers disease and vascular dementia. Int. J. Vitam. Nutr. Res. 79, 166–172. doi: 10.1024/0300-9831.79.3.166
Wahlin, A., Hill, R. D., Winblad, B., and Bäckman, L. (1996). Effects of serum vitamin B2 and folate status on episodic memory performance in very old age: a population-based study. Psychol. Aging 11, 487–496. doi: 10.1037/0882-7974.11.3.487
Wang, H. X., Wahlin, A., Basun, H., Fastbom, J., Winblad, B., and Fratiglioni, L. (2001). Vitamin B(12) and folate in relation to the development of Alzheimer's disease. Neurology 56, 1188–1194. doi: 10.1212/WNL.56.9.1188
Wang, X., Zhou, X., Li, G., Zhang, Y., Wu, Y., and Song, W. (2017). Modifications and trafficking of APP in the pathogenesis of Alzheimer's disease. Front. Mol. Neurosci. 10:294. doi: 10.3389/fnmol.2017.00294
WHO (2015). Serum and Red Blood Cell Folate Concentrations for Assessing Folate Status in Populations. Available online at: https://apps.who.int/iris/handle/10665/75584 (accessed October 16, 2020).
Zeng, J., Chen, L., Wang, Z., Chen, Q., Fan, Z., Jiang, H., et al. (2017). Marginal vitamin A deficiency facilitates Alzheimer's pathogenesis. Acta Neuropathol. 133, 967–982. doi: 10.1007/s00401-017-1669-y
Zhang, C. E., Wei, W., Liu, Y. H., Peng, J. H., Tian, Q., Liu, G. P., et al. (2009). Hyperhomocysteinemia increases beta-amyloid by enhancing expression of gamma-secretase and phosphorylation of amyloid precursor protein in rat brain. Am. J. Pathol. 174, 1481–1491. doi: 10.2353/ajpath.2009.081036
Zhang, S., Cai, F., Wu, Y., Bozorgmehr, T., Wang, Z., Huang, D., et al. (2020). A presenilin-1 mutation causes Alzheimer disease without affecting Notch signaling. Mol. Psychiatr. 25, 603–613. doi: 10.1038/s41380-018-0101-x
Zhong, X., Li, Y., Du, C., Li, G., Li, H., and Zhu, A. (2016). Changes in serum homocysteine and its correlation with altitude, folacin and high-sensitivity C-reactive protein in Tibetan patients with mild-to-moderate Alzheimer's disease at different altitudes. Chin. J. Geriatri. 35, 934–938. doi: 10.3760/cma.j.issn.0254-9026.2016.09.003
Keywords: Alzheimer's disease, folate level, folate deficiency, sufficient folate intake, meta-analaysis
Citation: Zhang X, Bao G, Liu D, Yang Y, Li X, Cai G, Liu Y and Wu Y (2021) The Association Between Folate and Alzheimer's Disease: A Systematic Review and Meta-Analysis. Front. Neurosci. 15:661198. doi: 10.3389/fnins.2021.661198
Received: 30 January 2021; Accepted: 17 March 2021;
Published: 14 April 2021.
Edited by:
Johanna O. Ojala, University of Eastern Finland, FinlandReviewed by:
May A. Beydoun, National Institutes of Health (NIH), United StatesDavid Smith, University of Oxford, United Kingdom
Copyright © 2021 Zhang, Bao, Liu, Yang, Li, Cai, Liu and Wu. This is an open-access article distributed under the terms of the Creative Commons Attribution License (CC BY). The use, distribution or reproduction in other forums is permitted, provided the original author(s) and the copyright owner(s) are credited and that the original publication in this journal is cited, in accordance with accepted academic practice. No use, distribution or reproduction is permitted which does not comply with these terms.
*Correspondence: Yili Wu, yili_wu2004@yahoo.ca; wuyili@mail.jnmc.edu.cn; Yan Liu, hakunaly@163.com