- 1School of Medicine, University of Western Sydney, Penrith, NSW, Australia
- 2National Institute of Complementary Medicine, University of Western Sydney, Penrith, NSW, Australia
- 3Molecular Medicine Research Group, University of Western Sydney, Penrith, NSW, Australia
Chronic Neuroinflammation and Alzheimer's Disease
Alzheimer's disease (AD) is a neurodegenerative disorder that impacts the daily lives of many sufferers through memory loss as well as behavioral and cognitive changes. AD is the most common form of dementia. One in ten people over the age of 65, and around half of those over 85 have AD. AD can be divided into familial (early-onset) and sporadic (late-onset) cases, with the familial form (<1%) linked to mutations in three major genes (amyloid precursor protein, presenilin-1 and 2), and the sporadic form (>99% of cases) caused by a variety of genetic (e.g., apolipoprotein E), metabolic and environmental factors.
The AD brain is characterized macroscopically by cortical atrophy, caused by degeneration of the cholinergic axonal arborisation and shrinkage of the dendritic tree. Microscopically, amyloid beta peptide deposits (senile plaques) and neurofibrillary tangles are present in affected areas (Gil-Bea et al., 2012). AD is also characterized by chronic neuroinflammation, driven by activation of astroglia and microglia (Rosenblum, 2014). In addition, levels of pro-inflammatory mediators or cytokines which include chemokines, interferons, interleukins, lymphokines, and tumor necrosis factors are elevated in the brains of patients with AD (Latta et al., 2014). Furthermore, nuclear translocation of NF-κB and STAT-1α, transcription factors involved in pro-inflammatory gene expression, indicates the presence of a sustained pro-inflammatory process (Lawrence, 2009).
Drug Discovery Difficulties Faced in Alzheimer's Disease
Drug discovery for AD has been strongly focused on β-amyloid (initially plaques, then soluble oligomers), as genetic evidence from the familial cases supported by the hypothesis that β-amyloid must be driving the disease process. Based on the “amyloid cascade hypothesis,” anti-amyloid therapies were hoped to deliver a cure for AD (Robinson et al., 2004). Unfortunately, numerous clinical trials with active and passive amyloid vaccines as well as ɣ-secretase inhibitors have failed (reviewed in Castello et al., 2014). Currently, there are no disease-modifying drugs available for AD. Consequently, alternative therapeutic targets, such as neuroinflammation have been suggested for the prevention and treatment of AD (Shi et al., 2013; Latta et al., 2014). As the expression of many pro-inflammatory cytokines is driven by the transcription factor NF-κB (Hoffmann et al., 2006), we propose that brain-permeable inhibitors of NF-κB signaling have the potential to prevent or slow down the progression of AD.
Role of Nuclear Factor-κB (NF-κB) in Inflammation
Stimulation of microglia, the resident macrophages in the brain, initiates an inflammatory cascade, which involves NF-κB signaling. NF-κB is a ubiquitous transcription factor found in almost all animal cell types. NF-κB regulates the expression of many cytokines and chemokines, such as interferons, interleukins, lymphokines and tumor necrosis factors. Microglial cells express membrane receptors as toll-like receptors (TLRs), C-type lectin receptors (CLRs), nucleotide-binding oligomerization domain proteins (NLRs), the receptor for advanced glycation endproducts, RAGE and receptor for interferons and cytokines (Figuera-Losada et al., 2014). TLRs recognize pro-inflammatory ligands such as pathogen-associated molecular patterns (PAMPs) and damage-associated molecular patterns (DAMPs) (Figure 1). PAMPs include bacterial, fungal, parasitic, and viral molecules such as α- and β-glucans, viral RNA and DNA, flagellin, chitin, and microbial cell wall components (Figuera-Losada et al., 2014). DAMPs include blood-clotting factors, RNA, DNA and a variety of intracellular proteins released from damaged and dying cells (Figuera-Losada et al., 2014).
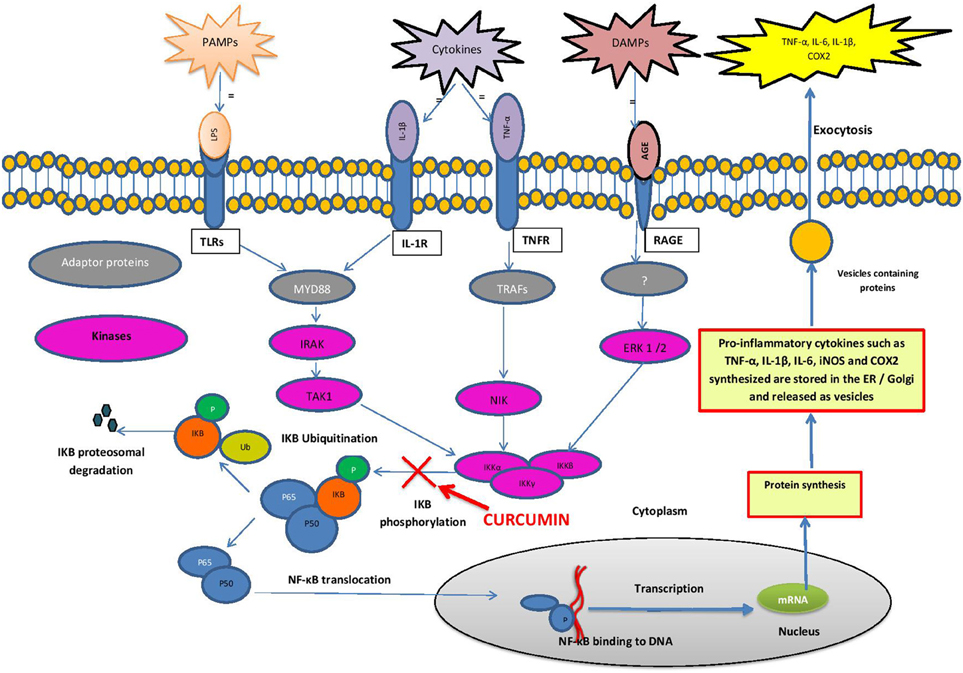
Figure 1. Schematic diagram of the NF-κB pathway. Cells express membrane receptors such as toll-like receptors (TLRs), interleukin-1β receptors (IL-1R), tumor necrosis factor receptors (TNFR) and receptor for advanced glycation end products (RAGE). These receptors recognize pro-inflammatory stimuli such as pathogen-associated molecular patterns (PAMPs), damage-associated molecular patterns (DAMPs) and cytokines. Ligand bound PAMPs, DAMPs and cytokines activate downstream adapter proteins such as myeloid differentiation primary response protein 88 (MyD88) and tumor necrosis factor associated factors (TRAF). MyD88 and TRAF activates specific protein kinases such as mitogen activated protein kinases (MAPK) such as IRAK, TAK1, NIK, and ERK 1/2. These kinases activate IκB kinases (IKKα, IKKβ, IKKγ) that phosphorylate IkB-α. In stimulated cells, phosphorylation of IkB leads to its dissociation from the complex, and its proteasomal degradation, allowing NF-kB to translocate to the nucleus, where it binds to specific DNA sequences present in the promoters of numerous target genes, encoding the pro inflammatory cytokines (e.g., IL-1, IL-2, IL-6, TNF-α), chemokines (e.g., IL-8, MIP-1α, MCP1, RANTES, eotaxin), adhesion molecules (e.g., ICAM, VCAM, E-selectin) as well as Cyclooxygenase-2 (Cox-2) and inducible nitric oxide synthase (iNOS).
In unstimulated cells, NF-kB is present in the cytoplasm as an inactive heterodimer composed of two subunits, p50 and p65 (relA). The heterodimer forms a complex with the inhibitory proteins IkB-α or IkB-β, retaining it in the cytoplasm. When stimulated by a PAMP, DAMP or cytokine, it triggers a cascade of signaling events initiated by stimulation of adapter proteins in the cytoplasm. In the case of many TLRs, myeloid differentiation primary response protein 88 (MYD88) is such an adaptor protein (Mogensen, 2009; Figuera-Losada et al., 2014). MYD88 activates specific protein kinases downstream, such as mitogen activated protein kinase (MAPK) and IκB kinase which phosphorylate IkB-α. Phosphorylation of IκB-α and its proteasomal degradation leads to its dissociation from the NF-κB complex. NF-κB then translocates into the nucleus, where it binds to the promotor regions of specific genes (Brasier, 2006; Gilmore, 2006; Perkins, 2007). NF-κB then recruits other proteins (coactivators and RNA polymerase), which finally lead to the expression of many chemokines (e.g., IL-8, MIP-1α, MCP1, RANTES and eotaxin), proinflammatory cytokines (e.g., IL-1, IL-2, IL-6 and TNF-α), adhesion molecules (e.g., ICAM, VCAM, E-selectin) as well as inducible pro-inflammatory enzymes (COX-2 and iNOS), which exacerbate and perpetuate the inflammatory process (Barnes, 1997; Ghosh and Karin, 2002; Figuera-Losada et al., 2014).
Plant Polyphenols and their Ability to Prevent Age-Related Degenerative Diseases
Many dietary plants including fruits, vegetables and whole grains contain substantial amounts of polyphenols (Carlsen et al., 2010; Gunawardena et al., 2014). Polyphenols are characterized as compounds with phenolic structural features (Liu, 2004; Tsao, 2010). Dietary polyphenols have been suggested to aid in the prevention of degenerative diseases, including cancer, cardiovascular disease and neurodegenerative diseases such as AD due to their anti-inflammatory and anti-oxidant properties (Liu, 2004; Tsao, 2010). The high diversification of plant polyphenols have led to different ways of categorizing these naturally occurring compounds (Liu, 2004; Tsao, 2010). Polyphenols differ by their source of origin, chemical structure and biological effects (Liu, 2004; Tsao, 2010; Bellik et al., 2012a; Ebrahimi and Schluesener, 2012). They are secondary plant metabolites produced to aid in the defense mechanism against herbivores, insects, ultraviolet radiation, and microorganisms (Yoon and Baek, 2005; Tsao, 2010). More than 8000 plant polyphenols are currently known and among them more than 4000 flavonoids have been identified (Tsao, 2010). Some polyphenols lead to growth inhibition in laboratory animals (Ebrahimi and Schluesener, 2012). However, epidemiological data suggest that intake of small amounts of polyphenols in foods and beverages has a potent effect on reducing chronic diseases (Gotsis et al., 2015). There are studies that have investigated the effectiveness of fruit and vegetable rich diets such as the Mediterranean diet against degenerative diseases, indicating that these diets lead to a reduced incidence of degenerative and age-related diseases including cancer, cardiovascular diseases and neurodegenerative diseases (Calabrese et al., 2003; Yoon and Baek, 2005; Pan et al., 2010; Sofi et al., 2010; Bellik et al., 2012b; Vetrani et al., 2013; Gunawardena et al., 2014).
Anti-Inflammatory Activity of Plant Polyphenols
Many plant polyphenolic compounds including curcumin, apigenin, quercetin, (E)-cinnamaldehyde and (E) -resveratrol have been shown to have anti-inflammatory activities in cell culture studies (Gautam and Jachak, 2009). Molecular targets of plant polyphenols acting as anti-inflammatory compounds include arachidonic acid (AA) dependent pathways and AA independent pathways. In the AA-dependent pathway, the anti-inflammatory effect of plant polyphenols is related to their ability to inhibit COX (the isoform Cox-2, also regulated by NF-κB), which converts AA into prostaglandins. AA-independent pathways involve AA signaling through nuclear factor-kappa B (NF-κB) (Yoon and Baek, 2005).
Polyphenols have been shown to interfere at two specific sites in the pathway leading from receptors to NF-kB. Some polyphenols inhibit kinases by inhibiting their phosphorylation or ubiquitination and therefore prevent the subsequent degradation of IkB (Ruiz and Haller, 2006). This prevents NF-κB translocation into the nucleus and transcription of pro-inflammatory cytokines. Additionally, inhibition of the interaction of NF-κB subunits with target DNA has also been proposed as a mode of action of anti-inflammatory polyphenols (Ruiz and Haller, 2006). Both modes of action ultimately lead to the inhibition of expression of various NF-κB regulated pro-inflammatory proteins (cytokines, chemokines) and enzymes (iNOS, COX-2).
Among the polyphenols that have been shown to modulate pro-inflammatory gene expression are curcumin (Jobin et al., 1999), apigenin (Wang et al., 2014), resveratrol (Kundu et al., 2006), quercetin (Endale et al., 2013), silymarin (Saliou et al., 1998) cinnamaldehyde (Reddy et al., 2004), pathenolode (Saadane et al., 2007), ergolide (Chun et al., 2007), 2β,5-epoxy-5,10-dihydroxy-6α-angeloyloxy-9β-isobutyloxy-germacran-8α,12-olide (Lee et al., 2011), andalusol (Heras et al., 1999), ent-kaur-16-ene-19-oic acid (Wu et al., 2013), kamebanin (Hwang et al., 2001), kamebacetal A (Hwang et al., 2001), kamebakaurin (Hwang et al., 2001), excisanin A (Hwang et al., 2001), hypoestoxide(Ojo-Amaize et al., 2001), helenalin (Lyss et al., 1997), pristimerin (Tiedemann et al., 2009), epigallocatechin gallate (Kim et al., 2010), avicin (Haridas et al., 2001), capsaicin (Singh et al., 1996), and oleandrin (Sreenivasan et al., 2003), just to name a few. In this opinion paper, we will focus on curcumin as the prime example as the manuscript format does not allow to elaborate on the specific targets of each of the compounds in the NF-κB pathway.
Curcumin is a Prototype Inhibitor of NF-κB Signaling Acting Upstream of IκB
Curcumin occurs naturally in the root of plant Curcuma longa which belongs to the Zingiberaceae family. The root of this plant is powdered and used in cooking for centuries in Asian countries. It has also been used extensively in traditional Indian and Chinese medicine to treat diabetic wounds, hepatic disorders, sinusitis and rheumatism (Chainani-Wu, 2003).
Curcumin has been shown to have various anti-inflammatory properties. Curcumin has a broad cytokine-suppressive anti-inflammatory action, it down-regulates the expression of cyclooxygenase-2 (COX-2), lipoxygenase, and inducible nitric oxide synthase (iNOS) enzymes. Curcumin also inhibits the production of the pro-inflammatory cytokines such as TNF-α, IL-1, -2, -6, -8, and -12, and the neurotoxic factors in LPS-stimulated monocytes and alveolar macrophages (Abe et al., 1999). Furthermore, the pharmacokinetic properties of curcumin are also favorable. Curcumin crosses the brain-blood-barrier and bioavailable curcumin preparations (orally applied) can achieve therapeutic concentrations in the brain. For example, brain levels of the curcuminoids reached concentrations of up to 3 μM for curcumin, and up to 6 μM for tetrahydrocurcumin (TC). The EC50 value for iNOS mRNA inhibition in vivo was 1.2 and 0.7 μM for curcumin and TC, respectively.
Inhibition of NF-κB signaling is the best described mechanism of anti-inflammatory action of curcumin (Ambegaokar et al., 2003; Shakibaei et al., 2007). It has been shown that curcumin inhibits the phosphorylation and degradation of IκBα and the subsequent translocation of the p65 subunit of NF-κB to the nucleus (Singh and Aggarwal, 1995). Although the exact target is not known, the study indicates that curcumin interferes with the NF-κB pathway upstream of IκBα phosphorylation (Figure 1). In a further study in intestinal epithelial cells, curcumin has also been shown to block a signal leading to IKK activation (Jobin et al., 1999).
Conclusions
We suggest that inhibitors of NF-kB activation should have the potential to prevent and delay the onset or even treat Alzheimer's disease (Bremner and Heinrich, 2002). However, clinical trials are needed to confirm the effectiveness of some of these plant-based inhibitors of NF-κB pathway as a treatment option for Alzheimer's disease. Nonetheless, in preparation of those trials, critical pharmacokinetic parameters have to be addressed beforehand. Comprehensive investigation models with multidisciplinary approach, including epidemiological, clinical and cellular molecular studies should be employed to ensure that the doses extrapolated from the in vitro data determined in cell cultures have pharmacological relevance (e.g., the tissue concentration matching the EC50 values). Their efficacy has to be tested in animal models of AD, which exhibits some degree of chronic inflammation generated by the pathological deposits such as beta-amyloid or tau, or in a “pure” animal model of chronic neuroinflammation such as the IL-1β or IL-6 overexpressing mouse (Ebrahimi and Schluesener, 2012; Millington et al., 2014). If these animal studies are successful, and show not only a decrease in NF-κB regulated pro-inflammatory mediators, but also an improvement in cognition and memory, then plant polyphenols might have the potential to be the first disease modifying drugs for the treatment of Alzheimer's disease.
Conflict of Interest Statement
The authors declare that the research was conducted in the absence of any commercial or financial relationships that could be construed as a potential conflict of interest.
Acknowledgments
We thank Dr. David Mahns for inspiring guidance. The support of the University of Western Sydney with HDR funding is acknowledged.
References
Abe, Y., Hashimoto, S., and Horie, T. (1999). Curcumin inhibition of inflammatory cytokine production by human peripheral blood monocytes and alveolar macrophages. Pharmacol. Res. 39, 41–47. doi: 10.1006/phrs.1998.0404
Ambegaokar, S. S., Wu, L., Alamshahi, K., Lau, J., Jazayeri, L., Chan, S., et al. (2003). Curcumin inhibits dose-dependently and time-dependently neuroglial cell proliferation and growth. Neuro Endocrinol. Lett. 24, 469–473.
Barnes, P. J. (1997). Nuclear factor-kappa B. Int. J. Biochem. Cell Biol. 29, 867–870. doi: 10.1016/S1357-2725(96)00159-8
Bellik, Y., Boukraa, L., Alzahrani, H. A., Bakhotmah, B. A., Abdellah, F., Hammoudi, S. M., et al. (2012a). Molecular mechanism underlying anti-inflammatory and anti-allergic activities of phytochemicals: an update. Molecules 18, 322–353. doi: 10.3390/molecules18010322
Bellik, Y., Hammoudi, S. M., Abdellah, F., Iguer-Ouada, M., and Boukraa, L. (2012b). Phytochemicals to prevent inflammation and allergy. Recent Pat. Inflamm. Allergy Drug Discov. 6, 147–158. doi: 10.2174/187221312800166886
Brasier, A. R. (2006). The NF-kappaB regulatory network. Cardiovasc. Toxicol. 6, 111–130. doi: 10.1385/CT:6:2:111
Bremner, P., and Heinrich, M. (2002). Natural products as targeted modulators of the nuclear factor-kappaB pathway. J. Pharm. Pharmacol. 54, 453–472. doi: 10.1211/0022357021778637
Calabrese, V., Scapagnini, G., Colombrita, C., Ravagna, A., Pennisi, G., Giuffrida Stella, A. M., et al. (2003). Redox regulation of heat shock protein expression in aging and neurodegenerative disorders associated with oxidative stress: a nutritional approach. Amino Acids 25, 437–444. doi: 10.1007/s00726-003-0048-2
Carlsen, M. H., Halvorsen, B. L., Holte, K., Bohn, S. K., Dragland, S., Sampson, L., et al. (2010). The total antioxidant content of more than 3100 foods, beverages, spices, herbs and supplements used worldwide. Nutr. J. 9:3. doi: 10.1186/1475-2891-9-3
Castello, M. A., Jeppson, J. D., and Soriano, S. (2014). Moving beyond anti-amyloid therapy for the prevention and treatment of Alzheimer's disease. BMC Neurol. 14:169. doi: 10.1186/s12883-014-0169-0
Chainani-Wu, N. (2003). Safety and anti-inflammatory activity of curcumin: a component of tumeric (Curcuma longa). J. Altern. Comp. Med. 9, 161–168. doi: 10.1089/107555303321223035
Chun, J. K., Seo, D. W., Ahn, S. H., Park, J. H., You, J. S., Lee, C. H., et al. (2007). Suppression of the NF-kappaB signalling pathway by ergolide, sesquiterpene lactone, in HeLa cells. J. Pharm. Pharmacol. 59, 561–566. doi: 10.1211/jpp.59.4.0011
Ebrahimi, A., and Schluesener, H. (2012). Natural polyphenols against neurodegenerative disorders: potentials and pitfalls. Ageing Res. Rev. 11, 329–345. doi: 10.1016/j.arr.2012.01.006
Endale, M., Park, S. C., Kim, S., Kim, S. H., Yang, Y., Cho, J. Y., et al. (2013). Quercetin disrupts tyrosine-phosphorylated phosphatidylinositol 3-kinase and myeloid differentiation factor-88 association, and inhibits MAPK/AP-1 and IKK/NF-kappaB-induced inflammatory mediators production in RAW 264.7 cells. Immunobiology 218, 1452–1467. doi: 10.1016/j.imbio.2013.04.019
Figuera-Losada, M., Rojas, C., and Slusher, B. S. (2014). Inhibition of microglia activation as a phenotypic assay in early drug discovery. J. Biomol. Screen. 19, 17–31. doi: 10.1177/1087057113499406
Gautam, R., and Jachak, S. M. (2009). Recent developments in anti-inflammatory natural products. Med. Res. Rev. 29, 767–820. doi: 10.1002/med.20156
Ghosh, S., and Karin, M. (2002). Missing pieces in the NF-kappaB puzzle. Cell 109(Suppl.), S81–S96. doi: 10.1016/S0092-8674(02)00703-1
Gil-Bea, F. J., Gerenu, G., Aisa, B., Kirazov, L. P., Schliebs, R., and Ramirez, M. J. (2012). Cholinergic denervation exacerbates amyloid pathology and induces hippocampal atrophy in Tg2576 mice. Neurobiol. Dis. 48, 439–446. doi: 10.1016/j.nbd.2012.06.020
Gilmore, T. D. (2006). Introduction to NF-kappaB: players, pathways, perspectives. Oncogene 25, 6680–6684. doi: 10.1038/sj.onc.1209954
Gotsis, E., Anagnostis, P., Mariolis, A., Vlachou, A., Katsiki, N., and Karagiannis, A. (2015). Health benefits of the mediterranean diet: an update of research over the last 5 years. Angiology 66, 304–318. doi: 10.1177/0003319714532169
Gunawardena, D., Shanmugam, K., Low, M., Bennett, L., Govindaraghavan, S., Head, R., et al. (2014). Determination of anti-inflammatory activities of standardised preparations of plant- and mushroom-based foods. Eur. J. Nutr. 53, 335–343. doi: 10.1007/s00394-013-0531-9
Haridas, V., Arntzen, C. J., and Gutterman, J. U. (2001). Avicins, a family of triterpenoid saponins from Acacia victoriae (Bentham), inhibit activation of nuclear factor-kappaB by inhibiting both its nuclear localization and ability to bind DNA. Proc. Natl. Acad. Sci. U.S.A. 98, 11557–11562. doi: 10.1073/pnas.191363498
Heras, B. N. A., Jose, M., Guerra, D., Bermejo, P., Castrillo, A., Bosca, L., et al. (1999). Inhibition of NOS-2 expression in macrophages through the inactivation of NF-kB by andalusol. Br. J. Pharmacol. 128, 605–612. doi: 10.1038/sj.bjp.0702844
Hoffmann, A., Natoli, G., and Ghosh, G. (2006). Transcriptional regulation via the NF-kappaB signaling module. Oncogene 25, 6706–6716. doi: 10.1038/sj.onc.1209933
Hwang, B. Y., Lee, J. H., Koo, T. H., Kim, H. S., Hong, Y. S., Ro, J. S., et al. (2001). Kaurane diterpenes from Isodon japonicus inhibit nitric oxide and prostaglandin E2 production and NF-kappaB activation in LPS-stimulated macrophage RAW264.7 cells. Planta Med. 67, 406–410. doi: 10.1055/s-2001-15808
Jobin, C., Bradham, C. A., Russo, M. P., Juma, B., Narula, A. S., Brenner, D. A., et al. (1999). Curcumin blocks cytokine-mediated NF-kappa B activation and proinflammatory gene expression by inhibiting inhibitory factor I-kappa B kinase activity. J. Immunol. 163, 3474–3483.
Kim, J., Lee, H. J., and Lee, K. W. (2010). Naturally occurring phytochemicals for the prevention of Alzheimer's disease. J. Neurochem. 112, 1415–1430. doi: 10.1111/j.1471-4159.2009.06562.x
Kundu, J. K., Shin, Y. K., Kim, S. H., and Surh, Y. J. (2006). Resveratrol inhibits phorbol ester-induced expression of COX-2 and activation of NF-kappaB in mouse skin by blocking IkappaB kinase activity. Carcinogenesis 27, 1465–1474. doi: 10.1093/carcin/bgi349
Latta, C. H., Brothers, H. M., and Wilcock, D. M. (2014). Neuroinflammation in Alzheimer's disease; A source of heterogeneity and target for personalized therapy. Neuroscience. doi: 10.1016/j.neuroscience.2014.09.061. [Epub ahead of print].
Lawrence, T. (2009). The nuclear factor NF-kappaB pathway in inflammation. Cold Spring Harb. Perspect. Biol. 1:a001651. doi: 10.1101/cshperspect.a001651
Lee, H. J., Jung, H., Kwon, J., Li, H., Lee da, Y., Lim, H. J., et al. (2011). A germacranolide sesquiterpene lactone suppressed inducible nitric oxide synthase by downregulating NF-kappaB activity. Can. J. Physiol. Pharmacol. 89, 232–237. doi: 10.1139/Y11-004
Liu, R. H. (2004). Potential synergy of phytochemicals in cancer prevention: mechanism of action. J. Nutr. 134(12 Suppl.), 3479S–3485S.
Lyss, G., Schmidt, T. J., Merfort, I., and Pahl, H. L. (1997). Helenalin, an anti-inflammatory sesquiterpene lactone from Arnica, selectively inhibits transcription factor NF-kappaB. Biol. Chem. 378, 951–961. doi: 10.1515/bchm.1997.378.9.951
Millington, C., Sonego, S., Karunaweera, N., Rangel, A., Aldrich-Wright, J. R., Campbell, I. L., et al. (2014). Chronic neuroinflammation in Alzheimer's disease: new perspectives on animal models and promising candidate drugs. Biomed Res. Int. 2014:309129. doi: 10.1155/2014/309129
Mogensen, T. H. (2009). Pathogen recognition and inflammatory signaling in innate immune defenses. Clin. Microbiol. Rev. 22, 240–273. doi: 10.1128/CMR.00046-08
Ojo-Amaize, E. A., Kapahi, P., Kakkanaiah, V. N., Takahashi, T., Shalom-Barak, T., Cottam, H. B., et al. (2001). Hypoestoxide, a novel anti-inflammatory natural diterpene, inhibits the activity of IkappaB kinase. Cell. Immunol. 209, 149–157. doi: 10.1006/cimm.2001.1798
Pan, M. H., Lai, C. S., and Ho, C. T. (2010). Anti-inflammatory activity of natural dietary flavonoids. Food Funct. 1, 15–31. doi: 10.1039/c0fo00103a
Perkins, N. D. (2007). Integrating cell-signalling pathways with NF-kappaB and IKK function. Nat. Rev. Mol. Cell Biol. 8, 49–62. doi: 10.1038/nrm2083
Reddy, A. M., Seo, J. H., Ryu, S. Y., Kim, Y. S., Kim, Y. S., Min, K. R., et al. (2004). Cinnamaldehyde and 2-methoxycinnamaldehyde as NF-kappaB inhibitors from Cinnamomum cassia. Planta Med. 70, 823–827. doi: 10.1055/s-2004-827230
Robinson, S. R., Bishop, G. M., Lee, H. G., and Münch, G. (2004). Lessons from the AN 1792 Alzheimer vaccine: lest we forget. Neurobiol. Aging 25, 609–615. doi: 10.1016/j.neurobiolaging.2003.12.020
Rosenblum, W. I. (2014). Why Alzheimer trials fail: removing soluble oligomeric beta amyloid is essential, inconsistent, and difficult. Neurobiol. Aging 35, 969–974. doi: 10.1016/j.neurobiolaging.2013.10.085
Ruiz, P. A., and Haller, D. (2006). Functional diversity of flavonoids in the inhibition of the proinflammatory NF-kappaB, IRF, and Akt signaling pathways in murine intestinal epithelial cells. J. Nutr. 136, 664–671.
Saadane, A., Masters, S., DiDonato, J., Li, J., and Berger, M. (2007). Parthenolide inhibits IkappaB kinase, NF-kappaB activation, and inflammatory response in cystic fibrosis cells and mice. Am. J. Respir. Cell Mol. Biol. 36, 728–736. doi: 10.1165/rcmb.2006-0323OC
Saliou, C., Rihn, B., Cillard, J., Okamoto, T., and Packer, L. (1998). Selective inhibition of NF-kappaB activation by the flavonoid hepatoprotector silymarin in HepG2. Evidence for different activating pathways. FEBS Lett. 440, 8–12. doi: 10.1016/S0014-5793(98)01409-4
Shakibaei, M., John, T., Schulze-Tanzil, G., Lehmann, I., and Mobasheri, A. (2007). Suppression of NF-kappaB activation by curcumin leads to inhibition of expression of cyclo-oxygenase-2 and matrix metalloproteinase-9 in human articular chondrocytes: implications for the treatment of osteoarthritis. Biochem. Pharmacol. 73, 1434–1445. doi: 10.1016/j.bcp.2007.01.005
Shi, S., Wang, Z., and Qiao, Z. (2013). The multifunctional anti-inflammatory drugs used in the therapy of Alzheimer's disease. Curr. Med. Chem. 20, 2583–2588. doi: 10.2174/0929867311320200006
Singh, S., and Aggarwal, B. B. (1995). Activation of transcription factor NF-kappa B is suppressed by curcumin (diferuloylmethane) [corrected]. J. Biol. Chem. 270, 24995–25000. doi: 10.1074/jbc.270.42.24995
Singh, S., Natarajan, K., and Aggarwal, B. B. (1996). Capsaicin (8-methyl-N-vanillyl-6-nonenamide) is a potent inhibitor of nuclear transcription factor-kappa B activation by diverse agents. J. Immunol. 157, 4412–4420.
Sofi, F., Macchi, C., Abbate, R., Gensini, G. F., and Casini, A. (2010). Effectiveness of the Mediterranean diet: can it help delay or prevent Alzheimer's disease? J. Alzheimers. Dis. 20, 795–801.
Sreenivasan, Y., Sarkar, A., and Manna, S. K. (2003). Oleandrin suppresses activation of nuclear transcription factor-kappa B and activator protein-1 and potentiates apoptosis induced by ceramide. Biochem. Pharmacol. 66, 2223–2239. doi: 10.1016/j.bcp.2003.07.010
Tiedemann, R. E., Schmidt, J., Keats, J. J., Shi, C. X., Zhu, Y. X., Palmer, S. E., et al. (2009). Identification of a potent natural triterpenoid inhibitor of proteosome chymotrypsin-like activity and NF-kappaB with antimyeloma activity in vitro and in vivo. Blood 113, 4027–4037. doi: 10.1182/blood-2008-09-179796
Tsao, R. (2010). Chemistry and biochemistry of dietary polyphenols. Nutrients 2, 1231–1246. doi: 10.3390/nu2121231
Vetrani, C., Costabile, G., Di Marino, L., and Rivellese, A. A. (2013). Nutrition and oxidative stress: a systematic review of human studies. Int. J. Food Sci. Nutr. 64, 312–326. doi: 10.3109/09637486.2012.738651
Wang, J., Liu, Y. T., Xiao, L., Zhu, L., Wang, Q., and Yan, T. (2014). Anti-inflammatory effects of apigenin in lipopolysaccharide-induced inflammatory in acute lung injury by suppressing COX-2 and NF-kB pathway. Inflammation 37, 2085–2090. doi: 10.1007/s10753-014-9942-x
Wu, K., Liu, Y., Lv, Y., Cui, L., Li, W., Chen, J., et al. (2013). Ent-11alpha-hydroxy-15-oxo-kaur-16-en-19-oic-acid induces apoptosis and cell cycle arrest in CNE-2Z nasopharyngeal carcinoma cells. Oncol. Rep. 29, 2101–2108.
Keywords: Alzheimer's disease, cytokine, neutraceuticals, inflammation, disease-modifying drug, treatment
Citation: Karunaweera N, Raju R, Gyengesi E and Münch G (2015) Plant polyphenols as inhibitors of NF-κB induced cytokine production—a potential anti-inflammatory treatment for Alzheimer's disease? Front. Mol. Neurosci. 8:24. doi: 10.3389/fnmol.2015.00024
Received: 16 February 2015; Accepted: 29 May 2015;
Published: 16 June 2015.
Edited by:
Jun Yan, University of Queensland, AustraliaReviewed by:
Mythily Srinivasan, Indiana University, USACopyright © 2015 Karunaweera, Raju, Gyengesi and Münch. This is an open-access article distributed under the terms of the Creative Commons Attribution License (CC BY). The use, distribution or reproduction in other forums is permitted, provided the original author(s) or licensor are credited and that the original publication in this journal is cited, in accordance with accepted academic practice. No use, distribution or reproduction is permitted which does not comply with these terms.
*Correspondence: Gerald Münch,Zy5tdWVuY2hAdXdzLmVkdS5hdQ==