- 1Unit for Degradomics of the Protease Web, Institute of Biochemistry, University of Kiel, Kiel, Germany
- 2Institute for Pathobiochemistry, University Medical Center of the Johannes Gutenberg-University Mainz, Mainz, Germany
The membrane bound metalloprotease meprin β is important for collagen fibril assembly in connective tissue formation and for the detachment of the intestinal mucus layer for proper barrier function. Recent proteomic studies revealed dozens of putative new substrates of meprin β, including the amyloid precursor protein (APP). It was shown that APP is cleaved by meprin β in distinct ways, either at the β-secretase site resulting in increased levels of Aβ peptides, or at the N-terminus releasing 11 kDa, and 20 kDa peptide fragments. The latter event was discussed to be rather neuroprotective, whereas the ectodomain shedding of APP by meprin β reminiscent to BACE-1 is in line with the amyloid hypothesis of Alzheimer's disease, promoting neurodegeneration. The N-terminal 11 kDa and 20 kDa peptide fragments represent physiological cleavage products, since they are found in human brains under different diseased or non-diseased states, whereas these fragments are completely missing in brains of meprin β knock-out animals. Meprin β is not only a sheddase of adhesion molecules, such as APP, but was additionally demonstrated to cleave within the prodomain of ADAM10. Activated ADAM10, the α-secretase of APP, is then able to shed meprin β from the cell surface thereby abolishing the β-secretase activity. All together meprin β seems to be a novel player in APP processing events, even influencing other enzymes involved in APP cleavage.
Introduction
To date, more than 35,000 research articles dealing with the amyloid precursor protein (APP) are annotated in Pubmed (13.10.2016) and most of these papers are related to Alzheimer's disease. Nevertheless, APP is still an enigma in terms of its physiological and pathophysiological functions.
APP is a multi-domain glycosylated type 1 transmembrane protein. Earlier studies reported that the ectodomains of APP family proteins have zinc- (Bush et al., 1993) and copper binding-properties (Simons et al., 2002) and that APP is able to reduce bound Cu2+ to Cu+ (Multhaup et al., 1996). Moreover, APP has been proposed to bind extracellular matrix proteins like heparin and collagen (Small et al., 1994), and to have a receptor-like function (Beher et al., 1996). In this context, it became more and more challenging, whether APP can form cellular cis-dimers (Scheuermann et al., 2001), reminiscent of classical receptor dimerization described for the EGF receptor (Schlessinger, 2002). However, there is accumulating evidence from biochemical and structural data that APP can form homodimers (Scheuermann et al., 2001; Kaden et al., 2009; Isbert et al., 2012) as well as heterodimers with its homologs APLP1 and APLP2 (Soba et al., 2005).
To date, at least three domains have been reported to promote APP dimerization: first the E1 domain containing the N-terminal Growth factor like domain (GFLD) and Copper binding domain (CuBD) (Soba et al., 2005). The second dimerization interface is represented by the E2 domain (amino acids 365–699), the largest subdomain of the APP ectodomain, containing the carbohydrate- and the juxtamembrane region. Crystallographic and X-ray structure modeling revealed that the E2 region can reversibly dimerize in an antiparallel orientation in solution (Wang and Ha, 2004) and it has been reported that binding of extracellular matrix components, such as heparin, to this domain may also regulate dimerization (Gralle et al., 2006). However, in contrast to Wang and colleagues a study by Dulubova and colleagues could not confirm that the E2 domain does dimerize in solution (Dulubova et al., 2004). A third dimerization interface is located at the extracellular juxtamembrane/transmembrane (JM/TM) boundary, where APP contains three consecutive glycine-xxx-glycine (GxxxG) motifs (Munter et al., 2007; Gorman et al., 2008; Kienlen-Campard et al., 2008) one embedded within the Aβ sequence.
Interestingly, detection of APP dimerization in vivo showed a possibility that the efficient processing of APP by α- and β-secretases (see below) may depend on its oligomerization state that results in cooperative effects for these allosteric enzymes (Schmidt et al., 2012).
Although the German psychiatrist Alois Alzheimer was the first to demonstrate a relationship between specific cognitive changes, neurological lesions in the human brain, and clinical history (Alzheimer, 1907), much later the amyloid cascade hypothesis attributed these observations to the presence of the cleavage products of APP in the brain (Hardy and Selkoe, 2002). Alzheimer reported the results of an autopsy on a 55-year-old woman named Auguste Deter and noted the presence of two distinct pathological lesions in Deters brain, which now define Alzheimer's disease (AD): first, the neurofibrillary tangles (NFTs), which accumulate intraneuronal (later shown to be composed of paired helical filaments (PHFs) containing the microtubule-associated protein tau; Goedert et al., 1988, 1989); second, extracellular amyloid deposits in the form of diffuse or neuritic senile plaques (Price et al., 1997). Senile plaques accumulate extracellular and were isolated and purified in 1984 by Glenner and Wong, who showed that it was a ~4 kDa peptide (Aβ), primarily 40 or 42 amino acids in length, which they speculated was cleaved from a larger precursor (Glenner and Wong, 1984). Subsequently, it has been demonstrated that this peptide fragment originated from a larger precursor protein, named the amyloid-β precursor protein (AβPP, or APP as used here) and was characterized from the analysis of a full-length cDNA encoding a translational product of 695 residues (Kang et al., 1987).
Conventional APP Processing
Multiple enzymes have been shown to process APP during its lifetime. The non amyloidogenic pathway, in which APP is cleaved within the sequence of the amyloid peptide by a generally named enzyme group called α-secretase, precludes the formation of the full-length Aβ which is found in the amyloid core of senile plaques (Zheng and Koo, 2006). One other pathway leads to the production of Aβ peptides from its precursor after the initial cleavage by a generally named enzyme group called β-secretase (Hussain et al., 1999; Sinha et al., 1999; Vassar et al., 1999; Yan et al., 1999). The first β–secretase identified was then named β-site APP-cleaving enzyme (BACE-1). BACE-1 is a type I membrane-bound aspartyl protease located in the endosomal/lysosomal compartment (Sinha et al., 1999; Vassar et al., 1999). Cleavage of APP by BACE-1 (Vassar, 2002) occurs between methionine 596 and aspartate 597 of APP695 (Figure 1), producing two fragments, the secreted N-terminal ectodomain sAPPβ and a 10 kDa, 99-amino-acid-long fragment C99, encompassing the Aβ peptide and the remaining C-terminal part. The optimal pH of BACE-1 activity is ~4.5, suggesting that the β-site cleavage of APP occurs preferentially in more acidic compartments, such as in endosomes and lysosomes (Vassar et al., 1999).
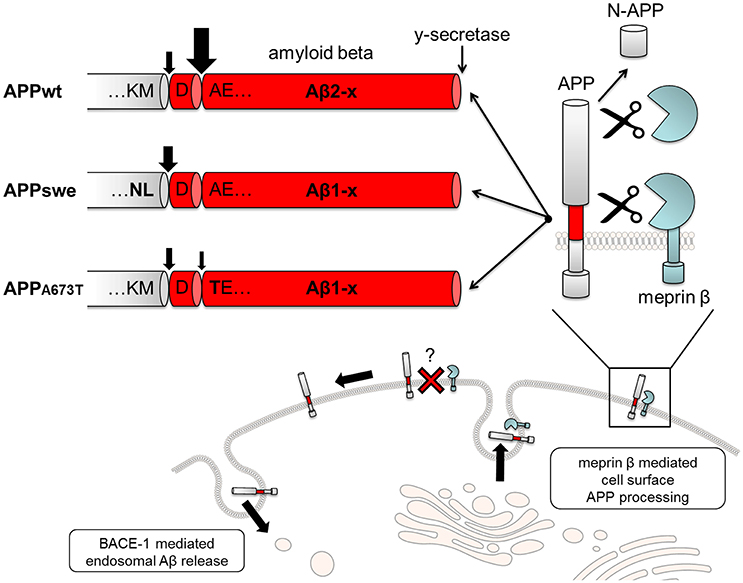
Figure 1. Proteolytic processing of APP by Meprin β. APP is cleaved by meprin β in two distinct ways. On the one hand non-amyloidogenic N-APP fragments are produced, and on the other hand, meprin β acts as a β-secretase, inducing Aβ2-x generation. Remarkably, APPswe completely abolishes Aβ2-x release. The AD protective mutant APPA673T is also much less cleaved by meprin β.
After α- or β-cleavage, the carboxyl terminal fragments (CTFs) of APP, known as αCTF (C83) and βCTF (C99), respectively, remain membrane-associated and are further cleaved by the γ-secretase-complex (Edbauer et al., 2003). The γ-secretase is an aspartyl protease complex (Wolfe et al., 1999), which unlike α- and β-secretases, acts within the membrane and cleaves APP at multiple sites (Zhao et al., 2004), releasing either, Aβ and intracellular C-terminal domain fragments (ICDs) or p3 and ICDs (Figure 1). This process is called regulated intramembrane proteolysis (RIP) (Brown et al., 2000). However, while the two predominant forms of Aβ and p3 terminate at valine 637 (Aβ40 and p3/40) and alanine 639 (Aβ42 and p3/42) (Haass et al., 1992a), some isolated ICDs are shorter than expected and begin at sites 9–10 amino acid downstream of those residues (Gu et al., 2001).
BACE-1 is described to be the major Aβ generating β-secretase (Hussain et al., 1999; Sinha et al., 1999; Vassar et al., 1999; Yan et al., 1999; Lin et al., 2000). This was convincingly shown when a genetic knock-out of the protease in mice abolished Aβ generation almost completely (Luo et al., 2001; Roberds et al., 2001; Dominguez et al., 2005). In accordance to that, BACE-1 was found to be upregulated in brains of sporadic AD patients (Fukumoto et al., 2002). However, there is strong evidence that certain amounts of Aβ are generated independently of BACE-1. This was supported, when using potent BACE-1 inhibitors in vitro and in vivo (Asai et al., 2006; Nishitomi et al., 2006; Hussain et al., 2007; Stanton et al., 2007; Sankaranarayanan et al., 2008). Interestingly, some studies showed that by inhibition of Aβ1-x generating β-secretase activity, alternative N-terminally truncated Aβ peptides increase (Haass et al., 1995; Schrader-Fischer and Paganetti, 1996; Takeda et al., 2004; Schieb et al., 2010; Mattsson et al., 2012). Analysis of Aβ species in BACE-1 knock-out mice likewise revealed that the generation of Aβ1-x peptides was completely abolished while N-terminally truncated Aβ variants could still be generated (Nishitomi et al., 2006). These N-terminally truncated Aβ peptides are also found in the cerebrospinal fluid, brain tissue, and human blood plasma (Wiltfang et al., 2001; Lewczuk et al., 2004; Takeda et al., 2004; Güntert et al., 2006; Lewis et al., 2006; Maler et al., 2007; Murayama et al., 2007). Later it was demonstrated that BACE-1 invariably generates two Aβ variants beginning with the aspartate in p1 or p11, therefore other proteases might account for the production of N-terminally truncated peptides (Citron et al., 1995; Vassar et al., 1999). Indeed, heterogeneity of alternative β-secretase cleavage events has been described (Golde et al., 1992; Haass et al., 1992b; Seubert et al., 1992; Busciglio et al., 1993) leading to alternative Aβ peptides other than Aβ1/11-x (Vigo-Pelfrey et al., 1993; Asami-Odaka et al., 1995; Wang et al., 1996), which could also be found in amyloid plaques in vivo (Masters et al., 1985; Güntert et al., 2006). It is not clear whether N-terminally truncated Aβ species are generated via cleavage of APP by yet unknown proteases or via truncation of Aβ1-x after its γ-secretase mediated release, e.g., by aminopeptidase A (Sevalle et al., 2009). In contrast to further subsequent cleavage of already released Aβ peptides, Cathepsin B (Hook et al., 2005, 2014; Kindy et al., 2012), S and L (Schechter and Ziv, 2011) have been discussed to be directly involved in Aβ generation, acting as alternative β-secretases. The enzymatic cleavage events of cathepsins on APP are not fully understood since some groups showed that cathepsins are rather involved in Aβ degradation lowering total Aβ burden (Mueller-Steiner et al., 2006; Letronne et al., 2016).
The amyloid peptides Aβ2-40/42 cannot be assigned to BACE-1 activity and are most likely generated due to an alternative β-secretase cleaving APP between 672Asp/673Ala (Wiltfang et al., 2001; Schieb et al., 2010, 2011). Aβ2-x might act as a precursor and can likewise be processed to Aβ3-x by the alanyl-aminopeptidase activity of aminopeptidase N (Hosoda et al., 1998). This is supposed to occur even under physiological conditions due to activity of cortical aminopeptidase N (Kuda et al., 1997; Wiltfang et al., 2001). It was also discussed that N-terminally truncated Aβ peptides arise when Aβ is degraded by a variety of Aβ degrading enzymes e.g., myelin basic protein, neprilysin, and angiotensin-converting enzyme (Saido and Leissring, 2012). But until recently no proof about the exact mechanisms leading to N-terminally truncated Aβ variants could be given, which changed by the identification of the metalloprotease meprin β as an alternative β-secretase described below.
Alternative APP Processing
In the last years, more and more focus has been put on modified N-terminally truncated Aβ variants. Increased levels of Aβ2-42 were detected in AD brains (Wiltfang et al., 2001). This is in line with results showing decreased levels of Aβ2-42 in CSF of AD patients (Bibl et al., 2012). Since BACE-1 is not capable in directly generating this peptide, a suggested model for the emergence of N-terminal truncation is the subsequent cleavage of the N-terminus of BACE generated Aβ1-x by either Aβ degrading enzymes like insulin-degrading enzymes (IDE) or neprilysin or the aminopeptidase A (APA) (Arai et al., 1999; Wiltfang et al., 2001; Wang et al., 2006). A candidate directly generating N-terminally truncated Aβ independent of BACE-1 is the metalloprotease meprin β. Meprin β is a multi-domain type I transmembrane protein, member of the astacin family of zinc-endopeptidases that is predominantly present as a dimer at the cell surface (Arolas et al., 2012; Figure 2). In 2011 meprin β was introduced as an alternative enzyme involved in APP processing (Jefferson et al., 2011). In 2012, N-terminally truncated Aβ2-40 peptides generated by meprin β (Figure 1), dependent on subsequent cleavage of the γ-secretase, but independent of BACE-1, were detected in supernatants of overexpressing cells (Bien et al., 2012). Interestingly, increased mRNA levels of meprin β were measured in AD brain homogenates supporting a potential role for this enzyme in neurodegeneration. Various posttranslational modifications of Aβ peptides have been described ranging from oxidation (Hou et al., 2002; Palmblad et al., 2002) to phosphorylation (Kumar et al., 2011, 2012), nitration (Kummer et al., 2011), glycosylation (Halim et al., 2011) or pyroglutamation of Glu3 of Aβ3-40 (Russo et al., 2002; Wittnam et al., 2012). These modifications have been shown to have an effect on the properties of the peptide. The oxidation at Met35 for example impedes the formation of protofibrils and fibrils from monomers (Hou et al., 2002). Nitration and pyroglutamation both increase the aggregation of Aβ (Schilling et al., 2004; Kummer et al., 2011). Meprin β was demonstrated to cleave APP at p3 position in a peptide derived in vitro assay (Bien et al., 2012), which would eventually lead to the release of Aβ3-40 peptides, containing an N-terminal pyroglumate modification. This cleavage site for meprin β, however, was so far only found in peptide cleavage assays and not in coexpression experiments with full length APP in cellular systems (Bien et al., 2012).
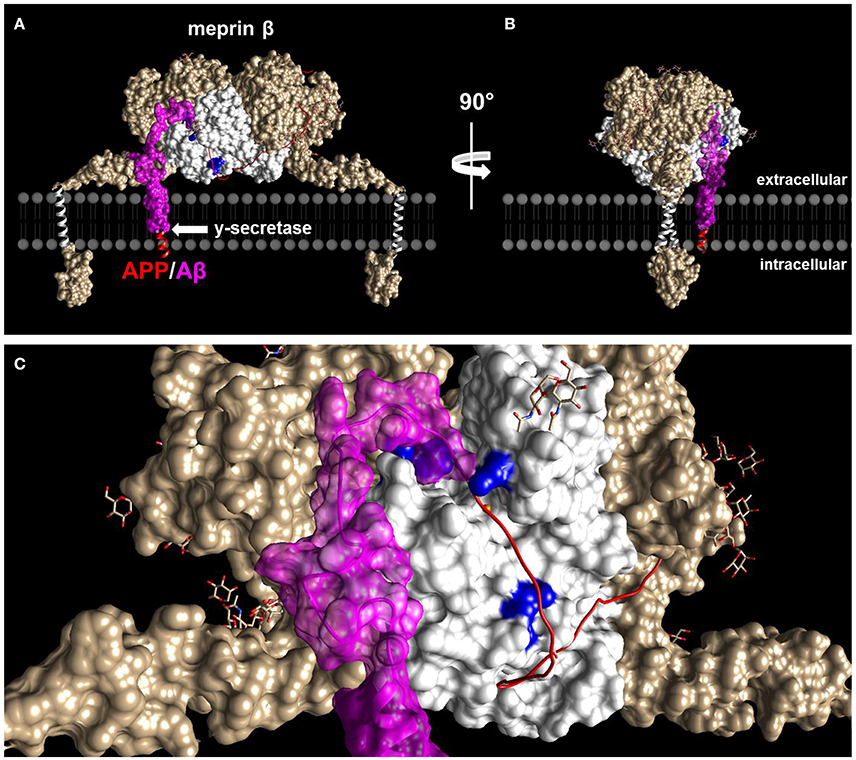
Figure 2. Structural features of meprin β and APP interaction. (A) Model of dimeric membrane bound meprin β (white and brownish) based on the crystal structure of the ectodomain (PDB 4GWN) in complex with part of the APP (red/magenta). (B) As in (A) but turned by 90° to the right. (C) Close up of the active site cleft of meprin β as shown in (A). Positively charged amino acid residues important for the cleavage specificity are highlighted in blue. Part of the APP that builds the Aβ peptide is displayed as surface model. Glycans in meprin β are depicted as stick models.
Several mutations within the APP sequence have been shown to have an impact on β-secretase cleavage by BACE-1. The recently described APP mutation A673T that has been shown to protect against AD as well as against cognitive decline in the elderly independent of AD was analyzed (Jonsson et al., 2012). The mutation is located at p2 of Aβ (Aβ-A/T) and has been shown to reduce BACE-1 mediated Aβ generation by 40% using synthetic peptides as substrates. Moreover, a significantly decreased Aβ production in human APP A673T-overexpressing primary neurons has been observed (Benilova et al., 2014; Maloney et al., 2014). Additionally, a decreased aggregation propensity of Aβ-A/T could be measured, which is showing the complexity of the protective effects of the substitution. As meprin β was shown to be involved in APP processing close to the BACE-1 cleavage site Schoenherr and colleagues investigated the effect of the APP A673T mutation on meprin β activity (Schönherr et al., 2016). The authors revealed a significant decrease of ~70% in the Aβ2-40/1-40 ratio compared to wildtype APP sequence in meprin β transfected cells and in a peptide cleavage assay using the APP A673T constructs. The decreased cleavage of APP by meprin β in the presence of the A673T substitution can nicely be explained by the cleavage preference of meprin β revealed by proteomics (Becker-Pauly et al., 2011). Here, a preference of alanine over threonine in P1' position was observed. As the activity of meprin β on APP processing varies with mutations around the original BACE-1 cleavage site Schoenherr and colleagues investigated whether the Swedish mutation of APP (K670N/M671L; APPswe) may affect meprin β cleavage activity. Surprisingly, Aβ2-x variants were completely missing in cells overexpressing meprin β and APP bearing the Swedish double mutation K670N/M671L (APPswe) which is located in close vicinity of the β-secretase cleavage site (Figure 1). This clearly shows a significant influence of amino acid substitutions around the β-secretase cleavage site for meprin β mediated Aβ generation.
Although BACE-1 is clearly the most prominent enzyme responsible for the generation of Aβ1-40 and Aβ1-42 peptides from the APP wildtype or APPswe sequences, meprin β may be responsible for generating small amounts of N-terminal truncated Aβ2-40 and Aβ2-42 peptides. N-terminal truncated Aβ peptides are almost exclusively generated by meprin β from the complete APP wildtype sequences or from APP carrying familiar Alzheimer disease mutations at the γ–secretase cleavage site but bearing the wildtype sequence around the β-cleavage site.
AD Mouse Models
To analyze AD in an in vivo situation, different mouse models were already generated in the 1990's. However, these mouse models always show potential weaknesses which have to be considered before translating the results obtained from the mouse studies into the human situation. The major drawback is that cleavage of endogenous murine APP via the amyloidogenic pathway was never observed to lead to an AD-like phenotype. Hence, overexpression of different human APP forms in mice was and still is the most promising way to establish appropriate animal models. There are common models to study Aβ plaque pathology that all bear the APP Swedish mutation, such as 5xFAD mice, carrying mutations in the APP and PSEN1 genes [APP K670N/M671L (Swedish), APP I716V (Florida), APP V717I (London), PSEN1 M146L, and PSEN1 L286V; (Oakley et al., 2006)], J20 mice, carrying mutations only in the APP gene [K670N/M671L (Swedish) and the APP V717F (Indiana; Mucke et al., 2000)], or the 3xTg mice, carrying mutations in the APP, PSEN1, and the MAPT genes [K670N/M671L (Swedish), MAPT P301L, and PSEN1 M146V; (Oddo et al., 2003)]. These models all manifest an amyloid pathology although varying between animal models as well as differential learning and memory deficits. Thus, they appear to be appropriate models to mimic AD phenotypes at first sight. Notably, the human sequence of the Swedish familiar Alzheimer disease mutation (APPswe) is used in almost all AD animal models as it serves as a better substrate for BACE-1, thereby increasing production of total Aβ and specifically 1-X Aβ peptides (Citron et al., 1992; Cai et al., 1993). However, in light of the result put forward by Schoenherr and colleagues Aβ2-42 peptides which have been detected in brains of AD patients will not be generated in these mouse models. Therefore, it is likely that the actual effect of meprin β has been overlocked in many studies focusing on APP processing. This issue must be considered when analyzing the results from the ongoing clinical trials, using BACE-1 inhibitors for the treatment of AD patients.
Meprin β and APP Beyond AD
As mentioned above in it has been shown that meprin β additionally cleaves APP apart from the Aβ sequence resulting in N-terminal APP fragments (NTF) (Jefferson et al., 2011). These fragments were also detected in human brain homogenates suggesting that this interaction not only occurs in overexpressing cell systems, but probably also under endogenous levels in the human brain. The in vivo relevance for this proteolytic event was further supported by analyzing brain lysates from meprin β deficient mice where this particular N-APP cleavage was abolished (Jefferson et al., 2011). Interestingly, Tessier-Lavigne and colleagues showed that an N-terminal APP fragment found in AD patients binds the death receptor 6 (DR6) thereby inducing neurodegeneration (Nikolaev et al., 2009). Thus, it was speculated whether meprin β might be the responsible protease in this regard. However, purification and characterization of the meprin β generated N-APP fragments showed neither negative nor positive influence on neuronal cell viability (Jefferson et al., 2011). Therefore, it is likely that APP cleavage by meprin β in the N-terminal region has rather protective function.
Physiological Functions of Meprin β
Meprin β is strongly expressed in the intestinal epithelium and in kidney proximal tubular cells, and to minor levels in several other tissues, e.g., in skin, certain immune cells, and the brain (Broder et al., 2013). Besides many potential substrates analyzed in vitro only few in vivo functions of meprin β have been reported so far. In the intestine, where meprin β is found at the apical site of epithelial cells, the protease is responsible for the detachment of the mucus by cleaving mucin 2, an important step for proper barrier function (Schütte et al., 2014). Along the same line, meprin β cleaves type 1 pili of adherent-invasive E. coli, thereby preventing colonization of these bacteria in the ileal mucosa of Crohn's disease patients (Vazeille et al., 2011). Several other studies provide evidence for an important immunological function of meprin β (Banerjee and Bond, 2008; Bylander et al., 2008; Banerjee et al., 2009, 2011; Yura et al., 2009; Broder and Becker-Pauly, 2013; Zhang et al., 2015). As known for other members of the astacin family, namely BMP-1 (bone morphogenetic protein 1) and tolloids, meprin β is involved in the maturation of procollagens I and III (Kronenberg et al., 2010; Broder et al., 2013; Prox et al., 2015). Collagen, the most abundant protein in human body, is a crucial factor for the integrity of connective tissue, tendon, and bone. To prevent fibril assembly already in intracellular compartments, collagens contain C- and N-terminal prodomains that need to be removed proteolytically by extracellular proteases. Meprin β is such an enzyme, and Mep1b−/− mice show severe impairments of the connective tissue in skin characterized by reduced tensile strength and decreased collagen deposition (Broder et al., 2013). On the other hand, under pathological conditions, overexpression of meprin β is associated with fibrotic diseases, such as keloids of the skin (Kronenberg et al., 2010) and pulmonary hypertension (PH) (Biasin et al., 2014). PH is a severe fibrotic condition of the lung with very bad prognosis for the patients that die 2–3 years after diagnosis. In genetic screens of lung tissues from patients and a mouse model of PH meprin β was found amongst the most up-regulated genes (Biasin et al., 2014). Here, AP-1 transcription factor complex was identified as an inducer of Mep1b mRNA expression. Whether meprin β is only involved in the progression of fibrosis by collagen maturation and deposition, or if the protease also contributes to the onset of the disease as a pro-inflammatory enzyme has to be further investigated.
Regulation of Meprin β
As meprin β associated pathologies, such as fibrosis, cancer, and AD, are mostly based on increased expression and activity of the protease, information about the regulation of the enzyme is important.
Activation
Meprin β is expressed as an inactive zymogen and requires proteolytic removal of its propeptide to gain full enzymatic activity. Several tryptic serine proteases have been identified as activators of latent meprin β, amongst them kallikreins (KLKs) 4, 5, and 8, as well as pancreatic trypsin (Ohler et al., 2010). The latter is supposed to be the physiological activator in the intestine, thereby contributing to the mucus-cleaving activity of meprin β (Schütte et al., 2014), whereas KLKs may rather be important in skin and mesenchymal tissues (Ohler et al., 2010). Based on the crystal structure of the ectodomain of human meprin β it became evident that the activation site at amino acid position Arg61 is in very close proximity to the cell surface (Arolas et al., 2012). Therefore, it was doubtful whether the previously described soluble tryptic activators, which were identified in in vitro assays using recombinant soluble promeprin β, are capable of activating the membrane bound meprin β. Indeed, not even trypsin was able to cleave off the propeptide of full length meprin β, which led to the assumption that possible candidates are most likely membrane bound serine proteases. In this regard, matriptase-2 (MT-2), a type 2 transmembrane protein, was found to fully activate meprin β at the cell surface (Jäckle et al., 2015). Consequently, MT-2 mediated activation of meprin β resulted in increased APP shedding and subsequently decreased sAPPα levels. If this proteolytic interaction may have impact on neurodegenerative disorders has to be shown. Surprisingly, however, in a different study MT-2 was found to directly cleave neuronal APP695, but was effectively inhibited by the Kunitz protease inhibitor (KPI) domain present in other APP isoforms (APP751 and APP770) from the periphery (Beckmann et al., 2016). Of note, the additional domains in APP751 (KPI) and APP770 (KPI/OX2) do not lead to altered proteolytic processing by meprin β (Jefferson et al., 2011). This demonstrates how complex the proteolytic processing of APP can be and how important it is to elucidate the time-dependent and site-specific cleavage events with regard to the different proteases, such as ADAM10, BACE-1, meprin β, or MT-2.
Inhibition
The tissue inhibitors of metalloproteinases (TIMPs) are effective regulators of the catalytic activity of matrix metalloproteases (MMPs) and ADAMs (Yamamoto et al., 2015). TIMPs, however, do not inhibit meprin β, and so far only one rather unspecific endogenous inhibitor was identified, namely fetuin-A (Kruse et al., 2004; Hedrich et al., 2010). Interestingly, calcium was found to inhibit the proteolytic activity of meprin β by binding to a cluster of negatively charged amino acids in close proximity to the active site, thereby inducing conformational changes (Arnold et al., 2015). However, the inhibition constant of calcium for meprin β is about 11 mM, which resembles the concentration in the endoplasmic reticulum and not at the cell surface. The amino acid residues forming the calcium binding site in meprin β contribute to correct folding of the protease. Mutations within the calcium binding site resulted in protein that stacks to the ER and is not properly secreted (Arnold et al., 2015). The calcium concentration needed for the inhibition of meprin β is rather not relevant for extracellular inhibition, at least under physiological conditions. Thus, regulation of meprin β's activity must occur on a different level. One possibility is the ectodomain shedding of meprin β by ADAM10 or ADAM17 (Jefferson et al., 2013). Very importantly, only membrane bound meprin β is capable of generating aggregation prone Aβ2-x peptides and not the shed solubilized protease (Bien et al., 2012). Therefore, ADAM10 does not only prevent Aβ release by cleaving APP at the α-secretase site, but additionally by the shedding of meprin β and thereby preventing its activity toward the β-secretase site.
Localization
As mentioned above, shedding of APP by meprin β occurs predominantly at the cell surface and thus competes with ADAM10 for the substrate (Schönherr et al., 2016). Recent studies demonstrated that ADAM10 localization and maturation is influenced by tetraspanins (TSPANs), building microdomains of protein clusters at the cell surface (Prox et al., 2012). In a yeast-two-hybrid approach TSPAN8 was identified as an interaction partner of meprin β, which was further proven by split-RFP and luciferase complementation assays (Schmidt et al., 2016). It was further demonstrated that APP together with meprin β is located in TSPAN8 enriched microdomains. However, overexpression of TSPAN8 had no obvious influence on meprin β activity and APP cleavage. Nevertheless, orchestration of proteases and substrates at the cell surface by regulatory factors has to be further studied to fully understand the complex proteolytic processing of APP by different enzymes.
Concluding, the protease meprin β appears as an important candidate for further studies on APP processing and Aβ generation and may have a contributing role to the physiological and pathophysiological function of APP itself.
Author Contributions
All authors (CP, CB) contributed to the concept and drafting the work. The authors ensure that questions related to the accuracy or integrity of any part of the work are appropriately investigated and resolved.
Funding
This work was supported by the Deutsche Forschungsgemeinschaft, grant BE 4086/2-2 (to CB), and grant PI 379 6-2 (to CP).
Conflict of Interest Statement
The authors declare that the research was conducted in the absence of any commercial or financial relationships that could be construed as a potential conflict of interest.
References
Alzheimer, A. (1907). Über eine eigenartige Erkrankung der Hirnrinde. Allgemeine Zeitschrift Psychiatr. Physc. Gerichtliche Medizin. 64, 146–148.
Arai, T., Akiyama, H., Ikeda, K., Kondo, H., and Mori, H. (1999). Immunohistochemical localization of amyloid beta-protein with amino-terminal aspartate in the cerebral cortex of patients with Alzheimer's disease. Brain Res. 823, 202–206. doi: 10.1016/S0006-8993(98)01366-3
Arnold, P., Schmidt, F., Prox, J., Zunke, F., Pietrzik, C., Lucius, R., et al. (2015). Calcium negatively regulates meprin beta activity and attenuates substrate cleavage. FASEB J. 29, 3549–3557. doi: 10.1096/fj.15-272310
Arolas, J. L., Broder, C., Jefferson, T., Guevara, T., Sterchi, E. E., Bode, W., et al. (2012). Structural basis for the sheddase function of human meprin β metalloproteinase at the plasma membrane. Proc. Natl. Acad. Sci. U.S.A. 109, 16131–16136. doi: 10.1073/pnas.1211076109
Asai, M., Hattori, C., Iwata, N., Saido, T. C., Sasagawa, N., Szabó, B., et al. (2006). The novel beta-secretase inhibitor KMI-429 reduces amyloid beta peptide production in amyloid precursor protein transgenic and wild-type mice. J. Neurochem. 96, 533–540. doi: 10.1111/j.1471-4159.2005.03576.x
Asami-Odaka, A., Ishibashi, Y., Kikuchi, T., Kitada, C., and Suzuki, N. (1995). Long amyloid beta-protein secreted from wild-type human neuroblastoma IMR-32 cells. Biochemistry 34, 10272–10278. doi: 10.1021/bi00032a022
Banerjee, S., and Bond, J. S. (2008). Prointerleukin-18 is activated by meprin beta in vitro and in vivo in intestinal inflammation. J. Biol. Chem. 283, 31371–31377. doi: 10.1074/jbc.M802814200
Banerjee, S., Jin, G., Bradley, S. G., Matters, G. L., Gailey, R. D., Crisman, J. M., et al. (2011). Balance of meprin A and B in mice affects the progression of experimental inflammatory bowel disease. Am. J. Physiol. Gastrointest. Liver Physiol. 300, G273–G282. doi: 10.1152/ajpgi.00504.2009
Banerjee, S., Oneda, B., Yap, L. M., Jewell, D. P., Matters, G. L., Fitzpatrick, L. R., et al. (2009). MEP1A allele for meprin A metalloprotease is a susceptibility gene for inflammatory bowel disease. Mucosal Immunol. 2, 220–231. doi: 10.1038/mi.2009.3
Becker-Pauly, C., Barré, O., Schilling, O., Auf dem Keller, U., Ohler, A., Broder, C., et al. (2011). Proteomic analyses reveal an acidic prime side specificity for the astacin metalloprotease family reflected by physiological substrates. Mol. Cell. Proteomics 10, M111 009233. doi: 10.1074/mcp.M111.009233
Beckmann, A. M., Glebov, K., Walter, J., Merkel, O., Mangold, M., Schmidt, F., et al. (2016). The intact Kunitz domain protects the amyloid precursor protein from being processed by matriptase-2. Biol. Chem. 397, 777–790. doi: 10.1515/hsz-2015-0263
Beher, D., Hesse, L., Masters, C. L., and Multhaup, G. (1996). Regulation of amyloid protein precursor (APP) binding to collagen and mapping of the binding sites on APP and collagen type I. J. Biol. Chem. 271, 1613–1620. doi: 10.1074/jbc.271.3.1613
Benilova, I., Gallardo, R., Ungureanu, A. A., Castillo Cano, V., Snellinx, A., Ramakers, M., et al. (2014). The Alzheimer disease protective mutation A2T modulates kinetic and thermodynamic properties of amyloid-beta (Abeta) aggregation. J. Biol. Chem. 289, 30977–30989. doi: 10.1074/jbc.M114.599027
Biasin, V., Marsh, L. M., Egemnazarov, B., Wilhelm, J., Ghanim, B., Klepetko, W., et al. (2014). Meprin beta, a novel mediator of vascular remodelling underlying pulmonary hypertension. J. Pathol. 233, 7–17. doi: 10.1002/path.4303
Bibl, M., Gallus, M., Welge, V., Esselmann, H., Wolf, S., Ruther, E., et al. (2012). Cerebrospinal fluid amyloid-β 2-42 is decreased in Alzheimer's, but not in frontotemporal dementia. J. Neural Transm. 119, 805–813. doi: 10.1007/s00702-012-0801-3
Bien, J., Jefferson, T., Causevic, M., Jumpertz, T., Muenter, L., Multhaup, G., et al. (2012). The metalloprotease meprin β generates amino terminal truncated Abeta-peptide species. J. Biol. Chem. 287, 33304–33313. doi: 10.1074/jbc.M112.395608
Broder, C., Arnold, P., Vadon-Le Goff, S., Konerding, M. A., Bahr, K., Müller, S., et al. (2013). Metalloproteases meprin alpha and meprin β are C- and N-procollagen proteinases important for collagen assembly and tensile strength. Proc. Natl. Acad. Sci. U.S.A. 110, 14219–14224. doi: 10.1073/pnas.1305464110
Broder, C., and Becker-Pauly, C. (2013). The metalloproteases meprin α and meprin β: unique enzymes in inflammation, neurodegeneration, cancer and fibrosis. Biochem. J. 450, 253–264. doi: 10.1042/BJ20121751
Brown, M. S., Ye, J., Rawson, R. B., and Goldstein, J. L. (2000). Regulated intramembrane proteolysis: a control mechanism conserved from bacteria to humans. Cell 100, 391–398. doi: 10.1016/S0092-8674(00)80675-3
Busciglio, J., Gabuzda, D. H., Matsudaira, P., and Yankner, B. A. (1993). Generation of beta-amyloid in the secretory pathway in neuronal and nonneuronal cells. Proc. Natl. Acad. Sci. U.S.A. 90, 2092–2096. doi: 10.1073/pnas.90.5.2092
Bush, A. I., Multhaup, G., Moir, R. D., Williamson, T. G., Small, D. H., Rumble, B., et al. (1993). A novel zinc(II) binding site modulates the function of the beta A4 amyloid protein precursor of Alzheimer's disease. J. Biol. Chem. 268, 16109–16112.
Bylander, J., Li, Q., Ramesh, G., Zhang, B., Reeves, W. B., and Bond, J. S. (2008). Targeted disruption of the meprin metalloproteinase beta gene protects against renal ischemia-reperfusion injury in mice. Am. J. Physiol. Renal Physiol. 294, F480–F490. doi: 10.1152/ajprenal.00214.2007
Cai, X. D., Golde, T. E., and Younkin, S. G. (1993). Release of excess amyloid beta protein from a mutant amyloid beta protein precursor. Science 259, 514–516. doi: 10.1126/science.8424174
Citron, M., Oltersdorf, T., Haass, C., McConlogue, L., Hung, A. Y., Seubert, P., et al. (1992). Mutation of the beta-amyloid precursor protein in familial Alzheimer's disease increases beta-protein production. Nature 360, 672–674. doi: 10.1038/360672a0
Citron, M., Teplow, D. B., and Selkoe, D. J. (1995). Generation of amyloid beta protein from its precursor is sequence specific. Neuron 14, 661–670. doi: 10.1016/0896-6273(95)90323-2
Dominguez, D., Tournoy, J., Hartmann, D., Huth, T., Cryns, K., Deforce, S., et al. (2005). Phenotypic and biochemical analyses of BACE1- and BACE2-deficient mice. J. Biol. Chem. 280, 30797–30806. doi: 10.1074/jbc.M505249200
Dulubova, I., Ho, A., Huryeva, I., Südhof, T. C., and Rizo, J. (2004). Three-dimensional structure of an independently folded extracellular domain of human amyloid-beta precursor protein. Biochemistry 43, 9583–9588. doi: 10.1021/bi049041o
Edbauer, D., Winkler, E., Regula, J. T., Pesold, B., Steiner, H., and Haass, C. (2003). Reconstitution of gamma-secretase activity. Nat. Cell Biol. 5, 486–488. doi: 10.1038/ncb960
Fukumoto, H., Cheung, B. S., Hyman, B. T., and Irizarry, M. C. (2002). Beta-secretase protein and activity are increased in the neocortex in Alzheimer disease. Arch. Neurol. 59, 1381–1389. doi: 10.1001/archneur.59.9.1381
Glenner, G. G., and Wong, C. W. (1984). Alzheimer's disease and Down's syndrome: sharing of a unique cerebrovascular amyloid fibril protein. Biochem. Biophys. Res. Commun. 122, 1131–1135. doi: 10.1016/0006-291X(84)91209-9
Goedert, M., Spillantini, M. G., Jakes, R., Rutherford, D., and Crowther, R. A. (1989). Multiple isoforms of human microtubule-associated protein tau: sequences and localization in neurofibrillary tangles of Alzheimer's disease. Neuron 3, 519–526. doi: 10.1016/0896-6273(89)90210-9
Goedert, M., Wischik, C. M., Crowther, R. A., Walker, J. E., and Klug, A. (1988). Cloning and sequencing of the cDNA encoding a core protein of the paired helical filament of Alzheimer disease: identification as the microtubule-associated protein tau. Proc. Natl. Acad. Sci. U.S.A. 85, 4051–4055. doi: 10.1073/pnas.85.11.4051
Golde, T. E., Estus, S., Younkin, L. H., Selkoe, D. J., and Younkin, S. G. (1992). Processing of the amyloid protein precursor to potentially amyloidogenic derivatives. Science 255, 728–730. doi: 10.1126/science.1738847
Gorman, P. M., Kim, S., Guo, M., Melnyk, R. A., McLaurin, J., Fraser, P. E., et al. (2008). Dimerization of the transmembrane domain of amyloid precursor proteins and familial Alzheimer's disease mutants. BMC Neurosci. 9:17. doi: 10.1186/1471-2202-9-17
Gralle, M., Oliveira, C. L., Guerreiro, L. H., McKinstry, W. J., Galatis, D., Masters, C. L., et al. (2006). Solution conformation and heparin-induced dimerization of the full-length extracellular domain of the human amyloid precursor protein. J. Mol. Biol. 357, 493–508. doi: 10.1016/j.jmb.2005.12.053
Gu, Y., Misonou, H., Sato, T., Dohmae, N., Takio, K., and Ihara, Y. (2001). Distinct intramembrane cleavage of the beta-amyloid precursor protein family resembling gamma-secretase-like cleavage of Notch. J. Biol. Chem. 276, 35235–35238. doi: 10.1074/jbc.C100357200
Güntert, A., Döbeli, H., and Bohrmann, B. (2006). High sensitivity analysis of amyloid-beta peptide composition in amyloid deposits from human and PS2APP mouse brain. Neuroscience 143, 461–475. doi: 10.1016/j.neuroscience.2006.08.027
Haass, C., Capell, A., Citron, M., Teplow, D. B., and Selkoe, D. J. (1995). The vacuolar H(+)-ATPase inhibitor bafilomycin A1 differentially affects proteolytic processing of mutant and wild-type beta-amyloid precursor protein. J. Biol. Chem. 270, 6186–6192. doi: 10.1074/jbc.270.11.6186
Haass, C., Koo, E. H., Mellon, A., Hung, A. Y., and Selkoe, D. J. (1992a). Targeting of cell-surface beta-amyloid precursor protein to lysosomes: alternative processing into amyloid-bearing fragments. Nature 357, 500–503. doi: 10.1038/357500a0
Haass, C., Schlossmacher, M. G., Hung, A. Y., Vigo-Pelfrey, C., Mellon, A., Ostaszewski, B. L., et al. (1992b). Amyloid beta-peptide is produced by cultured cells during normal metabolism. Nature 359, 322–325. doi: 10.1038/359322a0
Halim, A., Brinkmalm, G., Rüetschi, U., Westman-Brinkmalm, A., Portelius, E., Zetterberg, H., et al. (2011). Site-specific characterization of threonine, serine, and tyrosine glycosylations of amyloid precursor protein/amyloid beta-peptides in human cerebrospinal fluid. Proc. Natl. Acad. Sci. U.S.A. 108, 11848–11853. doi: 10.1073/pnas.1102664108
Hardy, J., and Selkoe, D. J. (2002). The amyloid hypothesis of Alzheimer's disease: progress and problems on the road to therapeutics. Science 297, 353–356. doi: 10.1126/science.1072994
Hedrich, J., Lottaz, D., Meyer, K., Yiallouros, I., Jahnen-Dechent, W., Stocker, W., et al. (2010). Fetuin-A and cystatin C are endogenous inhibitors of human meprin metalloproteases. Biochemistry 49, 8599–8607. doi: 10.1021/bi1004238
Hook, G., Yu, J., Toneff, T., Kindy, M., and Hook, V. (2014). Brain pyroglutamate amyloid-β is produced by cathepsin B and is reduced by the cysteine protease inhibitor E64d, representing a potential Alzheimer's disease therapeutic. J. Alzheimers Dis. 41, 129–149. doi: 10.3233/JAD-131370.
Hook, V., Toneff, T., Bogyo, M., Greenbaum, D., Medzihradszky, K. F., Neveu, J., et al. (2005). Inhibition of cathepsin B reduces β-amyloid production in regulated secretory vesicles of neuronal chromaffin cells: evidence for cathepsin B as a candidate beta-secretase of Alzheimer's disease. Biol. Chem. 386, 931–940. doi: 10.1515/BC.2005.108
Hosoda, R., Saido, T. C., Otvos, L. Jr., Arai, T., Mann, D. M., Lee, V. M., et al. (1998). Quantification of modified amyloid beta peptides in Alzheimer disease and Down syndrome brains. J. Neuropathol. Exp. Neurol. 57, 1089–1095. doi: 10.1097/00005072-199811000-00012
Hou, L., Kang, I., Marchant, R. E., and Zagorski, M. G. (2002). Methionine 35 oxidation reduces fibril assembly of the amyloid abeta-(1-42) peptide of Alzheimer's disease. J. Biol. Chem. 277, 40173–40176. doi: 10.1074/jbc.C200338200
Hussain, I., Hawkins, J., Harrison, D., Hille, C., Wayne, G., Cutler, L., et al. (2007). Oral administration of a potent and selective non-peptidic BACE-1 inhibitor decreases beta-cleavage of amyloid precursor protein and amyloid-beta production in vivo. J. Neurochem. 100, 802–809. doi: 10.1111/j.1471-4159.2006.04260.x
Hussain, I., Powell, D., Howlett, D. R., Tew, D. G., Meek, T. D., Chapman, C., et al. (1999). Identification of a novel aspartic protease (Asp 2) as beta-secretase. Mol. Cell. Neurosci. 14, 419–427. doi: 10.1006/mcne.1999.0811
Isbert, S., Wagner, K., Eggert, S., Schweitzer, A., Multhaup, G., Weggen, S., et al. (2012). APP dimer formation is initiated in the endoplasmic reticulum and differs between APP isoforms. Cell. Mol. Life Sci. 69, 1353–1375. doi: 10.1007/s00018-011-0882-4
Jäckle, F., Schmidt, F., Wichert, R., Arnold, P., Prox, J., Mangold, M., et al. (2015). Metalloprotease meprin beta is activated by transmembrane serine protease matriptase-2 at the cell surface thereby enhancing APP shedding. Biochem. J. 470, 91–103. doi: 10.1042/BJ20141417
Jefferson, T., Auf dem Keller, U., Bellac, C., Metz, V. V., Broder, C., Hedrich, J., et al. (2013). The substrate degradome of meprin metalloproteases reveals an unexpected proteolytic link between meprin β and ADAM10. Cell. Mol. Life Sci. 70, 309–333. doi: 10.1007/s00018-012-1106-2
Jefferson, T., Cauševic, M., auf dem Keller, U., Schilling, O., Isbert, S., Geyer, R., et al. (2011). Metalloprotease meprin beta generates nontoxic N-terminal amyloid precursor protein fragments in vivo. J. Biol. Chem. 286, 27741–27750. doi: 10.1074/jbc.M111.252718
Jonsson, T., Atwal, J. K., Steinberg, S., Snaedal, J., Jonsson, P. V., Bjornsson, S., et al. (2012). A mutation in APP protects against Alzheimer's disease and age-related cognitive decline. Nature 488, 96–99. doi: 10.1038/nature11283
Kaden, D., Voigt, P., Munter, L. M., Bobowski, K. D., Schaefer, M., and Multhaup, G. (2009). Subcellular localization and dimerization of APLP1 are strikingly different from APP and APLP2. J. Cell Sci. 122, 368–377. doi: 10.1242/jcs.034058
Kang, J., Lemaire, H. G., Unterbeck, A., Salbaum, J. M., Masters, C. L., Grzeschik, K. H., et al. (1987). The precursor of Alzheimer's disease amyloid A4 protein resembles a cell-surface receptor. Nature 325, 733–736. doi: 10.1038/325733a0
Kienlen-Campard, P., Tasiaux, B., Van Hees, J., Li, M., Huysseune, S., Sato, T., et al. (2008). Amyloidogenic processing but not amyloid precursor protein (APP) intracellular C-terminal domain production requires a precisely oriented APP dimer assembled by transmembrane GXXXG motifs. J. Biol. Chem. 283, 7733–7744. doi: 10.1074/jbc.M707142200
Kindy, M. S., Yu, J., Zhu, H., El-Amouri, S. S., Hook, V., and Hook, G. R. (2012). Deletion of the cathepsin B gene improves memory deficits in a transgenic ALZHeimer's disease mouse model expressing AβPP containing the wild-type beta-secretase site sequence. J. Alzheimers Dis. 29, 827–840. doi: 10.3233/JAD-2012-111604.
Kronenberg, D., Bruns, B. C., Moali, C., Vadon-Le Goff, S., Sterchi, E. E., Traupe, H., et al. (2010). Processing of procollagen III by meprins: new players in extracellular matrix assembly? J. Invest. Dermatol. 130, 2727–2735. doi: 10.1038/jid.2010.202
Kruse, M. N., Becker, C., Lottaz, D., Köhler, D., Yiallouros, I., Krell, H. W., et al. (2004). Human meprin α and β homo-oligomers: cleavage of basement membrane proteins and sensitivity to metalloprotease inhibitors. Biochem. J. 378, 383–389. doi: 10.1042/bj20031163
Kuda, T., Shoji, M., Arai, H., Kawashima, S., and Saido, T. C. (1997). Reduction of plasma glutamyl aminopeptidase activity in sporadic Alzheimer's disease. Biochem. Biophys. Res. Commun. 231, 526–530. doi: 10.1006/bbrc.1996.5920
Kumar, S., Rezaei-Ghaleh, N., Terwel, D., Thal, D. R., Richard, M., Hoch, M., et al. (2011). Extracellular phosphorylation of the amyloid β-peptide promotes formation of toxic aggregates during the pathogenesis of Alzheimer's disease. EMBO J. 30, 2255–2265. doi: 10.1038/emboj.2011.138
Kumar, S., Singh, S., Hinze, D., Josten, M., Sahl, H. G., Siepmann, M., et al. (2012). Phosphorylation of amyloid-β peptide at serine 8 attenuates its clearance via insulin-degrading and angiotensin-converting enzymes. J. Biol. Chem. 287, 8641–8651. doi: 10.1074/jbc.M111.279133
Kummer, M. P., Hermes, M., Delekarte, A., Hammerschmidt, T., Kumar, S., Terwel, D., et al. (2011). Nitration of tyrosine 10 critically enhances amyloid β aggregation and plaque formation. Neuron 71, 833–844. doi: 10.1016/j.neuron.2011.07.001
Letronne, F., Laumet, G., Ayral, A. M., Chapuis, J., Demiautte, F., Laga, M., et al. (2016). ADAM30 downregulates APP-linked defects through cathepsin D activation in Alzheimer's Disease. EBioMedicine 9, 278–292. doi: 10.1016/j.ebiom.2016.06.002
Lewczuk, P., Esselmann, H., Groemer, T. W., Bibl, M., Maler, J. M., Steinacker, P., et al. (2004). Amyloid β peptides in cerebrospinal fluid as profiled with surface enhanced laser desorption/ionization time-of-flight mass spectrometry: evidence of novel biomarkers in Alzheimer's disease. Biol. Psychiatry 55, 524–530. doi: 10.1016/j.biopsych.2003.10.014
Lewis, H., Beher, D., Cookson, N., Oakley, A., Piggott, M., Morris, C. M., et al. (2006). Quantification of Alzheimer pathology in ageing and dementia: age-related accumulation of amyloid-β(42) peptide in vascular dementia. Neuropathol. Appl. Neurobiol. 32, 103–118. doi: 10.1111/j.1365-2990.2006.00696.x
Lin, X., Koelsch, G., Wu, S., Downs, D., Dashti, A., and Tang, J. (2000). Human aspartic protease memapsin 2 cleaves the beta-secretase site of beta-amyloid precursor protein. Proc. Natl. Acad. Sci. U.S.A. 97, 1456–1460. doi: 10.1073/pnas.97.4.1456
Luo, Y., Bolon, B., Kahn, S., Bennett, B. D., Babu-Khan, S., Denis, P., et al. (2001). Mice deficient in BACE1, the Alzheimer's beta-secretase, have normal phenotype and abolished beta-amyloid generation. Nat. Neurosci. 4, 231–232. doi: 10.1038/85059
Maler, J. M., Klafki, H. W., Paul, S., Spitzer, P., Groemer, T. W., Henkel, A. W., et al. (2007). Urea-based two-dimensional electrophoresis of beta-amyloid peptides in human plasma: evidence for novel Abeta species. Proteomics 7, 3815–3820. doi: 10.1002/pmic.200700311
Maloney, J. A., Bainbridge, T., Gustafson, A., Zhang, S., Kyauk, R., Steiner, P., et al. (2014). Molecular mechanisms of Alzheimer disease protection by the A673T allele of amyloid precursor protein. J. Biol. Chem. 289, 30990–31000. doi: 10.1074/jbc.M114.589069
Masters, C. L., Multhaup, G., Simms, G., Pottgiesser, J., Martins, R. N., and Beyreuther, K. (1985). Neuronal origin of a cerebral amyloid: neurofibrillary tangles of Alzheimer's disease contain the same protein as the amyloid of plaque cores and blood vessels. EMBO J. 4, 2757–2763.
Mattsson, N., Rajendran, L., Zetterberg, H., Gustavsson, M., Andreasson, U., Olsson, M., et al. (2012). BACE1 inhibition induces a specific cerebrospinal fluid beta-amyloid pattern that identifies drug effects in the central nervous system. PLoS ONE 7:e31084. doi: 10.1371/journal.pone.0031084
Mucke, L., Masliah, E., Yu, G. Q., Mallory, M., Rockenstein, E. M., Tatsuno, G., et al. (2000). High-level neuronal expression of abeta 1-42 in wild-type human amyloid protein precursor transgenic mice: synaptotoxicity without plaque formation. J. Neurosci. 20, 4050–4058.
Mueller-Steiner, S., Zhou, Y., Arai, H., Roberson, E. D., Sun, B., Chen, J., et al. (2006). Antiamyloidogenic and neuroprotective functions of cathepsin B: implications for Alzheimer's disease. Neuron 51, 703–714. doi: 10.1016/j.neuron.2006.07.027
Multhaup, G., Schlicksupp, A., Hesse, L., Beher, D., Ruppert, T., Masters, C. L., et al. (1996). The amyloid precursor protein of Alzheimer's disease in the reduction of copper(II) to copper(I). Science 271, 1406–1409. doi: 10.1126/science.271.5254.1406
Munter, L. M., Voigt, P., Harmeier, A., Kaden, D., Gottschalk, K. E., Weise, C., et al. (2007). GxxxG motifs within the amyloid precursor protein transmembrane sequence are critical for the etiology of Abeta42. EMBO J. 26, 1702–1712. doi: 10.1038/sj.emboj.7601616
Murayama, K. S., Kametani, F., Tabira, T., and Araki, W. (2007). A novel monoclonal antibody specific for the amino-truncated beta-amyloid Abeta5-40/42 produced from caspase-cleaved amyloid precursor protein. J. Neurosci. Methods 161, 244–249. doi: 10.1016/j.jneumeth.2006.11.010
Nikolaev, A., McLaughlin, T., O'Leary, D. D., and Tessier-Lavigne, M. (2009). APP binds DR6 to trigger axon pruning and neuron death via distinct caspases. Nature 457, 981–989. doi: 10.1038/nature07767
Nishitomi, K., Sakaguchi, G., Horikoshi, Y., Gray, A. J., Maeda, M., Hirata-Fukae, C., et al. (2006). BACE1 inhibition reduces endogenous Abeta and alters APP processing in wild-type mice. J. Neurochem. 99, 1555–1563. doi: 10.1111/j.1471-4159.2006.04178.x
Oakley, H., Cole, S. L., Logan, S., Maus, E., Shao, P., Craft, J., et al. (2006). Intraneuronal beta-amyloid aggregates, neurodegeneration, and neuron loss in transgenic mice with five familial Alzheimer's disease mutations: potential factors in amyloid plaque formation. J. Neurosci. 26, 10129–10140. doi: 10.1523/JNEUROSCI.1202-06.2006
Oddo, S., Caccamo, A., Shepherd, J. D., Murphy, M. P., Golde, T. E., Kayed, R., et al. (2003). Triple-transgenic model of Alzheimer's disease with plaques and tangles: intracellular Abeta and synaptic dysfunction. Neuron 39, 409–421. doi: 10.1016/S0896-6273(03)00434-3
Ohler, A., Debela, M., Wagner, S., Magdolen, V., and Becker-Pauly, C. (2010). Analyzing the protease web in skin: meprin metalloproteases are activated specifically by KLK4, 5 and 8 vice versa leading to processing of proKLK7 thereby triggering its activation. Biol. Chem. 391, 455–460. doi: 10.1515/bc.2010.023
Palmblad, M., Westlind-Danielsson, A., and Bergquist, J. (2002). Oxidation of methionine 35 attenuates formation of amyloid beta -peptide 1-40 oligomers. J. Biol. Chem. 277, 19506–19510. doi: 10.1074/jbc.M112218200
Price, J. M., Sutton, E. T., Hellermann, A., and Thomas, T. (1997). beta-Amyloid induces cerebrovascular endothelial dysfunction in the rat brain. Neurol. Res. 19, 534–538. doi: 10.1080/01616412.1997.11740853
Prox, J., Arnold, P., and Becker-Pauly, C. (2015). Meprin α and meprin β: procollagen proteinases in health and disease. Matrix Biol. 44–46, 7–13. doi: 10.1016/j.matbio.2015.01.010
Prox, J., Willenbrock, M., Weber, S., Lehmann, T., Schmidt-Arras, D., Schwanbeck, R., et al. (2012). Tetraspanin15 regulates cellular trafficking and activity of the ectodomain sheddase ADAM10. Cell. Mol. Life Sci. 69, 2919–2932. doi: 10.1007/s00018-012-0960-2
Roberds, S. L., Anderson, J., Basi, G., Bienkowski, M. J., Branstetter, D. G., Chen, K. S., et al. (2001). BACE knockout mice are healthy despite lacking the primary beta-secretase activity in brain: implications for Alzheimer's disease therapeutics. Hum. Mol. Genet. 10, 1317–1324. doi: 10.1093/hmg/10.12.1317
Russo, C., Violani, E., Salis, S., Venezia, V., Dolcini, V., Damonte, G., et al. (2002). Pyroglutamate-modified amyloid beta-peptides–AbetaN3(pE)–strongly affect cultured neuron and astrocyte survival. J. Neurochem. 82, 1480–1489. doi: 10.1046/j.1471-4159.2002.01107.x
Saido, T., and Leissring, M. A. (2012). Proteolytic degradation of amyloid beta-protein. Cold Spring Harb. Perspect. Med. 2:a006379. doi: 10.1101/cshperspect.a006379
Sankaranarayanan, S., Price, E. A., Wu, G., Crouthamel, M. C., Shi, X. P., Tugusheva, K., et al. (2008). In vivo beta-secretase 1 inhibition leads to brain Abeta lowering and increased alpha-secretase processing of amyloid precursor protein without effect on neuregulin-1. J. Pharmacol. Exp. Ther. 324, 957–969. doi: 10.1124/jpet.107.130039
Schechter, I., and Ziv, E. (2011). Cathepsins, S., B and L with aminopeptidases display beta-secretase activity associated with the pathogenesis of Alzheimer's disease. Biol. Chem. 392, 555–569. doi: 10.1515/bc.2011.054
Scheuermann, S., Hambsch, B., Hesse, L., Stumm, J., Schmidt, C., Beher, D., et al. (2001). Homodimerization of amyloid precursor protein and its implication in the amyloidogenic pathway of Alzheimer's disease. J. Biol. Chem. 276, 33923–33929. doi: 10.1074/jbc.M105410200
Schieb, H., Kratzin, H., Jahn, O., Möbius, W., Rabe, S., Staufenbiel, M., et al. (2011). Beta-amyloid peptide variants in brains and cerebrospinal fluid from amyloid precursor protein (APP) transgenic mice: comparison with human Alzheimer amyloid. J. Biol. Chem. 286, 33747–33758. doi: 10.1074/jbc.M111.246561
Schieb, H., Weidlich, S., Schlechtingen, G., Linning, P., Jennings, G., Gruner, M., et al. (2010). Structural design, solid-phase synthesis and activity of membrane-anchored β-secretase inhibitors on Aβ generation from wild-type and Swedish-mutant APP. Chemistry 16, 14412–14423. doi: 10.1002/chem.201002878
Schilling, S., Hoffmann, T., Manhart, S., Hoffmann, M., and Demuth, H. U. (2004). Glutaminyl cyclases unfold glutamyl cyclase activity under mild acid conditions. FEBS Lett. 563, 191–196. doi: 10.1016/S0014-5793(04)00300-X
Schlessinger, J. (2002). Ligand-induced, receptor-mediated dimerization and activation of EGF receptor. Cell 110, 669–672. doi: 10.1016/S0092-8674(02)00966-2
Schmidt, F., Müller, M., Prox, J., Arnold, P., Schönherr, C., Tredup, C., et al. (2016). Tetraspanin 8 is an interactor of the metalloprotease meprin β within tetraspanin-enriched microdomains. Biol. Chem. 397, 857–869. doi: 10.1515/hsz-2016-0126
Schmidt, V., Baum, K., Lao, A., Rateitschak, K., Schmitz, Y., Teichmann, A., et al. (2012). Quantitative modelling of amyloidogenic processing and its influence by SORLA in Alzheimer's disease. EMBO J. 31, 187–200. doi: 10.1038/emboj.2011.352
Schönherr, C., Bien, J., Isbert, S., Wichert, R., Prox, J., Altmeppen, H., et al. (2016). Generation of aggregation prone N-terminally truncated amyloid β peptides by meprin β depends on the sequence specificity at the cleavage site. Mol. Neurodegener. 11, 19. doi: 10.1186/s13024-016-0084-5
Schrader-Fischer, G., and Paganetti, P. A. (1996). Effect of alkalizing agents on the processing of the beta-amyloid precursor protein. Brain Res. 716, 91–100. doi: 10.1016/0006-8993(96)00002-9
Schütte, A., Ermund, A., Becker-Pauly, C., Johansson, M. E., Rodriguez-Pineiro, A. M., Backhed, F., et al. (2014). Microbial-induced meprin β cleavage in MUC2 mucin and a functional CFTR channel are required to release anchored small intestinal mucus. Proc. Natl. Acad. Sci. U.S.A. 111, 12396–12401. doi: 10.1073/pnas.1407597111
Seubert, P., Vigo-Pelfrey, C., Esch, F., Lee, M., Dovey, H., Davis, D. S., et al. (1992). Isolation and quantification of soluble Alzheimer's beta-peptide from biological fluids. Nature 359, 325–327. doi: 10.1038/359325a0
Sevalle, J., Amoyel, A., Robert, P., Fournié-Zaluski, M. C., Roques, B., et al. (2009). Aminopeptidase A contributes to the N-terminal truncation of amyloid beta-peptide. J. Neurochem. 109, 248–256. doi: 10.1111/j.1471-4159.2009.05950.x
Simons, A., Ruppert, T., Schmidt, C., Schlicksupp, A., Pipkorn, R., Reed, J., et al. (2002). Evidence for a copper-binding superfamily of the amyloid precursor protein. Biochemistry 41, 9310–9320. doi: 10.1021/bi0258647
Sinha, S., Anderson, J. P., Barbour, R., Basi, G. S., Caccavello, R., Davis, D., et al. (1999). Purification and cloning of amyloid precursor protein beta-secretase from human brain. Nature 402, 537–540. doi: 10.1038/990114
Small, D. H., Nurcombe, V., Reed, G., Clarris, H., Moir, R., Beyreuther, K., et al. (1994). A heparin-binding domain in the amyloid protein precursor of Alzheimer's disease is involved in the regulation of neurite outgrowth. J. Neurosci. 14, 2117–2127.
Soba, P., Eggert, S., Wagner, K., Zentgraf, H., Siehl, K., Kreger, S., et al. (2005). Homo- and heterodimerization of APP family members promotes intercellular adhesion. EMBO J. 24, 3624–3634. doi: 10.1038/sj.emboj.7600824
Stanton, M. G., Stauffer, S. R., Gregro, A. R., Steinbeiser, M., Nantermet, P., Sankaranarayanan, S., et al. (2007). Discovery of isonicotinamide derived β-secretase inhibitors: in vivo reduction of β-amyloid. J. Med. Chem. 50, 3431–3433. doi: 10.1021/jm070272d
Takeda, K., Araki, W., Akiyama, H., and Tabira, T. (2004). Amino-truncated amyloid beta-peptide (Abeta5-40/42) produced from caspase-cleaved amyloid precursor protein is deposited in Alzheimer's disease brain. FASEB J. 18, 1755–1757. doi: 10.1096/fj.03-1070fje
Vassar, R. (2002). Beta-secretase (BACE) as a drug target for Alzheimer's disease. Adv. Drug Deliv. Rev. 54, 1589–1602. doi: 10.1016/S0169-409X(02)00157-6
Vassar, R., Bennett, B. D., Babu-Khan, S., Kahn, S., Mendiaz, E. A., Denis, P., et al. (1999). Beta-secretase cleavage of Alzheimer's amyloid precursor protein by the transmembrane aspartic protease BACE. Science 286, 735–741. doi: 10.1126/science.286.5440.735
Vazeille, E., Bringer, M. A., Gardarin, A., Chambon, C., Becker-Pauly, C., Pender, S. L., et al. (2011). Role of meprins to protect ileal mucosa of Crohn's disease patients from colonization by adherent-invasive E. coli. PLoS ONE 6:e21199. doi: 10.1371/journal.pone.0021199
Vigo-Pelfrey, C., Lee, D., Keim, P., Lieberburg, I., and Schenk, D. B. (1993). Characterization of beta-amyloid peptide from human cerebrospinal fluid. J. Neurochem. 61, 1965–1968. doi: 10.1111/j.1471-4159.1993.tb09841.x
Wang, D. S., Dickson, D. W., and Malter, J. S. (2006). beta-Amyloid degradation and Alzheimer's disease. J. Biomed. Biotechnol. 2006:58406. doi: 10.1155/JBB/2006/58406
Wang, R., Sweeney, D., Gandy, S. E., and Sisodia, S. S. (1996). The profile of soluble amyloid beta protein in cultured cell media. Detection and quantification of amyloid beta protein and variants by immunoprecipitation-mass spectrometry. J. Biol. Chem. 271, 31894–31902. doi: 10.1074/jbc.271.50.31894
Wang, Y., and Ha, Y. (2004). The X-ray structure of an antiparallel dimer of the human amyloid precursor protein E2 domain. Mol. Cell. 15, 343–353. doi: 10.1016/j.molcel.2004.06.037
Wiltfang, J., Esselmann, H., Cupers, P., Neumann, M., Kretzschmar, H., Beyermann, M., et al. (2001). Elevation of beta-amyloid peptide 2-42 in sporadic and familial Alzheimer's disease and its generation in PS1 knockout cells. J. Biol. Chem. 276, 42645–42657. doi: 10.1074/jbc.M102790200
Wittnam, J. L., Portelius, E., Zetterberg, H., Gustavsson, M. K., Schilling, S., Koch, B., et al. (2012). Pyroglutamate amyloid β (Aβ) aggravates behavioral deficits in transgenic amyloid mouse model for Alzheimer disease. J. Biol. Chem. 287, 8154–8162. doi: 10.1074/jbc.M111.308601
Wolfe, M. S., Xia, W., Moore, C. L., Leatherwood, D. D., Ostaszewski, B., Rahmati, T., et al. (1999). Peptidomimetic probes and molecular modeling suggest that Alzheimer's gamma-secretase is an intramembrane-cleaving aspartyl protease. Biochemistry 38, 4720–4727. doi: 10.1021/bi982562p
Yamamoto, K., Murphy, G., and Troeberg, L. (2015). Extracellular regulation of metalloproteinases. Matrix Biol. 44–46, 255–263. doi: 10.1016/j.matbio.2015.02.007
Yan, R., Bienkowski, M. J., Shuck, M. E., Miao, H., Tory, M. C., Pauley, A. M., et al. (1999). Membrane-anchored aspartyl protease with Alzheimer's disease beta-secretase activity. Nature 402, 533–537. doi: 10.1038/990107
Yura, R. E., Bradley, S. G., Ramesh, G., Reeves, W. B., and Bond, J. S. (2009). Meprin A metalloproteases enhance renal damage and bladder inflammation after LPS challenge. Am. J. Physiol. Renal Physiol. 296, F135–F144. doi: 10.1152/ajprenal.90524.2008
Zhang, A., Uaesoontrachoon, K., Shaughnessy, C., Das, J. R., Rayavarapu, S., Brown, K. J., et al. (2015). The use of urinary and kidney SILAM proteomics to monitor kidney response to high dose morpholino oligonucleotides in the mdx mouse. Toxicol. Rep. 2, 838–849. doi: 10.1016/j.toxrep.2015.05.008
Zhao, G., Mao, G., Tan, J., Dong, Y., Cui, M. Z., Kim, S. H., et al. (2004). Identification of a new presenilin-dependent zeta-cleavage site within the transmembrane domain of amyloid precursor protein. J. Biol. Chem. 279, 50647–50650. doi: 10.1074/jbc.C400473200
Keywords: meprin β, N-terminal truncated Aβ, APP, shedding, proteolysis
Citation: Becker-Pauly C and Pietrzik CU (2017) The Metalloprotease Meprin β Is an Alternative β-Secretase of APP. Front. Mol. Neurosci. 9:159. doi: 10.3389/fnmol.2016.00159
Received: 26 October 2016; Accepted: 09 December 2016;
Published: 05 January 2017.
Edited by:
Ulrike C. Müller, Heidelberg University, GermanyReviewed by:
Oliver Schilling, University of Freiburg, GermanyUlrich Auf Dem Keller, ETH Zurich, Switzerland
Copyright © 2017 Becker-Pauly and Pietrzik. This is an open-access article distributed under the terms of the Creative Commons Attribution License (CC BY). The use, distribution or reproduction in other forums is permitted, provided the original author(s) or licensor are credited and that the original publication in this journal is cited, in accordance with accepted academic practice. No use, distribution or reproduction is permitted which does not comply with these terms.
*Correspondence: Christoph Becker-Pauly, Y2JlY2tlcnBhdWx5QGJpb2NoZW0udW5pLWtpZWwuZGU=
Claus U. Pietrzik, cGlldHJ6aWtAdW5pLW1haW56LmRl