- Department of Pharmacology and Chemical Biology, University of Pittsburgh School of Medicine, Pittsburgh, PA, United States
α5 subunit containing GABA type A receptors (GABAARs) have long been an enigmatic receptor subtype of interest due to their specific brain distribution, unusual surface localization and key role in synaptic plasticity, cognition and memory. These receptors are uniquely positioned to sculpt both the developing and mature hippocampal circuitry due to high overall expression and a distinct peak within the critical synapse formation period during the second postnatal week. Unlike the majority of other GABAARs, they exhibit both receptor clustering at extrasynaptic sites via interactions with the radixin scaffold as well as synaptic sites via gephyrin, thus contributing respectively to tonic currents and synaptic GABAergic neurotransmission. α5 GABAAR signaling can be altered in neurodevelopmental disorders including autism and mental retardation and by inflammation in CNS injury and disease. Due to the unique physiology and pharmacology of α5 GABAARs, drugs targeting these receptors are being developed and tested as treatments for neurodevelopmental disorders, depression, schizophrenia, and mild cognitive impairment. This review article focuses on advances in understanding how the α5 subunit contributes to GABAAR neurobiology. In particular, I discuss both recent insights and remaining knowledge gaps for the functional role of these receptors, pathologies associated with α5 GABAAR dysfunction, and the effects and potential therapeutic uses of α5 receptor subtype targeted drugs.
Introduction
Structure, Distribution and Composition
GABA type A receptors (GABAARs) are heteropentameric ligand-gated chloride (Cl−) ion channels typically composed of two α (α1–6), two β (β1–3), and one γ (γ1–3) or δ subunit (Figure 1A). The common structure of individual subunits consists of a large extracellular N-terminus (NT), four transmembrane α-helices (M1–4) and a barely extruding extracellular C-terminus (CT). The conserved hydrophobic M domains are connected by small regions with a larger cytoplasmic domain between M3 and M4 (CD) that mediates interactions with intracellular proteins critical for receptor trafficking and surface localization (Figure 1B). Receptors can contain two different α or β subunits that are arranged in a counterclockwise configuration of γ-β-α-β-α (Figure 1C). The two αβ NT interfaces form GABA binding sites composed of the principal (+) side of the β subunit and the complementary α subunit (−) side, while a single α+(1, 2, 3 or 5)/γ2- interface generates the primary binding site for benzodiazepines, which are allosteric positive modulators of the GABAAR and an important clinical sedative-hypnotic-anxiolytic drug class. Several recent high resolution cryo-electron microscopy studies have provided unprecedented structural information for GABAAR (Phulera et al., 2018; Zhu et al., 2018; Laverty et al., 2019; Masiulis et al., 2019), advancing understanding of receptor architecture, principles of assembly, and binding of various ligands: GABA, bicuculline (antagonist), picrotoxin (channel blocker), and benzodiazepines. The channel properties, subcellular localization and pharmacological sensitivity of a GABAAR are defined by the subunit composition. While α5 containing GABAARs makeup only approximately 5% of the total receptor population in the brain, they are highly expressed in both the hippocampus and olfactory bulb. They represent close to 25% of all hippocampal GABAAR (Olsen and Sieghart, 2009) and are particularly abundant in CA1 and CA3. In the olfactory bulb, over a third of the neurons in the internal granule cell layer have α5 GABAARs (Sur et al., 1999), although the function here is unknown. α5 GABAARs are also expressed in the spinal cord, where they contribute to presynaptic inhibitory control over sensory-motor transmission (Lucas-Osma et al., 2018) and are also implicated in resolution of hyperalgesia (Perez-Sanchez et al., 2017). Other brain regions where these receptors are found at lower levels include the cortex, subiculum, hypothalamus, sympathetic preganglionic neurons, and amygdala (Martin et al., 2009a).
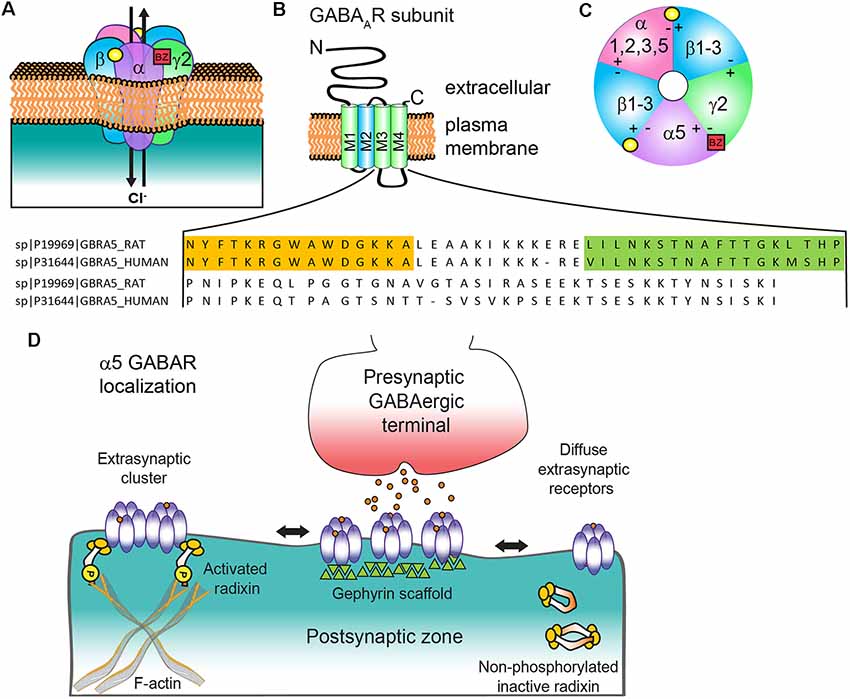
Figure 1. α5 subunit containing GABA type A receptor (α5 GABAAR) structure and subunit topology. (A) Generic synaptic GABAAR heteropentamer. Binding of the neurotransmitter GABA (yellow circle) at the αβ interface triggers ion channel opening and allows the rapid Cl− influx and membrane hyperpolarization. Benzodiazepines (BZ, red box) bind at the interface of an α1/2/3/5 and γ2 subunit. (B) All subunits have a common topology including an extracellular N-terminal domain (NT), short C-terminal tail (CT), and four transmembrane regions (M1–4) which compose the transmembrane domain. M2 (blue) contributes to formation of the receptor ion channel pore, while the large cytoplasmic domain between M3 and M4 (CD) contains sites for protein interactions and post translational modifications that modulate channel function and/or trafficking: amino acid residue alignment of rat and human α5 CD with radixin binding domain (orange highlighted residues, from Loebrich et al., 2006) and gephyrin interacting region (green highlighted residues, from Brady and Jacob, 2015). (C) α5 GABAAR extracellular representation with potential subunit combinations. (D) Schematic of α5 GABAAR clustering mechanisms at extrasynaptic and synaptic locations with radixin and gephyrin. Phosphorylated radixin interacts with receptors and actin, while with dephosphorylation radixin N-terminal FERM and C-terminal F-actin binding domains interact and form inactive monomers or dimers.
Early pharmacological analysis indicated rat and human hippocampal α5 GABAARs have α5β3γ2 characteristics (Sur et al., 1998). However, sequential immunoprecipitation from hippocampal tissue identified that α1/α5 heteromers constitute approximately 9% of the α1 GABAARs and α2/α5 heteromers constitute about 20% of the α2 population in the hippocampus (Araujo et al., 1999; del Río et al., 2001). More recent mass spectrometry analysis of affinity purified α5 GABAARs from mouse hippocampus supported association of α5 with α1–3, β1–3 and both γ2S and γ2L isoforms (Ju et al., 2009). A recent comparison of α5β1–3γ2L GABAARs in HEK cells co-cultured with neurons revealed robust inhibitory postsynaptic currents (IPSCs) with slow decay rates and isoform-specific effects of pharmacological inhibitors (Chen et al., 2017). Importantly, in mixed alpha subunit GABAARs there appears to be preferential assembly of α5 and γ2 together, generating a benzodiazepine binding site with α5 subunit pharmacology (Araujo et al., 1999; del Río et al., 2001). Thus for a mixed α5 GABAAR, the other alpha subunit is essentially pharmacologically inactive for benzodiazepines and other alpha/gamma subunit interface binding drugs (i.e., the “Z-drugs” for insomnia treatment zolpidem, zopiclone, zaleplon). Mutation of the α5 subunit H105 residue, a key alpha subunit residue required for forming the benzodiazepine binding site with the γ2 subunit, led to repositioning of α5 H105R subunits into the pharmacologically inactive alpha subunit location (Balic et al., 2009). Interestingly, our recent mass spectrometry analysis identified a specific increase in α5βγ2 containing receptors in the cortex following diazepam injection, consistent with benzodiazepine exposure leading to modification of GABAAR composition and potentially drug effects through α5 plasticity (Lorenz-Guertin et al., 2019).
Cellular and Circuit Localization
Subcellular Localization
Controversies regarding α5 GABAAR subcellular localization in the literature have mirrored debates about its functional impact on GABAergic neurotransmission. Due to their initial identification as a key generator of hippocampal tonic current (Caraiscos et al., 2004; Glykys and Mody, 2006; Bonin et al., 2007), α5 GABAARs were generally considered extrasynaptic receptors, despite earlier evidence for synaptic clustering on dendrites and the axon initial segment (Brünig et al., 2002; Christie and de Blas, 2002; Serwanski et al., 2006). α5 GABAARs predominantly mediate tonic inhibition in hippocampal CA3 and CA1 pyramidal neurons, cortical neurons (layer 5) and are contributors to tonic inhibition in dentate gyrus granule cells (Glykys et al., 2008; Herd et al., 2008). Immunocytochemistry indicates an extensive extrasynaptic presence of α5 GABAARs (Brünig et al., 2002; Crestani et al., 2002). However, this receptor subtype is unique in displaying surface clustering at extrasynaptic locations rather than a uniformly diffuse extrasynaptic distribution. Regions within the large cytoplasmic domain between M3 and M4 regulate subcellular clustering of α5 GABAARs via interactions with radixin and gephyrin scaffolds (Figure 1D). Extrasynaptic clustering is mediated by radixin, an ezrin/radixin/moesin (ERM) family member that links actin to the plasma membrane (Loebrich et al., 2006). Phosphorylated radixin scaffolds α5βγ2 receptors to the actin cytoskeleton, ultimately reducing diffusion rates and concentrating channel activity away from axon terminals (Hausrat et al., 2015). Treatment with GABA promotes radixin phosphorylation and retention of α5 GABAARs extrasynaptically, while AMPA, a ligand for ionotropic glutamatergic GluA type receptors, leads to dephosphorylation, an increase in synaptic α5-subunit receptors and an increase in slowly decaying miniature IPSCs (mIPSCs). Further support for the specific contribution of α5 GABAARs to slowly decaying IPSCs is seen in early neurodevelopment during the switch from α5 to α1 and α3 subunit expression (Pangratz-Fuehrer et al., 2016). Important areas of further investigation include assessment of the level and role of α5 GABAARs associated with radixin or gephyrin in the developing and adult brain and plasticity mechanisms regulating these interactions.
Functional studies indicate the α5 subunit is also important for phasic events including: spontaneous inhibitory postsynaptic currents (sIPSCs), evoked IPSCs (eIPSCs) and GABAslow IPSCs (Collinson et al., 2002; Prenosil et al., 2006; Zarnowska et al., 2009; Vargas-Caballero et al., 2010). Consistent with a synaptic role for α5 GABAARs, we demonstrated that the α5 subunit directly interacts with the gephyrin synaptic scaffold, with approximately half of surface α5 GABAARs being synaptically localized throughout the first 3 weeks of circuit development (Brady and Jacob, 2015). Single particle tracking studies measured reduced diffusion of surface α5 GABAARs at synapses (Renner et al., 2012) and similar to other synaptic receptors, α5 GABAARs showed an increase in diffusion with negative modulator DMCM treatment (Lévi et al., 2015). Further studies are needed to determine both acute and prolonged effects of α5 preferring GABAAR drugs on receptor diffusive properties and surface stability.
Cell Type and Input-Specific Expression
α5 GABAARs show input-specific synaptic localization and function in different brain regions both for pyramidal cells and interneurons. Recent work demonstrates preferential localization of α5 GABAARs to inhibitory synapses on dendrites of somatostatin-expressing interneurons in CA1 that are targeted by vasoactive intestinal peptide and calretinin-positive interneurons (Magnin et al., 2019). Somatostatin interneurons and NO-synthase-positive neurogliaform cells target α5 GABAARs on dendrites of hippocampal CA1 pyramidal neurons to generate slow IPSCs (Schulz et al., 2018). Importantly, these outward-rectifying α5-GABAARs generate a greater hyperpolarizing current at slightly depolarized membrane potentials, thereby having a large impact on NMDA-receptor-activation and action potential firing in pyramidal neurons. In the cortex, pyramidal cells exhibit dendritically localized α5 GABAARs at sites innervated by bitufted interneurons (an SST positive neuron class; Ali and Thomson, 2008). A recent human and mouse prefrontal cortex gene expression study determined that the majority of α5 GABAARs are in pyramidal cells, followed by parvalbumin interneurons (Hu et al., 2018). Interestingly, α5 GABAAR mRNA was uniquely expressed in human SST interneurons, albeit at a low level. As deficits in both GABAergic signaling and SST signaling (Fuchs et al., 2017) have been identified as contributors to major depressive disorder, this data suggests positive modulation of α5 GABAAR could be therapeutic by multiple mechanisms. It is clear that improving understanding of GABAAR subtype subcellular (extrasynaptic vs. synaptic) and circuit-specific localization and function are critical areas of current research and future pharmacological development (reviewed in Engin et al., 2018).
Functional Role of α5 GABAARs
Neuronal Excitability, Learning and Memory
Genetic and pharmacological studies in rodents demonstrate that α5 GABAARs are key in learning and memory processes (reviewed in Martin et al., 2009a). The two primary mouse models used in studying the α5 GABAAR contribution to cognitive processes are the α5 subunit knockout mice (Gabra5−/−) and the α5H105R point mutation mice. Although originally generated to render α5 receptors insensitive to benzodiazepines, α5H105R mice also have a 25% decrease in hippocampal α5 protein level (Crestani et al., 2002). As described earlier, Gabra5−/− mice showed a reduction in diverse types of phasic GABAAR currents and the tonic current. Behaviorally, the increased excitability of Gabra5−/− hippocampal pyramidal neurons was correlated with improved performance in a spatial learning behavior (Collinson et al., 2002), though later studies were not able to replicate this result (Cheng et al., 2006; Martin et al., 2009b). However, both Gabra5−/− and α5H105R mice show enhanced trace fear conditioning, a hippocampal learning task, while performing similarly to wild-type mice in a cued fear conditioning assay, which relies on the amygdala, hippocampus, and cortex (Crestani et al., 2002; Martin et al., 2009b). Long-term potentiation (LTP), the cellular correlate of learning and memory, is constrained by GABAAR-mediated inhibition. Gabra5−/− mice showed a reduced threshold for LTP induction with 10–20 Hz stimulation (Martin et al., 2010). In addition, Gabra5−/− mice showed greater power of kainate-induced gamma frequency oscillations (Towers et al., 2004), and knockout of delta and α5 subunits led to spontaneous gamma oscillations in CA3 (Glykys et al., 2008). Gamma oscillations occur in a range of cognitive states including memory processing, are thought to support neural coding of environmental information and are disturbed in some psychiatric disorders (reviewed in Lisman and Buzsáki, 2008). In summary, a reduction in α5 inhibition may improve learning and memory through enhanced neuronal firing and network oscillatory activity.
Development
In contrast to their inhibitory role in the mature nervous system, GABAARs can promote excitation in newly forming circuits, allowing chloride efflux to produce membrane depolarization which promotes calcium entry, dendritic outgrowth, synaptogenesis and unsilencing of glutamatergic synapses (reviewed in Ben-Ari et al., 2007). α5 GABAARs are particularly well positioned to sculpt early hippocampal circuit development due to exceptionally high expression that peaks in the first two postnatal weeks (Liu et al., 1998; Ramos et al., 2004; Yu et al., 2014; Bader et al., 2017), and receptor localization at both extrasynaptic and synaptic sites. During the first postnatal week, tonic α5 currents enhance cell excitability and synaptic activity, facilitating the induction of giant depolarizing potentials, which are important for early network maturation (Ben-Ari, 2002; Marchionni et al., 2007). Importantly, GABAergic activation of circuit formation also occurs with newborn neurons integrating into networks in the adult mammalian brain in vivo (Ge et al., 2006). A few in vitro pharmacological and genetic studies have supported the role of α5 GABAARs in dendritic development. Cultured hippocampal neurons treated with an α5-specific negative allosteric modulator (NAM; RY-80) exhibited decreased dendritic arborization and reduced expression of the AMPA type glutamate receptor GluA2 subunit (Giusi et al., 2009). To investigate the role of α5 GABAARs in emerging circuits, we genetically manipulated α5 binding to gephyrin, increasing or decreasing the ratio of extrasynaptic/synaptic α5 GABAARs (Brady and Jacob, 2015). Interestingly, reducing synaptic α5 GABAARs promoted dendritic outgrowth at the expense of dendritic spine maturation in hippocampal neurons. Consistent with these findings, recent work showed that single-cell deletion of Gabra5 in adult-born dentate gyrus granule cells caused severe alterations of migration and dendrite development (Deprez et al., 2016). Further research is needed to elucidate the specific role of the α5 subunit in dendritic architecture, both during development and in adult neurogenesis.
Genetic Disorders with Altered α5 GABAAR Neurotransmission
While acute reduction in α5 GABAARs has shown potential for improving cognition and memory, further studies both in mouse models and human patients link long term reduction with significant pathologies. Reduced α5 GABAAR levels, function or protein interactions have been observed in patients with neurodevelopmental disorders including intellectual disability, epilepsy and autism. Common conditions among these disorders include cognitive impairments, increased anxiety, autism-related behaviors, sleep disorders and epilepsy susceptibility. Analogous behavioral changes and pathologies are observed in mouse models including Gabra5−/−mice (Zurek et al., 2016; Mesbah-Oskui et al., 2017), Fragile X syndrome model mice (Fmr1−/− mice, Bakker and Oostra, 2003), and other mouse models of ASD (reviewed in Kazdoba et al., 2016). Fmr1−/− mice show downregulation of α5 GABAAR and a deficit in tonic inhibition (Curia et al., 2009). Subsequent studies of α5H105R mice identified behavioral changes including hyperactivity and impaired encoding of object location memories (Hauser et al., 2005; Prut et al., 2010), although some behavioral changes may be attributed to subunit ordering rearrangements in a mixed alpha subunit GABAAR (see earlier, Composition).
The most commonly reported loci of chromosomal abnormalities in ASD patients are found in the q11.2–13 region on chromosome 15 (Hogart et al., 2010). Among the genes in this region are the α5, β3, and γ3 subunits. An autism patient exome study identified mutations including α5G113A (NT), α5V204I (NT) and mutations in the extrasynaptic anchor radixin: T516I, P471T, D197H, A496V (Zurek et al., 2016). Exome sequencing of sporadic genetic epilepsy patients identified α5V204I (NT), α5W280R (M1), α5S402A (CD) and α5P453L (CT) mutations (Hernandez et al., 2016). Recombinant studies of these mutant α5β3γ2 GABAARs indicated no pronounced changes in surface or total α5 levels, while functional deficiencies ranged from reduced currents and gating defects to altered channel activation and deactivation. A V294L (M2, pore-lining helix) mutation identified in a patient with severe early-onset epilepsy and developmental delay showed receptors with 10 times greater GABA sensitivity, although maximal GABA currents were reduced by increased receptor desensitization (Butler et al., 2018). An autism patient pilot PET imaging study with the α5 preferring tracer [11C]Ro15-4513 identified reduced α5 binding across multiple brain regions (Mendez et al., 2013), while another recent study showed changes in a GABA-sensitive perceptual task without differences in binding (Horder et al., 2018). As both studies were without genetic information, this suggests further testing with patient stratification by exome data could provide greater insight. Despite being a genetically heterogeneous disorder, the potential utility for mechanism-based GABAAR pharmacologic treatment with ASDs is supported by shared pathologies both in patients and related mouse models.
α5 GABAAR Therapeutics
NAMs that selectively reduce α5 GABAAR function have been heavily pursued for the potential development of cognitive enhancing or “smart” drugs. The following are a selection of α5 GABAAR NAMs: L-655,708, α5IA, Ro15-4513, MRK-016, RO4938581, and RY-80 (reviewed in Clayton et al., 2015; Sieghart and Savic, 2018). Importantly, α5 NAMs did not exhibit the convulsant or pro-convulsant activity of more general alpha subunit NAMs, had good oral bioavailability and easily crossed the blood brain barrier (reviewed in Atack, 2011). In contrast to NAMs which act via the GABAAR benzodiazepine binding site, S44819 was recently identified as a competitive antagonist of GABA at α5 GABAAR and showed similar pro-cognitive effects as NAMs: blocking α5-GABAAR tonic current, enhancing LTP, reversing scopolamine-induced impairment of spatial working memory and enhancing object recognition memory (Ling et al., 2015; Etherington et al., 2017). Finally, recent evidence for beneficial effects of positive allosteric modulators (PAMs) in aged brain cognition, autism, depression and schizophrenia has bolstered α5 PAM drug development. A selection of α5 preferring PAMs includes SH-053-R-CH3-2′F, MP-III-022, and GL-II-73 (Sieghart and Savic, 2018; Prevot et al., 2019). Potential therapeutic applications for α5 preferring NAMs and PAMs are discussed below with a focus on CNS specific uses (Table 1), although important remaining questions exist for both in vivo specificity and receptor subtype selectivity as recently reviewed (Sieghart and Savic, 2018).
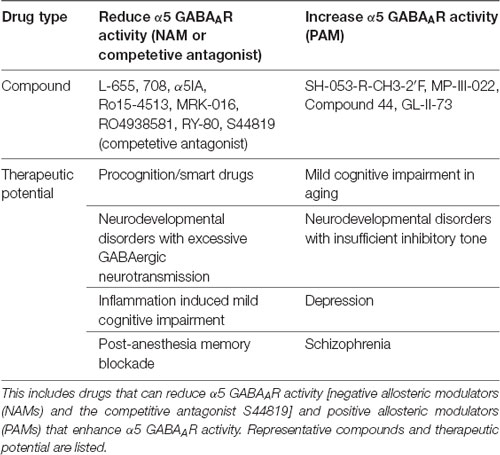
Table 1. Summary table of α5 subunit containing GABA type A receptor (α5 GABAAR) targeted drugs and potential utility.
NAM α5 GABAAR Therapeutic Applications
Pro-cognition
The ability of α5 preferring NAMs to enhance learning and memory in rodents provided crucial evidence for the importance of α5 GABAARs in these processes (Chambers et al., 2002, 2003; Street et al., 2004). The α5 NAM L-655,708, which shows approximately 50–100-fold selectivity for α5 GABAARs, reduced tonic inhibition, enhanced LTP, improved performance in the Morris water maze and generated spontaneous gamma oscillations in the CA3 region of the hippocampus (Caraiscos et al., 2004; Atack et al., 2006; Glykys et al., 2008). However anxiogenic activity and pharmacokinetics (reviewed in Atack, 2011) prevented its use in humans. Although α5IA was non-anxiogenic and reduced ethanol-induced learning impairment in young volunteers, prolonged use was prevented by high dose renal toxicity (Atack, 2010). MRK-016 showed pro-cognitive efficacy and was non-anxiogenic; poor compound tolerance in the elderly stopped further clinical development (Atack et al., 2009). Efforts to develop clinically successful α5 NAM are ongoing.
Developmental Disorders
Down syndrome mice (Ts65Dn) show cognitive impairment due to excessive GABAergic inhibition. Acute treatment with α5IA reversed deficits in novel object recognition and spatial learning and was able to restore deficits of immediate early genes expression during memory processing (Braudeau et al., 2011). Although Ts65Dn mice show no major changes in α5 GABAAR levels (Deidda et al., 2015), growing evidence indicates increased α5 GABAAR activity is an important pathological component, as genetic ablation of α5 GABAARs partially rescues learning, LTP and neuromorphological changes (Vidal et al., 2018). Furthermore, a recent study revealed a specific increase in GABAAR dendritic inhibition in Ts65Dn mice that led to reduced NMDAR activation and impaired LTP that could be restored with α5 NAM treatment (Schulz et al., 2019). Rdx−/−mice have increased GABAergic inhibition via enhanced α5 synaptic levels, impaired short-term memory and a reversal learning deficit, with the latter being improved with α5IA treatment (Hausrat et al., 2015). The subsequently identified α5 NAM RO4938581, with high affinity and efficacy at α5 GABAARs vs. α1–3 GABAARs (Ballard et al., 2009), demonstrated efficacy in Ts65Dn mice at improving spatial memory, reversing LTP deficits, and restoring neurogenesis while reducing both hyperactivity and the enhanced density of hippocampal GABAergic boutons (Martínez-Cué et al., 2013). Although these pharmacological successes led to a Phase II clinical trial for a related compound RG1662 (Hoffman-La Roche) in Down syndrome patients, the trial did not meet the primary and secondary endpoints of improved cognition and function.
Inflammation Induced Mild Cognitive Impairment and Post Anesthesia Memory Blockade
Increased systemic inflammation caused by pathological events such as stroke, infection, and traumatic brain injury is associated with memory problems during recovery from the initial insult. In an acute inflammation model, increased tonic α5 GABAAR current and surface levels via P38 MAPK signaling was central to generating inflammation induced memory deficits (Wang et al., 2012). Importantly, these inflammation induced memory impairments were absent in Gabra5−/− mice and could be blocked by treatment with the α5 NAMs L-655,708 or MRK-016. Similarly, following stroke injury, tonic inhibition is increased in the peri-infarct zone, and L-655,708 treatment from 3-days post-stroke increases functional recovery (Clarkson et al., 2010). Gabra5−/− mice also exhibited improved motor recovery post-stroke. Sustained upregulation of α5 GABAARs is also indicated in memory blockade following anesthesia (Zurek et al., 2014). Both the injectable anesthetic etomidate and the inhaled anesthetic isoflurane increase α5 GABAAR tonic conductance, promoting the amnesic properties of these drugs (Cheng et al., 2006; Martin et al., 2009b; Saab et al., 2010). Pharmacological inhibition of α5 GABAARs reduces anesthetic potentiation of GABAARs (Lecker et al., 2013) and restores recognition memory in mice after anesthesia. Recent investigation of age-dependent efficacy of L-655,708 showed that α5 NAM treatment prior or following anesthesia restored spatial learning and memory in young rats, while aged rats only showed improvement with α5 NAM treatment prior to anesthesia (Zhao et al., 2019). Importantly, low dose isoflurane downregulated α5 mRNA in aging hippocampal neurons but upregulated α5 mRNA in neurons from young animals. This suggests different approaches will be needed to improve post anesthesia memory blockade in young vs. aged populations.
PAM α5 GABAAR Therapeutic Applications
Neurodevelopmental Disorders
Mouse models of neurodevelopmental disorders that present with insufficient inhibitory tone show improvement with positive modulators of GABAAR signaling. In the Scn1a+/− mouse model of Dravet syndrome, a severe childhood epileptic encephalopathy syndrome with hyperactivity and autism behaviors, abnormal social behaviors and fear memory deficits were rescued following treatment with a benzodiazepine, clonazepam (Han et al., 2014). In an ASD mouse model with reduced GABAAR-mediated inhibition, the BTBR T+tf/J mouse, the α2,3 and 5 PAM L-838,417, improved deficits in social interaction, repetitive behaviors, and spatial learning (Han et al., 2014).
Mild Cognitive Impairment in Aging
Although α5 GABAAR NAMs enhance memory in young rodents, it appears positive modulation may be more therapeutic in aging brains impaired by excess activity. Particularly in disorders such as Alzheimer’s which are hallmarked by overexcitation (Ambrad Giovannetti and Fuhrmann, 2019), enhanced cognition may be achieved with reducing pathological excitability, as observed with the FDA approved NMDAR antagonist memantine. Furthermore, there is growing evidence for a general decline in GABAergic inhibitory tone in aging humans, monkeys and rodents (Rozycka and Liguz-Lecznar, 2017; Lissemore et al., 2018). From this newer perspective, an α5 GABAAR PAM focused approach (Compound 44) identified improved hippocampal-dependent memory in aged rats with cognitive impairment (Koh et al., 2013).
Depression and Schizophrenia
Another important unmet need where α5 GABAARs PAM pharmacotherapy may be applicable is in the development of new fast-acting anti-depressant drugs. Most current antidepressants act on the monoaminergic systems, and are only moderately therapeutically efficacious after dosing for several weeks. Significant evidence links GABAergic deficits with major depressive disorders (MDD) (Luscher et al., 2011). Investigation of anti-depressant activity of the α5 PAM SH-053-2′F-R-CH3 showed stress reduction in female mice both as an acute and chronic treatment (Piantadosi et al., 2016). Although male mice did not respond to PAM treatment, they also failed to show the upregulation of Gabra5 gene expression following unpredictable chronic mild stress seen in female mice. This particular PAM was also able to reverse pathological increases in dopaminergic activity in the MAM-model of schizophrenia (Gill et al., 2011). GL-II-73 a recently developed α5 preferring PAM showed anxiolytic and antidepressant efficacy, reversing stress-induced and age-related working memory deficits both in male and female mice (Prevot et al., 2019). Somewhat contradictory to this data and the GABA deficit hypothesis of MDD, α5 NAM have also shown rapid antidepressant actions in mice, potentially via ketamine like mechanisms of disinhibition (Fischell et al., 2015; Zanos et al., 2017).
Conclusion
Due to the unique physiology and pharmacology of α5 GABAARs, these receptors are being targeted and tested as treatments for neurodevelopmental disorders, mild cognitive impairment, depression and schizophrenia. The recent cryo-EM studies of heteropentameric synaptic GABAARs and binding of GABA, antagonists, and benzodiazepines should further advance α5 subtype specific structure-based drug design. Despite the progress in understanding of α5 GABAAR neurobiology, comparatively little is understood regarding mechanisms that regulate α5 GABAAR trafficking, stability, and both synaptic and extrasynaptic clustering. Furthermore, understanding of α5 GABAAR plasticity occurring from endogenous signaling mechanisms and from drug treatments in the developing, mature and aging brain will be needed to effectively and safely advance therapeutic application of α5 GABAAR preferring drugs.
Author Contributions
TJ prepared the figure, table and wrote the manuscript.
Funding
This work was supported by National Institute of Mental Health (NIMH) 1R01MH114908-01A1.
Conflict of Interest Statement
The author declares that the research was conducted in the absence of any commercial or financial relationships that could be construed as a potential conflict of interest.
References
Ali, A. B., and Thomson, A. M. (2008). Synaptic α5 subunit-containing GABAA receptors mediate IPSPs elicited by dendrite-preferring cells in rat neocortex. Cereb. Cortex 18, 1260–1271. doi: 10.1093/cercor/bhm160
Ambrad Giovannetti, E., and Fuhrmann, M. (2019). Unsupervised excitation: GABAergic dysfunctions in Alzheimer’s disease. Brain Res. 1707, 216–226. doi: 10.1016/j.brainres.2018.11.042
Araujo, F., Ruano, D., and Vitorica, J. (1999). Native γ-aminobutyric acid type A receptors from rat hippocampus, containing both α 1 and α 5 subunits, exhibit a single benzodiazepine binding site with α 5 pharmacological properties. J. Pharmacol. Exp. Ther. 290, 989–997.
Atack, J. R. (2010). Preclinical and clinical pharmacology of the GABAA receptor α5 subtype-selective inverse agonist α5IA. Pharmacol. Ther. 125, 11–26. doi: 10.1016/j.pharmthera.2009.09.001
Atack, J. R. (2011). GABAA receptor subtype-selective modulators: II. α5-selective inverse agonists for cognition enhancement. Curr. Top. Med. Chem. 11, 1203–1214. doi: 10.2174/156802611795371314
Atack, J. R., Bayley, P. J., Seabrook, G. R., Wafford, K. A., Mckernan, R. M., and Dawson, G. R. (2006). L-655,708 enhances cognition in rats but is not proconvulsant at a dose selective for α5-containing GABAA receptors. Neuropharmacology 51, 1023–1029. doi: 10.1016/j.neuropharm.2006.04.018
Atack, J. R., Maubach, K. A., Wafford, K. A., O’Connor, D., Rodrigues, A. D., Evans, D. C., et al. (2009). In vitro and in vivo properties of 3-tert-butyl-7-(5-methylisoxazol-3-yl)-2-(1-methyl-1H-1,2,4-triazol-5-ylmethoxy)- pyrazolo[1,5-d]-[1,2,4]triazine (MRK-016), a GABAA receptor α5 subtype-selective inverse agonist. J. Pharmacol. Exp. Ther. 331, 470–484. doi: 10.1124/jpet.109.157636
Bader, B. M., Steder, A., Klein, A. B., Frølund, B., Schroeder, O. H. U., and Jensen, A. A. (2017). Functional characterization of GABAA receptor-mediated modulation of cortical neuron network activity in microelectrode array recordings. PLoS One 12:e0186147. doi: 10.1371/journal.pone.0186147
Bakker, C. E., and Oostra, B. A. (2003). Understanding fragile X syndrome: insights from animal models. Cytogenet. Genome Res. 100, 111–123. doi: 10.1159/000072845
Balic, E., Rudolph, U., Fritschy, J.-M., Mohler, H., and Benke, D. (2009). The α5(H105R) mutation impairs α5 selective binding properties by altered positioning of the α5 subunit in GABAA receptors containing two distinct types of α subunits. J. Neurochem. 110, 244–254. doi: 10.1111/j.1471-4159.2009.06119.x
Ballard, T. M., Knoflach, F., Prinssen, E., Borroni, E., Vivian, J. A., Basile, J., et al. (2009). RO4938581, a novel cognitive enhancer acting at GABAA α5 subunit-containing receptors. Psychopharmacology 202, 207–223. doi: 10.1007/s00213-008-1357-7
Ben-Ari, Y. (2002). Excitatory actions of gaba during development: the nature of the nurture. Nat. Rev. Neurosci. 3, 728–739. doi: 10.1038/nrn920
Ben-Ari, Y., Gaiarsa, J. L., Tyzio, R., and Khazipov, R. (2007). GABA: a pioneer transmitter that excites immature neurons and generates primitive oscillations. Physiol. Rev. 87, 1215–1284. doi: 10.1152/physrev.00017.2006
Bonin, R. P., Martin, L. J., Macdonald, J. F., and Orser, B. A. (2007). α5GABAA receptors regulate the intrinsic excitability of mouse hippocampal pyramidal neurons. J. Neurophysiol. 98, 2244–2254. doi: 10.1152/jn.00482.2007
Brady, M. L., and Jacob, T. C. (2015). Synaptic localization of α5 GABAA receptors via gephyrin interaction regulates dendritic outgrowth and spine maturation. Dev. Neurobiol. 75, 1241–1251. doi: 10.1002/dneu.22280
Braudeau, J., Dauphinot, L., Duchon, A., Loistron, A., Dodd, R. H., Hérault, Y., et al. (2011). Chronic treatment with a promnesiant GABA-A α5-selective inverse agonist increases immediate early genes expression during memory processing in mice and rectifies their expression levels in a down syndrome mouse model. Adv. Pharmacol. Sci. 2011:153218. doi: 10.1155/2011/153218
Brünig, I., Scotti, E., Sidler, C., and Fritschy, J. M. (2002). Intact sorting, targeting, and clustering of γ-aminobutyric acid A receptor subtypes in hippocampal neurons in vitro. J. Comp. Neurol. 443, 43–55. doi: 10.1002/cne.10102
Butler, K. M., Moody, O. A., Schuler, E., Coryell, J., Alexander, J. J., Jenkins, A., et al. (2018). De novo variants in GABRA2 and GABRA5 alter receptor function and contribute to early-onset epilepsy. Brain 141, 2392–2405. doi: 10.1093/brain/awy171
Caraiscos, V. B., Elliott, E. M., You-Ten, K. E., Cheng, V. Y., Belelli, D., Newell, J. G., et al. (2004). Tonic inhibition in mouse hippocampal CA1 pyramidal neurons is mediated by α5 subunit-containing γ-aminobutyric acid type A receptors. Proc. Natl. Acad. Sci. U S A 101, 3662–3667. doi: 10.1073/pnas.0307231101
Chambers, M. S., Atack, J. R., Bromidge, F. A., Broughton, H. B., Cook, S., Dawson, G. R., et al. (2002). 6,7-Dihydro-2-benzothiophen-4(5H)-ones: a novel class of GABA-A α5 receptor inverse agonists. J. Med. Chem. 45, 1176–1179. doi: 10.1021/jm010471b
Chambers, M. S., Atack, J. R., Broughton, H. B., Collinson, N., Cook, S., Dawson, G. R., et al. (2003). Identification of a novel, selective GABAA α5 receptor inverse agonist which enhances cognition. J. Med. Chem. 46, 2227–2240. doi: 10.1021/jm020582q
Chen, X., Keramidas, A., and Lynch, J. W. (2017). Physiological and pharmacological properties of inhibitory postsynaptic currents mediated by α5β1γ2, α5β2γ2 and α5β3γ2 GABAA receptors. Neuropharmacology 125, 243–253. doi: 10.1016/j.neuropharm.2017.07.027
Cheng, V. Y., Martin, L. J., Elliott, E. M., Kim, J. H., Mount, H. T., Taverna, F. A., et al. (2006). α5GABAA receptors mediate the amnestic but not sedative-hypnotic effects of the general anesthetic etomidate. J. Neurosci. 26, 3713–3720. doi: 10.1523/JNEUROSCI.5024-05.2006
Christie, S. B., and de Blas, A. L. (2002). α5 subunit-containing GABAA receptors form clusters at GABAergic synapses in hippocampal cultures. Neuroreport 13, 2355–2358. doi: 10.1097/00001756-200212030-00037
Clarkson, A. N., Huang, B. S., Macisaac, S. E., Mody, I., and Carmichael, S. T. (2010). Reducing excessive GABA-mediated tonic inhibition promotes functional recovery after stroke. Nature 468, 305–309. doi: 10.1038/nature09511
Clayton, T., Poe, M. M., Rallapalli, S., Biawat, P., Savic, M. M., Rowlett, J. K., et al. (2015). A review of the updated pharmacophore for the α 5 GABAA benzodiazepine receptor model. Int. J. Med. Chem. 2015:430248. doi: 10.1155/2015/430248
Collinson, N., Kuenzi, F. M., Jarolimek, W., Maubach, K. A., Cothliff, R., Sur, C., et al. (2002). Enhanced learning and memory and altered GABAergic synaptic transmission in mice lacking the α5 subunit of the GABAA receptor. J. Neurosci. 22, 5572–5580. doi: 10.1523/jneurosci.22-13-05572.2002
Crestani, F., Keist, R., Fritschy, J. M., Benke, D., Vogt, K., Prut, L., et al. (2002). Trace fear conditioning involves hippocampal α5 GABAA receptors. Proc. Natl. Acad. Sci. U S A 99, 8980–8985. doi: 10.1073/pnas.142288699
Curia, G., Papouin, T., Séguéla, P., and Avoli, M. (2009). Downregulation of tonic GABAergic inhibition in a mouse model of fragile X syndrome. Cereb. Cortex 19, 1515–1520. doi: 10.1093/cercor/bhn159
Deidda, G., Parrini, M., Naskar, S., Bozarth, I. F., Contestabile, A., and Cancedda, L. (2015). Reversing excitatory GABAA signaling restores synaptic plasticity and memory in a mouse model of Down syndrome. Nat. Med. 21, 318–326. doi: 10.1038/nm.3827
del Río, J. C., Araujo, F., Ramos, B., Ruano, D., and Vitorica, J. (2001). Prevalence between different α subunits performing the benzodiazepine binding sites in native heterologous GABAA receptors containing the α2 subunit. J. Neurochem. 79, 183–191. doi: 10.1046/j.1471-4159.2001.00551.x
Deprez, F., Vogt, F., Floriou-Servou, A., Lafourcade, C., Rudolph, U., Tyagarajan, S. K., et al. (2016). Partial inactivation of GABAA receptors containing the α5 subunit affects the development of adult-born dentate gyrus granule cells. Eur. J. Neurosci. 44, 2258–2271. doi: 10.1111/ejn.13329
Engin, E., Benham, R. S., and Rudolph, U. (2018). An emerging circuit pharmacology of GABAA receptors. Trends Pharmacol. Sci. 39, 710–732. doi: 10.1016/j.tips.2018.04.003
Etherington, L. A., Mihalik, B., Pálvölgyi, A., Ling, I., Pallagi, K., Kertesz, S., et al. (2017). Selective inhibition of extra-synaptic α5-GABAA receptors by S44819, a new therapeutic agent. Neuropharmacology 125, 353–364. doi: 10.1016/j.neuropharm.2017.08.012
Fischell, J., Van Dyke, A. M., Kvarta, M. D., LeGates, T. A., and Thompson, S. M. (2015). Rapid antidepressant action and restoration of excitatory synaptic strength after chronic stress by negative modulators of alpha5-containing GABAA receptors. Neuropsychopharmacology 40, 2499–2509. doi: 10.1038/npp.2015.112
Fuchs, T., Jefferson, S. J., Hooper, A., Yee, P. H., Maguire, J., and Luscher, B. (2017). Disinhibition of somatostatin-positive GABAergic interneurons results in an anxiolytic and antidepressant-like brain state. Mol. Psychiatry 22, 920–930. doi: 10.1038/mp.2016.188
Ge, S., Goh, E. L. K., Sailor, K. A., Kitabatake, Y., Ming, G.-L., and Song, H. (2006). GABA regulates synaptic integration of newly generated neurons in the adult brain. Nature 439, 589–593. doi: 10.1038/nature04404
Gill, K. M., Lodge, D. J., Cook, J. M., Aras, S., and Grace, A. A. (2011). A novel α5GABAAR-positive allosteric modulator reverses hyperactivation of the dopamine system in the MAM model of schizophrenia. Neuropsychopharmacology 36, 1903–1911. doi: 10.1038/npp.2011.76
Giusi, G., Facciolo, R. M., Rende, M., Alo, R., Di Vito, A., Salerno, S., et al. (2009). Distinct α subunits of the GABAA receptor are responsible for early hippocampal silent neuron-related activities. Hippocampus 19, 1103–1114. doi: 10.1002/hipo.20584
Glykys, J., Mann, E. O., and Mody, I. (2008). Which GABAA receptor subunits are necessary for tonic inhibition in the hippocampus? J. Neurosci. 28, 1421–1426. doi: 10.1523/jneurosci.4751-07.2008
Glykys, J., and Mody, I. (2006). Hippocampal network hyperactivity after selective reduction of tonic inhibition in GABAA receptor α5 subunit-deficient mice. J. Neurophysiol. 95, 2796–2807. doi: 10.1152/jn.01122.2005
Han, S., Tai, C., Jones, C. J., Scheuer, T., and Catterall, W. A. (2014). Enhancement of inhibitory neurotransmission by GABAA receptors having α2,3-subunits ameliorates behavioral deficits in a mouse model of autism. Neuron 81, 1282–1289. doi: 10.1016/j.neuron.2014.01.016
Hauser, J., Rudolph, U., Keist, R., Möhler, H., Feldon, J., and Yee, B. K. (2005). Hippocampal α5 subunit-containing GABAA receptors modulate the expression of prepulse inhibition. Mol. Psychiatry 10, 201–207. doi: 10.1038/sj.mp.4001554
Hausrat, T. J., Muhia, M., Gerrow, K., Thomas, P., Hirdes, W., Tsukita, S., et al. (2015). Radixin regulates synaptic GABAA receptor density and is essential for reversal learning and short-term memory. Nat. Commun. 6:6872. doi: 10.1038/ncomms7872
Herd, M. B., Haythornthwaite, A. R., Rosahl, T. W., Wafford, K. A., Homanics, G. E., Lambert, J. J., et al. (2008). The expression of GABAAβ subunit isoforms in synaptic and extrasynaptic receptor populations of mouse dentate gyrus granule cells. J. Physiol. 586, 989–1004. doi: 10.1113/jphysiol.2007.146746
Hernandez, C. C., Klassen, T. L., Jackson, L. G., Gurba, K., Hu, N., Noebels, J. L., et al. (2016). Deleterious rare variants reveal risk for loss of GABAA receptor function in patients with genetic epilepsy and in the general population. PLoS One 11:e0162883. doi: 10.1371/journal.pone.0162883
Hogart, A., Wu, D., Lasalle, J. M., and Schanen, N. C. (2010). The comorbidity of autism with the genomic disorders of chromosome 15q11.2-q13. Neurobiol. Dis. 38, 181–191. doi: 10.1016/j.nbd.2008.08.011
Horder, J., Andersson, M., Mendez, M. A., Singh, N., Tangen, A., Lundberg, J., et al. (2018). GABAA receptor availability is not altered in adults with autism spectrum disorder or in mouse models. Sci. Transl. Med. 10:eaam8434. doi: 10.1126/scitranslmed.aam8434
Hu, X., Rocco, B. R., Fee, C., and Sibille, E. (2018). Cell type-specific gene expression of α 5 subunit-containing γ-aminobutyric acid subtype A receptors in human and mouse frontal cortex. Mol. Neuropsychiatry 4, 204–215. doi: 10.1159/000495840
Ju, Y. H., Guzzo, A., Chiu, M. W., Taylor, P., Moran, M. F., Gurd, J. W., et al. (2009). Distinct properties of murine α5 γ-aminobutyric acid type a receptors revealed by biochemical fractionation and mass spectroscopy. J. Neurosci. Res. 87, 1737–1747. doi: 10.1002/jnr.21991
Kazdoba, T. M., Leach, P. T., Yang, M., Silverman, J. L., Solomon, M., and Crawley, J. N. (2016). Translational mouse models of autism: advancing toward pharmacological therapeutics. Curr. Top. Behav. Neurosci. 28, 1–52. doi: 10.1007/7854_2015_5003
Koh, M. T., Rosenzweig-Lipson, S., and Gallagher, M. (2013). Selective GABAA α5 positive allosteric modulators improve cognitive function in aged rats with memory impairment. Neuropharmacology 64, 145–152. doi: 10.1016/j.neuropharm.2012.06.023
Laverty, D., Desai, R., Uchanski, T., Masiulis, S., Stec, W. J., Malinauskas, T., et al. (2019). Cryo-EM structure of the human α1β3γ2 GABAA receptor in a lipid bilayer. Nature 565, 516–520. doi: 10.1038/s41586-018-0833-4
Lecker, I., Yin, Y., Wang, D. S., and Orser, B. A. (2013). Potentiation of GABAA receptor activity by volatile anaesthetics is reduced by α5GABAA receptor-preferring inverse agonists. Br. J. Anaesth. 110, i73–i81. doi: 10.1093/bja/aet038
Lévi, S., Le Roux, N., Eugène, E., and Poncer, J. C. (2015). Benzodiazepine ligands rapidly influence GABAA receptor diffusion and clustering at hippocampal inhibitory synapses. Neuropharmacology 88, 199–208. doi: 10.1016/j.neuropharm.2014.06.002
Ling, I., Mihalik, B., Etherington, L. A., Kapus, G., Pálvölgyi, A., Gigler, G., et al. (2015). A novel GABAA α 5 receptor inhibitor with therapeutic potential. Eur. J. Pharmacol. 764, 497–507. doi: 10.1016/j.ejphar.2015.07.005
Lisman, J., and Buzsáki, G. (2008). A neural coding scheme formed by the combined function of gamma and theta oscillations. Schizophr. Bull. 34, 974–980. doi: 10.1093/schbul/sbn060
Lissemore, J. I., Bhandari, A., Mulsant, B. H., Lenze, E. J., Reynolds, C. F. III., Karp, J. F., et al. (2018). Reduced GABAergic cortical inhibition in aging and depression. Neuropsychopharmacology 43, 2277–2284. doi: 10.1038/s41386-018-0093-x
Liu, Z. F., Kamatchi, G. L., Moreira, T., Mu, W., and Burt, D. R. (1998). The α5 subunit of the murine type A GABA receptor. Mol. Brain Res. 59, 84–89. doi: 10.1016/s0169-328x(98)00144-2
Loebrich, S., Bähring, R., Katsuno, T., Tsukita, S., and Kneussel, M. (2006). Activated radixin is essential for GABAA receptor α5 subunit anchoring at the actin cytoskeleton. EMBO J. 25, 987–999. doi: 10.1038/sj.emboj.7600995
Lorenz-Guertin, J. M., Bambino, M. J., Das, S., Weintraub, S. T., and Jacob, T. C. (2019). Diazepam accelerates GABAAR synaptic exchange and alters intracellular trafficking. Cell. Neurosci. 13:163. doi: 10.3389/fncel.2019.00163
Lucas-Osma, A. M., Li, Y., Lin, S., Black, S., Singla, R., Fouad, K., et al. (2018). Extrasynaptic α5GABAA receptors on proprioceptive afferents produce a tonic depolarization that modulates sodium channel function in the rat spinal cord. J. Neurophysiol. 120, 2953–2974. doi: 10.1152/jn.00499.2018
Luscher, B., Shen, Q., and Sahir, N. (2011). The GABAergic deficit hypothesis of major depressive disorder. Mol. Psychiatry 16, 383–406. doi: 10.1038/mp.2010.120
Magnin, E., Francavilla, R., Amalyan, S., Gervais, E., David, L. S., Luo, X., et al. (2019). Input-specific synaptic location and function of the α5 GABAA receptor subunit in the mouse CA1 hippocampal neurons. J. Neurosci. 39, 788–801. doi: 10.1523/JNEUROSCI.0567-18.2018
Marchionni, I., Omrani, A., and Cherubini, E. (2007). In the developing rat hippocampus a tonic GABAA-mediated conductance selectively enhances the glutamatergic drive of principal cells. J. Physiol. 581, 515–528. doi: 10.1113/jphysiol.2006.125609
Martin, L. J., Bonin, R. P., and Orser, B. A. (2009a). The physiological properties and therapeutic potential of α5-GABAA receptors. Biochem. Soc. Trans. 37, 1334–1337. doi: 10.1042/BST0371334
Martin, L. J., Oh, G. H., and Orser, B. A. (2009b). Etomidate targets α5 γ-aminobutyric acid subtype A receptors to regulate synaptic plasticity and memory blockade. Anesthesiology 111, 1025–1035. doi: 10.1097/ALN.0b013e3181bbc961
Martin, L. J., Zurek, A. A., MacDonald, J. F., Roder, J. C., Jackson, M. F., and Orser, B. A. (2010). α5GABAA receptor activity sets the threshold for long-term potentiation and constrains hippocampus-dependent memory. J. Neurosci. 30, 5269–5282. doi: 10.1523/JNEUROSCI.4209-09.2010
Martínez-Cué, C., Martínez, P., Rueda, N., Vidal, R., García, S., Vidal, V., et al. (2013). Reducing GABAA α5 receptor-mediated inhibition rescues functional and neuromorphological deficits in a mouse model of down syndrome. J. Neurosci. 33, 3953–3966. doi: 10.1523/JNEUROSCI.1203-12.2013
Masiulis, S., Desai, R., Uchanski, T., Serna Martin, I., Laverty, D., Karia, D., et al. (2019). GABAA receptor signalling mechanisms revealed by structural pharmacology. Nature 565, 454–459. doi: 10.1038/s41586-018-0832-5
Mendez, M. A., Horder, J., Myers, J., Coghlan, S., Stokes, P., Erritzoe, D., et al. (2013). The brain GABA-benzodiazepine receptor α-5 subtype in autism spectrum disorder: a pilot [11C]Ro15–4513 positron emission tomography study. Neuropharmacology 68, 195–201. doi: 10.1016/j.neuropharm.2012.04.008
Mesbah-Oskui, L., Penna, A., Orser, B. A., and Horner, R. L. (2017). Reduced expression of α5GABAA receptors elicits autism-like alterations in EEG patterns and sleep-wake behavior. Neurotoxicol. Teratol. 61, 115–122. doi: 10.1016/j.ntt.2016.10.009
Olsen, R. W., and Sieghart, W. (2009). GABAA receptors: subtypes provide diversity of function and pharmacology. Neuropharmacology 56, 141–148. doi: 10.1016/j.neuropharm.2008.07.045
Pangratz-Fuehrer, S., Sieghart, W., Rudolph, U., Parada, I., and Huguenard, J. R. (2016). Early postnatal switch in GABAA receptor α-subunits in the reticular thalamic nucleus. J. Neurophysiol. 115, 1183–1195. doi: 10.1152/jn.00905.2015
Perez-Sanchez, J., Lorenzo, L. E., Lecker, I., Zurek, A. A., Labrakakis, C., Bridgwater, E. M., et al. (2017). α5GABAA receptors mediate tonic inhibition in the spinal cord dorsal horn and contribute to the resolution of hyperalgesia. J. Neurosci. Res. 95, 1307–1318. doi: 10.1002/jnr.23981
Phulera, S., Zhu, H., Yu, J., Claxton, D. P., Yoder, N., Yoshioka, C., et al. (2018). Cryo-EM structure of the benzodiazepine-sensitive α1β1γ2S tri-heteromeric GABAA receptor in complex with GABA. eLife 7:e39383. doi: 10.7554/elife.39383
Piantadosi, S. C., French, B. J., Poe, M. M., Timic, T., Markovic, B. D., Pabba, M., et al. (2016). Sex-dependent anti-stress effect of an α5 subunit containing GABAA receptor positive allosteric modulator. Front. Pharmacol. 7:446. doi: 10.3389/fphar.2016.00446
Prenosil, G. A., Schneider Gasser, E. M., Rudolph, U., Keist, R., Fritschy, J. M., and Vogt, K. E. (2006). Specific subtypes of GABAA receptors mediate phasic and tonic forms of inhibition in hippocampal pyramidal neurons. J. Neurophysiol. 96, 846–857. doi: 10.1152/jn.01199.2006
Prevot, T. D., Li, G., Vidojevic, A., Misquitta, K. A., Fee, C., Santrac, A., et al. (2019). Novel benzodiazepine-like ligands with various anxiolytic, antidepressant, or pro-cognitive profiles. Mol. Neuropsychiatry 5, 84–97. doi: 10.1159/000496086
Prut, L., Prenosil, G., Willadt, S., Vogt, K., Fritschy, J. M., and Crestani, F. (2010). A reduction in hippocampal GABAA receptor α5 subunits disrupts the memory for location of objects in mice. Genes Brain Behav. 9, 478–488. doi: 10.1111/j.1601-183x.2010.00575.x
Ramos, B., Lopez-Tellez, J. F., Vela, J., Baglietto-Vargas, D., Del Rio, J. C., Ruano, D., et al. (2004). Expression of α 5 GABAA receptor subunit in developing rat hippocampus. Dev. Brain Res. 151, 87–98. doi: 10.1111/j.0953-816x.2004.03349.x
Renner, M., Schweizer, C., Bannai, H., Triller, A., and Lévi, S. (2012). Diffusion barriers constrain receptors at synapses. PLoS One 7:e43032. doi: 10.1371/journal.pone.0043032
Rozycka, A., and Liguz-Lecznar, M. (2017). The space where aging acts: focus on the GABAergic synapse. Aging Cell 16, 634–643. doi: 10.1111/acel.12605
Saab, B. J., Maclean, A. J., Kanisek, M., Zurek, A. A., Martin, L. J., Roder, J. C., et al. (2010). Short-term memory impairment after isoflurane in mice is prevented by the α5 γ-aminobutyric acid type A receptor inverse agonist L-655,708. Anesthesiology 113, 1061–1071. doi: 10.1097/ALN.0b013e3181f56228
Schulz, J. M., Knoflach, F., Hernandez, M.-C., and Bischofberger, J. (2018). Dendrite-targeting interneurons control synaptic NMDA-receptor activation via nonlinear α5-GABAA receptors. Nat. Commun. 9:3576. doi: 10.1038/s41467-018-06004-8
Schulz, J. M., Knoflach, F., Hernandez, M.-C., and Bischofberger, J. (2019). Enhanced dendritic inhibition and impaired NMDAR activation in a mouse model of down syndrome. J. Neurosci. 39, 5210–5221. doi: 10.1523/JNEUROSCI.2723-18.2019
Serwanski, D. R., Miralles, C. P., Christie, S. B., Mehta, A. K., Li, X., and De Blas, A. L. (2006). Synaptic and nonsynaptic localization of GABAA receptors containing the α5 subunit in the rat brain. J. Comp. Neurol. 499, 458–470. doi: 10.1002/cne.21115
Sieghart, W., and Savic, M. M. (2018). International union of basic and clinical pharmacology. CVI: GABAA receptor subtype- and function-selective ligands: key issues in translation to humans. Pharmacol. Rev. 70, 836–878. doi: 10.1124/pr.117.014449
Street, L. J., Sternfeld, F., Jelley, R. A., Reeve, A. J., Carling, R. W., Moore, K. W., et al. (2004). Synthesis and biological evaluation of 3-Heterocyclyl-7,8,9,10-tetrahydro-(7,10-ethano)-1,2,4-triazolo[3,4-a]phthalazines and analogues as subtype-selective inverse agonists for the GABAAα5 benzodiazepine binding site. J. Med. Chem. 47, 3642–3657.
Sur, C., Fresu, L., Howell, O., McKernan, R. M., and Atack, J. R. (1999). Autoradiographic localization of α5 subunit-containing GABAA receptors in rat brain. Brain Res. 822, 265–270. doi: 10.1016/s0006-8993(99)01152-x
Sur, C., Quirk, K., Dewar, D., Atack, J., and McKernan, R. (1998). Rat and human hippocampal α5 subunit-containing γ-aminobutyric AcidA receptors have α5 β3 γ2 pharmacological characteristics. Mol. Pharmacol. 54, 928–933. doi: 10.1124/mol.54.5.928
Towers, S. K., Gloveli, T., Traub, R. D., Driver, J. E., Engel, D., Fradley, R., et al. (2004). α 5 subunit-containing GABAA receptors affect the dynamic range of mouse hippocampal kainate-induced γ frequency oscillations in vitro. J. Physiol. 559, 721–728. doi: 10.1113/jphysiol.2004.071191
Vargas-Caballero, M., Martin, L. J., Salter, M. W., Orser, B. A., and Paulsen, O. (2010). α5 Subunit-containing GABAA receptors mediate a slowly decaying inhibitory synaptic current in CA1 pyramidal neurons following Schaffer collateral activation. Neuropharmacology 58, 668–675. doi: 10.1016/j.neuropharm.2009.11.005
Vidal, V., Garcia-Cerro, S., Martínez, P., Corrales, A., Lantigua, S., Vidal, R., et al. (2018). Decreasing the expression of GABAA α5 subunit-containing receptors partially improves cognitive, electrophysiological, and morphological hippocampal defects in the Ts65Dn model of down syndrome. Mol. Neurobiol. 55, 4745–4762. doi: 10.1007/s12035-017-0675-3
Wang, D.-S., Zurek, A. A., Lecker, I., Yu, J., Abramian, A. M., Avramescu, S., et al. (2012). Memory deficits induced by inflammation are regulated by α5-subunit-containing GABAA receptors. Cell Rep. 2, 488–496. doi: 10.1016/j.celrep.2012.08.022
Yu, Y., Fuscoe, J. C., Zhao, C., Guo, C., Jia, M., Qing, T., et al. (2014). A rat RNA-Seq transcriptomic BodyMap across 11 organs and 4 developmental stages. Nat. Commun. 5:3230. doi: 10.1038/ncomms4230
Zanos, P., Nelson, M. E., Highland, J. N., Krimmel, S. R., Georgiou, P., Gould, T. D., et al. (2017). A negative allosteric modulator for alpha5 subunit-containing GABA receptors exerts a rapid and persistent antidepressant-like action without the side effects of the NMDA receptor antagonist ketamine in mice. eNeuro 4. doi: 10.1523/ENEURO.0285-16.2017
Zarnowska, E. D., Keist, R., Rudolph, U., and Pearce, R. A. (2009). GABAA receptor α5 subunits contribute to GABAA,slow synaptic inhibition in mouse hippocampus. J. Neurophysiol. 101, 1179–1191. doi: 10.1152/jn.91203.2008
Zhao, Z.-F., Du, L., Gao, T., Bao, L., Luo, Y., Yin, Y.-Q., et al. (2019). Inhibition of α5 GABAA receptors has preventive but not therapeutic effects on isoflurane-induced memory impairment in aged rats. Neural Regen. Res. 14, 1029–1036. doi: 10.4103/1673-5374.250621
Zhu, S., Noviello, C. M., Teng, J., Walsh, R. M. Jr., Kim, J. J., and Hibbs, R. E. (2018). Structure of a human synaptic GABAA receptor. Nature 559, 67–72. doi: 10.1038/s41586-018-0255-3
Zurek, A. A., Kemp, S. W., Aga, Z., Walker, S., Milenkovic, M., Ramsey, A. J., et al. (2016). α5GABAA receptor deficiency causes autism-like behaviors. Ann. Clin. Transl. Neurol. 3, 392–398. doi: 10.1002/acn3.303
Keywords: GABA A receptor, alpha 5 subunit, autism, cognition, memory, development, negative and positive allosteric modulators
Citation: Jacob TC (2019) Neurobiology and Therapeutic Potential of α5-GABA Type A Receptors. Front. Mol. Neurosci. 12:179. doi: 10.3389/fnmol.2019.00179
Received: 04 June 2019; Accepted: 08 July 2019;
Published: 24 July 2019.
Edited by:
Andrea Barberis, Istituto Italiano di Tecnologia, ItalyReviewed by:
Bernhard Lüscher, Pennsylvania State University, United StatesMarianne Renner, Sorbonne Universités, France
Copyright © 2019 Jacob. This is an open-access article distributed under the terms of the Creative Commons Attribution License (CC BY). The use, distribution or reproduction in other forums is permitted, provided the original author(s) and the copyright owner(s) are credited and that the original publication in this journal is cited, in accordance with accepted academic practice. No use, distribution or reproduction is permitted which does not comply with these terms.
*Correspondence: Tija C. Jacob, dGNqMTFAcGl0dC5lZHU=