- Department of Pediatrics, Columbia University, NewYork-Presbyterian Morgan Stanley Children’s Hospital, New York, NY, United States
Developmental changes in GABAergic and glutamatergic systems during frontal lobe development have been hypothesized to play a key role in neurodevelopmental disorders seen in children born very preterm or at/with low birth weight, but the associated cellular changes have not yet been identified. Here we studied the molecular development of the GABAergic system specifically in the dorsolateral prefrontal cortex, a region that has been implicated in neurodevelopmental and psychiatric disorders. The maturation state of the GABAergic system in this region was assessed in human post-mortem brain samples, from term infants ranging in age from 0 to 8 months (n = 17 male, 9 female). Gene expression was measured for 47 GABAergic genes and used to calculate a maturation index. This maturation index was significantly more dynamic in male than female infants. To evaluate the impact of premature birth on the GABAergic system development, samples from 1-month-old term (n = 9 male, 4 female) and 1-month corrected-age very preterm (n = 8 male, 6 female) infants, were compared using the same gene list and methodology. The maturation index for the GABAergic system was significantly lower (−50%, p < 0.05) in male preterm infants, with major alterations in genes linked to GABAergic function in astrocytes, suggesting astrocytic GABAergic developmental changes as a new cellular mechanism underlying preterm brain injury.
Introduction
Premature birth, affecting 10% of live births, is a leading cause of neonatal morbidity that can induce long-term neurological impairment (Adams-Chapman and Stoll, 2006; Arpino et al., 2010; Marín, 2012; Blencowe et al., 2013; Meldrum et al., 2013; Knuesel et al., 2014). Extensive alterations in frontal lobe development have been reported in children born very preterm (<32 weeks gestation) and at very low birth weight (Hodel, 2018). Alterations include decreased prefrontal gray matter volume and surface area throughout childhood and adolescence. Decreased frontal white matter volume and poorer white matter integrity in frontal lobe tracts have also been reported, suggesting that cortical development may be delayed or permanently impaired (Volpe, 2009). Specific deficits in executive functioning in preterm children, particularly in males, have been documented across childhood and adolescence (Nosarti et al., 2006; Hodel, 2018).
Mid-to-late gestational brain development includes neurogenesis, migration, dendrite arborization and synaptogenesis, which are heavily dependent on GABA and glutamatergic neural systems and are at risk of disruption in the extra-uterine environment after preterm birth (Represa and Ben-Ari, 2005; Ben-Ari, 2006, 2014). Both neurons and astrocytes participate to the metabolism of GABA and glutamate as well as their transport and signaling (Ishibashi et al., 2019). Consistent with these observations, ex vivo studies of the preterm brain have demonstrated specific loss of GABAergic neuron populations in males (Lacaille et al., 2019), alteration of GABA receptor subunits and disorganized neuronal migration and differentiation (Robinson et al., 2006; Shaw et al., 2015, 2018).
Recent magnetic resonance spectroscopy imaging studies have suggested that GABA concentrations may be lower in preterm than term infants, but cannot assess the components of the GABAergic system that may have been altered during development. Recent reports have shown lower GABA and glutamate concentrations at term-equivalent age and a negative correlation with functional connectivity in small cohorts of preterm infants (born <35 weeks gestational age) compared to healthy full-term infants (Kwon et al., 2014; Tanifuji et al., 2017; Tomiyasu et al., 2017). Additionally, GABA concentrations were shown to correlate negatively with increasing gestational age at birth and were lower in the preterm infant brain at term equivalent age compared with healthy term controls and older children (6–16 years) (Basu et al., 2020). Similar imbalance between neuronal excitation and inhibition in the prefrontal cortex (PFC) (Marín, 2012) and decrease in prefrontal GABA concentrations have been associated with a number of psychiatric disorders, including autism spectrum disorder (ASD) (Schür et al., 2016), known to affect a high proportion of prematurely born surviving adults (Adams-Chapman and Stoll, 2006; Arpino et al., 2010; Marín, 2012; Meldrum et al., 2013; Knuesel et al., 2014; Ream and Lehwald, 2018).
Despite emerging links between psychiatric disorders and prematurity, the specific impact of preterm birth on the early maturation of neurotransmitter systems needs to be determined and compared between sexes. Thorough assessment of maturation assessment of the GABAergic system, which comprises the proteins necessary for GABA biosynthesis, degradation, release, signaling and reuptake by neurons and astrocytes, in the developing human frontal cortex is necessary to understand how prematurity results in psychiatric disorders in the absence of visible lesion and why male infants are more susceptible to develop such pathologies.
Here we delineate the molecular development of the GABAergic system in the dorsolateral PFC (Brodmann area 10; BA10), which plays a role in attention and working memory (Wang et al., 2018) and is altered in preterm infants and cases of psychiatric disorders (Hodel, 2018). Using an array of genes, we first assessed the maturation state of the GABAergic system from 0 to 8 months in human term post-mortem brain samples. We then showed that the development of the GABAergic system is delayed in male preterm infants, with major alterations on the astrocytic function. Taken together, this study shows that the maturation of the GABAergic system is more dynamic in male infants, potentially rendering them more susceptible than females to perinatal insults.
Materials and Methods
Human Samples
Human samples were obtained from the NIH Neurobiobank at University of Maryland, Baltimore, MD, United States (ID #709). BA10 sections from 17 male and 9 female term infants ranging from 0 to 8 months were obtained to assess the normal maturation of the system at perinatal ages (i.e., cross sectional study, Tables 1A,B). Additionally, sections from 7 male and 6 female preterm infants were obtained to compare their development to age-matched term infants (i.e., comparative study, Tables 1A,C). Mean age of death (absolute age) was 1.5 months in term infants and 3.7 months for preterm infants delivered between 26 and 34 weeks of gestation (average corrected age 1.1 months). Sex, completed gestational weeks and cause of death varied, but the majority were due to sudden infant death syndrome and none were attributed to CNS infection, hemorrhage or malformation (Tables 1B,C). Genetic diseases or anatomic birth defects were excluded. Brodmann Area 10 (BA10) formalin-fixed brain samples were cut into 0.5-cm-thick coronal slices and preserved in 10% neutral buffered formalin; matched frozen tissues were preserved at −80°C.
Real-Time PCR
BA10 tissues were homogenized in TRIzol™ Reagent (Thermo Fisher, Waltham, MA, United States, 15596018); total RNA was extracted with the RNeasy Mini Kit (Qiagen Venlo, Netherlands, 74104) and quantified with a Nanodrop ND-2000C (Thermo Fisher). 1 μg of RNA was used to make cDNA with the iScript cDNA Synthesis Kit (Bio-Rad, 1708891). All primer pairs were designed and validated in-house for efficiency and specificity. RT-PCR experiments were performed on cDNA samples in presence of SsoAdvanced Universal SYBR Green Supermix (Bio-Rad, Hercules, CA, United States, 1725271) with specific primers at 100 nM using the ABI Prism 7500 Sequence Detection System (Thermo Fisher). The cDNA-generated signals for target genes were normalized with transferrin receptor protein 1 (tfrc). The regulation was determined with the 2–ΔΔCq method. Results are expressed as fold change (FC) to the control group.
Western Blot
Human samples were homogenized in RIPA lysis buffer with proteinase inhibitors (Santa Cruz Biotechnology, Dallas, TX, United States, sc24948). Protein extracts, 40 μg per lane, were loaded onto 4–20% gradient gels (NuSep Inc., Germantown, MD, United States, NB10-420). Gels were electrotransferred to a 0.2 μm nitrocellulose membrane (Bio-Rad, 1620174). Blots were blocked in 5% milk in tris-buffered saline, 0.1% Tween 20 (TBST) for 1 h, and then incubated at 4 °C overnight with one of the following antibodies raised against: AATM (1:1000, sc-271702, Santa Cruz Biotechnology), AQP4 (1:1000, sc-390488), CX30 (1:1000, sc-81802), CX43 (1:1000, sc-271837), EAAT1 (1:500, sc-515839), GABRA1-6 (1:250, sc-376282), GAPDH (1:2000, sc-32233), GAT-3 (1:250, sc-376001), GDH1/2 (1:1000, sc-515542), GEPH (1:1000, sc-25311), GS (1:1000, sc-74430), MAOB (1:250, sc-515354), SNAT5 (1:1000, sc-515813), VIAAT (1:500, sc-393373), GBRD (1:250, Novus Biologicals, Littleton, CO, United States, NB300-200) or GABRG1 (1:100, Alomone Labs, Jerusalem, Israel, AGA-016). Bands were detected with appropriate horseradish peroxide-conjugated secondary antibodies, reacted with chemiluminescent ECL substrate (Bio-Rad, 1705060) and visualized with a Bio-Rad ChemiDoc Imaging system. Band intensity was measured using the ImageJ program (NIH) and normalized with GAPDH.
Immunohistochemical Procedure
Formalin-fixed tissues were cryoprotected in a 30% sucrose solution and embedded in Tissue-Tek® O.C.T. Compound (Sakura® FineTek, Torrance, CA, United States). Blocks were cut into 25-μm-thick sections on a cryostat and mounted on Superfrost Plus (Thermo Fisher) glass slides. Frozen sections were allowed to equilibrate to room temperature for 2 h before staining.
Procedure
Tissue sections were rinsed in PBS-Triton 0.3% (PBS-T) then blocked in PBS-T with 10% normal donkey serum (NDS) followed by overnight incubation at 4°C in PBS-T-10% NDS with primary antibodies raised against: Calbindin (1:1000, Swant, Marly, Switzerland, CB300 or CB38), Calretinin (1:1000, Millipore Sigma, AB1550), Gad65-67 (1:200 Santa Cruz Biotechnology, sc-365180), GFAP (1:1000, Agilent Dako, Glostrup, Denmark, Z0334), Neuropeptide Y (1:500, Immunostar, Hudson, WI, United States, 22940) or Somatostatin (1:300, Santa Cruz Biotechnology, sc7819). For secondary detection, appropriately matched Alexa Fluor-conjugated secondary antibodies (1:500, Thermo Fisher) were incubated 90 min in PBS-T at room temperature. Sections were incubated with DAPI, mounted in Fluoromount G (Thermo Fisher) and coverslipped before epifluorescence examination with a slide scanner (Leica DMI6000 B, Leica, Wetzlar, Germany).
Quantification
Cell density was assessed in the upper layers (UL), lower layers (LL), and the subcortical white matter (SC-WM) of BA10 and expressed in cells per mm2. Cortical layering was determined with DAPI counterstaining. Cell quantification was performed using the Qpath software (Edinburgh, United Kingdom). All counts were performed blind to condition.
Statistics
All experiments and analyses were performed blind to conditions. Statistical analysis was performed using PRISM software (GraphPad Software 6.0, San Diego, CA, United States). Normal distribution of each dataset was analyzed by Shapiro–Wilk test. When two conditions were compared, data were analyzed with a non-parametric Mann–Whitney test. When four experimental groups were assessed and two variables were taken into consideration, data were analyzed with a two-way ANOVA with Fisher LSD, Sidak’s or Tukey’s multiple comparisons. The maturation index and the developmental trajectories of transcripts and proteins in term infants was quantified by fitting linear regression slopes to measure development through postnatal months. To compare the difference of two slopes, data were analyzed with an ANCOVA The null hypothesis was rejected for alpha greater than 5%.
Results
BA10, also known as dorsolateral prefrontal cortex, is one of the brain regions most frequently altered in psychiatric diseases. To address whether prematurity alters the maturation of the GABAergic system, a cross sectional developmental study was designed. The expression of 47 transcripts related to the GABA system were determined in pathology specimens from male and female term infant across multiple early postnatal time points (Tables 1A,B and Figures 1A–D). Screened genes encoding different components of the GABAergic signaling system included: GABA receptor subunits, metabolic enzymes, transporters, ion channels associated to the GABAergic function, interneuron specific markers, GABA receptor anchorage proteins and enzymes involved in the metabolism of glutamate and GABA (Table 2).
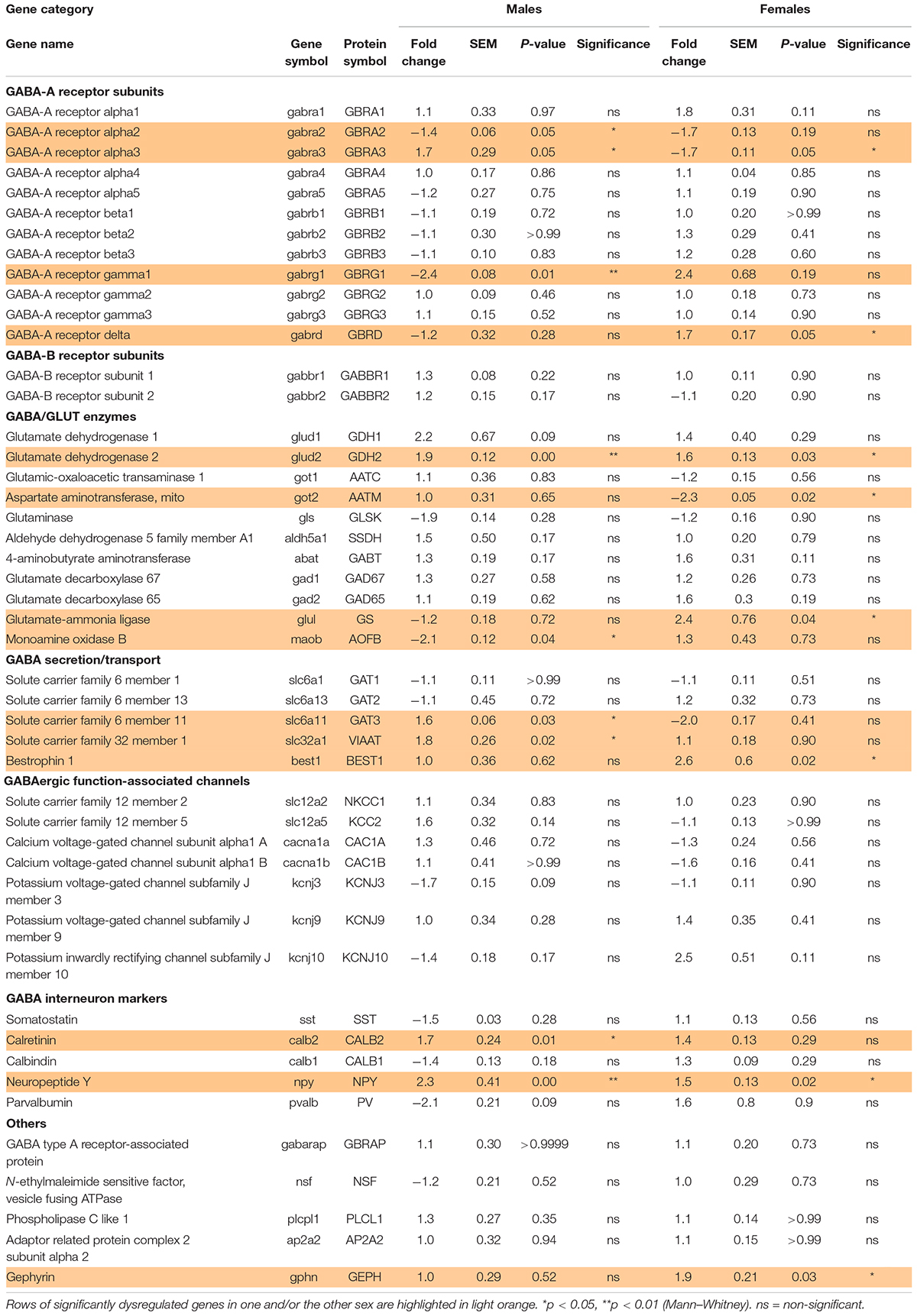
Table 2. Effect of preterm birth on the expression of GABA system-associated gene in the BA10 of male and female infants determined by qRT-PCR (comparative study).
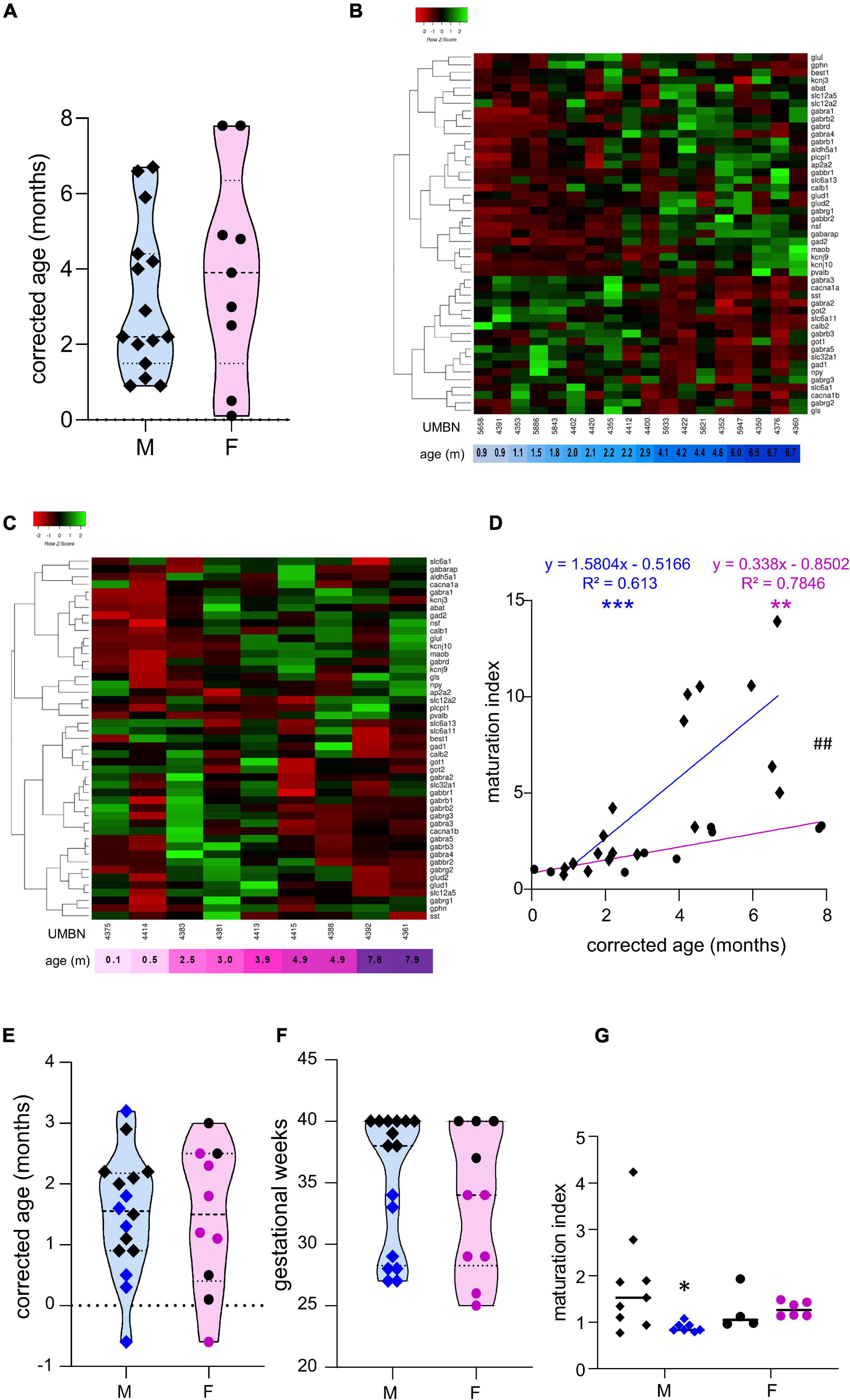
Figure 1. Preterm birth alters the maturation of the GABAergic system. (A) Violin plot displaying the distribution of male (M, blue, diamonds) and female (F, pink, circles) term infants included in the cross sectional study. Violin plots show individual values and include minima, maxima and median values. (B,C) Heatmap of GABA-associated gene expression means in the BA10 of (B) male and (C) female term infants, arranged using hierarchical clustering (complete linkage with Pearson distance measurement method). (D) Maturation index based on the heatmap clustering of male (blue, diamonds) and female (purple, circles) term infants cross sectional study, modeled with a linear regression, **p < 0.01, ***p < 0.001. ##p < 0.01 (ANCOVA, analysis of co-variance). (E,F) Violin plot displaying the distribution of male (M, blue, diamonds) and female (F, purple, circles) infants, term (black) versus preterm (colored) infants included in the comparative study according to (E) their corrected age (in months) and (F) gestational weeks. (G) Maturation index of male and female term (black) and preterm (colored) infants comparative study, based on the heatmap clustering of the term infants cross-sectional study. *p < 0.05 (two-way ANOVA with Sidak’s multiple comparisons).
The Maturation of the GABAergic System Is More Dynamic in Male Than Female Term Infants
To estimate the overall maturation of the GABAergic system based on gene expression level, a maturation index was calculated as previously described (Gandal et al., 2012). Expression levels for most genes changed monotonically across development, either increasing or decreasing. The ratio of the average of developmentally upregulated over downregulated genes also increases monotonically with time. The genes analyzed were plotted into a heatmap and separated into up- and down-regulated genes using the first linkage of the heatmap for male (Figure 1B) and female infants independently (Figure 1C). Equal weight was given to all genes within the maturation index. The ratio of averaged upregulated (29 genes) over averaged downregulated genes (18 genes) was used as a maturation index for the development of the GABAergic system in term males; and similarly in term female infants with 24 upregulated and 23 downregulated genes (Figure 1D). As expected, the maturation index significantly correlated with the age of the donor in males (R2 = 0.61, p < 0.0001, Figure 1D) and in females (R2 = 0.78, p < 0.0001, Figure 1D). Notably, the slope of the maturation index was significantly greater in males compared to females (p < 0.001, Figure 1D), indicating broader maturation dynamic in the former.
The Maturation of the GABAergic System Is Altered in Preterm Infants
To investigate the possibility of a maturation delay of the GABAergic system in preterm infants, a comparative study was designed. Corrected age matched preterm infants and the youngest term infants (0–3 months) were compared (Tables 1A–C and Figures 1E–G). The same transcripts were analyzed in male and female term and preterm cerebral cortices. The maturation index of term and preterm infants was calculated as ratio of the average of developmentally upregulated over downregulated genes, as previously defined with the cross-sectional developmental study. Interestingly, the maturation index was decreased in male (−50%, p < 0.05, Figure 1G) but not female preterm infants (−6% ns, Figure 1G) when compared, respectively, to their matched term group. The reduced maturation index in preterm male infants signifies that prematurity induces gene expression changes in the opposite direction from that occurring normally during GABAergic system development. To gain insight into the function of these genes, their expression was analyzed individually (Table 2).
Preterm Birth Delays the Development of the GABAergic System in Male Infants
Out of 47 transcripts, nine were significantly dysregulated in male preterm infants. Only gabra2 varied according to its predicted developmental trajectory by linear regression, which suggest an accelerated maturation. Indeed, gabra2 expression is predicted to decrease over time (p < 0.01, Figure 2A) and was decreased in preterm male infants (FC = −1.4, p < 0.5, Table 2 and Figure 2A’). Most of the transcripts varied oppositely to their predicted developmental trajectory suggesting an overall developmental delay (Table 2 and Figure 2). The dysregulated transcripts included: two GABAA receptor subunits, gabra3 (FC = 1.7, p < 0.05, Table 2) and gabrag1 (FC = −2.4, p < 0.01, Table 2 and Figures 2B,B’); two enzymes: glud2 (enzyme catalyzing the reversible interconversion of glutamate to α-ketoglutarate, FC = 1.9, p < 0.01, Table 2 and Figures 2C,C’) and maob (enzyme responsible for the production of GABA in astrocytes, FC = −2.1, p < 0.05, Table 2 and Figures 2D,D’); two transporters: slc6a11 (gaba transporter in astrocytes, FC = 1.6, p < 0.05, Table 2 and Figures 2E,E’) and slc32a1 (vesicular transporter, FC = 1.6 p < 0.05, Table 2); two markers of GABAergic interneuron subpopulation: crt (calretinin, FC = 1.7, p < 0.05, Table 2) and npy (neuropeptide Y, FC = 2.3, p < 0.001, Table 2). Further examination revealed that four of these genes were associated to astrocytic functions including gabrg1, gdh2, maob, and gat3. Additional astrocyte-related genes were trending toward significance (Table 2; glud1 FC = 2.2, gls FC = −1.9, and aldh5a1 FC = 1.5, enzymes part of the TCA cycle). The majority of protein expression levels assessed by western blot covaried positively with the mRNA changes in males born preterm, suggesting that the mRNAs of interest were actively synthetized (Figure 2).
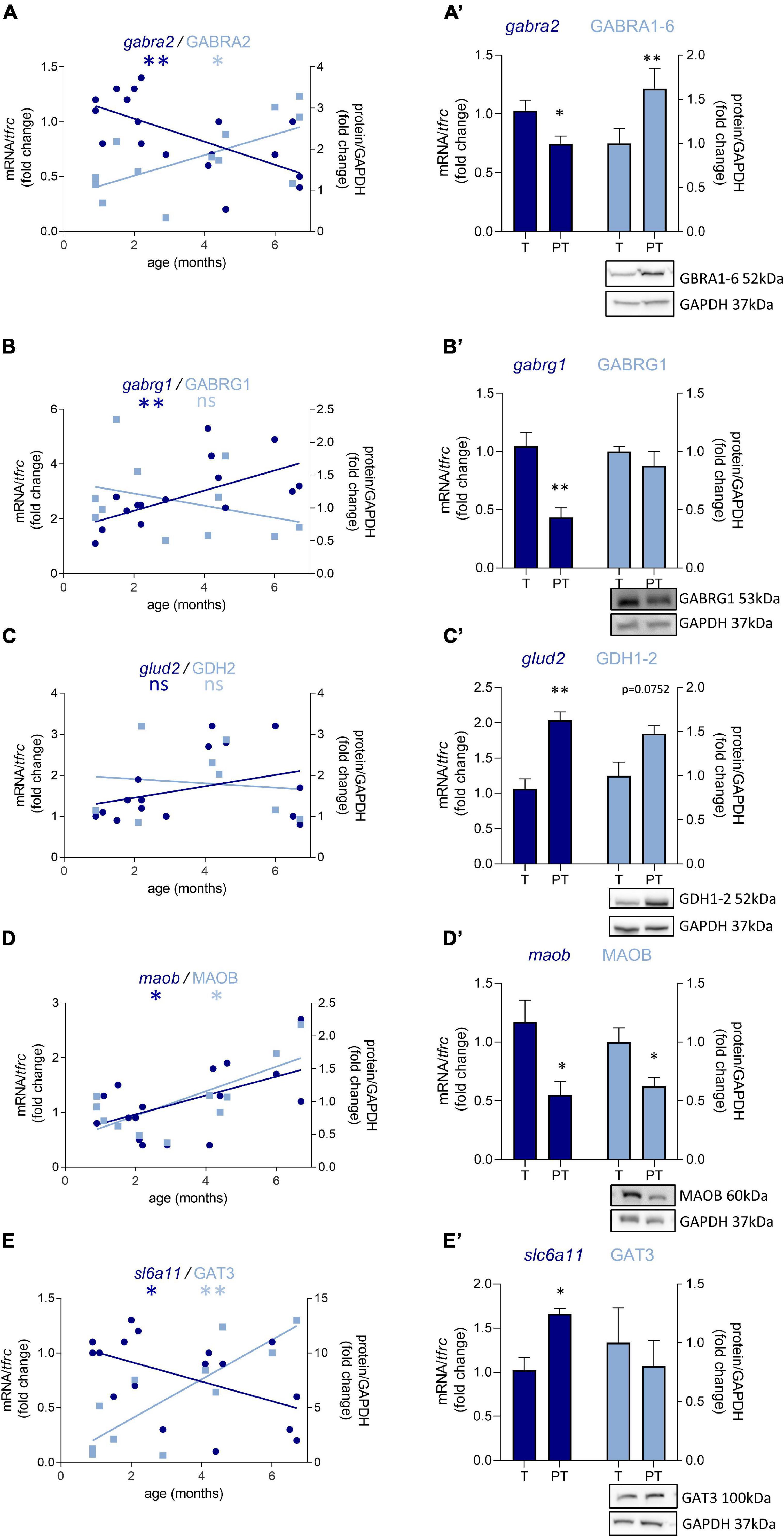
Figure 2. Preterm birth alters the expression of GABA-related transcripts (dark blue) and proteins (light blue) in male infant BA10. (A–E) Developmental regulation in term infants included in the cross sectional study, modeled with a linear regression *p < 0.05, **p < 0.01. (A’–E’) Effect of preterm birth (comparative study) on the expression of GABA-related transcripts and proteins. Quantification of mRNA level changes by qRT-PCR of (A,A’) gabra2, (B,B’) gabrg1, (C,C’) glud2, (D,D’) maob, and (E,E’) slc6a11. tfrc was used for normalization. Quantification of protein expression changes by Western blot of (A,A’) GABRA1-6, (B,B’) GABRG1, (C,C’) GDH2, (D,D’) MAOB, and (E,E’) GAT3. GAPDH was used for normalization. *p < 0.05, **p < 0.01 (Mann–Whitney). Representative blot below the graph. PT, preterm; T, term.
Preterm Birth Accelerates the Development of the GABAergic System in Female Infants
In female preterm infants, 8 out of 47 transcripts were significantly dysregulated, most varied accordingly to their developmental trajectory suggesting an accelerated development or to resemble their non-corrected age counterpart (Table 2 and Figure 3) -gabra3 and gabrd (GABAA receptor subunits) -glud2, got2, and glul (enzymes responsible for the glutamine to glutamate conversion) –best1 (GABA transporter in astrocytes) -npy (interneuron subtype) and geph (responsible for GABA receptor anchorage). Similarly to males, the majority of protein expression mostly covaried with mRNA in female preterm infants (Figure 3).
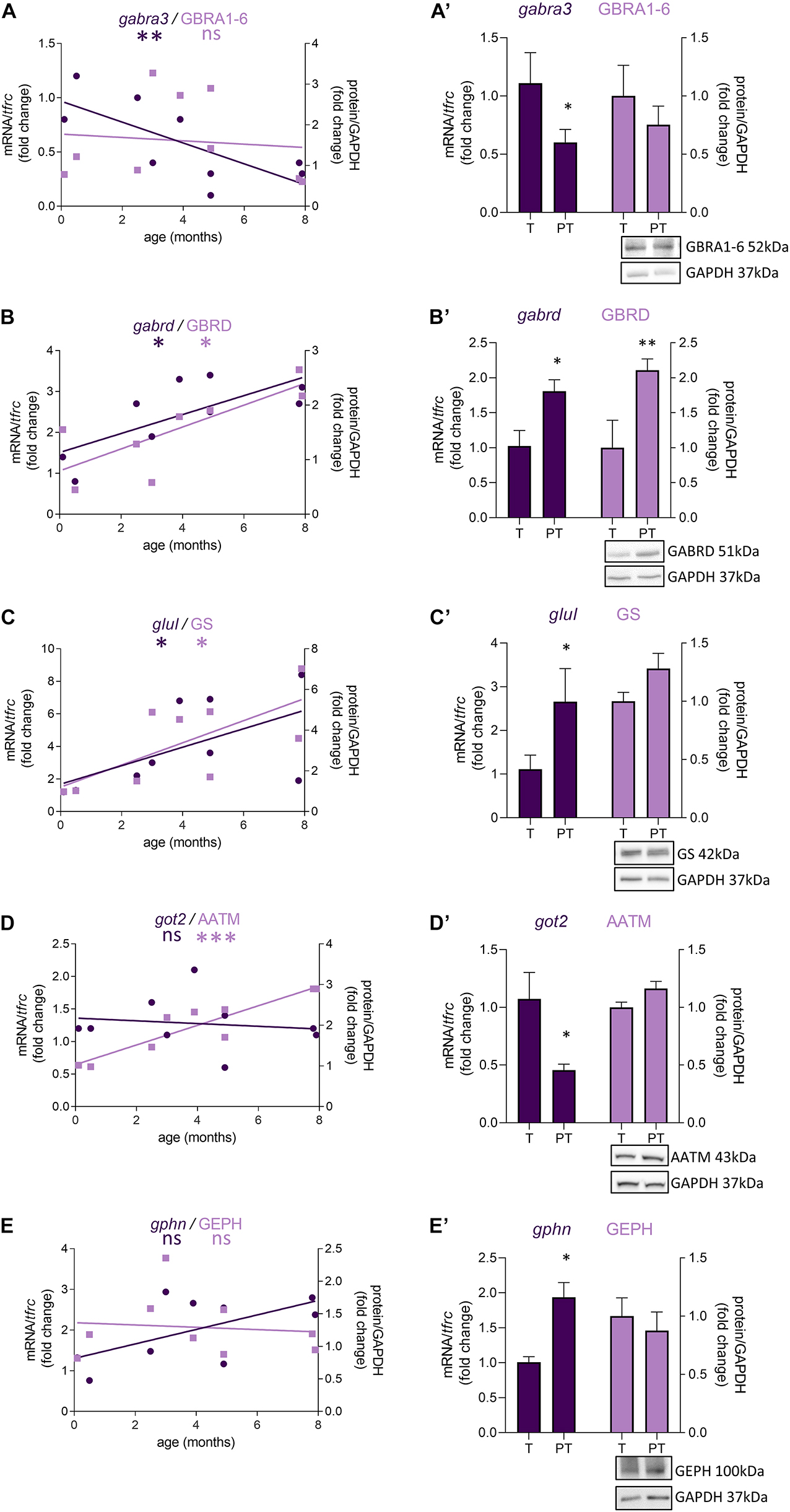
Figure 3. Preterm birth impacts the expression of GABA-related transcripts (dark purple) and proteins (light purple) in female infants. (A–E) Developmental regulation in term infants included in the cross sectional study, modeled with a non-linear regression *p < 0.05, ***p < 0.01, ***p < 0.001. (A’–E’) Effect of preterm birth on the expression of GABA-related transcripts and proteins (comparative study). Quantification of mRNA level changes by qRT-PCR of (A,A’) gabra3, (B,B’) gabrd, (C,C’) glul, (D,D’) got2, and (E,E’) gphn. tfrc was used for normalization. Quantification of protein expression changes by Western blot of (A,A’) GABRA1-6, (B,B’) GABRD, (C,C’) GS, (D,D’) AATM, and (E,E’) GEPHN. GAPDH was used for normalization. *p < 0.05, **p < 0.01 (Mann–Whitney). Representative blot below the graph. PT, preterm; T, term.
Preterm Birth Primarily Affects the Astrocytic GABA Function in Male Infants
Since most of the perturbations observed in male preterm infants seemed to be related to the astrocytic regulation of GABAergic signaling, 10 supplementary transcripts were analyzed to evaluate specifically the metabolism and morphogenesis, and ten others to evaluate the involvement of astrocytes in the GABAergic system (Figure 4 and Table 3). A maturation index specific to astrocytes was calculated with the averaged upregulated over averaged downregulated genes using the first linkage of the astrocyte-specific heatmap, individually in males (Figure 4A) and females (Figure 4B). The genes plotted were 14 genes related to astrocytes and GABA included in Table 2 (glul, best1, abat, aldh5a1, glud2, glud1, gabrg1, maob, kcnj10, slc6a11, got1, gabrg3, gls, and got2) and 20 newly added genes (Table 3). The maturation index was significantly correlated with the age of the donors both in males (R2 = 0.54, p < 0.001, Figure 4C) and in females (R2 = 0.44, p < 0.001, Figure 4C). Across the spam of ages examined (0–8 months), astrocytic maturation was significantly more dynamic in male infants since the slope of their maturation index was significantly superior to the one in female infants (p < 0.01, Figure 4C). The same transcripts were analyzed in term and preterm infants to estimate their astrocyte-related maturation indices. The astrocyte-maturation index was significantly decreased in male (−50%, p < 0.05, Figure 4D) but not female preterm infants (−13% ns, Figure 4D).
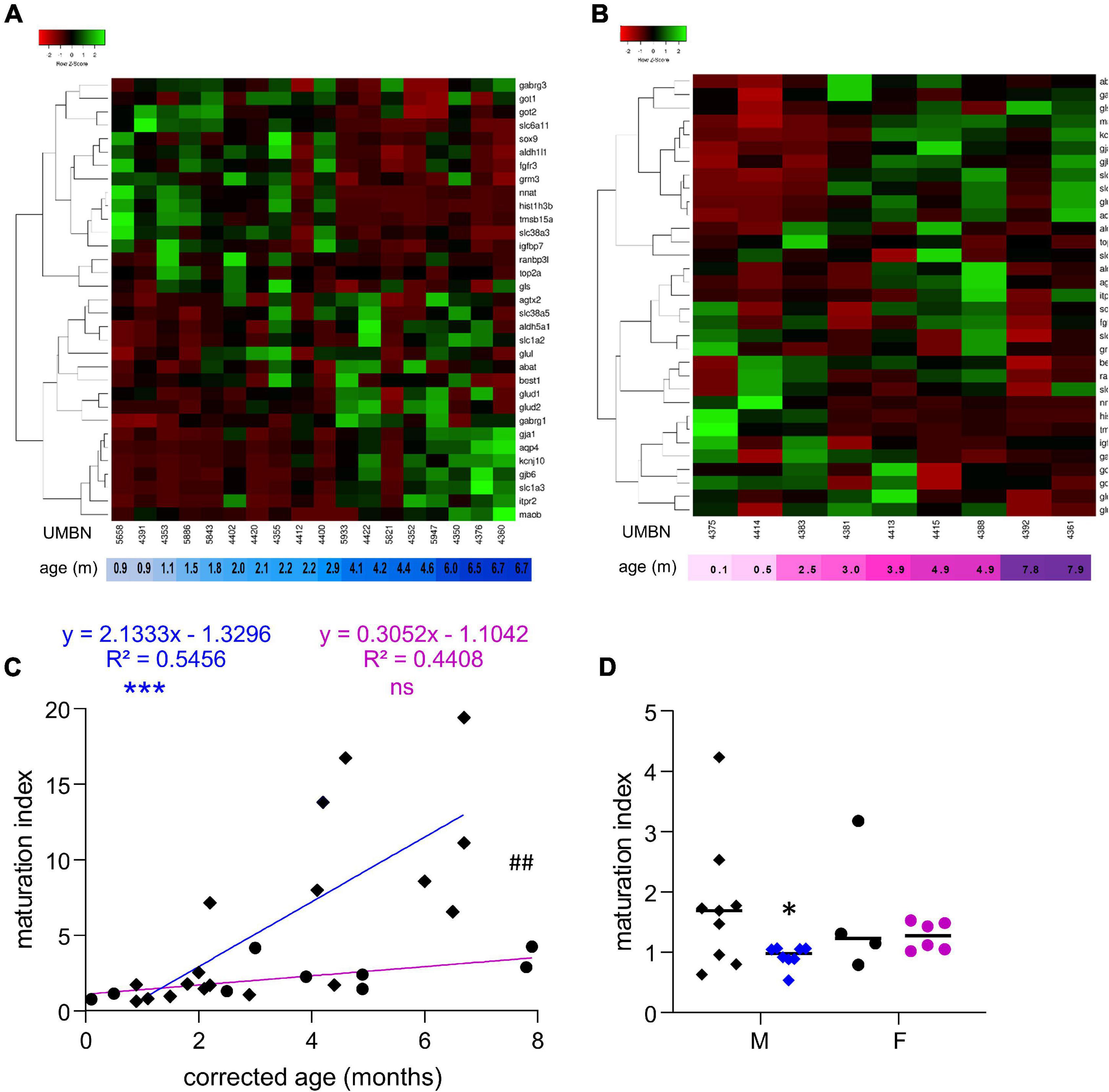
Figure 4. Preterm birth alters the maturation of the GABAergic system in astrocytes. (A,B) Heatmap of astrocyte-related gene expression means in the BA10 of (A) male and (B) female term included in the cross sectional study, arranged using hierarchical clustering (complete linkage with Pearson distance measurement method). (C) Maturation index based on the heatmap clustering of male (blue, diamonds) and female (purple, circles) term infants cross sectional study, modeled with a linear regression ***p < 0.001). ##p < 0.01 (ANCOVA, analysis of co-variance). (D) Maturation index of male and female term (black) and preterm (colored) infants comparative study, based on the heatmap clustering of the term infants cross-sectional study. *p < 0.05 (two-way ANOVA with Sidak’s multiple comparisons).
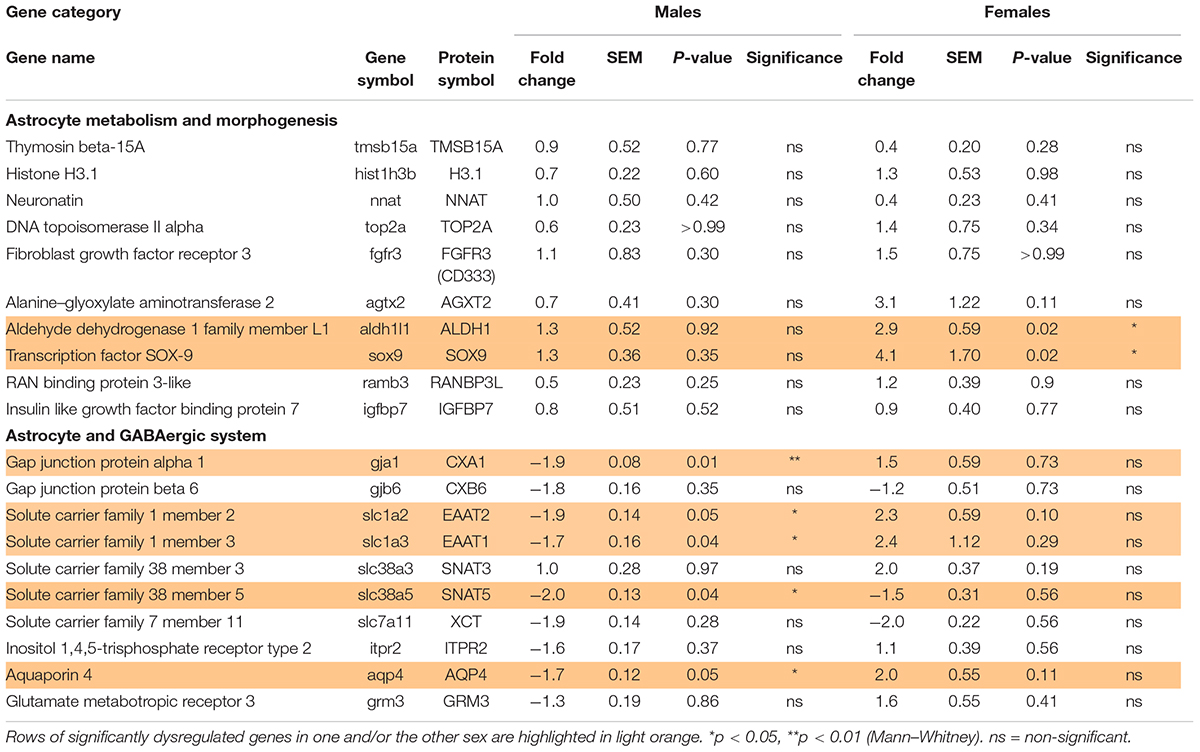
Table 3. Effect of preterm birth on the expression of astrocyte-associated genes in the BA10 of male and female infants determined by qRT-PCR (comparative study).
Out of the 10 genes related to astrocyte metabolism and morphogenesis, two were up-regulated in females only, both linked to astrocyte intrinsic metabolism: aldh1l1 (metabolic enzyme, FC = 2.9, p < 0.05, Table 3) and sox9 (transcription factor, FC = 4.1, p < 0.05, Table 3). Regarding the genes associated with the astrocytic GABA function, five were significantly regulated in males. In accordance with the decreased maturation index, these transcripts varied in the opposite way to their developmental trajectories, predicted by linear regression (Figure 5). The dysregulated transcripts included: gja1 (gap junction allowing intercellular communication between astrocytes, FC = −1.9, p < 0.01, Table 3 and Figure 5A) slc1a2 and slc1a3 (glutamate transporters, FC = −1.9, p < 0.05 and FC = −1.7, p < 0.05, Table 3 and Figures 5B,C) slc38a5 (glutamine transporter, FC = −2.0, p < 0.05, Table 3 and Figure 5D) and aqp4 (water channel, FC = −1.7, p < 0.05; Table 3 and Figure 5E). Protein and mRNA expression levels followed the same pattern in preterm infant samples (Figure 5).
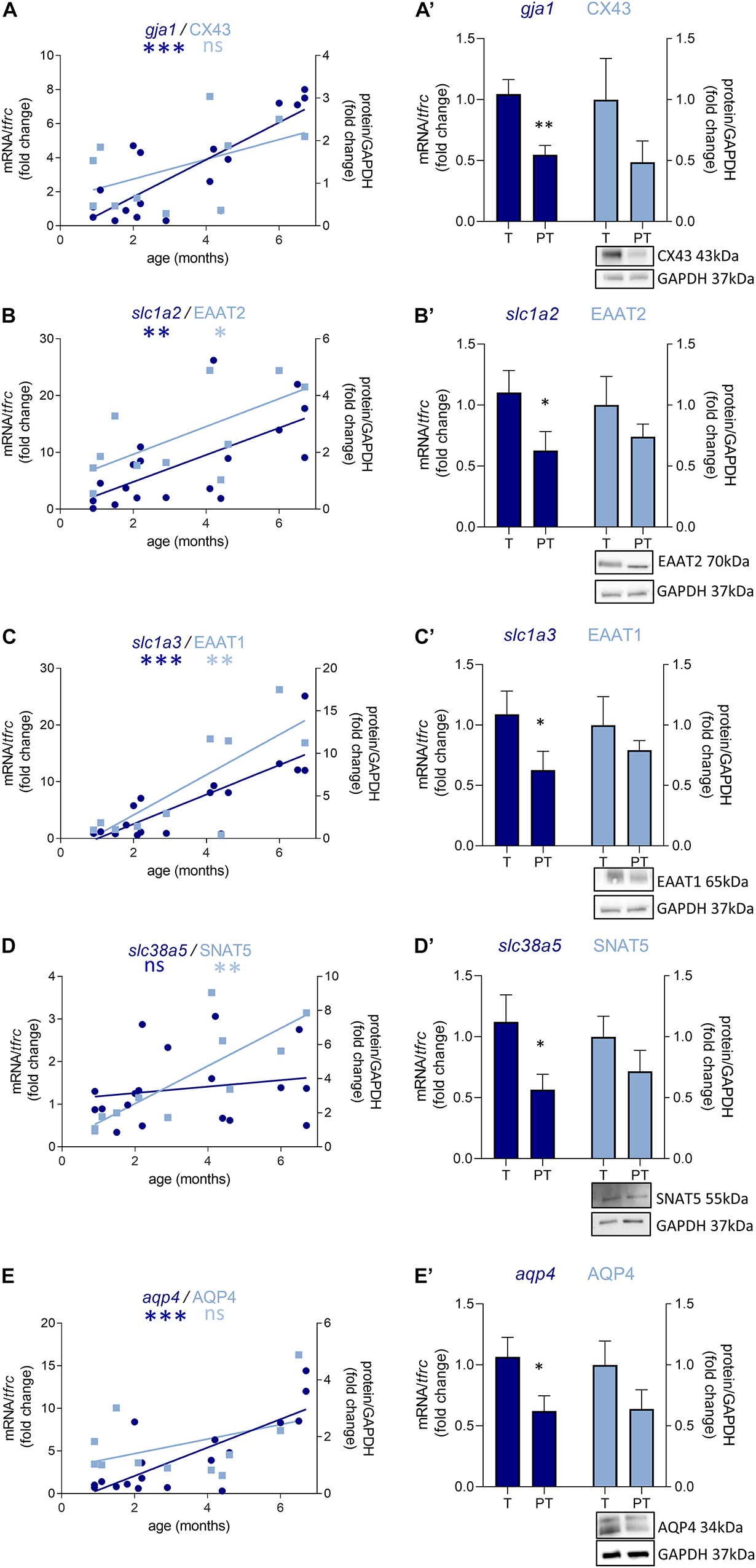
Figure 5. Preterm birth impacts the expression of astrocyte-related transcripts (dark blue) and proteins (light blue) in the BA10 of males infants. (A–E) Developmental regulation in term infants included in the cross sectional study, modeled with a non-linear regression (*p < 0.05, **p < 0.01, ***p < 0.001). (A’–E’) Effect of preterm birth on GABA-related transcripts and proteins (comparative study). Quantification of mRNA level changes by qRT-PCR of (A,A’) gja1, (B,B’) slc1a2, (C,C’) slc1a3, (D,D’) slc38a5, and (E,E’) aqp4. tfrc was used for normalization. Quantification of protein expression changes by Western blot of (A,A’) CX43, (B,B’) EAAT2, (C,C’) EAAT1, (D,D’) SNAT5, and (E,E’) AQP4. GAPDH was used for normalization. *p < 0.05, **p < 0.01 (Mann–Whitney). Representative blot below the graph. PT, preterm; T, term.
The Effect of Preterm Birth on the Maturation of the GABAergic System Is Not Due to a Change in the Number of Astrocytes
To address whether the observed molecular changes were due to a reduced number of cells or to a cellular maturation defect, both GABA-producing cell (interneurons) and astrocyte densities were assessed in three subdivisions of BA10: the upper layers (ULs), lower layers (LLs), and the sub-cortical white matter (SC-WM). The density of major subclasses of cortical interneurons was assessed by immunostaining for GAD65-67, SST, CLB, CRT, and NPY (Figure 6). GAD65-67-positive cell density was significantly decreased in the ULs of preterm male infants as compared with term male infants (UL −37% p < 0.05, LL −28% ns, SC-WM −27% ns, Figure 6A). A significant reduction in the density of SST was observed in both UL and LL (UL −43% p < 0.05, LL −30% p < 0.05, SC-WM −23% ns, Figure 6B) and of CLB interneurons in the UP but LL (UL −47% p < 0.05, LL −17% ns, SCWM: −1% ns, Figure 6C). No change was observed in the density of CRT or NPY interneurons (Figures 6D,E). PV cells were not detected in this set of human samples, although these cells could be detected at later developmental stages (data not shown). No statistically significant change in total cortical layer widths was discernable based on Nissl staining (data not shown), suggesting overall preservation of pyramidal cells at this age. No difference was observed in female infants, but statistical power was limited by the small sample size (Figures 6A’–E’).
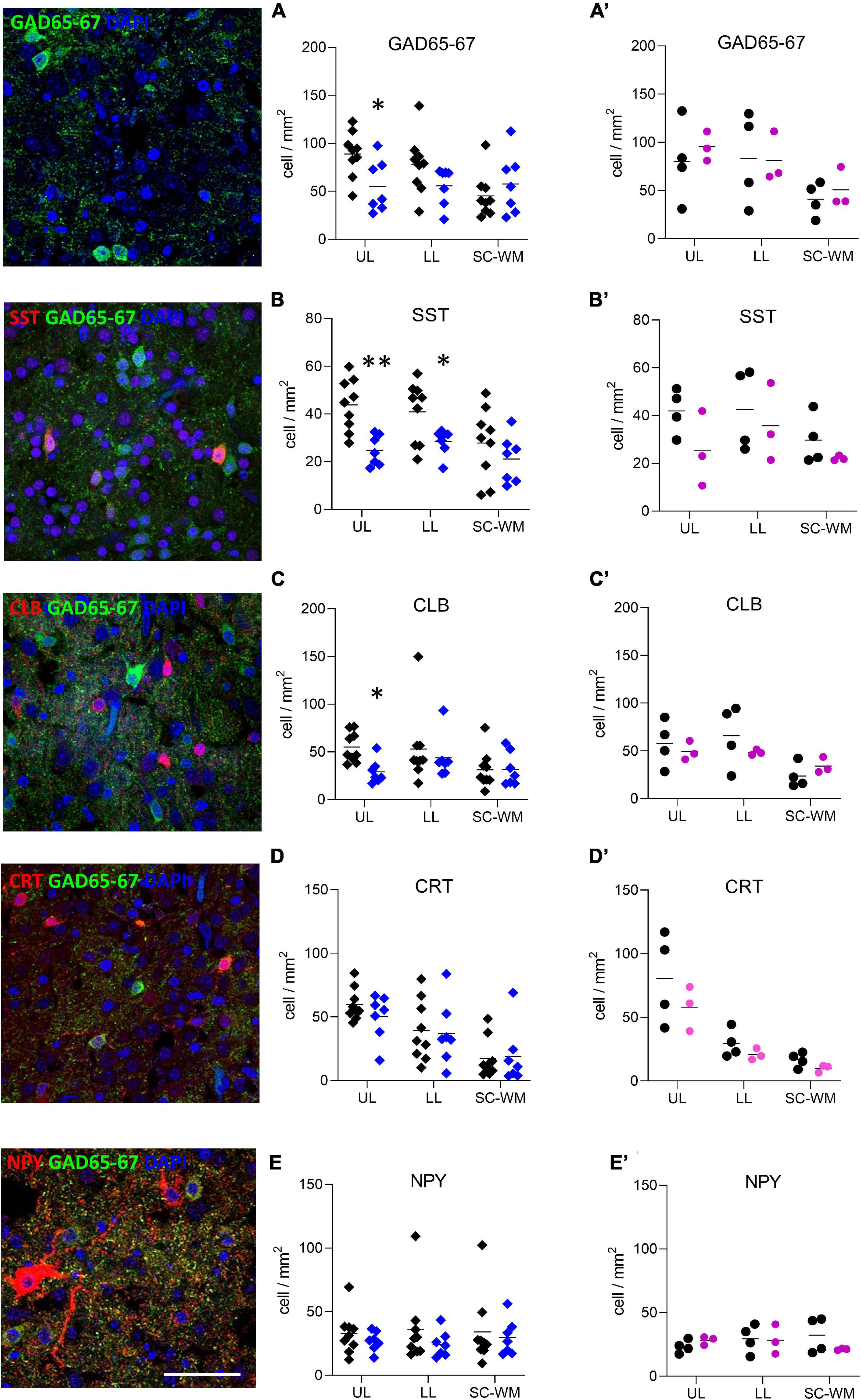
Figure 6. Preterm birth impacts interneuron density in the BA10. Illustrations of (A) glutamate decarboxylase 65 and 67 (GAD65-67, green), (B) SST (red), (C) CLB (red), (D) CRT (red), and (E) NPY (red) immunofluorescent staining. Scale bar 50 μm. Respective quantification of immunopositive cells in the upper layers (UL), lower layers (LL), and subcortical white matter (SC-WM) in the BA10 of (A–E) male (blue, diamonds) and (A’–E’) female (pink, circles) term (black) versus preterm (colored) infants included in the comparative study. Scatter dot plots show the mean and individual dispersion of term (colored circles) and preterm infants (black circles); *p < 0.05, **p < 0.01 (two-way ANOVAs were performed followed by Fisher’s LSD tests for post hoc comparisons).
GFAP-positive cell density was not significantly affected by preterm birth in males (UL −14% ns, LL −62% ns, SC-WM −15% ns, Figure 7A) and in females (UL −41% ns, LL −25% ns, SC-WM −9% ns, Figure 7A’). In accordance with the cell counts, GFAP mRNA and protein levels were not altered in premature infants (Figures 7B,B’,C,C’). Notably, the expression of gfap represented by a linear regression, increased in the postnatal period in males, confirming the dynamic aspect of the system being broader than in females (p < 0.05, Figure 7B; ns, Figure 7C).
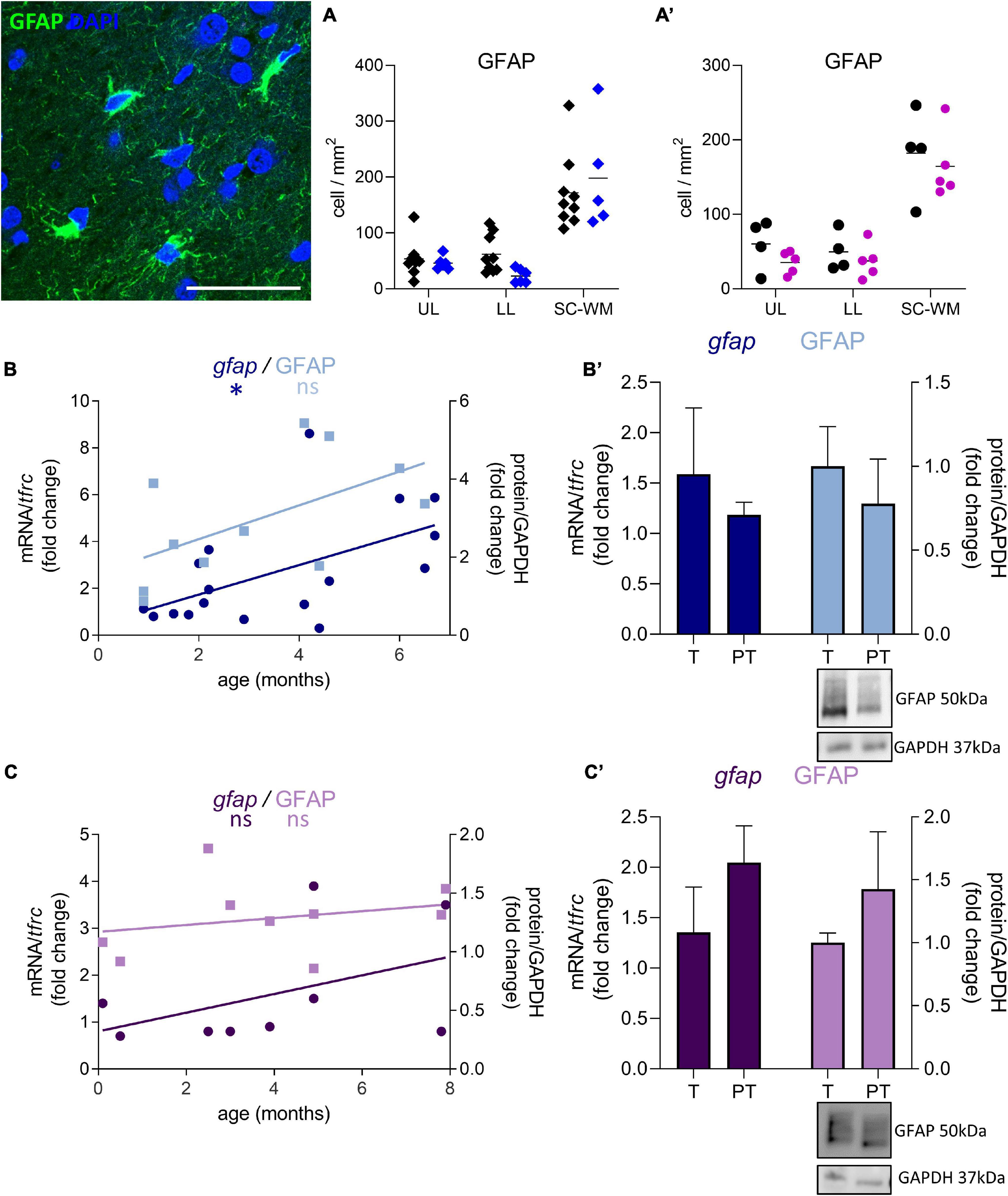
Figure 7. Effect of preterm birth on BA10 GFAP expression. Illustrations of GFAP-immunofluorescent staining. Scale bar X μm. (A,A’) Quantification of GFAP positive cells in the UL, LL, and SC-WM in the BA10 of (A) male (blue, diamonds) and (A’) female (pink, circles) term (black) versus preterm (colored) infants included in the comparative study. Scatter dot plots show the mean and individual dispersion of term (black circles) and preterm infants (colored circles); (two-way ANOVAs were performed followed by Fisher’s LSD tests for post hoc comparisons). (B,C) Developmental regulation of GFAP transcripts (dark) and proteins (light) in the BA10 of (B) male (blue) and (C) female (purple) infants, included in the cross sectional study, modeled with a non-linear regression *p < 0.05. (B’,C’) Effect of preterm birth on GFAP expression (comparative study). Quantification of mRNA level changes by qRT-PCR of gfap. tfrc was used for normalization. Quantification of protein expression changes by Western blot of GFAP. GAPDH was used for normalization (Mann–Whitney). Representative blot below the graph. PT, preterm; T, term.
Discussion
Understanding the effects of perinatal insults on GABAergic system development in male and female infants is critical to elucidating the mechanistic role it plays in the pathogenesis of neuropsychiatric disorders as well as the greater susceptibility of male infants to these pathologies. To assess the development of the GABAergic system, 47 genes related to GABA were analyzed in a cross-sectional study, in the BA10 (dorsolateral prefrontal cortex) of young male and female term infants (0- to 8-month-old). A maturation index was calculated based on the ratio of up-regulated versus down-regulated genes, as previously described (Gandal et al., 2012). The index was correlated with the age of the donor and was more dynamic in male than in female infants, as suggested by the steeper slope of the linear regression. Prior studies have shown that developmentally regulated differences in gene- and exon level expression exist between male and female brains in specific regions, including the PFC, and can have long lasting effects on brain development and plasticity (Mottron et al., 2015). Specifically, males are more susceptible than females to perturbations in genes involved in synaptic plasticity (Mottron et al., 2015). The flexibility of neuronal networks depends on both excitatory and inhibitory synapse plasticity, which notably relies on the number of postsynaptic GABAergic receptors (Barberis, 2020). Such GABAergic plasticity, elicited by the ventral hippocampus and basolateral amygdala, has been demonstrated in the PFC (Caballero et al., 2014). Within a local network, the GABAergic system can also adapt its efficacy to the overall activity of the network, being upregulated in hyperactive networks and downregulated under conditions of reduced activity. This homeostatic plasticity is mediated by multiple pre- and postsynaptic mechanisms, including the concentration of GABA itself, regulated by synthetizing enzymes and transporters (Roth and Draguhn, 2012).
To evaluate the impact of premature birth on GABAergic system development, the prefrontal cortices from 1-month-old term and 1-month corrected-age very preterm infants were compared, using the same gene list. The calculation of the maturation index showed a decrease in male but not in female preterm infants, suggesting a maturation delay in the formers. The basal higher dynamicity in male infants could explain this difference, rendering the genes associated to the GABAergic system more susceptible to perturbation. The maturation of the GABAergic system could either be delayed to compensate for the immature excitatory system, or be the result of various noxious events suffered by preterm infants.
Out of the 47 genes analyzed, the differentially expressed genes (DEGs) affected by preterm birth were distinct between males and females. Male DEGs varied in opposition to their predicted developmental trajectory resulting in the overall developmental delay displayed by the maturation index. Closely looking at individual DEGs revealed that most of the gene regulation in male were tied to the astrocytic GABAergic function. For instance, prematurity markedly reduced the expression of two GABAA receptor subunits: gabra2, enriched is astrocytes (Zhang et al., 2016), and gabrg1, which is specifically expressed by astrocytes (Batiuk et al., 2020). In these cells, GABA is depolarizing and contributes to maintaining GABAergic neuronal transmission via Cl– efflux, buffering the Cl– concentration of the GABAergic synapse (Isomura et al., 2003). Astrocytes also control the biosynthesis and turnover of GABA, both affected by prematurity. Maob, responsible for the synthesis of GABA from putrescine in astrocytes (Yoon et al., 2014), was downregulated while gdh2 was upregulated, an enzyme in charge of the turnover of glutamate, which catabolism is tied with GABA through the tricarboxylic acid cycle (Hertz et al., 1978). Our findings suggest that this decreased synthesis and increased turnover would lead to a decrease of total GABA content in preterm male infants. A recently published study demonstrated that GABA concentration in the PFC was lower in preterm infants when measured in vivo (Basu et al., 2020). Lower levels of brain GABA and glutamate concentrations have been associated with a variety of neurologic disorders including epilepsy, ASD and ADHD (Schür et al., 2016), which are more common in surviving premature infants (Ream and Lehwald, 2018).
In female preterm infants, the DEGs varied accordingly to their developmental trajectory suggesting either an accelerated development or compensatory mechanisms to resemble their non-corrected age counterpart. Preterm birth is a leading cause of psychiatric disorders such as ASD, schizophrenia or ADHD, which are tightly linked to the GABAergic system, and males are more likely to develop these pathologies. Preterm infants are 4 times more likely to develop ASD (Joseph et al., 2017). The possibility has been raised that this sex bias is an intrinsic female protective mechanism. This hypothesizes that multiple genetic factors contribute to the liability for developing ASD and that a higher threshold of genetic liability results in female pathological presentation (Jacquemont et al., 2014). The “extreme male brain theory” is another prominent hypothesis to explain this gender bias, which suggests that fetal testosterone exposure may underlie gender differences in autistic traits (Baron-Cohen, 2002).
Since most of the perturbations observed in male preterm infants were related to the astrocytic regulation of GABAergic signaling, additional astrocytic transcripts related to either metabolism and morphogenesis or GABAergic system were analyzed. GABA-associated astrocytic genes were solely downregulated in males, oppositely to their normal developmental trajectory. For instance, glutamine and glutamate transporter transcripts were downregulated in preterm male infants. These neurotransmitter metabolisms are tied to the metabolism of GABA and their dysregulation has often been reported in psychiatric disorders (O’Donovan et al., 2017).
In human, astrocytes are born during the second part of gestation (deAzevedo et al., 2003) leaving these cells highly susceptible to perinatal insults. Their proliferation occurs in tandem with birth and refinement of synapses within individual circuits, making astrocytes critical regulators of brain development (Perez-Catalan et al., 2021). Since preterm male infants maintain the same number of astrocytes than their term counterpart, it seems that prematurity mainly affected the function of these cells through their maturation rather than their proliferation or survival. In accordance, preclinical studies highlighted the absence of reactive astrogliosis in the immature white matter following chronic hypoxia, with decreased glutamate transporter expression suggesting a more immature phenotype of astrocytes (Back et al., 2006; Raymond et al., 2011). However, the number of BA10 GABA-producing cells was decreased in preterm male infants, which is consistent with our former study (Lacaille et al., 2019) as well as other studies of preterm birth brain (Robinson et al., 2006; Panda et al., 2018) and psychiatric disorders (Hashimoto et al., 2008; Mellios et al., 2009; Hashemi et al., 2017). Pharmacological effects of neuroprotective agents such as the GABA agonist and anti-inflammatory compound allopregnanolone are being investigated in the context of preterm birth and show promising results (Vacher et al., 2021). New cellular models have been developed and will give the opportunity to investigate the environmental and genetic factors underlying injury in the developing human brain (Paşca et al., 2019). Even though prematurity is a significant contributor to psychiatric disorders, many preterm infants survive neurologically intact, implying than most of the time, the preterm male infant GABA system could undergo a coordinated developmental delay, potentially conserving a stable excitatory-inhibitory balance.
Data Availability Statement
The original contributions presented in the study are included in the article/supplementary material, further inquiries can be directed to the corresponding author.
Ethics Statement
Ethical review and approval was not required for the study on human participants in accordance with the local legislation and institutional requirements. Written informed consent from the participants’ legal guardian/next of kin was not required to participate in this study in accordance with the national legislation and the institutional requirements.
Author Contributions
HL, C-MV, and AAP conceived the project, designed the experiments, and wrote, reviewed, and revised the manuscript. HL performed the experiments. HL and C-MV analyzed and interpreted the results. All authors reviewed and revised the manuscript.
Conflict of Interest
The authors declare that the research was conducted in the absence of any commercial or financial relationships that could be construed as a potential conflict of interest.
Publisher’s Note
All claims expressed in this article are solely those of the authors and do not necessarily represent those of their affiliated organizations, or those of the publisher, the editors and the reviewers. Any product that may be evaluated in this article, or claim that may be made by its manufacturer, is not guaranteed or endorsed by the publisher.
Acknowledgments
We thank Dr. D. Bakalar, Dr. J. O’reilly, and Ms. J. Salzbank for insightful comments on the manuscript, and Janine Burgess and Sacha Parchment for expert assistance. We thank Dr. V. Gallo and Dr. E. Passegue for hosting us in their respective facilities. We also thank the contribution of families to the NIH NeuroBioBank, as well as J. Cottrell (NIH NeuroBioBank), who assisted us throughout the process of human tissue selection.
References
Adams-Chapman, I., and Stoll, B. J. (2006). Neonatal infection and long-term neurodevelopmental outcome in the preterm infant. Curr. Opin Infect. Dis. 19, 290–297. doi: 10.1097/01.qco.0000224825.57976.87
Arpino, C., Compagnone, E., Montanaro, M. L., Cacciatore, D., De Luca, A., Cerulli, A., et al. (2010). Preterm birth and neurodevelopmental outcome: a review. Childs Nerv. Syst. 26, 1139–1149. doi: 10.1007/s00381-010-1125-y
Back, S. A., Craig, A., Luo, N. L., Ren, J., Akundi, R. S., Ribeiro, I., et al. (2006). Protective effects of caffeine on chronic hypoxia-induced perinatal white matter injury. Ann. Neurol. 60, 696–705. doi: 10.1002/ana.21008
Barberis, A. (2020). Postsynaptic plasticity of GABAergic synapses. Neuropharmacology 169:107643. doi: 10.1016/j.neuropharm.2019.05.020
Baron-Cohen, S. (2002). The extreme male brain theory of autism. Trends Cogn. Sci. 6, 248–254. doi: 10.1016/s1364-6613(02)01904-6
Basu, S. K., Pradhan, S., Jacobs, M. B., Said, M., Kapse, K., Murnick, J., et al. (2020). Age and Sex Influences Gamma-aminobutyric Acid Concentrations in the Developing Brain of Very Premature Infants. Sci. Rep. 10:10549. doi: 10.1038/s41598-020-67188-y
Batiuk, M. Y., Martirosyan, A., Wahis, J., de Vin, F., Marneffe, C., Kusserow, C., et al. (2020). Identification of region-specific astrocyte subtypes at single cell resolution. Nat. Commun. 11:1220. doi: 10.1038/s41467-019-14198-8
Ben-Ari, Y. (2006). Basic developmental rules and their implications for epilepsy in the immature brain. Epileptic Disord. 8, 91–102.
Ben-Ari, Y. (2014). The GABA excitatory/inhibitory developmental sequence: a personal journey. Neuroscience 279, 187–219. doi: 10.1016/j.neuroscience.2014.08.001
Blencowe, H., Cousens, S., Chou, D., Oestergaard, M., Say, L., Moller, A. B., et al. (2013). Born too soon: the global epidemiology of 15 million preterm births. Reprod. Health 1(Suppl. 1), S2. doi: 10.1186/1742-4755-10-s1-s2
Caballero, A., Thomases, D. R., Flores-Barrera, E., Cass, D. K., and Tseng, K. Y. (2014). Emergence of GABAergic-dependent regulation of input-specific plasticity in the adult rat prefrontal cortex during adolescence. Psychopharmacology 231, 1789–1796. doi: 10.1007/s00213-013-3216-4
deAzevedo, L. C., Fallet, C., Moura-Neto, V., Daumas-Duport, C., Hedin-Pereira, C., and Lent, R. (2003). Cortical radial glial cells in human fetuses: depth-correlated transformation into astrocytes. J. Neurobiol. 55, 288–298. doi: 10.1002/neu.10205
Gandal, M. J., Nesbitt, A. M., McCurdy, R. M., and Alter, M. D. (2012). Measuring the maturity of the fast-spiking interneuron transcriptional program in autism, schizophrenia, and bipolar disorder. PLoS One 7:e41215. doi: 10.1371/journal.pone.0041215
Hashemi, E., Ariza, J., Rogers, H., Noctor, S. C., and Martínez-Cerdeño, V. (2017). The Number of Parvalbumin-Expressing Interneurons Is Decreased in the Prefrontal Cortex in Autism. Cereb. Cortex 27, 1931–1943. doi: 10.1093/cercor/bhw021
Hashimoto, T., Arion, D., Unger, T., Maldonado-Avilés, J. G., Morris, H. M., Volk, D. W., et al. (2008). Alterations in GABA-related transcriptome in the dorsolateral prefrontal cortex of subjects with schizophrenia. Mol. Psychiatry 13, 147–161. doi: 10.1038/sj.mp.4002011
Hertz, L., Wu, P. H., and Schousboe, A. (1978). Evidence for net uptake of GABA into mouse astrocytes in primary cultures–its sodium dependence and potassium independence. Neurochem. Res. 3, 313–323. doi: 10.1007/bf00965577
Hodel, A. S. (2018). Rapid Infant Prefrontal Cortex Development and Sensitivity to Early Environmental Experience. Dev. Rev. 48, 113–144. doi: 10.1016/j.dr.2018.02.003
Ishibashi, M., Egawa, K., and Fukuda, A. (2019). Diverse Actions of Astrocytes in GABAergic Signaling. Int. J. Mol. Sci. 20:ijms20122964. doi: 10.3390/ijms20122964
Isomura, Y., Sugimoto, M., Fujiwara-Tsukamoto, Y., Yamamoto-Muraki, S., Yamada, J., and Fukuda, A. (2003). Synaptically activated Cl-accumulation responsible for depolarizing GABAergic responses in mature hippocampal neurons. J. Neurophysiol. 90, 2752–2756. doi: 10.1152/jn.00142.2003
Jacquemont, S., Coe, B. P., Hersch, M., Duyzend, M. H., Krumm, N., Bergmann, S., et al. (2014). A higher mutational burden in females supports a “female protective model” in neurodevelopmental disorders. Am. J. Hum. Genet. 94, 415–425. doi: 10.1016/j.ajhg.2014.02.001
Joseph, R. M., O’Shea, T. M., Allred, E. N., Heeren, T., Hirtz, D., Paneth, N., et al. (2017). Prevalence and associated features of autism spectrum disorder in extremely low gestational age newborns at age 10 years. Autism Res. 10, 224–232. doi: 10.1002/aur.1644
Knuesel, I., Chicha, L., Britschgi, M., Schobel, S. A., Bodmer, M., Hellings, J. A., et al. (2014). Maternal immune activation and abnormal brain development across CNS disorders. Nat. Rev. Neurol. 10, 643–660. doi: 10.1038/nrneurol.2014.187
Kwon, S. H., Scheinost, D., Lacadie, C., Benjamin, J., Myers, E. H., Qiu, M., et al. (2014). GABA, resting-state connectivity and the developing brain. Neonatology 106, 149–155. doi: 10.1159/000362433
Lacaille, H., Vacher, C. M., Bakalar, D., O’Reilly, J. J., Salzbank, J., and Penn, A. A. (2019). Impaired Interneuron Development in a Novel Model of Neonatal Brain Injury. eNeuro 6:2019. doi: 10.1523/eneuro.0300-18.2019
Marín, O. (2012). Interneuron dysfunction in psychiatric disorders. Nat. Rev. Neurosci. 13, 107–120. doi: 10.1038/nrn3155
Meldrum, S. J., Strunk, T., Currie, A., Prescott, S. L., Simmer, K., and Whitehouse, A. J. (2013). Autism spectrum disorder in children born preterm-role of exposure to perinatal inflammation. Front. Neurosci. 7:123. doi: 10.3389/fnins.2013.00123
Mellios, N., Huang, H. S., Baker, S. P., Galdzicka, M., Ginns, E., and Akbarian, S. (2009). Molecular determinants of dysregulated GABAergic gene expression in the prefrontal cortex of subjects with schizophrenia. Biol. Psychiatry 65, 1006–1014. doi: 10.1016/j.biopsych.2008.11.019
Mottron, L., Duret, P., Mueller, S., Moore, R. D., Forgeot d’Arc, B., et al. (2015). Sex differences in brain plasticity: a new hypothesis for sex ratio bias in autism. Mol. Autism 6:33. doi: 10.1186/s13229-015-0024-1
Nosarti, C., Rubia, K., Smith, A. B., Frearson, S., Williams, S. C., Rifkin, L., et al. (2006). Altered functional neuroanatomy of response inhibition in adolescent males who were born very preterm. Dev. Med. Child Neurol. 48, 265–271. doi: 10.1017/s0012162206000582
O’Donovan, S. M., Sullivan, C. R., and McCullumsmith, R. E. (2017). The role of glutamate transporters in the pathophysiology of neuropsychiatric disorders. NPJ Schizophr. 3:32. doi: 10.1038/s41537-017-0037-1
Panda, S., Dohare, P., Jain, S., Parikh, N., Singla, P., Mehdizadeh, R., et al. (2018). Estrogen Treatment Reverses Prematurity-Induced Disruption in Cortical Interneuron Population. J. Neurosci. 38, 7378–7391. doi: 10.1523/jneurosci.0478-18.2018
Paşca, A. M., Park, J. Y., Shin, H. W., Qi, Q., Revah, O., Krasnoff, R., et al. (2019). Human 3D cellular model of hypoxic brain injury of prematurity. Nat. Med. 25, 784–791. doi: 10.1038/s41591-019-0436-0
Perez-Catalan, N. A., Doe, C. Q., and Ackerman, S. D. (2021). The role of astrocyte-mediated plasticity in neural circuit development and function. Neural Dev. 16:1. doi: 10.1186/s13064-020-00151-9
Raymond, M., Li, P., Mangin, J. M., Huntsman, M., and Gallo, V. (2011). Chronic perinatal hypoxia reduces glutamate-aspartate transporter function in astrocytes through the Janus kinase/signal transducer and activator of transcription pathway. J. Neurosci. 31, 17864–17871. doi: 10.1523/jneurosci.3179-11.2011
Ream, M. A., and Lehwald, L. (2018). Neurologic Consequences of Preterm Birth. Curr. Neurol. Neurosci. Rep. 18:48. doi: 10.1007/s11910-018-0862-2
Represa, A., and Ben-Ari, Y. (2005). Trophic actions of GABA on neuronal development. Trends Neurosci. 28, 278–283. doi: 10.1016/j.tins.2005.03.010
Robinson, S., Li, Q., Dechant, A., and Cohen, M. L. (2006). Neonatal loss of gamma-aminobutyric acid pathway expression after human perinatal brain injury. J. Neurosurg. 104(6 Suppl.), 396–408. doi: 10.3171/ped.2006.104.6.396
Roth, F. C., and Draguhn, A. (2012). GABA metabolism and transport: effects on synaptic efficacy. Neural Plast. 2012:805830. doi: 10.1155/2012/805830
Schür, R. R., Draisma, L. W., Wijnen, J. P., Boks, M. P., Koevoets, M. G., Joëls, M., et al. (2016). Brain GABA levels across psychiatric disorders: A systematic literature review and meta-analysis of (1) H-MRS studies. Hum. Brain Mapp. 37, 3337–3352. doi: 10.1002/hbm.23244
Shaw, J. C., Palliser, H. K., Dyson, R. M., Berry, M. J., and Hirst, J. J. (2018). Disruptions to the cerebellar GABAergic system in juvenile guinea pigs following preterm birth. Int. J. Dev. Neurosci. 65, 1–10. doi: 10.1016/j.ijdevneu.2017.10.002
Shaw, J. C., Palliser, H. K., Walker, D. W., and Hirst, J. J. (2015). Preterm birth affects GABAA receptor subunit mRNA levels during the foetal-to-neonatal transition in guinea pigs. J. Dev. Orig. Health Dis. 6, 250–260. doi: 10.1017/s2040174415000069
Tanifuji, S., Akasaka, M., Kamei, A., Araya, N., Asami, M., Matsumoto, A., et al. (2017). Temporal brain metabolite changes in preterm infants with normal development. Brain Dev. 39, 196–202. doi: 10.1016/j.braindev.2016.10.006
Tomiyasu, M., Aida, N., Shibasaki, J., Umeda, M., Murata, K., Heberlein, K., et al. (2017). In vivo estimation of gamma-aminobutyric acid levels in the neonatal brain. NMR Biomed. 30:3666. doi: 10.1002/nbm.3666
Vacher, C. M., Lacaille, H., O’Reilly, J. J., Salzbank, J., Bakalar, D., Sebaoui, S., et al. (2021). Placental endocrine function shapes cerebellar development and social behavior. Nat. Neurosci. [Preprint]. doi: 10.1038/s41593-021-00896-4
Volpe, J. J. (2009). The encephalopathy of prematurity–brain injury and impaired brain development inextricably intertwined. Semin Pediatr. Neurol. 16, 167–178. doi: 10.1016/j.spen.2009.09.005
Wang, M., Yang, P., Wan, C., Jin, Z., Zhang, J., and Li, L. (2018). Evaluating the Role of the Dorsolateral Prefrontal Cortex and Posterior Parietal Cortex in Memory-Guided Attention With Repetitive Transcranial Magnetic Stimulation. Front. Hum. Neurosci. 12:236. doi: 10.3389/fnhum.2018.00236
Yoon, B. E., Woo, J., Chun, Y. E., Chun, H., Jo, S., Bae, J. Y., et al. (2014). Glial GABA, synthesized by monoamine oxidase B, mediates tonic inhibition. J. Physiol. 592, 4951–4968. doi: 10.1113/jphysiol.2014.278754
Keywords: prematurity, GABA, prefrontal cortex, neurodevelopment, maturation, astrocyte
Citation: Lacaille H, Vacher C-M and Penn AA (2022) Preterm Birth Alters the Maturation of the GABAergic System in the Human Prefrontal Cortex. Front. Mol. Neurosci. 14:827370. doi: 10.3389/fnmol.2021.827370
Received: 01 December 2021; Accepted: 31 December 2021;
Published: 03 February 2022.
Edited by:
Arie Kaffman, Yale University, United StatesReviewed by:
Yuri Bozzi, University of Trento, ItalyAlberto Cruz-Martín, Boston University, United States
Copyright © 2022 Lacaille, Vacher and Penn. This is an open-access article distributed under the terms of the Creative Commons Attribution License (CC BY). The use, distribution or reproduction in other forums is permitted, provided the original author(s) and the copyright owner(s) are credited and that the original publication in this journal is cited, in accordance with accepted academic practice. No use, distribution or reproduction is permitted which does not comply with these terms.
*Correspondence: Anna A. Penn, YWFwMjIxNkBjb2x1bWJpYS5lZHU=